- 1Shengli Clinical Medical College of Fujian Medical University, Fuzhou, Fujian, China
- 2The Fourth Department of Critical Care Medicine, Fuzhou University Affiliated Provincial Hospital, Fuzhou, Fujian, China
- 3Fujian Provincial Key Laboratory of Emergency Medicine, Fuzhou, Fujian, China
- 4Fujian Emergency Medical Center, Fuzhou, Fujian, China
- 5Department of Emergency, Fuzhou University Affiliated Provincial Hospital, Fuzhou, Fujian, China
Purpose: This study aimed to compare the performance of lactate and CO2-derived parameters in predicting major postoperative complications (MPC) after cardiac surgery with cardiopulmonary bypass.
Methods: Lactate and CO2-derived parameters, including the venous-arterial difference in CO2 partial pressure (Pv-aCO2), the venous-arterial difference in CO2 partial pressure to arterial-venous O2 content ratio (Pv-aCO2/Ca-vO2), and the venous-arterial difference in CO2 content to arterial-venous O2 content ratio (Cv-aCO2/Ca-vO2) at ICU admission, 3 h, 6 h, and 12 h later were collected. Receiver-operating characteristics (ROC) curve analysis was carried out to assess the predictive performance. Univariate and multivariate logistic regression analyses were performed to identify independent predictors of MPC.
Results: MPC occurred in 77 (54.2%) of 142 patients. No significant difference was observed between the MPC and no-MPC groups regarding lactate and CO2-derived parameters. The area under the curves (AUCs) were 0.532 (0.446–0.616) for lactate, 0.559 (0.473–0.642) for Pv-aCO2, 0.617 (0.532–0.697) for Pv-aCO2/Ca-vO2, and 0.625 (0.540–0.705) for Cv-aCO2/Ca-vO2, respectively, and there was no significant difference between the parameters. In the post-hoc analysis, all parameters' AUCs were lower than 0.75 in predicting acute renal failure, and there was no significant difference between these parameters. Cv-aCO2/Ca-vO2 at 12 h yielded the highest AUC of 0.853 (0.784–0.907) in predicting mortality and the highest AUC of 0.808 (0.733–0.869) in predicting delirium. In multivariate analysis, hypertension, surgery duration, and PaO2/FiO2 were identified as independent predictors of MPC, while lactate and CO2-derived parameters lost statistical significance after adjustment for covariates.
Conclusions: Lactate and CO2-derived parameters cannot be used as reliable indicators to predict the occurrence of MPC after cardiopulmonary bypass. Instead, traditional clinical factors such as hypertension, extended surgical duration, and impaired oxygenation emerged as the most reliable risk indicators.
Study registration
The study was registered on January 26th, 2020, at the Chinese Clinical Trial Registry (ChiCTR2000029365). URL of trial registry record: https://www.chictr.org.cn/bin/project/edit?pid=48744.
Introduction
The prompt response to tissue hypoxia following surgery is essential in preventing organ dysfunction and related complications. Biomarkers such as lactate have been proposed for the detection of tissue hypoxia (1–3). However, the presence of cardiopulmonary bypass (CPB) during cardiac surgery can complicate the interpretation of tissue hypoxia. Postoperative hyperlactatemia occurs in approximately 10%–20% of cardiac surgery patients, which may reflect either transient tissue hypoxia due to CPB (which is usually a benign phenomenon) or sustained tissue hypoxia resulting from postoperative circulatory failure (4). While numerous studies have established a link between elevated lactate levels and adverse outcomes post-cardiac surgery, the association is not definitive as elevated lactate levels are not always indicative of sustained tissue hypoxia and anaerobic metabolism (4, 5).
A series of parameters derived from CO₂ have emerged as alternative biomarkers for detecting tissue hypoxia and anaerobic metabolism. These include the venous-arterial difference in CO₂ partial pressure (Pv-aCO₂ or PCO₂ gap), the venous-arterial difference in CO₂ partial pressure to arterial-venous O₂ content ratio (Pv-aCO₂/Ca-vO₂), and the venous-arterial difference in CO₂ content to arterial-venous O₂ content ratio (Cv-aCO₂/Ca-vO₂) (6–9). Pv-aCO₂ primarily reflects the adequacy of cardiac output, as CO₂ accumulates in tissues when perfusion is inadequate, resulting in elevated venous CO₂. However, Pv-aCO₂ alone lacks specificity as a marker of tissue hypoxia and anaerobic metabolism (10). In contrast, both Pv-aCO₂/Ca-vO₂ and Cv-aCO₂/Ca-vO₂ ratios serve as surrogates for the respiratory quotient (RQ)—the ratio of CO₂ production to O₂ consumption—which increases above 1.0 during anaerobic metabolism (11). While the relationship between CO₂ content and CO₂ partial pressure follows a nearly linear relationship under physiological conditions (Pv-aCO₂ = κ × Cv-aCO₂, where κ represents the relationship factor), this linearity can be disrupted during metabolic acidosis, which is common after cardiac surgery (12–15). This potential Pv-aCO₂-Cv-aCO₂ decoupling has led to questions about whether Pv-aCO₂/Ca-vO₂ and Cv-aCO₂/Ca-vO₂ are truly interchangeable in clinical settings, particularly in cardiac surgery patients where acid-base disturbances are frequent. While these CO₂-derived parameters have been increasingly employed to identify low-flow states and guide resuscitation in septic shock, their clinical utility in cardiac surgery patients remains inadequately explored.
The CO2-derived parameters mentioned above, such as Pv-aCO2, Cv-aCO2/Ca-vO2, and Pv-aCO2/Ca-vO2, are increasingly employed to identify the low-flow status or anaerobic metabolism in septic shock for guiding fluid resuscitation (16, 17). However, limited investigation has been conducted on their application in patients undergoing cardiac surgery. This study aims to assess the efficacy of lactate and CO2-derived parameters in predicting major postoperative complications (MPC) following cardiac surgery with CPB.
Methods
Study design and population
The present study is a prospective, single-center diagnostic accuracy study involving patients undergoing elective cardiac surgery. The study protocol and consent forms were approved by the Ethics Committee of Fujian Provincial Hospital (Approval # K2020-01-022). The study was registered on January 26th, 2020, at the Chinese Clinical Trial Registry (ChiCTR2000029365). Informed consent was obtained from all subjects and/or their legal guardians. The study protocol was published on October 1st, 2021 (18). The major change in the protocol was that we also compared the performance of lactate and CO2-derived parameters in predicting mortality, acute kidney injury (AKI), and delirium, respectively, which are the potential consequences of tissue hypoxia.
Patients who received cardiac surgery with CPB were screened. The inclusion criteria were (1) Age ≥ 18; (2) Elective cardiac surgery with CPB; (3) Admitted into the cardiosurgical intensive care unit (ICU) after the operation; (4) With an arterial and a central venous catheter in place. Exclusion criteria were: (1) Known acute or chronic kidney disease according to international diagnostic standards (19–21); (2) Known hepatic insufficiency prior to surgery; (3) Malpositioning of the central venous catheter confirmed by chest x-ray; (4) History of alcohol abuse; (5) Pregnant; (6) Unwilling to provide consent.
Data collection and follow-up
Baseline characteristics were collected, including demographic data, medical history, preoperative ejection fraction, and the New York Heart Association (NYHA) functional classification. Intraoperative data were also recorded, including the type of surgery, duration of operation, CPB and aortic clamping, transfusion, fluid balance, and the doses of vasopressors and inotropic agents. The sequential organ failure assessment score (SOFA) and Acute Physiology and Chronic Health Evaluation (APACHE) II score at enrollment and the daily highest score during the follow-up period were recorded. Arterial and venous blood samples were collected at ICU admission (T0), and 3 h (T3), 6 h (T6), and 12 h (T12) after admission for the measurement of lactate and the calculation of CO2-derived parameters, by using the bedside blood gas analyzer (GEM 3500, Instrumentation Laboratory Co., MA, USA). CO2 content was calculated according to the Douglas formula (22), formulas were also provided in our published protocol (18) and the Supplementary File.
Patients were followed-up for seven days starting from the time of ICU admission. MPC was recorded, as previously defined in the protocol (18). Briefly, we defined the MPC as any of the following conditions: (1) AKI; (2) acute respiratory distress syndrome (ARDS) or respiratory failure; (3) cardiac arrest; (4) neurological dysfunction (including delirium); (5) myocardial infarction; (6) circulatory failure; (7) abdominal compartment syndrome; (8) reoperation; (9) death from any cause. Delirium was assessed daily during the 7-day follow-up period using the Confusion Assessment Method for the Intensive Care Unit (CAM-ICU), a validated tool for detecting delirium in critically ill patients. Assessments were performed by trained ICU nurses or physicians (23). The following postoperative data were also collected: the duration of mechanical ventilation and length of ICU stay; results of C-reactive protein, N-terminal pro-B type natriuretic peptide and procalcitonin; and the daily highest vasoactive-inotropic score (VIS) (24).
Statistical analysis
Perioperative characteristics were compared according to the occurrence or not of MPC. Continuous variables were presented as means and standard deviations (for normal distribution) or medians and interquartile ranges (for non-normal distribution). Student's t-test was used for normally distributed variables, and the Mann–Whitney U-test was used for non-normally distributed variables. Categorical variables were presented as numbers and percentages and analyzed using the χ2-test or Fisher's exact tests as appropriate. Changes over time were examined using Friedman RM-ANOVA on ranks where group distribution was not normal or the two-way ANOVA where distribution was normal and variance equal.
Receiver-operating characteristics (ROC) curve analyses were used to assess the predictive performance of lactate and CO2-derived parameters. The optimal thresholds for predicting the outcomes were determined by maximizing the Youden index. Sensitivity, specificity, predictive values, and likelihood ratios were calculated. The performance of the ROC curves was compared using DeLong's method (25).
Model 1 employed univariate Cox proportional hazards regression to assess the independent association of each variable with the outcome without covariate adjustment. Model 2 used a forced entry Cox proportional hazards model, retaining key variables and adjusting for additional covariates via forward selection. Model 3 applied forward stepwise multivariate logistic regression, incrementally including variables meeting significance thresholds (p < 0.05 for entry, p > 0.10 for removal), adjusted for the same covariates as Model 2. A p-value < 0.05 (two-tailed) was considered significant. Analyses were performed using SPSS Statistical Software (Ver. 23.0, IBM Co., NY, USA) and MedCalc Statistical Software (to compare ROC curves).
Results
A total of 142 patients were included between October 1st, 2021 and January 1st, 2024 (Figure 1). MPC occurred in 77 (54.2%) patients. The three leading MPC components were respiratory failure or ARDS, AKI, and shock (n = 54, 20, and 16, respectively, Figure 2). The baseline pre- and perioperative patient characteristics are summarized in Table 1. In brief, patients with MPC had greater body mass index, more hypertension, and worse NYHA cardiac functional classification at baseline. Preoperative blood gas analysis showed no significant difference between the MPC and no-MPC groups, except for a slightly but significantly lower PaO2 and PaO2/FiO2 ratio in patients with MPC (although both were within the normal range in both groups). Patients with MPC had longer surgery duration [310 (270, 405) vs. 290 (242.5, 338.8) min, p = 0.006], but there was no difference in the CBP duration and aortic clamping duration. Patients with MPC had higher SOFA scores at ICU admission [12 (11, 13) vs. 11 (10, 12.8), p = 0.022]. The median (QL, QU) mechanical ventilation duration was 16 (10, 21) hours, with a longer duration in the MPC group [19 (15, 48) h] compared to the no-MPC group [10 (7, 16) h, p < 0.001]. The length of ICU stay was 2 (1, 3) days, with a longer stay in the MPC group [3 (2, 4) days] compared to the no-MPC group [1 (1, 2) day, p < 0.001]. All deaths occurred in the ICU, and the mortality rate was 4.9% (n = 7).
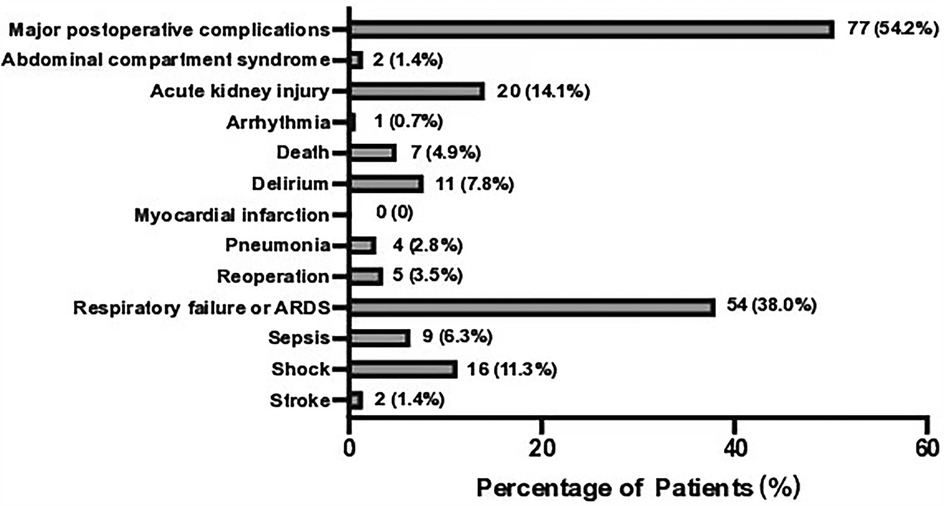
Figure 2. Incidence of major postoperative complications components. Showing the number and percentage of patients. ARDS, acute respiratory distress syndrome.
No statistically significant difference was observed between the MPC and no-MPC groups in terms of lactate and CO2-derived parameters. However, a significant difference was observed between the time points, except for Cv-aCO2/Ca-vO2 (Table 2 and Figure 3). Similar time effects were observed in arterial pH, PaCO2, PvCO2, arterial and venous blood CO2 content, Cv-aCO2, and hemoglobin, and no group effect was observed except hemoglobin. On the other hand, O2-derived parameters (i.e., PaO2, PvO2, and PaO2/FiO2) showed a significant difference between the MPC and no-MPC groups, but there was no intra-group difference in Ca-vO2 (Table 2).
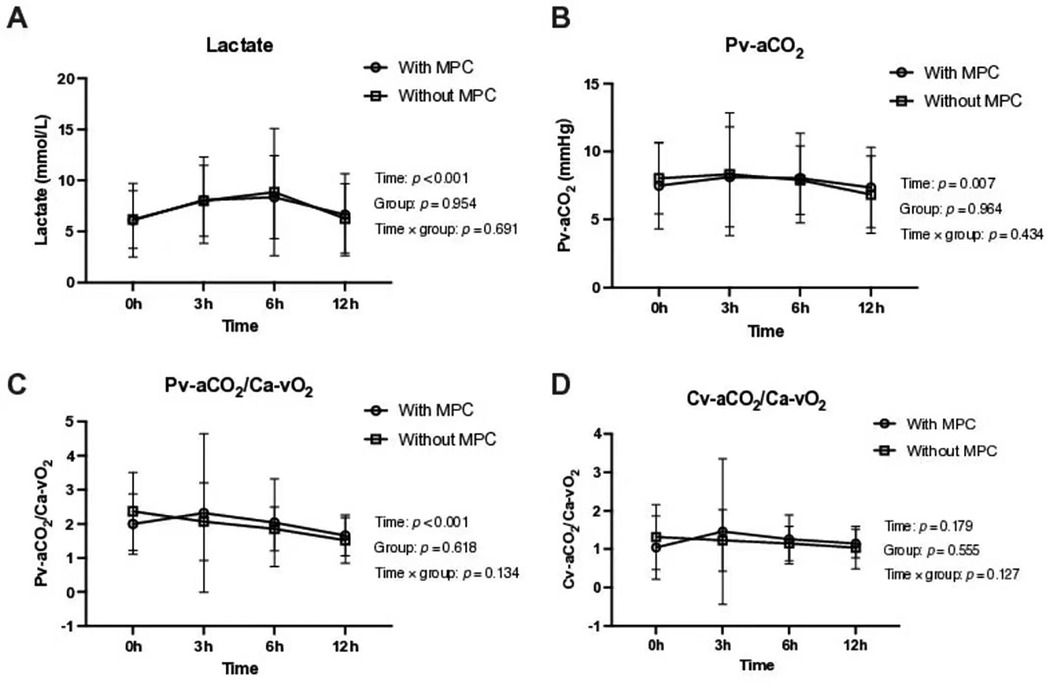
Figure 3. Lactate and CO₂-derived parameters in the first 12 postoperative hours according to major postoperative complications groups. (A) Lactate levels over time. (B) Venous-arterial difference in CO₂ partial pressure (Pv-aCO₂) over time. (C) Venous-arterial difference in CO₂ partial pressure to arterial-venous O₂ content ratio (Pv-aCO₂/Ca-vO₂) over time. (D) Venous-arterial difference in CO₂ content to arterial-venous O₂ content ratio (Cv-aCO₂/Ca-vO₂) over time. All parameters were assessed in patients with and without major postoperative complications (MPC).
For the ROC analysis, we only considered the time points with the highest AUCs for comparing different parameters. AUCs of all the time points are presented in the Supplementary File (S1, Table S1). Lactate and CO2-derived parameters had the greatest AUCs at 0 h in predicting MPC, and there was no significant difference between these four parameters. Generally, lactate and CO2-derived parameters had poor performance in predicting MPC, with AUCs of 0.532 (95% CI: 0.446–0.616) for lactate, 0.559 (0.473–0.642) for Pv-aCO2, 0.617 (0.532–0.697) for Pv-aCO2/Ca-vO2, and 0.625 (0.540–0.705) for Cv-aCO2/Ca-vO2, respectively (Figure 4A and Table 3).
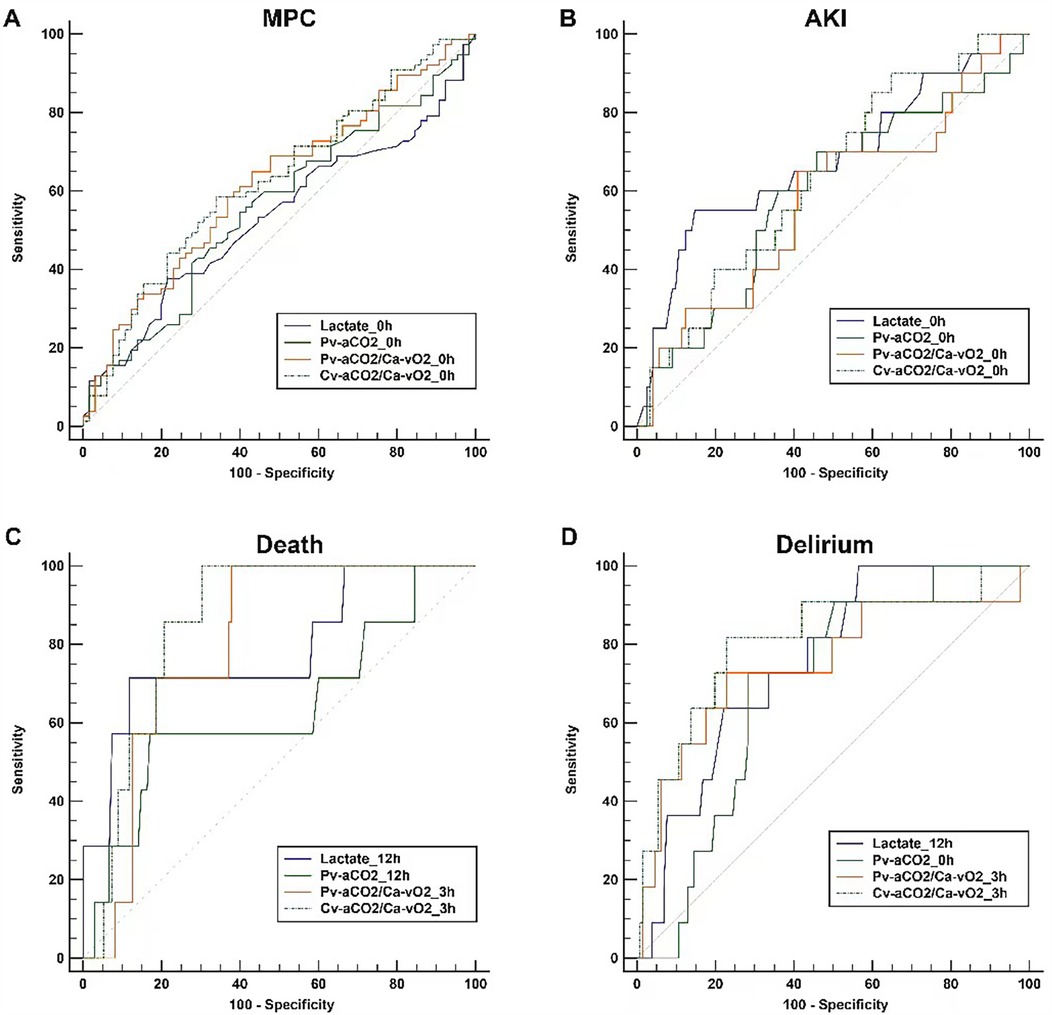
Figure 4. Comparisons of the receiver-operating characteristics curves. For each parameter, only the curve with the highest AUC among different time points is presented. Panel (A) Major postoperative complications (MPC) as the outcome; Panel (B) Acute renal failure (AKI) as the outcome; Panel (C) Death as the outcome; Panel (D) Delirium as the outcome.
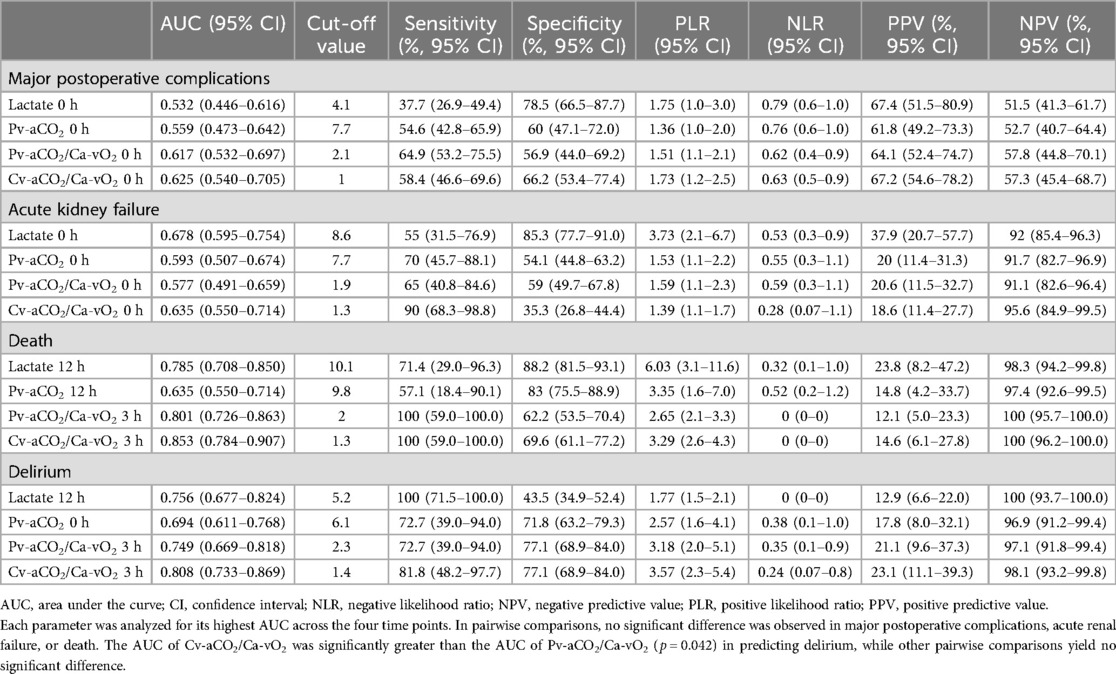
Table 3. Comparisons of receiver-operating characteristics curves of postoperative lactate and CO2-derived parameters.
In addition, we conducted a post-hoc analysis to examine how well lactate and CO2-derived parameters can predict MPC components that are more likely related to tissue hypoxia, including AKI, delirium, and death. Lactate levels in patients with AKI significantly differed over time and between groups. Pv-aCO2 levels only had a significant difference over time, while no significant differences were observed in Pv-aCO2/Ca-vO2 and Cv-aCO2/Ca-vO2 between groups or over time (Supplementary File S1, Figure S1). In the ROC analysis, all parameters had the greatest AUCs at 0 h in predicting AKI. However, all AUCs were lower than 0.75, and these parameters had no significant difference (Table 3 and Figure 4B).
With death as the grouping factor, lactate significantly changed over time (time effect), while no group effect or time×group interaction was observed. No significant time or group effect was observed in Pv-aCO2, Pv-aCO2/Ca-vO2, or Cv-aCO2/Ca-vO2 (Supplementary File S1, Figure S2). In the ROC analysis, Pv-aCO2/Ca-vO2 and Cv-aCO2/Ca-vO2 at 3 h, and lactate and Pv-aCO2 at 12 h had the greatest AUCs in predicting mortality (Figure 4C). Cv-aCO2/Ca-vO2 yielded the highest AUC of 0.853 (95% CI: 0.784–0.907) among these parameters with a cut-off value of 1.3, the sensitivity was 100% (95% CI: 59.0–100.0%), and the specificity was 69.6% (95% CI: 61.1–77.2%); however, the difference of the AUCs did not reach the significance level (Table 3). With delirium as the grouping factor, a significant time effect was observed in all these four parameters. Meanwhile, a significant group effect and time×group interaction was observed in Cv-aCO2/Ca-vO2 (Supplementary File S1, Figure S3). In the ROC analysis, Pv-aCO2/Ca-vO2 and Cv-aCO2/Ca-vO2 at 3 h, lactate at 12 h, and Pv-aCO2 at 0 h had the greatest AUCs in predicting delirium (Figure 4D). Cv-aCO2/Ca-vO2 yielded the highest AUC of 0.808 (95% CI: 0.733–0.869) among these parameters with a cut-off value of 1.4, the sensitivity was 81.8% (95% CI: 48.2–97.7%), and the specificity was 77.1% (95% CI: 68.9–84.0%); a significant difference was observed between the AUCs of Pv-aCO2/Ca-vO2 and Cv-aCO2/Ca-vO2 (p = 0.042, Table 3).
Hypertension was significantly associated with the outcome across all models, with hazard ratios (HRs) ranging from 2.03 (95% CI: 1.45–13.89, p = 0.001) in Model 1 to 1.40 (95% CI: 1.40–11.21, p = 0.01) in Model 3. Surgery Duration also showed a consistent association, with HRs of 1.002 (95% CI: 1.002–1.011, p = 0.003) in Model 1 and 1.001 (95% CI: 1.001–1.011, p = 0.015) in Model 3. PaO2/FiO2 was significantly predictive in all models, with HRs of 0.98 (95% CI: 0.98–0.994, p = 0.001) in Model 1 and 0.982 (95% CI: 0.982–0.997, p = 0.007) in Model 3. Lactate 0 h and CO2-derived parameters (e.g., Pv-aCO2/Ca-vO2 0 h) did not show significant associations in Models 2 and 3 (p > 0.05), except for Pv-aCO2/Ca-vO2 0 h in Model 1 (HR: 0.45, 95% CI: 0.45–0.98, p = 0.039, Table 4).
Discussion
The main findings of the present study were: (1) there was no difference in lactate and CO2-derived parameters between patients with or without MPC, and these parameters had limited predictive performance; (2) the performance of lactate and CO2-derived parameters improved in predicting delirium and death; (3) Cv-aCO2/Ca-vO2 had the best predictive performance in predicting delirium and mortality.
Complications after cardiac surgery are associated with high morbidity and mortality (26–28). Although multifactorial, one crucial factor of postoperative organ dysfunction is the mismatch of oxygen delivery and consumption (29, 30). The imbalance of oxygen delivery and consumption leads to tissue hypoxia, which eventually leads to organ injury and dysfunction if uncorrected. In this regard, parameters reflecting tissue hypoxia may help manage patients undergoing cardiac surgery, and there is increasing interest in these indicators of hypoxia. The majority of previous studies focused on Pv-aCO2/Ca-vO2 (31–34). Cv-aCO2/Ca-vO2 was investigated in one prospective observational study involving adult postoperative cardiac surgery patients. However, the aim was to evaluate the ability of Cv-aCO2/Ca-vO2 and Pv-aCO2/Ca-vO2 to predict an increase in oxygen consumption upon fluid challenge rather than organ dysfunctions or clinical outcomes (27). To the best of our knowledge, this study is the first to compare the performance of Cv-aCO2/Ca-vO2 and Pv-aCO2/Ca-vO2 in predicting outcomes directly.
We found no difference in lactate and CO2-derived parameters between patients with or without MPC, and these parameters had limited performance in predicting MPC. One potential explanation is the complex relationship between MPC and anaerobic metabolism. An impaired function of oxygenation of the lung (for example, ARDS or respiratory failure, which is the component of MPC) or an impaired ability of the heart to deliver blood flow to tissues (e.g., shock, circulatory failure, etc.) is the cause of the imbalance of oxygen delivery and consumption rather than the result.
Our finding is consistent with recently published studies (32, 34). A retrospective study found that Pv-aCO2 was associated with postoperative adverse outcomes but showed poor diagnostic performance (34). Zhang and colleagues found that Pv-aCO2 and Pv-aCO2/Ca-vO2 cannot be used as reliable indicators to predict the occurrence of organ dysfunction in a prospective observational study (32). In contrast to these negative findings, Mukai et al. reported that postoperative Pv-aCO2 was an independent predictor of major organ morbidity and mortality with an AUC of 0.804 (95% CI: 0.688–0.921) (35). However, it is worth noting that the measurements were obtained right at the end of the surgery in their study, which may be a reason for the inconsistent findings. In addition, selected outcomes vary from one study to the other, which is another factor of the discrepancy.
We conducted a post-hoc analysis using mortality, AKI, and delirium as the outcome, respectively. The reason for choosing these outcomes was that they are more likely the consequences than the cause of tissue hypoxia. We hypothesized that biomarkers of tissue hypoxia and anaerobic metabolism could have better performance in predicting the consequences of tissue hypoxia. Biomarkers are considered to have good discriminative performance when the AUC is greater than 0.75 (36). Our data suggest that lactate, Pv-aCO2/Ca-vO2, and Cv-aCO2/Ca-vO2 had good performance in discriminating mortality and delirium. However, statistical significance was not observed in pairwise comparisons except for the comparison between Pv-aCO2/Ca-vO2 and Cv-aCO2/Ca-vO2 in delirium. This finding could be attributed to the limited statistical power of the test, as the number of positive cases was small. On the other hand, lactate and CO2-derived parameters had limited performance in predicting AKI. Post-cardiac-surgery AKI is multifactorial, with the spectrum of insults involving micro-embolism, neurohormonal activation, endogenous and exogenous nephrotoxins, metabolic and hemodynamic factors, inflammation, ischemia-reperfusion injury, and oxidative stress (37). A single biomarker of tissue hypoxia may be insufficient to predict AKI.
One aim of the study was to compare the performance of Pv-aCO2/Ca-vO2 and Cv-aCO2/Ca-vO2. Our data demonstrate that although Cv-aCO2/Ca-vO2 had numerically higher AUCs than Pv-aCO2/Ca-vO2 in predicting MPC, AKI, and mortality, these differences did not reach statistical significance. The only statistically significant difference was observed in predicting delirium (p = 0.042). These findings should be interpreted with caution, particularly for the non-significant differences, which should not be over-interpreted as evidence of superiority. The clinical relevance of these statistical differences also warrants consideration. Even for the statistically significant difference observed in predicting delirium, the actual clinical benefit of using Cv-aCO2/Ca-vO2 over Pv-aCO2/Ca-vO2 may be modest. While the calculation of Cv-aCO2/Ca-vO2 does not require additional blood samples compared to Pv-aCO2/Ca-vO2, the minimal improvement in predictive performance needs to be balanced against the added complexity of calculation in routine clinical settings. Further studies with larger sample sizes are needed to confirm whether the observed trends in favor of Cv-aCO2/Ca-vO2 represent clinically meaningful advantages over Pv-aCO2/Ca-vO2, and to establish whether these differences are substantial enough to warrant changes in clinical practice.
We utilized a composite endpoint (MPC) in our study. This approach has both strengths and limitations. First, composite endpoints are well-established in cardiovascular research as they capture the overall burden of morbidity, which is particularly relevant in cardiac surgery populations where patients often experience multiple complications with shared underlying mechanisms of tissue hypoperfusion and oxygen supply-demand imbalance (6). Second, composite endpoints provide greater statistical efficiency when individual complications may be relatively uncommon, enhancing statistical power while maintaining clinical relevance. The composite endpoint enhances statistical efficiency and captures the overall burden of morbidity, which is clinically relevant in the cardiac surgical setting where tissue hypoperfusion may manifest in multiple organ systems. However, the heterogeneity within our composite endpoint should be considered when interpreting our findings. The stronger associations observed for certain complications suggest that lactate and CO2-derived parameters may be particularly useful for predicting and potentially preventing specific complications rather than the entire spectrum of postoperative morbidity. Future research may benefit from more targeted approaches focusing on homogeneous pathophysiological pathways or organ-specific complications, potentially enhancing both biological plausibility and clinical application of these parameters.
The multivariate analysis identified three independent predictors of MPC after cardiac surgery: preexisting hypertension, extended surgery duration, and reduced PaO₂/FiO₂ ratios. These associations persisted after rigorous adjustment for confounding variables, suggesting their robust prognostic value. Interestingly, while CO₂-derived parameters—specifically Pv-aCO₂/Ca-vO₂ ratio—demonstrated potential predictive capacity in univariate analysis, they failed to maintain statistical significance in multivariate models. This pattern of attenuated association after covariate adjustment suggests that tissue hypoxia biomarkers may reflect downstream consequences of other pathophysiological processes rather than serving as independent causal factors for MPC.
The loss of statistical significance for CO₂-derived parameters in multivariate models should not entirely negate their clinical relevance. Instead, it suggests that these biomarkers may provide complementary information within specific clinical contexts, particularly when interpreted alongside traditional risk factors. Their limited independent predictive value may reflect the complex, multifactorial nature of postoperative complications, which likely extend beyond tissue hypoxia alone.
There were several limitations of the study. The primary limitation was the sample size issue. While our sample size (n = 142) was calculated based on a previously reported major postoperative complication rate of 56.5% and provided adequate statistical power (β = 0.1, power 90%) for evaluating the ability of lactate and CO2-derived parameters to predict MPC, it was evidently insufficient for rare secondary outcomes such as mortality (n = 7). This creates a risk of type II errors and, consequently, results regarding these secondary outcomes should be interpreted with caution and considered primarily hypothesis-generating rather than definitive conclusions. The original sample size calculation was based on an expected area under the ROC curve (AUC) of 0.7, a type I error probability (α) of 0.05, a type II error probability (β) of 0.1, and an anticipated incidence rate of approximately 56.5% for the primary outcome (18). This calculation was appropriate for analyzing the primary outcome but inadequate for secondary endpoints with lower incidence rates. Future research should consider larger multi-center studies to validate our findings, particularly those related to rare outcomes such as mortality. Second, although one important purpose of the study was to compare the performance of the surrogates of the respiratory quotient, no “gold standard” method for measuring respiratory quotient, such as indirect calorimetry (38), was used. Third, central venous rather than mixed venous blood samples were taken to calculate all the CO2-derived parameters. Fourth, the current study was a single-center study, which limits the external validity of the results.
Conclusions
Lactate and CO2-derived parameters including Pv-aCO2, Pv-aCO2/Ca-vO2, and Cv-aCO2/Ca-vO2 cannot be used as reliable indicators to predict the occurrence of MPC after CBP. Instead, traditional clinical factors such as hypertension, extended surgical duration, and impaired oxygenation emerged as the most reliable risk indicators. Future research should focus on developing multimodal approaches that combine traditional risk factors with novel biomarkers to better identify patients at risk for specific complications in cardiac surgical populations.
Data availability statement
The raw data supporting the conclusions of this article will be made available by the authors, without undue reservation.
Ethics statement
The studies involving humans were approved by the Ethics Committee of Fujian Provincial Hospital (Approval # K2020-01-022). The studies were conducted in accordance with the local legislation and institutional requirements. The participants provided their written informed consent to participate in this study. Written informed consent was obtained from the individual(s) for the publication of any potentially identifiable images or data included in this article.
Author contributions
X-FZ: Conceptualization, Data curation, Formal analysis, Funding acquisition, Investigation, Methodology, Project administration, Resources, Software, Supervision, Validation, Visualization, Writing – original draft, Writing – review & editing. HC: Data curation, Formal analysis, Investigation, Methodology, Project administration, Resources, Supervision, Validation, Writing – original draft, Writing – review & editing. JK: Formal analysis, Investigation, Methodology, Project administration, Supervision, Visualization, Writing – review & editing. S-RL: Formal analysis, Investigation, Methodology, Project administration, Supervision, Visualization, Writing – review & editing. T-FH: Data curation, Formal analysis, Investigation, Methodology, Project administration, Visualization, Writing – review & editing. B-YC: Data curation, Formal analysis, Investigation, Project administration, Visualization, Writing – review & editing. X-DJ: Data curation, Formal analysis, Investigation, Methodology, Project administration, Visualization, Writing – review & editing. FC: Conceptualization, Data curation, Formal analysis, Funding acquisition, Investigation, Methodology, Project administration, Resources, Software, Supervision, Validation, Visualization, Writing – original draft, Writing – review & editing.
Funding
The author(s) declare that financial support was received for the research and/or publication of this article. FC is supported by the Major Health Research Project of Fujian Province (Grant Number: 2022ZD01008).HC is supported by the General Program of Natural Science Foundation of Fujian Province (Grant Number: 2021J01369) and the Fujian Provincial Health Technology Project (Grant Number: 2021GGA002). XFZ is supported by the General Program of Natural Science Foundation of Fujian Province (Grant Number: 2023J011179). The funders had no role in study design, data collection, and analysis, the decision to publish, or manuscript preparation.
Conflict of interest
The authors declare that the research was conducted in the absence of any commercial or financial relationships that could be construed as a potential conflict of interest.
Generative AI statement
The author(s) declare that no Generative AI was used in the creation of this manuscript.
Publisher's note
All claims expressed in this article are solely those of the authors and do not necessarily represent those of their affiliated organizations, or those of the publisher, the editors and the reviewers. Any product that may be evaluated in this article, or claim that may be made by its manufacturer, is not guaranteed or endorsed by the publisher.
Supplementary material
The Supplementary Material for this article can be found online at: https://www.frontiersin.org/articles/10.3389/fcvm.2025.1504431/full#supplementary-material
References
1. Hare GMT, Han K, Leshchyshyn Y, Mistry N, Kei T, Dai SY, et al. Potential biomarkers of tissue hypoxia during acute hemodilutional anemia in cardiac surgery: a prospective study to assess tissue hypoxia as a mechanism of organ injury. Can J Anaesth. (2018) 65:901–13. doi: 10.1007/s12630-018-1140-0
2. Andersen LW. Lactate elevation during and after Major cardiac surgery in adults: a review of etiology, prognostic value, and management. Anesth Analg. (2017) 125:743–52. doi: 10.1213/ane.0000000000001928
3. Raggi F, Cangelosi D, Becherini P, Blengio F, Morini M, Acquaviva M, et al. Transcriptome analysis defines myocardium gene signatures in children with ToF and ASD and reveals disease-specific molecular reprogramming in response to surgery with cardiopulmonary bypass. J Transl Med. (2020) 18:21. doi: 10.1186/s12967-020-02210-5
4. Minton J, Sidebotham DA. Hyperlactatemia and cardiac surgery. J Extra Corpor Technol. (2017) 49:7–15. doi: 10.1051/ject/201749007
5. Renew JR, Barbara DW, Hyder JA, Dearani JA, Rivera M, Pulido JN. Frequency and outcomes of severe hyperlactatemia after elective cardiac surgery. J Thorac Cardiovasc Surg. (2016) 151:825–30. doi: 10.1016/j.jtcvs.2015.10.063
6. Moussa MD, Durand A, Leroy G, Vincent L, Lamer A, Gantois G, et al. Central venous-to-arterial PCO2 difference, arteriovenous oxygen content and outcome after adult cardiac surgery with cardiopulmonary bypass: a prospective observational study. Eur J Anaesthesiol. (2019) 36:279–89. doi: 10.1097/eja.0000000000000949
7. Huette P, Ellouze O, Abou-Arab O, Guinot PG. Venous-to-arterial pCO2 difference in high-risk surgical patients. J Thorac Dis. (2019) 11:S1551–s7. doi: 10.21037/jtd.2019.01.109
8. He H, Long Y, Liu D, Wang X, Tang B. The prognostic value of central venous-to-arterial CO2 difference/arterial-central venous O2 difference ratio in septic shock patients with central venous O2 saturation ≥80. Shock. (2017) 48:551–7. doi: 10.1097/shk.0000000000000893
9. Mallat J, Lemyze M, Meddour M, Pepy F, Gasan G, Barrailler S, et al. Ratios of central venous-to-arterial carbon dioxide content or tension to arteriovenous oxygen content are better markers of global anaerobic metabolism than lactate in septic shock patients. Ann Intensive Care. (2016) 6:10. doi: 10.1186/s13613-016-0110-3
10. Bakker J, Vincent JL, Gris P, Leon M, Coffernils M, Kahn RJ. Veno-arterial carbon dioxide gradient in human septic shock. Chest. (1992) 101:509–15. doi: 10.1378/chest.101.2.509
11. Ospina-Tascon GA, Umana M, Bermudez W, Bautista-Rincon DF, Hernandez G, Bruhn A, et al. Combination of arterial lactate levels and venous-arterial CO2 to arterial-venous O 2 content difference ratio as markers of resuscitation in patients with septic shock. Intensive Care Med. (2015) 41:796–805. doi: 10.1007/s00134-015-3720-6
12. McHardy GJ. The relationship between the differences in pressure and content of carbon dioxide in arterial and venous blood. Clin Sci. (1967) 32:299–309.6022823
13. Jensen FB. Comparative analysis of autoxidation of haemoglobin. J Exp Biol. (2001) 204:2029–33. doi: 10.1242/jeb.204.11.2029
14. Stef A, Bodolea C, Bocsan IC, Cainap SS, Achim A, Serban A, et al. The value of biomarkers in Major cardiovascular surgery necessitating cardiopulmonary bypass. Rev Cardiovasc Med. (2024) 25:355. doi: 10.31083/j.rcm2510355
15. Lin X, Wang F, Wang Y. Study on the predictive value of a pulmonary edema imaging score for delayed extubation in patients after heart valve surgery on cardiopulmonary bypass. Rev Cardiovasc Med. (2024) 25:387. doi: 10.31083/j.rcm2510387
16. Vallet B, Pinsky MR, Cecconi M. Resuscitation of patients with septic shock: please “mind the gap"!. Intensive Care Med. (2013) 39:1653–5. doi: 10.1007/s00134-013-2998-5
17. Diaztagle Fernandez JJ, Rodriguez Murcia JC, Sprockel Diaz JJ. Venous-to-arterial carbon dioxide difference in the resuscitation of patients with severe sepsis and septic shock: a systematic review. Med Intensiva. (2017) 41:401–10. doi: 10.1016/j.medin.2017.03.008
18. Zhou X-F, Yu R-G, Chen Q, Xue Y-M, Chen H. Performance of lactate and CO2-derived parameters in predicting Major postoperative complications after cardiac surgery with cardiopulmonary bypass: protocol of a diagnostic accuracy study. Front Cardiovasc Med. (2021) 8:724713. doi: 10.3389/fcvm.2021.724713
19. Stevens PE, Levin A. Evaluation and management of chronic kidney disease: synopsis of the kidney disease: improving global outcomes 2012 clinical practice guideline. Ann Intern Med. (2013) 158:825–30. doi: 10.7326/0003-4819-158-11-201306040-00007
20. Kellum JA, Lameire N. Diagnosis, evaluation, and management of acute kidney injury: a KDIGO summary (part 1). Crit Care. (2013) 17:204. doi: 10.1186/cc11454
21. Lameire N, Kellum JA. Contrast-induced acute kidney injury and renal support for acute kidney injury: a KDIGO summary (part 2). Crit Care. (2013) 17:205. doi: 10.1186/cc11455
22. Douglas AR, Jones NL, Reed JW. Calculation of whole blood CO2 content. J Appl Physiol (1985). (1988) 65:473–7. doi: 10.1152/jappl.1988.65.1.473
23. Ely EW, Inouye SK, Bernard GR, Gordon S, Francis J, May L, et al. Delirium in mechanically ventilated patients: validity and reliability of the confusion assessment method for the intensive care unit (CAM-ICU). Jama. (2001) 286:2703–10. doi: 10.1001/jama.286.21.2703
24. Chen H, Cheng ZB, Yu RG. Procalcitonin as a predictor of moderate to severe acute respiratory distress syndrome after cardiac surgery with cardiopulmonary bypass: a study protocol for a prospective cohort study. BMJ Open. (2014) 4:e006344. doi: 10.1136/bmjopen-2014-006344
25. DeLong ER, DeLong DM, Clarke-Pearson DL. Comparing the areas under two or more correlated receiver operating characteristic curves: a nonparametric approach. Biometrics. (1988) 44:837–45. doi: 10.2307/2531595
26. Kunst G, Milojevic M, Boer C, De Somer F, Gudbjartsson T, van den Goor J, et al. 2019 EACTS/EACTA/EBCP guidelines on cardiopulmonary bypass in adult cardiac surgery. Br J Anaesth. (2019) 123:713–57. doi: 10.1016/j.bja.2019.09.012
27. Abou-Arab O, Braik R, Huette P, Bouhemad B, Lorne E, Guinot PG. The ratios of central venous to arterial carbon dioxide content and tension to arteriovenous oxygen content are not associated with overall anaerobic metabolism in postoperative cardiac surgery patients. PLoS One. (2018) 13:e0205950. doi: 10.1371/journal.pone.0205950
28. O'Brien SM, Feng L, He X, Xian Y, Jacobs JP, Badhwar V, et al. The society of thoracic surgeons 2018 adult cardiac surgery risk models: part 2-statistical methods and results. Ann Thorac Surg. (2018) 105:1419–28. doi: 10.1016/j.athoracsur.2018.03.003
29. Lannemyr L, Bragadottir G, Hjarpe A, Redfors B, Ricksten SE. Impact of cardiopulmonary bypass flow on renal oxygenation in patients undergoing cardiac operations. Ann Thorac Surg. (2019) 107:505–11. doi: 10.1016/j.athoracsur.2018.08.085
30. Schultz JM, Karamlou T, Shen I, Ungerleider RM. Cardiac output augmentation during hypoxemia improves cerebral metabolism after hypothermic cardiopulmonary bypass. Ann Thorac Surg. (2006) 81:625–32. discussion 32-3. doi: 10.1016/j.athoracsur.2005.06.042
31. Caruso V, Besch G, Nguyen M, Pili-Floury S, Bouhemad B, Guinot PG. Treatment of hyperlactatemia in acute circulatory failure based on CO(2)-O(2)-derived indices: study protocol for a prospective, multicentric, single, blind, randomized, superiority study (the LACTEL study). Front Cardiovasc Med. (2022) 9:898406. doi: 10.3389/fcvm.2022.898406
32. Zhang S, Zheng D, Chu XQ, Jiang YP, Wang CG, Zhang QM, et al. ΔPCO(2) and ΔPCO(2)/C((a-cv))O(2) are not predictive of organ dysfunction after cardiopulmonary bypass. Front Cardiovasc Med. (2021) 8:759826. doi: 10.3389/fcvm.2021.759826
33. Insom G, Marinari E, Scolari AF, Garisto C, Vitale V, Di Chiara L, et al. Veno-arterial CO(2) difference and cardiac index in children after cardiac surgery. Cardiol Young. (2021) 31:597–601. doi: 10.1017/s1047951120004357
34. Huette P, Beyls C, Mallat J, Martineau L, Besserve P, Haye G, et al. Central venous-to-arterial CO(2) difference is a poor tool to predict adverse outcomes after cardiac surgery: a retrospective study. Can J Anaesth. (2021) 68:467–76. doi: 10.1007/s12630-020-01881-4
35. Mukai A, Suehiro K, Kimura A, Funai Y, Matsuura T, Tanaka K, et al. Comparison of the venous-arterial CO(2) to arterial-venous O(2) content difference ratio with the venous-arterial CO(2) gradient for the predictability of adverse outcomes after cardiac surgery. J Clin Monit Comput. (2020) 34:41–53. doi: 10.1007/s10877-019-00286-z
36. Ray P, Le Manach Y, Riou B, Houle TT. Statistical evaluation of a biomarker. Anesthesiology. (2010) 112:1023–40. doi: 10.1097/ALN.0b013e3181d47604
37. Milne B, Gilbey T, De Somer F, Kunst G. Adverse renal effects associated with cardiopulmonary bypass. Perfusion. (2023) 39:452–68. doi: 10.1177/02676591231157055
Keywords: lactate, pv-aCO2/Ca-vO2, cv-aCO2/Ca-vO2, postoperative complications, diagnostic accuracy study
Citation: Zhou X-F, Chen H, Ke J, Lin S-R, Huang T-F, Chen B-Y, Jiang X-D and Chen F (2025) Lactate and CO2-derived parameters are not predictive factors of major postoperative complications after cardiac surgery with cardiopulmonary bypass: a diagnostic accuracy study. Front. Cardiovasc. Med. 12:1504431. doi: 10.3389/fcvm.2025.1504431
Received: 30 September 2024; Accepted: 25 March 2025;
Published: 11 April 2025.
Edited by:
Antonino S. Rubino, Kore University of Enna, ItalyReviewed by:
Robert Jeenchen Chen, Stanford University, United StatesGuo-wei Tu, Fudan University, China
Copyright: © 2025 Zhou, Chen, Ke, Lin, Huang, Chen, Jiang and Chen. This is an open-access article distributed under the terms of the Creative Commons Attribution License (CC BY). The use, distribution or reproduction in other forums is permitted, provided the original author(s) and the copyright owner(s) are credited and that the original publication in this journal is cited, in accordance with accepted academic practice. No use, distribution or reproduction is permitted which does not comply with these terms.
*Correspondence: Feng Chen, ZmpzbGNoZW5mZW5nQDEyNi5jb20=
†These authors have contributed equally to this work
‡ORCID:
Feng Chen
orcid.org/0000-0002-4419-9707