- Department of Pharmaceutical Sciences, Jerry H. Hodge School of Pharmacy, Texas Tech University Health Sciences Center (TTUHSC), Amarillo, TX, United States
Nicotine, the major component of tobacco smoke (TS) and electronic cigarette (e-cig) vape, has been reported in some cases to be prodromal to cerebrovascular toxicity as well as a promoting factor for the onset of various neurological diseases. In some conditions, pre-exposure to nicotine can lead to a state of compromised blood-brain barrier (BBB) integrity, including altered BBB-related protein expression, BBB leakage, and defective ion and glucose homeostasis within the brain. Moreover, drugs used to treat central nervous system disorders (CNS) have been reported to interact with nicotine and other components of TS/e-cig through both transporter and enzyme-based mechanisms. Herein we discuss nicotine’s potential toxicity at the brain cerebrovasculature and explain how nicotine (from smoking/vaping) may interfere with the uptake of CNS drugs through a CNS drug interaction perspective.
Introduction
Exposure to nicotine and combustion products comprising tobacco smoke (TS) and electronic cigarettes (e-cigs) or vapes are prominent risk factors implicated in ischemic stroke and possibly other neurological diseases such as Alzheimer’s disease (AD), schizophrenia, neuro-AIDS, and multiple sclerosis (MS) (Health and Services 2006; Salokangas et al., 2006; Moreno-Gonzalez et al., 2013; Kaisar et al., 2018; Sivandzade et al., 2019; McIntosh et al., 2021). TS is thought to be one of the greatest sources of toxic chemical exposure to humans and is accountable for the death of ∼400,000 individuals alone in the US (Mathers and Loncar 2006; Krist et al., 2021). A single puff of TS consists of around chemical 7,000 compounds such as polycyclic aromatic hydrocarbons, ammonia, aromatic amines, and various other chemicals and gaseous particulates that are hazardous (Mathers and Loncar 2006). Nevertheless, what makes TS so addictive is the primary psychoactive substance, nicotine, and this is based on how it acts nicotinic acetylcholine receptors and the release of the chemical dopamine, which is euphoric and reinforcing (Pontieri et al., 1996; Fowles and Dybing 2003). Nicotine is ranked by experts amongst the top-most addictive substance known to date to science (Mansvelder and McGehee 2002; Shoaib et al., 2002). Acute exposure to nicotine signals rewarding action, while continued exposure is co-related with desensitization leading to cellular tolerance (Laviolette and van der Kooy 2003). Molecular alteration occurs in the central dopamine systems that underlie continued propensity for nicotine consumption as well as adverse effects from its withdrawal (Laviolette and Van Der Kooy 2004). Of note, the oxidative stress-driven inflammatory potential of TS promotes cerebrovascular toxicity and compromises various recovery mechanisms in neurological pathologies (Pratico 2008; Arnson et al., 2010; Naik et al., 2014).
Since being introduced in the US market in 2006, there has been a steady rise in the use of e-cigs among both adults and youths, tobacco smokers, and non-smokers because it is perceived that e-cigs are a safer alternative and possess potentially less health risk than that of TS (McCubbin et al., 2017; Perikleous et al., 2018). E-cigs were not much regulated until 2016 when the US food and drug administration (US-FDA) extended its tobacco regulatory authority to products deemed to meet the definition of a tobacco product (Kasza et al., 2017). In recent years, the FDA has been active in funding projects that involve e-cig research via National Institute of Health grants (Backinger et al., 2016). Nevertheless, with limited research and lack of knowledge of the content of vaping solutions, other than nicotine, like polycyclic aromatic hydrocarbons, aldehydes, and nitrosamine, e-cigs are one of the major health concerns among the public (Goniewicz et al., 2014; Varlet et al., 2015; Gillman et al., 2016).
Nicotine, from TS and e-cigs, has been associated with the development of a number of potential toxic mechanisms inflicted on the brain, including increased oxidative stress (Bernard et al., 2019; Kuntic et al., 2020), neuroinflammation (Stamatovic et al., 2011; Li et al., 2019), cerebral thrombosis (Kaisar et al., 2017; Qasim et al., 2018), changes in BBB-related protein function or expression (Wang et al., 1994; Abbruscato et al., 2004; Kaisar et al., 2018), BBB permeability (Abbruscato et al., 2002; Hawkins et al., 2004; Kaisar et al., 2017), and cerebral blood flow (Hans et al., 1993; Zubieta et al., 2001). Furthermore, nicotine and chemical constituents of both TS and e-cigs have been reported to interact with the liver cytochrome P450 (CYP-P450) enzymes and affect plasma drug levels of certain central nervous system (CNS) drugs (Zevin and Benowitz 1999; Molden and Spigset 2009; Ellingrod 2013). Also, it has also been reported that CNS drugs utilize similar nicotine transport systems at the BBB interface, and in the presence of nicotine, their transport to the brain can be directly affected (Tega et al., 2018). Through this review, we report the known effects of TS and e-cig vaping on BBB dysfunction and worsening neurological disorders, primarily ischemic stroke, as several studies from our laboratory, fellow collaborators, and stroke researchers suggest smoking as comorbidity. In addition, we are drawing attention for the need to evaluate TS and e-cig interaction with some CNS drug therapy.
BBB and Effects of Tobacco Smoking/Electronic Cigarette Vaping
BBB Physiology and Properties
The BBB is a dynamic and specialized semipermeable boundary that forms the interface between circulating blood and brain parenchyma and functions to facilitate blood-based communication between the periphery and CNS (Abbott et al., 2010; Archie et al., 2021). It prevents the non-selective crossing of potentially harmful substances and permits the uptake of various essential nutrients and molecules from the blood and to the brain (Zhao et al., 2015; Archie et al., 2022). The primary anatomical unit of the BBB are the brain endothelial cells that closely interact with other cell types such as astrocytes, microglia, pericytes, and neurons for induction and maintenance of BBB properties (Sweeney et al., 2019; Sharma et al., 2021). Brain endothelial cells express tight junction (TJ) proteins responsible for limiting the paracellular diffusion of molecules to the brain by formation of a molecular seal. Therefore, circulating ions or molecules in the plasma rely mostly on the transcellular route to gain access to the brain. The effectiveness of the TJs results from the strong linkage of trans membranal claudins and occludins to intracellular actin and the cytoskeleton induced by cytoplasmic scaffolding proteins, zonula occludentes (ZO-1, 2, and 3) (Bagchi et al., 2019; Pandit et al., 2020).
The BBB is crucial for transporter-mediated influx and efflux of ions, nutrients, and drugs into and out of the brain (Sifat et al., 2019). Solute carrier transporters (SLC) and ATP-binding cassette (ABC) are the two major superfamilies of transporters present either at the apical or basolateral side of the BBB (Miyajima et al., 2011). The SLC transporters are primarily responsible for the movement of solutes and ions across the BBB. Glucose transporters are the SLC transporters present at the BBB interface responsible for the transport and uptake of glucose (Shah and Abbruscato 2014). Various ion transporters like Na+-K+-ATPase and Na+-K+-2Cl− co-transporter (NKCC) are also present at the BBB interface that play a significant role in maintaining normal brain physiology (O’donnell et al., 2004). Organic anion and cation transporters are other members of the SLC transporter superfamily expressed at the BBB interface are responsible for drug uptake and transport across the brain (Ronaldson and Davis 2013). In contrast, the ABC transporters efflux various drugs and xenobiotics using active-energy-dependent transport mechanisms (Abdullahi et al., 2017). P-Glycoprotein and breast cancer resistance protein are examples of ABC transporters.
Effects of Nicotine, Tobacco Smoking, and E-Cigarette Vaping on BBB Integrity
Nicotine and its major metabolite cotinine exert their action by stimulating nicotinic acetylcholine receptors (nAchRs). nAchRs are inotropic receptors proteins consisting of five subunits that form a central transmembrane cation channel that, upon stimulation, causes inward movement of sodium and calcium (Mansvelder and McGehee 2002). Bronchial epithelial cells, aortic endothelial cells, keratinocytes, and brain endothelial cells express various subtypes of these receptors. The pharmacologic effect of nicotine at BBB is partly due to stimulation of nAchRs in brain endothelial cells that express α-3, α-5, α-7, β-2, and β-5 of its subtypes (Abbruscato et al., 2002; Moccia et al., 2004). Nicotine, the principal component of TS and e-cig, can negatively affect tight junctional protein expression, compromising BBB integrity (Abbruscato et al., 2002; Hawkins et al., 2004). When incubated with brain endothelial cells, cotinine and nicotine decrease ZO-1 expression (Abbruscato et al., 2002).
Moreover, the comparative effect of TS and e-cig extract showed that downregulation of ZO-1 protein from e-cig was not dissimilar to that from TS extract treatment (Kaisar et al., 2017). TS and e-cig extract treatment in iPSC-derived brain microvascular endothelial cells also showed a similar pattern of causing downregulation of claudin-5 and altered distribution pattern of ZO-1 and occludin (Kadry et al., 2021). Downregulation and dysregulation of distributions TJ proteins can lead to paracellular gaps between the endothelial cells and leak within the barrier. This study showed that TS and E-cig extract treatment caused an increased paracellular permeability of sucrose and mannitol; both are used as in vitro paracellular permeability markers of the BBB. (Kadry et al., 2021). The increased paracellular permeability of the markers is directly correlated to the downregulation of claudin-5, which is known to contribute to endothelial cell integrity. Also, the transendothelial electrical resistance of brain endothelial cell monolayers decreased with TS and E-cig extracts exposure.
Ion transporters at the BBB, such as Na+-K+-ATPase and NKCC, contribute to maintaining brain potassium levels during both normal and pathophysiologic conditions. A study from our laboratory reported in the presence of nicotine, the expression level of these transporters alters and could exacerbate vasogenic brain edema formation and negatively affect brain extracellular fluid potassium levels during an in vitro and in vivo model of ischemia (Abbruscato et al., 2004). Similarly, the expression of glucose transporters by brain endothelial cells decreases in the presence of nicotine, leading to decreased glucose transport across the BBB (Shah et al., 2015). Moreover, there is decreased brain glucose utilization in animals exposed to e-cig vapor (Sifat et al., 2018).
Nicotine Transport System in the Brain
Nicotine is the principal addictive and pharmacologic substance in TS and e-cigs. Nicotine is rapidly metabolized by liver cytochrome P450s into cotinine and distributed across the cerebrovascular system (Fukada et al., 2002a). The plasma level of nicotine in tobacco smokers or vapers generally averages about 20–60 ng/ml depending on frequency, the number of cigarettes and/or puffs of aerosols, age, and gender (Benowitz et al., 2009; Trehy et al., 2011; Cheng 2014; Barrington-Trimis and Leventhal 2018). In contrast, the plasma level of cotinine is significantly higher, with concentrations ranging between 250 and 300 ng/ml and a greater half-life of 16 h compared to a short half-life of approximately 2 h for nicotine (Marsot and Simon 2016). It has been reported that nicotine uses a specific transport system to cross the BBB, which might affect the brain uptake of certain CNS drugs from a CNS drug-drug interaction perspective.
Nicotine has been shown to be a substrate and/or inhibitor of SLC transporters subtype organic cation transporters (OCTs) such as OCT1 -3, organic cation/carnitine transporters (OCTn), multidrug and toxin extrusion protein 1 (MATE 1), and plasma monoamine transporters (PMAT) in various cell lines that were transfected with these transporters (Urakami et al., 1998; Wu et al., 2000; Tsuda et al., 2007; Itagaki et al., 2012). Additionally, carrier-mediated transport has also been reported for nicotine in pig kidney epithelial cell line (LLC-PK1) and human intestinal epithelial cell line (Caco-2) (Takami et al., 1998, Fukada et al., 2002b). Furthermore, studies showed nicotine interacts with brain monoamine transporter such as serotonin transporter (SERT), dopamine transporter (DAT), and norepinephrine transporter (NET), a major transporter system for CNS drugs (Table 1).
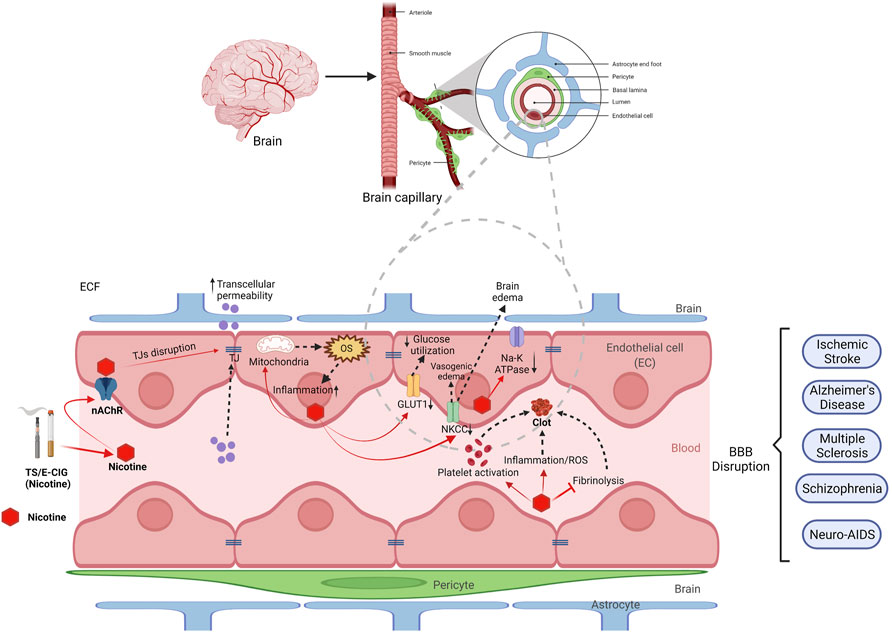
FIGURE 1. Effect of nicotine from tobacco smoke (TS) and electronic cigarette (e-cig) on blood-brain barrier (BBB) disruption. BBB is mainly composed of endothelial cells (red), astrocytes (blue), pericytes (green) and basal lamina. Nicotine enters the brain through nicotinic acetyl choline receptors (nAChRs) and disrupts the function of BBB by several mechanism. Nicotine can downregulate tight junction proteins (TJs) like ZO-1, claudins and occludins which results in increased transcellular permeability. Nicotine also disrupts mitochondrial function leading to increased oxidative stress (OS) and elevated inflammation and cytokines. Nicotine downregulates several transporters and receptors such as GLUT-1, NKCC and NA-K ATPase resulting in decreased brain glucose utilization and increased brain edema respectively. Moreover, nicotine causes platelet activation, increases inflammation, and inhibits fibrinolysis which ultimately results in clot or thrombus formation. This clot increases the chance of ischemic stroke. Thus, nicotine disrupts the BBB and plays a major role in pathogenesis of several cerebrovascular dysfunction including ischemic stroke, Alzheimer’s disease, multiple sclerosis, schizophrenia and neuro-AIDS.
Interestingly, nicotine uptake in an in vitro rat brain endothelial capillary cell line, TR-BBB13, showed hydrophilic cationic molecules such as 1-methyl-4-phenylpyridinium (MPP+), tetraethylammonium (TEA), and l-carnitine that are substrates of OCTs had no inhibitory effects (Tega et al., 2018). In contrast, hydrophobic cationic drugs such as pyrilamine, verapamil, and clonidine significantly reduced nicotine uptake. Additionally, the uptake clearance for carrier-mediated transport of nicotine is significantly higher in the BBB model than any other cell line, suggesting an involvement of transport driven by outward H+ gradient in nicotine transport across the brain. Pyrilamine is reported to be taken up from carrier-mediated transport with the involvement of H+/organic cation antiporter that drives H+ outward for its uptake, similar to that of nicotine, suggesting both molecules share a similar transport system for its uptake into the brain endothelial cells (Okura et al., 2008). This was supported by an in vivo experiment with carotid artery injection where nicotine brain uptake showed decreased value following pre-treatment with the hydrophobic cationic drug pyrilamine (Tega et al., 2013).
Additionally, efflux transporters like P-gp, that usually interact with moderately hydrophobic molecules, were reported not to influence brain uptake index of nicotine in the presence of known P-gp substrate verapamil (Toda et al., 2011). Furthermore, there was no difference in apparent permeability of nicotine in wild-type vs. P-gp knockout mice (Cisternino et al., 2013). Therefore, these studies support that P-gp is not involved in the nicotine transport system at the blood and brain interface. Overall, the carrier-mediated transport driven by outward H+ gradient is involved in most nicotine transport in rat brain endothelial cells. Nevertheless, additional studies are required to explore nicotine transport system in human brain endothelial cells for possible involvement of OCTs, MATE, and PMAT transporters that showed substrate specificity for nicotine.
Tobacco Smoke and Electronic Cigarette Vaping on Exacerbation of Ischemic Stroke
Pathophysiology of Ischemic Stroke
The prevalence of stroke, a debilitating central nervous system disorder impacting brain arteries that supply the brain with nutrients, continues to be identified as the fifth leading cause of mortality and severe long-term or lifelong disability throughout the US (Virani et al., 2021). There are two primary classes of stroke that predominate the clinical landscape, ischemic and hemorrhagic, with ischemic responsible for 87% of all strokes (Virani et al., 2021). Induction of acute ischemic stroke stems from the formation of a cerebrovascular occlusion by an embolus or a thrombotic event, resulting in a temporary or permanent loss of blood flow to a region of the brain. The sudden obstruction of blood circulation rapidly introduces a hypoxic and nutrient-deprived condition in the adjacent brain region, initiating several pathophysiological secondary injury pathways that progress with time, ultimately impairing intracellular homeostasis and resulting in a localized cerebral infarction (Heiss et al., 1999; Brzica et al., 2017). This primary brain injury can be attributed to the activation of secondary injury pathways that include ionic imbalance, oxidative stress, excitotoxicity, mitochondrial dysfunction, inflammation, and BBB breakdown (Dirnagl et al., 1999; Onwuekwe and Ezeala-Adikaibe 2012).
Early disruption of the BBB may result in a temporary, non-selective passage of toxic intracellular and extracellular substances that may increase the risk of secondary brain injury, in addition to progressing the pathogenesis of ischemic stroke (Howard et al., 1998; DiNapoli et al., 2008; Hossain et al., 2009). Furthermore, activation of microglia plays a crucial role in neuroinflammation and is followed by cellular or extracellular brain edema, both of which serve to aggravate disruption of the BBB further and worsen stroke outcome (Lipton 1999). Surrounding the ischemic cerebral infarction, where brain cells are irreversibly damaged and mostly necrotic, is the penumbral region consisting of functionally inactive neurons that are potentially salvageable (Kaufmann et al., 1999). Recovery of this region, by preventing the activation of further secondary brain injury cascades, is a major investigational neuroprotective strategy and therapeutic goal aiming to ultimately improve neuronal survivability and ischemic stroke outcome (Chamorro et al., 2016).
Blood Clotting, Thrombus Formation, and Use of Thrombolytics in Ischemic Stroke
Thrombin is a strong platelet agonist, with activated platelets serving to amplify additional thrombin generation through feedback activation of blood clotting factors V, VIII, and XI. Additionally, it further promotes vascular thrombosis by facilitating the cleavage of the fibrin zymogen (fibrinogen), as well as by activating factor XI, preventing fibrinolysis, and factor XIII, which further stabilizes the fibrin clot. Thrombin also functions as an anticoagulant when bound to thrombomodulin, a receptor protein expressed on the surface of the vascular endothelium, with thrombin-thrombomodulin binding ultimately leading to fibrinolysis. The dynamic nature of thrombin thus plays a critical role in response to inflammation and injury of the vascular endothelium (Levi et al., 2004; Nemmar et al., 2007).
Previous studies that focused on thrombosis have shown the presence of wide-spread crosstalk between clotting factors and inflammatory cytokines, indicating the existence of an interdependent and complementary relationship between coagulation and inflammation (Nemmar et al., 2009; Nemmar et al., 2011). Further evidence has shown that disruption of the mechanisms responsible for maintaining the antithrombotic and anti-inflammatory properties of the cerebral microvascular endothelium lead to an increased risk of ischemic stroke (del Zoppo and Hallenbeck 2000; Iadecola and Anrather 2011). A major therapeutic approach used to reduce the risk of stroke often involves antithrombotic agents that aim to prevent or reduce thrombosis. To date, intravenous administration (IV) of recombinant tissue plasminogen activator (r-tPA), a thrombolytic protein capable of conducting fibrinolysis, is the only US-FDA approved therapeutic for use in acute ischemic stroke. Although useful, the safety and efficacy of r-tPA significantly diminishes if not administered quickly following the onset of stroke (Gurewich 2016). Furthermore, lower thrombus extent and greater thrombus permeability have been associated with successful recanalization of the occluded artery in cerebral ischemia (Menon et al., 2018).
Effects of Prior Nicotine, Tobacco Smoke, or E-Cig Exposure on Thrombosis and Use of r-tPA
Smoking of tobacco, a well-known risk factor of ischemic stroke, increases the risk of cerebrovascular thrombosis and worsens stroke outcome (Shah and Cole 2010). Promotion of thrombosis proceeds through the mechanisms involved in vascular endothelial dysfunction (Noronha-Dutra et al., 1993; Nagy et al., 1997; Raij et al., 2001; Chen et al., 2004; Naik et al., 2014), resulting in increased platelet activation (Roy 1999), inflammation (Arnson et al., 2010), and impaired fibrinolysis (Jaffre et al., 2015). TS induced vascular endothelial dysfunction has been shown to be mediated by the deleterious properties of TS, primarily those relating to reactive oxygen species (ROS) content (Naik et al., 2014), nicotine, and oxidative stress derived inflammation, in a dose-dependent manner (Gill et al., 1989; Das et al., 2009; Arnson et al., 2010; Paulson et al., 2010; Naik et al., 2014). Interestingly when the effects of e-cig vapor and TS were compared, it was found that the oxidative stress promoted through nicotine exposure from either source induced similar detrimental effects, from a thrombolytic perspective, on BBB integrity, cellular inflammation, and stroke outcome (Kaisar et al., 2017). Such effects include platelet activation, as a result of upregulated endothelial synthesis and release of circulatory tissue factor, platelet-activating factor, catecholamines, thromboxane, and von Willebrand factor (Lip and Blann 1997; Girdhar et al., 2008; Togna et al., 2008).
Additionally, nicotine can increase the risk of thrombosis through the increased cellular expression of the pro-inflammatory cytokine TNF-α and plasminogen activator inhibitor-1, as well as the downregulation of NrF2, which regulates the antioxidative response system, and thrombomodulin, responsible for the crucial anticoagulant properties of thrombin (Lau et al., 2006; Prasad et al., 2015; Kaisar et al., 2017; Prasad et al., 2017). Upregulation of C-reactive protein has also been observed, functioning to promote endothelial dysfunction by diminishing nitric oxide (NO) synthesis and NO bioactivity (Verma et al., 2002). Collectively, these nicotine-mediated factors result in altered blood homeostasis that consequentially promotes platelet-dependent thrombosis, increases the risk of ischemic stroke, and exacerbates secondary brain injury (Wannamethee et al., 2005; Al-Awadhi et al., 2008). While nicotine has been implicated in vascular endothelial dysfunction and subsequent thrombus formation, other factors such as the combustion of e-liquid solvents, typically propylene glycol and glycerin, may also play an important role. Further, e-liquid combustion is known to form a variety of highly toxic aldehydes, such as acrolein, formaldehyde, and acetaldehyde, that ultimately function as biocides that may promote endothelial dysfunction and thrombus formation (Beauchamp et al., 1985; Conklin et al., 2009; Wheat et al., 2011; Erickson 2015).
Although tobacco use increases the risk of ischemic stroke, some studies have demonstrated a paradoxical association between current smoking and favorable stroke outcome following intravenous thrombolysis with r-tPA or endovascular treatment (Ovbiagele and Saver 2005; Ali et al., 2013; Kufner et al., 2013; Kvistad et al., 2014; Hussein et al., 2017; Kufner et al., 2021). Studies of this smoking-thrombolysis paradox have shown that TS use is associated with reduced infarct growth, as well as a higher rate of recanalization and reperfusion in TS users treated with IV r-tPA, following ischemic stroke (Kufner et al., 2013). The pathophysiology responsible for this paradox and the apparent increase in r-tPA treatment efficacy is thought to be based on clot formation and the reduced release of endogenous tPA from a smoker’s vascular endothelium (Rosenberg and Aird 1999; Sambola et al., 2003; Kufner et al., 2021). Although this TS-induced dysfunction of the vascular endothelium ultimately results in blood hypercoagulability and increased risk of thrombosis, the thrombi formed from such dysfunction are likely more fibrin-rich (Meade et al., 1987; Barua et al., 2010). As these thrombi are composed of elevated levels of fibrin, it has been hypothesized that they may be more receptive to treatment with exogenous r-tPA compared to thrombi with unelevated levels of fibrin (Gomez et al., 1993; Grines et al., 1995). In other words, this modification of clot dynamics, originating from TS use, may result in earlier recanalization of the occluded artery and favorable stroke outcome in patients treated with r-tPA.
Effect of Nicotine on Nutrient and Ion Transport Functioning During Ischemia
The brain microenvironment heavily depends on the supply of solutes, nutrients, and ions for its normal physiological functioning and metabolism (Bélanger et al., 2011). The brain uses glucose as its primary fuel for ATP production, and the transporters involved in its uptake are the isoforms GLUT1 and GLUT3 within various cells in the brain (Maher et al., 1994; Vannucci et al., 1997; Lundgaard et al., 2015). However, the transport of glucose across the BBB mostly occurs from facilitative GLUT1 expressed by the brain endothelial cells in a 1:4 ratio on its luminal and abluminal membranes, respectively (Shah et al., 2012). Once transported into the brain’s extracellular space, it is primarily utilized by neurons and by additional components of the neurovascular unit to maintain brain activity (Lundgaard et al., 2015).
During ischemia, the demand for energy increases due to the loss of blood supply into the brain (Sims and Muyderman 2010). There is an initial increase in glucose utilization, followed by decreased glucose metabolism within the brain (McCall et al., 1996). Furthermore, studies have shown that, as a regulatory mechanism, there is an increase in expression of GLUT1 through activation of phosphoinositide-3 kinase (PI3K)/Akt pathway via hypoxia-inducible factor-1 (HIF-1) (Yeh et al., 2008). This is supported by another study that showed the absence of hypoxic GLUT1 regulation in HIF-1 knock-out animals (Wood et al., 1998). Overall, HIF-1 induction of GLUT1 is crucial in causing increased glucose uptake for adaptation of energy demand in ischemia. Also, since glucose transport becomes the rate-limiting step for the cerebral glucose metabolism in ischemic conditions, determination of expression of GLUT1 on both luminal and abluminal for understanding glucose transport kinetics is vital in ischemic conditions (Simpson et al., 2007). Interestingly, pre-exposure to nicotine reduced ischemia-enhanced GLUT1 expression across the brain and caused exacerbation of ischemia-induced brain damage, adversely affecting stroke outcome (Shah et al., 2015). Also, nicotine and cotinine treatment decreased neuronal glucose uptake in ischemic conditions mediated through upregulation of a7 subtype of nAchR (Sifat et al., 2018). Moreover, e-cig vaping in vivo showed that both GLUT1 and GLUT3 expressions were decreased in both normoxic and ischemic conditions (Sifat et al., 2018). Although these studies suggest that nicotine in e-cig vaping plays a crucial role in compromising stroke outcome, other chemical constituents such as glycerin, propylene glycol, and flavoring agents (Kaur et al., 2018), shown by studies to have specific toxicities, need to be investigated separately for effects on ischemic stroke outcome and potential therapy.
Ischemic stroke is characterized by cellular swelling due to neuronal depolarization caused by GABAA receptor activation and a resulting influx of potassium and chloride (Payne et al., 2003). The cellular edema that occurs is followed by vasogenic edema that worsens the damage, and overall, the phenomenon is observed as BBB opening (Unterberg et al., 2004). As a neuroprotective mechanism, the NKCC activity at the BBB basolateral side increases during ischemia as it is phosphorylated by protein kinase C (PKC) to remove excess potassium from the brain (Yerby et al., 1997; Abbruscato et al., 2004). Furthermore, shuttle of sodium, potassium, and chloride occur away from the brain extracellular space, reducing the ability of GABAA receptors to cause depolarization and cellular swelling (Abbruscato et al., 2004). However, this neuroprotective compensatory increase in NKCC activity following ischemia is altered in the presence of nicotine (Paulson et al., 2006). Additionally, to understand the effects of tobacco smoke constituents on NKCC activity, nicotine-free tobacco smoke extract treatment was evaluated and reported that it did not negatively alter the activity of the transporter (Paulson et al., 2006). This finding supports nicotine as the responsible player for altering the NKCC activity, therefore worsening ischemic stroke compensatory mechanism. Opposite to NKCC, the Na, K-ATPase transporter at the BBB interface, in hypoxic conditions, has shown to have decreased function (Kawai et al., 1996). With nicotine treatment, the expression of Na, K-ATPase further decreases and worsens focal cerebral ischemia (Wang et al., 1996) (Figure 1).
Tobacco Smoking and E-Cig Vaping in Worsening of Other Neurological Diseases and Nicotine-CNS Drug Interactions
Alzheimer’s Disease
Alzheimer’s disease (AD) is the most common cause of elderly-age dementia and is characterized by the accumulation of amyloid-β (Aβ) protein in extracellular senile plaques and neurofibrillary tangles due to intraneuronal tau deposition (Mucke 2009). Additionally, cerebrovascular dysfunction exists, partially due to impaired cerebral blood flow characterized by decreased nitric oxide synthesis derived from endothelium and nitrergic nerves (de La Torre 2012). Moreover, cerebral hypoperfusion is believed to decrease the clearance of intraneuronal tau and induce accumulation of Aβ protein (Zhiyou et al., 2009; Mawuenyega et al., 2010).
Nicotine has been shown to increase the mRNA expression of amyloid precursor protein in the amygdala and hippocampus (Gutala et al., 2006). In an animal model of AD, TS exposure increased neuroinflammation, amyloidogenesis, and tau phosphorylation (Moreno-Gonzalez et al., 2013). Furthermore, nicotine has been shown to negate NO-induced vasodilation and cause cerebral hypoperfusion by degrading the NO, possibly resulting from oxidative stress (Arnson et al., 2010; Toda and Toda 2010). However, it cannot be excluded that except for nicotine, chemicals like acrolein and methyl vinyl ketone, present in TS and e-cigs have been shown to cause oxidative stress by the generation of ROS through activation of protein kinase C via NADPH oxidase (Asano et al., 2012). A retrospective population-based study reported that people with TS exposure are at increased risk for AD (Ott et al., 1998). The correlation is based on findings that showed smokers without the APOE-4 allele have a higher incidence of developing this risk, and by contrast, smokers with this allele are not at increased risk.
The current pharmacotherapy for AD is based on helping patients alleviate the symptoms and preserve their mental well-being by regulating the neurotransmitters acetylcholine and glutamate (Anand et al., 2017). Acetyl-cholinesterase inhibitors, such as donepezil, help elevate acetylcholine levels in the brain by inhibiting its breakdown by the enzyme acetylcholinesterase (McGleenon et al., 1999). This therapy is mostly used to treat mild to moderate AD. Similarly, NMDA receptor antagonists such as memantine reverse the adverse effects of increased levels of glutamate in the brain (Olivares et al., 2012) and is often prescribed to treat moderate to severe AD. Interestingly, data from an in vitro BBB model showed that donepezil and memantine significantly reduced nicotine uptake (Nakanishi et al., 2018). Moreover, memantine has been reported to be transported by a cationic influx H+ antiporter similar to the brain’s nicotine transport system (Mehta et al., 2013). Thus, it can be extrapolated that nicotine and anti-AD drugs like donepezil and memantine may share a similar transport system and compete for their transport into the brain. Given these data, it is highly likely that nicotine taken up by TS or e-cig affects the transport of donepezil and memantine; in vivo uptake studies in an animal model of AD are warranted to investigate CNS drug interaction due to TS or e-cig exposure.
Schizophrenia
Schizophrenia is a heterogenous psychotic disorder where patients experience delusions, negative symptoms, hallucinations, and behavioral and cognitive dysfunction (Najjar et al., 2017). The pathophysiological mechanisms are believed to be interconnected between immune, inflammatory, neurotransmitter, genetic, and oxidative pathways (Pun et al., 2009; Anderson et al., 2013). Growing preclinical and clinical studies show that in schizophrenia, neurovascular uncoupling occurs due to oxidative stress and neuroinflammation, which ultimately leads to increased BBB permeability and its breakdown (Abbott 2002; Grove et al., 2015). These alterations have been shown to worsen behavioral and cognitive symptoms in schizophrenic patients and are correlated to reduced cerebral perfusion and defective innate and adaptive immunity that signals neuroinflammatory responses in the brain (Serlin et al., 2011).
TS has been shown to cause higher mortality in schizophrenic patients and is linked to more severe symptoms that require higher doses of anti-psychotics (Schwartz et al., 2005; Salokangas et al., 2006). Literature suggests that people with schizophrenia smoke heavily to relieve pyramidal symptoms associated with adverse effects from anti-psychotic treatment; however, smoking is known to contribute to disease progression and cannot be recommended as a viable source of symptom relief (Levin 2006). Nicotine, TS, and e-cig exposure leading to BBB dysfunction are well-reported; nevertheless, BBB-related loss of cerebrovascular integrity in smokers that might play a role in the exacerbation of schizophrenia has not been studied well. Moreover, it is essential to note that pharmacokinetic interactions have been reported with smokers undergoing pharmacotherapy for schizophrenia (Desai et al., 2001).
Olanzapine and clozapine are atypical anti-psychotics that are the first-line treatment choice in schizophrenia. A pharmacokinetic study showed that TS increased clearance of olanzapine by 23% because of the induction of CYP1A2 enzyme activity in the liver (Callaghan et al., 1999). Another study reported that with TS there was a 33% increase in clearance of clozapine in smokers compared to the non-smoking population (Ng et al., 2009). This would then affect the drug plasma levels and thus cause inadequate concentration in the brain to exert therapeutic activity. Therefore, careful consideration of dosage is required in the smoking population of schizophrenia. Nevertheless, smoking regularity should also be assessed simultaneously with anti-psychotic courses because studies reported that patients who quit smoking had their plasma drug concentration levels rise by 50–70%, potentially leading to adverse drug reactions (Lowe and Ackman 2010). Additionally, it must be noted that both nicotine and by-products of TS and e-cigs such as polycyclic aromatic hydrocarbons are inducers of CYP1A2, hence deciphering their individual role in interaction with anti-psychotics can be beneficial for clinical translation (Zevin and Benowitz 1999; Hukkanen et al., 2011).
Neuro-AIDS
NeuroAIDS is a neurodegenerative disease that manifests on a spectrum of neurological disorders (Minagar et al., 2008). Manifestations can range from decreased attention and concentration, decreased psychomotor speed, decreased memory and learning, and an overall decrease in executive functions of the brain. There is also a slowing of motor functions that can lead to tremors, extrapyramidal symptoms, and paralysis. The clinical manifestations of this disease range from asymptomatic neurocognitive dysfunction to mild neurocognitive disorder to full-blown HIV-associated dementia (Shapshak et al., 2011).
It is hypothesized that during an HIV infection, the virus invades the BBB, causing dysregulation using viral proteins and cytokines (Kaul et al., 2001). One thing of note here is that NeuroAIDS is not caused by the infection of the virus in the neurons, it is the neurotoxic nature of the virus: such as viral proteins gp120, and oxidative stress exerted by the cytokine dysregulation causing the breakdown of the BBB, leading to neurocognitive dysfunction (Bhalerao and Cucullo 2020). It has been described earlier that TS causes endothelial dysregulation and oxidative stress on the BBB, leading to an increased coagulative state in the blood. Moreover, this can be dangerous to the cerebrovascular system, especially in the presence of infectious agents that affect the permeability of the BBB (Atluri et al., 2015). When it comes to TS and NeuroAIDS, reports vary in the extent to which it plays a role in the disease progression. A study reported that having a nicotine concentration of 300 μM in the blood plasma increased the HIV-1 expression (Abbud et al., 1995).
When comparing HIV-1 positive smokers to healthy individuals who smoke, another study found that through the induction of cytochrome P450 enzymes, the metabolism of nicotine is enhanced in patients with HIV-1 (Earla et al., 2014). Enhanced nicotine metabolism is known to form reactive metabolites. These reactive metabolites cause oxidative stress, which has been shown to cause an increase in viral load and replication (Ande et al., 2013). It is clear then that the optimal way to treat a severe case of NeuroAIDS is to stop viral replication. Since the diagnosis of NeuroAIDS is made using physiological function tests and clinical symptoms, these tests fail to incorporate the minute phenotypic variations that might be present from person to person (Owe-Larsson et al., 2009). With the use of biomarkers looking for specific endophenotypes, some progress has been made when it comes to determining the extent of neurodegeneration in the brain due to HIV infection (Rahimian and He 2017). However, these biomarkers cannot be used for therapeutic monitoring because they do not show if the damaged tissue is healing. The damage and inflammation caused by ROS due to chronic smoking has been well established. There is hope that the discovery of common biomarkers for both NeuroAIDS and smoking can help identify more synergy between the two and give rise to better treatments and outcomes for HIV-infected patients.
HIV patients have depleted immune systems, and the use and abuse of addictive substances like nicotine are injurious to their overall health (Friedman et al., 2003). From this point of view, tobacco smoking is more prevalent in HIV patients than the general population (Rahmanian et al., 2011). In fact, a study reported that this prevalence is > 2-times higher than the general population who are known smokers (Desai et al., 2020). Nicotine, absorbed from smoking and vaping, is primarily metabolized by CYP2A6 and CYP2B6 (Lucas et al., 2017; Bloom et al., 2019). A study reported that smoking negatively affects the metabolism of antiretroviral therapies (ARTs) such as efavirenz and nevirapine, which are metabolized through CYP2A6 and CYP2B6 (Gong et al., 2019). Moreover, smokers have increased expression of CYP1A1, which can increase the metabolism ARTs that undergo metabolism through this pathway, such as dolutegravir resulting in a higher drug clearance value (Zhu et al., 2018). Nicotine has also been reported to induce UGT1A1 in HIV-infected patients and thus could directly affect the metabolism of abacavir, raltegravir, and elvitegravir that use similar enzyme metabolism systems (Chastain et al., 2017; Parant et al., 2019).
Multiple Sclerosis
Multiple sclerosis (MS) is primarily a neurodegenerative disease that is caused by chronic inflammatory demyelination of nervous tissue in the brain and spinal cord (Rothhammer and Quintana 2015). During this demyelination, triggered by oxidative stress, astrocytes become reactive and promote further tissue damage (Ponath et al., 2018). Furthermore, macrophages of the brain secrete chemical signals that upregulate the secretion of cytokines such as TNF-α, IL-1β, and IL-6 (Contreras et al., 2016). These cytokines, along with neurotrophic factors such as brain-derived neurotrophic factor, nerve growth factor, and vascular endothelial growth factor, all exacerbate the state of chronic inflammation in the CNS (Ashraf-Uz-Zaman et al., 2022). Since MS has been correlated with an impaired immune system, it can be assumed that the pathology of the disease is attributed to genetic and environmental factors (Mitrovic et al., 1995). Thus, recognizing these factors that play a role in the development of the disease is essential for preventing the progression of MS.
One modifiable risk factor for poor outcomes is smoking (Riise et al., 2003). In recent years, it has been implied that TS may play a role in MS pathogenesis and that it might also affect how the disease progresses and how severe it will be (Ghasemi et al., 2017). An essential protein that plays a role in the pathogenesis of MS is calcium-binding protein B (S100B) (Bartosik Psujek et al., 2011). It is an important biomarker for disease activity because it detects specific T cells against S100B. Interestingly, the level of S100B was significantly higher among smokers with MS than with non-smokers (Paknejad et al., 2019). Additionally, oxidative stress is an important factor when it comes to MS because, more recently, it has been tied to both inflammation and demyelination (Haider et al., 2011). TS plays a major role in neuroinflammation than neurodegeneration (Socha et al., 2014). A subclass of T cells called mucosal-associated invariant T-cells has been shown to have a protective role in MS by expressing more proinflammatory chemokines (Ammitzbøll et al., 2019). Due to the constant irritation of smoke on the cell surfaces of the lungs, these cells are expressed more in chronic smokers (Fischer et al., 2012). They release chemokines that keep the immune system busy, creating inflammation in the lungs, thereby having a pseudo-protective effect from MS in the CNS.
Since MS is not curable, most of the therapies around it are to treat the symptoms. These therapies are called disease-modifying treatments (DMTs) (Doshi and Chataway 2017). The first-generation DMTs are all injectable drugs and have a linear PK relationship, while the second generation of DMT consists of three oral agents: monomethyl fumarate, dimethyl fumarate, and teriflunomide (Scolding et al., 2015). Of the three oral agents, teriflunomide acts on the cytochrome P450 system by inhibiting CYP2C8 while inducing CYP1A2 (Ferreira et al., 2021). Furthermore, smoking also induces CYP1A2, and therefore, an MS patient who is a smoker and taking teriflunomide puts themselves at high risk for hepatotoxicity (van der Weide et al., 2003). MS occurs due to a combination of environmental and genetic risk factors; therefore, it is difficult to say with certainty how significant of a role nicotine and TS play in the pathogenesis of the disease. However, most of the reviewed literature does agree that TS could be associated with triggering the onset of symptoms of the disease, increasing the likelihood of relapse, and increasing the risk of hepatotoxicity with DMTs (Jasielski et al., 2020). The role of smoking in MS and other neurodegenerative disorder needs to be investigated further. In the case of MS, there is a clear lack of knowledge when it comes to how nicotine, TS or e-cig exposure affect the disease.
In addition to effects of nicotine in aforementioned neurological disorders, TS has been linked as a predisposition factor in the development of depression (Anda et al., 1990; Mendelsohn 2012). Conversely, there is evidence that depressive symptoms lead to smoking or smoking cessation could precipitate depression and induce a return to smoking (Glassman et al., 1988; Anda et al., 1990). Moreover, CYP1A2 induction from TS causes increased clearance of anti-depressant drugs such as imipramine and fluvoxamine, and can mimic side effects from anti-depressants such as tremors (Heishma et al., 1994; Spina and Scordo 2002). Therefore, careful dosage adjustment is required for smoking populations that are on anti-depressants. It is also important to note that a significant number of studies have reported beneficial effects of nicotine in neurological disorders such as Parkinson’s disease (PD) (Morens et al., 1995; Quik and Kulak 2002; Quik 2004). This protective effect of nicotine is related to stimulation of the selectively damaged nigrostriatal dopamine neurons and protection against neuronal insults in experimental PD models (Champtiaux et al., 2003; Quik et al., 2003). Additionally, epidemiological studies from >40 independent studies showed that PD is less prevalent in smokers (Quik 2004). Contrary to these findings, some studies reported that chronic nicotine exposure reduces dopamine turnover and decreases catecholamine free radical formation that might damage the dopaminergic neurons (Kirch et al., 1988; Newhouse and Hughes 1991).
Conclusion
Overall, nicotine, TS, and e-cig vaping could be prodromal to cerebrovascular impairment and worsen ischemic stroke and a variety of other neurological disorders. Moreover, components, in addition to nicotine, in TS and e-cigs, might play a role in the deterioration of CNS diseases; thus, an individual toxic potential should be assessed in normal and pathological states for future investigations. Also, detailed transporter and enzyme-based studies on nicotine interactions in brain uptake of CNS drugs are warranted to understand the effects of smoking/vaping on CNS drug interactions. These types of studies should be routine for preclinical screening of new and old neurotherapeutics.
Author Contributions
Conceptualization, SS and TA; investigation, SS, SR, VK, KM, MR, YZ, and TA; funding acquisition, TA. All authors have read and agreed to the published version of the manuscript.
Funding
This work was supported by the National Institutes of Health National Institute of Neurologic Disorders and Stroke (R01NS106879) and National Institute on Drug Abuse (R01DA049737, R01DA029121).
Conflict of Interest
The authors declare that the research was conducted in the absence of any commercial or financial relationships that could be construed as a potential conflict of interest.
Publisher’s Note
All claims expressed in this article are solely those of the authors and do not necessarily represent those of their affiliated organizations, or those of the publisher, the editors and the reviewers. Any product that may be evaluated in this article, or claim that may be made by its manufacturer, is not guaranteed or endorsed by the publisher.
References
Abbott, N. J. (2002). Astrocyte-endothelial Interactions and Blood-Brain Barrier Permeability. J. Anat. 200 (5), 523–534. doi:10.1046/j.1469-7580.2002.00047_13.x
Abbott, N. J., Patabendige, A. A. K., Dolman, D. E. M., Yusof, S. R., and Begley, D. J. (2010). Structure and Function of the Blood-Brain Barrier. Neurobiol. Dis. 37 (1), 13–25. doi:10.1016/j.nbd.2009.07.030
Abbruscato, T. J., Lopez, S. P., Mark, K. S., Hawkins, B. T., and Davis, T. P. (2002). Nicotine and Cotinine Modulate Cerebral Microvascular Permeability and Protein Expression of ZO-1 through Nicotinic Acetylcholine Receptors Expressed on Brain Endothelial Cells. J. Pharm. Sci. 91 (12), 2525–2538. doi:10.1002/jps.10256
Abbruscato, T. J., Lopez, S. P., Roder, K., and Paulson, J. R. (2004). Regulation of Blood-Brain Barrier Na,K,2Cl-Cotransporter through Phosphorylation during In Vitro Stroke Conditions and Nicotine Exposure. J. Pharmacol. Exp. Ther. 310 (2), 459–468. doi:10.1124/jpet.104.066274
Abbud, R. A., Finegan, C. K., Guay, L. A., and Rich, E. A. (1995). Enhanced Production of Human Immunodeficiency Virus Type 1 by In Vitro-infected Alveolar Macrophages from Otherwise Healthy Cigarette Smokers. J. Infect. Dis. 172 (3), 859–863. doi:10.1093/infdis/172.3.859
Abdullahi, W., Davis, T. P., and Ronaldson, P. T. (2017). Functional Expression of P-Glycoprotein and Organic Anion Transporting Polypeptides at the Blood-Brain Barrier: Understanding Transport Mechanisms for Improved CNS Drug Delivery? Aaps J. 19 (4), 931–939. doi:10.1208/s12248-017-0081-9
Al-Awadhi, A. M., AlFadhli, S. M., Mustafa, N. Y., and Sharma, P. N. (2008). Effects of cigarette smoking on hematological parameters and von Willebrand factor functional activity levels in asymptomatic male and female Arab smokers. Med. Princ Pract. 17 (2), 149–153. doi:10.1159/000112970
Ali, S. F., Smith, E. E., Bhatt, D. L., Fonarow, G. C., and Schwamm, L. H. (2013). Paradoxical Association of Smoking with In-Hospital Mortality Among Patients Admitted with Acute Ischemic Stroke. J. Am. Heart Assoc. 2 (3), e000171. doi:10.1161/JAHA.113.000171
Ammitzbøll, C., Börnsen, L., Petersen, E. R., Oturai, A. B., Søndergaard, H. B., Grandjean, P., et al. (2019). Perfluorinated Substances, Risk Factors for Multiple Sclerosis and Cellular Immune Activation. J. neuroimmunology 330, 90–95. doi:10.1016/j.jneuroim.2019.03.002
Anand, A., Patience, A. A., Sharma, N., and Khurana, N. (2017). The Present and Future of Pharmacotherapy of Alzheimer's Disease: A Comprehensive Review. Eur. J. Pharmacol. 815, 364–375. doi:10.1016/j.ejphar.2017.09.043
Anda, R. F., Williamson, D. F., Escobedo, L. G., Mast, E. E., Giovino, G. A., and Remington, P. L. (1990). Depression and the Dynamics of Smoking. Jama 264 (12), 1541–1545. doi:10.1001/jama.1990.03450120053028
Ande, A., McArthur, C., Kumar, A., and Kumar, S. (2013). Tobacco Smoking Effect on HIV-1 Pathogenesis: Role of Cytochrome P450 Isozymes. Expert Opin. Drug Metab. Toxicol. 9 (11), 1453–1464. doi:10.1517/17425255.2013.816285
Anderson, G., Berk, M., Dodd, S., Bechter, K., Altamura, A. C., Dell'Osso, B., et al. (2013). Immuno-inflammatory, Oxidative and Nitrosative Stress, and Neuroprogressive Pathways in the Etiology, Course and Treatment of Schizophrenia. Prog. Neuro-Psychopharmacology Biol. Psychiatry 42, 1–4. doi:10.1016/j.pnpbp.2012.10.008
Archie, S. R., Al Shoyaib, A., and Cucullo, L. (2021). Blood-Brain Barrier Dysfunction in CNS Disorders and Putative Therapeutic Targets: An Overview. Pharmaceutics 13 (11), 1779. doi:10.3390/pharmaceutics13111779
Archie, S. R., Sharma, S., Burks, E., and Abbruscato, T. (2022). Biological Determinants Impact the Neurovascular Toxicity of Nicotine and Tobacco Smoke: a Pharmacokinetic and Pharmacodynamic Perspective. NeuroToxicology 89, 140–160. doi:10.1016/j.neuro.2022.02.002
Arnson, Y., Shoenfeld, Y., and Amital, H. (2010). Effects of Tobacco Smoke on Immunity, Inflammation and Autoimmunity. J. Autoimmun. 34 (3), J258–J265. doi:10.1016/j.jaut.2009.12.003
Asano, H., Horinouchi, T., Mai, Y., Sawada, O., Fujii, S., Nishiya, T., et al. (2012). Nicotine- and Tar-free Cigarette Smoke Induces Cell Damage through Reactive Oxygen Species Newly Generated by PKC-dependent Activation of NADPH Oxidase. J. Pharmacol. Sci. 118 (2), 275–287. doi:10.1254/jphs.11166fp
Ashraf-Uz-Zaman, M., Ji, G., Tidwell, D., Yin, L., Thakolwiboon, S., Pan, J., et al. (2022). Evaluation of Urea-Based Inhibitors of the Dopamine Transporter Using the Experimental Autoimmune Encephalomyelitis Model of Multiple Sclerosis. ACS Chem. Neurosci. 13 (2), 217–228. doi:10.1021/acschemneuro.1c00647
Atluri, V. S. R., Hidalgo, M., Samikkannu, T., Kurapati, K. R. V., Jayant, R. D., Sagar, V., et al. (2015). Effect of Human Immunodeficiency Virus on Blood-Brain Barrier Integrity and Function: an Update. Front. Cel. Neurosci. 9, 212. doi:10.3389/fncel.2015.00212
Awtry, T. L., and Werling, L. L. (2003). Acute and Chronic Effects of Nicotine on Serotonin Uptake in Prefrontal Cortex and hippocampus of Rats. Synapse 50 (3), 206–211. doi:10.1002/syn.10259
Backinger, C. L., Meissner, H. I., and Ashley, D. L. (2016). The FDA "Deeming Rule" and Tobacco Regulatory Research. Tobacco Reg. Sci. 2 (3), 290–293. doi:10.18001/trs.2.3.8
Bagchi, S., Chhibber, T., Lahooti, B., Verma, A., Borse, V., and Jayant, R. D. (2019). In-vitro Blood-Brain Barrier Models for Drug Screening and Permeation Studies: an Overview. Dddt Vol. 13, 3591–3605. doi:10.2147/dddt.s218708
Barrington-Trimis, J. L., and Leventhal, A. M. (2018). Adolescents' Use of "Pod Mod" E-Cigarettes - Urgent Concerns. N. Engl. J. Med. 379 (12), 1099–1102. doi:10.1056/nejmp1805758
Bartosik‐Psujek, H., Psujek, M., Jaworski, J., and Stelmasiak, Z. (2011). Total Tau and S100b Proteins in Different Types of Multiple Sclerosis and during Immunosuppressive Treatment with Mitoxantrone. Acta Neurol. Scand. 123 (4), 252–256. doi:10.1111/j.1600-0404.2010.01393.x
Barua, R. S., Sy, F., Srikanth, S., Huang, G., Javed, U., Buhari, C., et al. (2010). Effects of Cigarette Smoke Exposure on Clot Dynamics and Fibrin Structure. Atvb 30 (1), 75–79. doi:10.1161/atvbaha.109.195024
Beauchamp, R. O., Andjelkovich, D. A., Kligerman, A. D., Morgan, K. T., Heck, H. d. A., and Feron, V. J. (1985). A Critical Review of the Literature on Acrolein Toxicity. CRC Crit. Rev. Toxicol. 14, 309–380. doi:10.3109/10408448509037461
Bélanger, M., Allaman, I., and Magistretti, P. J. (2011). Brain Energy Metabolism: Focus on Astrocyte-Neuron Metabolic Cooperation. Cel Metab. 14 (6), 724–738. doi:10.1016/j.cmet.2011.08.016
Benowitz, N. L., Hukkanen, J., and Jacob, P. (2009). Nicotine Chemistry, Metabolism, Kinetics and Biomarkers. Handb Exp. Pharmacol. 2009 (192), 29–60. doi:10.1007/978-3-540-69248-5_2
Bernard, A., Ku, J. M., Vlahos, R., and Miller, A. A. (2019). Cigarette Smoke Extract Exacerbates Hyperpermeability of Cerebral Endothelial Cells after Oxygen Glucose Deprivation and Reoxygenation. Sci. Rep. 9 (1), 15573. doi:10.1038/s41598-019-51728-2
Bhalerao, A., and Cucullo, L. (2020). Impact of Tobacco Smoke in HIV Progression: a Major Risk Factor for the Development of NeuroAIDS and Associated CNS Disorders. J. Public Health (Berl.) 28 (3), 259–270. doi:10.1007/s10389-019-01062-8
Bloom, A. J., Wang, P. F., and Kharasch, E. D. (2019). Nicotine Oxidation by Genetic Variants of CYP 2B6 and in Human Brain Microsomes. Pharmacol. Res. Perspect. 7 (2), e00468. doi:10.1002/prp2.468
Boudanova, E., Navaroli, D. M., and Melikian, H. E. (2008). Amphetamine-induced Decreases in Dopamine Transporter Surface Expression Are Protein Kinase C-independent. Neuropharmacology 54 (3), 605–612. doi:10.1016/j.neuropharm.2007.11.007
Boulton, D. W., DeVane, C. L., Liston, H. L., and Markowitz, J. S. (2002). In Vitro P-glycoprotein Affinity for Atypical and Conventional Antipsychotics. Life Sci. 71 (2), 163–169. doi:10.1016/s0024-3205(02)01680-6
Brzica, H., Abdullahi, W., Ibbotson, K., and Ronaldson, P. T. (2017). Role of Transporters in central Nervous System Drug Delivery and Blood-Brain Barrier protection: Relevance to Treatment of Stroke. J. Cent. Nerv Syst. Dis. 9, 1179573517693802. doi:10.1177/1179573517693802
Callaghan, J. T., Bergstrom, R. F., Ptak, L. R., and Beasley, C. M. (1999). Olanzapine. Clin. Pharmacokinet. 37 (3), 177–193. doi:10.2165/00003088-199937030-00001
Chamorro, Á., Dirnagl, U., Urra, X., and Planas, A. M. (2016). Neuroprotection in Acute Stroke: Targeting Excitotoxicity, Oxidative and Nitrosative Stress, and Inflammation. Lancet Neurol. 15 (8), 869–881. doi:10.1016/s1474-4422(16)00114-9
Champtiaux, N., Gotti, C., Cordero-Erausquin, M., David, D. J., Przybylski, C., Léna, C., et al. (2003). Subunit Composition of Functional Nicotinic Receptors in Dopaminergic Neurons Investigated with Knock-Out Mice. J. Neurosci. 23 (21), 7820–7829. doi:10.1523/jneurosci.23-21-07820.2003
Chastain, D. B., Franco-Paredes, C., and Stover, K. R. (2017). Addressing Antiretroviral Therapy-Associated Drug-Drug Interactions in Patients Requiring Treatment for Opportunistic Infections in Low-Income and Resource-Limited Settings. J. Clin. Pharmacol. 57 (11), 1387–1399. doi:10.1002/jcph.978
Chen, H.-W., Chien, M.-L., Chaung, Y.-H., Lii, C.-K., and Wang, T.-S. (2004). Extracts from Cigarette Smoke Induce DNA Damage and Cell Adhesion Molecule Expression through Different Pathways. Chemico-biological interactions 150 (3), 233–241. doi:10.1016/j.cbi.2004.09.014
Cheng, T. (2014). Chemical Evaluation of Electronic Cigarettes. Tob. Control. 23 Suppl 2 (Suppl. 2), ii11–7. doi:10.1136/tobaccocontrol-2013-051482
Cisternino, S., Chapy, H., André, P., Smirnova, M., Debray, M., and Scherrmann, J.-M. (2013). Coexistence of Passive and Proton Antiporter-Mediated Processes in Nicotine Transport at the Mouse Blood-Brain Barrier. Aaps J. 15 (2), 299–307. doi:10.1208/s12248-012-9434-6
Conklin, D. J., Haberzettl, P., Prough, R. A., and Bhatnagar, A. (2009). Glutathione-S-transferase P Protects against Endothelial Dysfunction Induced by Exposure to Tobacco Smoke. Am. J. Physiology-Heart Circulatory Physiol. 296 (5), H1586–H1597. doi:10.1152/ajpheart.00867.2008
Contreras, F., Prado, C., González, H., Franz, D., Osorio-Barrios, F., Osorio, F., et al. (2016). Dopamine Receptor D3 Signaling on CD4+ T Cells Favors Th1- and Th17-Mediated Immunity. J.I. 196 (10), 4143–4149. doi:10.4049/jimmunol.1502420
Das, S., Gautam, N., Dey, S. K., Maiti, T., and Roy, S. (2009). Oxidative Stress in the Brain of Nicotine-Induced Toxicity: Protective Role ofAndrographis paniculataNees and Vitamin E. Appl. Physiol. Nutr. Metab. 34 (2), 124–135. doi:10.1139/h08-147
de La Torre, J. C. (2012). Cardiovascular Risk Factors Promote Brain Hypoperfusion Leading to Cognitive Decline and Dementia. Cardiovasc. Psychiatry Neurol. 2012, 367516. doi:10.1155/2012/367516
del Zoppo, G. J., and Hallenbeck, J. M. (2000). Advances in the Vascular Pathophysiology of Ischemic Stroke. Thromb. Res. 98 (3), 73–81. doi:10.1016/s0049-3848(00)00218-8
Desai, H. D., Seabolt, J., and Jann, M. W. (2001). Smoking in Patients Receiving Psychotropic Medications. CNS drugs 15 (6), 469–494. doi:10.2165/00023210-200115060-00005
Desai, N., Burns, L., Gong, Y., Zhi, K., Kumar, A., Summers, N., et al. (2020). An Update on Drug-Drug Interactions between Antiretroviral Therapies and Drugs of Abuse in HIV Systems. Expert Opin. Drug Metab. Toxicol. 16 (11), 1005–1018. doi:10.1080/17425255.2020.1814737
DiNapoli, V. A., Huber, J. D., Houser, K., Li, X., and Rosen, C. L. (2008). Early Disruptions of the Blood-Brain Barrier May Contribute to Exacerbated Neuronal Damage and Prolonged Functional Recovery Following Stroke in Aged Rats. Neurobiol. Aging 29 (5), 753–764. doi:10.1016/j.neurobiolaging.2006.12.007
Dirnagl, U., Iadecola, C., and Moskowitz, M. A. (1999). Pathobiology of Ischaemic Stroke: an Integrated View. Trends Neurosciences 22 (9), 391–397. doi:10.1016/s0166-2236(99)01401-0
Doran, A., Obach, R. S., Smith, B. J., Hosea, N. A., Becker, S., Callegari, E., et al. (2005). The Impact of P-Glycoprotein on the Disposition of Drugs Targeted for Indications of the central Nervous System: Evaluation Using the MDR1A/1B Knockout Mouse Model. Drug Metab. Dispos 33 (1), 165–174. doi:10.1124/dmd.104.001230
Doshi, A., and Chataway, J. (2017). Multiple Sclerosis, a Treatable Disease. Clin. Med. 17 (6), 530–536. doi:10.7861/clinmedicine.17-6-530
Earla, R., Ande, A., McArthur, C., Kumar, A., and Kumar, S. (2014). Enhanced Nicotine Metabolism in HIV-1-Positive Smokers Compared with HIV-Negative Smokers: Simultaneous Determination of Nicotine and its Four Metabolites in Their Plasma Using a Simple and Sensitive Electrospray Ionization Liquid Chromatography-Tandem Mass Spectrometry Technique. Drug Metab. Dispos 42 (2), 282–293. doi:10.1124/dmd.113.055186
Einarson, T., Addis, A., Mittmann, N., and Iskedjian, M. (1998). Meta-analysis of Venlafaxine, SSRIs and TCAs in the Treatment of Major Depressive Disorder. Can. J. Clin. Pharmacol. 5, 205–216.
Ellingrod, V. L. (2013). Drug Interactions with Tobacco Smoke: Implications for Patient Care. Curr. Psychiatry 12 (1), 12–16.
Erickson, B. E. (2015). Boom in E-Cigarettes sparks Debate. Chem. Eng. News Archive 93 (7), 10–13. doi:10.1021/cen-09307-cover
Ferreira, C. M., Vasconcelos-Pereira, E. F., Oliveira, V. M., Salgado, P. R., Domingos, J. A., Monreal, M. T. F. D., et al. (2021). Hepatotoxicity Associated with the Use of Teriflunomide in a Patient with Multiple Sclerosis: A Case Report. Medicine (Baltimore) 100 (51), e28246. doi:10.1097/MD.0000000000028246
Fischer, M. T., Sharma, R., Lim, J. L., Haider, L., Frischer, J. M., Drexhage, J., et al. (2012). NADPH Oxidase Expression in Active Multiple Sclerosis Lesions in Relation to Oxidative Tissue Damage and Mitochondrial Injury. Brain 135 (3), 886–899. doi:10.1093/brain/aws012
Fowles, J., and Dybing, E. (2003). Application of Toxicological Risk Assessment Principles to the Chemical Constituents of Cigarette Smoke. Tob. Control 12 (4), 424–430. doi:10.1136/tc.12.4.424
Friedman, H., Newton, C., and Klein, T. W. (2003). Microbial Infections, Immunomodulation, and Drugs of Abuse. Clin. Microbiol. Rev. 16 (2), 209–219. doi:10.1128/cmr.16.2.209-219.2003
Fukada, A., Saito, H., and Inui, K.-i. (2002a). Transport Mechanisms of Nicotine across the Human Intestinal Epithelial Cell Line Caco-2. J. Pharmacol. Exp. Ther. 302 (2), 532–538. doi:10.1124/jpet.102.034629
Fukada, A., Saito, H., Urakami, Y., Okuda, M., and Inui, K.-i. (2002b). Involvement of Specific Transport System of Renal Basolateral Membranes in Distribution of Nicotine in Rats. Drug Metab. Pharmacokinet. 17 (6), 554–560. doi:10.2133/dmpk.17.554
Ghasemi, N., Razavi, S., and Nikzad, E. (2017). Multiple Sclerosis: Pathogenesis, Symptoms, Diagnoses and Cell-Based Therapy. Cell J 19 (1), 1–10. doi:10.22074/cellj.2016.4867
Gill, J. S., Shipley, M. J., Tsementzis, S. A., Hornby, R., Gill, S. K., Hitchcock, E. R., et al. (1989). Cigarette Smoking. A Risk Factor for Hemorrhagic and Nonhemorrhagic Stroke. Arch. Intern. Med. 149 (9), 2053–2057. doi:10.1001/archinte.149.9.2053
Gillman, I. G., Kistler, K. A., Stewart, E. W., and Paolantonio, A. R. (2016). Effect of Variable Power Levels on the Yield of Total Aerosol Mass and Formation of Aldehydes in E-Cigarette Aerosols. Regul. Toxicol. Pharmacol. 75, 58–65. doi:10.1016/j.yrtph.2015.12.019
Girdhar, G., Xu, S., Jesty, J., and Bluestein, D. (2008). In Vitro model of Platelet-Endothelial Activation Due to Cigarette Smoke under Cardiovascular Circulation Conditions. Ann. Biomed. Eng. 36 (7), 1142–1151. doi:10.1007/s10439-008-9503-2
Glassman, A. H., Stetner, F., Walsh, B. T., Raizman, P. S., Fleiss, J. L., Cooper, T. B., et al. (1988). Heavy Smokers, Smoking Cessation, and Clonidine. Jama 259 (19), 2863–2866. doi:10.1001/jama.1988.03720190031026
Gomez, M. A., Karagounis, L. A., Allen, A., Anderson, J. L., and investigators, T.-. (1993). Effect of Cigarette Smoking on Coronary Patency after Thrombolytic Therapy for Myocardial Infarction. Am. J. Cardiol. 72 (5), 373–378. doi:10.1016/0002-9149(93)91125-2
Gong, Y., Haque, S., Chowdhury, P., Cory, T. J., Kodidela, S., Yallapu, M. M., et al. (2019). Pharmacokinetics and Pharmacodynamics of Cytochrome P450 Inhibitors for HIV Treatment. Expert Opin. Drug Metab. Toxicol. 15 (5), 417–427. doi:10.1080/17425255.2019.1604685
Goniewicz, M. L., Knysak, J., Gawron, M., Kosmider, L., Sobczak, A., Kurek, J., et al. (2014). Levels of Selected Carcinogens and Toxicants in Vapour from Electronic Cigarettes. Tob. Control. 23 (2), 133–139. doi:10.1136/tobaccocontrol-2012-050859
Grines, C. L., Topol, E. J., O’Neill, W. W., George, B. S., Kereiakes, D., Phillips, H. R., et al. (1995). Effect of Cigarette Smoking on Outcome after Thrombolytic Therapy for Myocardial Infarction. Circulation 91 (2), 298–303. doi:10.1161/01.cir.91.2.298
Grove, T., Taylor, S., Dalack, G., and Ellingrod, V. (2015). Endothelial Function, Folate Pharmacogenomics, and Neurocognition in Psychotic Disorders. Schizophr Res. 164 (1-3), 115–121. doi:10.1016/j.schres.2015.02.006
Gurewich, V. (2016). Thrombolysis: a Critical First-Line Therapy with an Unfulfilled Potential. Am. J. Med. 129 (6), 573–575. doi:10.1016/j.amjmed.2015.11.033
Gutala, R., Wang, J., Hwang, Y. Y., Haq, R., and Li, M. D. (2006). Nicotine Modulates Expression of Amyloid Precursor Protein and Amyloid Precursor-like Protein 2 in Mouse Brain and in SH-Sy5y Neuroblastoma Cells. Brain Res. 1093 (1), 12–19. doi:10.1016/j.brainres.2006.03.100
Haider, L., Fischer, M. T., Frischer, J. M., Bauer, J., Hoftberger, R., Botond, G., et al. (2011). Oxidative Damage in Multiple Sclerosis Lesions. Brain 134 (7), 1914–1924. doi:10.1093/brain/awr128
Hans, F. J., Wei, L., Bereczki, D., Acuff, V., Demaro, J., Chen, J. L., et al. (1993). Nicotine Increases Microvascular Blood Flow and Flow Velocity in Three Groups of Brain Areas. Am. J. Physiology-Heart Circulatory Physiol. 265 (6), H2142–H2150. doi:10.1152/ajpheart.1993.265.6.h2142
Hawkins, B. T., Abbruscato, T. J., Egleton, R. D., Brown, R. C., Huber, J. D., Campos, C. R., et al. (2004). Nicotine Increases In Vivo Blood-Brain Barrier Permeability and Alters Cerebral Microvascular Tight junction Protein Distribution. Brain Res. 1027 (1-2), 48–58. doi:10.1016/j.brainres.2004.08.043
Health and Services (2006). The Health Consequences of Involuntary Exposure to Tobacco Smoke: A Report of the Surgeon General. Atlanta, GA: US Department of Health and Human Services, Centers for Disease.
Heishma, S. J., Taylor, R. C., and Henningfield, J. E. (1994). Nicotine and Smoking: a Review of Effects on Human Performance. Exp. Clin. Psychopharmacol. 2 (4), 345–395. doi:10.1037/1064-1297.2.4.345
Heiss, W.-D., Thiel, A., Grond, M., and Graf, R. (1999). Which Targets Are Relevant for Therapy of Acute Ischemic Stroke? Stroke 30 (7), 1486–1489. doi:10.1161/01.str.30.7.1486
Hossain, M., Sathe, T., Fazio, V., Mazzone, P., Weksler, B., Janigro, D., et al. (2009). Tobacco Smoke: A Critical Etiological Factor for Vascular Impairment at the Blood-Brain Barrier. Brain Res. 1287, 192–205. doi:10.1016/j.brainres.2009.06.033
Howard, G., Wagenknecht, L. E., Cai, J., Cooper, L., Kraut, M. A., and Toole, J. F. (1998). Cigarette Smoking and Other Risk Factors for Silent Cerebral Infarction in the General Population. Stroke 29 (5), 913–917. doi:10.1161/01.str.29.5.913
Hukkanen, J., Jacob III, P., Peng, M., Dempsey, D., and Benowitz, N. L. (2011). Effect of Nicotine on Cytochrome P450 1A2 Activity. Br. J. Clin. Pharmacol. 72 (5), 836–838. doi:10.1111/j.1365-2125.2011.04023.x
Hussein, H. M., Niemann, N., Parker, E. D., and Qureshi, A. I. (2017). Searching for the Smoker's Paradox in Acute Stroke Patients Treated with Intravenous Thrombolysis. Nicotine Tob. Res. 19 (7), 871–876. doi:10.1093/ntr/ntx020
Iadecola, C., and Anrather, J. (2011). The Immunology of Stroke: from Mechanisms to Translation. Nat. Med. 17 (7), 796–808. doi:10.1038/nm.2399
Itagaki, S., Ganapathy, V., Ho, H. T. B., Zhou, M., Babu, E., and Wang, J. (2012). Electrophysiological Characterization of the Polyspecific Organic Cation Transporter Plasma Membrane Monoamine Transporter. Drug Metab. Dispos 40 (6), 1138–1143. doi:10.1124/dmd.111.042432
Itoh, H., Toyohira, Y., Ueno, S., Saeki, S., Zhang, H., Furuno, Y., et al. (2010). Upregulation of Norepinephrine Transporter Function by Prolonged Exposure to Nicotine in Cultured Bovine Adrenal Medullary Cells. Naunyn-schmied Arch. Pharmacol. 382 (3), 235–243. doi:10.1007/s00210-010-0540-7
Jaffre, A., Ruidavets, J. B., Nasr, N., Guidolin, B., Ferrieres, J., and Larrue, V. (2015). Tobacco Use and Cryptogenic Stroke in Young Adults. J. Stroke Cerebrovasc. Dis. 24 (12), 2694–2700. doi:10.1016/j.jstrokecerebrovasdis.2015.07.022
Jasielski, P., Piędel, F., Rocka, A., Petit, V., and Rejdak, K. (2020). Smoking as a Risk Factor of Onset and Relapse of Multiple Sclerosis - a Review. Neurol. Neurochir Pol. 54 (3), 243–251. doi:10.5603/pjnns.a2020.0032
Kadry, H., Noorani, B., Bickel, U., Abbruscato, T. J., and Cucullo, L. (2021). Comparative Assessment of In Vitro BBB Tight junction Integrity Following Exposure to Cigarette Smoke and E-Cigarette Vapor: a Quantitative Evaluation of the Protective Effects of Metformin Using Small-Molecular-Weight Paracellular Markers. Fluids Barriers CNS 18 (1), 28–15. doi:10.1186/s12987-021-00261-4
Kaisar, M. A., Sivandzade, F., Bhalerao, A., and Cucullo, L. (2018). Conventional and Electronic Cigarettes Dysregulate the Expression of Iron Transporters and Detoxifying Enzymes at the Brain Vascular Endothelium: In Vivo Evidence of a Gender-specific Cellular Response to Chronic Cigarette Smoke Exposure. Neurosci. Lett. 682, 1–9. doi:10.1016/j.neulet.2018.05.045
Kaisar, M. A., Villalba, H., Prasad, S., Liles, T., Sifat, A. E., Sajja, R. K., et al. (2017). Offsetting the Impact of Smoking and E-Cigarette Vaping on the Cerebrovascular System and Stroke Injury: Is Metformin a Viable Countermeasure? Redox Biol. 13, 353–362. doi:10.1016/j.redox.2017.06.006
Kasza, K. A., Ambrose, B. K., Conway, K. P., Borek, N., Taylor, K., Goniewicz, M. L., et al. (2017). Tobacco-product Use by Adults and Youths in the United States in 2013 and 2014. N. Engl. J. Med. 376 (4), 342–353. doi:10.1056/nejmsa1607538
Kaufmann, A. M., Firlik, A. D., Fukui, M. B., Wechsler, L. R., Jungries, C. A., and Yonas, H. (1999). Ischemic Core and Penumbra in Human Stroke. Stroke 30 (1), 93–99. doi:10.1161/01.str.30.1.93
Kaul, M., Garden, G. A., and Lipton, S. A. (2001). Pathways to Neuronal Injury and Apoptosis in HIV-Associated Dementia. Nature 410 (6831), 988–994. doi:10.1038/35073667
Kaur, G., Muthumalage, T., and Rahman, I. (2018). Mechanisms of Toxicity and Biomarkers of Flavoring and Flavor Enhancing Chemicals in Emerging Tobacco and Non-tobacco Products. Toxicol. Lett. 288, 143–155. doi:10.1016/j.toxlet.2018.02.025
Kawai, N., McCarron, R. M., and Spatz, M. (1996). Effect of Hypoxia on Na(+)-K(+)-Cl- Cotransport in Cultured Brain Capillary Endothelial Cells of the Rat. J. Neurochem. 66 (6), 2572–2579. doi:10.1046/j.1471-4159.1996.66062572.x
Kirch, D. G., Alho, A.-M., and Wyatt, R. J. (1988). Hypothesis: a Nicotine-Dopamine Interaction Linking Smoking with Parkinson's Disease and Tardive Dyskinesia. Cell Mol Neurobiol 8 (3), 285–291. doi:10.1007/bf00711170
Krist, A. H., Krist, A. H., Davidson, K. W., Mangione, C. M., Barry, M. J., Cabana, M., et al. (2021). Interventions for Tobacco Smoking Cessation in Adults, Including Pregnant Persons: US Preventive Services Task Force Recommendation Statement. Jama 325 (3), 265–279. doi:10.1001/jama.2020.25019
Kufner, A., Ali, H. F., Ebinger, M., Fiebach, J. B., Liebeskind, D. S., Endres, M., et al. (2021). The Smoking Paradox in Ischemic Stroke Patients Treated with Intra-arterial Thrombolysis in Combination with Mechanical thrombectomy-VISTA-Endovascular. PloS one 16 (5), e0251888. doi:10.1371/journal.pone.0251888
Kufner, A., Nolte, C. H., Galinovic, I., Brunecker, P., Kufner, G. M., Endres, M., et al. (2013). Smoking-Thrombolysis Paradox. Stroke 44 (2), 407–413. doi:10.1161/strokeaha.112.662148
Kuntic, M., Oelze, M., Steven, S., Kröller-Schön, S., Stamm, P., Kalinovic, S., et al. (2020). Short-term E-Cigarette Vapour Exposure Causes Vascular Oxidative Stress and Dysfunction: Evidence for a Close Connection to Brain Damage and a Key Role of the Phagocytic NADPH Oxidase (NOX-2). Eur. Heart J. 41 (26), 2472–2483. doi:10.1093/eurheartj/ehz772
Kvistad, C. E., Oeygarden, H., Logallo, N., Thomassen, L., Waje-Andreassen, U., and Naess, H. (2014). Is Smoking Associated with Favourable Outcome in tPA-Treated Stroke Patients? Acta Neurol. Scand. 130 (5), 299–304. doi:10.1111/ane.12225
Lau, P. P., Li, L., Merched, A. J., Zhang, A. L., Ko, K. W. S., and Chan, L. (2006). Nicotine Induces Proinflammatory Responses in Macrophages and the Aorta Leading to Acceleration of Atherosclerosis in Low-Density Lipoprotein Receptor −/− Mice. Atvb 26 (1), 143–149. doi:10.1161/01.atv.0000193510.19000.10
Laviolette, S. R., and van der Kooy, D. (2003). Blockade of Mesolimbic Dopamine Transmission Dramatically Increases Sensitivity to the Rewarding Effects of Nicotine in the Ventral Tegmental Area. Mol. Psychiatry 8 (1), 50–59. doi:10.1038/sj.mp.4001197
Laviolette, S. R., and Van Der Kooy, D. (2004). The Neurobiology of Nicotine Addiction: Bridging the gap from Molecules to Behaviour. Nat. Rev. Neurosci. 5 (1), 55–65. doi:10.1038/nrn1298
Lee, N.-Y., Choi, H.-O., and Kang, Y.-S. (2012). The Acetylcholinesterase Inhibitors Competitively Inhibited an Acetyl L-Carnitine Transport through the Blood-Brain Barrier. Neurochem. Res. 37 (7), 1499–1507. doi:10.1007/s11064-012-0723-3
Levi, M., van der Poll, T., and Büller, H. R. (2004). Bidirectional Relation between Inflammation and Coagulation. Circulation 109 (22), 2698–2704. doi:10.1161/01.cir.0000131660.51520.9a
Levin, E. D. (2006). Neurotransmitter Interactions and Cognitive Function. Springer Science & Business Media.
Li, H., Li, X., Gao, S., Wang, D., Gao, X., Li, Y., et al. (2019). Exposure to Cigarette Smoke Augments post-ischemic Brain Injury and Inflammation via Mobilization of Neutrophils and Monocytes. Front. Immunol. 10, 2576. doi:10.3389/fimmu.2019.02576
Linnet, K., and Ejsing, T. B. (2008). A Review on the Impact of P-Glycoprotein on the Penetration of Drugs into the Brain. Focus on Psychotropic Drugs. Eur. Neuropsychopharmacol. 18 (3), 157–169. doi:10.1016/j.euroneuro.2007.06.003
Lip, G., and Blann, A. (1997). von Willebrand factor: a marker of endothelial dysfunction in vascular disorders? Cardiovasc. Res. 34 (2), 255–265. doi:10.1016/s0008-6363(97)00039-4
Lipton, P. (1999). Ischemic Cell Death in Brain Neurons. Physiol. Rev. 79 (4), 1431–1568. doi:10.1152/physrev.1999.79.4.1431
Lowe, E. J., and Ackman, M. L. (2010). Impact of Tobacco Smoking Cessation on Stable Clozapine or Olanzapine Treatment. Ann. Pharmacother. 44 (4), 727–732. doi:10.1345/aph.1m398
Lucas, C. J., Patel, J., and Martin, J. H. (2017). Predicting Drug Interactions in Addiction Treatment. Intern. Med. J. 47 (8), 872–878. doi:10.1111/imj.13500
Lundgaard, I., Li, B., Xie, L., Kang, H., Sanggaard, S., Haswell, J. D., et al. (2015). Direct Neuronal Glucose Uptake Heralds Activity-dependent Increases in Cerebral Metabolism. Nat. Commun. 6 (1), 6807–6812. doi:10.1038/ncomms7807
Maher, F., Vannucci, S. J., and Simpson, I. A. (1994). Glucose Transporter Proteins in Brain. FASEB j. 8 (13), 1003–1011. doi:10.1096/fasebj.8.13.7926364
Mansvelder, H. D., and McGehee, D. S. (2002). Cellular and Synaptic Mechanisms of Nicotine Addiction. J. Neurobiol. 53 (4), 606–617. doi:10.1002/neu.10148
Marsot, A., and Simon, N. (2016). Nicotine and Cotinine Levels with Electronic Cigarette. Int. J. Toxicol. 35 (2), 179–185. doi:10.1177/1091581815618935
Mathers, C. D., and Loncar, D. (2006). Projections of Global Mortality and burden of Disease from 2002 to 2030. Plos Med. 3 (11), e442. doi:10.1371/journal.pmed.0030442
Mawuenyega, K. G., Sigurdson, W., Ovod, V., Munsell, L., Kasten, T., Morris, J. C., et al. (2010). Decreased Clearance of CNS β-Amyloid in Alzheimer's Disease. Science 330 (6012), 1774. doi:10.1126/science.1197623
McCall, A. L., Van Bueren, A. M., Nipper, V., Moholt-Siebert, M., Downes, H., and Lessov, N. (1996). Forebrain Ischemia Increases GLUT1 Protein in Brain Microvessels and Parenchyma. J. Cereb. Blood Flow Metab. 16 (1), 69–76. doi:10.1097/00004647-199601000-00008
McCubbin, A., Fallin-Bennett, A., Barnett, J., and Ashford, K. (2017). Perceptions and Use of Electronic Cigarettes in Pregnancy. Health Educ. Res. 32 (1), 22–32. doi:10.1093/her/cyw059
McGleenon, B., Dynan, K., and Passmore, A. (1999). Acetylcholinesterase Inhibitors in Alzheimer's Disease. Br. J. Clin. Pharmacol. 48 (4), 471–480. doi:10.1046/j.1365-2125.1999.00026.x
McIntosh, E. C., Tureson, K., Rotblatt, L. J., Singer, E. J., and Thames, A. D. (2021). HIV, Vascular Risk Factors, and Cognition in the Combination Antiretroviral Therapy Era: a Systematic Review and Meta-Analysis. J. Int. Neuropsychol. Soc. 27 (4), 365–381. doi:10.1017/s1355617720001022
Meade, T. W., Imeson, J., and Stirling, Y. (1987). Effects of Changes in Smoking and Other Characteristics on Clotting Factors and the Risk of Ischaemic Heart Disease. The Lancet 330 (8566), 986–988. doi:10.1016/s0140-6736(87)92556-6
Mehta, D. C., Short, J. L., and Nicolazzo, J. A. (2013). Memantine Transport across the Mouse Blood-Brain Barrier Is Mediated by a Cationic Influx H+ Antiporter. Mol. Pharmaceutics 10 (12), 4491–4498. doi:10.1021/mp400316e
Menon, B. K., Al-Ajlan, F. S., Najm, M., Puig, J., Castellanos, M., Dowlatshahi, D., et al. (2018). Association of Clinical, Imaging, and Thrombus Characteristics with Recanalization of Visible Intracranial Occlusion in Patients with Acute Ischemic Stroke. Jama 320 (10), 1017–1026. doi:10.1001/jama.2018.12498
Minagar, A., Commins, D., Alexander, J. S., Hoque, R., Chiappelli, F., Singer, E. J., et al. (2008). NeuroAIDS. Mol. Diag Ther. 12 (1), 25–43. doi:10.1007/bf03256266
Mitrovic, B., Ignarro, L. J., Vinters, H. V., Akers, M.-A., Schmid, I., Uittenbogaart, C., et al. (1995). Nitric Oxide Induces Necrotic but Not Apoptotic Cell Death in Oligodendrocytes. Neuroscience 65 (2), 531–539. doi:10.1016/0306-4522(94)00491-m
Miyajima, M., Kusuhara, H., Fujishima, M., Adachi, Y., and Sugiyama, Y. (2011). Organic Anion Transporter 3 Mediates the Efflux Transport of an Amphipathic Organic Anion, Dehydroepiandrosterone Sulfate, across the Blood-Brain Barrier in Mice. Drug Metab. Dispos 39 (5), 814–819. doi:10.1124/dmd.110.036863
Moccia, F., Frost, C., Berra-Romani, R., Tanzi, F., and Adams, D. J. (2004). Expression and Function of Neuronal Nicotinic ACh Receptors in Rat Microvascular Endothelial Cells. Am. J. Physiology-Heart Circulatory Physiol. 286 (2), H486–H491. doi:10.1152/ajpheart.00620.2003
Molden, E., and Spigset, O. (2009). Tobakksrøyking Og Interaksjoner Med Legemidler. Tidsskriftetny raekke 129 (7), 632–633. doi:10.4045/tidsskr.08.0122
Moreno-Gonzalez, I., Estrada, L. D., Sanchez-Mejias, E., and Soto, C. (2013). Smoking Exacerbates Amyloid Pathology in a Mouse Model of Alzheimer's Disease. Nat. Commun. 4 (1), 1–10. doi:10.1038/ncomms2494
Morens, D. M., Grandinetti, A., Reed, D., White, L. R., and Ross, G. W. (1995). Cigarette Smoking and protection from Parkinson's Disease: False Association or Etiologic Clue? Neurology 45 (6), 1041–1051. doi:10.1212/wnl.45.6.1041
Nagy, J., Demaster, E. G., Wittman, I., Shultz, P., and Raij, L. (1997). Induction of Endothelial Cell Injury by Cigarette Smoke. Endothelium 5 (4), 251–263. doi:10.3109/10623329709052590
Naik, P., Fofaria, N., Prasad, S., Sajja, R. K., Weksler, B., Couraud, P. O., et al. (2014). Oxidative and Pro-inflammatory Impact of Regular and Denicotinized Cigarettes on Blood Brain Barrier Endothelial Cells: Is Smoking Reduced or Nicotine-free Products Really Safe? BMC Neurosci. 15 (1), 51–14. doi:10.1186/1471-2202-15-51
Najjar, S., Pahlajani, S., De Sanctis, V., Stern, J. N. H., Najjar, A., and Chong, D. (2017). Neurovascular Unit Dysfunction and Blood-Brain Barrier Hyperpermeability Contribute to Schizophrenia Neurobiology: A Theoretical Integration of Clinical and Experimental Evidence. Front. Psychiatry 8, 83. doi:10.3389/fpsyt.2017.00083
Nakanishi, T., Tamai, I., and Deguchi, Y. (2018). Recent Advances in Research on Biological Membranes that Regulate the Central Nervous System Foreword, PHARMACEUTICAL SOC JAPAN 2-12-15 SHIBUYA, SHIBUYA-KU, TOKYO, 150-0002, JAPAN. Biol. Pharm. Bull. 41, 1322–1323. doi:10.1248/bpb.b18-ctf4109
Nemmar, A., Al-Salam, S., Dhanasekaran, S., Sudhadevi, M., and Ali, B. H. (2009). Pulmonary Exposure to Diesel Exhaust Particles Promotes Cerebral Microvessel Thrombosis: Protective Effect of a Cysteine Prodrug L-2-Oxothiazolidine-4-Carboxylic Acid. Toxicology 263 (2-3), 84–92. doi:10.1016/j.tox.2009.06.017
Nemmar, A., Al-Salam, S., Zia, S., Marzouqi, F., Al-Dhaheri, A., Subramaniyan, D., et al. (2011). Contrasting Actions of Diesel Exhaust Particles on the Pulmonary and Cardiovascular Systems and the Effects of Thymoquinone. Br. J. Pharmacol. 164 (7), 1871–1882. doi:10.1111/j.1476-5381.2011.01442.x
Nemmar, A., Hoet, P. H. M., Vandervoort, P., Dinsdale, D., Nemery, B., and Hoylaerts, M. F. (2007). Enhanced Peripheral Thrombogenicity after Lung Inflammation Is Mediated by Platelet-Leukocyte Activation: Role of P-Selectin. J. Thromb. Haemost. 5 (6), 1217–1226. doi:10.1111/j.1538-7836.2007.02557.x
Newhouse, P. A., and Hughes, J. R. (1991). The Role of Nicotine and Nicotinic Mechanisms in Neuropsychiatric Disease. Addiction 86 (5), 521–526. doi:10.1111/j.1360-0443.1991.tb01801.x
Ng, W., Uchida, H., Ismail, Z., Mamo, D. C., Rajji, T. K., Remington, G., et al. (2009). Clozapine Exposure and the Impact of Smoking and Gender: a Population Pharmacokinetic Study. Ther. Drug Monit. 31 (3), 360–366. doi:10.1097/ftd.0b013e31819c7037
Noronha-Dutra, A. A., Epperlein, M. M., and Woolf, N. (1993). Effect of Cigarette Smoking on Cultured Human Endothelial Cells. Cardiovasc. Res. 27 (5), 774–778. doi:10.1093/cvr/27.5.774
O'donnell, M. E., Tran, L., Lam, T. I., Liu, X. B., and Anderson, S. E. (2004). Bumetanide Inhibition of the Blood-Brain Barrier Na-K-Cl Cotransporter Reduces Edema Formation in the Rat Middle Cerebral Artery Occlusion Model of Stroke. J. Cereb. Blood Flow Metab. 24 (9), 1046–1056. doi:10.1097/01.wcb.0000130867.32663.90
Okura, T., Hattori, A., Takano, Y., Sato, T., Hammarlund-Udenaes, M., Terasaki, T., et al. (2008). Involvement of the Pyrilamine Transporter, a Putative Organic Cation Transporter, in Blood-Brain Barrier Transport of Oxycodone. Drug Metab. Dispos 36 (10), 2005–2013. doi:10.1124/dmd.108.022087
Olivares, D., K. Deshpande, V., Shi, Y., K. Lahiri, D., H. Greig, N., T. Rogers, J., et al. (2012). N-Methyl D-Aspartate (NMDA) Receptor Antagonists and Memantine Treatment for Alzheimer's Disease, Vascular Dementia and Parkinson's Disease. Car 9 (6), 746–758. doi:10.2174/156720512801322564
Onwuekwe, I., and Ezeala-Adikaibe, B. (2012). Ischemic Stroke and Neuroprotection. Ann. Med. Health Sci. Res. 2 (2), 186–190. doi:10.4103/2141-9248.105669
Ott, A., Slooter, A., Hofman, A., van Harskamp, F., Witteman, J., Van Broeckhoven, C., et al. (1998). Smoking and Risk of Dementia and Alzheimer's Disease in a Population-Based Cohort Study: the Rotterdam Study. The Lancet 351 (9119), 1840–1843. doi:10.1016/s0140-6736(97)07541-7
Ovbiagele, B., and Saver, J. L. (2005). The Smoking-Thrombolysis Paradox and Acute Ischemic Stroke. Neurology 65 (2), 293–295. doi:10.1212/01.wnl.0000168163.72351.f3
Owe-Larsson, B., Säll, L., Salamon, E., and Allgulander, C. (2009). HIV Infection and Psychiatric Illness. Afr. J. Psychiatry (Johannesbg) 12 (2), 115–128. doi:10.4314/ajpsy.v12i2.43729
Paknejad, B., Shirkhanloo, H., and Aliomrani, M. (2019). Is There Any Relevance between Serum Heavy Metal Concentration and BBB Leakage in Multiple Sclerosis Patients? Biol. Trace Elem. Res. 190 (2), 289–294. doi:10.1007/s12011-018-1553-1
Pandit, R., Chen, L., and Götz, J. (2020). The Blood-Brain Barrier: Physiology and Strategies for Drug Delivery. Adv. Drug Deliv. Rev. 165-166, 1–14. doi:10.1016/j.addr.2019.11.009
Parant, F., Miailhes, P., Brunel, F., and Gagnieu, M.-C. (2019). Dolutegravir Population Pharmacokinetics in a Real-Life Cohort of People Living with HIV Infection: a Covariate Analysis. Ther. Drug Monit. 41 (4), 444–451. doi:10.1097/ftd.0000000000000618
Paulson, J. R., Roder, K. E., McAfee, G., Allen, D. D., Van der Schyf, C. J., and Abbruscato, T. J. (2006). Tobacco Smoke Chemicals Attenuate Brain-To-Blood Potassium Transport Mediated by the Na,K,2Cl-Cotransporter during Hypoxia-Reoxygenation. J. Pharmacol. Exp. Ther. 316 (1), 248–254. doi:10.1124/jpet.105.090738
Paulson, J. R., Yang, T., Selvaraj, P. K., Mdzinarishvili, A., Van der Schyf, C. J., Klein, J., et al. (2010). Nicotine Exacerbates Brain Edema during In Vitro and In Vivo Focal Ischemic Conditions. J. Pharmacol. Exp. Ther. 332 (2), 371–379. doi:10.1124/jpet.109.157776
Payne, J. A., Rivera, C., Voipio, J., and Kaila, K. (2003). Cation-chloride Co-transporters in Neuronal Communication, Development and Trauma. Trends Neurosciences 26 (4), 199–206. doi:10.1016/s0166-2236(03)00068-7
Perikleous, E. P., Steiropoulos, P., Paraskakis, E., Constantinidis, T. C., and Nena, E. (2018). E-cigarette Use Among Adolescents: an Overview of the Literature and Future Perspectives. Front. Public Health 6, 86. doi:10.3389/fpubh.2018.00086
Ponath, G., Park, C., and Pitt, D. (2018). The Role of Astrocytes in Multiple Sclerosis. Front. Immunol. 9, 217. doi:10.3389/fimmu.2018.00217
Pontieri, F. E., Tanda, G., Orzi, F., and Chiara, G. D. (1996). Effects of Nicotine on the Nucleus Accumbens and Similarity to Those of Addictive Drugs. Nature 382 (6588), 255–257. doi:10.1038/382255a0
Prasad, S., Sajja, R. K., Park, J. H., Naik, P., Kaisar, M. A., and Cucullo, L. (2015). Impact of Cigarette Smoke Extract and Hyperglycemic Conditions on Blood-Brain Barrier Endothelial Cells. Fluids Barriers CNS 12 (1), 18–13. doi:10.1186/s12987-015-0014-x
Prasad, S., Sajja, R. K., Kaisar, M. A., Park, J. H., Villalba, H., Liles, T., et al. (2017). Role of Nrf2 and Protective Effects of Metformin against Tobacco Smoke-Induced Cerebrovascular Toxicity. Redox Biol. 12, 58–69. doi:10.1016/j.redox.2017.02.007
Praticò, D. (2008). Oxidative Stress Hypothesis in Alzheimer's Disease: a Reappraisal. Trends Pharmacological Sciences 29 (12), 609–615. doi:10.1016/j.tips.2008.09.001
Pun, P. B. L., Lu, J., and Moochhala, S. (2009). Involvement of ROS in BBB Dysfunction. Free Radic. Res. 43 (4), 348–364. doi:10.1080/10715760902751902
Qasim, H., Karim, Z. A., Silva-Espinoza, J. C., Khasawneh, F. T., Rivera, J. O., Ellis, C. C., et al. (2018). Short-Term E-Cigarette Exposure Increases the Risk of Thrombogenesis and Enhances Platelet Function in Mice. J. Am. Heart Assoc. 7 (15), e009264. doi:10.1161/JAHA.118.009264
Quik, M., and Kulak, J. M. (2002). Nicotine and Nicotinic Receptors; Relevance to Parkinson's Disease. Neurotoxicology 23 (4-5), 581–594. doi:10.1016/s0161-813x(02)00036-0
Quik, M. (2004). Smoking, Nicotine and Parkinson's Disease. Trends Neurosciences 27 (9), 561–568. doi:10.1016/j.tins.2004.06.008
Quik, M., Sum, J. D., Whiteaker, P., McCallum, S. E., Marks, M. J., Musachio, J., et al. (2003). Differential Declines in Striatal Nicotinic Receptor Subtype Function after Nigrostriatal Damage in Mice. Mol. Pharmacol. 63 (5), 1169–1179. doi:10.1124/mol.63.5.1169
Rahimian, P., and He, J. J. (2017). HIV/neuroAIDS Biomarkers. Prog. Neurobiol. 157, 117–132. doi:10.1016/j.pneurobio.2016.04.003
Rahmanian, S., Wewers, M. E., Koletar, S., Reynolds, N., Ferketich, A., and Diaz, P. (2011). Cigarette Smoking in the HIV-Infected Population. Proc. Am. Thorac. Soc. 8 (3), 313–319. doi:10.1513/pats.201009-058wr
Raij, L., DeMaster, E. G., and Jaimes, E. A. (2001). Cigarette Smoke-Induced Endothelium Dysfunction: Role of Superoxide Anion. J. Hypertens. 19 (5), 891–897. doi:10.1097/00004872-200105000-00009
Riise, T., Nortvedt, M. W., and Ascherio, A. (2003). Smoking Is a Risk Factor for Multiple Sclerosis. Neurology 61 (8), 1122–1124. doi:10.1212/01.wnl.0000081305.66687.d2
Ronaldson, P. T., and Davis, T. P. (2013). Targeted Drug Delivery to Treat Pain and Cerebral Hypoxia. Pharmacol. Rev. 65 (1), 291–314. doi:10.1124/pr.112.005991
Rosenberg, R. D., and Aird, W. C. (1999). Vascular-Bed-Specific Hemostasis and Hypercoagulable States. N. Engl. J. Med. 340 (20), 1555–1564. doi:10.1056/nejm199905203402007
Rothhammer, V., and Quintana, F. J. (2015). “Control of Autoimmune CNS Inflammation by Astrocytes,” in Seminars in Immunopathology (Springer). doi:10.1007/s00281-015-0515-3
Roy, S. (1999). Effects of Smoking on Prostacyclin Formation and Platelet Aggregation in Users of Oral Contraceptives. Am. J. Obstet. Gynecol. 180 (6), S364–S368. doi:10.1016/s0002-9378(99)70697-6
Salokangas, R. K. R., Honkonen, T., Stengård, E., Koivisto, A.-M., and Hietala, J. (2006). Cigarette Smoking in Long-Term Schizophrenia. Eur. Psychiatr. 21 (4), 219–223. doi:10.1016/j.eurpsy.2005.07.008
Sambola, A., Osende, J., Hathcock, J., Degen, M., Nemerson, Y., Fuster, V., et al. (2003). Role of Risk Factors in the Modulation of Tissue Factor Activity and Blood Thrombogenicity. Circulation 107 (7), 973–977. doi:10.1161/01.cir.0000050621.67499.7d
Schwartz, K., Iancu, I., Stryjer, R., Chelben, J., Kotler, M., Weizman, A., et al. (2005). Reduced Platelet Vesicular Monoamine Transporter Density in Smoking Schizophrenia Patients. Eur. Neuropsychopharmacol. 15 (5), 557–561. doi:10.1016/j.euroneuro.2005.02.005
Scolding, N., Barnes, D., Cader, S., Chataway, J., Chaudhuri, A., Coles, A., et al. (2015). Association of British Neurologists: Revised (2015) Guidelines for Prescribing Disease-Modifying Treatments in Multiple Sclerosis. Pract. Neurol. 15 (4), 273–279. doi:10.1136/practneurol-2015-001139
Serlin, Y., Levy, J., and Shalev, H. (2011). Vascular Pathology and Blood-Brain Barrier Disruption in Cognitive and Psychiatric Complications of Type 2 Diabetes Mellitus. Cardiovasc. Psychiatry Neurol. 2011, 609202. doi:10.1155/2011/609202
Shah, K., DeSilva, S., and Abbruscato, T. (2012). The Role of Glucose Transporters in Brain Disease: Diabetes and Alzheimer’s Disease. Int. J. Mol. Sci. 13 (10), 12629–12655. doi:10.3390/ijms131012629
Shah, K., and Abbruscato, T. (2014). The Role of Blood-Brain Barrier Transporters in Pathophysiology and Pharmacotherapy of Stroke. Cpd 20 (10), 1510–1522. doi:10.2174/13816128113199990465
Shah, K. K., Boreddy, P. R., and Abbruscato, T. J. (2015). Nicotine Pre-exposure Reduces Stroke-Induced Glucose Transporter-1 Activity at the Blood-Brain Barrier in Mice. Fluids Barriers CNS 12 (1), 10–19. doi:10.1186/s12987-015-0005-y
Shah, R. S., and Cole, J. W. (2010). Smoking and Stroke: the More You Smoke the More You Stroke. Expert Rev. Cardiovasc. Ther. 8 (7), 917–932. doi:10.1586/erc.10.56
Shapshak, P., Kangueane, P., Fujimura, R. K., Commins, D., Chiappelli, F., Singer, E., et al. (2011). Editorial neuroAIDS Review. AIDS (London, England) 25 (2), 123–141. doi:10.1097/qad.0b013e328340fd42
Sharma, S., Nozohouri, S., Vaidya, B., and Abbruscato, T. (2021). Repurposing Metformin to Treat Age-Related Neurodegenerative Disorders and Ischemic Stroke. Life Sci. 274, 119343. doi:10.1016/j.lfs.2021.119343
Shoaib, M., Gommans, J., Morley, A., Stolerman, I. P., Grailhe, R., and Changeux, J.-P. (2002). The Role of Nicotinic Receptor Beta-2 Subunits in Nicotine Discrimination and Conditioned Taste Aversion. Neuropharmacology 42 (4), 530–539. doi:10.1016/s0028-3908(01)00194-0
Sifat, A. E., Vaidya, B., Kaisar, M. A., Cucullo, L., and Abbruscato, T. J. (2018). Nicotine and Electronic Cigarette (E-Cig) Exposure Decreases Brain Glucose Utilization in Ischemic Stroke. J. Neurochem. 147 (2), 204–221. doi:10.1111/jnc.14561
Sifat, A. E., Vaidya, B., Villalba, H., Albekairi, T. H., and Abbruscato, T. J. (2019). Neurovascular Unit Transport Responses to Ischemia and Common Coexisting Conditions: Smoking and Diabetes. Am. J. Physiology-Cell Physiol. 316 (1), C2–C15. doi:10.1152/ajpcell.00187.2018
Simpson, D., and Plosker, G. L. (2004). Atomoxetine. Drugs 64 (2), 205–222. doi:10.2165/00003495-200464020-00005
Simpson, I. A., Carruthers, A., and Vannucci, S. J. (2007). Supply and Demand in Cerebral Energy Metabolism: the Role of Nutrient Transporters. J. Cereb. Blood Flow Metab. 27 (11), 1766–1791. doi:10.1038/sj.jcbfm.9600521
Sims, N. R., and Muyderman, H. (2010). Mitochondria, Oxidative Metabolism and Cell Death in Stroke. Mol. Basis Dis. 1802 (1), 80–91. doi:10.1016/j.bbadis.2009.09.003
Sivandzade, F., Prasad, S., Bhalerao, A., and Cucullo, L. (2019). NRF2 and NF-Қb Interplay in Cerebrovascular and Neurodegenerative Disorders: Molecular Mechanisms and Possible Therapeutic Approaches. Redox Biol. 21, 101059. doi:10.1016/j.redox.2018.11.017
Socha, K., Kochanowicz, J., Karpińska, E., Soroczyńska, J., Jakoniuk, M., Mariak, Z., et al. (2014). Dietary Habits and Selenium, Glutathione Peroxidase and Total Antioxidant Status in the Serum of Patients with Relapsing-Remitting Multiple Sclerosis. Nutr. J. 13 (1), 62–66. doi:10.1186/1475-2891-13-62
Spina, E., and Scordo, M. G. (2002). Clinically Significant Drug Interactions with Antidepressants in the Elderly. Drugs & Aging 19 (4), 299–320. doi:10.2165/00002512-200219040-00004
Stahl, S. M., Pradko, J. F., Haight, B. R., Modell, J. G., Rockett, C. B., and Learned-Coughlin, S. (2004). A Review of the Neuropharmacology of Bupropion, a Dual Norepinephrine and Dopamine Reuptake Inhibitor. Prim. Care Companion J. Clin. Psychiatry 6 (4), 159–166. doi:10.4088/pcc.v06n0403
Stamatovic, S., Dondeti, R., Keep, R., and Andjelkovic, A. (2011). Nicotine Aggravates the Brain Postischemic Inflammatory Response. Am. J. Physiol. Heart Circ. Physiol. 300, H1518–H1529. doi:10.1152/ajpheart.00928.2010
Sweeney, M. D., Zhao, Z., Montagne, A., Nelson, A. R., and Zlokovic, B. V. (2019). Blood-brain Barrier: from Physiology to Disease and Back. Physiol. Rev. 99 (1), 21–78. doi:10.1152/physrev.00050.2017
Takami, K., Saito, H., Okuda, M., Takano, M., and Inui, K. I. (1998). Distinct Characteristics of Transcellular Transport between Nicotine and Tetraethylammonium in LLC-PK1 Cells. J. Pharmacol. Exp. Ther. 286 (2), 676–680.
Tega, Y., Akanuma, S.-i., Kubo, Y., Terasaki, T., and Hosoya, K.-i. (2013). Blood-to-brain Influx Transport of Nicotine at the Rat Blood?brain Barrier: Involvement of a Pyrilamine-Sensitive Organic Cation Transport Process. Neurochem. Int. 62 (2), 173–181. doi:10.1016/j.neuint.2012.11.014
Tega, Y., Yamazaki, Y., Akanuma, S.-i., Kubo, Y., and Hosoya, K.-i. (2018). Impact of Nicotine Transport across the Blood-Brain Barrier: Carrier-Mediated Transport of Nicotine and Interaction with Central Nervous System Drugs. Biol. Pharm. Bull. 41 (9), 1330–1336. doi:10.1248/bpb.b18-00134
Toda, N., and Toda, H. (2010). Nitric Oxide-Mediated Blood Flow Regulation as Affected by Smoking and Nicotine. Eur. J. Pharmacol. 649 (1-3), 1–13. doi:10.1016/j.ejphar.2010.09.042
Toda, R., Kawazu, K., Oyabu, M., Miyazaki, T., and Kiuchi, Y. (2011). Comparison of Drug Permeabilities across the Blood-Retinal Barrier, Blood-Aqueous Humor Barrier, and Blood-Brain Barrier. J. Pharm. Sci. 100 (9), 3904–3911. doi:10.1002/jps.22610
Togna, A. R., Latina, V., Orlando, R., and Togna, G. I. (2008). Cigarette Smoke Inhibits Adenine Nucleotide Hydrolysis by Human Platelets. Platelets 19 (7), 537–542. doi:10.1080/09537100802272626
Trehy, M. L., Ye, W., Hadwiger, M. E., Moore, T. W., Allgire, J. F., Woodruff, J. T., et al. (2011). Analysis of Electronic Cigarette Cartridges, Refill Solutions, and Smoke for Nicotine and Nicotine Related Impurities. J. Liquid Chromatogr. Relat. Tech. 34 (14), 1442–1458. doi:10.1080/10826076.2011.572213
Tsuda, M., Terada, T., Asaka, J.-i., Ueba, M., Katsura, T., and Inui, K.-i. (2007). Oppositely Directed H+ Gradient Functions as a Driving Force of Rat H+/organic Cation Antiporter MATE1. Am. J. Physiology-Renal Physiol. 292 (2), F593–F598. doi:10.1152/ajprenal.00312.2006
Unterberg, A. W., Stover, J., Kress, B., and Kiening, K. L. (2004). Edema and Brain Trauma. Neuroscience 129 (4), 1019–1027. doi:10.1016/j.neuroscience.2004.06.046
Urakami, Y., Okuda, M., Masuda, S., Saito, H., and Inui, K. I. (1998). Functional Characteristics and Membrane Localization of Rat Multispecific Organic Cation Transporters, OCT1 and OCT2, Mediating Tubular Secretion of Cationic Drugs. J. Pharmacol. Exp. Ther. 287 (2), 800–805.
van der Weide, J., Steijns, L. S., and van Weelden, M. J. (2003). The Effect of Smoking and Cytochrome P450 CYP1A2 Genetic Polymorphism on Clozapine Clearance and Dose Requirement. Pharmacogenetics 13 (3), 169–172. doi:10.1097/00008571-200303000-00006
Vannucci, S. J., Maher, F., and Simpson, I. A. (1997). Glucose Transporter Proteins in Brain: Delivery of Glucose to Neurons and Glia. Glia 21 (1), 2–21. doi:10.1002/(sici)1098-1136(199709)21:1<2::aid-glia2>3.0.co;2-c
Varlet, V., Farsalinos, K., Augsburger, M., Thomas, A., and Etter, J.-F. (2015). Toxicity Assessment of Refill Liquids for Electronic Cigarettes. Ijerph 12 (5), 4796–4815. doi:10.3390/ijerph120504796
Verma, S., Wang, C.-H., Li, S.-H., Dumont, A. S., Fedak, P. W. M., Badiwala, M. V., et al. (2002). A Self-Fulfilling Prophecy. Circulation 106 (8), 913–919. doi:10.1161/01.cir.0000029802.88087.5e
Versiani, M., Cassano, G., Perugi, G., Benedetti, A., Mastalli, L., Savino, A., et al. (2002). Reboxetine, a Selective Norepinephrine Reuptake Inhibitor, Is an Effective and Well-Tolerated Treatment for Panic Disorder. J. Clin. Psychiatry 63 (1), 31–37. doi:10.4088/jcp.v63n0107
Virani, S. S., Alonso, A., Aparicio, H. J., Benjamin, E. J., Bittencourt, M. S., Callaway, C. W., et al. (2021). Heart Disease and Stroke Statistics-2021 Update: A Report from the American Heart Association. Circulation 143 (8), e254–e743. doi:10.1161/CIR.0000000000000950
Wang, F., Gerzanich, V., Wells, G. B., Anand, R., Peng, X., Keyser, K., et al. (1996). Assembly of Human Neuronal Nicotinic Receptor α5 Subunits with α3, β2, and β4 Subunits. J. Biol. Chem. 271 (30), 17656–17665. doi:10.1074/jbc.271.30.17656
Wang, L., McComb, J. G., Weiss, M. H., McDonough, A. A., and Zlokovic, B. V. (1994). Nicotine Downregulates α2 Isoform of Na,K-ATPase at the Blood-Brain Barrier and Brain in Rats. Biochem. biophysical Res. Commun. 199 (3), 1422–1427. doi:10.1006/bbrc.1994.1389
Wannamethee, S. G., Lowe, G. D. O., Shaper, A. G., Rumley, A., Lennon, L., and Whincup, P. H. (2005). Associations between Cigarette Smoking, Pipe/cigar Smoking, and Smoking Cessation, and Haemostatic and Inflammatory Markers for Cardiovascular Disease. Eur. Heart J. 26 (17), 1765–1773. doi:10.1093/eurheartj/ehi183
Wheat, L. A., Haberzettl, P., Hellmann, J., Baba, S. P., Bertke, M., Lee, J., et al. (2011). Acrolein Inhalation Prevents Vascular Endothelial Growth Factor-Induced Mobilization of Flk-1 +/Sca-1 + Cells in Mice. Atvb 31 (7), 1598–1606. doi:10.1161/atvbaha.111.227124
Wood, S. M., Wiesener, M. S., Yeates, K. M., Okada, N., Pugh, C. W., Maxwell, P. H., et al. (1998). Selection and Analysis of a Mutant Cell Line Defective in the Hypoxia-Inducible Factor-1 α-Subunit (HIF-1α). J. Biol. Chem. 273 (14), 8360–8368. doi:10.1074/jbc.273.14.8360
Wu, X., George, R. L., Huang, W., Wang, H., Conway, S. J., Leibach, F. H., et al. (2000). Structural and Functional Characteristics and Tissue Distribution Pattern of Rat OCTN1, an Organic Cation Transporter, Cloned from Placenta. Biochim. Biophys. Acta 1466 (1-2), 315–327. doi:10.1016/s0005-2736(00)00189-9
Yeh, W.-L., Lin, C.-J., and Fu, W.-M. (2008). Enhancement of Glucose Transporter Expression of Brain Endothelial Cells by Vascular Endothelial Growth Factor Derived from Glioma Exposed to Hypoxia. Mol. Pharmacol. 73 (1), 170–177. doi:10.1124/mol.107.038851
Yerby, T. R., Vibat, C. R., Sun, D., Payne, J. A., and O'Donnell, M. E. (1997). Molecular Characterization of the Na-K-Cl Cotransporter of Bovine Aortic Endothelial Cells. Am. J. Physiology-Cell Physiol. 273 (1), C188–C197. doi:10.1152/ajpcell.1997.273.1.c188
Zevin, S., and Benowitz, N. L. (1999). Drug Interactions with Tobacco Smoking. Clin. Pharmacokinet. 36 (6), 425–438. doi:10.2165/00003088-199936060-00004
Zhao, Z., Nelson, A. R., Betsholtz, C., and Zlokovic, B. V. (2015). Establishment and Dysfunction of the Blood-Brain Barrier. Cell 163 (5), 1064–1078. doi:10.1016/j.cell.2015.10.067
Zhiyou, C., Yong, Y., Shanquan, S., Jun, Z., Liangguo, H., Ling, Y., et al. (2009). Upregulation of BACE1 and β-Amyloid Protein Mediated by Chronic Cerebral Hypoperfusion Contributes to Cognitive Impairment and Pathogenesis of Alzheimer's Disease. Neurochem. Res. 34 (7), 1226–1235. doi:10.1007/s11064-008-9899-y
Zhu, J., Wang, P., Li, F., Lu, J., Shehu, A. I., Xie, W., et al. (2018). CYP1A1 and 1B1-Mediated Metabolic Pathways of Dolutegravir, an HIV Integrase Inhibitor. Biochem. Pharmacol. 158, 174–184. doi:10.1016/j.bcp.2018.10.012
Zubieta, J.-K., Lombardi, U., Minoshima, S., Guthrie, S., Ni, L., Ohl, L. E., et al. (2001). Regional Cerebral Blood Flow Effects of Nicotine in Overnight Abstinent Smokers. Biol. Psychiatry 49 (11), 906–913. doi:10.1016/s0006-3223(00)01070-2
Nomenclature
AD Alzheimer’s disease
ABC ATP-binding cassette
Aβ amyloid-β
ARTs antiretroviral therapies
BBB blood-brain barrier
CNS central nervous system
DAT dopamine transporter
DMTs disease-modifying treatments
E-cig electronic cigarette
HIF-1 hypoxia-inducible factor-1
MATE 1 multidrug and toxin extrusion protein-1
MPP+ 1-methyl-4-phenylpyridinium
MS multiple sclerosis
nAchRs nicotinic acetylcholine receptors
NET norepinephrine transporter
NKCC Na+-K+-2Cl− co-transporter
OCTs organic cation transporters
OCTn organic cation/carnitine transporters
PD Parkinson’s disease
PMAT plasma monoamine transporters
r-tPA tissue plasminogen activator
PI3K phosphoinositide-3 kinase
PKC protein kinase C
SERT serotonin transporter
SLC solute carrier transporters
TEA tetraethylammonium
TS tobacco smoke/tobacco smoking
TJ tight junction
US-FDA US food and drug administration
Keywords: neurological disorders, smoking, vaping, nicotine, blood-brain barrier, CNS drugs, transporters, ischemic stroke
Citation: Sharma S, Rahman Archie S, Kanchanwala V, Mimun K, Rahman MA, Zhang Y and Abbruscato T (2022) Effects of Nicotine Exposure From Tobacco Products and Electronic Cigarettes on the Pathogenesis of Neurological Diseases: Impact on CNS Drug Delivery. Front. Drug. Deliv. 2:886099. doi: 10.3389/fddev.2022.886099
Received: 28 February 2022; Accepted: 22 March 2022;
Published: 26 April 2022.
Edited by:
Patrick T. Ronaldson, University of Arizona, United StatesReviewed by:
Nicolas Tournier, Commissariat à l'Energie Atomique et aux Energies Alternatives (CEA), FranceIbolya Andras, University of Miami, United States
Copyright © 2022 Sharma, Rahman Archie, Kanchanwala, Mimun, Rahman, Zhang and Abbruscato. This is an open-access article distributed under the terms of the Creative Commons Attribution License (CC BY). The use, distribution or reproduction in other forums is permitted, provided the original author(s) and the copyright owner(s) are credited and that the original publication in this journal is cited, in accordance with accepted academic practice. No use, distribution or reproduction is permitted which does not comply with these terms.
*Correspondence: Thomas Abbruscato, dGhvbWFzLmFiYnJ1c2NhdG9AdHR1aHNjLmVkdQ==