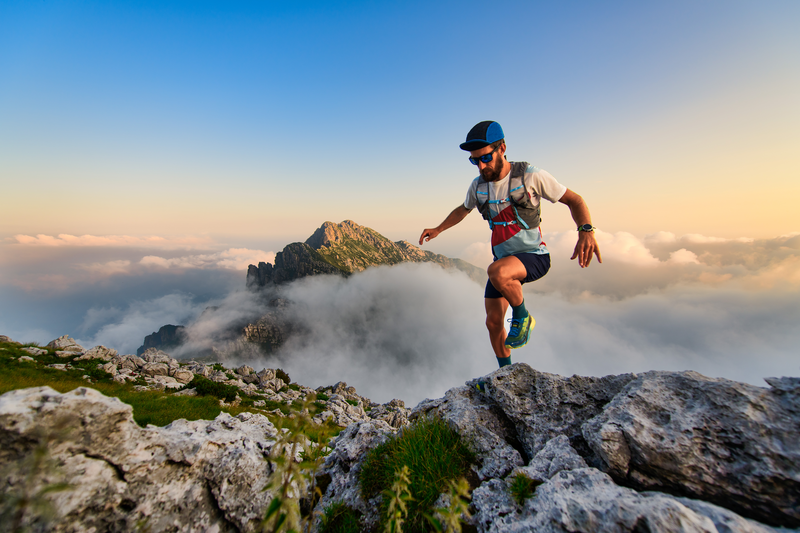
95% of researchers rate our articles as excellent or good
Learn more about the work of our research integrity team to safeguard the quality of each article we publish.
Find out more
REVIEW article
Front. Cardiovasc. Med. , 14 January 2025
Sec. Cardiovascular Imaging
Volume 11 - 2024 | https://doi.org/10.3389/fcvm.2024.1504063
This article is part of the Research Topic Revolutionizing Cardiovascular Diagnosis: Advances in Functional Imaging Technologies View all 8 articles
Valvular heart disease (VHD) leading to inadequate hemodynamic circulation is a major cause of cardiovascular morbidity and mortality worldwide. Right ventricular-pulmonary artery (RV–PA) coupling integrates the ability of RV contractility to adapt to increased pulmonary arterial afterload. If the right ventricle cannot adapt to the elevated afterload by increasing its contractile function, RV-PA uncoupling occurs. RV-PA uncoupling has been shown to be associated with poor outcomes in VHD. This review summarizes the prognostic significance of RV-PA coupling in patients with VHD.
Valvular heart disease (VHD), including congenital and acquired valvular diseases, is a major cause of cardiovascular morbidity and mortality worldwide, affecting more than 2% of the population (1). Previous studies have demonstrated the impact of right ventricular (RV) dysfunction on the poor prognosis in patients with VHD (2, 3). In recent years, right ventricular-pulmonary artery (RV-PA) uncoupling has emerged as a strong predictor of poor prognosis in VHD (4–6). RV-PA coupling integrates the ability of the right ventricle to maintain adequate output in the face of increased pulmonary arterial afterload. When the RV-PA coupling is normal, the right ventricle can adapt to an elevated afterload by increasing its contractile function, and the ratio may remain within the normal range (7–9). However, with prolonged elevation of RV afterload, RV remodeling occurs, leading to a progressive impairment of RV systolic function (8–10). This inadequacy compromises the maintenance of normal cardiac output, leading to RV uncoupling from the PA and ultimately to RV dysfunction and RV failure (11, 12). Moreover, RV-PA uncoupling indicates an advanced stage of disease progression (5, 13). Therefore, identification of such high-risk patients in clinical practice is of paramount importance for clinical decision making. With the increasing focus on the measurement of RV-PA coupling in VHD patients, a growing number of studies have demonstrated its strong impact on prognosis. In this review, we focus on the prognostic significance of RV-PA coupling in patients with VHD.
RV-PA coupling has gained more attention in various clinical practice, such as heart failure, pulmonary hypertension, VHD and congenital heart defect (CHD) (14–16). RV-PA coupling can be assessed by invasive and noninvasive metrics, and the gold standard for evaluating RV-PA coupling is the ratio of single-beat and multi-beat RV end-systolic elastance (Ees) to PA elastance (Ea) derived from invasive pressure-volume loops by right heart catheterization(RHC) (7, 9, 17–19). Measurement of Ees/Ea is shown in Figure 1. An Ees/Ea ratio between 1.5 and 2.0 is considered to be normal range according to previous studies (20), indicating that RV contractility adapts to the afterload with the highest efficiency (21, 22). Some of the studies use Ees/Ea to represent RV-PA coupling, and the most favorable RV-PA coupling is acquired when Ees/Ea = 1 (23). This method is considered to be more sensitive and accurate, however, it is difficult to apply conventionally due to its high cost, technical skill requirements and critical conducting condition (24). Additionally, researchers have found that Ees and Ea may be abnormal, but the ratio remains within the normal range. Thus, non-invasive alternative indicators have been introduced to meet the demand for clinical use.
Figure 1. Invasive measurement of RV-PA coupling derived from RHC. RHC, right heart catheterization; Ees, end-systolic elastance; Ea, elastance; EDPVR, end systole pulmonary vascular resistance.
Cardiac magnetic resonance (CMR) imaging has been considered the gold standard for the non-invasive assessment of RV function and RV-PA coupling (25). The ratio of CMR-derived RV end-systolic volume (ESV) to stroke volume (SV) has been shown to correlate with cardiac catheterization-derived RV-PA coupling (26).
Currently, the most commonly used echocardiographic surrogate in daily practice is the ratio of tricuspid annular plane systolic excursion (TAPSE) obtained from M-mode imaging in the apical RV-focused four-chamber view to pulmonary arterial systolic pressure (PASP) derived from RV systolic pressure (RVSP) (9, 10, 19, 27). PASP is calculated from peak tricuspid regurgitant velocity using the Bernoulli equation summing right atrial pressure. TAPSE and PASP represent RV contraction and RV afterload, separately. However, TAPSE tends to decrease after cardiac surgery even when overall RV function remains normal (28), which may underestimate RV-PA coupling.
Novel methods to account for RV-PA coupling, such as three-dimensional echocardiography (3DE) and RV strain, are gaining increasing attention. Acquisition of ESV/SV by 3DE using offline software has been shown to be associated with invasively measured RV-PA coupling from cardiac catheterization in patients with pulmonary hypertension (29). 3DE can be performed postoperatively to avoid assessment of TAPSE and RV geometric hypothesis. The ratio of RV free wall longitudinal strain (RVFWLS) obtained by speckle-tracking echocardiography (STE) to PASP has also been used in clinical practice (30), and has shown its potential value in pulmonary hypertension patients compared with conventional echocardiographic parameters (9). Apart from the above-mentioned indices, the ratios between fractional area change (FAC), right ventricular ejection fraction (RVEF) or tricuspid annular peak systolic velocity (S′) measured by tissue Doppler imaging and PASP or RVSP are less commonly used (13, 31). Non-invasive measurement of RV function and RV-PA coupling parameters are shown in Figure 2.
Figure 2. Non-invasive measurement of RV function and RV-PA coupling parameters derived from echocardiography in a patient with HFpEF. (A) RVFAC; (B) TAPSE; (C) RVFWLS; (D) S′; (E) 3D-TTE derived RV ESV, RV SV, RVEF; (F) Tricuspid regurgitation spectrum. HFpEF, heart failure with preserved ejection fraction; RVFAC, right ventricular fractional area change; TAPSE, tricuspid annular plane systolic excursion; RVFWLS, right ventricular free wall longitudinal strain; S′, tricuspid annular systolic velocity; ESV, end systolic volume; SV, stroke volume; RVEF, right ventricular ejection fraction. Yellow arrows represent measurements for parameters.
Acquired valvular diseases refer to situations that develop after birth due to non-congenital factors. These diseases can result from a range of causes, including degenerative processes, infections, inflammatory conditions, and other acquired heart diseases (32). These acquired valvular diseases can significantly impact cardiac function and require medical or surgical intervention, including the use of medications, valve repair, or valve replacement (33). The management of these conditions often involves a multidisciplinary approach, taking into account the patient's overall health and the specific valve involved. In this review, we choose three main categories that have been discussed more often to represent acquired valvular diseases.
Severe aortic stenosis (AS) results in inadequate output, leading to left ventricular (LV) remodeling, heart failure (HF) or even death (34). In patients with severe AS, reduced LV output leads to chronic pressure overload in the LV chambers, retrograde transmission of the pressure through the pulmonary vasculature, and results in compensatory RV and pulmonary vasculature remodeling, RV dilatation or RV dysfunction (35, 36). A previous study has also revealed that RV dysfunction is common in AS patients due to RV-LV interdependence (37). Both RV dysfunction and pulmonary hypertension have been shown to be associated with worse outcomes in patients undergoing aortic valve implantation (38, 39), confirming the fundamental role of preoperative assessment of RV function. RV-PA coupling as a novel marker of RV function and pulmonary hypertension has demonstrated its prognostic value in postoperative patients.
Transcatheter aortic valve replacement (TAVR) is a less invasive approach for patients with severe AS requiring intervention, associated with a milder recovery and shorter hospital stay (40, 41). However, mortality varies from 19.3%–67.8% during 2–5 years of postoperative follow-up, requiring sensitive and detailed assessment of the factors associated with mortality and outcome (42, 43). Thus, the predictive value of baseline RV-PA coupling in TAVR candidates with severe symptomatic AS has gained significant interest. The impact of RV-PA coupling after TAVR is shown in Table 1.
Sultan et al. (5) performed a retrospective analysis of 457 patients undergoing TAVR procedure. Based on the TAPSE/PASP ratio, patients were divided into quartiles. The baseline TAPSE/PASP ratio had a linear relationship with 2-year all-cause mortality in TAVR recipients. After multivariable adjustment, RV-PA coupling remained independently associated with outcomes. They concluded that RV-PA coupling has a dose-response relationship with survival and that pre-TAVR TAPSE/PASP evaluation should be incorporated into the shared decision-making process as an integrative approach. Similarly, in a 10-year follow-up study of 56 TAVR candidates with HF and AS, Vizzardi et al. (45) found that RV-PA coupling was a better predictor for risk stratification. They emphasized the role of comprehensive assessment of deformation and RV-PA coupling in predicting long-term outcomes in TAVR candidates.
Recent studies have found that pre-TAVR RV-PA coupling is predictive of cardiovascular death. Meucci et al. (36) studied 900 patients who underwent TAVR at 2 tertiary centers to evaluate the prognostic impact of RV-PA coupling and its association with all-cause mortality. RV-PA coupling was assessed by TAPSE/PASP. More than half of the patients had RV-PA uncoupling before TAVR (58%; severe in 15%). After TAVR, 45% of patients had RV-PA uncoupling and 10% had severe uncoupling. Among all 407 patients with post-TAVR uncoupling, 85% (347) patients had persistent uncoupling, while 15% had new-onset uncoupling. They found that either new-onset or persistent post-TAVR RV-PA uncoupling was associated with higher mortality at a median follow-up of 40 months. In contrast, there was no significant difference in survival between normal pre-TAVR RV-PA coupling and uncoupling. Notably, patients with “recovered coupling” tended to have better outcomes. The reason why the indicator of RV-PA coupling elevated possibly attributed to favorable unloading effects of TAVR on the LV and ultimately a reduction of PASP (46, 47, 51). Parasca et al. (47) used the RVFWLS/PASP ratio as an estimate of RV-PA coupling, and normal coupling was defined as RVFWLS/PASP ≥0.63. 160 patients with severe AS who underwent TTE examinations including STE myocardial deformation analysis of RV function before and one month after TAVR were enrolled (47). They found that the RVFWLS/PASP ratio was a novel non-invasive metric of RV-PA coupling and a predictor of outcome in patients with severe AS undergoing TAVR (47).
Most of the aforementioned investigators emphasized the role of comprehensive assessment of deformation and RV-PA coupling in predicting long-term outcomes in TAVR candidates. Thus, measurement of RV-PA coupling before and after TAVR is helpful in determining appropriate clinical strategies and risk stratification.
Surgical aortic valve replacement (SAVR) is more commonly used in patients younger than 75 years with low risk (the Society of Thoracic Surgeons predicted risk of mortality score or the European System for Cardiac Operative Risk Evaluation II < 4%) for surgical replacement (33). Compared to TAVR, there are fewer studies focusing on the relationship between RV-PA coupling and SAVR. Cahill et al. (6) conducted a study of 570 patients, 301 underwent TAVR, and 269 underwent SAVR in the PARTNER (Placement of Aortic Transcatheter Valve) 3 trial. Baseline RV-PA uncoupling was defined by TAPSE/PASP, and patients were stratified as “normal” with a ratio greater than 0.55 mm/mmHg, and “uncoupling” with a ratio less than 0.55 mm/mmHg. After 2-year follow-up, RV-PA uncoupling patients had an increased incidence of the endpoint, indicating that it was associated with higher risk of worse outcomes. What's more, RV-PA coupling decreased in patients who underwent SAVR at the early stage, and was sustained for 2 years (6). Another study also found that the TAPSP/PASP ratio was significantly reduced at 30 days and 1 year after SAVR (50). They concluded that reduction in RV-PA coupling is an independent predictor of increased risk of cardiac mortality at 5 years following SAVR (50). Hence, RV-PA coupling may be helpful in guiding treatment in patients undergoing SAVR.
Moderate to severe TR can cause right heart failure (52, 53), and is associated with increased morbidity and mortality (54–56). Secondary TR is more common than primary TR, and is significantly associated with left-sided valvular disease and LV dysfunction (57–59). In clinical practice, TR interventions are usually initiated too late due to other comorbidities (60, 61), and irreversible RV dilatation and even RV failure may occur (62). In a study of 180 patients with moderate or severe secondary TR, researchers found that RV-PA coupling was associated with mortality and hospitalization for HF (30). RV-PA coupling was measured by 3DE using RV forward SV to ESV to avoid underestimation of PASP. The findings showed that RV forward SV/ESV < 0.40 was strongly associated with higher rates of all-cause mortality and hospitalization for HF, and RV-PA coupling could be considered as a marker to detect RV dysfunction and to predict outcomes before conventional RV function parameters fail (30). Fortuni et al. (63) performed a study of 1,149 patients with secondary TR using TAPSE/PASP to estimate RV-PA coupling. Of these, 41% had RV-PA uncoupling with a TAPSE/PASP ratio less than 0.31 mm/mmHg, and during follow-up, these patients tended to have a higher risk of HF syndromes and higher mortality (63). Thus, they found that RV-PA coupling could be used to improve risk stratification in patients with TR.
In patients with severe TR, surgical treatment carries non-negligible risks and morbidity, therefore, transcatheter tricuspid valve replacement (TTVR) has been developed as an alternative treatment option. TTVR has been shown to be feasible with improved outcomes (64–66). After TTVR, regurgitant blood flowing through the tricuspid valve into the right atrium was reduced and additional afterload was introduced, requiring the right ventricle to contract to compensate for the increased afterload burden (67). Thus, evaluation of RV-PA coupling to predict outcome is an ideal method. Recent studies investigating the predictive value of RV-PA coupling in patients undergoing TTVR are shown in Table 2.
In 444 patients undergoing TTVR, the degree of TR reduction in TTVR was independently associated with a reduction in post-TTVR RV-PA coupling. Multivariable Cox regression analysis showed that a higher TAPSE/PASP ratio was associated with a lower risk of all-cause mortality (68), confirming RV-PA coupling as a powerful marker for predicting all-cause mortality and helping to select patients suitable for TTVR (68). In a study of 206 patients undergoing TTVR at high surgical risk, the RV-PA coupling ratio was calculated by TAPSE/PASP at baseline (70). PASP was measured echocardiographically or invasively and referred to as ePASP and iPASP respectively. Patients with a lower TAPSE/PASP ratio tended to have a higher risk of death or HF rehospitalization within one year. Additionally, they demonstrated that TAPSE/iPASP (c-statistic 0.695) had a greater predictive value for outcomes compared with TAPSE/ePASP (c-statistic 0.565) (70). Stolz et al. (69) conducted a study of 502 patients who underwent tricuspid valve edge-to-edge repair (TEER) for severe TR. Patients with RV-PA uncoupling had a lower survival rate at 1- and 2-year follow-up, and TAPSE/iPASP was better in predicting mortality (69). In another multicenter study of 737 patients undergoing TTVR for severe TR, RV-PA coupling was defined as the ratio of TAPSE to mean pulmonary artery pressure (mPAP) measured invasively by right heart catheterization (67). In addition, they used an extreme gradient boosting (XGB) algorithm to predict mPAP levels after training on 10 echocardiographic parameters, which could refine risk stratification before TTVR without invasive surgery, and provided the ability to calculate mPAP levels using echocardiographic input parameters (67). Moreover, by multivariate regression analysis, they found that predicted TAPSE/mPAP levels were independently associated with 2-year mortality after TTVR (67). In the end, they revealed that patients with preserved predicted RV-PA coupling, defined by TAPSE/mPAP values greater than 0.617 mm/mmHg, had significantly better survival than patients with reduced RV-PA coupling, and XGB may help to develop treatment strategies to improve survival outcomes in patients with reduced RV-PA coupling (67). Thus, RV-PA coupling plays a key role in risk stratification and patient selection for TTVR.
Mitral regurgitation (MR) has the highest incidence of valvular heart disease worldwide (33, 71). Untreated moderate to severe MR is responsible for the progressive LV dilatation and dysfunction, leading to reduced quality of life, high rates of morbidity, mortality, and HF hospitalization (72). Depending on the pathogenesis, MR can be of primary or secondary (PMR/SMR) (73, 74). Conventional surgical approach used to be a feasible therapy for patients with symptomatic MR. However, since SMR is often associated with dilated left ventricle with markedly reduced ejection fraction (33), less invasive therapeutic options have emerged as a preferred alternative to surgery (75). Transcatheter mitral valve repair (TMVR) using the MitraClip and the PASCAL edge-to-edge devices is classified in recent guidelines as a treatment that should be considered to improve outcomes in patients with PMR and SMR, especially those at high surgical risk (76, 77). Owing to the improvement in prognosis and quality of life, mitral valve TEER has been performed more frequently in clinical practice (33, 76). Therefore, predicting the outcome after TEER seemed to be more important than ever. In recent years, RV-PA coupling has been proposed as a novel parameter for risk stratification in the TEER population. The relevant studies are listed in Table 3.
Recent evidence demonstrates that improvement in RV-PA coupling after TEER is associated with favorable outcomes. In a study of 156 patients with PMR or SMR undergoing TEER, impaired baseline RV–PA coupling was present in 64% of individuals with a TAPSE/PASP ratio <0.36 (84). Patients with reduced RV-PA coupling had a strong association with HF hospitalization and death, as well as a high risk of non-cardiac conditions such as renal dysfunction (84). Using multivariable Cox regression analyses, Koschutnik et al. (84) found that a TAPSE/PASP ratio <0.36 was independently associated with outcomes. Additionally, the authors found that after TEER, RV remodeling and RV-PA coupling may be reversed to some extent in individuals with impaired RV-PA coupling at baseline, which may provide additional prognostic information for poor outcomes (84). A similar message was delivered by Adamo et al., who elegantly demonstrated that SMR patients with lower baseline TAPSE, higher baseline PASP and postprocedural mitral gradient ≥2+ were more likely to improve their TAPSE/PASP ratio after TEER, and may be associated with lower mortality compared with nonresponders with little change in TAPSE/PASP (82). In addition, several studies have focused on the prognostic value of RV-PA coupling, and emphasizing that higher TAPSE/PASP is associated with a favorable post-procedural outcome. Shechter et al. demonstrated that TAPSE/PASP <0.37 mm/mmHg at baseline was associated with worse preprocedural status and immediate postprocedural outcome (83). Thus, a low TAPSE/PASP ratio was strongly associated with higher post-TEER mortality and HF hospitalization in SMR patients (83). In another series of 165 SMR patients with the same TAPSE/PASP cut-off who underwent MitraClip implantation, a baseline RV–PA uncoupling was associated with a higher rate of all-cause mortality (15). Brener et al. (80) performed their subanalysis of the COAPT (Cardiovascular Outcomes Assessment of the MitraClip Percutaneous Therapy for Heart Failure Patients With Functional Mitral Regurgitation) trial using the RVFWLS/PASP ratio, which also demonstrated the prognostic utility of RV-PA coupling. In conclusion, RV-PA coupling may provide further insight into strategy-making and should be accurately assessed when planning a TEER procedure for severe SMR to improve prognosis and subsequently reverse RV remodeling.
CHD is the most common congenital disease, including malformations of the cardiac structures and great vessels (85). Nearly twenty percent of newborns with CHD have right ventricular outflow tract obstruction (86), modern treatments for CHD, such as cardiac intervention and surgical repair, significantly prolong survival (87, 88). Along with surgery [such as surgery for tetralogy of Fallot (TOF), pulmonary artery atresia, patent truncus arteriosus, Ross procedure, etc.], new diseases have emerged, including pulmonary valve dysfunction as a short- or long-term outcome (89). Take TOF for instance, after transannular patch repair, most of these patients will ultimately encounter pulmonary regurgitation (PR), pulmonary stenosis and eventually RV dilatation (90). If left untreated, RV failure would occur during long-term following up (91). Thus, pulmonary valve replacement (PVR) is necessary to prevent irreversible RV failure (90, 92). Previous studies have focused on the assessment of RV dimensions and function, whereas the right ventricle is coupled to a highly compliant low-pressure PA system (93). Hence, the right ventricle and PA should be considered as a unit to assess prognosis after PVR. In or after the surgical treatment of TOF, pulmonary valve repair or replacement is often required to address issues such as pulmonary stenosis or PR (94, 95). Studies focusing on RV-PA coupling in repaired TOF have recently attracted more attention, but research on RV-PA coupling in postoperative TOF patients undergoing PVR has rarely been reported. The most relevant publications are listed in Table 4.
In a retrospective study of 135 postoperative TOF patients for PVR, noninvasive measurements by CMR imaging were performed in all patients, and the ratio of ESV/SV equal to Ea/Ees was introduced to define RV-PA coupling (ESV/SV ≤ 1 for coupling and >1 for uncoupling). RV-PA uncoupling was observed in 90 patients and 45 patients had a coupled RV-PA by CMR (23). Patients who underwent PVR had improved RV-PA coupling, supporting the idea that timely PVR can improve RV function. PA system maladapted to chronic RV loading explained the occurrence of uncoupling. Moreover, Sandeep et al. (23) found that Ea/Ees was negatively correlated with RVEF, which represents RV function that is directly correlated with volume overload and pulsatile afterload in the meantime; thus, assessment of RV-PA coupling following TOF repair is a more comprehensive way to reflect the hemodynamic burden of the right ventricle (23). Additionally, Kim SJ and colleagues (100) studied a total of 48 patients with repaired TOF and performed both cardiac catheterization and CMR imaging to assess hemodynamic parameters; 30 patients received PVR and most of them had RV-PA uncoupling. They found that no significant change in RV-PA coupling ratio was found after PVR. By univariate analysis, higher ESV/SV ratio was identified as a risk factor for adverse outcome, and they then concluded that RV-PA coupling is an important predictor for postoperative outcome (100).
As mentioned above, researchers have paid more attention to the RV-PA coupling in repaired TOF without PVR. In a study of 60 patients with repaired TOF, RVFAC/end-systolic area (ESA) ratio and peak tricuspid annular s velocity/indexed RV ESA ratio were assessed to represent RV-PA coupling. They concluded that RV-PA coupling is impaired in rTOF, while demonstrating for the first time that RV and LV mechanics, as well as severity of PR and tricuspid regurgitation (TR) in patients had correlations with RV-PA coupling (98). Buddhe et al. (97) compared RV-PA coupling in repaired standard TOF and those with repaired TOF-pulmonary atresia using the ratio of RV ESV to pulmonary artery SV. RV-PA coupling was impaired in both cohorts, but was worse in repaired TOF- pulmonary atresia. Notably, after regression analysis, they found that RV-PA coupling was associated with New York Heart Association (NYHA) class, and saw the promising future that RV-PA coupling emerge as an indicator of PA compliance and cardiovascular performance (97). Therefore, RV-PA coupling exerts a major impact on risk stratification and prognosis assessment in patients after TOF with or without PVR.
Although RV-PA coupling has been proven to be a strong marker to predict VHD prognosis postoperatively, limitations of which are not negligible. Invasive measurement method using RHC has high sensitivity and accuracy, but it is highly technically demanding, expensive and unpractical to operate bedside (24). Among non-invasive measurement methods, TAPSE/PASP ratio is the most commonly used due to its convenience, however, in case of modest to severe TR, PASP may be underestimated that leads to inaccurate RV-PA coupling measurement. In addition, TAPSE together with S′ is angle dependent. 3D-TTE derived SV/ESV avoids estimation of PASP but have high quality image requirements. Thus, further advancements in non-invasive techniques are in great demand. Another limitation is that the calculated cutoff value of RV-PA coupling via echocardiography varies significantly. It can decrease the importance of its measurement and building prognostic model.
RV-PA coupling has been considered as a strong marker to be implied to multiple conditions, among which is VHD. With the development of technology, treatments for VHD are diversiform and efficient enough to handle conditions that used to be troublesome. However, if unable to distinguish advanced stage of disease progression, advanced treatments to change the disease may be futile. Moreover, some patients may choose conservative treatments, monitoring their daily behavior of various risk conditions are vital to survival. RV-PA coupling has been shown to be a sensitive index for predicting prognosis both in conditions of congenital and acquired valvular diseases. Therefore, it should be routinely assessed as part of the comprehensive echocardiographic or CMR evaluation to help clinicians stratify risk, guide treatment, and improve prognosis. It is vital to develop new method to increase the accuracy, reproducibility, and time efficiency of the assessment of RV-PA coupling in clinical practice. For example, artificial intelligence approach has the potential to automatedly obtain RV-PA coupling and predict poor prognosis in VHD patients.
ZW: Conceptualization, Methodology, Software, Visualization, Writing – original draft, Writing – review & editing. MX: Funding acquisition, Methodology, Writing – original draft. LZ: Conceptualization, Funding acquisition, Methodology, Writing – original draft. QH: Software, Writing – original draft, Writing – review & editing. LG: Methodology, Writing – original draft, Writing – review & editing. MJ: Software, Writing – original draft, Writing – review & editing. YL: Software, Writing – original draft, Writing – review & editing. YL: Funding acquisition, Methodology, Writing – original draft.
The author(s) declare financial support was received for the research, authorship, and/or publication of this article. The research was supported by grants from National Natural Science Foundation of China (grant numbers 82371991, 82230066, 82371990, 82171964); Key Research and Development Program of Hubei (grant numbers 2020DCD015, 2021BCA138).
The authors declare that the research was conducted in the absence of any commercial or financial relationships that could be construed as a potential conflict of interest.
The authors declare that no Generative AI was used in the creation of this manuscript.
All claims expressed in this article are solely those of the authors and do not necessarily represent those of their affiliated organizations, or those of the publisher, the editors and the reviewers. Any product that may be evaluated in this article, or claim that may be made by its manufacturer, is not guaranteed or endorsed by the publisher.
1. Nkomo VT, Gardin JM, Skelton TN, Gottdiener JS, Scott CG, Enriquez-Sarano M. Burden of valvular heart diseases: a population-based study. Lancet. (2006) 368:1005–11. doi: 10.1016/S0140-6736(06)69208-8
2. Grose R, Strain J, Yipintosoi T. Right ventricular function in valvular heart disease: relation to pulmonary artery pressure. J Am Coll Cardiol. (1983) 2:225–32. doi: 10.1016/S0735-1097(83)80157-0
3. Prihadi EA, van der Bijl P, Dietz M, Abou R, Vollema EM, Marsan NA, et al. Prognostic implications of right ventricular free wall longitudinal strain in patients with significant functional tricuspid regurgitation. Circ Cardiovasc Imaging. (2019) 12:e008666. doi: 10.1161/CIRCIMAGING.118.008666
4. Leurent G, Auffret V, Donal E. Right ventricular–pulmonary artery coupling: a simple marker to guide cmplex clinical decisions?. JACC: Cardiovasc Interventions. (2022) 15:1834–6. doi: 10.1016/j.jcin.2022.08.020
5. Sultan I, Cardounel A, Abdelkarim I, Kilic A, Althouse AD, Sharbaugh MS, et al. Right ventricle to pulmonary artery coupling in patients undergoing transcatheter aortic valve implantation. Heart. (2019) 105:117–21. doi: 10.1136/heartjnl-2018-313385
6. Cahill TJ, Pibarot P, Yu X, Babaliaros V, Blanke P, Clavel M-A, et al. Impact of right ventricle-pulmonary artery coupling on clinical outcomes in the PARTNER 3 trial. JACC: Cardiovasc Interventions. (2022) 15:1823–33. doi: 10.1016/j.jcin.2022.07.005
7. Kubba S, Davila CD, Forfia PR. Methods for evaluating right ventricular function and ventricular-arterial coupling. Prog Cardiovasc Dis. (2016) 59:42–51. doi: 10.1016/j.pcad.2016.06.001
8. Tello K, Dalmer A, Axmann J, Vanderpool R, Ghofrani HA, Naeije R, et al. Reserve of right ventricular-arterial coupling in the setting of chronic overload. Circ Heart Fail. (2019) 12:e005512. doi: 10.1161/CIRCHEARTFAILURE.118.005512
9. Ünlü S, Bézy S, Cvijic M, Duchenne J, Delcroix M, Voigt J-U. Right ventricular strain related to pulmonary artery pressure predicts clinical outcome in patients with pulmonary arterial hypertension. Eur Heart J Cardiovasc Imaging. (2023) 24:635–42. doi: 10.1093/ehjci/jeac136
10. Bragança B, Trêpa M, Santos R, Silveira I, Fontes-Oliveira M, Sousa MJ, et al. Echocardiographic assessment of right ventriculo-arterial coupling: clinical correlates and prognostic impact in heart failure patients undergoing cardiac resynchronization therapy. J Cardiovasc Imaging. (2020) 28:109–20. doi: 10.4250/jcvi.2019.0094
11. Rosenkranz S, Gibbs JSR, Wachter R, De Marco T, Vonk-Noordegraaf A, Vachiéry J-L. Left ventricular heart failure and pulmonary hypertension. Eur Heart J. (2016) 37:942–54. doi: 10.1093/eurheartj/ehv512
12. Bhatia M, Jia S, Smeltz A, Kumar PA. Right heart failure management: focus on mechanical support options. J Cardiothorac Vasc Anesth. (2022) 36:3278–88. doi: 10.1053/j.jvca.2022.02.022
13. Nochioka K, Querejeta Roca G, Claggett B, Biering-Sørensen T, Matsushita K, Hung C-L, et al. Right ventricular function, right ventricular-pulmonary artery coupling, and heart failure risk in 4 US communities: the atherosclerosis risk in communities (ARIC) study. JAMA Cardiol. (2018) 3:939–48. doi: 10.1001/jamacardio.2018.2454
14. Wright SP, Groves L, Vishram-Nielsen JKK, Karvasarski E, Valle FH, Alba AC, et al. Elevated pulmonary arterial elastance and right ventricular uncoupling are associated with greater mortality in advanced heart failure. J Heart Lung Transplant. (2020) 39:657–65. doi: 10.1016/j.healun.2020.02.013
15. Tua L, Mandurino-Mirizzi A, Colombo C, Morici N, Magrini G, Nava S, et al. The impact of transcatheter edge-to-edge repair on right ventricle-pulmonary artery coupling in patients with functional mitral regurgitation. Eur J Clin Invest. (2023) 53:e13869. doi: 10.1111/eci.13869
16. Latus H, Binder W, Kerst G, Hofbeck M, Sieverding L, Apitz C. Right ventricular-pulmonary arterial coupling in patients after repair of tetralogy of Fallot. J Thorac Cardiovasc Surg. (2013) 146:1366–72. doi: 10.1016/j.jtcvs.2013.02.039
17. Humbert M, Kovacs G, Hoeper MM, Badagliacca R, Berger RMF, Brida M, et al. 2022 ESC/ERS guidelines for the diagnosis and treatment of pulmonary hypertension. Eur Heart J. (2022) 43:3618–731. doi: 10.1093/eurheartj/ehac237
18. Vonk Noordegraaf A, Westerhof BE, Westerhof N. The relationship between the right ventricle and its load in pulmonary hypertension. J Am Coll Cardiol. (2017) 69:236–43. doi: 10.1016/j.jacc.2016.10.047
19. Tello K, Wan J, Dalmer A, Vanderpool R, Ghofrani HA, Naeije R, et al. Validation of the tricuspid annular plane systolic excursion/systolic pulmonary artery pressure ratio for the assessment of right ventricular-arterial coupling in severe pulmonary hypertension. Circ Cardiovasc Imaging. (2019) 12:e009047. doi: 10.1161/CIRCIMAGING.119.009047
20. Lahm T, Douglas IS, Archer SL, Bogaard HJ, Chesler NC, Haddad F, et al. Assessment of right ventricular function in the research setting: knowledge gaps and pathways forward. An official American thoracic society research statement. Am J Respir Crit Care Med. (2018) 198:e15–43. doi: 10.1164/rccm.201806-1160ST
21. Sunagawa K, Maughan WL, Burkhoff D, Sagawa K. Left ventricular interaction with arterial load studied in isolated canine ventricle. Am J Physiol. (1983) 245:H773–780. doi: 10.1152/ajpheart.1983.245.5.H773
22. He Q, Lin Y, Zhu Y, Gao L, Ji M, Zhang L, et al. Clinical usefulness of right ventricle–pulmonary artery coupling in cardiovascular disease. J Clin Med. (2023) 12:2526. doi: 10.3390/jcm12072526
23. Sandeep B, Huang X, Li Y, Wang X, Mao L, Kan Y, et al. Evaluation of right ventricle pulmonary artery coupling on right ventricular function in post operative tetralogy of Fallot patients underwent for pulmonary valve replacement. J Cardiothorac Surg. (2020) 15:241. doi: 10.1186/s13019-020-01281-1
24. Ikonomidis I, Vlastos D. Corrigendum to ‘The role of ventricular–arterial coupling in cardiac disease and heart failure: assessment, clinical implications and therapeutic interventions. A consensus document of the European Society of Cardiology Working Group on Aorta & Peripheral Vascular Diseases, European Association of Cardiovascular Imaging, and Heart Failure Association’ [Eur J Heart Fail 2019;21:402–424]. Eur J Heart Fail. (2022) 24:1452. doi: 10.1002/ejhf.2452
25. Vonk Noordegraaf A, Haddad F, Bogaard HJ, Hassoun PM. Noninvasive imaging in the assessment of the cardiopulmonary vascular unit. Circulation. (2015) 131:899–913. doi: 10.1161/CIRCULATIONAHA.114.006972
26. Truong U, Patel S, Kheyfets V, Dunning J, Fonseca B, Barker AJ, et al. Non-invasive determination by cardiovascular magnetic resonance of right ventricular-vascular coupling in children and adolescents with pulmonary hypertension. J Cardiovasc Magn Reson. (2015) 17:81. doi: 10.1186/s12968-015-0186-1
27. Forton K, Motoji Y, Caravita S, Faoro V, Naeije R. Exercise stress echocardiography of the pulmonary circulation and right ventricular-arterial coupling in healthy adolescents. Eur Heart J Cardiovasc Imaging. (2021) 22:688–94. doi: 10.1093/ehjci/jeaa085
28. Raina A, Vaidya A, Gertz ZM, Chambers S, Forfia PR. Marked changes in right ventricular contractile pattern after cardiothoracic surgery: implications for post-surgical assessment of right ventricular function. J Heart Lung Transplant. (2013) 32:777–83. doi: 10.1016/j.healun.2013.05.004
29. Aubert R, Venner C, Huttin O, Haine D, Filippetti L, Guillaumot A, et al. Three-dimensional echocardiography for the assessment of right ventriculo-arterial coupling. J Am Soc Echocardiogr. (2018) 31:905–15. doi: 10.1016/j.echo.2018.04.013
30. Gavazzoni M, Badano LP, Cascella A, Heilbron F, Tomaselli M, Caravita S, et al. Clinical value of a novel three-dimensional echocardiography-derived index of right ventricle-pulmonary artery coupling in tricuspid regurgitation. J Am Soc Echocardiogr. (2023) 36:1154–1166.e3. doi: 10.1016/j.echo.2023.06.014
31. Kiamanesh O, Prosperi-Porta G, Harper L, Solverson K, Boiteau P, Helmersen D, et al. Ventricular-arterial decoupling is associated with in-hospital adverse events in normotensive pulmonary embolism. Int J Cardiovasc Imaging. (2022) 38:2655–65. doi: 10.1007/s10554-022-02677-x
32. Yang Y, Wang Z, Chen Z, Wang X, Zhang L, Li S, et al. Current status and etiology of valvular heart disease in China: a population-based survey. BMC Cardiovasc Disord. (2021) 21:339. doi: 10.1186/s12872-021-02154-8
33. Vahanian A, Beyersdorf F, Praz F, Milojevic M, Baldus S, Bauersachs J, et al. 2021 ESC/EACTS guidelines for the management of valvular heart disease. Eur Heart J. (2022) 43:561–632. doi: 10.1093/eurheartj/ehab395
34. Boskovski MT, Gleason TG. Current therapeutic options in aortic stenosis. Circ Res. (2021) 128:1398–417. doi: 10.1161/CIRCRESAHA.121.318040
35. Cavalcante JL, Rijal S, Althouse AD, Delgado-Montero A, Katz WE, Schindler JT, et al. Right ventricular function and prognosis in patients with low-flow, low-gradient severe aortic stenosis. J Am Soc Echocardiogr. (2016) 29:325–33. doi: 10.1016/j.echo.2015.12.001
36. Meucci MC, Malara S, Butcher SC, Hirasawa K, van der Kley F, Lombardo A, et al. Evolution and prognostic impact of right ventricular-pulmonary artery coupling after transcatheter aortic valve replacement. JACC Cardiovasc Interv. (2023) 16:1612–21. doi: 10.1016/j.jcin.2023.05.003
37. Galli E, Guirette Y, Feneon D, Daudin M, Fournet M, Leguerrier A, et al. Prevalence and prognostic value of right ventricular dysfunction in severe aortic stenosis. Eur Heart J Cardiovasc Imaging. (2015) 16:531–8. doi: 10.1093/ehjci/jeu290
38. Cavalcante JL, Simon MA, Chan SY. Comprehensive right-sided assessment for transcatheter aortic valve replacement risk stratification: time for a change. J Am Soc Echocardiogr. (2017) 30:47–51. doi: 10.1016/j.echo.2016.11.006
39. Schwartz LA, Rozenbaum Z, Ghantous E, Kramarz J, Biner S, Ghermezi M, et al. Impact of right ventricular dysfunction and tricuspid regurgitation on outcomes in patients undergoing transcatheter aortic valve replacement. J Am Soc Echocardiogr. (2017) 30:36–46. doi: 10.1016/j.echo.2016.08.016
40. Baron SJ, Wang K, House JA, Magnuson EA, Reynolds MR, Makkar R, et al. Cost-effectiveness of transcatheter versus surgical aortic valve replacement in patients with severe aortic stenosis at intermediate risk. Circulation. (2019) 139:877–88. doi: 10.1161/CIRCULATIONAHA.118.035236
41. Tam DY, Hughes A, Wijeysundera HC, Fremes SE. Cost-effectiveness of self-expandable transcatheter aortic valves in intermediate-risk patients. Ann Thorac Surg. (2018) 106:676–83. doi: 10.1016/j.athoracsur.2018.03.069
42. Leon MB, Smith CR, Mack M, Miller DC, Moses JW, Svensson LG, et al. Transcatheter aortic-valve implantation for aortic stenosis in patients who cannot undergo surgery. N Engl J Med. (2010) 363:1597–607. doi: 10.1056/NEJMoa1008232
43. Mack MJ, Leon MB, Smith CR, Miller DC, Moses JW, Tuzcu EM, et al. 5-year outcomes of transcatheter aortic valve replacement or surgical aortic valve replacement for high surgical risk patients with aortic stenosis (PARTNER 1): a randomised controlled trial. Lancet. (2015) 385:2477–84. doi: 10.1016/S0140-6736(15)60308-7
44. Eleid MF, Padang R, Pislaru SV, Greason KL, Crestanello J, Nkomo VT, et al. Effect of transcatheter aortic valve replacement on right ventricular-pulmonary artery coupling. JACC Cardiovasc Interv. (2019) 12:2145–54. doi: 10.1016/j.jcin.2019.07.025
45. Vizzardi E, Gavazzoni M, Sciatti E, Dallapellegrina L, Bernardi N, Raddino R, et al. Right ventricular deformation and right ventricular-arterial coupling in patients with heart failure due to severe aortic stenosis undergoing TAVI: long-term results. Am J Cardiovasc Dis. (2020) 10:150–63.32923096
46. Adamo M, Maccagni G, Fiorina C, Giannini C, Angelillis M, Costa G, et al. Prognostic value of right ventricle to pulmonary artery coupling in transcatheter aortic valve implantation recipients. J Cardiovasc Med. (2022) 23:615. doi: 10.2459/JCM.0000000000001336
47. Parasca CA, Calin A, Cadil D, Mateescu A, Rosca M, Botezatu SB, et al. Right ventricle to pulmonary artery coupling after transcatheter aortic valve implantation—determinant factors and prognostic impact. Front Cardiovasc Med. (2023) 10:1150039. doi: 10.3389/fcvm.2023.1150039
48. Alwan L, Tomii D, Heg D, Okuno T, Lanz J, Praz F, et al. Impact of right ventricular-pulmonary arterial coupling on clinical outcomes in patients undergoing transcatheter aortic valve implantation. Cardiovasc Revasc Med. (2023) 56:27–34. doi: 10.1016/j.carrev.2023.05.008
49. Hakgor A, Kahraman BC, Dursun A, Yazar A, Savur U, Akhundova A, et al. The influence of preoperative right ventricle to pulmonary arterial coupling on short- and long-term prognosis in patients who underwent transcatheter aortic valve implantation. Angiology. (2024). doi: 10.1177/00033197241232723
50. Silva I, Ternacle J, Hahn RT, Salah-Annabi M, Dahou A, Krapf L, et al. Left and right ventricular longitudinal systolic function following aortic valve replacement in the PARTNER 2 trial and registry. Eur Heart J Cardiovasc Imaging. (2024) 25:1276–86. doi: 10.1093/ehjci/jeae114
51. Haddad F, Denault AY, Couture P, Cartier R, Pellerin M, Levesque S, et al. Right ventricular myocardial performance index predicts perioperative mortality or circulatory failure in high-risk valvular surgery. J Am Soc Echocardiogr. (2007) 20:1065–72. doi: 10.1016/j.echo.2007.02.017
52. Benfari G, Antoine C, Miller WL, Thapa P, Topilsky Y, Rossi A, et al. Excess mortality associated with functional tricuspid regurgitation complicating heart failure with reduced ejection fraction. Circulation. (2019) 140:196–206. doi: 10.1161/CIRCULATIONAHA.118.038946
53. Hahn RT, Asch F, Weissman NJ, Grayburn P, Kar S, Lim S, et al. Impact of tricuspid regurgitation on clinical outcomes: the COAPT trial. J Am Coll Cardiol. (2020) 76:1305–14. doi: 10.1016/j.jacc.2020.07.035
54. Nath J, Foster E, Heidenreich PA. Impact of tricuspid regurgitation on long-term survival. J Am Coll Cardiol. (2004) 43:405–9. doi: 10.1016/j.jacc.2003.09.036
55. Topilsky Y, Nkomo VT, Vatury O, Michelena HI, Letourneau T, Suri RM, et al. Clinical outcome of isolated tricuspid regurgitation. JACC Cardiovasc Imaging. (2014) 7:1185–94. doi: 10.1016/j.jcmg.2014.07.018
56. Topilsky Y, Maltais S, Medina Inojosa J, Oguz D, Michelena H, Maalouf J, et al. Burden of tricuspid regurgitation in patients diagnosed in the community setting. JACC Cardiovasc Imaging. (2019) 12:433–42. doi: 10.1016/j.jcmg.2018.06.014
57. Arsalan M, Walther T, Smith RL II, Grayburn PA. Tricuspid regurgitation diagnosis and treatment. Eur Heart J. (2017) 38:634–8. doi: 10.1093/eurheartj/ehv487
58. Badano LP, Muraru D, Enriquez-Sarano M. Assessment of functional tricuspid regurgitation. Eur Heart J. (2013) 34:1875–85. doi: 10.1093/eurheartj/ehs474
59. Prihadi EA, Delgado V, Leon MB, Enriquez-Sarano M, Topilsky Y, Bax JJ. Morphologic types of tricuspid regurgitation: characteristics and prognostic implications. JACC: Cardiovasc Imaging. (2019) 12:491–9. doi: 10.1016/j.jcmg.2018.09.027
60. Taramasso M, Vanermen H, Maisano F, Guidotti A, La Canna G, Alfieri O. The growing clinical importance of secondary tricuspid regurgitation. J Am Coll Cardiol. (2012) 59:703–10. doi: 10.1016/j.jacc.2011.09.069
61. Rogers JH, Bolling SF. The tricuspid valve: current perspective and evolving management of tricuspid regurgitation. Circulation. (2009) 119:2718–25. doi: 10.1161/CIRCULATIONAHA.108.842773
62. Dreyfus J, Ghalem N, Garbarz E, Cimadevilla C, Nataf P, Vahanian A, et al. Timing of referral of patients with severe isolated tricuspid valve regurgitation to surgeons (from a French nationwide database). Am J Cardiol. (2018) 122:323–6. doi: 10.1016/j.amjcard.2018.04.003
63. Fortuni F, Butcher SC, Dietz MF, van der Bijl P, Prihadi EA, De Ferrari GM, et al. Right ventricular-pulmonary arterial coupling in secondary tricuspid regurgitation. Am J Cardiol. (2021) 148:138–45. doi: 10.1016/j.amjcard.2021.02.037
64. Lurz P, Besler C, Noack T, Forner AF, Bevilacqua C, Seeburger J, et al. Transcatheter treatment of tricuspid regurgitation using edge-to-edge repair: procedural results, clinical implications and predictors of success. EuroIntervention. (2018) 14:e290–7. doi: 10.4244/EIJ-D-17-01091
65. Orban M, Besler C, Braun D, Nabauer M, Zimmer M, Orban M, et al. Six-month outcome after transcatheter edge-to-edge repair of severe tricuspid regurgitation in patients with heart failure. Eur J Heart Fail. (2018) 20:1055–62. doi: 10.1002/ejhf.1147
66. Schlotter F, Orban M, Rommel K-P, Besler C, von Roeder M, Braun D, et al. Aetiology-based clinical scenarios predict outcomes of transcatheter edge-to-edge tricuspid valve repair of functional tricuspid regurgitation. Eur J Heart Fail. (2019) 21:1117–25. doi: 10.1002/ejhf.1547
67. Fortmeier V, Lachmann M, Stolz L, von Stein J, Unterhuber M, Kassar M, et al. Artificial intelligence-enabled assessment of right ventricular to pulmonary artery coupling in patients undergoing transcatheter tricuspid valve intervention. Eur Heart J Cardiovasc Imaging. (2023) 25:jead324. doi: 10.1093/ehjci/jead324
68. Brener MI, Lurz P, Hausleiter J, Rodés-Cabau J, Fam N, Kodali SK, et al. Right ventricular-pulmonary arterial coupling and afterload reserve in patients undergoing transcatheter tricuspid valve repair. J Am Coll Cardiol. (2022) 79:448–61. doi: 10.1016/j.jacc.2021.11.031
69. Stolz L, Weckbach LT, Karam N, Kalbacher D, Praz F, Lurz P, et al. Invasive right ventricular to pulmonary artery coupling in patients undergoing transcatheter edge-to-edge tricuspid valve repair. JACC Cardiovasc Imaging. (2023) 16:564–6. doi: 10.1016/j.jcmg.2022.10.004
70. Sugiura A, Tanaka T, Kavsur R, Öztürk C, Silaschi M, Goto T, et al. Refining accuracy of RV-PA coupling in patients undergoing transcatheter tricuspid valve treatment. Clin Res Cardiol. (2024) 113:177–86. doi: 10.1007/s00392-023-02339-5
71. Dziadzko V, Dziadzko M, Medina-Inojosa JR, Benfari G, Michelena HI, Crestanello JA, et al. Causes and mechanisms of isolated mitral regurgitation in the community: clinical context and outcome. Eur Heart J. (2019) 40:2194–202. doi: 10.1093/eurheartj/ehz314
72. El Sabbagh A, Reddy YNV, Nishimura RA. Mitral valve regurgitation in the contemporary era: insights into diagnosis, management, and future directions. JACC Cardiovasc Imaging. (2018) 11:628–43. doi: 10.1016/j.jcmg.2018.01.009
73. Writing Committee Members, Otto CM, Nishimura RA, Bonow RO, Carabello BA, Erwin JP, et al. 2020 ACC/AHA guideline for the management of patients with valvular heart disease: executive summary: a report of the American college of cardiology/American heart association joint committee on clinical practice guidelines. J Am Coll Cardiol. (2021) 77:450–500. doi: 10.1016/j.jacc.2020.11.035
74. Stolz L, Orban M, Braun D, Stark K, Steffen J, Orban M, et al. Anatomy and outcome of secondary mitral regurgitation subtypes undergoing transcatheter mitral valve edge-to-edge repair. JACC Cardiovasc Interv. (2021) 14:110–1. doi: 10.1016/j.jcin.2020.09.035
75. Raman J, Narang A, Appadurai V, Puthumana JJ. The increasing use of mitral transcatheter edge-to-edge repair in complex and high-risk patients. Heart Lung Circ. (2023) 32:653–5. doi: 10.1016/j.hlc.2023.05.015
76. Mack MJ, Lindenfeld J, Abraham WT, Kar S, Lim DS, Mishell JM, et al. 3-year outcomes of transcatheter mitral valve repair in patients with heart failure. J Am Coll Cardiol. (2021) 77:1029–40. doi: 10.1016/j.jacc.2020.12.047
77. Feldman T, Kar S, Elmariah S, Smart SC, Trento A, Siegel RJ, et al. Randomized comparison of percutaneous repair and surgery for mitral regurgitation: 5-year results of EVEREST II. J Am Coll Cardiol. (2015) 66:2844–54. doi: 10.1016/j.jacc.2015.10.018
78. Karam N, Stolz L, Orban M, Deseive S, Praz F, Kalbacher D, et al. Impact of right ventricular dysfunction on outcomes after transcatheter edge-to-edge repair for secondary mitral regurgitation. JACC Cardiovasc Imaging. (2021) 14:768–78. doi: 10.1016/j.jcmg.2020.12.015
79. Trejo-Velasco B, Estevez-Loureiro R, Carrasco-Chinchilla F, Fernández-Vázquez F, Arzamendi D, Pan M, et al. Prognostic role of TAPSE to PASP ratio in patients undergoing MitraClip procedure. J Clin Med. (2021) 10:1006. doi: 10.3390/jcm10051006
80. Brener MI, Grayburn P, Lindenfeld J, Burkhoff D, Liu M, Zhou Z, et al. Right ventricular-pulmonary arterial coupling in patients with HF secondary MR: analysis from the COAPT trial. JACC Cardiovasc Interv. (2021) 14:2231–42. doi: 10.1016/j.jcin.2021.07.047
81. Popolo Rubbio A, Testa L, Granata G, Salvatore T, De Marco F, Casenghi M, et al. Prognostic significance of right ventricle to pulmonary artery coupling in patients with mitral regurgitation treated with the MitraClip system. Catheter Cardiovasc Interv. (2022) 99:1277–86. doi: 10.1002/ccd.30044
82. Adamo M, Inciardi RM, Tomasoni D, Dallapellegrina L, Estévez-Loureiro R, Stolfo D, et al. Changes in right ventricular-to-pulmonary artery coupling after transcatheter edge-to-edge repair in secondary mitral regurgitation. JACC Cardiovasc Imaging. (2022) 15:2038–47. doi: 10.1016/j.jcmg.2022.08.012
83. Shechter A, Vaturi M, Kaewkes D, Koren O, Koseki K, Solanki A, et al. Prognostic value of baseline tricuspid annular plane systolic excursion to pulmonary artery systolic pressure ratio in mitral transcatheter edge-to-edge repair. J Am Soc Echocardiogr. (2023) 36:391–401.e19. doi: 10.1016/j.echo.2022.12.026
84. Koschutnik M, Donà C, Nitsche C, Kammerlander AA, Dannenberg V, Brunner C, et al. Impact of right ventricular-to-pulmonary artery coupling on remodeling and outcome in patients undergoing transcatheter edge-to-edge mitral valve repair. Clin Res Cardiol. (2023). doi: 10.1007/s00392-023-02318-w
85. Triedman JK, Newburger JW. Trends in congenital heart disease. Circulation. (2016) 133:2716–33. doi: 10.1161/CIRCULATIONAHA.116.023544
86. Verheugt CL, Uiterwaal CS, van der Velde ET, Meijboom FJ, Pieper PG, van Dijk AP, et al. Mortality in adult congenital heart disease. Eur Heart J. (2010) 31:1220–9. doi: 10.1093/eurheartj/ehq032
87. Stout KK, Daniels CJ, Aboulhosn JA, Bozkurt B, Broberg CS, Colman JM, et al. 2018 AHA/ACC guideline for the management of adults with congenital heart disease: a report of the American college of cardiology/American heart association task force on clinical practice guidelines. Circulation. (2019) 139:e698–800. doi: 10.1161/CIR.0000000000000603
88. Baumgartner H, De Backer J, Babu-Narayan SV, Budts W, Chessa M, Diller G-P, et al. 2020 ESC guidelines for the management of adult congenital heart disease. Eur Heart J. (2021) 42:563–645. doi: 10.1093/eurheartj/ehaa554
89. Cleveland JD, Wells WJ. The surgical approach to pulmonary valve replacement. Semin Thorac Cardiovasc Surg. (2022) 34:1256–61. doi: 10.1053/j.semtcvs.2022.05.006
90. Ferraz Cavalcanti PE, Sá MP, Santos CA, Esmeraldo IM, Escobar RR, Menezes AM, et al. Pulmonary valve replacement after operative repair of tetralogy of Fallot: meta-analysis and meta-regression of 3,118 patients from 48 studies. J Am Coll Cardiol. (2013) 62:2227–43. doi: 10.1016/j.jacc.2013.04.107
91. Voelkel NF, Quaife RA, Leinwand LA, Barst RJ, McGoon MD, Meldrum DR, et al. Right ventricular function and failure: report of a national heart, lung, and blood institute working group on cellular and molecular mechanisms of right heart failure. Circulation. (2006) 114:1883–91. doi: 10.1161/CIRCULATIONAHA.106.632208
92. Oosterhof T, van Straten A, Vliegen HW, Meijboom FJ, van Dijk APJ, Spijkerboer AM, et al. Preoperative thresholds for pulmonary valve replacement in patients with corrected tetralogy of Fallot using cardiovascular magnetic resonance. Circulation. (2007) 116:545–51. doi: 10.1161/CIRCULATIONAHA.106.659664
93. Buddhe S, Shah A, Lai WW. Progression of right ventricular dilation in repaired tetralogy of Fallot. J Magn Reson Imaging. (2015) 41:730–7. doi: 10.1002/jmri.24610
94. Bokma JP, Geva T, Sleeper LA, Narayan SVB, Wald R, Hickey K, et al. A propensity score-adjusted analysis of clinical outcomes after pulmonary valve replacement in tetralogy of Fallot. Heart. (2018) 104:738–44. doi: 10.1136/heartjnl-2017-312048
95. Geva T, Mulder B, Gauvreau K, Babu-Narayan SV, Wald RM, Hickey K, et al. Preoperative predictors of death and sustained ventricular tachycardia after pulmonary valve replacement in patients with repaired tetralogy of Fallot enrolled in the INDICATOR cohort. Circulation. (2018) 138:2106–15. doi: 10.1161/CIRCULATIONAHA.118.034740
96. Egbe AC, Kothapalli S, Miranda WR, Pislaru S, Ammash NM, Borlaug BA, et al. Assessment of right ventricular-pulmonary arterial coupling in chronic pulmonary regurgitation. Can J Cardiol. (2019) 35:914–22. doi: 10.1016/j.cjca.2019.03.009
97. Buddhe S, Jani V, Sarikouch S, Gaur L, Schuster A, Beerbaum P, et al. Differences in right ventricular-pulmonary vascular coupling and clinical indices between repaired standard tetralogy of Fallot and repaired tetralogy of Fallot with pulmonary atresia. Diagn Interv Imaging. (2021) 102:85–91. doi: 10.1016/j.diii.2020.05.008
98. Cheng S, Li VW-Y, So EK-F, Cheung Y-F. Right ventricular-pulmonary arterial coupling in repaired tetralogy of Fallot. Pediatr Cardiol. (2022) 43:207–17. doi: 10.1007/s00246-021-02719-6
99. Vitarelli A, Miraldi F, Capotosto L, Galea N, Francone M, Marchitelli L, et al. Comprehensive echocardiographic assessment of right ventricular function, pulmonary arterial elastic properties and ventricular-vascular coupling in adult patients with repaired tetralogy of Fallot: clinical significance of 3D derived indices. Int J Cardiovasc Imaging. (2023) 39:1631–41. doi: 10.1007/s10554-023-02857-3
Keywords: right ventricular-pulmonary artery coupling, valvular heart disease, pulmonary hypertension, congenital heart defect, right ventricular function, clinical utility
Citation: Wu Z, Xie M, Zhang L, He Q, Gao L, Ji M, Lin Y and Li Y (2025) Prognostic implication of right ventricular-pulmonary artery coupling in valvular heart disease. Front. Cardiovasc. Med. 11:1504063. doi: 10.3389/fcvm.2024.1504063
Received: 30 September 2024; Accepted: 20 December 2024;
Published: 14 January 2025.
Edited by:
Wen Qin, Tianjin Medical University General Hospital, ChinaReviewed by:
Natallia Maroz-Vadalazhskaya, Belarusian State Medical University, BelarusCopyright: © 2025 Wu, Xie, Zhang, He, Gao, Ji, Lin and Li. This is an open-access article distributed under the terms of the Creative Commons Attribution License (CC BY). The use, distribution or reproduction in other forums is permitted, provided the original author(s) and the copyright owner(s) are credited and that the original publication in this journal is cited, in accordance with accepted academic practice. No use, distribution or reproduction is permitted which does not comply with these terms.
*Correspondence: Yuman Li, bGl5bUBodXN0LmVkdS5jbg==
†These authors have contributed equally to this work
Disclaimer: All claims expressed in this article are solely those of the authors and do not necessarily represent those of their affiliated organizations, or those of the publisher, the editors and the reviewers. Any product that may be evaluated in this article or claim that may be made by its manufacturer is not guaranteed or endorsed by the publisher.
Research integrity at Frontiers
Learn more about the work of our research integrity team to safeguard the quality of each article we publish.