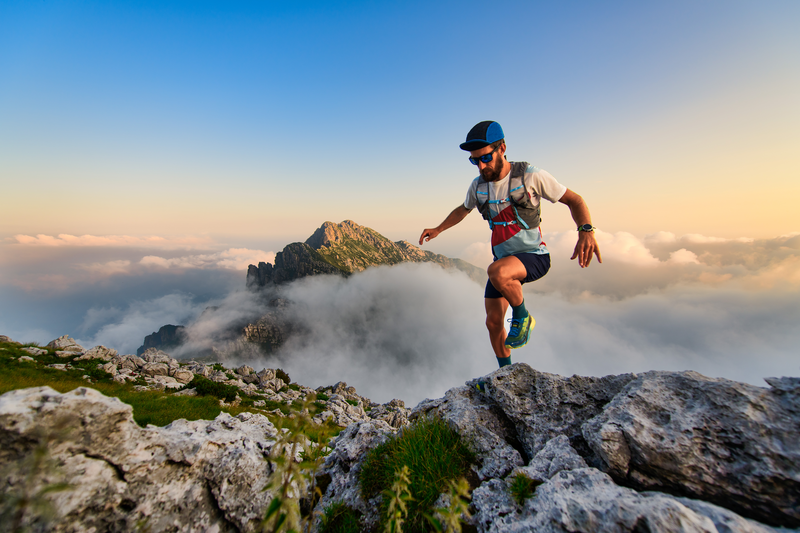
95% of researchers rate our articles as excellent or good
Learn more about the work of our research integrity team to safeguard the quality of each article we publish.
Find out more
ORIGINAL RESEARCH article
Front. Cardiovasc. Med. , 17 September 2024
Sec. Heart Failure and Transplantation
Volume 11 - 2024 | https://doi.org/10.3389/fcvm.2024.1415238
Background: Most studies have indicated that peripheral insulin-like growth levels factor-1 (IGF-1) is valuable in diagnosing heart failure, although the results have been inconsistent. To help solve the debate, we performed a meta-analysis to explore the relationship between IGF-1 and heart failure (HF).
Methods: We conducted an extensive search across various databases such as Embase, Cochrane Library, Pubmed, Medline, and Web of Science on May 30, 2023. From the extensive pool of studies, we selected 16 relevant articles, encompassing a total of 1,380 cases and 1,153 controls, to conduct a rigorous meta-analysis.
Results: The total results indicated that there is an association between lower IGF-1 level and HF. The random-effects model yielded a pooled standardized mean difference (SMD) of −0.598 (95% CI: −1.081 to −0.116, P = 0.015). Further subgroup analysis also showed that IGF-1 levels were associated with HF in the age difference ≥5 years subgroup and body mass index difference >1 subgroup. Additionally, significant association between IGF-1 levels and HF were detected in the “serum” samples and “Europe” subgroups. Importantly, we observed IGF-1 showed significant lower levels in patients with reduced ejection fraction (HFrEF) compared to the controls, not in patients with preserved ejection fraction (HFpEF). The Begg’s and Egger’s tests revealed no indication of publication bias.
Conclusions: Our meta-analysis has provided evidence suggesting a substantial correlation between reduced levels of IGF-1 and the occurrence of HF. Further prospective studies are necessary to ascertain the use of IGF-1 as a reliable biomarker for diagnosing HF, especially for HFrEF. But the diagnosis of HFpEF should be cautious.
Heart failure (HF), which affects approximately 64.3 million people globally, is the primary cause of death for those afflicted with cardiovascular diseases (1). The global prevalence of heart failure is expected to rise rapidly in the upcoming decades (2). Despite notable advancements in diagnostic and treatment approaches for heart failure in recent years, this condition continues to be a significant contributor to morbidity and mortality globally. Therefore, it is crucial to explore the underlying mechanisms of this condition and develop more efficacious diagnostic and treatment methods.
Identifying HF patients at an early stage who are at a higher risk can result in timely intervention, which has the potential to enhance outcomes (3). In recent decades, there has been an abundance of studies investigating prognostic biomarkers for HF; however, it is still difficult to predict both short-term and long-term outcomes. Several biomarkers, including B-type natriuretic peptide (BNP) (4), N-terminal proBNP (NT-proBNP) (4), sST2 (5), Gal3 (6), and GDF-15 (7) have been previously identified as having prognostic value for HF. Current guidelines recommend only BNP and NT-proBNP among these biomarkers.
The growth hormone/insulin-like growth factor-1 (GH/IGF-1) system is crucial for regulating growth and cellular differentiation in various tissues. IGF-1, homologous to pro-insulin, is a peptide consisting of 70 amino acids and is mainly synthesized in the liver and kidney. It is generally known as an anabolic growth hormone, responsible for cell growth, differentiation, proliferation and survival in almost all body organs, such as the heart, brain, breast, hepatocytes, skin, and so on (8–14). Numerous reports advocate the hypothesis that IGF-1 participates in the homeostasis of cardiovascular physiology. In particular, IGF-1's roles in the cardiovascular system include maintaining cellular homeostasis by regulating vascular vasoconstriction/vasodilatation, cardiac apoptosis and autophagy, and inflammatory responses (15–18). Many studies have attempted to explore the correlation between alterations in serum IGF-1 levels in individuals suffering from HF; nonetheless, researchers have obtained conflicting outcomes. In certain studies, a considerable decrease in serum IGF-1 levels has been observed among HF patients in comparison to control groups (19–25). However, some studies found that HF patients have higher serum IGF-1 levels than normal individuals (26, 27). Therefore, this study aims to conduct a comprehensive and critical meta-analysis of previous studies to arrive at a clearer and evidence-based conclusion regarding the association between serum IGF-1 levels and HF.
This study provided a summary of the research conducted on the correlation between IGF-1 and the risk of heart failure. Initially, our aim is to investigate if there is a connection between heart failure and changes in serum IGF-1 levels. Additionally, we aim to identify potential factors that could explain the significant variations observed in the results reported by these studies.
The present systematic review adheres to the Preferred Reporting Items for Systematic Reviews and Meta-Analyses (PRISMA) reporting guideline.
Two investigators performed an unbiased systematic review of English-language literature using multiple databases included “PubMed”, “Medline”, “Web of Science”, “Cochrane Library”, and “Embase”. The search terms used for each database were “insulin-like growth factor-1 OR IGF-1 AND heart failure.” The search was conducted until May 30, 2023. Additional information and a detailed search record are available in the Supplementary Materials.
The meta-analysis comprised eligible studies meeting the following criteria: (1) exploration of the association between blood IGF-1 concentrations and HF risk; (2) inclusion of a case group of patients with HF and a control group of healthy individuals; (3) utilization of qualified clinical criteria for HF diagnosis; (4) provision of IGF-1 data; (5) studies conducted in human subjects; and (6) availability of full English text.
The study's exclusion criteria were: (1) Insufficient data on IGF-1 concentrations. (2) Sole reporting of a single group of subjects. (3) Repetitive reporting about the same group of subjects. (4) Extraction of heart tissue or postmortem samples. (5) Study types that include non-human studies, case reports, commentaries, reviews, meta-analyses, conference abstracts or unrelated topics.
Information regarding the first author, publication year, study location (country), study design, number of participants for patients and controls, diagnostic criteria, average age of each group, gender distribution of each group, classification of HF (HFpEF: heart failure with preserved fraction, HFrEF: heart failure with reduced ejection fraction), sample type (serum, plasma), patient medication and detection technology (ELISA: enzyme-linked immunosorbent assay; RIA: radioimmunoassay; IRM: immunochemical assays), BMI, IGF-1 concentrations, the mean and standard deviation (SD) or median and the minimum and maximum values or median and interquartile range (IQR) were recorded from the included studies. If the case or control groups were further segmented into subgroups, the data from each subgroup were combined as , and .
In instances where a study solely provided medians and IQRs in lieu of means and SDs, and omitted the minimum or maximum values, we regarded the medians as synonymous with to the means. Subsequently, we derived the SDs by dividing the IQR by 1.35 (28). Alternatively, if the study supplied the minimum and maximum values, we estimated the means and SDs, as per the methodology outlined by Hozo et al. (29).
Two investigators carried out independent data extraction to identify any inaccuracies or omissions. Any discrepancies were resolved through discussions including a third author during the extraction process.
The quality of the studies included was appraised through the Newcastle-Ottawa Scale (NOS), suggested by the Agency for Healthcare Research and Quality in the United States. For the purpose of evaluating comparability of design or analysis between cases and controls, age and gender matching was exercised. Studies that secured a score below 5 on the NOS scale were excluded following assessments of low quality (Supplementary Material Table 1).
The relationship between the blood IGF-1 level and HF risk was evaluated by calculating the pooled standardized mean difference (SMD) and the two-sided 95% confidence intervals (CIs) for the meta-analysis. SMD denotes the mean difference in outcomes between cases and controls divided by the pooled standard deviation (SD), and yields a unit-free measure of effect size. The significance of the pooled SMD was determined utilising a Z test with the significance level set at p < 0.05. Heterogeneity amongst the studies was assessed using the Q test and the I2 statistic. When significant heterogeneity was identified (p < 0.1 in the Q test, and I2 > 50%), a random effects model was employed to combine the data from the primary studies. STATA 17.0 software (Stata Corporation, College Station, TX, United States) was used to do the meta-analysis and draw the forest plots.
To examine the potential impacts of age difference, sample type, and study location, we performed the subgroup analysis. Subgroup analyses were carried out based on age difference (<5 years or ≥5 years), BMI difference (≤1 or >1), sample type (serum or plasma) and region (Europe, East Asia, etc.). Random effects models were also used in subgroup analyses through STATA 17.0 software (Stata Corporation, College Station, TX, United States).
Network meta-analysis is a broadened form of pairwise meta-analysis that compares multiple different classifications across several diseases for the same condition (30). We then used the network meta-analysis to compare the IGF-1 value between HFrEF and HFpEF. A test for global inconsistency was performed, using the “network meta i” command, with a p-value more than 0.05; therefore, a consistency model that can be fitted was used to perform network meta-analysis. Between-studies heterogeneity was tested using the “network sidesplit” command through STATA 17.0 software (Stata Corporation, College Station, TX, United States). Direct and indirect comparison effects were jointly estimated using the node-splitting method.
A meta-regression using restricted maximum likelihood estimation (REML) was conducted to evaluate the potentially significant covariates exerting a substantial impact on the between-study heterogeneity through STATA 17.0 software (Stata Corporation, College Station, TX, United States). The meta-regression analysis included age difference (<5 years or ≥5 years), sampling type (plasma or serum), and study location (Europe, East Asia, and North America).
To assess result stability, the sensitivity analysis excluded individual studies on a step-by-step basis. Publication bias was evaluated by Begg-Kendall test with p < 0.05 representing significant publication bias and displayed using a funnel plot. STATA 17.0 software (Stata Corporation, College Station, TX, United States) was used to carry out all the analyses including meta-analysis, subgroup analysis, meta-regression, and publication bias assessment. A two-sided p-value lower than 0.05 was applied as the threshold for significance in all statistical tests.
The initial search yielded 295 records from Pubmed, 1,227 from Web of Science, 604 from Embase, 362 from the Cochrane Library and 101 from Medline. Following the removal of duplicates and a review of titles and abstracts, a total of 151 pertinent articles on the subject at hand were selected for full-text evaluation. Of these, 136 records were excluded: these were not IGF-1 and HF (n = 113), not heart failure (n = 1), not blood sample or venous blood sample (n = 4), review or meta-analysis (n = 1), the reports of studies that was no HF group (n = 2), or the studies of which did not have control group (n = 11), use duplicate samples (n = 1), not detailed data available (n = 4). Finally, we identified 16 studies using our inclusion and exclusion criteria. Please refer to Figure 1 for a flowchart showing the process of selecting studies.
Figure 1. Preferred reporting items for systematic reviews and meta-analyses (PRISMA) flow diagram of the study selection process. HF, heart failure; IGF-1, insulin-like growth factor-1.
Data from the 16 studies used in this meta-analysis were obtained either from the publications or directly provided by the authors where necessary. The characteristics of the studies were described in Table 1 and Supplementary Material Table 2. The total sample sizes consisted of 2,533 participants, comprising of 1,380 cases and 1,153 controls. The average ages of the participants in each study varied from 51.2 to 75.49 years for HF patients and 40.5 to 80 years for HC. Ten studies (62.5%) reported serum IGF-1 levels, and only 37.5% of studies reported plasma IGF-1 levels.
Table 1. The detailed characteristics of all the eligible studies for the association with the insulin-like growth factor-1 (IGF-1) levels and heart failure (HF).
The meta-analysis investigated the correlation between IGF-1 levels and the risk of HF, using data from 16 studies with 1,380 cases and 1,153 controls. The analysis indicated that lower levels of IGF-1 were associated with a higher risk of HF. A random-effects model showed the pooled SMD to be −0.598 (95% CI: −1.081 to −0.116; P = 0.015; I2 = 96.5%) (Figure 2).
Figure 2. Meta-analysis for the association of the IGF-1 level with HF risk using a random effects model for all included studies. HF, heart failure; IGF-1, insulin-like growth factor-1; SMD, standardized mean difference.
Among the 16 studies, eight had an age difference greater than or equal to 5 years, while eight had an age difference less than 5 years. When analyzing the subgroups based on age difference, we found that IGF-1 levels were significantly associated with HF in the subgroup of “age difference ≥5 years” [SMD = −0.73, 95% CI (−1.42, −0.04), P = 0.038; I2 = 96.5%], but not in the subgroup of “age difference < 5 years” (Figure 3A). Among the meta-analysis, nine studies included BMI data for HF. These studies were divided into two groups according to the BMI difference between HF and controls: diff BMI ≤1 (four studies) and diff BMI >1 (five studies). IGF-1 levels showed an association with HF in the subgroup of “diff BMI >1” [SMD = −0.75, 95% CI (−1.28, −0.22), P = 0.344; I2 = 87.7%]. However, there was no association observed in the subgroup of “diff BMI ≤ 1” [SMD = −0.60, 95% CI (−1.84, 0.64), P = 0.304; I2 = 98.3%] (Figure 3B).
Figure 3. Subgroup analysis of “age difference” (A) and “BMI difference” (B) using a random effects model between AD group and control group. HF, heart failure; SMD, standardized mean difference; BMI, body mass index.
Additionally, there were 12 studies that compared HFrEF and controls, and the result revealed that a reduced IGF-1 level was linked to a higher risk of HFrEF. [SMD = −0.66, 95% CI (−1.25, −0.07), P = 0.028; I2 = 97.1%] (Figure 4A). Regarding HFpEF data, only four studies were available, and further analysis of subgroups did not reveal any correlation between IGF-1 levels and HFpEF [SMD = −0.34, 95% CI (−0.87, 0.18), P = 0.202; I2 = 87.7%] (Figure 4B).
Figure 4. Meta-analysis for the association of the IGF-1 level with hFrEF (A) and hFpEF (B) risk using a random effects model. HFrEF, heart failure with reduced ejection fraction; HFpEF, heart failure with preserved ejection fraction; IGF-1, insulin-like growth factor-1; SMD, standardized mean difference.
Out of the 16 comparisons, six were samples taken from plasma, while ten were from serum. Analyzing the subgroup of “plasma” revealed no association between IGF-1 and HF [SMD = 0.02, 95% CI (−0.28, 0.33), P = 0.890; I2 = 72%]. However, subgroup analysis of “serum” showed a significant association between IGF-1 and HF [SMD = −1.03, 95% CI (−1.73, −0.33), P = 0.004; I2 = 97.3%] (Supplementary Material Figure 1). Furthermore, we conducted subgroup analyses based on region, dividing the studies into Europe, East Asia, and North America. The results indicated that only the subgroup analysis of “Europe” showed a significant association between IGF-1 and HF [SMD = −0.57, 95% CI (−1.08, −0.06), P = 0.029; I2 = 95.8%]. IGF-1 levels were not found to be associated with HF in the subgroup analyses of other regions (Supplementary Material Figure 2).
To discriminate between HFrEF and HFpEF, we assessed the value of IGF-1 in these groups using network meta-analysis (Supplementary Material Figure 3). Comparisons with controls, IGF-1 showed a significant lower level in HFrEF [SMD = −0.7, 95% CI (−1.25, −0.15)] not in HFpEF [SMD = −0.23, 95% CI (−1.12, 0.65)]. When comparing HFrEF and HFpEF, no significant difference was observed [SMD =0.46, 95% CI (−0.45, 1.38)].
Meta-regression analysis was carried out in order to explore the influence of sample source, age difference, and region on the effect size of the study. The outcomes uncovered that the sample source can account for 31.54% of the variation between studies (coefficient = −1.13, SE = 0.46, t = −2.49, P = 0.028) (Supplementary Material Table 3).
The Begg's and Egger's tests revealed no indication of publication bias (P = 0.386, P = 0.260, respectively). Additionally, the funnel plot for the overall results also did not exhibit any noteworthy bias (Supplementary Material Figure 4).
During the sensitivity analysis, we evaluated the impact of each eligible study on the combined SMD by excluding one dataset at a time. We observed no change in the corresponding pooled SMD or the statistical significance of the results (Supplementary Material Figure 5), indicating that our findings were highly resilient to the study-selection process.
Our meta-analysis included 16 reports, comprising a total of 1,380 cases and 1,153 controls. The findings showed a significant decrease in serum IGF-1 levels in HF patients compared to the control group. Subgroup analysis was conducted on various factors, including sample type (serum, plasma), geographic locations (Europe, East Asia, and North America), age difference (≥5, <5), BMI difference (≤1, >1), and LVEF (HFpEF ≥ 50%, HFrEF < 50%). We discovered that larger age differences and BMI difference between the case and control groups led to greater differences in IGF-1 levels between the groups. Additionally, subgroup analysis demonstrated a significant association between IGF-1 levels and HF in the “serum” samples and “Europe” subgroups. Importantly, significant differences in IGF-1 levels between HF and controls were observed in HFrEF subgroup not in HFpEF subgroup.
The GH/IGF-1 axis is considered the most potent natural anabolic system, driving post-natal growth mainly by promoting the elongation of bones and the development of muscle mass (31). IGF-1 is a pleiotropic factor, responsible for cell growth, differentiation, proliferation and survival. Despite systemic effects, IGF-1 exerts a wide array of influences in the cardiovascular system affecting metabolic homeostasis, autophagy, hypertension, cardiac contractility and hypertrophy, cardiac fibrosis, and inflammatory (32). It has been shown that IGF-1 prevents starvation-induced cardiac autophagy by increasing intracellular ATP levels, mitochondrial metabolism, mitochondrial Ca2+ uptake, and oxygen consumption (33). Norling et al. reported that IGF-1 deficiencies induce pathological vessel wall remodeling that result in a weakening of the cerebral arteries and are associated with functional maladaptation to hypertension (34). In addition, some pieces of evidence are suggesting IGF-1 protects against angiotensin II-induced cardiac fibrosis by targeting αSMA (35). Furthermore, it was found that IGF-1 attenuates the pro-inflammatory phenotype of neutrophils in myocardial infarction (18). The mechanisms explaining the connection between serum IGF-1 levels and HF remain incompletely comprehended. In patients with HF, the hemodynamic impairment is likely to change the blood flow in the pituitary gland, primarily due to the stagnation of venous drainage and/or poor supply of arterial blood. This alteration can result in the death of cells and subsequently cause a deficiency in IGF-1 (36). Besides, heart failure patients often suffer from secondary pulmonary hypertension and subsequently develop right heart failure and backward liver congestion, which may further hinder the secretion of IGF-1 (37). Isgaard et al. has been reported that IGF-1 can increase the contractility of cardiomyocytes primarily through elevating the level of intracellular calcium and sensitizing the myofilaments to calcium, and it can preserve capillary density. Therefore, decreased levels of IGF-1 can also exacerbate the process of HF (32). Research has shown that levels of serum IGF-1 were decreased in heart failure patients, and lower levels of peripheral IGF-1 were linked to an increased risk of heart failure (38). Our research also confirmed this. After exercise training treatment for HF, the lower IGF-1 level was also relieved with the improvement of cardiac function (39). These studies suggest that IGF-1 has the potential to be a disease biomarker in patients with HF.
Studies have recorded that HFrEF and HFpEF may exhibit different behavior regarding the anabolic drive, with HFrEF being altered and HFpEF remaining unmodified (40, 41). In this context, circulating protein biomarkers may offer valuable insights into early physiological changes and biological pathways that contribute to the subsequent development of HF, with the potential for improved understanding of similarities and differences in disease pathogenesis for HFrEF and HFpEF. Regarding the GH/IGF-1 axis, we found that HFrEF patients show a significant decrease in IGF-1 expression compared to healthy controls, and HFpEF patients do not. This finding may be related to the various regulatory roles of IGF-1 in the cardiovascular system as mentioned above. Then, we performed a further network analysis, and the results also indicate significance between HFrEF and the control group. This result indicates that IGF-1 is likely to become a specific biomarker for HFrEF. However, more research is needed to validate our findings and to further delineate the specific etiologies of and factors driving HF subtype development due to limited studies with data on this specific association in HF patients.
It was reported that serum IGF-1 peaks during puberty and declines thereafter during aging in humans (42). In our meta-analysis, we found a positive correlation between IGF-1 levels and the risk of HF in the overall analysis. Nevertheless, when we specifically considered studies with an age gap of less than 5 years between HF patients and controls, there was no evidence of a connection between IGF-1 levels and the risk of HF. Furthermore, when we combined the results of the subgroups with an age difference of ≥5 years, we observed a positive relationship between the age difference and the variance in IGF-1 levels among the control and HF groups. Because obesity and increased adiposity can affect GH secretions, it is reasonable to expect an association between BMI and IGF-1 levels, despite controversial results reported for the relationship between the two factors (43, 44). Additionally, several studies have indicated that obesity can result in a decrease in GH secretion, leading to low-normal IGF-1 levels (45–47). On this account, we analyzed the subgroups based on BMI difference. Our results indicated that IGF-1 levels showed an association with HF in the subgroup of “diff BMI >1”. However, there was no association observed in the subgroup of “diff BMI ≤ 1”. Thus, it is possible that age and BMI are two confounding factors, and the disparity in serum IGF-1 levels between the HF and control groups may be influenced by age difference and BMI difference. Hence, it is highly recommended that future studies incorporate age matching and BMI matching as two inclusion criterions for both groups, and meticulously consider the age and BMI of the control group.
In this meta-analysis, we observed that the lower IGF-1 level is related to HF risk. Our sensitivity analysis demonstrated that the variation caused by any individual study was not significant. Furthermore, we determined the absence of publication bias through the visualization of a funnel plot and the implementation of Begg's test. However, it is important to note that our study does possess certain limitations. Firstly, the number of studies included in our analysis is limited, and therefore, further research may be necessary to strengthen and support our findings. Secondly, there was a statistically significant heterogeneity among the trials, suggesting that caution should be exercised when drawing any conclusions. Additionally, we did not include conference proceedings, potentially resulting in the omission of some minor unpublished studies. Consequently, a detection bias cannot be completely ruled out in our analysis.
In conclusion, the evidence available supports an association between IGF-1 levels and HF. These findings indicate that serum IGF-1 has the potential to evaluate individuals with HF, especially for HFrEF. Its implementation can improve the identification of individuals with heart failure, offering an opportunity for early diagnosis in clinical practice. But caution is needed when diagnosing HFpEF.
The original contributions presented in the study are included in the article/Supplementary Material, further inquiries can be directed to the corresponding author.
Ethical approval was not required for the study involving humans in accordance with the local legislation and institutional requirements. Written informed consent to participate in this study was not required from the participants or the participants’ legal guardians/next of kin in accordance with the national legislation and the institutional requirements.
TL: Writing – review & editing, Conceptualization, Supervision, Writing – original draft, Data curation, Formal Analysis. FL: Conceptualization, Writing – original draft, Writing – review & editing, Formal Analysis, Methodology, Software, Data curation. YF: Data curation, Writing – original draft. FS: Conceptualization, Data curation, Writing – review & editing. MC: Data curation, Resources, Writing – original draft. XT: Data curation, Writing – original draft. WZ: Visualization, Writing – review & editing. ZZ: Project administration, Resources, Writing – review & editing. WW: Conceptualization, Funding acquisition, Writing – original draft, Writing – review & editing, Supervision.
The author(s) declare financial support was received for the research, authorship, and/or publication of this article. This work was funded by the National Natural Science Foundation of China (Nos. 82173795 to WW).
The authors declare that the research was conducted in the absence of any commercial or financial relationships that could be construed as a potential conflict of interest.
All claims expressed in this article are solely those of the authors and do not necessarily represent those of their affiliated organizations, or those of the publisher, the editors and the reviewers. Any product that may be evaluated in this article, or claim that may be made by its manufacturer, is not guaranteed or endorsed by the publisher.
The Supplementary Material for this article can be found online at: https://www.frontiersin.org/articles/10.3389/fcvm.2024.1415238/full#supplementary-material
1. Disease GBD, Injury I, and Prevalence C. Global, regional, and national incidence, prevalence, and years lived with disability for 354 diseases and injuries for 195 countries and territories, 1990–2017: a systematic analysis for the global burden of disease study 2017. Lancet. (2018) 392(10159):1789–858. doi: 10.1016/S0140-6736(18)32279-7
2. Savarese G, Lund LH. Global public health burden of heart failure. Card Fail Rev. (2017) 3(1):7–11. doi: 10.15420/cfr.2016:25:2
3. Cao TH, Quinn PA, Sandhu JK, Voors AA, Lang CC, Parry HM, et al. Identification of novel biomarkers in plasma for prediction of treatment response in patients with heart failure. Lancet. (2015) 385(Suppl 1):S26. doi: 10.1016/s0140-6736(15)60341-5
4. Cao Z, Jia Y, Zhu B. BNP and NT-proBNP as diagnostic biomarkers for cardiac dysfunction in both clinical and forensic medicine. Int J Mol Sci. (2019) 20(8):1820. doi: 10.3390/ijms20081820
5. Sciatti E, Merlo A, Scangiuzzi C, Limonta R, Gori M, D'Elia E, et al. Prognostic value of sST2 in heart failure. J Clin Med. (2023) 12(12):3970. doi: 10.3390/jcm12123970
6. Baccouche BM, Mahmoud MA, Nief C, Patel K, Natterson-Horowitz B. Galectin-3 is associated with heart failure incidence: a meta-analysis. Curr Cardiol Rev. (2023) 19(3):50–62. doi: 10.2174/1573403X19666221117122012
7. May BM, Pimentel M, Zimerman LI, Rohde LE. GDF-15 as a biomarker in cardiovascular disease. Arq Bras Cardiol. (2021) 116(3):494–500. doi: 10.36660/abc.20200426
8. Conover CA, Bale LK, Frye RL, Schaff HV. Cellular characterization of human epicardial adipose tissue: highly expressed PAPP-A regulates insulin-like growth factor I signaling in human cardiomyocytes. Physiol Rep. (2019) 7(4):e14006. doi: 10.14814/phy2.14006
9. Caicedo D, Díaz O, Devesa P, Devesa J. Growth hormone (GH) and cardiovascular system. Int J Mol Sci. (2018) 19(1):290. doi: 10.3390/ijms19010290
10. Macvanin M, Gluvic Z, Radovanovic J, Essack M, Gao X, Isenovic ER. New insights on the cardiovascular effects of IGF-1. Front Endocrinol. (2023) 14:1142644. doi: 10.3389/fendo.2023.1142644
11. Williams HC, Carlson SW, Saatman KE. A role for insulin-like growth factor-1 in hippocampal plasticity following traumatic brain injury. Vitam Horm. (2022) 118:423–55. doi: 10.1016/bs.vh.2021.11.009
12. Fajka-Boja R, Marton A, Tóth A, Blazsó P, Tubak V, Bálint B, et al. Increased insulin-like growth factor 1 production by polyploid adipose stem cells promotes growth of breast cancer cells. BMC Cancer. (2018) 18(1):872. doi: 10.1186/s12885-018-4781-z
13. Guo J, Cui L, Lu Q, Zhang Y, Liu Q, Wang X, et al. Cyadox regulates the transcription of different genes by activation of the PI3K signaling pathway in porcine primary hepatocytes. J Cell Biochem. (2019) 120(5):7623–34. doi: 10.1002/jcb.28037
14. Hu F, Huang K, Zhang H, Hu W, Tong S, Xu H. IGF-PLGA microspheres promote angiogenesis and accelerate skin flap repair and healing by inhibiting oxidative stress and regulating the Ang 1/tie 2 signaling pathway. Eur J Pharm Sci. (2024) 193:106687. doi: 10.1016/j.ejps.2023.106687
15. Higashi Y, Gautam S, Delafontaine P, Sukhanov S. IGF-1 and cardiovascular disease. Growth Horm IGF Res. (2019) 45:6–16. doi: 10.1016/j.ghir.2019.01.002
16. Lin M, Liu X, Zheng H, Huang X, Wu Y, Huang A, et al. IGF-1 enhances BMSC viability, migration, and anti-apoptosis in myocardial infarction via secreted frizzled-related protein 2 pathway. Stem Cell Res Ther. (2020) 11(1):22. doi: 10.1186/s13287-019-1544-y
17. Cirrik S, Schmid-Schönbein GW. IGF-1 receptor cleavage in hypertension. Hypertens Res. (2018) 41(6):406–13. doi: 10.1038/s41440-018-0023-7
18. Nederlof R, Reidel S, Spychala A, Gödecke S, Heinen A, Lautwein T, et al. Insulin-like growth factor 1 attenuates the pro-inflammatory phenotype of neutrophils in myocardial infarction. Front Immunol. (2022) 13:908023. doi: 10.3389/fimmu.2022.908023
19. Jankowska EA, Biel B, Majda J, Szklarska A, Lopuszanska M, Medras M, et al. Anabolic deficiency in men with chronic heart failure: prevalence and detrimental impact on survival. Circulation. (2006) 114(17):1829–37. doi: 10.1161/circulationaha.106.649426
20. Saeki H, Hamada M, Hiwada K. Circulating levels of insulin-like growth factor-1 and its binding proteins in patients with hypertrophic cardiomyopathy. Circ J. (2002) 66(7):639–44. doi: 10.1253/circj.66.639
21. Kontoleon PE, Anastasiou-Nana MI, Papapetrou PD, Alexopoulos G, Ktenas V, Rapti AC, et al. Hormonal profile in patients with congestive heart failure. Int J Cardiol. (2003) 87(2–3):179–83. doi: 10.1016/s0167-5273(02)00212-7
22. Hu Z, Mao L, Wang L. Levels of serum IGF-1, HCY, and plasma BNP in patients with chronic congestive heart failure and their relationship with cardiac function and short-term prognosis. Evid Based Complement Alternat Med. (2022) 2022:4118976. doi: 10.1155/2022/4118976
23. D'Assante R, Arcopinto M, Rengo G, Salzano A, Walser M, Gambino G, et al. Myocardial expression of somatotropic axis, adrenergic signalling, and calcium handling genes in heart failure with preserved ejection fraction and heart failure with reduced ejection fraction. ESC Heart Fail. (2021) 8(2):1681–6. doi: 10.1002/ehf2.13067
24. Broglio F, Benso A, Gottero C, Di Vito L, Aimaretti G, Fubini A, et al. Patients with dilated cardiomyopathy show reduction of the somatotroph responsiveness to GHRH both alone and combined with arginine. Eur J Endocrinol. (2000) 142(2):157–63. doi: 10.1530/eje.0.1420157
25. Barroso MC, Kramer F, Greene SJ, Scheyer D, Köhler T, Karoff M, et al. Serum insulin-like growth factor-1 and its binding protein-7: potential novel biomarkers for heart failure with preserved ejection fraction. BMC Cardiovasc Disord. (2016) 16(1):199. doi: 10.1186/s12872-016-0376-2
26. Al-Obaidi MK, Hon JK, Stubbs PJ, Barnes J, Amersey RA, Dahdal M, et al. T12 plasma insulin-like growth factor-1 elevated in mild-to-moderate but not severe heart failure. Am Heart J. (2001) 142(6):E10. doi: 10.1067/mhj.2001.118116
27. Toth MJ, Matthews DE. T16 whole-body protein metabolism in chronic heart failure: relationship to anabolic and catabolic hormones. JPEN J Parenter Enteral Nutr. (2006) 30(3):194–201. doi: 10.1177/0148607106030003194
28. Higgins JPT, Thomas J, Chandler J, Cumpston M, Li T, Page MJ, et al. Cochrane Handbook for Systematic Reviews of Interventions. 2nd ed. Chichester, UK: John Wiley & Sons (2019).
29. Hozo SP, Djulbegovic B, Hozo I. Estimating the mean and variance from the median, range, and the size of a sample. BMC Med Res Methodol. (2005) 5(1):13. doi: 10.1186/1471-2288-5-13
30. De Marzo V, Savarese G, Tricarico L, Hassan S, Iacoviello M, Porto I, et al. Network meta-analysis of medical therapy efficacy in more than 90,000 patients with heart failure and reduced ejection fraction. J Intern Med. (2022) 292(2):333–49. doi: 10.1111/joim.13487
31. Melmed S. New therapeutic agents for acromegaly. Nat Rev Endocrinol. (2016) 12(2):90–8. doi: 10.1038/nrendo.2015.196
32. Isgaard J, Arcopinto M, Karason K, Cittadini A. GH and the cardiovascular system: an update on a topic at heart. Endocrine. (2015) 48(1):25–35. doi: 10.1007/s12020-014-0327-6
33. Troncoso R, Vicencio JM, Parra V, Nemchenko A, Kawashima Y, del Campo A, et al. Energy-preserving effects of IGF-1 antagonize starvation-induced cardiac autophagy. Cardiovas Res. (2012) 93(2):320–9. doi: 10.1093/cvr/cvr321
34. Norling AM, Gerstenecker AT, Buford TW, Khan B, Oparil S, Lazar RM. The role of exercise in the reversal of IGF-1 deficiencies in microvascular rarefaction and hypertension. GeroScience. (2019) 42(1):141–58. doi: 10.1007/s11357-019-00139-2
35. Ock S, Ham W, Kang CW, Kang H, Lee WS, Kim J. IGF-1 protects against angiotensin II-induced cardiac fibrosis by targeting αSMA. Cell Death Dis. (2021) 12(7):688. doi: 10.1038/s41419-021-03965-5
36. Wang CY, Chung HW, Cho NY, Liu HS, Chou MC, Kao HW, et al. Idiopathic growth hormone deficiency in the morphologically normal pituitary gland is associated with perfusion delay. Radiology. (2011) 258(1):213–21. doi: 10.1148/radiol.10100504
37. Rosenkranz S, Gibbs JS, Wachter R, De Marco T, Vonk-Noordegraaf A, Vachiery JL. Left ventricular heart failure and pulmonary hypertension. Eur Heart J. (2016) 37(12):942–54. doi: 10.1093/eurheartj/ehv512
38. Barroso MC, Kramer F, Greene SJ, Scheyer D, Köhler T, Karoff M, et al. T14 serum insulin-like growth factor-1 and its binding protein-7: potential novel biomarkers for heart failure with preserved ejection fraction. BMC Cardiovasc Disord. (2016) 16(1):199. doi: 10.1186/s12872-016-0376-2
39. Hambrecht R, Schulze PC, Gielen S, Linke A, Möbius-Winkler S, Erbs S, et al. Effects of exercise training on insulin-like growth factor-I expression in the skeletal muscle of non-cachectic patients with chronic heart failure. Eur J Cardiovasc Prev Rehabil. (2005) 12(4):401–6. doi: 10.1097/01.hjr.0000173106.68485.b7
40. Faxen UL, Hage C, Benson L, Zabarovskaja S, Andreasson A, Donal E, et al. HFpEF and HFrEF display different phenotypes as assessed by IGF-1 and IGFBP-1. J Card Fail. (2017) 23(4):293–303. doi: 10.1016/j.cardfail.2016.06.008
41. Salzano A, Marra AM, Ferrara F, Arcopinto M, Bobbio E, Valente P, et al. Multiple hormone deficiency syndrome in heart failure with preserved ejection fraction. Int J Cardiol. (2016) 225:1–3. doi: 10.1016/j.ijcard.2016.09.085
42. Gong Z, Kennedy O, Sun H, Wu Y, Williams GA, Klein L, et al. Reductions in serum IGF-1 during aging impair health span. Aging Cell. (2014) 13(3):408–18. doi: 10.1111/acel.12188
43. Hjelholt A, Høgild M, Bak AM, Arlien-Søborg MC, Bæk A, Jessen N, et al. Growth hormone and obesity. Endocrinol Metab Clin North Am. (2020) 49(2):239–50. doi: 10.1016/j.ecl.2020.02.009
44. Roelfsema F, Veldhuis JD. Growth hormone dynamics in healthy adults are related to age and sex and strongly dependent on body mass index. Neuroendocrinology. (2016) 103(3–4):335–44. doi: 10.1159/000438904
45. Kempf E, Landgraf K, Vogel T, Spielau U, Stein R, Raschpichler M, et al. Associations of GHR, IGF-1 and IGFBP-3 expression in adipose tissue cells with obesity-related alterations in corresponding circulating levels and adipose tissue function in children. Adipocyte. (2022) 11(1):630–42. doi: 10.1080/21623945.2022.2148886
46. Kopchick JJ, Berryman DE, Puri V, Lee KY, Jorgensen JOL. The effects of growth hormone on adipose tissue: old observations, new mechanisms. Nat Rev Endocrinol. (2019) 16(3):135–46. doi: 10.1038/s41574-019-0280-9
Keywords: heart failure, IGF-1, meta-analysis, prognostic biomarker, HFrEF
Citation: Liu T, Li F, Fei Y, Sun F, Chen M, Tian X, Zheng W, Zhu Z and Wang W (2024) Serum insulin-like growth factor-1 as a potential prognostic biomarker for heart failure with reduced ejection fraction: a meta-analysis. Front. Cardiovasc. Med. 11:1415238. doi: 10.3389/fcvm.2024.1415238
Received: 10 April 2024; Accepted: 6 September 2024;
Published: 17 September 2024.
Edited by:
Inna P. Gladysheva, University of Arizona College of Medicine—Phoenix, United StatesCopyright: © 2024 Liu, Li, Fei, Sun, Chen, Tian, Zheng, Zhu and Wang. This is an open-access article distributed under the terms of the Creative Commons Attribution License (CC BY). The use, distribution or reproduction in other forums is permitted, provided the original author(s) and the copyright owner(s) are credited and that the original publication in this journal is cited, in accordance with accepted academic practice. No use, distribution or reproduction is permitted which does not comply with these terms.
*Correspondence: Wen Wang, bHp3d2FuZ0AxNjMuY29t
†These authors have contributed equally to this work
Disclaimer: All claims expressed in this article are solely those of the authors and do not necessarily represent those of their affiliated organizations, or those of the publisher, the editors and the reviewers. Any product that may be evaluated in this article or claim that may be made by its manufacturer is not guaranteed or endorsed by the publisher.
Research integrity at Frontiers
Learn more about the work of our research integrity team to safeguard the quality of each article we publish.