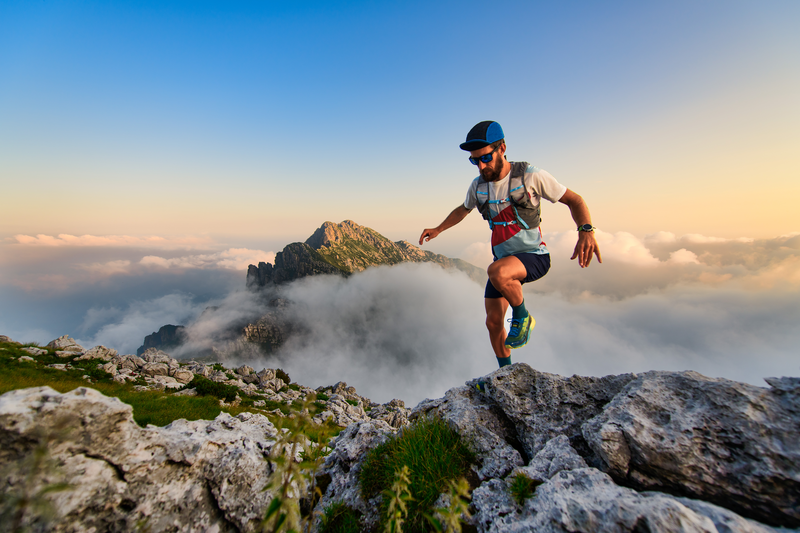
94% of researchers rate our articles as excellent or good
Learn more about the work of our research integrity team to safeguard the quality of each article we publish.
Find out more
ORIGINAL RESEARCH article
Front. Cardiovasc. Med. , 30 May 2024
Sec. Cardiovascular Biologics and Regenerative Medicine
Volume 11 - 2024 | https://doi.org/10.3389/fcvm.2024.1394453
This article is part of the Research Topic Current Status, Challenges and Future Scope of Stem Cells in Cardiovascular Regenerative Medicine View all 4 articles
Background: Mesenchymal stem cells (MSCs) have important research value and broad application prospects in cardiovascular diseases (CVDs). However, few bibliometric analyses on MSCs in cardiovascular diseases are available. This study aims to provide a thorough review of the cooperation and influence of countries, institutions, authors, and journals in the field of MSCs in cardiovascular diseases, with the provision of discoveries in the latest progress, evolution paths, frontier research hotspots, and future research trends in the regarding field.
Methods: The articles related to MSCs in cardiovascular diseases were retrieved from the Web of Science. The bibliometric study was performed by CiteSpace and VOSviewer, and the knowledge map was generated based on data obtained from retrieved articles.
Results: In our study, a total of 4,852 publications launched before August 31, 2023 were accessed through the Web of Science Core Collection (WoSCC) database via our searching strategy. Significant fluctuations in global publications were observed in the field of MSCs in CVDs. China emerged as the nation with the largest number of publications, yet a shortage of high-quality articles was noted. The interplay among countries, institutions, journals and authors is visually represented in the enclosed figures. Importantly, current research trends and hotspots are elucidated. Cluster analysis on references has highlighted the considerable interest in exosomes, extracellular vesicles, and microvesicles. Besides, keywords analysis revealed a strong emphasis on myocardial infarction, therapy, and transplantation. Treatment methods-related keywords were prominent, while keywords associated with extracellular vesicles gathered significant attention from the long-term perspective.
Conclusion: MSCs in CVDs have become a topic of active research interest, showcasing its latent value and potential. By summarizing the latest progress, identifying the research hotspots, and discussing the future trends in the advancement of MSCs in CVDs, we aim to offer valuable insights for considering research prospects.
Cardiovascular disease (CVD) is a pressing public health concern that remains the leading cause of death globally (1). However, traditional treatment methods for cardiovascular diseases, such as various drug therapies and surgical interventions, may result in significant adverse reactions or disease recurrence (2). Recent studies have highlighted the promising role of mesenchymal stem cells (MSCs) in the treatment of several kinds of CVDs (3–6).
MSCs, a type of cell with self-renewal ability, multi-differentiation potential and immune regulation functions, exist in a variety of tissues (7–9). MSCs stain positive for a series of cell surface markers including CD73, CD44, CD90, and CD105, yet stain negative for the hematopoietic markers such as CD34 and CD45 and HLA-DR (10). MSCs can differentiate into various cell types including chondrocytes, adipocytes, cardiomyocytes, muscle cells, etc., which makes them valuable for both in-vivo and in-vitro applications (10–14). MSCs can exhibit immunomodulatory function through cell-to-cell contact, and perform the release of cytokines to inhibit the proliferation and immune response of T cells (15). Based on the characteristics that have both regenerative and immune regulatory abilities, MSCs can be widely applied in clinical research and can show extremely high research value. The differentiation ability of MSCs can be utilized for stem cell therapy of cardiovascular diseases and it can to some extent avoid the occurrence of adverse reactions (16). Shin et al. discovered that the MSCs intravenously injected can specifically migrate to the inflammatory site caused by ischemic injury, and it has been confirmed that administration of MSCs after myocardial infarction injury can enhance adenosine production through CD73 activity and reduce reactive oxygen species (ROS) formation following MI/R (17). Under appropriate conditions, MSCs can differentiate into functional cardiomyocytes (18–20). For example, 5-azacytidine can induce MSCs to express proteins commonly associated with cardiomyocytes, including beta-MHC, desmin, and alpha-cardiac actin, thereby showing potential to repair injured myocardium (21). MSCs can also promote angiogenesis through differentiation. Quevedo et al. performed catheter-based transendocardial injections of MSCs on swine and found that MSCs can differentiate into vascular smooth muscle and endothelial cells, contributing to the formation of vessels of all sizes (22).
Bibliometric analysis evaluates and quantifies literature information by analyzing different features of articles, aiming to identify global research trends (23). It typically uses scientific articles found in the databases as input to create interactive visual representations for statistical analysis (24). The primary research issues and frontiers can be identified by swiftly assembling a large number of published articles, sketching and visualizing the structure and dynamics of a research field, and then conducting further analysis (25). Bibliometrics has unparalleled advantages in analyzing research hotspots and frontiers compared to other methods due to its specialization in identifying core researchers, institutions, and countries in the field, and their collaborative relationships as well (26). Using software for bibliometric analysis, a knowledge map is created by thoroughly reviewing the literature on the suggested research dimensions (27). Due to the advantages of this method, more and more scholars are using bibliometric analysis in various fields of medicine, such as gynecology (28), hypertension (29), lung diseases (30), inflammatory bowel disease (31), aortic disease (32), and autoimmune diseases (33).
This research aims to analyze the trends and hotspots in the utilization of MSCs for CVDs over the past decade using tools like CiteSpace and VOSviewer. By creating a knowledge map based on bibliometric analysis, the study provides insights into the latest progress, evolution, and future directions of MSC research in CVDs, benefiting both basic research and clinical treatment.
The Science Citation Index Expanded (SCI-E) and Social Science Citation Index (SSCI) of the core database of the document information index database Web of Science were selected as the target databases for bibliometric analysis. The search formula was set to TS = (“Mesenchymal Stem Cell*” OR “Bone Marrow Stromal Cell*” OR “Mesenchymal Stromal Cell*” OR “Mesenchymal Progenitor Cell*” OR “Bone Marrow Stromal Stem Cell*”OR “MSC*”) AND TS = (“high blood pressure” or hypertensi* or “peripheral arter*” disease* or “atrial fibrillat*” or tachycardi* or endocardi* or pericard* or ischem* or arrhythmi* or thrombo* or cardio* or cardiac* or “heart failure” or “heart beat” or “heart rate*” or “heart val*” or coronary* or angina* or ventric* or myocard*) AND DT = (Article OR Review) AND LA = (English) AND DOP = (2013-01-01/2023-08-31). A total of 10,388 publications were originally identified through WoSCC database searching. Only articles and reviews published in English that met the theme of MSCs in CVDs were selected for further analysis, while book chapters, proceedings papers, meeting abstracts, corrections, editorials, duplicates, retractions, or unpublished articles were excluded. Two independent investigators (Jiajing Yu and Houle Zhou) retrieved and filtered the publications to ensure the accuracy of the scientometric analysis. After removing literature that does not match this topic (n = 5,536), 4,852 studies were identified as eligible for our study, consisting of articles (n = 3,575) and reviews (n = 1,277). The detailed filtering process is demonstrated in Figure 1.
The software used in the study includes VOSviewer [version 1.6.20(0), Leiden University, Leiden, Netherlands], CiteSpace (version 6.2. R5, Chaomei Chen, Drexel University, Philadelphia, PA, United States), Microsoft Excel for Mac [version 16.78.3(23102801), WA, United States], the R language based Bibliometrix Package (4.1.3 Package) and Scimago Graphica (Beta 1.0.36). These applications were used to analyze the 10,388 exported articles and data information including the number and year of publications, total and average citations, titles, countries and institutions, authors, journals, keywords, and references. VOSviewer was used to construct and view bibliometric maps, which has functionality for zooming, scrolling, and searching (34). The VOSviewer map depicts weighting by nodes, collaborative relationships by links, and link strength by link thickness. To distinguish clusters, different colors are used in the module visualizing network. The Bibliometric package (4.1.3 package) based on R language is used to analyze three-field plots, displaying the relationships between co-cited references, authors, and keywords.
Figure 2 indicates the change in annual publications (reviews and articles) and citation frequency, which witnessed the progress of this field. From 2013 to 2023, the annual publications on MSCs in cardiovascular diseases included 4,852 related articles, generally presenting an ascending trend. The number of papers published on MSCs in CVDs experienced an upturn from 2013 to 2018, then declined from 2019 to 2022 compared to the previous years. The articles we obtained for this study were published before August 31, 2023. We speculate that by the end of 2023, the total number of publications will be equal to or less than the number in 2022. In addition, Figure 2 depicts the upward trend of citation frequency from 2013 to 2021. We have updated the citation data and the latest citation data displayed is as of April 23, 2024. The citations in 2022 had a modest drop compared to 2021. However, the citation count of 183 papers in the first 8 months of 2023 has exceeded the citation count of 392 articles in the entire year of 2022. This indicates that the interest and influence of this study in this field will continue to grow.
Figure 2. Trends in the growth of publications (reviews and articles), and citations worldwide from 2013 to 2023. Due to the need for article revisions, the citation data of the articles filtered was updated, and the latest citation data displayed is as of April 23, 2024.
Table 1 ranked 10 high-productivity countries that contributed to publications of MSCs in CVDs. China and the United States stand out as the two primary contributors in this research field, showcasing a contrast in the number of publications and citations compared to other countries. China leads with the highest number of publications (1,735 documents), which represents 37.36% of the total. The United States follows closely behind with 1,350 publications, accounting for 29.07% of the research output. Additionally, Italy, Germany, South Korea, and the United Kingdom have also played substantial roles in this field, with publication numbers of 233 (5.02%), 226 (4.87%), 226 (4.87%), and 201 (4.33%) respectively. While Canada, Iran, Japan, and the Netherlands have made noteworthy contributions, their publication numbers remain below 200. As is shown in Figure 3A, nodes of different sizes represent countries with different levels of participation, and different colors represent clusters of close cooperation. For example, the United States and China have collaborated closely in this research field. Interestingly, the USA has garnered the highest number of citations, totaling 62,761, while China closely follows with 51,896 citations, slightly below the USA. This data suggests that USA (1,350 documents, 62,761 citations, and 825 total link strength) stands out as the country with the most extensively collaborative network in publications related to MSCs in CVDs.
Figure 3. The collaboration of countries/regions and institutions in the field of MSCs in CVDs. (A) Collaboration map of the publications and co-occurrence network of countries/regions. (B) Institutional cooperation/contributions to publications.
Table 1 also displayed the top 10 most productive institutions in the field. Furthermore, the VOSviewer was applied to cluster the cooperating organizations, and formed a clustering diagram (Figure 3B). Zhejiang University leads in publication volume with 90 articles, establishing itself as a key contributor in terms of the number of publications. Following closely come the University of Miami (80 articles), Shanghai Jiao Tong University (76 articles), and Sun Yat-sen University (72 articles).
Notably, the University of Miami stands out with the highest number of citations, indicating a relatively high quality of articles produced by the institution. In Figure 3B, institutions closer to the yellow color spectrum are depicted to be more active recently, while those closer to the blue spectrum indicate a higher level of activity in the past.
The top 10 most productive journals and co-cited journals in the field of MSCs in CVDs are ranked in Table 2. The top three journals in number of publications were Stem Cell Research & Therapy (184 articles), International Journal of Molecular Sciences (131 articles) and Stem Cells International (128 articles), each of which possessed more than 120 documents. While referring to the H-index of the top 10 productive journals, Circulation Research (48 H-index) ranked first, which reflects the high quality of its production and academic influence, followed by Stem Cell Research & Therapy (47 H-index), which were the only two journals with an H-index exceeding 40. The influence factor is one of the indicators to measure the influence of a journal, which reflects the frequency of article citations. According to Table 2, the journal with the highest impact factor is Circulation Research, reaching 20.1. This indicates that the articles in this journal have great influence and importance in the academic community. Regarding the total link strength, Stem Cell Research & Therapy (13,676 citations and 1,312,401 total link strength) and International Journal of Molecular Sciences (13,430 citations and 1,308,475 total link strength) featured the most co-citations along with others, with a total link strength that surpassed 1,300,000. By analyzing these data, we can figure out which journals have more influence and importance in the academic community, as well as the correlation between them. For example, Circulation and Circulation Research are two widely cited journals, which also show a high link strength. This indicates that they have a great influence in the field of MSCs in CVDs, and there may be a cooperative relationship or mutual reference.
The bond between countries, institutions and journals based on a three-field plot for MSCs in CVDs is demonstrated in Figure 4. China exerts the greatest influence among the 20 countries, closely followed by the USA. The six dominant institutions lying foremost on the list are Chang Gung Memorial Hospital, the University of California System, Harvard University, Pennsylvania Commonwealth System of Higher Education (PCSHE), the University of Toronto and the University of Miami. Notably, from the last two columns of the chart, we can learn the information of the collaborations between institutions and journals. The six journals with the most connections are Stem Cell Research & Therapy, Circulation Research, Stem Cells, Scientific Reports, Plos One, and Stem Cells Translational Medicine (Figure 4).
Figure 4. The relationship of the countries, institutions, and research journals that produced articles in an alluvial flow map based on MSCs in CVDs.
Table 3 lists the top 10 authors who contributed most to the literature on MSCs in CVDs. Hare Joshua M. (49 Documents, 3,488 citations, and 33 H-index) ranks first with the most citations per document among the top ten authors in this field, reaching 71.18, indicating that the articles he published have been highly influential. Bolli Roberto, Zhu Wei, and Cheng Ke ranked second, third, and fourth, each of whom published 27 articles. Other top ten authors also published over 20 articles. These authors have made outstanding contributions to the field of MSCs in CVDs. Their articles have been widely cited and developed a high H-index, indicating that their research has been widely recognized by peers (Table 3).
As Table 3 shows, Gnecchi M. (893 citations, 30,501 total link strength) was the most high-yielding co-cited author. Hare Joshua M. with the highest total link strength, reaching 31,683, ranks second. His research focuses on MSCs in cardiomyogenesis and angiogenesis in postinfarct myocardium (35), reducing levels of apoptosis (36), increasing tissue perfusion (37), and stimulating the proliferation and differentiation of cardiac stem cells (38).
The top 10 references based on the number of citations are listed in Table 4, including three articles and seven reviews. The most cited reference written by Phinney Donald. et al. in 2017 was a review published in Stem Cells, entitled “Concise Review: MSC-Derived Exosomes for Cell-Free Therapy” (39), followed by a review entitled “Silk Fibroin Biomaterials for Tissue Regenerations” (40). Table 4 also illustrates the uppermost 10 co-cited references on the topic of MSCs in CVDs, with Dominici et al. (9), Pittenger et al. (41) and Hare et al. (42) ranking the highest.
As was shown in Figure 5A, CiteSpace was used to extract the information of co-cited references. A cluster analysis was performed on the extracted information, as shown in Figure 5B. In Figure 5B, there are 18 clusters formed and 9 clusters showed in the figure: (0) exosomes, (1) extracellular vesicles (EVs), (2) microvesicles, (3) bone marrow mononuclear cells, (4) fibrosis, (5) cardiac stem cells, (6) cardiovascular regeneration, (7) cell adhesion, (8) drug delivery. The three clusters of exosomes, EVs and microvesicles have the highest burst strength. To some extent, these clusters reflect the main research focus in the fields of MSCs in CVDs.
Figure 5. The collaboration of authors and co-cited references in the field of MSCs in CVDs. (A) The figure only shows the top 10 authors’ name. Cooperation network among the co-cited references in the studies of MSCs in CVDs. (B) A cluster of the co-cited references in the studies of MSCs in CVDs.
Figure 6 shows the top 25 references with the strongest citation bursts during 2013–2023. Through analysis of the references published over each specific period, we can identify the research hotspots during the corresponding time. As we can see in Figure 6, the first citation burst occurred between 2013 and 2014, and the latest reference with a strong citation burst was recorded from 2021 to the present. The highest burst strength was from Hare et al. (42) (50.39 strength), Williams et al. (2011) (45.82 strength), and Bolli et al. (2011) (41.45 strength). What's more, Kalluri et al. (2020) and Huang et al. (2020) have received wide attention in recent years (Figure 6).
Figure 6. The top 25 references with the strongest citation bursts, based on citeSpace, that were involved in MSCs in CVDs between 2013 and 2023. The blue line represents the time from its first appearance to 2023, and the red line represents the burst time.
The top 20 frequency keywords related to MSCs in CVDs are displayed in Table 5. “MSCs” (2,337 occurrences, 20,025 total link strength) was the keyword with the greatest number of occurrences. The second and third ranked keywords are “myocardial infarction” (793 occurrences, 7,317 total link strength) and “therapy” (722 occurrences, 7,035 total link strength). These data can help us understand which keywords are widely discussed and cited in the field of MSCs in CVDs, as well as their importance and research hotspots.
Moreover, we applied CiteSpace to group the keywords so that we could illustrate the timeline for keywords after clustering (Figure 7). The association between keywords is manifested in the map (Figure 7A). The larger the node is, the higher the frequency of the keyword was. A point has layers of different colors and thicknesses. The color represents the time of publication. The earlier keywords tend to be bluer; the later ones tend to be yellower. And the thickness represents the number of occurrences during that period.
Figure 7. Keyword-related mapping in studies in the field of MSCs in CVDs. (A) The figure only shows the top 10 keywords. Map of the keywords in the studies of MSCs in CVDs. (B) Subcategories of the main keywords that were utilized in the studies related to MSCs in CVDs. (C) A timeline view of keywords based on CiteSpace related to MSCs in CVDs.
According to the cluster map (Figure 7B), there are 9 clusters formed: (0) myocardial infarction, (1) EVs, (2) MSCs, (3) heart failure, (4) cell therapy, (5) tissue engineering, (6) bone marrow, (7) left ventricular function, and (8) endothelial cells. Obviously, they are all related to MSCs and exosomes, which to some extent indicate the research center of the intersection of MSCs and cardiovascular fields. By sorting the keywords in the cluster in chronological order based on the year of their first appearance, the timeline view was formed. As is depicted in Figure 7C, the keyword relevant to EVs has maintained a certain number of occurrences from 2013 to 2023, with several outbreaks in a few years. Besides, keywords relevant to myocardial infarction and EVs obtained attention for the longest period on MSCs in CVDs.
Moreover, the top 25 keywords with the strongest citation bursts are depicted in Figure 8. The keyword with the longest burst period was “injury”, which lasted from 2018 to 2023. And the highest burst strength related to MSCs in CVDs was “EVs” (strength = 70.05), “marrow stromal cells” (strength = 27.49), and “cardiac repair” (strength = 24.27). Additionally, “infarction” (2014) and “smooth muscle cells” (2014) have received considerable attention in recent years.
The association and connection between the top 20 co-cited references, authors and keywords (only 19 keywords were presented) in MSCs in CVDs are shown in Figure 9 as an alluvial flow map. It depicted that the top 20 co-cited references are more closely related to the research of MSCs, while various types of CVDs occurred (Figure 9).
Figure 9. The relationships of the top 20 co-cited references, authors, and keywords evolutions based on an alluvial flow map by R.
This study conducts a comprehensive bibliometric analysis utilizing CiteSpace and VOSviewer to investigate recent research developments, identify key research areas, and outline future growth directions in the field of MSCs in CVDs. Through this analysis, we aim to provide a detailed examination of research progress in this domain. By compiling and presenting data on nations and regions, institutions, authors, references, and keywords relevant to this subject, we generate clear and informative tables and figures. These findings are essential for recognizing research hotspots and trends in the field.
The founder of the field of MSC biology was Prof. Alexander Friedenstein, who was the first to discover the presence of a group of non-hematopoietic bone marrow stromal cells in the bone marrow, exhibiting clonal adherent growth and morphology similar to fibroblasts (43–45). In 1991, Prof. Arnold Caplan first named these cells as MSCs (46). In 1999, SCIENCE first reported that MSCs have the ability to differentiate into adipocytes, osteoblasts, and chondrocytes simultaneously in vitro (41), which ignites everyone’s enthusiasm for studying MSCs, focusing on the various differentiation functions of MSCs. In 2004, Le Blanc et al. reported the successful implementation of the first partially compatible heterogenic MSC transplantation for treating graft-versus-host disease (GVHD) (47). During the period under analysis from 2013 to 2023, this research field garnered significant attention.
In Figure 2, publication and citation reached their peaks in 2018 and 2021, respectively indicating the peak influence of the study during this timeframe. Although subsequent publications maintained a certain quantity, there was a slight decline in research activity levels. The decrease in the number of publications in 2019 may be related to the retraction of 31 papers related to stem cell research in top international journals by Harvard University in 2018. The retraction may have lagged and interfered with the development of stem cell research. However, it is worth noting that the period from 2019 to 2021 coincides with the global pandemic of COVID-19, but the number of publications has still increased to some extent (Figure 2). After analyzing the number of articles and reviews separately, we found that compared to 2019, the number of articles published in 2021 decreased by 2, while the number of reviews increased by 54. This shows that the growth of the number of papers issued during the period of COVID-19 from 2019 to 2021 basically comes from the growth of review type articles. This may be because under the influence of the COVID-19 epidemic, the scientific research work of the laboratory was restricted, but people spent more time writing reviews. This, to a certain extent, also explains the reason for the growth rate of citation numbers is even higher than before the outbreak of COVID-19 in 2019. This also indicates to some extent that the research on MSCs in CVDs has received widespread attention. However, by 2022, the number of articles and reviews were significantly reduced, which may be related to the fact that most countries attach importance to the epidemic and take relatively strict measures to control the COVID-19. And the negative impact of COVID-19 on scientific research may lag behind, and it was concentrated displayed in 2022. The citation count of a specific article dynamically changes over time. We have updated the citation data of the articles we have filtered, and the latest citation data displayed is as of April 23, 2024. As shown in Figure 2, even if only 183 papers in the first eight months were included in the analysis in 2023, the citation count in 2023 has exceeded the citation count of 392 articles in 2022. Therefore, we speculate that the research of stem cells in cardiovascular diseases will continue to develop steadily.
Chinese scholars lead in publication volume, followed by the US, surpassing the third-ranked Italy by nearly sevenfold. Notably, six of the top ten productive institutions are Chinese, with Zhejiang University standing out as the most prolific. As is well known, China has a huge population base, which may be one of the reasons for this result. In addition, China has the highest morbidity and mortality rates of cardiovascular diseases in the world, so it is in urgent need of cardiovascular disease research. While China may not have initiated this research, its subsequent studies and experiences have contributed significantly to the field's advancement.
Furthermore, in Table 4, we can observe that although China has a significant advantage in the number of publications, this advantage becomes less pronounced when evaluating the value of articles based on citation rates. Among the top ten most-cited references, only three were authored by Chinese scholars. Similarly, none of the top ten co-cited references were from Chinese scholars. This indicates that although China is the predominant country in terms of publishing articles in the field of MSCs in CVDs, there are still relatively few high-quality articles published. The possible reason is that Chinese scholars have paid less attention to the mechanism of MSCs, and more attention has been paid to the application and efficacy of MSCs in specific diseases.
Overall, bibliometric analyses indicate a flourishing research landscape on MSCs in CVDs, with countries contributing diverse and in-depth studies. Meanwhile, different countries and laboratories have their own advantages and research focuses, and there are still significant differences in their level in this research field. However, the continuous strengthening of international academic cooperation and exchange is driving rapid progress in this field.
Extensive experimental evidence suggests that MSCs play an indispensable role in potentially differentiation into a wide range of cell types, and they likewise have the ability to differentiate into cardiomyocytes or cardiomyocytes-like cells through a variety of strategies that focus on biological, physical, and chemical aspects (48–50). Studies have shown that women with pre-eclampsia will face a higher risk of CVD such as chronic hypertension, ischemic heart disease and late-life stroke (51), and MSC treatment has shown promise in alleviating pre-eclampsia symptoms through immune-modulating, pro-angiogenic, anti-inflammatory and antioxidant effects (52–55). In addition, in the realm of atherosclerosis, BMSCs affect the role of VSMC in plaque formation through different regulatory mechanisms (56, 57). The remarkable differentiation potential of MSCs into various cell types can also promote angiogenesis to enhance tissue perfusion in ischemic conditions (58–63).
The important role of MSCs-derived exosomes in cardiovascular diseases has received widespread attention. Zhang et al. found that the mechanism by which MSC-EXO prevents vascular remodeling in pulmonary hypertension through the regulation of the Wnt5a/BMP signaling pathway (64). In addition, exosomes produced by MSCs can also direct cardiomyocyte differentiation (65–69). The article with most citations (Table 4) has discussed about MSC-derived extracellular vehicles which play an important role in cell-to-cell communication, cell signaling, cell alteration and tissue metabolism at short or long distances in the body (39). Various groups have confirmed that MSC-derived exosomes exhibiting cardio-protective activity are efficacious in animal models of myocardial infarction (70–72), as well as in accelerating wound healing in chronic limb-threatening ischemia (73–76). The most cited literature, Concise Review: MSC-Derived Exosomes for Cell-Free Therapy, also mentioned that MSC-derived exosomes mainly exert their effects through mRNA, miRNA, and proteins, and then alter the activity of target cells through various mechanisms (39).
MiRNA, one of the MSC-sourced bioactive molecules, has the function of regulating gene expression (77–79). As we can see in Figure 8, the keyword “gene expression” has attracted a lot of attention since 2017. Cardiac repair can be enhanced by exosome-derived miRNA gene expression (80–84). By inhibiting the expression of miR-210, Wang et al. demonstrated that miR-210 may inhibit Efna3 at the transcription level, thereby stimulating angiogenesis (85). By comparing fetal dermal MSC EVs with WJ-MSC EVs, Chinnici et al. found that five miRNAs (27b-3p, 125a-5p, 146a-5p, 126-3p and 137) with higher levels have the ability to activate endogenous VEGF-A to promote angiogenesis (86). What's more, Pan et al. incubated LPS-exposed cardiomyocytes with EVs from mouse MSCs, confirming that miR-223-3p in EVs of MSCs can target and inhibit FOXO3 to reduce NLRP3 expression, and subsequently inhibit cardiac inflammation (87). In addition, MSC exo-miRNA has anti-apoptotic function (88–90) and anti-inflammatory function (91), which can enhance the pumping capacity of the heart and effectively treat heart failure (92).
Signaling pathways are essential to cellular physiological activities, and significant proteins can be regulated to modify particular cellular functions (93). In recent years, the role of miRNA in crucial cellular signaling pathways has also been stressed (94–96). Several experiments have demonstrated that miRNAs derived from MSCs regulate multiple signaling pathways, thereby affecting cardiomyocyte repair (97–100). For instance, Sun et al. injected purified exosomes into hypoxia/reoxygenation conditioned rat MIRI model, demonstrating that the MiR-486-5p carried by BMSC-Exos inhibits PTEN expression and activates PI3K/AKT signaling pathway, which ultimately inhibits apoptosis of injured cardiomyocytes (101). In addition, miR-96 in BMSC-Exos promoted BMSC-Exos protection on the cardiac function of DOX-induced rats through inhibiting the Rac1/NF-κB signaling pathway, which reduced free radicals and inhibited the toxicity of DOX, inflammatory reactions and fibrosis (102). The treatment conducted by Zhu et al. treatment with a miR-144 inhibitor in a hypoxic environment could induce increased PTEN expression and decreased p-AKT expression, which led to enhanced apoptosis of H9C2 cells, demonstrating that miR-144 inhibits apoptotic injury under hypoxic conditions by regulating the PTEN/AKT pathway (103). In recent years, a steady stream of pathways associated with miRNA regulation have been identified, but it is still unclear which exosomal miRNAs are the best regulators for the use in the development of MSC-based therapies. By comparing the roles of various types of exosomal miRNAs in the future, it may be possible to find the best therapeutic approaches regarding cardiovascular diseases such as myocardial repair.
MSCs promote healing in ischemic tissue-associated diseases through pro-angiogenic secretion of proteins, again in relation to MSC exosomes, making them a promising resource for regenerative medicine and a wide range of in vitro applications (104–106). In addition, a variety of exosome-derived proteases have been shown to favor cardiomyocyte repair and regeneration through multiple mechanisms (107–110). For example, ATP deficiency and initiation of apoptosis during ischemia and reperfusion are supported by proteomic defects in enzymes critical for fatty acid oxidation, glycolysis, and the tricarboxylic acid cycle as well as proteomic excesses of pro-apoptotic proteins. Lai et al. discovered that this glycolytic enzyme deficiency was complemented by an abundance of exosomes from MSCs, and that CD73 could increase survival through activation of the reperfusion injury salvage kinases (111). What's more, MSC-derived exosomes contain multiple proteins involved in signaling molecules, like cytokines, interleukins, chemokines, and growth factors (107). MSCs have been shown to secrete a number of growth factors and cytokines that reduce myocardial ischemia and reperfusion injury (79, 112–116). The therapeutic effects of many cardiovascular diseases are mediated by the reduction of fibrosis, tissue revascularization, cardiomyocyte proliferation, and progenitor cell recruitment, which are required to be mediated by growth factors such as VEGF, HGF, FGF, and neuromodulatory proteins, as well as by cytokines such as colony-stimulating factors and leukemia inhibitory factors (117–121).
In summary, MSCs can not only differentiate directly into cardiomyocytes, but also treat cardiovascular diseases through MSC-derived exosomes. These exosomes can be utilized for their therapeutic potential by leveraging their source miRNAs, proteins, etc., to regulate gene expression, signaling pathways, and growth factors, thus providing a new approach to cardiovascular medicine. By understanding and exploiting the complex interactions facilitated by these exosomes, we can develop more effective and personalized treatments for cardiovascular disease, marking an important step forward in regenerative medicine.
Recent clinical trials have delved into the efficacy of MSCs in treating CVDs. Clinical trials have demonstrated the safety and efficacy of MSCs in treating CVDs (122–128). MSC therapy has been shown to enhance regional function, tissue perfusion, and reduce fibrotic burden in patients undergoing cardiac surgery (129). Moreover, MSC treatment has proven beneficial for individuals with severe ischemic heart failure and non-ischemic heart disease, leading to improvements in myocardial function, ejection fraction, and quality of life (123, 126–128, 130). In patients with stable heart failure and reduced ejection fraction, MSC therapy has been deemed safe and feasible (124). Additionally, research indicates that MSC therapy may possess antiarrhythmic potential and can improve outcomes in non-ischemic dilated cardiomyopathy patients, irrespective of gender (131, 132). Meanwhile, a SCIENCE trial and a multi-center double-blinded placebo-controlled trial by Qayyum et al. found MSCs to be less effective than expected (133, 134). Correspondingly, the author analyzed that the possible reasons were that the cell retention may have been too short to initiate the paracrine effects, and the treatment may have been initiated too late. These findings underscore the promise of MSC therapy in managing a range of heart conditions and indicate the need for further exploration in larger clinical trials.
MSCs, with their broad origin, robust differentiation capacity, and regenerative potential, play an important role in regenerative medicine (135, 136). They have long been a focal point in clinical research for treating CVDs (137). The therapeutic effects of MSCs on various CVDs, including myocardial infarction, atherosclerosis, pulmonary hypertension, and so on, have been extensively investigated (22, 56, 138–141). The immense potential of MSCs in various applications remains undeniable in the future. However, recent research on MSCs may have reached a plateau, and the problems of low survival after transplantation and low differentiation efficiency still need to be solved (138, 142). What’s more, low retention, weak homing activity, invasive isolation process, and limited tissue volume obtained are also the key issues that limit the application of MSCs in the clinic (Figure 10) (143–145).
Figure 10. Challenges faced by MSCs in clinical applications. In order to realize the treatment of diseases like myocardial infarction and pulmonary hypertension, MSCs need to overcome the following five significant obstacles: (1) low survival rate, (2) low retention, (3) weak homing activity, (4) invasive isolation process, and (5) limited tissue volume obtained.
Indeed, compared to MSC cell therapy, cell-free treatments based on MSC secretomes and EVs in show significant potential in regenerative medicine (146–150). EVs have proven to be an effective drug delivery method for a variety of conditions, including tumors, neurodegenerative disorders, renal disease, and CVDs (151–157). Additionally, biological effectors can be transferred by EVs through natural or engineered means. MSC-derived EV-based therapy provides several benefits over MSC-based cell therapy, according to a growing number of studies: low immunogenicity, low tumorigenicity, low cytotoxicity, easy preservation, high stability, low degradability, blood–brain barrier penetration ability, possible intravenous injection, internalization into cells, potential cargo loading ability, intrinsic homing properties, immunotolerance properties, absence of genotoxic effects, and no endotoxin production (158, 159). Laboratory studies have shown that compared to MSCs, MSC-derived exosomes and their cargos also play an important role in cardiovascular diseases, which has been introduced in Section 4.2.1. So far, several clinical studies have evaluated the efficacy of EVs derived from MSCs in respiratory diseases (160–164). However, the efficacy of MSC-derived EVs in CVDs should be further explored.
Currently, biomaterials technology combined with cellular therapy plays a huge role in cardiovascular diseases (165, 166). For example, treatments with collagen can promote cardiomyogenic differentiation of MSCs (167, 168). In recent years, numerous nanomaterials have also been gradually combined with MSC therapy (169, 170). Studies have found that graphene as a culture substrate contributes to the survival rate of MSCs after transplantation (171, 172). In addition, hydrogels can be used as scaffolds to induce MSC differentiation (173, 174). Embedding MSCs in hydrogels for treatment can promote wound healing and angiogenesis, as well as anti-inflammatory effects, which are crucial in the treatment of cardiovascular diseases (175–178). In addition, in terms of non-extracellular matrix based, the use of cellular derivatives of MSCs as polymeric biomaterials (e.g., collagen, gelatin, hyaluronic acid, chitosan, etc.) for scaffold fabrication for cardiomyocytes can influence stem cell behavior (179–182).
In this study, we collected data from WoSCC through a retrieval strategy and analyzed it, thus obtaining certain reliable results. However, it is essential to acknowledge certain limitations. Firstly, the retrieval algorithm that served as the method to get our results may have ineluctable flaws, potentially leading to omissions in retrieving relevant literature. Secondly, relying solely on data from a single database restricted the scope of our study results. What's more, due to time constraints in obtaining retrieval literature, it was inevitable that some recent articles were not included in our research.
To explore the latest trends and key areas of interest concerning MSCs in CVDs, future research should encompass corresponding bibliometric analyses. Nonetheless, this bibliometric study conducted a meticulous analysis and thorough examination of the literature data, providing valuable insights into the progression of MSCs in CVDs. It also underscored promising directions for future development and research hotspots within the field.
Over recent years, research on MSCs has emerged as a beacon of hope across a wide spectrum of diseases, notably in the realm of CVDs. Our study, as a bibliometric analysis, has synthesized the latest advancements, identified the research hotspots, and deliberated on the prospects in the field of MSCs in CVDs. Currently, investigating the molecular mechanism underlying the role of MSCs in CVDs stands out as a prominent research trend. Noteworthy topics include the study of MSC-derived exosomes, regulation of gene expression and signaling pathways by miRNAs, and promotion of growth factors are also hot topics. In addition, the utilization of biomaterials plays a critical role in facilitating the transplantation and functionality of MSCs. By holding an escalating trend in international and inter-institutional collaborations, this field of research is poised to remain a key area of study, with a primary focus on advancing treatments for CVDs and enhancing longevity.
The original contributions presented in the study are included in the article/Supplementary Material, further inquiries can be directed to the corresponding authors.
ZJ: Writing – original draft, Formal Analysis, Conceptualization. JY: Project administration, Validation, Writing – original draft. HZ: Data curation, Writing – original draft. JF: Resources, Writing – original draft, Visualization. ZX: Investigation, Writing – original draft. MW: Writing – original draft, Investigation. WZ: Writing – original draft, Investigation. YH: Writing – original draft, Investigation. CJ: Writing – original draft, Investigation. SS: Writing – review & editing. HG: Writing – review & editing. BL: Writing – review & editing.
The author(s) declare financial support was received for the research, authorship, and/or publication of this article.
This work was supported by grants from the National Natural Science Foundation of China (82204831, 82174120), Natural Science Foundation of Shanghai (No. 21ZR1463100), Program of Shanghai Academic Research Leader (22XD1423400), Shanghai Sailing Program (No. 22YF1448800) and, the China Postdoctoral Science Foundation (No. 2021M692153).
We thank Wenlong Xue for the support in reading and revising the manuscript. Furthermore, we thank CiteSpace and VOSviewer for free access by researchers.
The authors declare that the research was conducted in the absence of any commercial or financial relationships that could be construed as a potential conflict of interest.
All claims expressed in this article are solely those of the authors and do not necessarily represent those of their affiliated organizations, or those of the publisher, the editors and the reviewers. Any product that may be evaluated in this article, or claim that may be made by its manufacturer, is not guaranteed or endorsed by the publisher.
1. Nitsa A, Toutouza M, Machairas N, Mariolis A, Philippou A, Koutsilieris M. Vitamin D in cardiovascular disease. In Vivo. (2018) 32(5):977–81. doi: 10.21873/invivo.11338
2. Faulx MD, Francis GS. Adverse drug reactions in patients with cardiovascular disease. Curr Probl Cardiol. (2008) 33(12):703–68. doi: 10.1016/j.cpcardiol.2008.08.002
3. Pan Y, Wu W, Jiang X, Liu Y. Mesenchymal stem cell-derived exosomes in cardiovascular and cerebrovascular diseases: from mechanisms to therapy. Biomed Pharmacother. (2023) 163:114817. doi: 10.1016/j.biopha.2023.114817
4. Guo Y, Yu Y, Hu S, Chen Y, Shen Z. The therapeutic potential of mesenchymal stem cells for cardiovascular diseases. Cell Death Dis. (2020) 11(5):349. doi: 10.1038/s41419-020-2542-9
5. de Oliveira MS, Saldanha-Araujo F, de Goes AM, Costa FF, de Carvalho JL. Stem cells in cardiovascular diseases: turning bad days into good ones. Drug Discov Today. (2017) 22(11):1730–9. doi: 10.1016/j.drudis.2017.07.012
6. Joladarashi D, Kishore R. Mesenchymal stromal cell exosomes in cardiac repair. Curr Cardiol Rep. (2022) 24(4):405–17. doi: 10.1007/s11886-022-01660-1
7. Tian C, Juan J, Zhi-Feng G. The Therapeutic Role of Mesenchymal Stem Cell-Derived Exosomes in the Autoimmune Diseases (2022).
8. Ding D-C, Shyu W-C, Lin S-Z. Mesenchymal stem cells. Cell Transplant. (2011) 20(1):5–14. doi: 10.3727/096368910X
9. Dominici M, Le Blanc K, Mueller I, Slaper-Cortenbach I, Marini FC, Krause DS. Minimal criteria for defining multipotent mesenchymal stromal cells. The international society for cellular therapy position statement. Cytotherapy. (2006) 8(4):315–7. doi: 10.1080/14653240600855905
10. Jiang B, Yan L, Wang X, Li E, Murphy K, Vaccaro K, et al. Concise review: mesenchymal stem cells derived from human pluripotent cells, an unlimited and quality-controllable source for therapeutic applications. Stem Cells. (2019) 37(5):572–81. doi: 10.1002/stem.2964
11. Squillaro T, Peluso G, Galderisi U. Clinical trials with mesenchymal stem cells: an update. Cell Transplant. (2016) 25(5):829–48. doi: 10.3727/096368915X689622
12. Deak E, Seifried E, Henschler R. Homing pathways of mesenchymal stromal cells (MSCs) and their role in clinical applications. Int Rev Immunol. (2010) 29(5):514–29. doi: 10.3109/08830185.2010.498931
13. Gupta S, Sharma A, A S, Verma RS. Mesenchymal stem cells for cardiac regeneration: from differentiation to cell delivery. Stem Cell Reviews and Reports. (2021) 17(5):1666–94. doi: 10.1007/s12015-021-10168-0
14. Liu Z-J, Zhuge Y, Velazquez OC. Trafficking and differentiation of mesenchymal stem cells. J Cell Biochem. (2009) 106(6):984–91. doi: 10.1002/jcb.22091
15. Lai P, Weng J, Guo L, Chen X, Du X. Novel insights into MSC-EVs therapy for immune diseases. Biomark Res. (2019) 7(1):6. doi: 10.1186/s40364-019-0156-0
16. White IA, Sanina C, Balkan W, Hare JM. Mesenchymal stem cells in cardiology. Methods Mol Biol. (2016) 1416:55–87. doi: 10.1007/978-1-4939-3584-0_4
17. Shin EY, Wang L, Zemskova M, Deppen J, Xu K, Strobel F, et al. Adenosine production by biomaterial-supported mesenchymal stromal cells reduces the innate inflammatory response in myocardial ischemia/reperfusion injury. J Am Heart Assoc. (2018) 7(2):e006949. doi: 10.1161/JAHA.117.006949
18. Xu H, Zhou Q, Yi Q, Tan B, Tian J, Chen X, et al. Islet-1 synergizes with Gcn5 to promote MSC differentiation into cardiomyocytes. Sci Rep. (2020) 10(1):1817. doi: 10.1038/s41598-020-58387-8
19. Fukuda K, Fujita J. Mesenchymal, but not hematopoietic, stem cells can be mobilized and differentiate into cardiomyocytes after myocardial infarction in mice. Kidney Int. (2005) 68(5):1940–3. doi: 10.1111/j.1523-1755.2005.00624.x
20. Carvalho PH, Daibert APF, Monteiro BS, Okano BS, Carvalho JL, da Cunha DNQ. Differentiation of adipose tissue-derived mesenchymal stem cells into cardiomyocytes. Arq Bras Cardiol. (2013) 100(1):82–9. doi: 10.1590/S0066-782X2012005000114
21. Xu W, Zhang X, Qian H, Zhu W, Sun X, Hu J. Mesenchymal stem cells from adult human bone marrow differentiate into a cardiomyocyte phenotype in vitro. Exp Biol Med (Maywood). (2004) 229(7):623–31. doi: 10.1177/153537020422900706
22. Quevedo HC, Hatzistergos KE, Oskouei BN, Feigenbaum GS, Rodriguez JE, Valdes D. Allogeneic mesenchymal stem cells restore cardiac function in chronic ischemic cardiomyopathy via trilineage differentiating capacity. Proc Natl Acad Sci USA. (2009) 106(33):14022–7. doi: 10.1073/pnas.0903201106
23. Zhang J, Zhang Y, Hu L, Huang X, Liu Y, Li J, et al. Global trends and performances of magnetic resonance imaging studies on acupuncture: a bibliometric analysis. Front Neurosci. (2021) 14:620555. doi: 10.3389/fnins.2020.620555
24. Liu X, Zhao S, Tan L, Tan Y, Wang Y, Ye Z, et al. Frontier and hot topics in electrochemiluminescence sensing technology based on CiteSpace bibliometric analysis. Biosens Bioelectron. (2022) 201:113932. doi: 10.1016/j.bios.2021.113932
25. Carve M, Allinson G, Nugegoda D, Shimeta J. Trends in environmental and toxicity research on organic ultraviolet filters: a scientometric review. Sci Total Environ. (2021) 773:145628. doi: 10.1016/j.scitotenv.2021.145628
26. Ma D, Yang B, Guan B, Song L, Liu Q, Fan Y, et al. A bibliometric analysis of pyroptosis from 2001 to 2021. Front Immunol. (2021) 12:731933. doi: 10.3389/fimmu.2021.731933
27. Chen C, Hu Z, Liu S, Tseng H. Emerging trends in regenerative medicine: a scientometric analysis in CiteSpace. Expert Opin Biol Ther. (2012) 12(5):593–608. doi: 10.1517/14712598.2012.674507
28. Brandt JS, Hadaya O, Schuster M, Rosen T, Sauer MV, Ananth CV. A bibliometric analysis of top-cited journal articles in obstetrics and gynecology. JAMA network Open. (2019) 2(12):e1918007. doi: 10.1001/jamanetworkopen.2019.18007
29. Devos P, Menard J. Bibliometric analysis of research relating to hypertension reported over the period 1997–2016. J Hypertens. (2019) 37(11):2116–22. doi: 10.1097/HJH.0000000000002143
30. Lin F, Chen Y, Mo W, Zhou H, Xiao Z, Hu S, et al. A bibliometric analysis of autophagy in lung diseases from 2012 to 2021. Front Immunol. (2022) 13:1092575. doi: 10.3389/fimmu.2022.1092575
31. Liu C, Yu R, Zhang J, Wei S, Xue F, Guo Y, et al. Research hotspot and trend analysis in the diagnosis of inflammatory bowel disease: a machine learning bibliometric analysis from 2012 to 2021. Front Immunol. (2022) 13:972079. doi: 10.3389/fimmu.2022.972079
32. Wang L, Zhou S, Liu Y, Li Y, Sun X. Bibliometric analysis of the inflammatory mechanism in aortic disease. Rev Cardiovasc Med. (2022) 23(2):67. doi: 10.31083/j.rcm2302067
33. Wu F, Gao J, Kang J, Wang X, Niu Q, Liu J, et al. Knowledge mapping of exosomes in autoimmune diseases: a bibliometric analysis (2002–2021). Front Immunol. (2022) 13:939433. doi: 10.3389/fimmu.2022.939433
34. van Eck NJ, Waltman L. Citation-based clustering of publications using CitNetExplorer and VOSviewer. Scientometrics. (2017) 111(2):1053–70. doi: 10.1007/s11192-017-2300-7
35. Zimmet JM, Hare JM. Emerging role for bone marrow derived mesenchymal stem cells in myocardial regenerative therapy. Basic Res Cardiol. (2005) 100(6):471–81. doi: 10.1007/s00395-005-0553-4
36. Mazhari R, Hare JM. Mechanisms of action of mesenchymal stem cells in cardiac repair: potential influences on the cardiac stem cell niche. Nat Clin Pract Cardiovasc Med. (2007) 4(Suppl. 1):S21–26. doi: 10.1038/ncpcardio0770
37. Schuleri KH, Amado LC, Boyle AJ, Centola M, Saliaris AP, Gutman MR, et al. Early improvement in cardiac tissue perfusion due to mesenchymal stem cells. Am J Physiol Heart Circ Physiol. (2008) 294(5):H2002–11. doi: 10.1152/ajpheart.00762.2007
38. Hatzistergos KE, Quevedo H, Oskouei BN, Hu Q, Feigenbaum GS, Margitich IS. Bone marrow mesenchymal stem cells stimulate cardiac stem cell proliferation and differentiation. Circ Res. (2010) 107(7):913–22. doi: 10.1161/CIRCRESAHA.110.222703
39. Phinney DG, Pittenger MF. Concise review: MSC-derived exosomes for cell-free therapy. Stem Cells. (2017) 35(4):851–8. doi: 10.1002/stem.2575
40. Kundu B, Rajkhowa R, Kundu SC, Wang X. Silk fibroin biomaterials for tissue regenerations. Adv Drug Delivery Rev. (2013) 65(4):457–70. doi: 10.1016/j.addr.2012.09.043
41. Pittenger MF, Mackay AM, Beck SC, Jaiswal RK, Douglas R, Mosca JD. Multilineage potential of adult human mesenchymal stem cells. Science. (1999) 284(5411):143–7. doi: 10.1126/science.284.5411.143
42. Hare JM, Fishman JE, Gerstenblith G, DiFede Velazquez DL, Zambrano JP, Suncion VY, et al. Comparison of allogeneic vs autologous bone marrow–derived mesenchymal stem cells delivered by transendocardial injection in patients with ischemic cardiomyopathy: the POSEIDON randomized trial. JAMA. (2012) 308(22):2369–79. doi: 10.1001/jama.2012.25321
43. Friedenstein AJ, Piatetzky-Shapiro II, Petrakova KV. Osteogenesis in transplants of bone marrow cells. J Embryol Exp Morphol. (1966) 16(3):381–90. PMID: 5336210
44. Friedenstein AJ, Chailakhjan RK, Lalykina KS. The development of fibroblast colonies in monolayer cultures of Guinea-pig bone marrow and spleen cells. Cell Tissue Kinet. (1970) 3(4):393–403. doi: 10.1111/j.1365-2184.1970.tb00347.x
45. Friedenstein AJ. Stromal mechanisms of bone marrow: cloning in vitro and retransplantation in vivo. Haematol Blood Transfus. (1980) 25:19–29. doi: 10.1007/978-3-642-67319-1_3
46. Caplan AI. Mesenchymal stem cells. J Orthop Res. (1991) 9(5):641–50. doi: 10.1002/jor.1100090504
47. Le Blanc K, Rasmusson I, Sundberg B, Götherström C, Hassan M, Uzunel M, et al. Treatment of severe acute graft-versus-host disease with third party haploidentical mesenchymal stem cells. Lancet. (2004) 363(9419):1439–41. doi: 10.1016/S0140-6736(04)16104-7
48. Sharma A, Gupta S, Archana S, Verma RS. Emerging trends in mesenchymal stem cells applications for cardiac regenerative therapy: current Status and advances. Stem Cell Rev Reports. (2022) 18(5):1546–602. doi: 10.1007/s12015-021-10314-8
49. Maldonado VV, Patel NH, Smith EE, Barnes CL, Gustafson MP, Rao RR, et al. Clinical utility of mesenchymal stem/stromal cells in regenerative medicine and cellular therapy. J Biol Eng. (2023) 17(1):44. doi: 10.1186/s13036-023-00361-9
50. Czerwiec K, Zawrzykraj M, Deptuła M, Skoniecka A, Tymińska A, Zieliński J, et al. Adipose-derived mesenchymal stromal cells in basic research and clinical applications. Int J Mol Sci. (2023) 24(4):3888. doi: 10.3390/ijms24043888
51. Lui NA, Jeyaram G, Henry A. Postpartum interventions to reduce long-term cardiovascular disease risk in women after hypertensive disorders of pregnancy: a systematic review. Front Cardiovasc Med. (2019) 6:160. doi: 10.3389/fcvm.2019.00160
52. Aggarwal S, Pittenger MF. Human mesenchymal stem cells modulate allogeneic immune cell responses. Blood. (2005) 105(4):1815–22. doi: 10.1182/blood-2004-04-1559
53. Wang L-L, Yu Y, Guan H-B, Qiao C. Effect of human umbilical cord mesenchymal stem cell transplantation in a rat model of preeclampsia. Reprod Sci. (2016) 23(8):1058–70. doi: 10.1177/1933719116630417
54. Zhang D, Fu L, Wang L, Lin L, Yu L, Zhang L, et al. Therapeutic benefit of mesenchymal stem cells in pregnant rats with angiotensin receptor agonistic autoantibody-induced hypertension: implications for immunomodulation and cytoprotection. Hypertens Pregnancy. (2017) 36(3):247–58. doi: 10.1080/10641955.2017.1329429
55. Vaka VR, McMaster KM, Cunningham MW, Ibrahim T, Hazlewood R, Usry N, et al. Role of mitochondrial dysfunction and reactive oxygen species in mediating hypertension in the reduced uterine perfusion pressure rat model of preeclampsia. Hypertension. (2018) 72(3):703–11. doi: 10.1161/HYPERTENSIONAHA.118.11290
56. Lin Y, Zhu W, Chen X. The involving progress of MSCs based therapy in atherosclerosis. Stem Cell Res Ther. (2020) 11(1):216. doi: 10.1186/s13287-020-01728-1
57. Grootaert MOJ, Bennett MR. Vascular smooth muscle cells in atherosclerosis: time for a re-assessment. Cardiovasc Res. (2021) 117(11):2326–39. doi: 10.1093/cvr/cvab046
58. Ji ST, Kim H, Yun J, Chung JS, Kwon S-M. Promising therapeutic strategies for mesenchymal stem cell-based cardiovascular regeneration: from cell priming to tissue engineering. Stem Cells Int. (2017) 2017:3945403. doi: 10.1155/2017/3945403
59. Wen Z, Huang W, Feng Y, Cai W, Wang Y, Wang X, et al. MicroRNA-377 regulates mesenchymal stem cell-induced angiogenesis in ischemic hearts by targeting VEGF. PLoS ONE. (2014) 9(9):e104666. doi: 10.1371/journal.pone.0104666
60. Bronckaers A, Hilkens P, Martens W, Gervois P, Ratajczak J, Struys T, et al. Mesenchymal stem/stromal cells as a pharmacological and therapeutic approach to accelerate angiogenesis. Pharmacol Ther. (2014) 143(2):181–96. doi: 10.1016/j.pharmthera.2014.02.013
61. Yao Z, Liu H, Yang M, Bai Y, Zhang B, Wang C, et al. Bone marrow mesenchymal stem cell-derived endothelial cells increase capillary density and accelerate angiogenesis in mouse hindlimb ischemia model. Stem Cell Res Ther. (2020) 11(1):221. doi: 10.1186/s13287-020-01710-x
62. Wang C, Li Y, Yang M, Zou Y, Liu H, Liang Z. Efficient differentiation of bone marrow mesenchymal stem cells into endothelial cells in vitro. Eur J Vasc Endovasc Surg. (2018) 55(2):257–65. doi: 10.1016/j.ejvs.2017.10.012
63. Shi S, Sun J, Meng Q, Yu Y, Huang H, Ma T. Sonic hedgehog promotes endothelial differentiation of bone marrow mesenchymal stem cells via VEGF-D. J Thorac Dis. (2018) 10(9):5476–88. doi: 10.21037/jtd.2018.09.50
64. Zhang Z, Ge L, Zhang S, Wang J, Jiang W, Xin Q, et al. The protective effects of MSC-EXO against pulmonary hypertension through regulating Wnt5a/BMP signalling pathway. J Cell Mol Med. (2020) 24(23):13938–48. doi: 10.1111/jcmm.16002
65. Sun S-J, Wei R, Li F, Liao S-Y, Tse H-F. Mesenchymal stromal cell-derived exosomes in cardiac regeneration and repair. Stem Cell Rep. (2021) 16(7):1662–73. doi: 10.1016/j.stemcr.2021.05.003
66. Suzuki E, Fujita D, Takahashi M, Oba S, Nishimatsu H. Stem cell-derived exosomes as a therapeutic tool for cardiovascular disease. World J Stem Cells. (2016) 8(9):297–305. doi: 10.4252/wjsc.v8.i9.297
67. Dong J, Wu B, Tian W. Exosomes derived from hypoxia-preconditioned mesenchymal stem cells (hypoMSCs-exo): advantages in disease treatment. Cell Tissue Res. (2023) 392(3):621–9. doi: 10.1007/s00441-023-03758-6
68. Nooshabadi VT, Mardpour S, Yousefi-Ahmadipour A, Allahverdi A, Izadpanah M, Daneshimehr F. The extracellular vesicles-derived from mesenchymal stromal cells: a new therapeutic option in regenerative medicine. J Cell Biochem. (2018) 119(10):8048–73. doi: 10.1002/jcb.26726
69. Giovannelli L, Bari E, Jommi C, Tartara F, Armocida D, Garbossa D, et al. Mesenchymal stem cell secretome and extracellular vesicles for neurodegenerative diseases: risk-benefit profile and next steps for the market access. Bioact Mater. (2023) 29:16–35. doi: 10.1016/j.bioactmat.2023.06.013
70. Arslan F, Lai RC, Smeets MB, Akeroyd L, Choo A, Aguor ENE. Mesenchymal stem cell-derived exosomes increase ATP levels, decrease oxidative stress and activate PI3K/AKT pathway to enhance myocardial viability and prevent adverse remodeling after myocardial ischemia/reperfusion injury. Stem Cell Res. (2013) 10(3):301–12. doi: 10.1016/j.scr.2013.01.002
71. Bian S, Zhang L, Duan L, Wang X, Min Y, Yu H. Extracellular vesicles derived from human bone marrow mesenchymal stem cells promote angiogenesis in a rat myocardial infarction model. J Mol Med. (2014) 92(4):387–97. doi: 10.1007/s00109-013-1110-5
72. Teng X, Chen L, Chen W, Yang J, Yang Z, Shen Z. Mesenchymal stem cell-derived exosomes improve the microenvironment of infarcted myocardium contributing to angiogenesis and anti-inflammation. Cell Physiol Biochem. (2015) 37(6):2415–24. doi: 10.1159/000438594
73. Huerta CT, Voza FA, Ortiz YY, Liu Z-J, Velazquez OC. Mesenchymal stem cell-based therapy for non-healing wounds due to chronic limb-threatening ischemia: a review of preclinical and clinical studies. Front Cardiovasc Med. (2023) 10:1113982. doi: 10.3389/fcvm.2023.1113982
74. Debin L, Youzhao J, Ziwen L, Xiaoyan L, Zhonghui Z, Bing C. Autologous transplantation of bone marrow mesenchymal stem cells on diabetic patients with lower limb ischemia. J Med Coll PLA. (2008) 23(2):106–15. doi: 10.1016/S1000-1948(08)60031-3
75. Park JS, Bae S-H, Jung S, Lee M, Choi D. Enrichment of vascular endothelial growth factor secreting mesenchymal stromal cells enhances therapeutic angiogenesis in a mouse model of hind limb ischemia. Cytotherapy. (2019) 21(4):433–43. doi: 10.1016/j.jcyt.2018.12.007
76. Shirbaghaee Z, Hassani M, Heidari Keshel S, Soleimani M. Emerging roles of mesenchymal stem cell therapy in patients with critical limb ischemia. Stem Cell Res Ther. (2022) 13(1):462. doi: 10.1186/s13287-022-03148-9
77. Oveili E, Vafaei S, Bazavar H, Eslami Y, Mamaghanizadeh E, Yasamineh S, et al. The potential use of mesenchymal stem cells-derived exosomes as microRNAs delivery systems in different diseases. Cell Commun Signal. (2023) 21(1):20. doi: 10.1186/s12964-022-01017-9
78. Shao L, Zhang Y, Lan B, Wang J, Zhang Z, Zhang L. MiRNA-sequence indicates that mesenchymal stem cells and exosomes have similar mechanism to enhance cardiac repair. BioMed Res Int. (2017) 2017:e4150705. doi: 10.1155/2017/4150705
79. Wei Z, Qiao S, Zhao J, Liu Y, Li Q, Wei Z, et al. miRNA-181a over-expression in mesenchymal stem cell-derived exosomes influenced inflammatory response after myocardial ischemia-reperfusion injury. Life Sci. (2019) 232:116632. doi: 10.1016/j.lfs.2019.116632
80. Zhang Z, Yang J, Yan W, Li Y, Shen Z, Asahara T. Pretreatment of cardiac stem cells with exosomes derived from mesenchymal stem cells enhances myocardial repair. J Am Heart Assoc. (2016) 5(1):e002856. doi: 10.1161/JAHA.115.002856
81. Ektesabi AM, Mori K, Tsoporis JN, Vaswani CM, Gupta S, Walsh C. Mesenchymal stem/stromal cells increase cardiac miR-187-3p expression in a polymicrobial animal model of sepsis. Shock. (2021) 56(1):133. doi: 10.1097/SHK.0000000000001701
82. Luther KM, Haar L, McGuinness M, Wang Y, Lynch IV TL, Phan A. Exosomal miR-21a-5p mediates cardioprotection by mesenchymal stem cells. J Mol Cell Cardiol. (2018) 119:125–37. doi: 10.1016/j.yjmcc.2018.04.012
83. Xu B, Luo Y, Liu Y, Li B-Y, Wang Y. Platelet-derived growth factor-BB enhances MSC-mediated cardioprotection via suppression of miR-320 expression. Am J Physiol Heart Circ Physiol. (2015) 308(9):H980–989. doi: 10.1152/ajpheart.00737.2014
84. Yang C, Luo M, Chen Y, You M, Chen Q. MicroRNAs as important regulators mediate the multiple differentiation of mesenchymal stromal cells. Front Cell Dev Biol. (2021) 9:619842. doi: 10.3389/fcell.2021.619842
85. Wang N, Chen C, Yang D, Liao Q, Luo H, Wang X, et al. Mesenchymal stem cells-derived extracellular vesicles, via miR-210, improve infarcted cardiac function by promotion of angiogenesis. Biochim Biophys Acta Mol Basis Dis. (2017) 1863(8):2085–92. doi: 10.1016/j.bbadis.2017.02.023
86. Chinnici CM, Iannolo G, Cittadini E, Carreca AP, Nascari D, Timoneri F, et al. Extracellular vesicle-derived microRNAs of human wharton’s jelly mesenchymal stromal cells may activate endogenous VEGF-A to promote angiogenesis. Int J Mol Sci. (2021) 22(4):2045. doi: 10.3390/ijms22042045
87. Pan L, Yan B, Zhang J, Zhao P, Jing Y, Yu J, et al. Mesenchymal stem cells-derived extracellular vesicles-shuttled microRNA-223-3p suppress lipopolysaccharide-induced cardiac inflammation, pyroptosis, and dysfunction. Int Immunopharmacol. (2022) 110:108910. doi: 10.1016/j.intimp.2022.108910
88. Shi B, Wang Y, Zhao R, Long X, Deng W, Wang Z. Bone marrow mesenchymal stem cell-derived exosomal miR-21 protects C-kit+ cardiac stem cells from oxidative injury through the PTEN/PI3K/AKT axis. PLoS ONE. (2018) 13(2):e0191616. doi: 10.1371/journal.pone.0191616
89. Wang K, Jiang Z, Webster KA, Chen J, Hu H, Zhou Y. Enhanced cardioprotection by human endometrium mesenchymal stem cells driven by exosomal MicroRNA-21. Stem Cells Transl Med. (2017) 6(1):209–22. doi: 10.5966/sctm.2015-0386
90. Yu B, Gong M, Wang Y, Millard RW, Pasha Z, Yang Y. Cardiomyocyte protection by GATA-4 gene engineered mesenchymal stem cells is partially mediated by translocation of miR-221 in microvesicles. PLoS ONE. (2013) 8(8):e73304. doi: 10.1371/journal.pone.0073304
91. Yu T, Li YJ, Bian AH, Zuo HB, Zhu TW, Ji SX. The regulatory role of activating transcription factor 2 in inflammation. Mediat Inflamm. (2014) 2014:950472. doi: 10.1155/2014/950472
92. Botello-Flores YA, Yocupicio-Monroy M, Balderrábano-Saucedo N, Contreras-Ramos A. A systematic review on the role of MSC-derived exosomal miRNAs in the treatment of heart failure. Mol Biol Rep. (2022) 49(9):8953–73. doi: 10.1007/s11033-022-07385-2
93. Kirsch K, Sok P, Reményi A. Structural reconstruction of protein-protein complexes involved in intracellular signaling. Adv Exp Med Biol. (2016) 896:315–26. doi: 10.1007/978-3-319-27216-0_20
94. Tian C, Gao L, Zucker IH. Regulation of Nrf2 signaling pathway in heart failure: role of extracellular vesicles and non-coding RNAs. Free Radic Biol Med. (2021) 167:218–31. doi: 10.1016/j.freeradbiomed.2021.03.013
95. Laura Francés J, Pagiatakis C, Di Mauro V, Climent M. Therapeutic potential of EVs: targeting cardiovascular diseases. Biomedicines. (2023) 11(7):1907. doi: 10.3390/biomedicines11071907
96. Chang C, Cai R-P, Su Y-M, Wu Q, Su Q. Mesenchymal stem cell-derived exosomal noncoding RNAs as alternative treatments for myocardial ischemia-reperfusion injury: current Status and future perspectives. J Cardiovasc Transl Res. (2023) 16(5):1085–98. doi: 10.1007/s12265-023-10401-w
97. Dai F, Du P, Chang Y, Ji E, Xu Y, Wei C, et al. Downregulation of miR-199b-5p inducing differentiation of bone-marrow mesenchymal stem cells (BMSCs) toward cardiomyocyte-like cells via HSF1/HSP70 pathway. Med Sci Monit. (2018) 24:2700–10. doi: 10.12659/MSM.907441
98. Azizidoost S, Farzaneh M. MicroRNAs as a novel player for differentiation of mesenchymal stem cells into cardiomyocytes. Curr Stem Cell Res Ther. (n.d.) 18(1):27–34. doi: 10.2174/1574888X17666220422094150
99. Xu R, Zhao M, Yang Y, Huang Z, Shi C, Hou X. MicroRNA-449c-5p inhibits osteogenic differentiation of human VICs through Smad4-mediated pathway. Sci Rep. (2017) 7(1):8740. doi: 10.1038/s41598-017-09390-z
100. Wang P, Zhang C, Li J, Luo L, Zhang S, Dong F. Adipose-derived mesenchymal stromal cells improve hemodynamic function in pulmonary arterial hypertension: identification of microRNAs implicated in modulating endothelial function. Cytotherapy. (2019) 21(4):416–27. doi: 10.1016/j.jcyt.2019.02.011
101. Sun X-H, Wang X, Zhang Y, Hui J. Exosomes of bone-marrow stromal cells inhibit cardiomyocyte apoptosis under ischemic and hypoxic conditions via miR-486-5p targeting the PTEN/PI3K/AKT signaling pathway. Thromb Res. (2019) 177:23–32. doi: 10.1016/j.thromres.2019.02.002
102. Lei B, Wu X, Xia K, Sun H, Wang J. Exosomal micro-RNA-96 derived from bone marrow mesenchymal stem cells inhibits doxorubicin-induced myocardial toxicity by inhibiting the Rac1/nuclear factor-κB signaling pathway. J Am Heart Assoc. (2021) 10(17):e020589. doi: 10.1161/JAHA.120.020589
103. Wen Z, Mai Z, Zhu X, Wu T, Chen Y, Geng D, et al. Mesenchymal stem cell-derived exosomes ameliorate cardiomyocyte apoptosis in hypoxic conditions through microRNA144 by targeting the PTEN/AKT pathway. Stem Cell Res Ther. (2020) 11(1):36. doi: 10.1186/s13287-020-1563-8
104. Anderson JD, Johansson HJ, Graham CS, Vesterlund M, Pham MT, Bramlett CS. Comprehensive proteomic analysis of mesenchymal stem cell exosomes reveals modulation of angiogenesis via nuclear factor-KappaB signaling. Stem Cells (Dayton, Ohio). (2016) 34(3):601–13. doi: 10.1002/stem.2298
105. Gonzalez-King H, García NA, Ontoria-Oviedo I, Ciria M, Montero JA, Sepúlveda P. Hypoxia inducible factor-1α potentiates jagged 1-mediated angiogenesis by mesenchymal stem cell-derived exosomes. Stem Cells. (2017) 35(7):1747–59. doi: 10.1002/stem.2618
106. Takeuchi R, Katagiri W, Endo S, Kobayashi T. Exosomes from conditioned media of bone marrow-derived mesenchymal stem cells promote bone regeneration by enhancing angiogenesis. PLoS ONE. (2019) 14(11):e0225472. doi: 10.1371/journal.pone.0225472
107. Deng H, Sun C, Sun Y, Li H, Yang L, Wu D. Lipid, protein, and MicroRNA composition within mesenchymal stem cell-derived exosomes. Cell Reprogram. (2018) 20(3):178–86. doi: 10.1089/cell.2017.0047
108. Lai RC, Yeo RWY, Lim SK. Mesenchymal stem cell exosomes. Semin Cell Dev Biol. (2015) 40:82–8. doi: 10.1016/j.semcdb.2015.03.001
109. Lai RC, Chen TS, Lim SK. Mesenchymal stem cell exosome: a novel stem cell-based therapy for cardiovascular disease. Regen Med. (2011) 6(4):481–92. doi: 10.2217/rme.11.35
110. Yin X, Lin L, Fang F, Zhang B, Shen C. Mechanisms and optimization strategies of paracrine exosomes from mesenchymal stem cells in ischemic heart disease. Stem Cells Int. (2023) 2023:6500831. doi: 10.1155/2023/6500831
111. Lai RC, Yeo RWY, Tan KH, Lim SK. Mesenchymal stem cell exosome ameliorates reperfusion injury through proteomic complementation. Regen Med. (2013) 8(2):197–209. doi: 10.2217/rme.13.4
112. Zhao J, Li X, Hu J, Chen F, Qiao S, Sun X, et al. Mesenchymal stromal cell-derived exosomes attenuate myocardial ischaemia-reperfusion injury through miR-182-regulated macrophage polarization. Cardiovasc Res. (2019) 115(7):1205–16. doi: 10.1093/cvr/cvz040
113. Lai RC, Arslan F, Lee MM, Sze NSK, Choo A, Chen TS. Exosome secreted by MSC reduces myocardial ischemia/reperfusion injury. Stem Cell Res. (2010) 4(3):214–22. doi: 10.1016/j.scr.2009.12.003
114. Liu L, Jin X, Hu C-F, Li R, Zhou Z, Shen C-X. Exosomes derived from mesenchymal stem cells rescue myocardial ischaemia/reperfusion injury by inducing cardiomyocyte autophagy via AMPK and AKT pathways. Cell Physiol Biochem. (2017) 43(1):52–68. doi: 10.1159/000480317
115. Tan SS, Yin Y, Lee T, Lai RC, Yeo RWY, Zhang B. Therapeutic MSC exosomes are derived from lipid raft microdomains in the plasma membrane. J Extracell Vesicles. (2013) 2(1):22614. doi: 10.3402/jev.v2i0.22614
116. Miceli V, Bulati M, Gallo A, Iannolo G, Busà R, Conaldi PG, et al. Role of mesenchymal stem/stromal cells in modulating ischemia/reperfusion injury: current state of the art and future perspectives. Biomedicines. (2023) 11(3):689. doi: 10.3390/biomedicines11030689
117. Okazaki T, Ebihara S, Asada M, Yamanda S, Saijo Y, Shiraishi Y. Macrophage colony-stimulating factor improves cardiac function after ischemic injury by inducing vascular endothelial growth factor production and survival of cardiomyocytes. Am J Pathol. (2007) 171(4):1093–103. doi: 10.2353/ajpath.2007.061191
118. Zgheib C, Zouein FA, Kurdi M, Booz GW. Chronic treatment of mice with leukemia inhibitory factor does not cause adverse cardiac remodeling but improves heart function. Eur Cytokine Network. (2012) 23(4):191–7. doi: 10.1684/ecn.2012.0319
119. Formiga FR, Pelacho B, Garbayo E, Imbuluzqueta I, Díaz-Herráez P, Abizanda G, et al. Controlled delivery of fibroblast growth factor-1 and neuregulin-1 from biodegradable microparticles promotes cardiac repair in a rat myocardial infarction model through activation of endogenous regeneration. J Control Release. (2014) 173:132–9. doi: 10.1016/j.jconrel.2013.10.034
120. Gu X, Liu X, Xu D, Li X, Yan M, Qi Y, et al. Cardiac functional improvement in rats with myocardial infarction by up-regulating cardiac myosin light chain kinase with neuregulin. Cardiovasc Res. (2010) 88(2):334–43. doi: 10.1093/cvr/cvq223
121. Palmen M, Daemen MJAP, De Windt LJ, Willems J, Dassen WRM, Heeneman S, et al. Fibroblast growth factor-1 improves cardiac functional recovery and enhances cell survival after ischemia and reperfusion: a fibroblast growth factor receptor, protein kinase C, and tyrosine kinase-dependent mechanism. J Am Coll Cardiol. (2004) 44(5):1113–23. doi: 10.1016/j.jacc.2004.05.067
122. Trachtenberg B, Velazquez DL, Williams AR, McNiece I, Fishman J, Nguyen K, et al. Rationale and design of the transendocardial injection of autologous human cells (bone marrow or mesenchymal) in chronic ischemic left ventricular dysfunction and heart failure secondary to myocardial infarction (TAC-HFT) trial: a randomized, double-blind, placebo-controlled study of safety and efficacy. Am Heart J. (2011) 161(3):487–93. doi: 10.1016/j.ahj.2010.11.024
123. Mathiasen AB, Qayyum AA, Jørgensen E, Helqvist S, Fischer-Nielsen A, Kofoed KF, et al. Bone marrow-derived mesenchymal stromal cell treatment in patients with severe ischaemic heart failure: a randomized placebo-controlled trial (MSC-HF trial). Eur Heart J. (2015) 36(27):1744–53. doi: 10.1093/eurheartj/ehv136
124. Bartolucci J, Verdugo FJ, González PL, Larrea RE, Abarzua E, Goset C, et al. Safety and efficacy of the intravenous infusion of umbilical cord mesenchymal stem cells in patients with heart failure: a phase 1/2 randomized controlled trial (RIMECARD trial (randomized clinical trial of intravenous infusion umbilical cord mesenchymal stem cells on cardiopathy)). Circ Res. (2017) 121(10):1192–204. doi: 10.1161/CIRCRESAHA.117.310712
125. Kaushal S, Wehman B, Pietris N, Naughton C, Bentzen SM, Bigham G, et al. Study design and rationale for ELPIS: a phase I/IIb randomized pilot study of allogeneic human mesenchymal stem cell injection in patients with hypoplastic left heart syndrome. Am Heart J. (2017) 192:48–56. doi: 10.1016/j.ahj.2017.06.009
126. Butler J, Epstein SE, Greene SJ, Quyyumi AA, Sikora S, Kim RJ, et al. Intravenous allogeneic mesenchymal stem cells for nonischemic cardiomyopathy: safety and efficacy results of a phase II-A randomized trial. Circ Res. (2017) 120(2):332–40. doi: 10.1161/CIRCRESAHA.116.309717
127. Kim SH, Cho JH, Lee YH, Lee JH, Kim SS, Kim MY, et al. Improvement in left ventricular function with intracoronary mesenchymal stem cell therapy in a patient with anterior wall ST-segment elevation myocardial infarction. Cardiovasc Drugs Ther. (2018) 32(4):329–38. doi: 10.1007/s10557-018-6804-z
128. Mathiasen AB, Qayyum AA, Jørgensen E, Helqvist S, Kofoed KF, Haack-Sørensen M, et al. Bone marrow-derived mesenchymal stromal cell treatment in patients with ischaemic heart failure: final 4-year follow-up of the MSC-HF trial. Eur J Heart Fail. (2020) 22(5):884–92. doi: 10.1002/ejhf.1700
129. Karantalis V, DiFede DL, Gerstenblith G, Pham S, Symes J, Zambrano JP, et al. Autologous mesenchymal stem cells produce concordant improvements in regional function, tissue perfusion, and fibrotic burden when administered to patients undergoing coronary artery bypass grafting: the prospective randomized study of mesenchymal stem cell therapy in patients undergoing cardiac surgery (PROMETHEUS) trial. Circ Res. (2014) 114(8):1302–10. doi: 10.1161/CIRCRESAHA.114.303180
130. Golpanian S, El-Khorazaty J, Mendizabal A, DiFede DL, Suncion VY, Karantalis V, et al. Effect of aging on human mesenchymal stem cell therapy in ischemic cardiomyopathy patients. J Am Coll Cardiol. (2015) 65(2):125–32. doi: 10.1016/j.jacc.2014.10.040
131. Ramireddy A, Brodt CR, Mendizabal AM, DiFede DL, Healy C, Goyal V, et al. Effects of transendocardial stem cell injection on ventricular proarrhythmia in patients with ischemic cardiomyopathy: results from the POSEIDON and TAC-HFT trials. Stem Cells Transl Med. (2017) 6(5):1366–72. doi: 10.1002/sctm.16-0328
132. Florea V, Rieger AC, Natsumeda M, Tompkins BA, Banerjee MN, Schulman IH, et al. The impact of patient sex on the response to intramyocardial mesenchymal stem cell administration in patients with non-ischaemic dilated cardiomyopathy. Cardiovasc Res. (2020) 116(13):2131–41. doi: 10.1093/cvr/cvaa004
133. Qayyum AA, van Klarenbosch B, Frljak S, Cerar A, Poglajen G, Traxler-Weidenauer D. Effect of allogeneic adipose tissue-derived mesenchymal stromal cell treatment in chronic ischaemic heart failure with reduced ejection fraction—the SCIENCE trial. Eur J Heart Fail. (2023) 25(4):576–87. doi: 10.1002/ejhf.2772
134. Qayyum AA, Mouridsen M, Nilsson B, Gustafsson I, Schou M, Nielsen OW, et al. Danish phase II trial using adipose tissue derived mesenchymal stromal cells for patients with ischaemic heart failure. ESC Heart Fail. (2023) 10(2):1170–83. doi: 10.1002/ehf2.14281
135. Olmedo-Moreno L, Aguilera Y, Baliña-Sánchez C, Martín-Montalvo A, Capilla-González V. Heterogeneity of in vitro expanded mesenchymal stromal cells and strategies to improve their therapeutic actions. Pharmaceutics. (2022) 14(5):1112. doi: 10.3390/pharmaceutics14051112
136. Schäfer R. Advanced cell therapeutics are changing the clinical landscape: will mesenchymal stromal cells be a part of it? BMC Med. (2019) 17(1):53. doi: 10.1186/s12916-019-1289-6
137. Bortolotti F, Ukovich L, Razban V, Martinelli V, Ruozi G, Pelos B, et al. In vivo therapeutic potential of mesenchymal stromal cells depends on the source and the isolation procedure. Stem Cell Rep. (2015) 4(3):332–9. doi: 10.1016/j.stemcr.2015.01.001
138. Reiss AB, Ahmed S, Johnson M, Saeedullah U, De Leon J. Exosomes in cardiovascular disease: from mechanism to therapeutic target. Metabolites. (2023) 13(4):479. doi: 10.3390/metabo13040479
139. Zhang X, Huang F, Chen Y, Qian X, Zheng SG. Progress and prospect of mesenchymal stem cell-based therapy in atherosclerosis. Am J Transl Res. (2016) 8(10):4017–24. PMID: 27829989
140. Kirwin T, Gomes A, Amin R, Sufi A, Goswami S, Wang B. Mechanisms underlying the therapeutic potential of mesenchymal stem cells in atherosclerosis. Regen Med. (2021) 16(7):669–82. doi: 10.2217/rme-2021-0024
141. Freyman T, Polin G, Osman H, Crary J, Lu M, Cheng L, et al. A quantitative, randomized study evaluating three methods of mesenchymal stem cell delivery following myocardial infarction. Eur Heart J. (2006) 27(9):1114–22. doi: 10.1093/eurheartj/ehi818
142. Yan W, Xia Y, Zhao H, Xu X, Ma X, Tao L. Stem cell-based therapy in cardiac repair after myocardial infarction: promise, challenges, and future directions. J Mol Cell Cardiol. (2024) 188:1–14. doi: 10.1016/j.yjmcc.2023.12.009
143. Karp JM, Leng Teo GS. Mesenchymal stem cell homing: the devil is in the details. Cell Stem Cell. (2009) 4(3):206–16. doi: 10.1016/j.stem.2009.02.001
144. Guo X, Bai Y, Zhang L, Zhang B, Zagidullin N, Carvalho K, et al. Cardiomyocyte differentiation of mesenchymal stem cells from bone marrow: new regulators and its implications. Stem Cell Res Ther. (2018) 9(1):44. doi: 10.1186/s13287-018-0773-9
145. Alzghoul Y, Issa HJB, Sanajleh AK, Alabduh T, Rababah F, Al-Shdaifat M, et al. Therapeutic and regenerative potential of different sources of mesenchymal stem cells for cardiovascular diseases. Biocell. (2024) 48(4):559–69. doi: 10.32604/biocell.2024.048056
146. Ma H, Siu W-S, Leung P-C. The potential of MSC-based cell-free therapy in wound healing—a thorough literature review. Int J Mol Sci. (2023) 24(11):9356. doi: 10.3390/ijms24119356
147. Torizal F, Fiano F, Khumaira A. Production of mesenchymal stem cell derived-secretome as cell-free regenerative therapy and immunomodulation: a biomanufacturing perspective. BIOCELL. (2022) 46(8):1885–91. doi: 10.32604/biocell.2022.019591
148. Ahangar P, Mills SJ, Cowin AJ. Mesenchymal stem cell secretome as an emerging cell-free alternative for improving wound repair. Int J Mol Sci. (2020) 21(19):7038. doi: 10.3390/ijms21197038
149. Teixeira FG, Salgado AJ. Mesenchymal stem cells secretome: current trends and future challenges. Neural Regen Res. (2019) 15(1):75–7. doi: 10.4103/1673-5374.264455
150. Sahoo S, Losordo DW. Exosomes and cardiac repair after myocardial infarction. Circ Res. (2014) 114(2):333–44. doi: 10.1161/CIRCRESAHA.114.300639
151. Kumar MA, Baba SK, Sadida HQ, Marzooqi SA, Jerobin J, Altemani FH, et al. Extracellular vesicles as tools and targets in therapy for diseases. Signal Transduct Target Ther. (2024) 9(1):27. doi: 10.1038/s41392-024-01735-1
152. Yue M, Hu S, Sun H, Tuo B, Jia B, Chen C, et al. Extracellular vesicles remodel tumor environment for cancer immunotherapy. Mol Cancer. (2023) 22:203. doi: 10.1186/s12943-023-01898-5
153. Kalluri R, McAndrews KM. The role of extracellular vesicles in cancer. Cell. (2023) 186(8):1610–26. doi: 10.1016/j.cell.2023.03.010
154. Grange C, Bussolati B. Extracellular vesicles in kidney disease. Nat Rev Nephrol. (2022) 18(8):499–513. doi: 10.1038/s41581-022-00586-9
155. Karpman D, Ståhl A-L, Arvidsson I. Extracellular vesicles in renal disease. Nat Rev Nephrol. (2017) 13(9):545–62. doi: 10.1038/nrneph.2017.98
156. Boulanger CM, Loyer X, Rautou P-E, Amabile N. Extracellular vesicles in coronary artery disease. Nat Rev Cardiol. (2017) 14(5):259–72. doi: 10.1038/nrcardio.2017.7
157. de Abreu RC, Fernandes H, da Costa Martins PA, Sahoo S, Emanueli C, Ferreira L. Native and bioengineered extracellular vesicles for cardiovascular therapeutics. Nat Rev Cardiol. (2020) 17(11):685–97. doi: 10.1038/s41569-020-0389-5
158. Garofalo M, Villa A, Crescenti D, Marzagalli M, Kuryk L, Limonta P, et al. Heterologous and cross-species tropism of cancer-derived extracellular vesicles. Theranostics. (2019) 9(19):5681–93. doi: 10.7150/thno.34824
159. Maji S, Yan IK, Parasramka M, Mohankumar S, Matsuda A, Patel T. In vitro toxicology studies of extracellular vesicles. J Appl Toxicol. (2017) 37(3):310–8. doi: 10.1002/jat.3362
160. Harrell CR, Miloradovic D, Sadikot R, Fellabaum C, Markovic BS, Miloradovic D, et al. Molecular and cellular mechanisms responsible for beneficial effects of mesenchymal stem cell-derived product ‘exo-d-MAPPS’ in attenuation of chronic airway inflammation. Anal Cell Pathol (Amst). (2020) 2020:3153891. doi: 10.1155/2020/3153891
161. Sengupta V, Sengupta S, Lazo A, Woods P, Nolan A, Bremer N. Exosomes derived from bone marrow mesenchymal stem cells as treatment for severe COVID-19. Stem Cells Dev. (2020) 29(12):747–54. doi: 10.1089/scd.2020.0080
162. Shi M-M, Yang Q-Y, Monsel A, Yan J-Y, Dai C-X, Zhao J-Y, et al. Preclinical efficacy and clinical safety of clinical-grade nebulized allogenic adipose mesenchymal stromal cells-derived extracellular vesicles. J Extracell Vesicles. (2021) 10(10):e12134. doi: 10.1002/jev2.12134
163. Chu M, Wang H, Bian L, Huang J, Wu D, Zhang R, et al. Nebulization therapy with umbilical cord mesenchymal stem cell-derived exosomes for COVID-19 pneumonia. Stem Cell Rev Reports. (2022) 18(6):2152–63. doi: 10.1007/s12015-022-10398-w
164. Lightner AL, Sengupta V, Qian S, Ransom JT, Suzuki S, Park DJ, et al. Bone marrow mesenchymal stem cell-derived extracellular vesicle infusion for the treatment of respiratory failure from COVID-19: a randomized, placebo-controlled dosing clinical trial. Chest. (2023) 164(6):1444–53. doi: 10.1016/j.chest.2023.06.024
165. Akbay E, Onur MA. Investigation of survival and migration potential of differentiated cardiomyocytes transplanted with decellularized heart scaffold. J Biomed Mater Res A. (2019) 107(3):561–70. doi: 10.1002/jbm.a.36572
166. Konoplyannikov M, Kotova S, Baklaushev V, Konoplyannikov A, Kalsin V, Timashev P, et al. Mesenchymal stem cell therapy for ischemic heart disease: advances and challenges. Curr Pharm Des. (2018) 24(26):3132–42. doi: 10.2174/1381612824666180913151059
167. Kang P-L, Chen C-H, Chen SY, Wu Y-J, Lin CY, Lin F-H, et al. Nano-sized collagen I molecules enhanced the differentiation of rat mesenchymal stem cells into cardiomyocytes. J Biomed Mater Res A. (2013) 101(10):2808–16. doi: 10.1002/jbm.a.34589
168. Wu Y-J, Chen SY, Chang SJ, Kuo SM. Enhanced differentiation of rat MSCs into cardiomyocytes with 5-azacytidine/collagen I nano-molecules. Annual International Conference of the IEEE Engineering in Medicine and Biology Society. IEEE Engineering in Medicine and Biology Society. Annual International Conference (2013). p. 322–5
169. Mohammadi Nasr S, Rabiee N, Hajebi S, Ahmadi S, Fatahi Y, Hosseini M, et al. Biodegradable nanopolymers in cardiac tissue engineering: from concept towards nanomedicine. Int J Nanomed. (2020) 15:4205–24. doi: 10.2147/IJN.S245936
170. Raghav PK, Mann Z, Ahlawat S, Mohanty S. Mesenchymal stem cell-based nanoparticles and scaffolds in regenerative medicine. Eur J Pharmacol. (2022) 918:174657. doi: 10.1016/j.ejphar.2021.174657
171. Park J, Kim B, Han J, Oh J, Park S, Ryu S, et al. Graphene oxide flakes as a cellular adhesive: prevention of reactive oxygen species mediated death of implanted cells for cardiac repair. ACS Nano. (2015) 9(5):4987–99. doi: 10.1021/nn507149w
172. Park J, Park S, Ryu S, Bhang SH, Kim J, Yoon J-K, et al. Graphene-regulated cardiomyogenic differentiation process of mesenchymal stem cells by enhancing the expression of extracellular matrix proteins and cell signaling molecules. Adv Healthcare Mater. (2014) 3(2):176–81. doi: 10.1002/adhm.201300177
173. Shou K, Huang Y, Qi B, Hu X, Ma Z, Lu A, et al. Induction of mesenchymal stem cell differentiation in the absence of soluble inducer for cutaneous wound regeneration by a chitin nanofiber-based hydrogel. J Tissue Eng Regen Med. (2018) 12(2):e867–80. doi: 10.1002/term.2400
174. Li J, Ding Z, Zheng X, Lu G, Lu Q, Kaplan DL. Injectable silk nanofiber hydrogels as stem cell carriers to accelerate wound healing. J Mater Chem B. (2021) 9(37):7771–81. doi: 10.1039/D1TB01320C
175. He S, Zhang Z, Luo R, Jiang Q, Yang L, Wang Y. Advances in injectable hydrogel strategies for heart failure treatment. Adv Healthcare Mater. (2023) 12(19):2300029. doi: 10.1002/adhm.202300029
176. Norahan MH, Pedroza-González SC, Sánchez-Salazar MG, Álvarez MM, Trujillo de Santiago G. Structural and biological engineering of 3D hydrogels for wound healing. Bioact Mater. (2022) 24:197–235. doi: 10.1016/j.bioactmat.2022.11.019
177. Chen S, Shi J, Zhang M, Chen Y, Wang X, Zhang L, et al. Mesenchymal stem cell-laden anti-inflammatory hydrogel enhances diabetic wound healing. Sci Rep. (2015) 5:18104. doi: 10.1038/srep18104
178. Lei Z., Singh G., Min Z., Shixuan C., Xu K., Pengcheng X, Lin Z. (2018). Bone marrow-derived mesenchymal stem cells laden novel thermo-sensitive hydrogel for the management of severe skin wound healing. Mater Sci Eng C Mater Biol Appl, 90, 159–67. doi: 10.1016/j.msec.2018.04.045
179. Reis LA, Chiu LLY, Feric N, Fu L, Radisic M. Biomaterials in myocardial tissue engineering. J Tissue Eng Regen Med. (2016) 10(1):11–28. doi: 10.1002/term.1944
180. Pascual-Gil S, Garbayo E, Díaz-Herráez P, Prosper F, Blanco-Prieto MJ. Heart regeneration after myocardial infarction using synthetic biomaterials. J Control Release. (2015) 203:23–38. doi: 10.1016/j.jconrel.2015.02.009
181. Wang H, Shi J, Wang Y, Yin Y, Wang L, Liu J, et al. Promotion of cardiac differentiation of brown adipose derived stem cells by chitosan hydrogel for repair after myocardial infarction. Biomaterials. (2014) 35(13):3986–98. doi: 10.1016/j.biomaterials.2014.01.021
Keywords: mesenchymal stem cells, cardiovascular diseases, exosome, therapeutic target, bibliometric study
Citation: Jiang Z, Yu J, Zhou H, Feng J, Xu Z, Wan M, Zhang W, He Y, Jia C, Shao S, Guo H and Liu B (2024) Research hotspots and emerging trends of mesenchymal stem cells in cardiovascular diseases: a bibliometric-based visual analysis. Front. Cardiovasc. Med. 11:1394453. doi: 10.3389/fcvm.2024.1394453
Received: 1 March 2024; Accepted: 17 May 2024;
Published: 30 May 2024.
Edited by:
Jyotsna Joshi, The Ohio State University, United StatesReviewed by:
Ajit Magadum, Temple University, United States© 2024 Jiang, Yu, Zhou, Feng, Xu, Wan, Zhang, He, Jia, Shao, Guo and Liu. This is an open-access article distributed under the terms of the Creative Commons Attribution License (CC BY). The use, distribution or reproduction in other forums is permitted, provided the original author(s) and the copyright owner(s) are credited and that the original publication in this journal is cited, in accordance with accepted academic practice. No use, distribution or reproduction is permitted which does not comply with these terms.
*Correspondence: Haidong Guo, aGRndW84QGhvdG1haWwuY29t
Baonian Liu, Ym5saXUxNUBmdWRhbi5lZHUuY24=
†These authors have contributed equally to this work
Disclaimer: All claims expressed in this article are solely those of the authors and do not necessarily represent those of their affiliated organizations, or those of the publisher, the editors and the reviewers. Any product that may be evaluated in this article or claim that may be made by its manufacturer is not guaranteed or endorsed by the publisher.
Research integrity at Frontiers
Learn more about the work of our research integrity team to safeguard the quality of each article we publish.