- 1The First Clinical College of Traditional Chinese Medicine, Hunan University of Chinese Medicine, Changsha, China
- 2Department of Urology, The First Affiliated Hospital of Jinzhou Medical University, Jinzhou Medical University, Jinzhou, China
- 3International Medical Department, The First Hospital of Hunan University of Chinese Medicine, Changsha, China
- 4Intensive Care Unit, The First Hospital of Hunan University of Chinese Medicine, Changsha, China
- 5Preventive Treatment Center, The First Hospital of Hunan University of Chinese Medicine, Changsha, China
Background: Previous studies have shown an association between lipid-lowering drugs, circulating inflammatory factors, and atrial fibrillation (AF), but the specific effects of lipid-lowering drugs on AF and whether they can be mediated by circulating inflammatory factors remain unclear.
Methods: We collected 10 genetic variants encoding lipid-lowering drug targets (LDLR, HMGCR, PCSK9, NPC1L1, APOB, APOB, ABCG5, ABCG8, LPL, APOC3, and PPARA) and AF based on genome-wide association study (GWAS) summary statistics. Drug target Mendelian randomization (MR) was used to explore the causal relationship between lipid-lowering drugs and AF. In addition, we performed a mediation analysis of 91 circulating inflammatory factors to explore potential mediators. Sensitivity analyses were performed to verify the reliability of the MR Results by MR-Egger intercept test, Cochran's Q test and leave-one-out test.
Results: The results of IVW method showed that LPL agonist had a protective effect on AF(OR = 0. 854, 95%CI: 0.816–0.894, P = 1.844E-11). However, the other nine lipid-lowering drug targets had no significant effect on AF. Notably, we found a mediator role of Fibroblast Growth Factor 5 (FGF5) in the protective effect of LPL agonist on AF with a mediator ratio of 9.22%. Sensitivity analyses supported the robustness of our findings, indicating a possible mediating pathway by which LPL agonists affect the risk of AF.
Conclusion: Our study provides new insights into the complex interactions among lipid-lowering agents, circulating inflammatory factors and AF, and also identified a potential mediating role of FGF5 in the pathogenesis of AF. Our findings highlight the potential of LPL agonists and targeting specific inflammatory factors for therapeutic intervention in AF, providing promising avenues for future research and clinical strategies for the management and prevention of AF.
1 Introduction
Atrial Fibrillation (AF), as one of the most common arrhythmias in clinical practice, has the characteristics of high disability rate and high mortality, and can cause a series of complications such as stroke, heart failure, cognitive dysfunction, which poses a serious threat (1–4). Although many studies have identified a variety of risk factors for atrial fibrillation, including age, hypertension and cardiac structural abnormalities, the pathogenesis of AF is still not fully understood, and the efficacy and safety of existing treatments are limited.
In recent years, abnormal lipid metabolism and inflammatory response have been considered to be important factors in the pathogenesis of AF. Elevated blood levels of low-density lipoprotein cholesterol (LDL-C) and triglycerides (TG), as well as inflammatory factors such as C-reactive protein (CRP) and interleukin-6 (IL-6), are associated with an increased risk of AF (5–10). And, several studies have indicated that dyslipidemia may further lead to inflammation (11, 12). Furthermore, it has been reported in some studies that in addition to their lipid-lowering properties, various lipid-lowering drugs may also exhibit anti-inflammatory and immunomodulatory effects, effectively reducing the incidence of AF (13, 14). However, some prospective studies have presented conflicting evidence regarding the correlation (15–18). Currently, the specific relationship between lipid-lowering drugs, circulating inflammatory factors and AF is not clear, especially the mediating role of inflammatory factors in the causal relationship between lipid-lowering drugs and AF. Therefore, it is crucial to explore the effects of lipid-lowering drugs and circulating inflammatory factors on AF.
Drug-target Mendelian randomization (MR) is a new research approach specifically designed for drug repurposing, finding new therapeutic targets, and revealing the harm of drugs. Compared with the traditional observational study, MR can effectively avoid the interference of external factors and potential confounding factors, being regarded as a natural randomized controlled trial that is more cost-effective. We explored the causal relationship between LDL-C (ABCG5, ABCG8, APOB, HMGCR, LDLR, NPC1L1 and PCSK9) and TG (LPL, APOC3 and PPARA) lowering targets and AF, as well as the mediating role of circulating inflammatory factors. We hypothesize that drugs lowering LDL-C and TG levels may indirectly reduce AF risk by dampening the inflammatory response. In this way, we hope to reveal the feasibility and effectiveness of lipid-lowering drugs as potential drug targets for AF, reveal the underlying biological mechanisms, and provide more precise therapeutic strategies for the prevention and treatment of AF.
2 Article types
Original Research
3 Materials and methods
3.1 Study design
In this study, single nucleotide polymorphisms (SNPs) from the GWAS data were used as instrumental variables (IVs) for MR Analysis. The selection of SNPs for MR Analysis is based on three core assumptions (19): (1) SNPs must be strongly associated with the exposure variables; (2) SNPs should be independent of any potential confounders; and (3) SNPs influence the outcome solely through the exposure variables. This study was reported following the Strengthening the Reporting of Observational Studies in Epidemiology Using Mendelian Randomization (20, 21) (STROBE-MR). The specific process of this study is illustrated in Figure 1.
3.2 Genetic instruments for lipid-lowering drugs
In this study, we selected commonly used lipid-lowering drugs and the latest treatment methods, such as statins, ezetimibe, PCSK9 inhibitors, bile acid sequestrants, mipomersen, fibrates, and antisense oligonucleotides targeting apolipoprotein C-III (APOC3) mRNA. Utilizing the DrugBank database, we have identified the genes responsible for encoding the pharmacological targets of these drugs. These target genes can be further categorized into those that reduce LDL-C (such as LDLR, HMGCR, NPC1L1, PCSK9, APOB, ABCG5, and ABCG8) and those that reduce TG (including LPL, APOC3, and PPARA). LDL-C serum levels and TG serum levels are reliable downstream biomarkers of lipid-lowering drugs, so we simulated the effect of lipid-lowering drugs by obtaining SNPs within ± 100 kb of the drug target sites associated with LDL-C or TG levels to estimate the downstream effect of lipid-lowering drugs on AF risk.
Summary data on LDL-C (accession number: ieu-b-110), consisting of 440,546 Europeans and 12,321,875 SNPs, and summary data on TG (accession number: ieu-b-111), consisting of 441,016 Europeans and 12,321,875 SNPs, were obtained from the UK Biobank (UKBB). Richardson TG et al. (22) conducted a GWAS of circulating non-fasted lipoprotein lipid traits in the UKBB for low-density LDL-C, TG, and apolipoprotein B to identify lipid-associated SNPs. We first screened SNPs that were strongly associated with LDL-C or TG levels under the condition of P < 5E-08. Secondly, in order to ensure the independence of IVs, we excluded the interference of linkage disequilibrium (LD). For screening SNPs, R2 < 0.3 was used as the criterion if more than three SNPs were identified. If fewer than three SNPs were obtained, R2 < 0.4 was applied to ensure the MR Analysis's reliability. In addition, only SNPs with an effect allele frequency (EAF) > 0.01 were considered. Finally, in order to avoid the interference of potential confounding factors, LD trait tool was used to find and remove possible confounding factors, such as obesity, body mass index, smoking, hypertension, and diabetes (23).
3.3 Genetic instruments for circulating inflammatory factors
The GWAS data set for circulating inflammatory factors is available in the GWAS Catalog from a genome-wide protein quantitative trait locus study (accession number: GCST90274758 to GCST90274848). Zhao JH et al. (24) performed genome-wide pQTL mapping for 91 plasma proteins measured using the Olink Target Inflammation panel in 11 cohorts totaling 14,824 European-ancestry participants. To obtain a sufficient number of SNPs to ensure the reliability of the MR Analysis, we screened SNPs that were significantly associated with circulating inflammatory factors under the condition of P < 5E-06, and then excluded the interference from LD (R2 < 0.001, KB = 10,000) to ensure the independence of IVs.
3.4 Source of results
The GWAS data for AF in this study are available on the IEU open GWAS database (accession number: ebi-a-GCST006414). Nielsen JB et al. (25) tested association between 34,740,186 genetic variants and AF, comparing a total of 60,620 cases and 970,216 controls of European ancestry from six contributing studies (HUNT, deCODE, MGI, DiscovEHR, UK Biobank, and the AFGen Consortium). As lipid-lowering drugs have been approved for the treatment of coronary heart disease (CHD), CHD was selected as a positive control to validate our chosen lipid-lowering drug instrument in this study. The GWAS data for CHD were obtained from CARDIoGRAMplusC4D (accession number: ieu-a-7). Nikpay M et al. (26) assembled 60,801 cases and 123,504 controls from 48 studies for a GWAS meta-analysis of coronary artery disease. All the datasets included in this study were sourced from European populations to maintain demographic consistency.
3.5 Mediation analysis
First, we conducted separate assessments of the total causal effect of each of the 10 lipid-lowering drug targets on AF (α) and the causal effect of the lipid-lowering drug targets that were significantly associated with AF on circulating inflammatory factors (β1). Second, we analyzed the causal relationship between circulating inflammatory factors that were significantly associated with lipid-lowering drugs and AF (β2). In this MR Analysis, we excluded SNPs in the MR Analysis of lipid-lowering drug targets and AF. Finally, we utilized the coefficient product method to calculate the indirect effect (β1 × β2) and mediating proportion [(β1 × β2)/α] of circulating inflammatory factors on the causal relationship between lipid-lowering drugs and AF.
3.6 Statistical analysis
Inverse variance weighted (IVW) was used as the main analysis method, and weighted median (WME) and MR Egger's method were used for supplementary analysis. The strength of instrumental variables was evaluated using the F-statistic, with a value greater than 10 indicating no weak instrument bias (27). Heterogeneity was assessed using Cochran's Q statistics based on IVW and MR Egger methods (28). In cases of heterogeneity, the inverse variance weighted multiplicative random effects (IVW-MRE) model was employed for MR Analysis (29).MR-Egger intercept test was used to detect pleiotropy, MR-PRESSO was used to identify and remove outliers, and leave-one-out sensitivity test was used to assess whether individual SNPs had a significant effect on the overall results.
All statistical analyses were performed using R 4.3.2 and the R package (TwoSampleMR, VariantAnnotation, ieugwasr, gwasglue, and MR-PRESSO). To correct for bias caused by multiple comparisons, P-values were adjusted through Bonferroni correction (30).
4 Results
4.1 Positive control analysis
We identified causal relationships between various lipid-lowering drug targets and CHD, and as expected, the results of the IVW showed that, all 10 lipid-lowering drugs significantly reduced the risk of CHD [Bonferroni-corrected P-value threshold = 0.005 (0.05/10)] (Figure 2 and Supplementary Table S1).
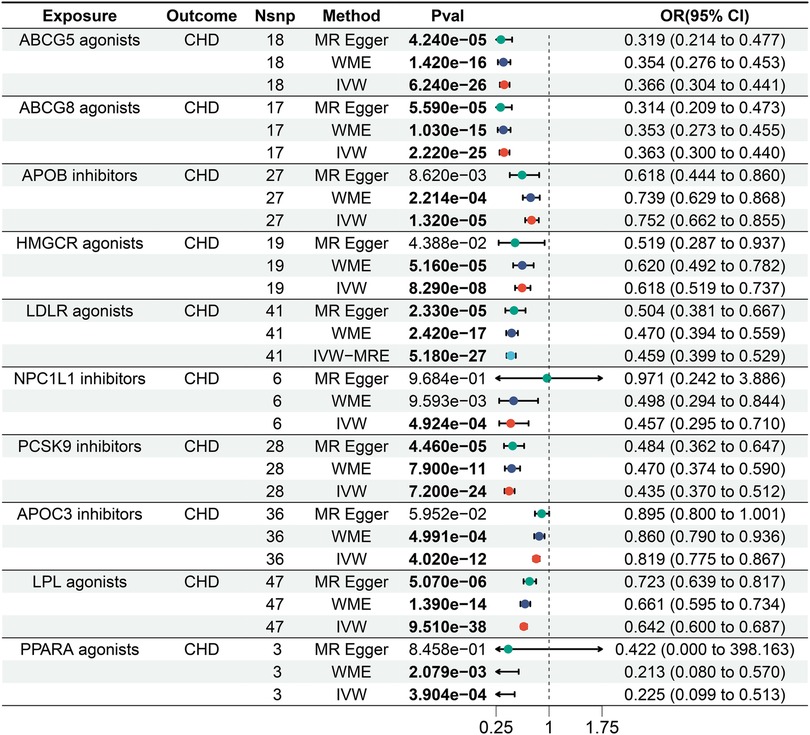
Figure 2. Forest plot of the results of the MR analysis between lipid-lowering drugs and coronary heart disease.
4.2 MR analysis between lipid-lowering drugs and AF
The IVW results showed that LPL agonist had a significant effect on AF [Bonferrai-corrected P-value threshold = 0.005 (0.05/10)] (OR = 0. 854, 95% CI: 0.816–0.894, P = 1.844E-11) (Supplementary Tables S2 and S3). However, there was no significant effect of other targets on AF (Figure 3).
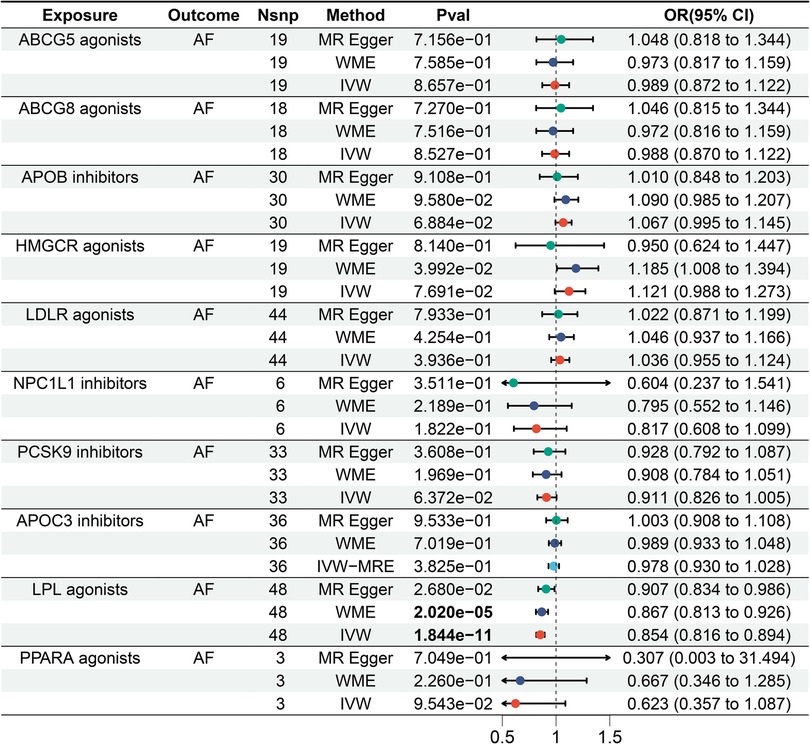
Figure 3. Forest plot of the results of the MR analysis between lipid-lowering drugs and atrial fibrillation.
4.3 MR analysis between lipid-lowering drugs and circulating inflammatory factors
We assessed the impact of LPL agonist on 91 circulating inflammatory factors. According to IVW results, we observed a causal association between LPL agonist and reduced levels of six circulating inflammatory factors after Bonferroni correction [Bonferrai-corrected P-value threshold = 5.495E-04 (0.05/91)] (Figure 4). Specifically, The results showed that LPL agonist was associated with decreased levels of Fibroblast growth factor (FGF) 19 (OR = 0.847, 95% CI = 0.777–0.924, P = 1.861E-04), FGF5 (OR = 0.817, 95% CI = 0.745–0.896, P = 1.781E-05), IL-6 (OR = 0.834, 95% CI = 0.765–0.909, P = 3.800E-05), Matrix metalloproteinase-1 (MMP-1) (OR = 0.837, 95% CI = 0.764–0.918, P = 1.534E-05), Oncostatin-M (OR = 0.836, 95% CI = 0.756–0.925, P = 5.092E-04) and Tumor necrosis factor (TNF) (OR = 0.836, 95%CI = 0.760–0.921, P = 2.630E-04) (Supplementary Tables S4 and 5).
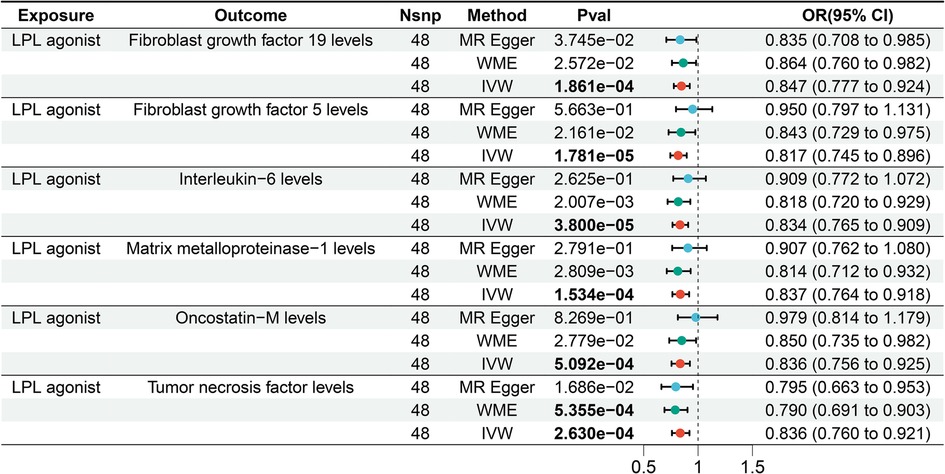
Figure 4. Forest plot of the results of the MR analysis between lipid-lowering drugs and circulating inflammatory factors.
4.4 MR analysis between circulating inflammatory factors and AF
Based on the IVW results, the MR analysis revealed a significant causal association between elevated FGF5 levels and an increased risk of AF [Bonferroni-corrected P-value threshold = 5.556E-03(0.05/9)] (OR = 1.075, 95% CI = 1.047–1.103, P = 6.436E-08) among the six LPL agonist related circulating inflammatory factors (Figure 5). However, there was no evidence of a significant causal relationship between the other 5 circulating inflammatory factors and AF.
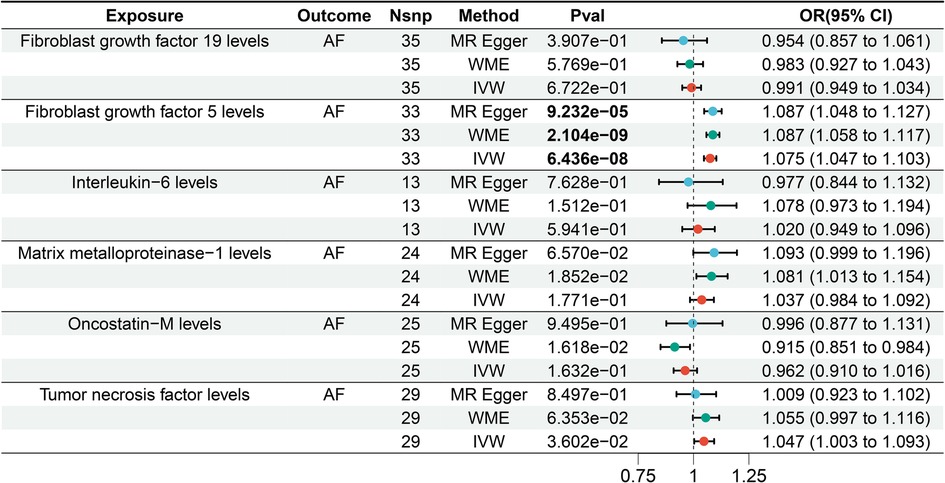
Figure 5. Forest plot of the results of the MR analysis between circulating inflammatory factors and atrial fibrillation.
4.5 Mediating role of circulating inflammatory factors in the causal relationship between lipid-lowering drugs and AF
We further investigated the mediating role of FGF5 levels in the causal relationship between LPL agonist and AF. Our findings suggest that LPL agonist may reduce the risk of AF by lowering FGF5 levels. Using the product of coefficient method, we calculated that LPL agonist is associated with a 9.22% reduction in FGF5 levels related to AF risk.
4.6 Results of sensitivity analysis
The MR-Egger intercept test indicated no horizontal pleiotropy in any of the results in this study. The Cochran Q-test showed heterogeneity in the MR Analysis between LDLR agonists and CHD, so we performed the MR Analysis using the IVW-MRE method to eliminate the bias caused by heterogeneity. In addition, an outlier (rs73015007) was identified in the MR Analysis between LDLR agonists and CHD. After removing this outlier, we repeated the MR Analysis to ensure result robustness. However, no heterogeneity or outliers were detected in the remaining MR Analyses (Supplementary Tables S7–S10). The results of leave-one-out method showed that there was no significant effect of individual SNP on the overall results in all the MR Analyses. In conclusion, the MR Results of this study are robust.
5 Discussion
In this study, we conducted a comprehensive exploration of the intricate relationship between LPL agonist, circulating inflammatory factors, and AF using the MR Method. The reliability of our findings was ensured through multiple sensitivity analyses and Bonferroni correction. Our study revealed a significant protective effect of LPL agonist against AF and highlighted the pivotal role of FGF5 in this process.
Through the analysis of the causal relationship between 10 lipid-lowering drug targets and AF, we discovered that LPL agonist exhibited a significant protective effect on AF. Subsequently, we conducted further investigation into the impact of LPL agonist on 91 circulating inflammatory factors. Our findings revealed that LPL agonist was significantly correlated with reduced levels of six inflammatory factors, including IL-6, TNF, and MMP-1, which was consistent with the conclusion that lipid-lowering drugs may have certain anti-inflammatory effects proposed in previous studies. LPL is an important lipid metabolic enzyme in the body, which can degrade TG in Triglyceride rich Lipoproteins (TRLs) and release free fatty acids (FFA) for the body to use (31–34). LPL agonist is a class of drugs that can reduce blood lipid levels by activating lipoprotein lipase (LPL) and accelerating the hydrolysis of TRLs.
In recent years, the association between TRLS and inflammation has been extensively studied. Moreno-Vedia et al. (35) have shown that TRL concentration and composition have a significant effect on the subclinical inflammatory state of patients with lipid metabolism disorders. Cesena et al. (36) found different patterns of association between TRL subparticles and inflammatory markers through cross-sectional analysis of the Brazilian Longitudinal Study of Adult Health (ELSA-Brasil) which supports the hypothesis that TRL may induce a low-grade inflammatory environment. A large number of previous studies have shown that inflammation plays an important role (37) in the occurrence and development of AF. Inflammation may increase the risk of AF by promoting atrial fibrosis, electrical remodeling, and causing endothelial dysfunction. Therefore, we suggest that LPL agonist may play a protective role in AF by accelerating the hydrolysis of TRLs, reducing the deposition of lipids in vascular endothelial cells, and reducing the activation and inflammatory response of endothelial cells.
However, conflicting evidence exists regarding the relationship between lipid levels and AF. A recent meta-analysis (38) found no significant correlation between TG levels and AF occurrence. This discrepancy may stem from the influence of age-related declines in TG levels or fluctuations due to dietary changes, which complicates the understanding of TG's long-term effect on AF. In contrast to simple blood lipid measurements, our study focuses on lipoprotein lipase (LPL) agonists, which dynamically regulate lipid metabolism and inflammation, offering a more comprehensive perspective on their potential protective role in AF. Therefore, while elevated TG levels may not be directly linked to AF, LPL agonists might confer protective effects through mechanisms that extend beyond lipid levels alone.
Due to the intricate nature of the LPL regulation mechanism, as well as the challenge of developing high specificity and selectivity for the target and the complexity of clinical trials, current understanding of LPL activation as the core mechanism for drug effects is still in its developmental stage (39). In this study, we found the protective effect of LPL agonist on AF using drug-target MR Method, which provides a theoretical basis for larger-scale research of LPL agonist in the future.
FGF5 is a member of the FGF family, which is mainly involved in cell proliferation, differentiation, survival and tissue repair (40). Previous studies have found that FGF5 is mainly associated with cancer and hair growth (41–44). Recent studies have indicated that FGF5 also plays a significant role in cardiovascular conditions such as hypertension and coronary artery disease (CAD) (45–48). Vatner SF et al. (49) demonstrated that FGF5 has the ability to inhibit cell apoptosis, induce cardiomyocyte hypertrophy and proliferation, ultimately leading to an increase in myocardial mass and myocardial wall thickening. Additionally, Hu et al. (50) established a causal relationship between FGF5 and Cardiac Remodeling using MR method. In addition, FGF5 also plays an important role in promoting tissue fibrosis. Hiromi H et al. (51) discovered that FGF5 was implicated in the progression of liver fibrosis and suggested that FGF5 could potentially serve as a therapeutic target for liver fibrosis in non-alcoholic steatohepatitis. In the present study, we identified a significant causal relationship between elevated levels of FGF5 and an increased AF risk. While there is no direct evidence linking FGF5 to AF, our findings suggest that this causal relationship may be attributed to the ability of FGF5 to induce cardiomyocyte hypertrophy and promote myocardial fibrosis. These effects ultimately lead to structural remodeling of the atrium, providing a potential mechanistic basis for the observed association (52, 53).
Through mediation analysis, we found that LPL agonist reduced the risk of AF by reducing the level of FGF5. The results of the mediation analysis indicated that changes in FGF5 levels accounted for 9.22% of the reduction in AF risk associated with LPL agonist treatment. This suggests that FGF5 plays a partial mediating role in the mechanism of action of LPL agonists in preventing AF. Previous studies have suggested that the protective effect of LPL agonists on AF may be linked to chronic inflammation and myocardial fibrosis, while FGF5 may increase the risk of AF through myocardial fibrosis and structural remodeling of the atrium. Therefore, it is possible that FGF5 mediates the causal relationship between LPL agonists and AF. Our study provides genetic evidence supporting the idea that FGF5 may mediate the protective effects of partial LPL agonists against AF. However, it is important to note that while our findings suggest a potential mediating association, further experimental studies are needed to confirm causality as well as to determine if other factors also play a role.
To the best of our knowledge, this is the first study to utilize MR analysis in examining the correlation between lipid-lowering drugs, circulating inflammatory factors, and AF in the general population. Our study provides new targets and strategies for the targeted treatment of AF, and points out the direction for future research. In terms of clinical implications, our findings suggest that LPL agonists could serve as novel therapeutic agents for AF prevention and management by targeting both lipid metabolism and inflammation. By reducing key inflammatory factors and influencing lipid dynamics, LPL agonists may address fundamental aspects of AF pathogenesis. Furthermore, identifying FGF5 as a mediator offers a new therapeutic target, potentially allowing for more effective interventions to prevent atrial remodeling and fibrosis associated with AF. From a research perspective, our study underscores the value of integrating genetic association studies with drug development processes. Utilizing MR analysis provides genetic evidence that supports causal relationships, aiding in the prioritization of drug targets. This approach is consistent with recent frameworks that advocate for leveraging human genetics to identify druggable targets for AF (54).
At the same time, this study also has certain limitations. First, all the data in the study were from persons of European ancestry. This reduced bias due to ethnic and regional differences but also limited the generalizability of the findings to other ethnic groups. Second, although we used a variety of sensitivity analyses in our study, potential horizontal pleiotropy may still exist. Therefore, future studies should further explore the role of LPL agonist and FGF5 in different populations and clinical settings, and verify the potential for practical application of these biomarkers in the prevention and treatment of AF.
6 Conclusion
In conclusion, this study supports the genetically predicted association between LPL agonist, circulating inflammatory factors, and AF. Specifically, FGF5 levels mediate the protective effect of LPL agonist against AF. These findings provide genetic evidence for the mechanism by which LPL agonists reduce the risk of AF and may inform future mechanistic and clinical studies.
Data availability statement
The datasets presented in this study can be found in online repositories. The names of the repository/repositories and accession number(s) can be found in the article/Supplementary Material.
Ethics statement
Ethical approval was not required for the studies involving humans because all data used in this study were obtained from publicly available databases; further ethical approval was not required. The studies were conducted in accordance with the local legislation and institutional requirements. The human samples used in this study were acquired from The datasets supporting this study are available from EMBL's European Bioinformatics Institute, GWAS Catalog and the UK Biobank database. Written informed consent to participate in this study was not required from the participants or the participants’ legal guardians/next of kin in accordance with the national legislation and the institutional requirements.
Author contributions
GO: Writing – original draft, Writing – review & editing, Conceptualization, Methodology. YZ: Conceptualization, Writing – original draft. HC: Data curation, Writing – review & editing. KY: Methodology, Writing – original draft. ZQ: Methodology, Writing – original draft. YC: Formal Analysis, Writing – original draft. YY: Visualization, Writing – original draft. QC: Writing – review & editing, Methodology, Conceptualization, Visualization. XC: Funding acquisition, Writing – original draft, Writing – review & editing.
Funding
The author(s) declare financial support was received for the research, authorship, and/or publication of this article. This study was funded by National Natural Science Foundation of China (81704061, 81173213), the National Famous Elderly Chinese Medicine Expert Chen Xinyu Inheritance Workshop Construction Project [National Chinese Medicine Human Education Letter (2022) No. 75], the R & D Plan for Key Areas of Hunan Provincial Department of Science and Technology (2019SK2321) and the Hunan Science and Technology Talent Hosting Project (2020TJ-N01).
Acknowledgments
We sincerely thank the investigators who provided the summary data on GWAS.
Conflict of interest
The authors declare that the research was conducted in the absence of any commercial or financial relationships that could be construed as a potential conflict of interest.
Publisher's note
All claims expressed in this article are solely those of the authors and do not necessarily represent those of their affiliated organizations, or those of the publisher, the editors and the reviewers. Any product that may be evaluated in this article, or claim that may be made by its manufacturer, is not guaranteed or endorsed by the publisher.
Supplementary material
The Supplementary Material for this article can be found online at: https://www.frontiersin.org/articles/10.3389/fcvm.2024.1446610/full#supplementary-material
Abbreviations
LPL, lipoprotein lipase; TG, triglyceride; FGF5, fibroblast growth factor 5; AF, atrial fibrillation; LDL-C, low-density lipoprotein cholesterol; CHD, coronary heart disease; MR, Mendelian randomization; IVW, inverse variance weighted; WME, weighted median; SNP, single nucleotide polymorphisms; NSNP, number of single nucleotide polymorphisms; POS, position; CHR, chromosome; SE, standard error; OR, odds ratio; CI, confidence interval.
References
1. Hindricks G, Potpara T, Dagres N, Arbelo E, Bax JJ, Blomström-Lundqvist C, et al. 2020 ESC guidelines for the diagnosis and management of atrial fibrillation developed in collaboration with the European association for cardio-thoracic surgery (EACTS): the task force for the diagnosis and management of atrial fibrillation of the European Society of Cardiology (ESC) developed with the special contribution of the European heart rhythm association (EHRA) of the ESC. Eur Heart J. (2021) 42(5):373–498. doi: 10.1093/eurheartj/ehaa612
2. Kirchhof P. The future of atrial fibrillation management: integrated care and stratified therapy. Lancet. (2017) 390(10105):1873–87. doi: 10.1016/S0140-6736(17)31072-3
3. Lip GY, Lane DA. Stroke prevention in atrial fibrillation: a systematic review. JAMA. (2015) 313(19):1950–62. doi: 10.1001/jama.2015.4369
4. Sepehri Shamloo A, Dagres N, Müssigbrodt A, Stauber A, Kircher S, Richter S, et al. Atrial fibrillation and cognitive impairment: new insights and future directions. Heart Lung Circ. (2020) 29(1):69–85. doi: 10.1016/j.hlc.2019.05.185
5. Li X, Peng S, Wu X, Guan B, Tse G, Chen S, et al. C-reactive protein and atrial fibrillation: insights from epidemiological and Mendelian randomization studies. Nutr Metab Cardiovasc Dis. (2022) 32(6):1519–27. doi: 10.1016/j.numecd.2022.03.008
6. Tanaka M, Imano H, Kubota Y, Yamagishi K, Umesawa M, Muraki I, et al. Serum high-sensitivity C-reactive protein levels and the risk of atrial fibrillation in Japanese population: the circulatory risk in communities study. J Atheroscler Thromb. (2021) 28(2):194–202. doi: 10.5551/jat.54064
7. Li X, Wu X, Chen X, Peng S, Chen S, Zhou G, et al. Selective blockade of interleukin 6 trans-signaling depresses atrial fibrillation. Heart Rhythm. (2023) 20(12):1759–70. doi: 10.1016/j.hrthm.2023.08.026
8. Amdur RL, Mukherjee M, Go A, Barrows IR, Ramezani A, Shoji J, et al. Interleukin-6 is a risk factor for atrial fibrillation in chronic kidney disease: findings from the CRIC study. PLoS One. (2016) 11(2):e0148189. doi: 10.1371/journal.pone.0148189
9. Karataş MB, Durmuş G, Zengin A, Gökalp M, Hayıroğlu Mİ, Çınar T, et al. Association of uric acid albumin ratio with recurrence of atrial fibrillation after cryoballoon catheter ablation. Medicina (Kaunas). (2022) 58(12):1872. doi: 10.3390/medicina58121872
10. Kalenderoglu K, Hayiroglu MI, Cinar T, Oz M, Bayraktar GA, Cam R, et al. Comparison of inflammatory markers for the prediction of atrial fibrillation recurrence following cryoablation. Biomark Med. (2024) 18(17–18):717–25. doi: 10.1080/17520363.2024.2395236
11. Hansen S, Madsen CM, Varbo A, Nordestgaard BG. Low-grade inflammation in the association between mild-to-moderate hypertriglyceridemia and risk of acute pancreatitis: a study of more than 115000 individuals from the general population. Clin Chem. (2019) 65(2):321–32. doi: 10.1373/clinchem.2018.294926
12. Welty FK. How do elevated triglycerides and low HDL-cholesterol affect inflammation and atherothrombosis. Curr Cardiol Rep. (2013) 15(9):400. doi: 10.1007/s11886-013-0400-4
13. Şaylık F, Çınar T, Akbulut T, Hayıroğlu Mİ. Comparison of catheter ablation and medical therapy for atrial fibrillation in heart failure patients: a meta-analysis of randomized controlled trials. Heart Lung. (2023) 57:69–74. doi: 10.1016/j.hrtlng.2022.08.012
14. Yang S, Shen W, Zhang HZ, Wang CX, Yang PP, Wu QH. Effect of PCSK9 monoclonal antibody versus placebo/ezetimibe on atrial fibrillation in patients at high cardiovascular risk: a meta-analysis of 26 randomized controlled trials. Cardiovasc Drugs Ther. (2023) 37(5):927–40. doi: 10.1007/s10557-022-07338-8
15. Rahimi K, Emberson J, McGale P, Majoni W, Merhi A, Asselbergs FW, et al. Effect of statins on atrial fibrillation: collaborative meta-analysis of published and unpublished evidence from randomised controlled trials. Br Med J. (2011) 342:d1250. doi: 10.1136/bmj.d1250
16. Macfarlane PW, Norrie J. The value of the electrocardiogram in risk assessment in primary prevention: experience from the west of Scotland coronary prevention study. J Electrocardiol. (2007) 40(1):101–9. doi: 10.1016/j.jelectrocard.2006.05.003
17. Haywood LJ, Ford CE, Crow RS, Davis BR, Massie BM, Einhorn PT, et al. Atrial fibrillation at baseline and during follow-up in ALLHAT (antihypertensive and lipid-lowering treatment to prevent heart attack trial). J Am Coll Cardiol. (2009) 54(22):2023–31. doi: 10.1016/j.jacc.2009.08.020
18. Lopez FL, Agarwal SK, Maclehose RF, Soliman EZ, Sharrett AR, Huxley RR, et al. Blood lipid levels, lipid-lowering medications, and the incidence of atrial fibrillation: the atherosclerosis risk in communities study. Circ Arrhythm Electrophysiol. (2012) 5(1):155–62. doi: 10.1161/CIRCEP.111.966804
19. Birney E. Mendelian Randomization. Cold Spring Harb Perspect Med. (2022) 12(4):a041302. doi: 10.1101/cshperspect.a041302
20. Skrivankova VW, Richmond RC, Woolf BAR, Yarmolinsky J, Davies NM, Swanson SA, et al. Strengthening the reporting ofobservational studies in epidemiology using Mendelian randomization: the STROBEMR statement. JAMA. (2021) 326(16):1614–21. doi: 10.1001/jama.2021.18236
21. Skrivankova VW, Richmond RC, Woolf BAR, Davies NM, Swanson SA, VanderWeele TJ, et al. Strengthening the reporting of observational studies in epidemiology using Mendelian randomisation (STROBE-MR): explanation and elaboration. Br Med J. (2021) 375:n2233. doi: 10.1136/bmj.n2233
22. Richardson TG, Sanderson E, Palmer TM, Ala-Korpela M, Ference BA, Davey Smith G, et al. Evaluating the relationship between circulating lipoprotein lipids and apolipoproteins with risk of coronary heart disease: a multivariable Mendelian randomisation analysis. PLoS Med. (2020) 17(3):e1003062. doi: 10.1371/journal.pmed.1003062
23. Staerk L, Sherer JA, Ko D, Benjamin EJ, Helm RH. Atrial fibrillation: epidemiology, pathophysiology, and clinical outcomes. Circ Res. (2017) 120(9):1501–17. doi: 10.1161/CIRCRESAHA.117.309732
24. Zhao JH, Stacey D, Eriksson N, Macdonald-Dunlop E, Hedman ÅK, Kalnapenkis A, et al. Genetics of circulating inflammatory proteins identifies drivers of immune-mediated disease risk and therapeutic targets. Nat Immunol. (2023) 24(9):1540–51. doi: 10.1038/s41590-023-01588-w
25. Nielsen JB, Thorolfsdottir RB, Fritsche LG, Zhou W, Skov MW, Graham SE, et al. Biobank-driven genomic discovery yields new insight into atrial fibrillation biology. Nat Genet. (2018) 50(9):1234–9. doi: 10.1038/s41588-018-0171-3
26. Nikpay M, Goel A, Won HH, Hall LM, Willenborg C, Kanoni S, et al. A comprehensive 1,000 genomes-based genome-wide association meta-analysis of coronary artery disease. Nat Genet. (2015) 47(10):1121–30. doi: 10.1038/ng.3396
27. Sanderson E, Glymour MM, Holmes MV, Kang H, Morrison J, Munafò MR, et al. Mendelian randomization. Nat Rev Methods Primers. (2022) 2:6. doi: 10.1038/s43586-021-00092-5
28. Greco MFD, Minelli C, Sheehan NA, Thompson JR. Detecting pleiotropy in Mendelian randomisation studies with summary data and a continuous outcome. Stat Med. (2015) 34(21):2926–40. doi: 10.1002/sim.6522
29. Bowden J, Spiller W, Del Greco MF, Sheehan N, Thompson J, Minelli C, et al. Improving the visualization, interpretation and analysis of two-sample summary data Mendelian randomization via the radial plot and radial regression. Int J Epidemiol. (2018) 47(4):1264–78. doi: 10.1093/ije/dyy101
30. Sedgwick P. Multiple hypothesis testing and Bonferroni’s correction. Br Med J. (2014) 349:g6284. doi: 10.1136/bmj.g6284
31. Wu SA, Kersten S, Qi L. Lipoprotein lipase and its regulators: an unfolding story. Trends Endocrinol Metab. (2021) 32(1):48–61. doi: 10.1016/j.tem.2020.11.005
32. Kersten S. Physiological regulation of lipoprotein lipase. Biochim Biophys Acta. (2014) 1841(7):919–33. doi: 10.1016/j.bbalip.2014.03.013
33. Laufs U, Parhofer KG, Ginsberg HN, Hegele RA. Clinical review on triglycerides. Eur Heart J. (2020) 41(1):99–109c. doi: 10.1093/eurheartj/ehz785
34. Tokgözoğlu L, Libby P. The Dawn of a new era of targeted lipid-lowering therapies. Eur Heart J. (2022) 43(34):3198–208. doi: 10.1093/eurheartj/ehab841
35. Moreno-Vedia J, Llop D, Rodríguez-Calvo R, Plana N, Amigó N, Rosales R, et al. Lipidomics of triglyceriderich lipoproteins derived from hyperlipidemic patients on inflammation. Eur J Clin Invest. (2024) 54(3):e14132. doi: 10.1111/eci.14132
36. Cesena FY, Generoso G, Santos RD, Pereira AC, Blaha MJ, Jones SR, et al. The association between triglyceriderich lipoproteins, circulating leukocytes, and low-grade inflammation: the Brazilian longitudinal study of adult health (ELSA-Brasil). J Clin Lipidol. (2023) 17(2):261–71. doi: 10.1016/j.jacl.2023.01.007
37. Aviles RJ, Martin DO, Apperson-Hansen C, Houghtaling PL, Rautaharju P, Kronmal RA, et al. Inflammation as a risk factor for atrial fibrillation. Circulation. (2003) 108(24):3006–10. doi: 10.1161/01.CIR.0000103131.70301.4F
38. Hayıroğlu Mİ, Şaylık F, Çınar T, Tokgözoğlu L. Meta-analysis of the current research on the relationship between blood lipid levels and the occurrence of atrial fibrillation. Heart Lung Circ. (2023) 32(10):1158–66. doi: 10.1016/j.hlc.2023.08.006
39. Geldenhuys WJ, Lin L, Darvesh AS, Sadana P. Emerging strategies of targeting lipoprotein lipase for metabolic and cardiovascular diseases. Drug Discov Today. (2017) 22(2):352–65. doi: 10.1016/j.drudis.2016.10.007
40. Chen B, Hu R, Min Q, Li Y, Parkinson DB, Dun XP. FGF5 Regulates Schwann cell migration and adhesion. Front Cell Neurosci. (2020) 14:237. doi: 10.3389/fncel.2020.00237
41. Higgins CA, Petukhova L, Harel S, Ho YY, Drill E, Shapiro L, et al. FGF5 Is a crucial regulator of hair length in humans. Proc Natl Acad Sci U S A. (2014) 111(29):10648–53. doi: 10.1073/pnas.1402862111
42. Fang F, Chang RM, Yu L, Lei X, Xiao S, Yang H, et al. MicroRNA-188-5p suppresses tumor cell proliferation and metastasis by directly targeting FGF5 in hepatocellular carcinoma. J Hepatol. (2015) 63(4):874–85. doi: 10.1016/j.jhep.2015.05.008
43. Allerstorfer S, Sonvilla G, Fischer H, Spiegl-Kreinecker S, Gauglhofer C, Setinek U, et al. FGF5 as an oncogenic factor in human glioblastoma multiforme: autocrine and paracrine activities. Oncogene. (2008) 27(30):4180–90. doi: 10.1038/onc.2008.61
44. Ota Y, Saitoh Y, Suzuki S, Ozawa K, Kawano M, Imamura T. Fibroblast growth factor 5 inhibits hair growth by blocking dermal papilla cell activation. Biochem Biophys Res Commun. (2002) 290(1):169–76. doi: 10.1006/bbrc.2001.6140
45. Nelson CP, Goel A, Butterworth AS, Kanoni S, Webb TR, Marouli E, et al. Association analyses based on false discovery rate implicate new loci for coronary artery disease. Nat Genet. (2017) 49(9):1385–91. doi: 10.1038/ng.3913
46. Jeong H, Jin HS, Kim SS, Shin D. Identifying interactions between dietary sodium, potassium, sodium-potassium ratios, and FGF5 rs16998073 variants and their associated risk for hypertension in Korean adults. Nutrients. (2020) 12(7):2121. doi: 10.3390/nu12072121
47. Franceschini N, Carty CL, Lu Y, Tao R, Sung YJ, Manichaikul A, et al. Variant discovery and fine mapping of genetic loci associated with blood pressure traits in Hispanics and African Americans. PLoS One. (2016) 11(10):e0164132. doi: 10.1371/journal.pone.0164132
48. Lu X, Wang L, Lin X, Huang J, Charles Gu C, He M, et al. Genome-wide association study in Chinese identifies novel loci for blood pressure and hypertension. Hum Mol Genet. (2015) 24(3):865–74. doi: 10.1093/hmg/ddu478
49. Vatner SF. FGF Induces hypertrophy and angiogenesis in hibernating myocardium. Circ Res. (2005) 96(7):705–7. doi: 10.1161/01.RES.0000164184.63158.6c
50. Hu Y, Lin L, Zhang L, Li Y, Cui X, Lu M, et al. Identification of circulating plasma proteins as a mediator of hypertension-driven cardiac remodeling: a mediation Mendelian randomization study. Hypertension. (2024) 81(5):1132–44. doi: 10.1161/HYPERTENSIONAHA.123.22504
51. Hanaka H, Hamada T, Ito M, Nakashima H, Tomita K, Seki S, et al. Fibroblast growth factor-5 participates in the progression of hepatic fibrosis. Exp Anim. (2014) 63(1):85–92. doi: 10.1538/expanim.63.85
52. Nattel S, Burstein B, Dobrev D. Atrial remodeling and atrial fibrillation: mechanisms and implications. Circ Arrhythm Electrophysiol. (2008) 1(1):62–73. doi: 10.1161/CIRCEP.107.754564
53. Voigt N, Heijman J, Wang Q, Chiang DY, Li N, Karck M, et al. Cellular and molecular mechanisms of atrial arrhythmogenesis in patients with paroxysmal atrial fibrillation. Circulation. (2014) 129(2):145–56. doi: 10.1161/CIRCULATIONAHA.113.006641
Keywords: lipid-lowering drugs, LPL agonist, atrial fibrillation, circulating inflammatory factors, fibroblast growth factor 5, drug treatment, Mendelian randomization study
Citation: Ou G, Zhang Y, Cai H, Yao K, Qiu Z, Chen Y, Yang Y, Chen Q and Chen X (2024) Lipid-lowering drugs, circulating inflammatory factors, and atrial fibrillation: a mediation Mendelian randomization study. Front. Cardiovasc. Med. 11:1446610. doi: 10.3389/fcvm.2024.1446610
Received: 10 June 2024; Accepted: 14 October 2024;
Published: 5 November 2024.
Edited by:
Martina Nesti, Toscana Gabriele Monasterio Foundation, ItalyReviewed by:
Rui Providencia, University College London, United KingdomMert İlker Hayıroğlu, Dr. Siyami Ersek Chest Cardiovascular Surgery Training and Research Hospital, Türkiye
Copyright: © 2024 Ou, Zhang, Cai, Yao, Qiu, Chen, Yang, Chen and Chen. This is an open-access article distributed under the terms of the Creative Commons Attribution License (CC BY). The use, distribution or reproduction in other forums is permitted, provided the original author(s) and the copyright owner(s) are credited and that the original publication in this journal is cited, in accordance with accepted academic practice. No use, distribution or reproduction is permitted which does not comply with these terms.
*Correspondence: Qingyang Chen, NjIzNDMzNzQ0QHFxLmNvbQ==; Xinyu Chen, Y3h5c215eEAxNjMuY29t