- Aerogen Pharma Corporation, San Mateo, CA, United States
The last half century of pulmonary product development is reviewed in the context of the main drivers of innovation, technology development, and the advancement of science. A perspective on development timeframes, patent lifetimes, and the odds of success of developing of new inhaler technologies is presented.
Introduction
Inhalation therapy has been documented in various forms for over 2000 years and has likely been practiced in some form for much longer than that (Stein and Theil, 2017). However, the modern era of inhalation therapy corresponds, not surprisingly, with the rise of modern pharmacology, a deeper understanding of the biology underlying respiratory diseases, and an elucidation of airway structure, aerosol transport, and deposition (Clark, 1995).
During the early part of the 19th century significant advances in the discovery of drugs to treat asthma (Andersen et al., 2022) (beta agonists, corticosteroids, and anticholinergics) were setting the stage for a transition from early nebulizer devices to more advanced delivery systems. In 1956, 3M invented the now ubiquitous pressurized metered dose inhaler (pMDI) (Thiel, 1996); and in the late 1960s, Fisons Pharmaceuticals invented lactose carrier blends and the Spinhaler (Bell et al., 1971), which became the precursor to the modern dry powder inhaler (DPI). The basic delivery technologies that have evolved over the past half century can best be described as small personal portable aerosol generators, and they can be divided into four major classes utilizing three physical “forms” of drugs. The various technologies have different operating characteristics, patient interface/user requirements, and dose limitations (Figure 1).
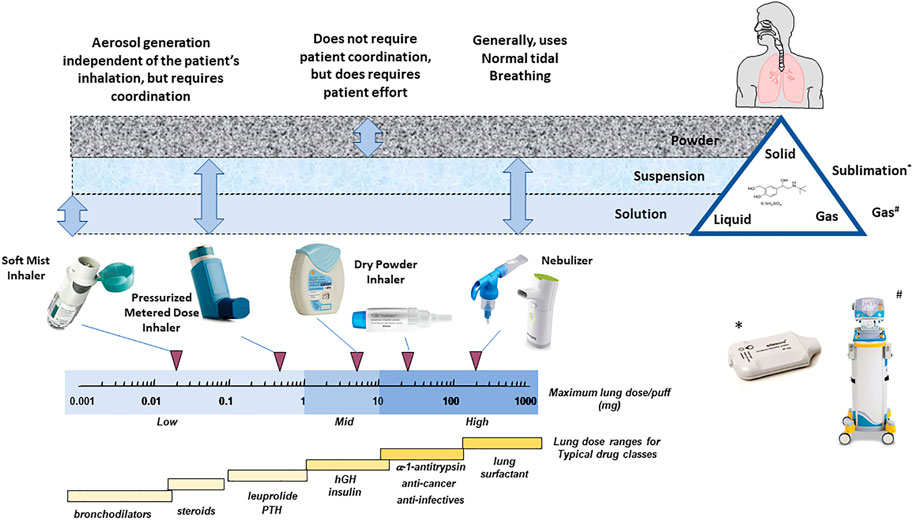
FIGURE 1. The “universe” of inhaler technology illustrating patient technique requirements, primary drug “form,” inhaler type, maximum achievable dose with each inhaler type, and the typical lung doses required for the major inhaled drug classes (adapted from Weers et al., 2004).
Amid steady progress in inhaler development, in the mid-1990s, chlorofluorocarbon (CFC) propellants were banned due to their ozone depletion potential (Federal Register, 1994). The realization that pMDIs, then as now the mainstay of inhalation therapy, might no longer be available, sparked enormous efforts to find alternatives, as exemplified in patent filings of that period. Between 1990 and 2010, nearly 1,000 patents describing inhalation technologies were filed, compared to less than 100 in the previous two decades (Stein and Theil, 2017). However, this drive for new technologies was short lived as alternative propellants were rapidly developed and the pMDI remains the mainstay of inhalation therapy to this day. Of the many attempts to use novel methods of generating aerosols for inhalation products, most failed. Electrostatic atomizers (Zimilich et al., 2000) failed primarily due to formulation limitations because they had to contain excipients to control conductivity that were incompatible with delivery to the airways. Active DPI, which used compressed air (Patton, 2005), mechanical impact (Nelson et al., 1999), or ultrasound (Fleming et al., 2012) to disperse drug powders, failed either due to a poor choice of development candidate and poor business partner or because the technology was too expensive or too hard to make work in a clinical environment. Several complex pMDI breath actuation devices also failed, again due to a poor choice of molecule development candidate or partner (Armer et al., 2007) or because they were simply too expensive or complex for the market (Farr S J et al., 1995). Several liquid atomizer technologies failed for a variety of reasons from financial to market forces (Farr S J et al., 2004). However, it is noteworthy that mesh nebulizers came of age (Lipworth et al., 2005) and since developed the capability to deliver 2 μm of aerosol at acceptable rates (Sweeny et al., 2019). During this period the Respimat, invented in the late 1980s, beat the odds and is now marketed with several drug combinations for the treatment of asthma and chronic obstructive pulmonary disease (COPD) (Dalby R et al., 2004). The Staccato inhaler, which sublimes loxapine to produce an inhaled aerosol, was also approved in 2012 (Dinh et al., 2011).
In the absence of worries of existing technologies being withdrawn from the market, one of the major factors controlling new technology adoption is whether it is enabling. In a conservative industry, the general trend is to stick with what is known rather than adopt additional technology risk, particularly if the development involves a new chemical entity (NCE) with a concomitant molecule risk. As a result, most of the hopeful technologies fell by the wayside. Of more than 25 new protype inhaler devices reported in the literature in the late 1990s, few more than five have survived and continued in development; even fewer have made it to market.
The evolution of delivery devices and therapeutic molecules has primarily been targeted at facilitating increased levels of patient adherence and compliance. From a molecule perspective, treatments have evolved from short durations of action to longer and longer durations of action (Andersen et al., 2022), reducing dosing requirement from multiple events per day to once a day. For example, the bronchodilators salbutamol, salmeterol, and vilanterol have moved from dosing four times a day, to twice a day, to once a day. Although single-dose devices utilizing single drugs and unit dose capsules are still being used (Lipp et al., 2002; Garcia et al., 2012; Yin et al., 2017), they have been largely replaced with multidose devices. Arguably these are easier to use, require use only once daily, can deliver up to three active molecules in a single dose (usually a B2 agonist, an antimuscarinic, and a steroid) and require replacement only once a month. Figure 2 presents a development sequence for NCEs and devices developed by GlaxoSmithKline. It should be noted that this sequence of development took over 23 years and one of the devices, the Diskhaler, was never approved with salbutamol in the United States.
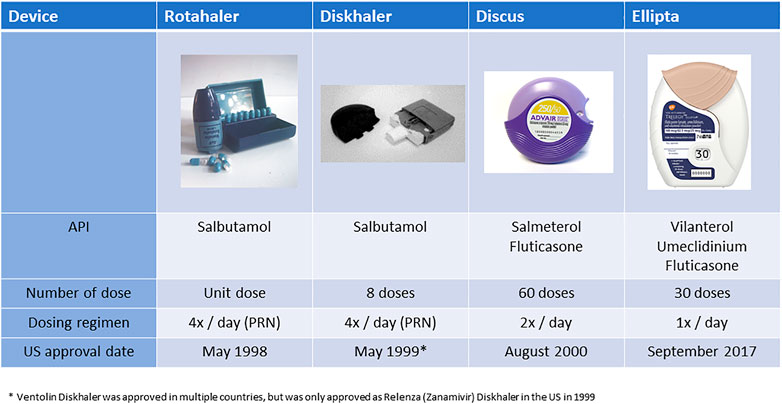
FIGURE 2. Illustration of the concomitant development of longer acting drugs and more convenient devices (Note. The device platforms have generally been used for more than one drug. The data presented have been limited to a single drug sequence for illustrative purposes).
However, despite these great advances, adherence and compliance remain major hurdles to effective therapy (Bourbeau and Bartlett, 2008; Restrepo et al., 2008; Vestbo et al., 2009). Figure 3 presents typical compliance curves for various drug delivery modalities and diseases. Compliance for respiratory products, in particular corticosteroids and bronchodilators in this example, is poor and shows a decline of more than 70% over the first 3 months of treatment. That said, even oncology, diabetes, and cardiovascular drugs show a decline of 50% over 6 months. This indicates that, in general, patients exhibit poor compliance regardless of the route of delivery or the severity of disease, but also that despite major improvements in inhaler design and inhaled drugs, respiratory therapy remains challenging (Newman, 2014).
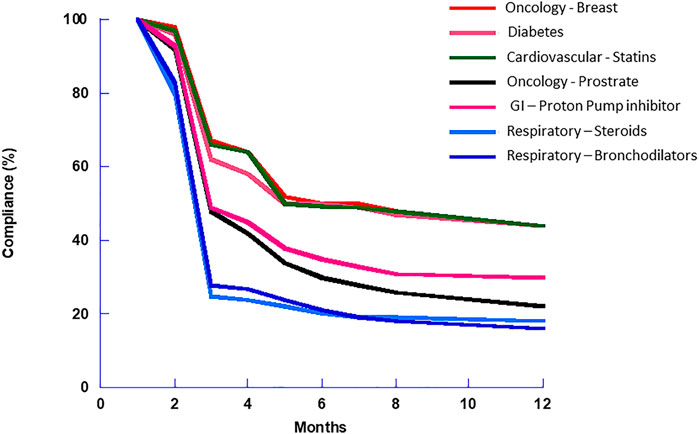
FIGURE 3. Adherence and compliance for various drug dosage forms. Of note is the 3-months fall-off in compliance apparent with all drugs, but it appears to be more dramatic with inhalers than the other dosage forms (Schroeder et al., 2004; Vermeire et al., 2005; Dew et al., 2007).
Recent Trends and Developments
With a backdrop of reduced dosing frequency, and inhalers that require fewer steps to operate and less frequent replacement, recent technological developments have been targeted at mitigating the impact of non-adherence to ensure the delivery of an adequate dose over a broader range of operating characteristics with products that are less reliant on patient technique. These developments have mainly focused on manufacturing and formulation technologies, so called “particle engineering”, to improve lung delivery efficiency, and thereby directly reduce inter- and intrasubject variability (Borgstrom et al., 2006). Figure 4 (data from Weers and Clark, 2017) describes one of the major problems encountered when developing inhaled products. While the lower airway dimensions of humans are reasonably consistent, intersubject variations in oropharyngeal dimensions are large (Grgic et al., 2004). This variation in geometry coupled to the typical performance range (particle size and inhaled flow rate, d2Q) of most commercial inhaler products is at the heart of a lot of the variability seen clinically (Weers et al., 2019). This has resulted in developments in technology, particularly in dry powder inhalers (DPIs), that produce more ideal aerosol particle size and function at lower inhaled flow rates. Reducing d2Q is paramount to making further reductions in intersubject variability (Weers et al., 2013).
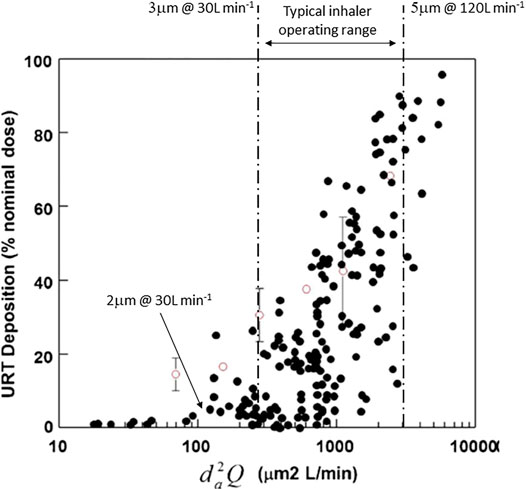
FIGURE 4. The effect of aerosol size and inhaled flow rate (d2Q) on interpatient variability in oropharyngeal deposition of inhaled aerosols. A typical range of aerosol size and inhaled flow rate is illustrated as is a size and flow rate that would minimize oropharyngeal losses and variability (adapted from Weers and Clark, 2017).
Although the conservative nature of drug development dictates that adoption of new pulmonary technologies is slow and fickle, great progress has ultimately been made in making some of these technologies commercially available. Table 1 presents four particle engineering technologies, their application, and the names of commercial products that utilize them. It should however be understood that progression from concept to product approval takes many years, which has consequences for development cost and the remaining patent life when a product finally makes it to market. For example, the original provisional patent for Pulmospheres™ was filed in 1997, the proof-of-concept study for TobiPodhaler was performed in 2000 (Duddu et al., 2002), and the product was approved for use in cystic fibrosis patients by the FDA in March 2013, some 16 years after the initial work and with only 4 years of original patent life remaining. Thus, development time and patent life influences the choice and adoption of new technologies for generic molecules that are being re-tasked for new indications or the inhaled route of delivery as well as for NCEs.
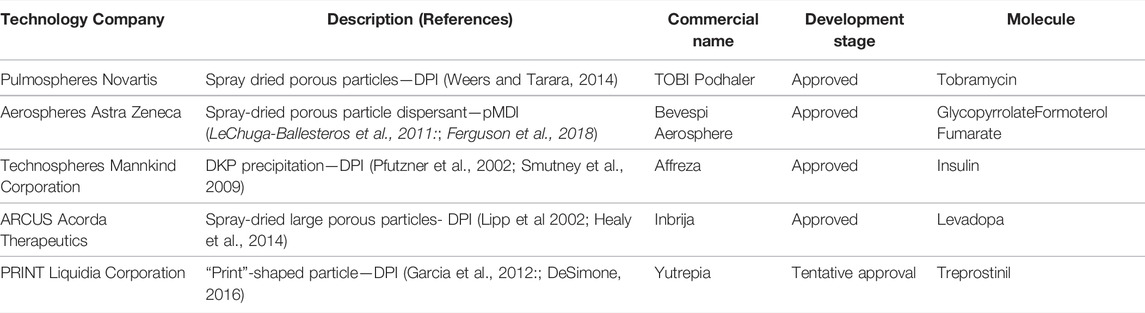
TABLE 1. Particle engineering coming of age—Approved pulmonary products containing engineered powders.
The Fundamentals of Inhalation Delivery
Alongside new developments in technology, there has been an accompanying improvement in the understanding of airway deposition mechanisms and the technical requirements for the design and development of inhalers.
The basic requirements for the delivery of drugs to the lung have been known for many years (Clark, 1995) and are based on the original work of the industrial hygiene community where aerosol deposition was studied in the context of occupational exposure during normal tidal breathing and exercise (Heyder et al., 1986). Generally, the window of opportunity for an inhaled aerosol to reach the lung is from about 0.5 to 5 µm in diameter–too big and the aerosol impacts in the mouth and oropharynx; too small and there is insufficient time for sedimentation in the peripheral airways and the aerosol particles are exhaled. However, these concepts were developed based on tidal breathing and they are not directly applicable to all inhalation modalities. For example, with conventional DPIs, the instruction is to inhale forcefully, which generates inhaled flow rates that are much higher than in normal tidal breathing. This results in a higher probability of mouth and oropharyngeal deposition and a requirement for a “finer” aerosol if oropharyngeal deposition is to be avoided. Many inhaler directions for use also instruct breath holds following inhalation, which gives a longer residence time in the airways and a greater chance for smaller particles to sediment and deposit. In fact, only with nebulizer delivery using tidal breathing is exhaled drug really an issue. Thus, while particle size range is a reasonable general guide, the optimum size range for efficient and consistent delivery varies markedly with inhaler and formulation design. This was exemplified by the modeling work of Egan and Clark (Egan and Clark, 1994) demonstrating the effects of high inhalation flow rate and breath holds compared to normal tidal breathing (Figure 5). Figure 3 also exemplifies the main challenge: inhalers must generate a sufficiently fine aerosol and deliver it at a sufficiently low rate to avoid significant losses in the mouth and oropharynx and deliver an effective dose to the lung (i.e., a low d2Q). They obviously must also be relatively inexpensive.
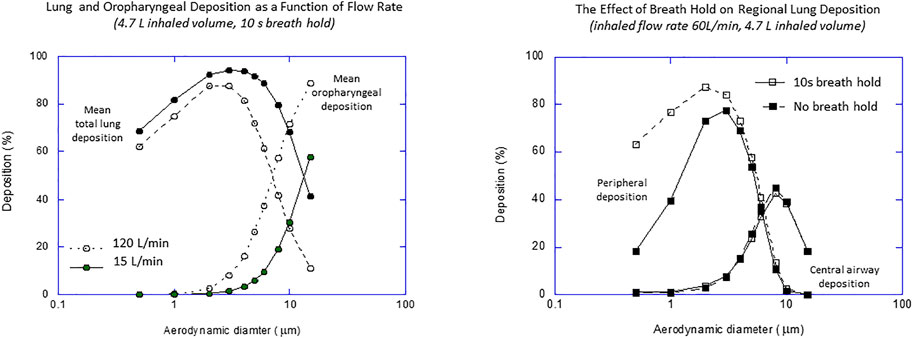
FIGURE 5. The effect of inhaled flow rate and breath hold on regional deposition from a dry powder inhaler (data from Egan and Clark, 1994).
The performance of a range of inhaler products (DPIs, pMDIs, pMDIs with spacer and holding chambers, and soft mist inhalers (SMIs)) under ideal laboratory conditions is presented in Figure 6 (Clark, 2012). This figure summarizes two decades of research into lung deposition and regional distribution from inhaled products using gamma scintigraphy. The y-axis is total lung deposition as a percentage of the inhaled dose. The lower x-axis is the peripheral-to-central ratio, which is a measure of how much drug reaches the peripheral air spaces. The upper x-axis is the percentage of the inhaled dose that remains in the lung after 24 h, which is a measure of the dose reaching the non-ciliated, alveolated airways that are not cleared by mucocillary clearance. The solid line represents a theoretical relationship between lung dose and regional deposition for various aerosols with mass median aerodynamic diameters of between 2 and 5 μm, geometric standard deviation of 2.0, and an inhaled dose of 60 L/min. First, it can be seen from the data that the majority of pMDIs and DPIs produce from 5 to 40% lung deposition with between 45 and 60 % of that dose reaching the peripheral airways. Most of the data points are well below the theoretical line indicating that peripheral deposition is higher than would be expected for a given lung deposition if the delivered aerosols were monomodal and/or were not dynamic in nature. As explained by Theil (Theil, 1998), this discrepancy is actually due to the ballistic nature of pMDI sprays and the bimodal nature of DPIs which usually generate a course mode that is large and causes substantial deposition in the mouth and oropharynx and a fine mode that penetrates to airways reasonably well. It is of note that pMDIs with spacers or chambers are closer to the theoretical line. Spacers and holding chambers remove the ballistic component of a pMDI spray prior to reaching the patient’s mouth. Also the DPI data point from Duddu et al. (2002) is noteworthy. This was generated from a Pulmospheres™ formulation (Weers and Tarara, 2014) that, because of its easily dispersed nature, delivered single-mode aerosol distribution in the region of 3–4 μm MMAD. This exemplifies the advantages of particle engineering technologies where interparticle forces can be controlled and manipulated to produce aerosols from DPIs that are relatively fine and delivered at relatively low flow rates (i.e., possessing low d2Q).
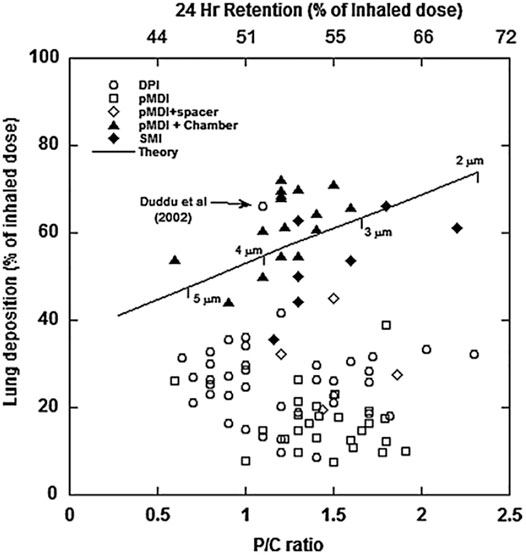
FIGURE 6. Summary of regional deposition from pharmaceutical inhalers (from Clark, 2012).
Understanding Dry Powder Inhaler Performance
With the advent of particle engineering and the growing use of DPIs, more attention is being paid to patient interactions with DPIs and whether they can inhale vigorously enough to be able to use a DPI effectively. The obvious advantage of a DPI is that it “automatically” coordinates drug delivery with inhalation because it is the inhalation that provides the energy to extract and disperse the inhaled dose. However, it is incumbent on the patient to inhale at a sufficiently high pressure drop/flow rate to ensure the dose is adequately delivered. Despite half a century of DPI use, this is a topic that has recently garnered much attention as the clinical community tries to understand the cause and impact of non-adherence and compliance on clinical outcomes (Bourbeau & Bartlett, 2008).
The basic relationship between DPI resistance and inspiratory pressure was defined by Clark (Clark & Hollingworth, 1993). The flow rate through a DPI is proportional to the square root of the pressure developed across it. The constant of proportionality is defined as the inhaler resistance. The resistance of DPIs varies depending upon their design and ranges from approximately 0.01 kPa ½ L/min to 0.06 kPa ½ L/min. The negative pressures that patients can produce at the mouthpiece of a DPI generally depends on age and gender and ranges from 12 kPa in adult men to 4 or 5 kPa in children and the elderly (Clark et al., 2020). Airway resistance, even with the presence of respiratory disease, is much lower than DPI resistances, and airway disease plays only a secondary part in inhaler use through possibly generating weakness in the respiratory muscles (Clark, 2015; Sahay et al., 2021).
The performance of DPIs depends on the design of the formulation as well as the inhaler itself, and so the clinical community’s current efforts to define a single threshold flow rate for all DPIs is inappropriate and dangerously misleading (Clark et al., 2020). Since the flow rates at which products deliver adequate lung doses are dependent upon the inhaler resistance and the pressure drop a patient can produce, a more appropriate metric is pressure, not inhaled flow rate (Clark, 2015).
Electronic Inhalers
In the continued pursuit of improved adherence and compliance, which even with newer inhaler technologies is far from optimal, modern electronics are beginning to playing their part. What started as monitoring dosing in clinical trials has evolved into smart inhalers that can help patients use correct inhaler techniques and allow clinicians to monitor dosing compliance. Mehta (2015) lists seven electronic monitoring devices for DPIs, and a similar number of monitoring device for pMDIs have been reported in the literature. Prichard (Pritchard et al., 2015) reviewed monitoring technologies across all inhaler modalities in the context of promoting adherence and compliance. However, to date there are few publications detailing actual improvement in clinical outcomes resulting from adherence monitoring, with most publications detailing the accuracy of monitoring devices and such things as the correspondence between patient diary cards and adherence measured by the electronics. Pinnock (2017) concluded that this “technology” is not a panacea in the context of asthma self-management, but self-management options might be improved by moving beyond performing specific functions such as patient reminders and counting doses. It remains to be seen if this approach will have a real and sustained impact on clinical outcomes.
The Respiratory Marketplace
As previously mentioned, issues with CFC propellants and global warming brought a renewed focus on other forms of inhaled drug delivery. Although many technologies were tried, the performance and cost constraints associated with small portable aerosol generators capable of delivering inhaled drugs have resulted in very few new technologies standing the test of time. Except for the smart mist inhaler, the mainstay of inhalation therapy remains the basic triad of pMDI, DPI, and nebulizer. Indeed, it was expected that DPIs would displace the pMDI and, in sales revenue terms, they have. However, the pMDI is still the most popular inhalation device by number of doses and units sold by a factor of about three (Fradley, 2006). While this is largely driven by low-cost generics, it is also bolstered by new binary and triple combination products in asthma and COPD (Self and Ellingson, 2017).
In 2018 there were 2,387 innovator drug products and 7,852 generic products approved for sale in the United States. Of these, 62% were oral dosage forms and only 1.2% (127) were pulmonary products (Zhong et al., 2018). This illustrates several points. First, and perhaps not surprisingly, oral products are the preferred route for drug delivery. People are used to ingesting food and supplements and adding tablets or capsules to the “diet” would seem straightforward. Tablets are convenient, if you remember to take them. In contrast, inhalation is one of the least popular routes of delivery and one might speculate that most patients find this route unfamiliar. It is also more technically demanding than swallowing a tablet—despite the great advances that have been made in pulmonary product design in recent years, specific inhaler techniques are still necessary to ensure effective dosing. A second observation is of the generic-to-innovator product ratio, which is 4.7 for oral dosage forms and only 0.9 for pulmonary products. While this is likely driven by the unattractiveness of some of the market opportunities, it is interesting to speculate that in large part, it is driven by the technical requirements for inhaled products and the need for specialist knowledge to be able to meet the FDA’s expectations when demonstrating equivalence. This is exemplified by the long development times and technical struggles to get the first generic salbutamol pMDI approved (Adams et al., 1994) and more recently with similar struggles to get a generic version of Advair approved (Donohue et al., 2021). An Advair J substitutable generic product represents a huge market opportunity, but it also presented huge technical challenges that have resulted in some high-profile development casualties.
Summary
Much progress has been made over the last half century, both in understanding the underlying rules of inhaler/formulation design and in producing better inhaled products. Initial developments were triggered by the emergence of new molecules that were effective in treating pulmonary disease. This was followed by issues with propellants and their ozone depletion potential. This drove attempts to mitigate the potential impact of the removal of pMDIs from the clinical arsenal with new device innovation, but most of the new device technologies that resulted fell by the wayside as new propellants were developed. During this time frame, steady progress was made in the discovery of longer acting molecules, and multidose DPIs were developed, all of which enhanced patient convenience with the inference that it could improve adherence and compliance. In recent years the focus has switched from inhaler design to formulation design. For many decades lactose blends and spheronized particles ruled supreme; in the last two decades, multiple particle engineering technologies have been developed and have made it to market. These range from spray drying to production of large or small porous particles (Lipp et al., 2002; Weers et al., 2007; LeChuga-Ballesteros et al., 2011) to PRINTing particles with defined shapes (Garcia et al., 2012), to controlled precipitation of novel excipients (Pfutzner et al., 2002). While these technologies have only recently made it through to approval and have had an impact on a relatively small number of products, they hold great potential for improving pulmonary products and reducing the patient burden of having to learn specific inhaler techniques.
Finally, from a marketed product perspective, pulmonary products are still relatively niche. They obviously offer direct access to the lung and most of the products that have been developed treat lung diseases. However, there are several products that have taken advantage of the lung as a portal of entry into the systemic circulation (Exubra, Patton, 2006; Inbrija, Lipp et al., 2002). The lung epithelium is thin and most small molecules are absorbed at a rate that is comparable to IV administration, provided they are relatively soluble and not dissolution-limited (Hasdelt et al., 2016). It remains to be seen if other technologies will emerge in future years, but either way it should be remembered that the development of any new pharmaceutical technology is a long and arduous task, and this is particularly so for pulmonary delivery technologies.
Author Contributions
The author confirms being the sole contributor of this work and has approved it for publication.
Conflict of Interest
AC is employed by Aerogen Pharma Corporation.
Publisher’s Note
All claims expressed in this article are solely those of the authors and do not necessarily represent those of their affiliated organizations, or those of the publisher, the editors, and the reviewers. Any product that may be evaluated in this article, or claim that may be made by its manufacturer, is not guaranteed or endorsed by the publisher.
References
Adams, W. P., Poochikian, G., Taylor, A. S., Patel, R. M., Burke, G. P., and Williams, R. L. (1994). Regulatory Aspects of Modification to Innovator Bronchodilator Metered Dose Inhalers and Development of Generic Substitutes. J. Aerosol Med. 7, 119–134. doi:10.1089/jam.1994.7.119
Andersen, S., Atkins, P., Backman, P., Cipolla, D., Clark, A., Daviskas, E., et al. (2022). Inhaled Medicines: Past, Present and Future. Pharmacol. Rev. 74, 1–71. doi:10.1124/pharmrev.120.000198
Armer, T. A., Shrewsbury, S. B., Newman, S. P., Pitcairn, G., and Ramadan, N. (2007). Aerosol Delivery of Ergotamine Tartrate via a Breath-Synchronized Plume-Control Inhaler in Humans. Curr. Med. Res. Opin. 23, 3177–3187. doi:10.1185/030079907x242881
Bell, J. H., Hartley, P. S., and Cox, J. S. G. (1971). Dry Powder Aerosols I: A New Powder Inhalation Device. J. Pharm. Sci. 60 (10), 1559–1564. doi:10.1002/jps.2600601028
Borgström, L., Olsson, B., and Thorsson, L. (2006). Degree of Throat Deposition Can Explain the Variability in Lung Deposition of Inhaled Drugs. J. Aerosol Med. 19, 473–483. doi:10.1089/jam.2006.19.473
Bourbeau, J., and Bartlett, S. J. (2008). Patient Adherence in COPD. Thorax 63, 831–838. doi:10.1136/thx.2007.086041
Clark, A. R. (2012). Understanding Penetration index Measurements and Regional Lung Targeting. J. Aerosol Med. Pulm. Drug Deliv. 25, 179–187. doi:10.1089/jamp.2011.0899
Clark, A. R., and Hollingworth, A. M. (1993). The Relationship between Powder Inhaler Resistance and Peak Inspiratory Conditions in Healthy Volunteers - Implications for In Vitro Testing. J. Aerosol Med. 6 (2), 99–110. doi:10.1089/jam.1993.6.99
Clark, A. R. (1995). Medical Aerosol Inhalers: Past, Present, and Future. Aerosol Sci. Techn. 22, 374–391. doi:10.1080/02786829408959755
Clark, A. R., Weers, J. G., and Dhand, R. (2020). The Confusing World of Dry Powder Inhalers: It Is All about Inspiratory Pressures, Not Inspiratory Flow Rates. J. Aerosol Med. Pulm. Drug Deliv. 33 (1), 1–11. doi:10.1089/jamp.2019.1556
Clark, A. (2015). The Role of Inspiratory Pressures in Determining the Flow Rates Though Dry Powder Inhalers; A Review. Cpd 2127, 3974–3983. doi:10.2174/1381612821666150820105800
Dalby, R., Spallek, M., and Voshaar, T. (2004). A Review of the Development of Respimat Soft Mist Inhaler. Int. J. Pharmaceutics 283, 1–9. doi:10.1016/j.ijpharm.2004.06.018
DeSimone, J. M. (2016) Co-opting Moore's Law: Therapeutics, Vaccines and Interfacially Active Particles Manufactured via PRINT. J. Controlled Release, 240, 541–543. doi:10.1016/j.jconrel.2016.07.019
Dew, M. A., DiMartini, A. F., De Vito Dabb, A., Myaskovsky, L., Steel, J., Unruh, M., et al. (2007). Rates and Risk Factors for Nonadherence to the Medical Regimen after Adult Solid Organ Transplantation. Transplantation 83, 858–873. doi:10.1097/01.tp.0000258599.65257.a6
Dinh, K., Myers, D. J., Glazer, M., Shmidt, T., Devereaux, C., SimisNoymer, K. P. D., et al. (2011). In Vitro aerosol Characterization of Staccato(®) Loxapine. Int. J. Pharm. 403 (1-2), 101–108. doi:10.1016/j.ijpharm.2010.10.030
Donohue, J. F., Burgoyne, D. S., Ward, J. K., Allan, R., Koltun, A., and Cooper, A. (2021). Wixela Inhub: A Generic Equivalent Treatment Option for Patients with Asthma or COPD. Pulm. Ther. 7, 47–57. doi:10.1007/s41030-020-00142-5
Duddu, S. P., Walter, Y. H., Tarara, T. E., Trimble, K. R., Clark, A. R., and Eldon, M. A. (2002). Improved Lung Delivery from a Passive Dry Powder Inhaler Using an Engineered PulmoSphere® Powder. Pharm. Res. 19, 689–695. doi:10.1023/A:101532261661
Egan, M., and Clark, A. R. (1994). Modelling the Deposition of Inhaled Powdered Drug Aerosols. J. Aerosol Sci. 25 (1), 175–186. doi:10.1016/0021-8502(94)90189-9
Farr, S. J., McElduff, A., Mather, L. E., Okikawa, J., Ward, M. E., Gonda, I., et al. (2004). Diabetes Tech. Thera 2, 2. doi:10.1089/jamp.2018.1508
Farr, S. J., Rowe, A. M., Rubsamen, R., and Taylor, G. (1995). Aerosol Deposition in the Human Lung Following Administration from a Microprocessor Controlled Pressurised Metered Dose Inhaler. Thorax 50, 639–644. doi:10.1136/thx.50.6.639
Federal Register (1994). Montreal Protocol on Substances that Deplete the Ozone Layer, Final Act (Nairobi: UNEP 1987) 59 FR, 56276–56298.
Ferguson, G. T., Hickey, A. J., and Dwivedi, S. (2018). Co-suspension Delivery Technology in Pressurized Metered-Dose Inhalers for Multi-Drug Dosing in the Treatment of Respiratory Diseases. Respir. Med. 134, 16–23. doi:10.1016/j.rmed.2017.09.012
Fleming, F., Akouka, H., Corcoran, T. E., Geller, D. E., Oakum, C. D., Watts, A. B., et al. (2012). Development and Optimization of a Novel Pulsed Dry Powder Nebulizer Using In Vitro Studies that Mimic In Vivo Conditions. Respir. Drug Deliv. 1, 377–38635.
Fradley, G. (2006). Living in Harmony – pMDIs & DPIs in the 21st century. Drug Deliv. Techn. 6 (7), 32–35.
Garcia, A., Mack, P., Williams, S., Fromen, C., Shen, T., Tully, J., et al. (2012). Microfabricated Engineered Particle Systems for Respiratory Drug Delivery and Other Pharmaceutical Applications. J. Drug Deliv. 2012, 941243. doi:10.1155/2012/941243
Grgic, B., Finlay, W. H., Burnell, P. K. P., and Heenan, A. F. (2004). In Vitro intersubject and Intrasubject Deposition Measurements in Realistic Mouth-Throat Geometries. J. Aerosol Sci. 35, 1025–1040. doi:10.1016/j.jaerosci.2004.03.003
Hasdelt, J. E., Backman, P., Clark, A. R., Doub, W., Hickey, A., Hochaus, G., et al. (2016). Scope and Relevance of a Pulmonary Biopharmaceutical Classification System AAPS/FDA/USP Workshop March 16-17th 2015 in Baltimore, MC. AAPS Open 2, 1.
Healy, A. M., Amaro, M., Paluch, K. J., and Tajber, L. (2014). Dry Powders for Oral Inhalation Free of Lactose Carrier Particles. Adv. Drug Deliv. Rev. 75, 32–52. doi:10.1016/j.addr.2014.04.005
Heyder, J., Gebhart, J., Rudolf, G., Schiller, C. F., and Stahlhofen, W. (1986). Deposition of Particles in the Human Respiratory Tract in the Size Range 0.005-15 μm. J. Aerosol Sci. 17 (5), 811–825. doi:10.1016/0021-8502(86)90035-2
Lechuga-Ballesteros, D., Noga, B., Vehring, R., Cummings, R. H., and Dwivedi, S. K. (2011). Novel Cosuspension Metered-Dose Inhalers for the Combination Therapy of Chronic Obstructive Pulmonary Disease and Asthma. Future Med. Chem. 3 (13), 1703–1718. doi:10.4155/fmc.11.133
Lipp, M., Hickey, A., Langer, R., and LeWitt, P. A. (2002). A Technology Evualtion of CVT-301 (Inbrija): an Inhalable Therapy Fro Treatment of Parkinson’s Disease. Expert Opin. Drug Deliv. 18 (11), 1159–1569. doi:10.1080/17425247.2021.1960820
Lipworth, B. J., Sims, E. J., Taylor, K., Cockburn, W., and Fishman, R. (2005). Dose-response to Salbutamol via a Novel palm Sized Nebuliser (AerodoseR Inhaler), Conventional Nebuliser (Pari LC Plus) and Metered Dose Inhaler (Ventolintm Evohalertm) in Moderate to Severe Asthmatics. Br. J. Clin. Pharmacol. 59, 5–13. doi:10.1111/j.1365-2125.2005.02168.x
Mehta, P. P. (2020). Dry Powder inhalers: A Concise Summary of the Electronic Monitoring Devices. Ther. Deliv. 12 (1), 6–232.
Nelson, H., Kemp, J., Bieler, S., Vaughan, L., and Hill, H. R. (1999). Comparative Efficacy and Safety of Albuterol Sulfate Spiros Inhaler and Albuterol Metered-Dose Inhaler in Asthma. Chest 115 (2), 329–335. doi:10.1378/chest.115.2.329
Newman, S. (2014). Improving Inhaler Technique, Adherence to Therapy and the Precision of Dosing: Major Challenges for Pulmonary Drug Delivery. Expert Opin. Drug Deliv. 11 (3), 365–378. doi:10.1517/17425247.2014.873402
Patton, J. S. (2006). Pulmonary Delivery of Insulin. Curr. Med. Res. Opin. 22 (Suppl 3), S5–S11. doi:10.1185/030079906X132695
Patton, J. S. (2005). Unlocking the Opportunity of Tight Glycemic Control: Innovative Delivery of Insulin via the Lung. Diabetes Obes. Metab. 7 (Suppl 1), S5–S8. doi:10.1111/j.1463-1326.2005.00530.x
Pfutzner, A., Mann, A., and Steiner, S. (2002). Technosphere/Insulin − A New Approach for Effective Delivery of Human Insulin via the Pulmonary Route. Diabetes Technol. Ther. 4 (5), 589–594. doi:10.1089/152091502320798204
Pinnock, H. (2017). Connecting Professionals and Patients: How Technology Can Support Asthma Self-Management. Respiratory Drug Delivery Europe 2017. Editors R. N. Dalby, J. Peart, J. D. Suman, P. M. Young, and D. Traini (Nice, France: Davis Healthcare International Publishing), 43–52.
Pritchard, J. N., and Nicholls, C. (2015). Emerging Technologies for Electronic Monitoring of Adherence, Inhaler Competence, and True Adherence. J. Aerosol. Med. Pulm. Drug Deliv. 28 (2), 69–81. doi:10.1089/jamp.2014.1163
Restrepo, R. D., Alvarez, M. T., Wittenebel, L. D., Sorenson, H., Wettstein, R., Sikkema-Ortiz, J., et al. (2008). Medication Adherence Issues in Patients Treated for COPD. Int. J. Chron. Obstruct. Pulmon. Dis. 3 (3), 371–384. doi:10.2147/copd.s3036
Sahay, S., Holy, R., Lyons, S., Parsley, E., Maurer, M., and Weers, J. (2021). Impact of Human Behavior on Inspiratory Flow Profiles in Patients with Pulmonary Hypertension Using the AOS™ Dry Powder Inhaler Device. Pulm Circ. 11 (1), 2045894020985345. doi:10.1177/2045894020985345
Schroeder, K., Fahey, T., and Ebrahim, S. (2004). Interventions for Improving Adherence to Treatment in Patients with High Blood Pressure in Ambulatory Settings. Cochrane Database Syst. Rev. (2), CD004804. doi:10.1002/14651858.CD004804
Self, T., and Ellingson, S. (2017). New Treatment Option for Chronic Obstructive Pulmonary Disease: Two Long-Acting Bronchodilators in a Single Metered-Dose Inhaler. Am. J. Med. 130 (11), 1251–1254. doi:10.1016/j.amjmed.2017.07.011
Smutney, C., Friedman, E. M., Polidoro, J. M., and Amin, N. (2009). Inspiratory Efforts Achieved in Use of the Technosphere™ Insulin Inhalation System. J. Diabetes Sci. Technol. 3 (5), 1175–1182. doi:10.1177/193229680900300524
Stein, S., and Thiel, C. (2017). The History of Therapeutic Aerosols: A Chronological Review. J. Aerosol. Med. Pulm. Drug Deliv. 30 (1), 20–41. doi:10.1089/jamp.2016.1297
Sweeny, L., McClosley, A. P., Higgins, G., Ramsey, J. M., Cryan, S.-A., and MacLoughlin, R. (2019). Effective Nebulization of Interferon- A Using a Novel Vibrating Mesh. Respir. Res. 20 (1), 60. doi:10.1186/s12931-019-1030-1
Theil, C. (1998). Can In Vitro Particle Size Measurements by Used to Predict Pulmonary Deposition of Aerosol from Inhalers. J. Aerosol. Med. 11 (Suppl 1), S43–S52.
Thiel, C. G. (1996). From Susie’s Question to CFC-Free: An Inventor’s Perspective on Forty Years of MDI Development and Regulation. Respir. Drug Deliv. 1, 115–123.
Vermeire, E., Wens, J., Van Royen, P., Biot, Y., Hearnshaw, H., and Lindenmeyer, A. (2005). Interventions for Improving Adherence to Treatment Recommendations in People with Type 2 Diabetes Mellitus. Cochrane Database Syst. Rev. (2), CD003638. doi:10.1002/14651858.CD003638.pub2
Vestbo, J., Anderson, J. A., Calverley, P. M. A., Celli, B., Ferguson, G. T., Jenkins, C., et al. (2009). Adherence to Inhaled Therapy, Mortality and Hospital Admission in COPD. Thorax. 64 (11), 939–943. doi:10.1136/thx.2009.113662
Weers, J., and Clark, A. (2017). The Impact of Inspiratory Flow Rate on Drug Delivery to the Lungs with Dry Powder Inhalers. Pharm. Res. 34 (3), 507–528. doi:10.1007/s11095-016-2050-x
Weers, J., Clark, A. R., and Challoner, P. (2004). “High Dose Inhaled Powder Delivery: Challenges and Techniques,”. Respiratory Drug Delivery VII. Editors R. N. Dalby, P. R. Byron, J. Peart, and S. J. Farr (Raleigh, NC: Serentec), 281–288.
Weers, J., Son, Y., Glusker, M., Haynes, A., Huang, D., Kadrichu, N., et al. (2019). Ideal Halers Versus Real Inhalers: Is it Possible to Bypass Deposition in the Upper Respiratory Tract. J. Aerosol. Med. Pulm. Drug Deliv. 32 (2), 55–69. doi:10.1089/jamp.2018.1497
Weers, J., and Tarara, T. (2014). The Pulmosphere™ Platform for Pulmonary Drug Delivery. Ther. Deliv. 5 (3), 277–295. doi:10.4155/tde.14.3
Weers, J., Tarara, T., and Clark, A. R. (2007). Design of Fine Particles for Pulmonary Drug Delivery. Expert Opin. Drug Deliv. 4 (3), 297–313. doi:10.1517/17425247.4.3.297
Weers, J., Ung, K., Le, J., Nagaraja, R., Ament, B., Axford, G., et al. (2013). Dose Emission Characteristics of Placebo Pulmosphere Particles are Unaffected by a Subject’s Inhalation Maneuver. J. Aerosol. Med. Pulm. Drug Deliv. 26 (1), 56–68. doi:10.1089/jamp.2012.0973
Yin, J., Bovert, J.-M., Smyth, H., Clark, A., and Hannon, J. (2017). An Add‐on to Dry Powder Inhaler Device that Can Improve Dose Delivery to the Lungs. Respiratory Drug Delivery Europe 2017. Editors R. N. Dalby, J. Peart, J. D. Suman, P. M. Young, and D. Traini (Nice, France: Davis Healthcare International Publishing), 1–4.
Zhong, H., Chan, G., Hu, Y., Hu, H., and Quyang, D. (2018). A Comprehensive Map of FDA-Approved Pharmaceutical Products. Pharmaceutics 10 (4), 263. doi:10.3390/pharmaceutics10040263
Zimlich, W. C., Ding, J. Y., Busick, A., Moutvic, R. R., Placke, M. E., Hirst, P. H., et al. (2000). “The Development of a Novel Electrohydrodynamic Pulmonary Drug Delivery Device,”. Respiratory Drug Delivery VII. Editors R. N. Dalby, P. R. Byron, J. Peart, and S. J. Farr (Raleigh, NC: Serentec), 241–246.
Keywords: pulmonary, inhaler aerosol, drug delivery, dry powder inhaler (DPI), PMDI (pressurized metered dose inhaler), nebulizer, drug deposition
Citation: Clark AR (2022) Half a Century of Technological Advances in Pulmonary Drug Delivery: A Personal Perspective. Front. Drug. Deliv. 2:871147. doi: 10.3389/fddev.2022.871147
Received: 09 February 2022; Accepted: 04 March 2022;
Published: 13 April 2022.
Edited by:
Mohammad Momin, Virginia Commonwealth University, United StatesReviewed by:
Hideyuki Sato, University of Shizuoka, JapanCopyright © 2022 Clark. This is an open-access article distributed under the terms of the Creative Commons Attribution License (CC BY). The use, distribution or reproduction in other forums is permitted, provided the original author(s) and the copyright owner(s) are credited and that the original publication in this journal is cited, in accordance with accepted academic practice. No use, distribution or reproduction is permitted which does not comply with these terms.
*Correspondence: Andrew R. Clark, YWNsYXJrQGFlcm9nZW5waGFybWEuY29t