- Beijing Key Laboratory of Precision Medicine of Coronary Atherosclerotic Disease, Department of Cardiology, Beijing Anzhen Hospital, Beijing Institute of Heart Lung and Blood Vessel Disease, Clinical Center for Coronary Heart Disease, Capital Medical University, Beijing, China
Background: The prognostic value of triglyceride-glucose (TyG) has been well described in patients with coronary artery disease (CAD). Hyperhomocysteinemia (HHcy) promotes insulin resistance and has also been regarded as a potential risk factor for cardiovascular disease. However, the prognostic value of TyG in acute coronary syndrome (ACS) patients undergoing percutaneous coronary intervention (PCI) and the interaction between TyG and HHcy remain unclear.
Methods: A total of 1,734 ACS patients undergoing PCI were continuously enrolled between June 2016 and November 2017 at Beijing Anzhen Hospital. Patients were categorized into four groups based on HHcy status and the optimal cut-off value of TyG. The primary endpoint was major adverse cardiovascular events (MACE), a composite of all-cause death, nonfatal myocardial infarction, nonfatal stroke, and unplanned repeat revascularization.
Results: Over a median follow-up of 927 days, 358 patients (20.6%) experienced MACE. The Kaplan-Meier curves showed significant differences in the cumulative incidence of MACE among prespecified groups (p < 0.001). Multivariable Cox regression analysis revealed that higher TyG was significantly associated with an increased risk of MACE in patients without HHcy (HR: 2.36, 95% CI: 1.53–3.64, p < 0.001), but not in patients with HHcy (HR: 1.31, 95% CI: 0.60–2.87, p = 0.503). Restricted cubic splines only demonstrated the prognostic value of TyG in patients without HHcy. A significant interaction was observed for MACE between TyG and HHcy (p for interaction = 0.01).
Conclusions: The prognostic value of TyG was modified by HHcy in ACS patients undergoing PCI. Higher TyG was only associated with an increased risk of MACE in ACS patients without HHcy, but not in ACS patients with HHcy.
1 Introduction
Coronary artery disease (CAD) remains a leading cause of global mortality and health loss, and causes an increasing public health burden worldwide (1, 2). Despite guideline-recommended medical therapy and percutaneous coronary intervention (PCI), patients with acute coronary syndrome (ACS) still faced relatively high cardiovascular risks (3). Therefore, it is necessary to further identify reliable prognostic factors for ACS patients undergoing PCI.
Insulin resistance (IR) and homocysteine (Hcy) are both metabolic risk factors for CAD. IR refers to decreased responsiveness of the body to insulin, resulting in the inability of insulin to stimulate tissue cells for glucose uptake and utilization. Although the hyperinsulinemic-euglycemic clamp (HEC) is the gold standard for assessing IR (4), it is rarely utilized in clinical practice due to its complexity, time consumption, expense, and invasiveness. Triglyceride glucose (TyG) is a novel index composed of blood lipids and glucose. It is considered as a reliable index reflecting insulin resistance, showing consistent accuracy with the hyperinsulinemic-euglycemic clamp, and is more economical and convenient (5–7). Homocysteine (Hcy) is a sulfhydryl-containing amino acid present in plasma and an important intermediary product in the metabolism of cysteine and methionine. Hyperhomocysteinemia (HHcy) is independently associated with blood lipid levels (8) and promotes IR through several synergistic mechanisms (9–11). Hcy promotes endothelial dysfunction, prothrombotic effects, and irreversible alteration in remodeling of the vessel wall (12–15).
Previous studies have demonstrated that higher TyG is independently associated with poor prognosis in CAD patients (16–19). For decades, HHcy has been recognized as one of the cardiovascular risk factors (20, 21). However, the prognostic value of TyG in ACS patients undergoing PCI and the interaction between TyG and Hcy remain unclear. In this study, we aimed to explore the prognostic value of TyG in ACS patients undergoing PCI and further evaluate the interaction between TyG and HHcy.
2 Materials and methods
2.1 Study population
This is a single-center, retrospective analysis including 1,734 ACS patients undergoing PCI between June 2016 and November 2017 at Beijing Anzhen Hospital. The exclusion criteria were as follows: Patients with (1) prior coronary artery bypass grafting; (2) cardiogenic shock; (3) estimated glomerular filtration rate (eGFR) < 15 ml/min/1.73 m2; (4) incomplete follow-up (Figure 1). In the final analysis, 1,734 patients were continuously enrolled and categorized into four groups based on HHcy status and the optimal cut-off value of TyG.
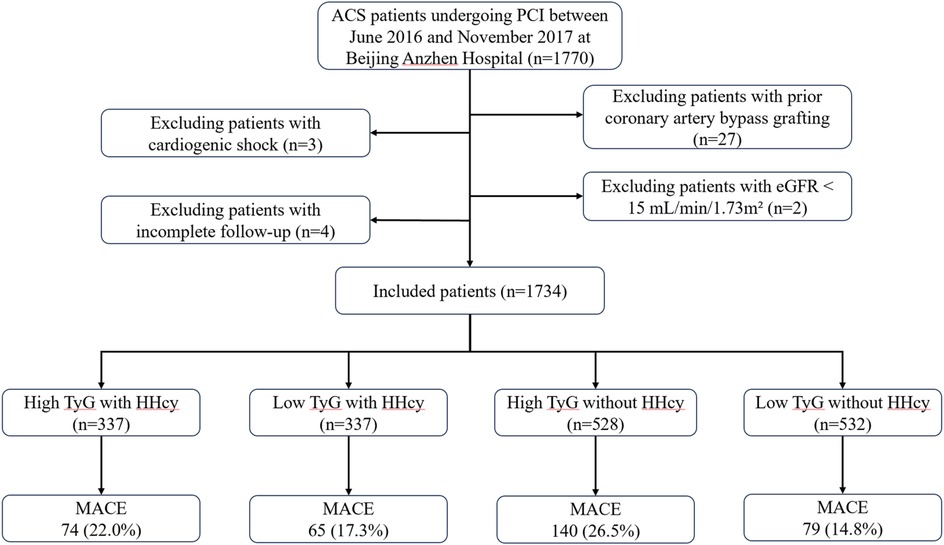
Figure 1. Study flow chart. ACS, acute coronary syndrome; PCI, percutaneous coronary intervention; HHcy, hyperhomocysteinemia; eGFR, estimated glomerular filtration rate; TyG, triglyceride glucose; MACE, major adverse cardiovascular events.
The diagnosis of ACS was determined according to the 2013 ACCF/AHA and 2014 AHA/ACC guidelines (22, 23). ST-segment elevation myocardial infarction (STEMI) was defined as myocardial ischemic symptoms accompanied by persistent electrocardiographic ST elevation and elevated levels of creatine kinase or cardiac troponin higher than the upper limit of the normal range. The diagnosis of non-ST-segment elevation myocardial infarction (NSTEMI) and unstable angina (UA) was determined based on the presence or absence of elevated cardiac biomarkers. HHcy was defined as Hcy > 15 μmol/L.
2.2 Data collection and definitions
Data on demographics, medical history, procedural results, and medications were collected from the electronic medical records system using standardized questionnaires. Body mass index (BMI) was calculated by weight/height2 (kg/m2). Fasting blood glucose (FBG), total cholesterol (TC), low-density lipoprotein-cholesterol (LDL-C), high-density lipoprotein-cholesterol (HDL-C), triglyceride (TG), and homocysteine levels were measured after admission in the central laboratory of Beijing Anzhen Hospital. TyG index was calculated using the formula Ln (TG[mg/dl] × FBG[mg/dl]/2). Hypertension was diagnosed when systolic blood pressure was ≥140 mmHg and/or diastolic blood pressure (DBP) was ≥90 mmHg and/or receiving antihypertension treatment. Diabetes was considered when 2-h blood glucose after an oral glucose tolerance test (OGTT) was ≥11.1 mmol/L, and/or FBG was ≥7.0 mmol/L and/or casual plasma glucose was ≥11.1 mmol/L along with diabetic symptoms, and/or taking antidiabetic medication. Chronic kidney disease (CKD) was defined as an estimated glomerular filtration rate (eGFR) ≤60 ml/min/1.73 m2. Heart failure (HF) was diagnosed in patients with symptoms/signs of congestive heart failure accompanied by elevated B-type natriuretic peptide (BNP) levels, receiving ongoing therapy for heart failure, or left ventricular ejection fraction (LVEF) ≤ 40%.
2.3 Follow-up and endpoints
Follow-up assessments were conducted at one month and subsequently every six months after discharge. Information of adverse events were obtained through telephone contacts and electronic medical records by trained personnel who were blinded to the patients' baseline data. The primary endpoint was major adverse cardiovascular events (MACE), a composite of all-cause death, nonfatal myocardial infarction (MI), nonfatal stroke, and unplanned repeat revascularization (URR). Any non-staged revascularization that occurred after the initial PCI was considered as an unplanned repeat revascularization.
2.4 Statistical analysis
Due to the lack of comparable studies evaluating the effect of HHcy on the prognostic value of TyG index, a power analysis to determine the required sample size was waived. Continuous variables with a normal distribution were reported as means ± standard deviations, while those with non-normal distribution were reported as medians and interquartile ranges. Categorical variables were presented as frequencies and percentages. Differences in continuous variables among groups were examined using unpaired t-test or Mann–Whitney U test. The comparison of categorical variables between groups was performed using the chi-squared test.
Correlation analysis was conducted using Pearson's or Spearman's tests. Survival analyses for MACE were carried out using Kaplan–Meier curves and p value was calculated by log-rank test. Cox proportional hazards analysis was used to calculate hazard ratios (HR) and 95% confidence intervals (CI). Variables that showed statistical significance in baseline characteristics, as well as those of clinical importance, were included in the multivariate Cox proportional hazards model. P for interaction was tested by adding an interaction term to the Cox proportional hazards model. The linear association and optimal cut-off value of TyG index was evaluated using restricted cubic spline (RCS) with four knots. A two-sided p value <0.05 was defined as statistically significant. All statistical analyses were performed using R (version 4.3.2) and SPSS (version 27.0).
3 Results
3.1 Baseline characteristics
A total of 1,734 patients (mean age 59.8 ± 10.5 years, male 76.6%), 674 (38.9%) of whom had HHcy, were included in this analysis. Baseline characteristics of the study population stratified by HHcy were summarized in Table 1. In comparison to patients with HHcy, patients without HHcy tended to be female, have higher BMI and increased prevalence of diabetes mellitus (DM), but lower proportions of current smoking, HF, CKD, and previous MI. Additionally, patients without HHcy demonstrated higher HDL-C, glycated hemoglobin (HbA1c), and FBG levels, along with lower high-sensitivity C-reactive protein (hsCRP) and serum creatinine (Scr) levels. Moreover, patients without HHcy had lower syntax scores and received a higher proportion of aspirin treatment. TyG index values between groups showed no significant difference.
The optimal cut-off value of TyG index for MACE was 8.86 (Figure 5A). Baseline characteristics of the study population according to HHcy and the optimal cut-off value of TyG index were shown in Table 2. Regardless of HHcy, patients with high TyG were more likely to be younger, have lower BMI, a history of DM and lower HDL-C levels, but higher levels of TC, TG, LDL-C, HbA1c, FBG, and syntax scores. Additionally, in patients without HHcy, those with high TyG were more likely to be current smokers, have higher levels of hsCRP, and be more frequently treated with β-blockers.
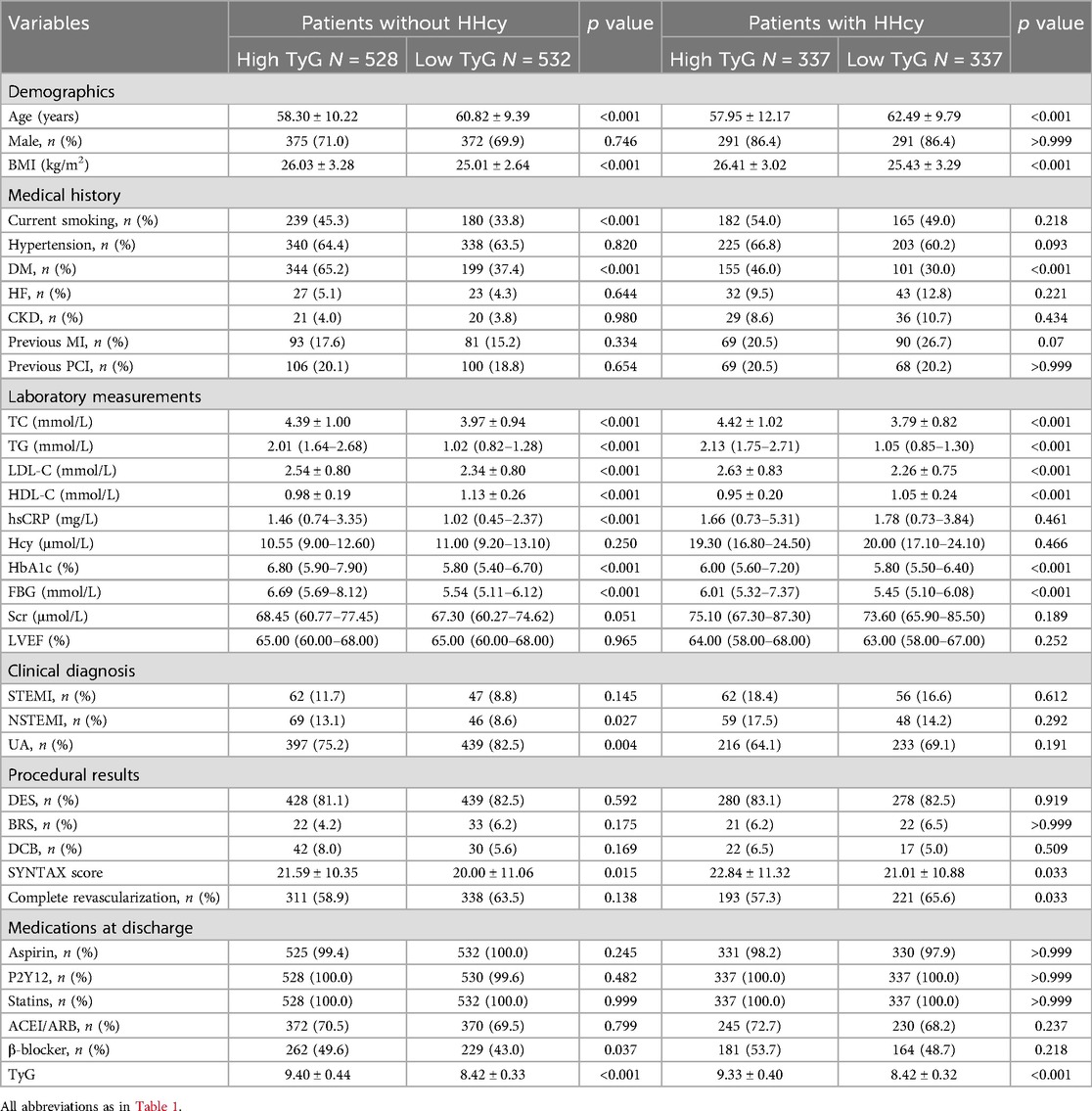
Table 2. Baseline characteristics of the study population according to HHcy and the optimal cut-off value of TyG index.
3.2 Correlation analysis between TyG and other variables
Figure 2 shows the correlation analysis between TyG and clinical risk factors. TyG showed a weak but significant positive correlation with BMI, current smoking, DM, TC, LDL-C, HbA1c, syntax score, and ACEI/ARB at discharge, while negatively correlating with age, HDL-C, and complete revascularization (all p < 0.05).
3.3 Prognostic value of TyG in patients with or without HHcy
Over a median follow-up of 927 days, 358 patients (20.6%) experienced MACE. Detailed information of MACE was described in Table 3. The Kaplan–Meier curves showed significant differences in cumulative incidence of MACE among prespecified groups (logrank p < 0.001, Figure 3). This difference was primarily driven by unplanned repeat revascularization (logrank p < 0.001, Figure 4). A significant interaction was observed between TyG and HHcy (p for interaction = 0.01). Multivariable Cox regression analysis revealed that higher TyG was significantly associated with an increased risk of MACE in patients without HHcy (Table 4) (Model 1: HR: 2.02, 95% CI: 1.62–2.47, p < 0.001; Model 2: HR: 2.18, 95% CI: 1.79–2.64, p < 0.001; Model 3: HR: 2.00, 95% CI: 1.67–2.47, p < 0.001; Model 4: HR: 2.39, 95% CI: 1.58–3.63, p < 0.001; Model 5: HR: 2.38, 95% CI: 1.55–3.65, p < 0.001; Model 6: HR: 2.36, 95% CI: 1.53–3.64, p < 0.001), but failed to demonstrate similar prognostic value in patients with HHcy (Table 4) (Model 1: HR: 1.28, 95% CI: 0.96–1.70, p = 0.016; Model 2: HR: 1.44, 95% CI: 1.08–1.93, p = 0.014; Model 3: HR: 1.37, 95% CI: 0.99–1.87, p = 0.052; Model 4: HR: 1.58, 95% CI: 0.73–3.42, p = 0.243; Model 5: HR: 1.27, 95% CI: 0.59–2.74, p = 0.542; Model 6: HR: 1.31, 95% CI: 0.60–2.87, p = 0.504). In the overall study population, Hcy was not associated with the risk of MACE (HR: 1.00, 95% CI: 0.99–1.02, p = 0.634).
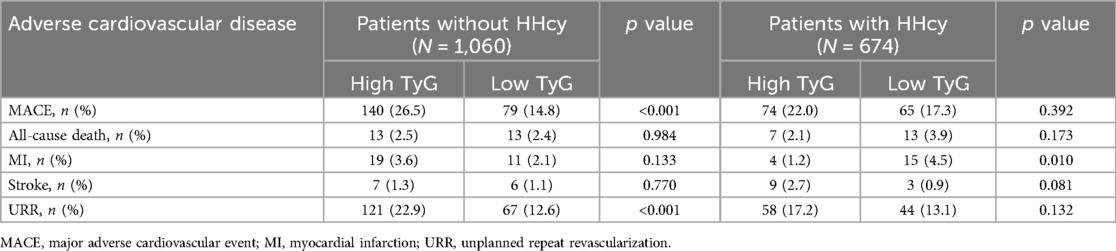
Table 3. Adverse cardiovascular events stratified by HHcy and the optimal cut-off value of TyG index.
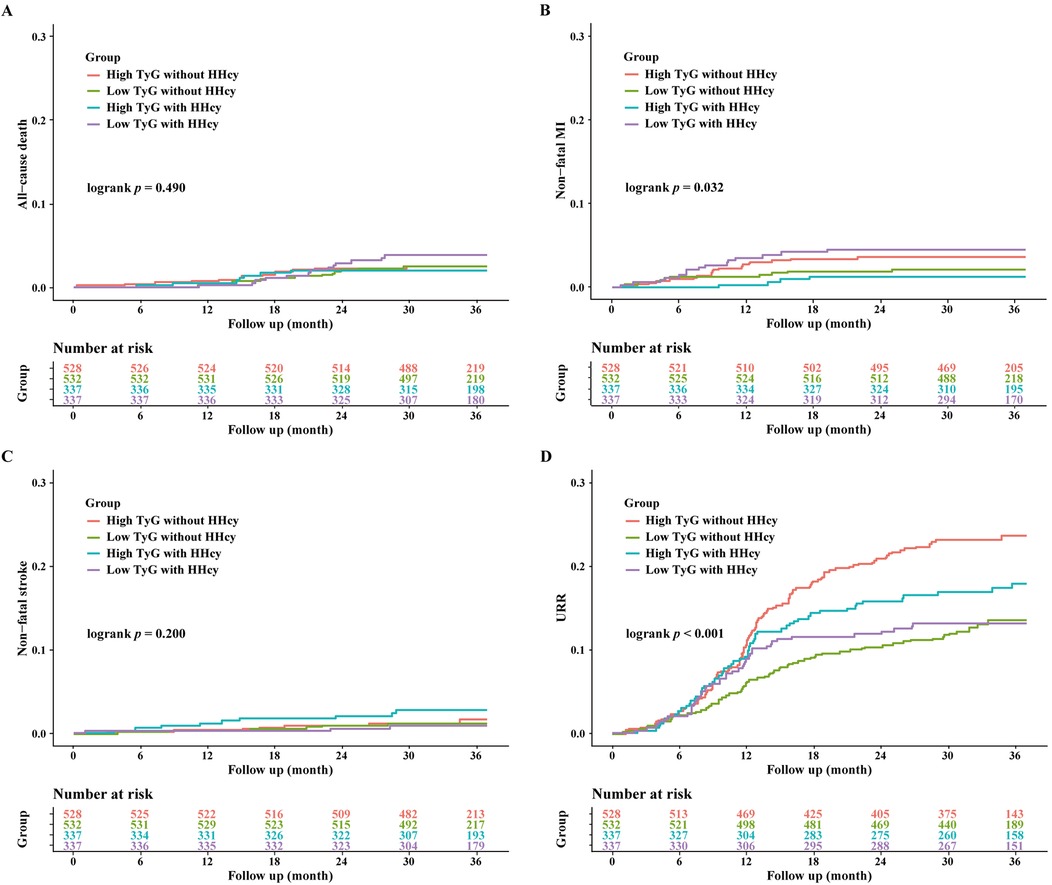
Figure 4. Kaplan-Meier curves for the secondary endpoint stratified by HHcy and TyG index. (A) Kaplan-Meier curves for all-cause death; (B) Kaplan-Meier curves for nonfatal MI; (C) Kaplan-Meier curves for nonfatal stroke; (D) Kaplan-Meier curves for URR.
RCS curves were used to visualize the linear association between TyG and MACE (Figure 5). In the overall ACS population, a linear association was observed between higher TyG and increased MACE risk (p for overall <0.001, p for nonlinear = 0.657). Among patients without HHcy, high TyG was linearly associated with an increased MACE risk (p for overall <0.001, p for nonlinear = 0.245). By contrast, among patients with HHcy, TyG was not associated with MACE risk (p for overall = 0.246).
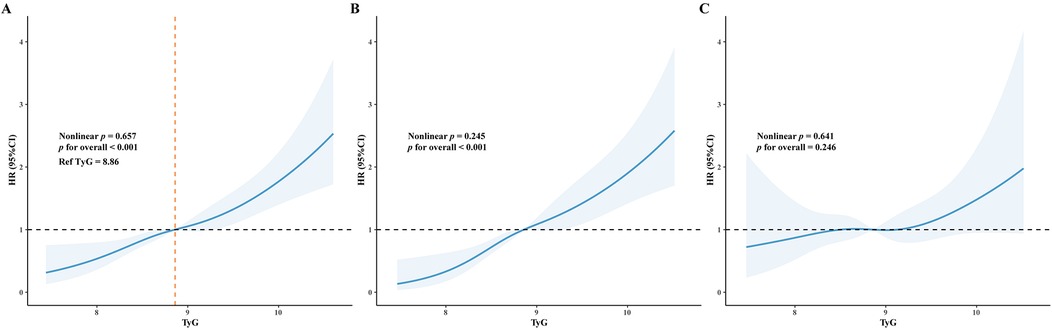
Figure 5. Restricted cubic splines (RCS) curve demonstrated the association between TyG index and MACE. The blue line represents the hazard ratio (HR), and the shaded area represents the 95% confidence interval (CI). (A) RCS curve for all patients; (B) RCS curve for patients without HHcy; (C) RCS curve for patients with HHcy.
3.4 Impact of HHcy on the prognostic value of TyG
Figure 6 presented the impact of HHcy on the prognostic value of TyG for MACE, all-cause death, nonfatal MI, nonfatal stroke, and unplanned repeat revascularization. A significant interaction between HHcy and TyG in predicting MACE was observed.
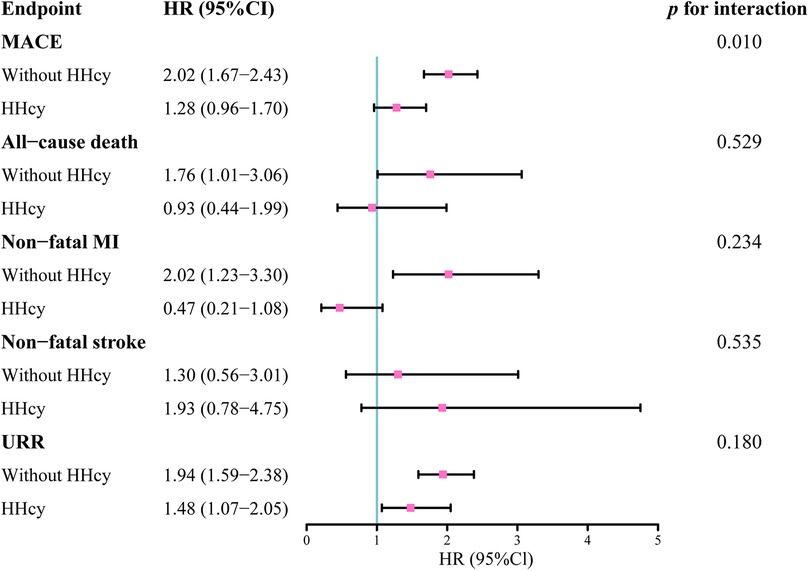
Figure 6. Hazard ratios of TyG index as a continuous variable on the endpoints in patients with and without HHcy.
4 Discussion
Based on our knowledge, the present study is the first to evaluate the prognostic value of TyG in patients with ACS undergoing PCI, and further investigate the interaction between TyG and HHcy. Our analysis yielded the following findings: (1) Regardless of continuous variable or categorical variable, TyG was independently associated with poorer prognosis in ACS patients without HHcy. (2) A linear relationship was observed between TyG and MACE. [3] The prognostic value of TyG was demonstrated only in patients without HHcy, but not in patients with HHcy. A significant interaction was observed between TyG and HHcy.
As early as 1969, McCully proposed the association between homocysteine and atherosclerosis (24). The vascular pathology and prognostic value of Hcy have been discussed for decades. Hcy contributes to increased blood coagulation and decreased fibrinolysis through synergistic mechanisms (25). Hcy also induces endothelial dysfunction by promoting oxidative stress, inflammation, elevated production of nitric oxide, hydrogen sulfide (H2S), adenosine, and oxidized low-density lipoprotein (LDL) (13, 14, 26–29). Furthermore, elevated Hcy levels lead to irreversible remodeling of the vessel wall, characterized by increased fibrosis, homocysteinylation of extracellular matrix proteins, and breaks in elastin (14, 15). Consequently, elevated Hcy is reasonably recognized as a risk factor for CVD. However, the effect of HHcy on cardiovascular disease remained controversial in clinic practice. Meta-analysis of observational studies indicated that elevated Hcy was associated with an increased risk of cardiovascular risk in healthy populations (20) and ACS patients (21). But it is worth noting that lowering Hcy levels through diet or medical therapy did not consistently reduce the risk of CVD (30). An early meta-analysis in 2010 showed that folic acid supplementation could reduce Hcy levels by 25%, yet it did not lower the risk of cardiovascular events and all-cause mortality (31). Another meta-analysis of 30 randomized controlled trials indicated a 10% lower risk of stroke and a 4% reduction in overall CVD risk by folic acid supplementation (32). Cochrane review in 2017 demonstrated that B-vitamins supplementation slightly reduced the risk of stroke in patients without end-stage renal disease, but did not reduce the risk of MI or all-cause mortality (33). Therefore, a deeper understanding of the relationship between HHcy and cardiovascular diseases is still required.
The association between IR and adverse cardiovascular outcomes has been widely acknowledged. While the hyperinsulinemic-euglycemic clamp (HEC) is regarded as the gold standard for IR assessment, its clinical utility is limited due to complexity, time consumption, expense, and the need for frequent blood tests. The TyG index provides high sensitivity and specificity in measuring insulin resistance, with the added advantages of convenience and cost-effectiveness, making it more suitable for clinical application (5–7). The prognostic value of TyG in ACS patients has been confirmed by previous studies (16, 19, 34–40). A meta-analysis including 13,684 ACS patients showed that the highest category of TyG was associated with twofold increased MACE risk compared to the lowest category (34). Liang SC et al. analyzed data of CAD patients from 41 studies and concluded that patients with higher TyG levels were at a higher risk of CAD, with more severe coronary artery lesions, and suffered poorer prognosis compared to those with lower TyG levels (19). A cohort study included 1,158 Chinese patients and reported that higher TyG was associated with worse prognosis in ACS patients with prior coronary artery bypass grafting undergoing PCI (16). Wang L et al. enrolled 2,531 DM patients undergoing coronary angiography for ACS and found that TyG could predict MACE risk in ACS patients with DM independently of known cardiovascular risk factors (35). Subgroup analyses in these studies generally incorporated clinical risk factors such as age, gender, hypertension, and diabetes, but never took the influence of HHcy into consideration. In our study, we found consistent results with previous evidence that higher TyG was associated with an increased risk of MACE. Moreover, serum Hcy levels showed no correlation with MACE risk. Importantly, a significant interaction between TyG and hcy was observed. TyG was only associated with increased MACE risk in ACS patients without HHcy, but not in ACS patients with HHcy. Therefore, we reasonably assumed that the prognostic value of TyG was modified by HHcy.
The underlying mechanisms behind these findings remain unclear and need further investigation. HHcy promotes insulin resistance through various synergistic mechanisms such as protein cysteine-homocysteinylation (9), MDM2-mediated ubiquitination of HSF1 (10), and modulation of M2 macrophage polarization via estrogen suppression (11). Additionally, supplementation with folic acid and vitamin B12 has been shown to improve insulin resistance in intrauterine growth retardation rats (41). Plasma homocysteine levels were also found to be independently associated with conventional atherogenic lipid profiles and remnant cholesterol in adults (8). These mechanisms may be relevant to the interaction between HHcy and TyG observed in our analysis.
In our study, the gender distribution differed between the HHcy and non-HHcy groups, with a relatively low proportion of females in the overall population. However, after adjusting for gender in the multivariable Cox regression analysis, the discrepancy in the prognostic value of the TyG index remained. To further elucidate potential sexual dimorphisms in the prognostic value of the TyG index, we analyzed and reported the baseline characteristics and prognostic value of TyG separately for males and females in both HHcy and non-HHcy groups (Supplementary Tables S1 and S2). Our findings suggest that, among patients with HHcy, gender appears to influence the prognostic value of the TyG index. However, possibly due to the limited sample size, we did not observe a significant interaction between TyG and gender (p for interaction = 0.069). Future studies should include a larger sample size and higher proportion of female participants to further validate and extend our conclusions.
The present study has several limitations. Firstly, this is a single-center, retrospective analysis of a prospective registry. Some confounding factors, such as unhealthy lifestyles and obstructive sleep apnea syndrome, could influence the results. Secondly, the analysis only relied on fasting TyG index and Hcy levels upon admission, without dynamic monitoring of TyG and Hcy. Thus, fluctuations in TyG and Hcy levels were not taken into consideration. Thirdly, the study did not document the prescription of homocysteine-lowering medications such as folic acid and vitamin B, which could potentially affect Hcy levels. Fourth, due to the lack of comparable studies evaluating the effect of HHcy on the prognostic value of TyG, a power analysis to determine the required sample size was waived. Given the substantial sample size of 1,734 patients, we believe our findings are acceptable and provide valuable insights into this area. Finally, the study population consisted entirely of Chinese, and external validation was not conducted. Therefore, the general applications of this finding should be made with caution.
5 Conclusion
The prognostic value of TyG was modified by HHcy in ACS patients undergoing PCI. Higher TyG was only associated with an increased risk of MACE in ACS patients without HHcy, but not in ACS patients with HHcy.
Data availability statement
The original contributions presented in the study are included in the article/Supplementary Material, further inquiries can be directed to the corresponding authors.
Ethics statement
The studies involving humans were approved by the institutional review board of Beijing Anzhen Hospital, Capital Medical University. The studies were conducted in accordance with the local legislation and institutional requirements. The human samples used in this study were acquired from a by- product of routine care or industry. Written informed consent for participation was not required from the participants or the participants' legal guardians/next of kin in accordance with the national legislation and institutional requirements.
Author contributions
YK: Conceptualization, Writing – original draft, Writing – review & editing. XM: Conceptualization, Writing – review & editing. ZZ: Investigation, Writing – original draft. SD: Software, Writing – original draft. YX: Data curation, Writing – original draft. YS: Methodology, Writing – original draft. YC: Supervision, Writing – original draft. DZ: Formal Analysis, Writing – original draft. YL: Project administration, Writing – review & editing. XL: Validation, Writing – review & editing. DS: Resources, Writing – review & editing. YZ: Funding acquisition, Visualization, Writing – review & editing.
Funding
The author(s) declare financial support was received for the research, authorship, and/or publication of this article. The study was reviewed and approved for funding by National Key Research and Development Program of China (2022YFC3602500) and Youth Program of National Natural Science Foundation of China (82200405).
Conflict of interest
The authors declare that the research was conducted in the absence of any commercial or financial relationships that could be construed as a potential conflict of interest.
Generative AI statement
The authors declare that no Generative AI was used in the creation of this manuscript.
Publisher's note
All claims expressed in this article are solely those of the authors and do not necessarily represent those of their affiliated organizations, or those of the publisher, the editors and the reviewers. Any product that may be evaluated in this article, or claim that may be made by its manufacturer, is not guaranteed or endorsed by the publisher.
Supplementary material
The Supplementary Material for this article can be found online at: https://www.frontiersin.org/articles/10.3389/fcvm.2024.1517437/full#supplementary-material
References
1. Mensah GA, Fuster V, Murray CJL, Roth GA. Global burden of cardiovascular diseases and risks, 1990–2022. J Am Coll Cardiol. (2023) 82(25):2350–473. doi: 10.1016/j.jacc.2023.11.007
2. Vaduganathan M, Mensah GA, Turco JV, Fuster V, Roth GA. The global burden of cardiovascular diseases and risk: a compass for future health. J Am Coll Cardiol. (2022) 80(25):2361–71. doi: 10.1016/j.jacc.2022.11.005
3. Szummer K, Jernberg T, Wallentin L. From early pharmacology to recent pharmacology interventions in acute coronary syndromes: jacc state-of-the-art review. J Am Coll Cardiol. (2019) 74(12):1618–36. doi: 10.1016/j.jacc.2019.03.531
4. American Diabetes Association. Consensus Development Conference on Insulin Resistance: 5–6 November 1997. Diabetes Care. (1998) 21(2):310–4. doi: 10.2337/diacare.21.2.310
5. Vasques AC, Novaes FS, de Oliveira Mda S, Souza JR, Yamanaka A, Pareja JC, et al. Tyg index performs better than homa in a Brazilian population: a hyperglycemic clamp validated study. Diabetes Res Clin Pract. (2011) 93(3):e98–e100. doi: 10.1016/j.diabres.2011.05.030
6. Guerrero-Romero F, Simental-Mendía LE, González-Ortiz M, Martínez-Abundis E, Ramos-Zavala MG, Hernández-González SO, et al. The product of triglycerides and glucose, a simple measure of insulin sensitivity. Comparison with the euglycemic-hyperinsulinemic clamp. J Clin Endocrinol Metab. (2010) 95(7):3347–51. doi: 10.1210/jc.2010-0288
7. Guerrero-Romero F, Villalobos-Molina R, Jiménez-Flores JR, Simental-Mendia LE, Méndez-Cruz R, Murguía-Romero M, et al. Fasting triglycerides and glucose index as a diagnostic test for insulin resistance in young adults. Arch Med Res. (2016) 47(5):382–7. doi: 10.1016/j.arcmed.2016.08.012
8. Zhou L, Liu J, An Y, Wang Y, Wang G. Plasma homocysteine level is independently associated with conventional atherogenic lipid profile and remnant cholesterol in adults. Front Cardiovasc Med. (2022) 9:898305. doi: 10.3389/fcvm.2022.898305
9. Zhang X, Qu YY, Liu L, Qiao YN, Geng HR, Lin Y, et al. Homocysteine inhibits pro-insulin receptor cleavage and causes insulin resistance via protein cysteine-homocysteinylation. Cell Rep. (2021) 37(2):109821. doi: 10.1016/j.celrep.2021.109821
10. Xiang W, Yang Y, Weng L, Ye Z, Ding P, Li H, et al. Hyperhomocysteinemia activates Nlrp3 inflammasome to cause hepatic steatosis and insulin resistance via Mdm2-mediated ubiquitination of Hsf1. Int Immunopharmacol. (2023) 118:110085. doi: 10.1016/j.intimp.2023.110085
11. Qi X, Zhang B, Zhao Y, Li R, Chang HM, Pang Y, et al. Hyperhomocysteinemia promotes insulin resistance and adipose tissue inflammation in pcos mice through modulating M2 macrophage polarization via estrogen suppression. Endocrinology. (2017) 158(5):1181–93. doi: 10.1210/en.2017-00039
12. Jakubowski H. Homocysteine modification in protein structure/function and human disease. Physiol Rev. (2019) 99(1):555–604. doi: 10.1152/physrev.00003.2018
13. Esse R, Barroso M, Tavares de Almeida I, Castro R. The contribution of homocysteine metabolism disruption to endothelial dysfunction: state-of-the-art. Int J Mol Sci. (2019) 20(4):867. doi: 10.3390/ijms20040867
14. Balint B, Jepchumba VK, Guéant JL, Guéant-Rodriguez RM. Mechanisms of homocysteine-induced damage to the endothelial, medial and adventitial layers of the arterial wall. Biochimie. (2020) 173:100–6. doi: 10.1016/j.biochi.2020.02.012
15. Balint B, Hergalant S, Camadro JM, Blaise S, Vanalderwiert L, Lignières L, et al. Fetal programming by methyl donor deficiency produces pathological remodeling of the ascending aorta. Arterioscler, Thromb, Vasc Biol. (2021) 41(6):1928–41. doi: 10.1161/atvbaha.120.315587
16. Dong S, Zhao Z, Huang X, Ma M, Yang Z, Fan C, et al. Triglyceride-glucose index is associated with poor prognosis in acute coronary syndrome patients with prior coronary artery bypass grafting undergoing percutaneous coronary intervention. Cardiovasc Diabetol. (2023) 22(1):286. doi: 10.1186/s12933-023-02029-6
17. Ding X, Wang X, Wu J, Zhang M, Cui M. Triglyceride-glucose index and the incidence of atherosclerotic cardiovascular diseases: a meta-analysis of cohort studies. Cardiovasc Diabetol. (2021) 20(1):76. doi: 10.1186/s12933-021-01268-9
18. Liu X, Tan Z, Huang Y, Zhao H, Liu M, Yu P, et al. Relationship between the triglyceride-glucose index and risk of cardiovascular diseases and mortality in the general population: a systematic review and meta-analysis. Cardiovasc Diabetol. (2022) 21(1):124. doi: 10.1186/s12933-022-01546-0
19. Liang S, Wang C, Zhang J, Liu Z, Bai Y, Chen Z, et al. Triglyceride-glucose index and coronary artery disease: a systematic review and meta-analysis of risk, severity, and prognosis. Cardiovasc Diabetol. (2023) 22(1):170. doi: 10.1186/s12933-023-01906-4
20. Homocysteine and. Risk of ischemic heart disease and stroke: a meta-analysis. JAMA. (2002) 288(16):2015–22. doi: 10.1001/jama.288.16.2015
21. Zhu M, Mao M, Lou X. Elevated homocysteine level and prognosis in patients with acute coronary syndrome: a meta-analysis. Biomarkers. (2019) 24(4):309–16. doi: 10.1080/1354750x.2019.1589577
22. O'Gara PT, Kushner FG, Ascheim DD, Casey DE Jr, Chung MK, de Lemos JA, et al. 2013 Accf/Aha guideline for the management of st-elevation myocardial infarction: a report of the American College of Cardiology Foundation/American Heart Association task force on practice guidelines. Circulation. (2013) 127(4):e362–425. doi: 10.1161/CIR.0b013e3182742cf6
23. Amsterdam EA, Wenger NK, Brindis RG, Casey DE Jr, Ganiats TG, Holmes DR Jr, et al. 2014 Aha/Acc guideline for the management of patients with non-st-elevation acute coronary syndromes: a report of the American College of Cardiology/American Heart Association task force on practice guidelines. J Am Coll Cardiol. (2014) 64(24):e139–228. doi: 10.1016/j.jacc.2014.09.017
24. McCully KS. Vascular pathology of homocysteinemia: implications for the pathogenesis of arteriosclerosis. Am J Pathol. (1969) 56(1):111–28.5792556
25. Undas A, Brozek J, Szczeklik A. Homocysteine and thrombosis: from basic science to clinical evidence. Thromb Haemostasis. (2005) 94(5):907–15. doi: 10.1160/th05-05-0313
26. Wu X, Zhang L, Miao Y, Yang J, Wang X, Wang CC, et al. Homocysteine causes vascular endothelial dysfunction by disrupting endoplasmic reticulum redox homeostasis. Redox Biol. (2019) 20:46–59. doi: 10.1016/j.redox.2018.09.021
27. Zhao Z, Liu X, Shi S, Li H, Gao F, Zhong X, et al. Exogenous hydrogen sulfide protects from endothelial cell damage, platelet activation, and neutrophils extracellular traps formation in hyperhomocysteinemia rats. Exp Cell Res. (2018) 370(2):434–43. doi: 10.1016/j.yexcr.2018.07.007
28. Paganelli F, Mottola G, Fromonot J, Marlinge M, Deharo P, Guieu R, et al. Hyperhomocysteinemia and cardiovascular disease: is the adenosinergic system the missing link? Int J Mol Sci. (2021) 22(4):1690. doi: 10.3390/ijms22041690
29. Perła-Kaján J, Jakubowski H. Dysregulation of epigenetic mechanisms of gene expression in the pathologies of hyperhomocysteinemia. Int J Mol Sci. (2019) 20(13):3140. doi: 10.3390/ijms20133140
30. Guéant JL, Guéant-Rodriguez RM, Oussalah A, Zuily S, Rosenberg I. Hyperhomocysteinemia in cardiovascular diseases: revisiting observational studies and clinical trials. Thromb Haemostasis. (2023) 123(3):270–82. doi: 10.1055/a-1952-1946
31. Clarke R, Halsey J, Lewington S, Lonn E, Armitage J, Manson JE, et al. Effects of lowering homocysteine levels with B vitamins on cardiovascular disease, cancer, and cause-specific mortality: meta-analysis of 8 randomized trials involving 37 485 individuals. Arch Intern Med. (2010) 170(18):1622–31. doi: 10.1001/archinternmed.2010.348
32. Li Y, Huang T, Zheng Y, Muka T, Troup J, Hu FB. Folic acid supplementation and the risk of cardiovascular diseases: a meta-analysis of randomized controlled trials. J Am Heart Assoc. (2016) 5(8):e003786. doi: 10.1161/jaha.116.003768
33. Martí-Carvajal AJ, Solà I, Lathyris D, Dayer M. Homocysteine-lowering interventions for preventing cardiovascular events. Cochrane Database Syst Rev. (2017) 8(8):Cd006612. doi: 10.1002/14651858.CD006612.pub5
34. Akbar MR, Pranata R, Wibowo A, Irvan ST, Martha JW. The association between triglyceride-glucose index and major adverse cardiovascular events in patients with acute coronary syndrome—dose-response meta-analysis. Nutr Metab Cardiovasc Dis. (2021) 31(11):3024–30. doi: 10.1016/j.numecd.2021.08.026
35. Wang L, Cong HL, Zhang JX, Hu YC, Wei A, Zhang YY, et al. Triglyceride-glucose index predicts adverse cardiovascular events in patients with diabetes and acute coronary syndrome. Cardiovasc Diabetol. (2020) 19(1):80. doi: 10.1186/s12933-020-01054-z
36. Zhu Y, Liu K, Chen M, Liu Y, Gao A, Hu C, et al. Triglyceride-glucose index is associated with in-stent restenosis in patients with acute coronary syndrome after percutaneous coronary intervention with drug-eluting stents. Cardiovasc Diabetol. (2021) 20(1):137. doi: 10.1186/s12933-021-01332-4
37. Zhao Q, Cheng YJ, Xu YK, Zhao ZW, Liu C, Sun TN, et al. Comparison of various insulin resistance surrogates on prognostic prediction and stratification following percutaneous coronary intervention in patients with and without type 2 diabetes mellitus. Cardiovasc Diabetol. (2021) 20(1):190. doi: 10.1186/s12933-021-01383-7
38. Hu C, Zhang J, Liu J, Liu Y, Gao A, Zhu Y, et al. Discordance between the triglyceride glucose index and fasting plasma glucose or Hba1c in patients with acute coronary syndrome undergoing percutaneous coronary intervention predicts cardiovascular events: a cohort study from China. Cardiovasc Diabetol. (2020) 19(1):116. doi: 10.1186/s12933-020-01091-8
39. Ma X, Dong L, Shao Q, Cheng Y, Lv S, Sun Y, et al. Triglyceride glucose index for predicting cardiovascular outcomes after percutaneous coronary intervention in patients with type 2 diabetes mellitus and acute coronary syndrome. Cardiovasc Diabetol. (2020) 19(1):31. doi: 10.1186/s12933-020-01006-7
40. Zhang Y, Chu C, Zhong Z, Luo YB, Ning FF, Guo N. High triglyceride-glucose index is associated with poor cardiovascular outcomes in Chinese acute coronary syndrome patients without diabetes mellitus who underwent emergency percutaneous coronary intervention with drug-eluting stents. Front Endocrinol (Lausanne). (2023) 14:1101952. doi: 10.3389/fendo.2023.1101952
Keywords: hyperhomocysteinemia, triglyceride glucose index, acute coronary syndrome, percutaneous coronary intervention, major adverse cardiovascular events
Citation: Kan Y, Ma X, Zhao Z, Dong S, Xu Y, Sun Y, Cheng Y, Zhang D, Liu Y, Liu X, Shi D and Zhou Y (2025) Effect of hyperhomocysteinemia on the prognostic value of triglyceride glucose index in patients with acute coronary syndrome. Front. Cardiovasc. Med. 11:1517437. doi: 10.3389/fcvm.2024.1517437
Received: 26 October 2024; Accepted: 30 December 2024;
Published: 10 January 2025.
Edited by:
Venkatesh Sundararajan, West Virginia University, United StatesReviewed by:
Brindha Jaya Chandran, Center for Discovery and Innovation, Hackensack Meridian Health, United StatesSaranya Kannan, Texas A&M Health Science Center, United States
Copyright: © 2025 Kan, Ma, Zhao, Dong, Xu, Sun, Cheng, Zhang, Liu, Liu, Shi and Zhou. This is an open-access article distributed under the terms of the Creative Commons Attribution License (CC BY). The use, distribution or reproduction in other forums is permitted, provided the original author(s) and the copyright owner(s) are credited and that the original publication in this journal is cited, in accordance with accepted academic practice. No use, distribution or reproduction is permitted which does not comply with these terms.
*Correspondence: Xiaoteng Ma, bWF4aWFvdGVuZ2FpQDE2My5jb20=; Yujie Zhou, YXp6eWoxMkAxNjMuY29t