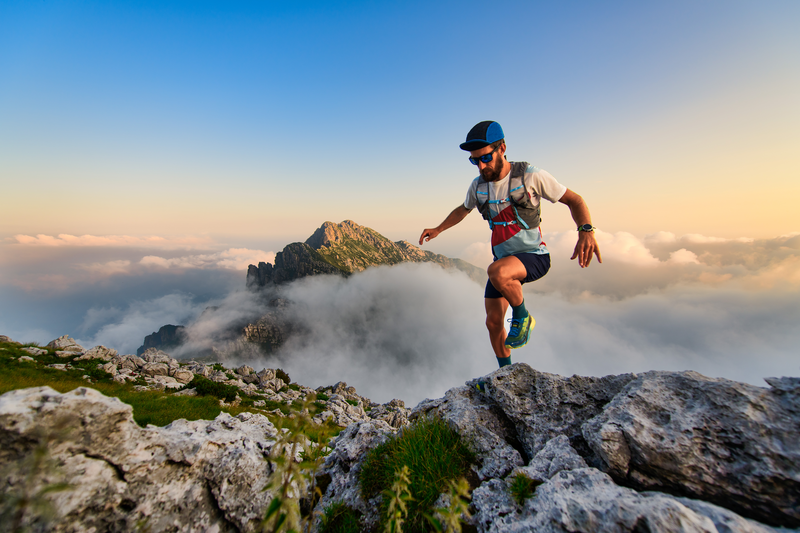
94% of researchers rate our articles as excellent or good
Learn more about the work of our research integrity team to safeguard the quality of each article we publish.
Find out more
ORIGINAL RESEARCH article
Front. Cardiovasc. Med. , 18 October 2024
Sec. General Cardiovascular Medicine
Volume 11 - 2024 | https://doi.org/10.3389/fcvm.2024.1435038
Background: COVID-19 vaccines are well tolerated and effective but may have adverse effects on the cardiovascular system. Vaccine-associated myocardial injury was analysed by measuring high-sensitive troponin T (hsTnT); mid-regional pro-adrenomedullin (MR-proADM) levels were evaluated to assess endothelial dysfunction.
Methods: This was a prospective study with a vulnerable population of healthcare workers (HCWs) and elderly patients (>70 years) who were vaccinated with either one dose of ChAdOx1 nCov-19 adenoviral vector vaccine (AZ) followed by one dose of the BNT162b2 messenger RNA vaccine (BNT), or with two doses of BNT (12th of January - 30th of November 2021). HsTnT and MR-proADM were measured in blood samples at three visits (V1: 1st immediately before vaccination; V2, 3: 3–4 weeks after 1st and 2nd vaccination). HsTnT of HCWs was compared to a healthy reference population.
Results: N = 162 volunteers were included (V1 = 161; V2, V3 = 162 each). N = 74 (45.7%) received AZ/BNT and n = 88 (54.3%) received BNT/BNT [elderly: n = 20 (12.3%), HCWs: n = 68 (42.0%)]. Median hsTnT levels were 4 ng/L, 5 ng/L and 4 ng/L (V1-V3) for AZ/BNT and at 5 ng/L, 6 ng/L and 6 ng/L (V1-V3) for BNT/BNT. Compared to the reference population (n = 300), hsTnT was significantly higher at all visits for both vaccination groups (p < 0.01), without differences between the AZ/BNT and BNT/BNT cohort. Median MR-proADM values were 0.43 nmol/L, 0.45 nmol/L, 0.44 nmol/L (V1-V3) in the AZ/BNT cohort and 0.49 nmol/L, 0.44 nmol/L, 0.47 nmol/L for BNT/BNT, respectively. Change of median hsTnT and MR-proADM between visits did not show significant increases. One HCW experienced a permanent and three a transient hsTnT increase ≥14 ng/L.
Conclusion: No overall subtle, persistent cardiovascular involvement was observed after the 2nd COVID-19 vaccination. Elevated cardiovascular biomarkers in clinically asymptomatic individuals need further investigations.
Graphical Abstract. Summary of the vaccination scheme and visiting points in the study population (of HCWs and seniors >70 years) between the 12th of January and the 30th of November 2021. The results showed no overall subtle, chronic myocardial or vascular involvement in our COVID-19 vaccinated cohorts. AZ ChAdOx1 nCov-19 adenoviral vector vaccine from Astra Zeneca, BNT BNT162b2 messenger ribonucleic acid vaccine from BioNTech, EDTA Ethylenediaminetetraacetic acid, HCWs health care workers, hsTnT high-sensitive troponin T, mid-regional pro-adrenomedullin, V1-V3 visiting times 1–3, w week(s).
Coronavirus disease 2019 (COVID-19) vaccines are generally safe and effective in reducing the severity of SARS-CoV-2 infections and their complications (1, 2). About 70.6% of the world's population has received at least one vaccine dose, and over 13 billion doses have been administered worldwide (3). To ensure the safety of COVID-19 vaccines, short- and long-term adverse events are continuously monitored by active and passive pharmacovigilance and post-marketing studies and vaccine safety is intensely studied (4, 5). The International Coalition of Medicines Regulatory Authorities, representing 38 regulatory agencies, recently issued a joint statement reassuring that safety data shows that COVID-19 vaccines have a very good safety profile in all age groups, including children and people with underlying medical conditions (6). Rare adverse events include complications of the cardiac system, like COVID-19 vaccine-associated myocarditis and pericarditis, as well as adverse effects on the vasculature, such as an impaired endothelial function as well as arterial and venous thrombosis (7–10).
Although rare, myo- and pericarditis are the most frequent adverse cardiac events following COVID-19 vaccinations (11, 12) and were first reported in April 2021 (12). Today, incidence of myo- and pericarditis is (region-specifically) estimated at 1–11 per 100,000 vaccinees (12, 13). Incidence is highest among young adult males, particularly following the second dose of Moderna (MOD) or BioNTech/Pfizer (BNT) messenger ribonucleic acid (mRNA) vaccine (12). In patients with mRNA-vaccine related myo- and/or pericarditis, cardiomyocyte necrosis was almost always detectable, as determined by measurements of the cardiac-specific biomarker high-sensitive troponin T (hsTnT) (14, 15). For instance, in a study by Oster et al., elevated hsTnT was observed in 97.9% (792/809) of COVID-19 vaccine-associated cases of myo- and/or pericarditis, as well as further major adverse cardiac events (14). Hence, hsTnT measurement is of high relevance for the diagnostic identification of patients with vaccine-related cardiac adverse events.
So far, cardiomyocyte injury in COVID-19 vaccine recipients was primarily analysed in study populations that presented with clinically manifest and acute cardiac symptoms (7). Yet, it has rarely been investigated if and to what extent a subtle, chronic post-vaccine myocardial involvement occurs in a vaccinated population even in the absence of typical symptoms. Mainly three studies actively monitored hsTnT levels in a BNT-vaccinated cohort. Mansanguan et al. analysed 301 adolescents of whom four had signs of a subclinical myocarditis, one suffered a clinically manifest myopericarditis and two a pericarditis after the 2nd BNT162b2 vaccine. Here, cardiac biomarkers were measured at baseline, day 3, 7 and 14 (optionally) (16). Levi and colleagues observed vaccine-associated cardiomyocyte injury in two participants (0.6%), with one being symptomatic and one asymptomatic, 2–4 days after having received their 4th BNT dose (17). Lastly, Buergin et al. detected the highest rate of 2.8% (women: 20/777; men: 2/777) in their hospital employee cohort presenting with a vaccine-induced myocardial injury on day 3 after receipt of MOD mRNA booster (18). All three studies described cardiac involvement as mild and transient and mainly defined it as an acute hsTnT elevation above the 99th percentile of upper reference limit (URL) (16, 18, 19). Electrocardiograms and echocardiography were normal in the majority of affected participants (17, 18).
In order to further strengthen safety data and confidence in COVID-19 vaccines, additional research is warranted to assess possible subtle and subclinical myocardial damage after COVID-19 vaccination, also with regards to varying vaccination regiments (vector vs. mRNA or heterologous vaccination). Furthermore, as emphasised in a recent editorial by Levi et al., longitudinal observations of hsTnT levels over time, e.g., including pre-vaccination status and follow-up samples after booster vaccination, would be of interest to evaluate if any occurring subclinical cardiac injury is likely self-limiting or becomes chronic (17).
Mainly one study has investigated possible acute effects of COVID-19 vaccines on the vascular system (20). The authors observed a short-term deterioration of endothelial function in the first 24 h after the 2nd BNT vaccine dose. The extent of endothelial involvement was described as far lower than in COVID-19, which in turn has been linked to endothelial cell infection and endotheliitis (20). In COVID-19 patients, the biomarker mid-regional pro-adrenomedullin (MR-proADM), an endothelium-related peptide with vasodilatory properties, was used to evaluate the severity of endothelial dysfunction (21). Measurements of MR-proADM levels to assess possible short- and long-term endothelial injury in COVID-19 vaccine recipients have not been analysed so far.
By conducting a prospective observational study, we investigated subacute, subtle, and subclinical cardiomyocyte injury in two risk populations with follow-ups until 4 weeks (optionally longer) after completion of the original COVID-19 basic vaccination regimen. We hypothesise that hsTnT levels remain unchanged during follow-up, indicating no significant rise in myocardial injury in the short- and long-term compared to baseline. Secondly, we sought to analyse endothelial function based on levels of MR-proADM throughout the study period. With these two approaches, we aimed to address current knowledge gaps concerning chronic cardiovascular effects of COVID-19 vaccination.
The participants were recruited within the multicentre prospective observational studies, EICOV, COVIMMUNIZE and COVIM and consisted of healthcare workers (HCWs) of Charité – Universitätsmedizin Berlin as well as elderly/senior patients (>70 years) at a general practitioner's office in Berlin, Germany; representing two different types of risk populations in the pandemic. While vulnerability of HCWs arises from their regular exposure to infectious patients, the elderly mainly belong into this category due to their compromised immune response and multimorbidity (22, 23). Thus, in the following, this group will be named “vulnerable population”. All participants received their first two COVID-19 vaccinations between the 12th of January and the 22nd of June 2021 with either a combination of AZ (AstraZeneca)/BNT (only for HCWs) or BNT/BNT (HCWs or elderly). Booster vaccinations (3rd and 4th) were only mRNA-based (BNT or MOD). Key inclusion criteria for our initial observational studies were the ability to give written informed consent by the participants or via their legal representative, no contraindications to receiving a COVID-19 vaccination and an age of ≥18 years at the time of enrolment. Out of our initial studies, 162 study participants were selected for this investigation with the following criteria: (a) availability of sufficient samples from the V1-V3 visits for analyses carried out in this specific investigation, (b) a balanced selection of male and female study participants, (c) a balanced selection of homologous (BNT/BNT) and heterologous (AZ/BNT) vaccination regimen (that does not apply for the elderly, since this cohort entirely received a homologous vaccination regimen) (d) no patient-reported infection with SARS-CoV-2, negative anti-SARS-CoV-2 spike immunoglobulin G (IgG) antibodies at baseline and negative anti-SARS-CoV-2 nucleocapsid antibodies at baseline and during follow-up (measured by SeraSpot), and no positive nucleic acid amplification test (NAAT) during weekly screening from V1-V3. Exclusion criteria for this investigation were (a) insufficient sample remaining for analysis and (b) infection with SARS-CoV-2 before enrolment or during follow-up until V3, determined by patient history and SeraSpot Anti-SARS-CoV-2 IgG microarray-based immunoassay including nucleocapsid and spike as antigens (Seramun Diagnostica GmbH).
Three main visit timepoints (V1-V3) were conducted. V1: at baseline, i.e., 7 to 0 days before the 1st vaccination; V2: 3–4 weeks after the 1st vaccination and V3: 3–4 weeks after the 2nd vaccination (Figure 1). Previous work on this topic has shown that cardiovascular biomarkers, if elevated in the acute setting, usually rise and fall within 1–2 weeks after vaccinations. By choosing an interval of 3–4 weeks post-vaccination for biomarker measurement, we aimed to assess subacute or persistent hsTnT elevation (16). Participants that opted to participate in the follow-up study COVIM-Boost, had a 4th visit (V4) and 5th visit (V5) captured, which is reported here for HCWs as part of an ancillary analysis up to 50 days after the 3rd and 4th vaccination (with either BNT or MOD), respectively (Supplementary Figure S1). At all visits, serum and ethylenediaminetetraacetic acid (EDTA) blood samples were collected. MR-proADM was measured in EDTA plasma [nmol/L] on the Kryptor Compact Plus device (Thermo Fisher Scientific, Waltham, MA, USA). The clinically relevant cut-off was set at ≥0.75 nmol/L (24). Depending on the sample availability, EDTA or serum samples were used for measuring hsTnT (via Cobas e801, Roche Diagnostics, Mannheim, Germany). In the diagnostic exclusion procedure of a myocardial infarction, relevant hsTnT levels are defined as being >the 99th percentile of URL (25). This definition was also adopted by prior studies with a research question similar to ours (16–18). However, in a study by Barbier et al. the authors pointed out that new, subclinical cardiomyocyte injury, detected in late enhancement magnetic resonance imaging, may only be reflected in minor troponin elevations (of 4.1 ng/L–5.9 ng/L) and slight increases also correlate with a negative prognosis (26). Additionally, in cases of SARS-CoV-2 infections with cardiac involvement, troponin rise was likewise mostly mild (27). Therefore, to also capture a possibly subtle but relevant troponin increase, we focused on all changes in hsTnT levels in the course of the visits and put it in relation to the initial baseline hsTnT value of the respective participant. Cases with a hsTnT elevation > the 99th percentile cut-off value were presented and interpreted separately. HsTnT levels below the limit of detection (3 ng/L), were set to 2.9 ng/L for analyses.
Figure 1. Study population diagram. Recruitment of vulnerable study population with cohort 1 including health care workers and cohort 2 including elderly general practitioner patients. Vaccinations with either a heterogenous combination of AZ/BNT or a homologous scheme of BNT/BNT were applied and three main visits V1 to V3 were performed. *One hospital employee of the BNT/BNT cohort did not participate in the enrolment visit V1 but received both BNT vaccinations regularly and took part in the visits V2, V3 and is, therefore, included in this study. AZ ChAdOx1 nCov-19 adenoviral vector vaccine from Astra Zeneca, BNT BNT162b2 messenger ribonucleic acid vaccine from BioNTech, V1-V5 visiting times 1-5, w week(s).
Demographic and clinical characteristics of all participants, including age and sex, comorbidities and pre-medication were collected at enrolment and used for comparison with the reference cohort.
As a reference population, participants of the BIC-1 (Biomarkers in Cardiology 1) study were used. This included HCWs of Charité – Universitätsmedizin Berlin that were recruited between the 6th of July 2007 and the 5th of November 2007. Clinical exclusion criteria were the presence of a heart failure, other heart diseases, kidney or metabolic diseases and the intake of permanent medication. Therefore, this cohort was defined as a presumably healthy reference and was used for comparison of hsTnT values with our HCWs of the vaccination groups. Further inclusion criteria were a full employment contract, age of 18 years or older and no direct dependence on the study leader. In this cohort, one visit (VR−1) was performed. HsTnT was measured in lithium-heparin blood samples on the same analyser module as in our vaccination cohorts (Cobas e801, Roche Diagnostics, Mannheim, Germany).
The primary hypothesis was that the distribution of hsTnT remains unchanged across all vaccination groups at the visits V1 to V3. Therefore, we assumed that neither the combination of AZ/BNT, nor the BNT/BNT scheme would lead to a significant elevation of hsTnT compared to baseline levels at enrolment visit V1. Secondly, we expected no relevant chronic endothelial dysfunction to be detected in the sense of a significant median difference between V1 to V3 of MRpro-ADM values in the observed time span.
A basic description of the vaccination and reference groups was performed, including characteristics of sex, age, Body-Mass-Index (BMI), smoking status, comorbidities, and pre-medication at enrolment. If not indicated otherwise, metric values are presented as median with the associated interquartile range (IQR). Due to the skewed distribution of hsTnT and MR-proADM values, non-parametric tests were performed. The Mann-Whitney U test was applied to test the difference of hsTnT and MR-proADM levels between the sexes and vaccination groups at single visits, and the Wilcoxon signed rank test to investigate potential signals of individual change between the visits for both biomarkers (differences V2-V1, V3-V1, V3-V1), which is in the following demonstrated as box plots. Correlation analyses were performed separately for sex between hsTnT and MR-proADM at each visit, and for both hsTnT and MR-proADM with age at V1; Spearman's correlation coefficients were reported. Comparisons of hsTnT values between the reference group and the two vaccination cohorts (solely HCWs included here) were performed with the Kruskal-Wallis-test and pairwise post-hoc tests with Bonferroni correction. Linear mixed-effects models were calculated to examine effects of the type of COVID-19 vaccination on the course of hsTnT and MR-proADM values adjusted for age (per 10 years) and sex, with random effects for the intercept. Since most visits were performed within a very close time window, the course of hsTnT and MR-proADM values were further explored in alternative linear mixed-effects regression models treating the visits as repeated measurements, again including random effects for the intercept.
All analyses were performed using IBM SPSS Version 29 (IBM Corporation, Armonk, NY, USA) and R version 4.3.1 (2023-06-16 ucrt). A p-value <0.05 was considered significant; for pairwise subgroup comparisons or parallel tests in visits V1 to V3, p-values were adjusted for multiple testing.
The COVIM study (EA4/245/20), its preceding studies EICOV (EA4/245/20) and COVIMMUNIZE (EA4/244/20), the follow-up study COVIM-Boost (EA4/261/21) and the BIC-1 study (EA2/030/07) were approved by the ethics committee of Charité – Universitätsmedizin Berlin. BIC-1 was registered in the German register for clinical studies (DRKS-ID DRKS00000310) and COVIM in the European clinical trial register (EudraCT-2021–001512–28).
Information on basic characteristics is provided in Table 1 and Supplementary Table S1. In total, the study population consisted of 162 people in the vaccination group, including 142 (87.7%) HCWs and 20 (12.3%) elderly participants (solely in the BNT/BNT group). Except for one person who missed the 1st visit, all participants were seen (at least) three times. Altogether, 74 (45.7%) received the combination of AZ/BNT, while 88 (54.3%) participants were vaccinated according to the regular BNT/BNT regimen. In the AZ/BNT group, 41 (55.4%) participants and in the BNT/BNT population 49 (55.7%) were female, respectively. The median age was 33.2 years in the AZ/BNT and 37.6 years in the BNT/BNT group. Median BMI was 23.9 kg/m2 and 23.8 kg/m2, respectively. Out of 148 available answers, 16 reported being either former or current smokers.
With regard to pre-medication, 9 (5.6%, 7/9 from the BNT/BNT cohort) reported receiving immunosuppressive therapy and 4 (2.5%, 3/4 from the BNT/BNT group) participants reported taking corticosteroids at enrolment. Furthermore, 14 (8.6%) participants reported taking antihypertensive drugs, 3 (1.9%) anticoagulants, 6 (3.7%) statin treatment and 4 (2.5%) antiplatelet drugs as a permanent medication; the majority of participants on this medication belonging to the elderly group (see Table 1).
Four (5.4%) participants in the AZ/BNT cohort, and 17 (19.3%) participants in the BNT/BNT cohort reported a cardiovascular disease at baseline, with hypertension (AZ/BNT: 4/74 (5.4%); BNT/BNT: 16/88 (18.2%)) as the most frequent condition. Chronic lung diseases were present in 10 (6.2%) participants, with 4 (5.4%) being in the AZ/BNT and 6 (6.8%) participants in the BNT/BNT cohort. Other comorbidities, with 7 cases each (4.3%), included kidney disease (6/7 BNT/BNT), rheumatologic/immunological diseases (4/7 BNT/BNT), and cancer (5/7 BNT/BNT). Diabetes, dementia, cerebrovascular or chronic haematological diseases were present in 5 (3.1%) cases, or less.
The reference population included 300 individuals with a 1:1 female to male ratio. The median age was 39.0 years. For all 300 participants, hsTnT values were available at VR−1 for analysis. Comorbidities and pre-medication are not reported for this cohort since both were exclusion criteria (Table 1).
The median values of hsTnT in the AZ/BNT group were 4 ng/L (IQR 4–6), 5 ng/L (IQR 4–6) and 4 ng/L (IQR 4–6) for V1, V2, V3, and for the BNT/BNT cohort 5 ng/L (IQR 4–8), 6 ng/L (IQR 4–9) and 6 ng/L (4–8.5), respectively. Separated by HCWs and elderly (BNT/BNT), the seniors showed higher median values of 18 ng/L (IQR 11–29), 15 ng/L (IQR 10.5–25.5) and 13.5 ng/L (IQR 10–22.5) during follow-up visits. HsTnT levels at V1-V3 in the AZ/BNT group were lower compared to the BNT/BNT cohort at all visits, including at baseline (pV1 = 0.048, pV2 = 0.003, pV3 < 0.001). The individual progression for V1-V3 is visualised in Figure 2A, separated by vaccination regimen and sex. Median hsTnT in the reference population was 3.6 ng/L (IQR 3.0–4.9, Figure 3). For all HCWs, median hsTnT values were higher in males at all visits, including baseline (V1: p = 0.010; V2: p = 0.003; V3: p = 0.006). Changes of median hsTnT values were 0 ng/L (IQR −1.0 to 1.0; V2-V1), 0 ng/L (IQR −1.0 to 0.0; V3-V2) and 0 ng/L (IQR −1.0 to 0.0; V3-V1) in the AZ/BNT cohort (Figure 2B). In the BNT/BNT population, changes were 0 ng/L (IQR 0.0 to 1.0; V2-V1), 0 ng/L (IQR −1.0 to 1.0; V3-V2) and 0 ng/L (IQR −1.0 to 1.0; V3-V1). There were no individual differences of hsTnT levels between all visits (AZ/BNT: V2-V1 p = 1.0; V3-V2 p = 0.25; V3-V1 p = 0.42; BNT/BNT: V2-V1 p = 0.24; V3-V2 p = 0.25; V3-V1 p = 1.0). Median changes of hsTnT for HCWs and elderly of the BNT/BNT cohort separately showed significant differences between V2 and V1 for HCWs [median 0 ng/L (IQR 0.0 to 1.0), p = 0.029] and between V3 and the two prior visits for elderly (V3-V2: median −1 ng/L (IQR −4.0 to 0.0), p = 0.038; V3-V1: median −2 ng/L (IQR −4.0 to 0.0), p = 0.008) were visible (Figure 2C).
Figure 2. (A) hsTnT values at the three main visiting points for participants of the AZ/BNT and BNT/BNT group (HCWs and elderly), separated by sex. The red dashed line indicates the 14 ng/L threshold. (B) Box plots of the change of hsTnT values between each visiting time (V1-V2, V1-V3, V1-V3), separated by AZ/BNT and BNT/BNT group. (C) Box plots of the change of hsTnT values between each visiting time (V1-V2, V1-V3, V1-V3), separated by AZ/BNT, HCW BNT/BNT and senior BNT/BNT group. AZ ChAdOx1 nCov-19 adenoviral vector vaccine from Astra Zeneca, BNT BNT162b2 messenger ribonucleic acid vaccine from BioNTech, hsTnT high-sensitive troponin T, V1-V3 visiting times 1-3.
Figure 3. Box plots of hsTnT levels of the reference group (orange) compared to the AZ/BNT group (blue) and the BNT/BNT group (HCWs: green; elderly: red) at V1 to V3. The red dashed line indicates the 14 ng/L threshold. AZ ChAdOx1 nCov-19 adenoviral vector vaccine from Astra Zeneca, BNT BNT162b2 messenger ribonucleic acid vaccine from BioNTech, hsTnT high-sensitive troponin T, V1-V3 visiting times 1-3.
Four HCW cases stand out regarding their individual hsTnT levels and are therefore reported separately in detail (Figure 2A). One BNT/BNT participant (“w”, male, in his 30s, HCW) showed a steady hsTnT rise with values of 15 ng/L, 21 ng/L and 25 ng/L (V1-V3). At enrolment, he reported having had muscle pain and fever in the 7 days prior. At a follow-up visit 6 months after V1, he stated having suffered from headache in the 7 days before presenting. Generally, no pre-existing medical conditions were reported by this participant. A second participant “x” (male, in his 20 s, HCW, BNT/BNT), had a noticeable but transient hsTnT elevation at V2 and returned to normal at V3 (7 ng/L, 17 ng/L, 5 ng/L). He reported the following baseline conditions: hypertensive heart disease, ulcer duodeni and vascular encephalopathy. Secondly, in the AZ/BNT population, two participants presented with a transient hsTnT elevation (participant “y” (male, in his 20 s): 8 ng/L, 14 ng/L, 5 ng/L; participant “z” (male, in his 20 s): 6 ng/L, 17 ng/L, 6 ng/L). Both did not report any symptoms, pre-medication or comorbidities.
In total, 16 study participants had at least one absolute hsTnT value > the 99th percentile of URL. Out of these, four participants presented with a normal baseline hsTnT value, while the other 12 participants showed levels of ≥14 ng/L already at enrolment, prior to COVID-19 vaccination (Figure 2A).
HsTnT levels in the reference cohort were significantly lower than in the AZ/BNT and BNT/BNT group at all three time points (at V1 and V2 compared to both vaccination groups: p < 0.001; at V3: for VR−1-AZ/BNT: p < 0.001 and for VR−1-BNT/BNT: p = 0.006) (Figure 3).
The MR-proADM median value was 0.43 nmol/L (IQR 0.39–0.47), 0.45 nmol/L (IQR 0.39–0.50) and 0.44 nmol/L (IQR 0.38–0.52) (V1-V3) in the AZ/BNT population. In the BNT/BNT group, median MR-proADM levels were 0.49 nmol/L (IQR 0.42–0.63), 0.44 nmol/L (IQR 0.40–0.55) and 0.47 nmol/L (IQR 0.41–0.64), respectively (Figure 4A). The comparison of MR-proADM between the vaccination groups revealed a significant difference at V1 and V3 (V1: p < 0.001; V2: p = 0.336; V3: p = 0.032). Generally, 17 participants (AZ/BNT: n = 2; BNT/BNT: n = 15) had at least one MR-pro ADM value > 0.75 nmol/L. Median changes of MR-proADM levels between the visits V1 and V2 were 0.01 nmol/L (IQR −0.02 to 0.06; AZ/BNT) and −0.02 nmol/L (IQR −0.05 to 0.02; BNT/BNT), with a small but significant difference between the vaccination groups (p = 0.014). Between V2 and V3 median values differed at −0.02 nmol/L (IQR −0.05 to 0.04; AZ/BNT) and 0.01 nmol/L (IQR −0.06 to 0.06; BNT/BNT) and median changes between the study visits V1 and V3 were 0.01 nmol/L (IQR −0.03 to 0.06; AZ/BNT) and 0.00 nmol/L (IQR −0.05 to 0.04; BNT/BNT), without statistical significance (Figures 4B,C). One participant presented with a striking rise in MR-proADM levels during the study course (BNT/BNT group, in his 80s, male; V1: 1.1 nmol/L, V2: 1.1 nmol/L, V3: 1.5 nmol/L) (Figure 4A). He reported one cardiovascular comorbidity (hypertension) as well as an active cancer disease (Hodgkin lymphoma).
Figure 4. (A) MR-proADM values at the three main visiting points for participants of the AZ/BNT and BNT/BNT group (including HCWs and elderly), separated by sex. The red dashed line indicates the 0.75 nmol/L threshold. (B) Box plots of the change of MR-proADM values between each visiting time (V1-V2, V1-V3, V1-V3). (C) Box plots of the change of MR-proADM values between each visiting time (V1-V2, V1-V3, V1-V3), separated by AZ/BNT, HCW BNT/BNT and senior BNT/BNT group. AZ ChAdOx1 nCov-19 adenoviral vector vaccine from Astra Zeneca, BNT BNT162b2 messenger ribonucleic acid vaccine from BioNTech, MR-proADM mid-regional pro-adrenomedullin, V1-V3 visiting times 1-3.
Correlation between hsTnT and MR-proADM values was assessed for all three visiting times (Supplementary Figure S4A). The Spearman's correlation coefficient was low in both males (V1: r = 0.36; V2: r = 0.08; V3: r = 0.38) and females (V1: r = 0.55; V2: r = 0.32; V3: r = 0.57), with a tendency to even lower correlation at V2. A stronger correlation was seen between hsTnT levels and age (female: r = 0.69; male: r = 0.45) as well as MR-proADM and age (female: r = 0.67; male: r = 0.56) in female participants (Supplementary Figures 4B,C).
Mixed-effect models were calculated for HCWs to identify factors that are associated with MR-proADM and hsTnT values over time, with the first vaccination as the anchor defining time 0 (Figures 2A, 4A). Sex, age (per 10 years), and the type of COVID-19 vaccine were used as independent variables. Results showed a significant effect of male sex and increasing age, but not the type of vaccine on both hsTnT and MRproADM levels. There was no linear effect over time, but when ignoring shifts in time of lab measurements relative to the first vaccination and treating the visits as repeated measurements, only V2 showed a significant increase compared to V1 with 0.41 ng/L (95%CI 0.12 to 0.70, p = 0.005); no difference between V3 and V1 (β = 0.13 ng/L, 95% CI −0.15 to 0.42, p = 0.356) was detected. When considering interaction, there was no interaction between this time effect and the type of vaccination, with the general time effect disappearing. For MRproADM, no significant effects except for age were seen (Table 2).
Table 2. Mixed-effects regression models for the course of hsTnT and MR-proADM in HCWs with visits as repeated measurements and random effects for the intercept. All other variables are treated as fixed effects.
Altogether in HCWs, 25 V4 visits after the 3rd vaccination and 9 V5 visits after the 4th vaccination were registered. Details are listed in Supplementary Figure S1. HsTnT median levels were 4 ng/L (V4) and 3 ng/L (V5), while median MR-proADM values were 0.46 nmol/L (V4) and 0.54 nmol/L (V5). The hsTnT and MR-proADM progression for all visits V1 to V5 is visualised in Supplementary Figures S2A and S3. Furthermore, we performed a post SARS-CoV-2 breakthrough infection measurement of hsTnT and MR-proADM up to 50 days after the infection. Participants with a reported breakthrough infection during the study course are marked in orange (Supplementary Figure S2B).
In this prospective observational study, we assessed if subtle, subacute and persistent myocardial involvement is detectable in a vulnerable population of HCWs and elderly patients following basic vaccination against COVID-19 with either an AZ/BNT or a BNT/BNT vaccination regimen. With scheduled follow-up visits, the biomarkers hsTnT and MR-proADM were measured at enrolment and at 3–4 weeks after the 1st and the 2nd vaccination, respectively.
Regarding baseline hsTnT values (V1), the HCW vaccination cohort had a median hsTnT level of 4–5 ng/L (IQR 4–6), whilst the reference population presented with a slightly lower median of 3.6 ng/L (IQR 3.0–4.9). As visible in our post-hoc pairwise comparisons, this difference in hsTnT at V1 was significant. As V1 was performed before or right at the time of the 1st vaccination, this difference in baseline hsTnT values must be viewed as an indicator of pre-existing differences in patient characteristics. For instance, this observation may be explained by the fact that the reference cohort did not include any participants with cardiological, nephrological or endocrinological diseases and were, therefore, presumably healthy, whereas in our study cohort, pre-existing diseases were not an exclusion criterion. Therefore, our results underline that the HCW group, if not preselected otherwise, represents a cohort that is working in healthcare, is <65 years of age, but is otherwise not free of pre-existing illnesses. Furthermore, hsTnT values in our BNT/BNT senior group were comparatively high with a median of 18 ng/L (IQR 11–29), with 10 out of 20 elderly participants already having an elevated hsTnT level > the 99th percentile of URL at baseline V1. This is in accordance with common literature, indicating that increasing age accounts for higher troponin levels (28, 29), either as an independently increasing variable and/or as a sign for an underlying (silent) disease. This was also reproduced in our correlation analysis. For instance, Orlev and colleagues reported a median hsTnT at 14 ng/L in nursing home patients at an age of >70 years even though the study population was described as asymptomatic (28). Self-evidently, when discussing slight troponin differences, analytical imprecision of hsTnT measurement, especially in values below the 99th percentile of URL, must also be considered, besides the mentioned biological variability (30, 31).
With regard to hsTnT time kinetics, median hsTnT values of all participants remained almost stable at 5 ng/L for V1, V2 and V3. When specifically analysing the vaccination cohorts stratified by vaccine type and age, changes of median hsTnT over time were partially statistically significant, however, median absolute differences were still at 0 ng/L for HCWs, indicating that these changes are not of clinical relevance. For the elderly, absolute changes were −1 ng/L and −2 ng/L, when comparing visit 3 to the prior visits, indicating, if anything, rather a slight post-vaccination decrease. In the group of medical personnel, four male participants stood out with a pronounced troponin elevation. All of them were young to mid-aged males, fitting the typical group of vaccine recipients with rare vaccine-related myo- and/or pericarditis (12). Participant “w” who showed a transient hsTnT rise to 17 ng/L at V2, did not report any corresponding symptoms. He had a prior known hypertensive heart disease. Here, the troponin elevation could be interpreted as a typical transient, subclinical myocarditis but without chronic residuals. Participant “x” presented with a steady increase in hsTnT levels up to 25 ng/L at V3. However, no cardiovascular comorbidity was stated by him before enrolment. Therefore, the increase in hsTnT may either be a result of a yet unknown underlying cardiac disease and/or it may be interpreted as a subtle post-vaccine myocardial injury. The same hypothesis is assumed for persons “y” and “z”. In all cases, the hsTnT elevation was already detected after the 1st BNT vaccination (V2) and hsTnT elevation was transient in all cases except for one. Those with a transient hsTnT rise returned to baseline levels or lower. The similar incidence of cardiac injury biomarker elevation following mRNA- and vector vaccines, is not in agreement with prior studies showing cardiomyocyte injury primarily after mRNA vaccination (12). Also, our findings partly deviate from the literature, which reported rare cardiomyocyte damage in the context of myocarditis and/or pericarditis, mainly after the 2nd vaccine dose (12). When taking a look at the individual median troponin values of the BNT/BNT vaccinated elderly, it is noticeable that all 10 seniors with high absolute hsTnT levels in the course of the study period (V2, V3), already showed a relevant troponin elevation of ≥14 ng/L at the enrolment visit. Apart from this, no relevant vaccine-induced additional hsTnT elevation was detectable until V3 among the elderly cohort. Therefore, our results support the notion that no overall subtle, subacute cardiomyocyte injury can be observed in this prospective cohort in temporal relation to the performed COVID-19 vaccinations. This appears to be the case irrespective of vaccination regimen, as indicated by our pairwise comparisons that showed no significant differences of hsTnT levels between the AZ/BNT and BNT/BNT groups (HCW sub-group) at V1 to V3.
Existing studies on hsTnT and myocardial injury have so far been performed either on HCWs or adolescents (16–18). Thus, previous studies are mainly comparable to the hsTnT results of our HCW sub-cohort, since the baseline characteristics of our elderly group differ strongly. Mansanguan and colleagues reported a relevant cardiac biomarker elevation in 7/301 (2.3%) cases, including 5 (1.6%) with cardiac troponin T ≥ 14 ng/L out of which 4 (1.3%) were described as occurrences of subclinical myocarditis with initially normal hsTnT levels, followed by a post-vaccine elevation that peaked at day 7 (16). These participants were between 13 and 17 years old; an age range that was not covered in our work, but according to the literature, includes most of the post-COVID-19-vaccine-associated myocarditis cases (12). However, for those 4 adolescents with subclinical troponin elevations, the researchers did not provide follow-up measurements at day 14 (16). Therefore, it is not possible to evaluate whether troponin rise persisted or declined with time. In general, the study objective, participant age and observation span differed largely from our work, making a direct comparison difficult. Regarding participant characteristics, our cohort is most similar to the one studied by Levi et al. who recruited 324 HCWs of whom 22 showed a hsTnT above the sex-specific 99th percentile at baseline and 27 showed elevated hsTnT within 2–4 days post-vaccination (4th BNT162b booster) (17). However, the authors primarily focused on 2 participants who additionally showed a hsTnT rise of >50% compared to enrolment measurements and interpreted this as vaccine-related myocardial injury; both did not have a clinical myocarditis (17). A detailed characterisation of the remaining HCWs with slightly elevated hsTnT would have also been of interest but was not provided in the publication. In contrast, we focused not only on hsTnT elevations above the URL and exceeding the reference change value, since slight elevations below the 99th percentile can, in the long term, also impact clinical outcomes (26). Our results differ from those of Buergin et al. who reported a mild and transient cardiomyocyte injury in a significantly higher proportion of participants in their cohort of hospital employees (2.8%; 22/777) 3 days after mRNA-1273 booster vaccination, with mainly female HCWs (2,57%; 20/777) being affected (18). This difference in sex distribution was not reproducible in our study. As described above, individual cases of a noticeable troponin rise were solely observed in male participants and, overall, median hsTnT levels, separated by sex, were significantly higher for our male population at all main visiting points. Also, the comparatively high cases of troponin elevation deviate from our results. However, here, it must be kept in mind that our first post-vaccination visit (V2) was performed up to 4 weeks after vaccination, with the aim of capturing persistent myocardial involvement. Therefore, our data does not allow for conclusions on possible acute troponin changes that may have occurred within the first days after vaccination. Yet, since other studies by Mansanguan et al. and Levi et al. did not find much lower incidences of myocardial injury in the immediate post-vaccine period (16, 17), possible other reasons for the deviating results of Buergin et al. must be considered. On the one hand, the authors chose the respective sex-specific URL, which was for females at 8.9 ng/L and, therefore, clearly lower than 14 ng/L (18). On the other hand, the distribution of sex was unbalanced in their study with an approximately 1:2 ratio of males:females (18). This difference in selection may have consequently resulted in higher numbers of cases with positive troponin amongst females than compared to prior trials. Further, Levi et al. discussed a higher immunological response, and subsequent cardiac involvement, observed after the former BNT1273 vaccination, contrasting to BNT162b2, as a plausible reason for the increased rate of troponin elevations (19, 32). Our study only investigated AZ or the BNT162b vaccine, which may also account for lower hsTnT levels. Importantly, one crucial limitation of Buergin and colleagues’ study was the missing baseline assessment of hsTnT levels (18). Elevated troponin levels may therefore have existed prior to vaccination and must, thus, be interpreted with caution.
MR-proADM is a biomarker for endothelial dysfunction and can be elevated for a broad spectrum of causes. Its release can be related to ongoing infectious processes, which is why MR-proADM is used as a prognostic marker for sepsis patients, including cases of severe COVID-19 (20, 21), but also for heart failure (24). Especially in the early stages of the pandemic, COVID-19 was discussed as a disease mainly affecting the vascular system (33). However, vaccine-associated endothelial dysfunction has been rarely investigated and, if so, predominantly in the acute post-vaccination phase (20). Therefore, this study is the first of its kind in which MR-proADM measurements were performed to assess persistent endothelial damage.
At baseline, the median MR-proADM was 0.44 nmol/L for the whole study population and ranged between 0.43 and 0.49 nmol/L in the AZ/BNT group and HCW-BNT/BNT sub-cohort; i.e., below the clinical cut-off of 0.75 nmol/L for all HCWs. In the elderly group, a higher median MR-proADM of 0.95 nmol/L was observed at V1. Accordingly, a significant difference in median MR-proADM levels was detected when comparing the HCWs and elderly groups; both at enrolment visit V1 and at V3. It can be assumed that these differences are mostly driven by known age-dependent, naturally higher values in the senior BNT/BNT sub-group. Furthermore, cardiovascular disease was reported in 70% of the elderly. From prior literature, we know that MR-proADM remains stably elevated in patients with pre-existing cardiovascular conditions (34). Focusing on the two younger and healthier HCW cohorts, no significant differences were detectable at any of the study visits. Moreover, changes in absolute median MR-proADM levels over time were nearly zero. Overall, 17 participants presented with at least one MR-proADM value above the clinical cut-off during the course of the study; however, 15 of them had elevated values already at enrolment, indicating that MR-proADM elevation was independent of vaccination. One case stood out because of a steep MR-proADM increase from visit V2 to V3. However, considering that the participant suffered from an active haematological malignancy, MR-proADM levels may be related to the underlying condition and were interpreted as a non-vaccine associated effect. The remaining participants showed no relevant kinetic and/or a return to baseline levels at V3. Therefore, our data indicate no evidence of subacute or persistent post-COVID-19 vaccination endotheliitis, based on MR-proADM measurements. This is in line with the results of a previous study by Terentes-Printzios et al. who reported no signs of permanent endothelial dysfunction after the COVID-19 vaccination by measuring, among other markers, brachial artery-flow mediated dilatation (20). However, this study reported a transient endothelial impairment in the immediate 24 h after vaccination (20); a time point that was not covered in our work.
Association between hsTnT and MR-proADM levels as analysed by Spearman's correlation was low for both females and males at all visiting times. A prior study by Theuerle et al. reported that MR-proADM may also function as a marker for myocardial damage and strong correlations between MR-proADM and hsTnT were discussed, without a further sex-dependent analysis. The researchers emphasised that a combined positive biomarker assay may be associated with a higher death risk in septic patients (35). While this may well be the case in severely ill patients with significant cardiovascular damage, our data suggest that hsTnT and MR-proADM correlation is weak in healthier cohorts with only mild biomarker elevations.
This is a prospective observational cohort study, in which we performed three study visits to assess persistent myocardial and vascular damage after the COVID-19 vaccination. The comparatively low sample size limits the statistical power and generalisability of the results. In this context, it must be acknowledged that the present work was conducted during the ongoing worldwide COVID-19 pandemic; a period characterised by significant political, social as well as research- and healthcare-related turbulence. Thus, the planning and execution of such a study within a constrained timeframe, involving vaccine-naive individuals was challenging and the number of participants difficult to regulate. Therefore, a certain validation in larger cohorts may be required in the future. However, the availability of baseline values and a reference cohort is a major strength over other existing and possible future studies, since it allows the characterisation of vaccine-associated elevation of biomarkers as compared to baseline and their trajectory over time. At the same time, since the observation span between V1 to V3 was several weeks, the data cannot definitively prove/exclude a causative association between COVID-19 vaccination and elevated markers of acute cardiovascular damage. Another limitation is the slightly distinct demographic baseline characteristic of the reference cohort and the study population and the lack of available MR-proADM data for the reference cohort. Another strength of our work is the fact that the study included the 1st COVID-19 vaccine dose, and also compared two different vaccination regimens, which allowed for a broad and longitudinal analysis of cardiovascular effects of COVID-19 vaccinations. By analysing all troponin changes, regardless of clinical symptoms and regardless of their absolute level above or below clinical cut-offs, our data also provides information on potentially mild subclinical adverse effects of COVID-19 vaccines. In addition, to our best of our knowledge, this is the first study that included an elderly sub-cohort and compared it to a young to mid-aged group. This is significant, since COVID-19 vaccinations are highly effective and most beneficial with regard to preventing severe diseases and mortality in older risk patients, most of whom present with pre-existing conditions.
This study shows no relevant hsTnT and MR-proADM increase in temporal association with COVID-19 vaccination in our cohort. Although the sample size is limited, the cohort is diverse and covers persons at high risk for both COVID-19 and cardiovascular disease. Thus, the study provides valuable data indicating that myocardial cell injury and endothelial dysfunction are not a common feature of COVID-19 vaccination and were not detectable up to 4 weeks after the 1st and 2nd homologous (BNT/BNT) or heterologous (AZ/BNT) COVID-19 vaccination. Hence, based on our data and other available evidence, a general cardiovascular peri-vaccine monitoring is not needed. However, based on the three individual cases of observed temporal subclinical troponin elevation, and one HCW case of persistent hsTnT rise, it should be investigated in future research if males under the age of 40, who suffer from pre-existing cardiovascular conditions or previously documented increases in hsTnT levels would benefit from active troponin surveillance following COVID-19 vaccination. However, since the vast majority of the population worldwide has had antigenic experience, often via multiple doses of vaccines and infections, generalisation of our findings to boosters and re-vaccinations should be cautioned. Future research is needed and as of now, all available safety data underscore the excellent safety and very good risk-benefit ratios of COVID-19 vaccines.
The raw data supporting the conclusions of this article will be made available by the authors, without undue reservation.
The studies involving humans were approved by the COVIM study (EA4/245/20), its preceding studies EICOV (EA4/245/20) and COVIMMUNIZE (EA4/244/20), the follow-up study COVIM-Boost (EA4/261/21) and the BIC-1 study (EA2/030/07) were approved by the ethics committee of Charité – Universitätsmedizin Berlin. The studies were conducted in accordance with the local legislation and institutional requirements. Written informed consent for participation in this study was provided by the participants themselves or (if necessary) by the participants' legal guardian/next of kin.
SP: Data curation, Formal Analysis, Methodology, Validation, Visualization, Writing – original draft. NL: Data curation, Formal Analysis, Investigation, Methodology, Validation, Visualization, Writing – review & editing. DHu: Data curation, Formal Analysis, Methodology, Validation, Visualization, Writing – original draft. FH: Formal Analysis, Writing – review & editing. DHi: Investigation, Writing – review & editing. PT-L: Investigation, Writing – review & editing. FK: Investigation, Writing – review & editing. LS: Conceptualization, Data curation, Funding acquisition, Investigation, Methodology, Project administration, Supervision, Validation, Writing – review & editing. MM: Conceptualization, Data curation, Formal Analysis, Funding acquisition, Investigation, Methodology, Project administration, Supervision, Validation, Visualization, Writing – original draft.
The author(s) declare financial support was received for the research, authorship, and/or publication of this article. The study was funded by “Forschungsnetzwerk der Universitätsmedizin zu COVID-19”, the Federal Ministry of Education and Research (BMBF), Zalando SE, the Federal Institute for Drugs and Medical Devices and intramural funds of Charité - Universitätsmedizin Berlin. The BIC-1 study was performed in collaboration with the Heidelberg university hospital. The funders of the study had no role in study design, data collection, data analysis, data interpretation, or writing of the report.
We thank all study participants of the EICOV, COVIMMUNIZE, COVIM, COVIM-Boost and BIC-1 study. We also thank all members of the EICOV/COVIM Study Group for sample acquisition and processing: Y. Ahlgrimm, B. Al-Rim, L. Bardtke, K. Behn, N. Bethke, H. Bias, D. Briesemeister, C. Conrad, V. M. Corman, C. Dang-Heine, S. Dieckmann, D. Frey, J.-A. Gabelich, J. Gerdes, U. Gläser, A. Hetey, L. Hasler, E. T. Helbig, A. Horn, C. Hülso, S. Jentzsch, C. von Kalle, L. Kegel, A. Krannich, W. Koch, P. Kopankiewicz, P. Kroneberg, I. Landgraf, L. J. Lippert, M. Lisy, C. Lüttke, P. de Macedo Gomes, B. Maeß, J. Michel, F. Münn, A. Nitsche, A.-M. Ollech, C. Peiser, A. Pioch, C. Pley, K. Pohl, A. Richter, M. Rönnefarth, C. Rubisch, L. Ruby, A. Sanchez Rezza, I. Schellenberger, V. Schenkel, J. Schlesinger, S. Schmidt, S. Schwalgun, G. Schwanitz, T. Schwarz, S. Senaydin, J. Seybold, A.-S. Sinnigen, A. Solarek, A. Stege, S. Steinbrecher, P. Stubbemann, C. Thibeault and D. Treue.
Independent of this work, MM received speakers and consulting fees from Bayer Healthcare, BMS, Boehringer Ingelheim, Daiichi Sankyo, Astra Zeneca, Sanofi, BRAHMS GmbH and Roche Diagnostics. He received research funding from German public funding authorities for Health Care Research and Roche Diagnostics. SP receives research funding from Roche Diagnostics.
The remaining authors declare that the research was conducted in the absence of any commercial or financial relationships that could be construed as a potential conflict of interest.
All claims expressed in this article are solely those of the authors and do not necessarily represent those of their affiliated organizations, or those of the publisher, the editors and the reviewers. Any product that may be evaluated in this article, or claim that may be made by its manufacturer, is not guaranteed or endorsed by the publisher.
The Supplementary Material for this article can be found online at: https://www.frontiersin.org/articles/10.3389/fcvm.2024.1435038/full#supplementary-material
1. Watson OJ, Barnsley G, Toor J, Hogan AB, Winskill P, Ghani AC. Global impact of the first year of COVID-19 vaccination: a mathematical modelling study. Lancet Infect Dis. (2022) 22(9):1293–302. doi: 10.1016/S1473-3099(22)00320-6
2. Rahmani K, Shavaleh R, Forouhi M, Disfani HF, Kamandi M, Oskooi RK, et al. The effectiveness of COVID-19 vaccines in reducing the incidence, hospitalization, and mortality from COVID-19: a systematic review and meta-analysis. Front Public Health. (2022) 10:873596. doi: 10.3389/fpubh.2022.873596
3. University of Oxford. Coronavirus (COVID-19) vaccinations (2023). Available online at: https://ourworldindata.org/covid-vaccinations (Accessed August 4, 2023).
4. Centers for Disease Control and Prevention. Selected Adverse Events Reported after COVID-19 Vaccination (2023). Available online at: https://www.cdc.gov/coronavirus/2019-ncov/vaccines/safety/adverse-events.html (Accessed August 4, 2023).
5. European Medicines Agency. Safety of COVID-19 vaccines (2023). Available online at: https://www.ema.europa.eu/en/human-regulatory/overview/public-health-threats/coronavirus-disease-covid-19/covid-19-medicines/safety-covid-19-vaccines#:∼:text=Reports%20of%20suspected%20side%20effects,-Information%20on%20reports&text=Almost%20768%20million%20vaccine%20doses,every%20100%20administered%20vaccine%20doses (Accessed August 4, 2023).
6. ICMRA. ICMRA statement on the safety of COVID-19 vaccines. Available online at: https://icmra.info/drupal/strategicinitiatives/vaccines/safety_statement (Accessed February 7, 2024).
7. Witberg G, Barda N, Hoss S, Richter I, Wiessman M, Aviv Y, et al. Myocarditis after COVID-19 vaccination in a large health care organization. N Engl J Med. (2021) 385(23):2132–9. doi: 10.1056/NEJMoa2110737
8. Wong HL, Hu M, Zhou CK, Lloyd PC, Amend KL, Beachler DC, et al. Risk of myocarditis and pericarditis after the COVID-19 mRNA vaccination in the USA: a cohort study in claims databases. Lancet. (2022) 399(10342):2191–9. doi: 10.1016/S0140-6736(22)00791-7
9. Xu SW, Ilyas I, Weng JP. Endothelial dysfunction in COVID-19: an overview of evidence, biomarkers, mechanisms and potential therapies. Acta Pharmacol Sin. (2023) 44(4):695–709. doi: 10.1038/s41401-022-00998-0
10. Yasmin F, Najeeb H, Naeem U, Moeed A, Atif AR, Asghar MS, et al. Adverse events following COVID-19 mRNA vaccines: a systematic review of cardiovascular complication, thrombosis, and thrombocytopenia. Immun Inflamm Dis. (2023) 11(3):e807. doi: 10.1002/iid3.807
11. Paknahad MH, Yancheshmeh FB, Soleimani A. Cardiovascular complications of COVID-19 vaccines: a review of case-report and case-series studies. Heart Lung. (2023) 59:173–80. doi: 10.1016/j.hrtlng.2023.02.003
12. Pillay J, Gaudet L, Wingert A, Bialy L, Mackie AS, Paterson DI, et al. Incidence, risk factors, natural history, and hypothesised mechanisms of myocarditis and pericarditis following COVID-19 vaccination: living evidence syntheses and review. Br Med J. (2022) 378:e069445. doi: 10.1136/bmj-2021-069445
13. Husby A, Hansen JV, Fosbol E, Thiesson EM, Madsen M, Thomsen RW, et al. SARS-CoV-2 vaccination and myocarditis or myopericarditis: population based cohort study. Br Med J. (2021) 375:e068665. doi: 10.1136/bmj-2021-068665
14. Oster ME, Shay DK, Su JR, Gee J, Creech CB, Broder KR, et al. Myocarditis cases reported after mRNA-based COVID-19 vaccination in the US from December 2020 to August 2021. JAMA. (2022) 327(4):331–40. doi: 10.1001/jama.2021.24110
15. Montgomery J, Ryan M, Engler R, Hoffman D, McClenathan B, Collins L, et al. Myocarditis following immunization with mRNA COVID-19 vaccines in members of the US military. JAMA Cardiol. (2021) 6(10):1202–6. doi: 10.1001/jamacardio.2021.2833
16. Mansanguan S, Charunwatthana P, Piyaphanee W, Dechkhajorn W, Poolcharoen A, Mansanguan C. Cardiovascular manifestation of the BNT162b2 mRNA COVID-19 vaccine in adolescents. Trop Med Infect Dis. (2022) 7(8):196. doi: 10.3390/tropicalmed7080196
17. Levi N, Moravsky G, Weitsman T, Amsalem I, Bar-Sheshet Itach S, Algur N, et al. A prospective study on myocardial injury after BNT162b2 mRNA COVID-19 fourth dose vaccination in healthy persons. Eur J Heart Fail. (2023) 25(2):313–8. doi: 10.1002/ejhf.2687
18. Buergin N, Lopez-Ayala P, Hirsiger JR, Mueller P, Median D, Glarner N, et al. Sex-specific differences in myocardial injury incidence after COVID-19 mRNA-1273 booster vaccination. Eur J Heart Fail. (2023) 25(10):1871–81. doi: 10.1002/ejhf.2978
19. Levi N, Hasin T. Myocardial injury related to SARS-CoV-2 mRNA vaccination: the plot thickens. Eur J Heart Fail. (2023) 25(10):1882–3. doi: 10.1002/ejhf.3013
20. Terentes-Printzios D, Gardikioti V, Solomou E, Emmanouil E, Gourgouli I, Xydis P, et al. The effect of an mRNA vaccine against COVID-19 on endothelial function and arterial stiffness. Hypertens Res. (2022) 45(5):846–55. doi: 10.1038/s41440-022-00876-6
21. Gregoriano C, Koch D, Kutz A, Haubitz S, Conen A, Bernasconi L, et al. The vasoactive peptide MR-pro-adrenomedullin in COVID-19 patients: an observational study. Clin Chem Lab Med. (2021) 59(5):995–1004. doi: 10.1515/cclm-2020-1295
22. Smith C. The structural vulnerability of healthcare workers during COVID-19: observations on the social context of risk and the equitable distribution of resources. Soc Sci Med. (2020) 258:113119. doi: 10.1016/j.socscimed.2020.113119
23. Liu E, Dean CA, Elder KT. Editorial: the impact of COVID-19 on vulnerable populations. Front Public Health. (2023) 11:1267723. doi: 10.3389/fpubh.2023.1267723
24. Mockel M, Koehler K, Anker SD, Vollert J, Moeller V, Koehler M, et al. Biomarker guidance allows a more personalized allocation of patients for remote patient management in heart failure: results from the TIM-HF2 trial. Eur J Heart Fail. (2019) 21(11):1445–58. doi: 10.1002/ejhf.1530
25. Ibanez B, James S, Agewall S, Antunes MJ, Bucciarelli-Ducci C, Bueno H, et al. 2017 ESC guidelines for the management of acute myocardial infarction in patients presenting with ST-segment elevation: the task force for the management of acute myocardial infarction in patients presenting with ST-segment elevation of the European Society of Cardiology (ESC). Eur Heart J. (2018) 39(2):119–77. doi: 10.1093/eurheartj/ehx393
26. Barbier CE, Themudo R, Bjerner T, Johansson L, Lindahl B, Venge P, et al. Cardiac troponin I associated with the development of unrecognized myocardial infarctions detected with MRI. Clin Chem. (2014) 60(10):1327–35. doi: 10.1373/clinchem.2014.222430
27. Lala A, Johnson KW, Januzzi JL, Russak AJ, Paranjpe I, Richter F, et al. Prevalence and impact of myocardial injury in patients hospitalized with COVID-19 infection. J Am Coll Cardiol. (2020) 76(5):533–46. doi: 10.1016/j.jacc.2020.06.007
28. Orlev A, Klempfner R, Rott D. Serum cardiac troponin T levels in asymptomatic elderly nursing home residents. Am J Med. (2018) 131(7):842–5. doi: 10.1016/j.amjmed.2017.11.054
29. Sedighi SM, Fulop T, Mohammadpour A, Nguyen M, Prud'Homme P, Khalil A. Elevated cardiac troponin levels in geriatric patients without ACS: role of comorbidities. CJC Open. (2021) 3(3):248–55. doi: 10.1016/j.cjco.2020.07.017
30. Herman DS, Kavsak PA, Greene DN. Variability and error in cardiac troponin testing: an ACLPS critical review. Am J Clin Pathol. (2017) 148(4):281–95. doi: 10.1093/ajcp/aqx066
31. Lan NSR, Bell DA. Revisiting the biological variability of cardiac troponin: implications for clinical practice. Clin Biochem Rev. (2019) 40(4):201–16. doi: 10.33176/AACB-19-00032
32. Abraham N, Spruin S, Rossi T, Fireman B, Zafack J, Blaser C, et al. Myocarditis and/or pericarditis risk after mRNA COVID-19 vaccination: a Canadian head to head comparison of BNT162b2 and mRNA-1273 vaccines. Vaccine. (2022) 40(32):4663–71. doi: 10.1016/j.vaccine.2022.05.048
33. Siddiqi HK, Libby P, Ridker PM. COVID-19—a vascular disease. Trends Cardiovasc Med. (2021) 31(1):1–5. doi: 10.1016/j.tcm.2020.10.005
34. Potocki M, Ziller R, Mueller C. Mid-regional pro-adrenomedullin in acute heart failure: a better biomarker or just another biomarker? Curr Heart Fail Rep. (2012) 9(3):244–51. doi: 10.1007/s11897-012-0096-6
Keywords: COVID-19 vaccination, high-sensitive troponin T, persistent myocardial injury, mid-regional pro-adrenomedullin, endothelial dysfunction
Citation: Pudasaini S, Le NH, Huscher D, Holert F, Hillus D, Tober-Lau P, Kurth F, Sander LE and Möckel M (2024) Levels of high-sensitive troponin T and mid-regional pro-adrenomedullin after COVID-19 vaccination in vulnerable groups: monitoring cardiovascular safety of COVID-19 vaccination. Front. Cardiovasc. Med. 11:1435038. doi: 10.3389/fcvm.2024.1435038
Received: 19 May 2024; Accepted: 30 September 2024;
Published: 18 October 2024.
Edited by:
Michiaki Nagai, University of Oklahoma Health Science Center, United StatesReviewed by:
Ricardo Adrian Nugraha, Airlangga University, IndonesiaCopyright: © 2024 Pudasaini, Le, Huscher, Holert, Hillus, Tober-Lau, Kurth, Sander and Möckel. This is an open-access article distributed under the terms of the Creative Commons Attribution License (CC BY). The use, distribution or reproduction in other forums is permitted, provided the original author(s) and the copyright owner(s) are credited and that the original publication in this journal is cited, in accordance with accepted academic practice. No use, distribution or reproduction is permitted which does not comply with these terms.
*Correspondence: Martin Möckel, bWFydGluLm1vZWNrZWxAY2hhcml0ZS5kZQ==
†These authors have contributed equally to this work and share last authorship
Disclaimer: All claims expressed in this article are solely those of the authors and do not necessarily represent those of their affiliated organizations, or those of the publisher, the editors and the reviewers. Any product that may be evaluated in this article or claim that may be made by its manufacturer is not guaranteed or endorsed by the publisher.
Research integrity at Frontiers
Learn more about the work of our research integrity team to safeguard the quality of each article we publish.