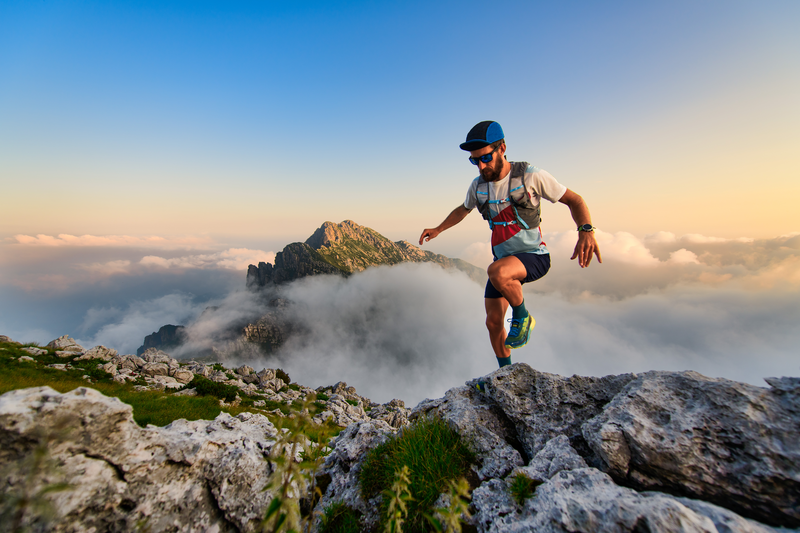
94% of researchers rate our articles as excellent or good
Learn more about the work of our research integrity team to safeguard the quality of each article we publish.
Find out more
ORIGINAL RESEARCH article
Front. Cardiovasc. Med. , 02 April 2024
Sec. Cardiovascular Imaging
Volume 11 - 2024 | https://doi.org/10.3389/fcvm.2024.1357349
Introduction: Myocardial tissue alterations in patients with post-Coronavirus disease 2019 syndrome (PCS) are often subtle and mild. Reports vary in the prevalence of non-ischemic and ischemic injuries as well as the extent of ongoing myocardial inflammation. The exact relevance of these myocardial alterations is not fully understood. This study aimed at describing the trajectories of myocardial alterations in PCS patients by mid-term follow-up with cardiovascular magnetic resonance (CMR).
Methods: This study entails a retrospective analysis of symptomatic PCS patients referred for follow-up CMR between August 2020 and May 2023 due to mildly affected or reduced left or right ventricular function (LV and RV, respectively) and structural myocardial alterations, e.g., focal and diffuse fibrosis, on baseline scans. Follow-up CMR protocol consisted of cine images and full coverage native T1 and T2 mapping. Baseline and follow-up scans were compared using t-tests or Wilcoxon tests. Post-hoc analysis was carried out in a subgroup based on the change of LV stroke volume (SV) between scans.
Results: In total, 43 patients [median age (interquartile range) 46 (37–56) years, 33 women] received follow-ups 347 (167–651) days after initial diagnosis. A decrease in symptoms was recorded on follow-ups (p < 0.03) with 23 patients being asymptomatic at follow-ups [symptomatic at baseline 43/43 (100%) vs. symptomatic at follow-up 21/43 (49%), p < 0.001]. Functional improvement was noted for LV-SV [83.3 (72.7–95.0) vs. 84.0 (77.0–100.3) ml; p = 0.045], global radial [25.3% (23.4%–27.9%) vs. 27.4% (24.4%–33.1%); p < 0.001], and circumferential strains [−16.5% (−17.5% to −15.6%) vs. −17.2% (−19.5% to −16.1%); p < 0.001]. In total, 17 patients had an LV-SV change >10% on follow-up scans (5 with a decrease and 12 with an increase), with LV-SV, RV-SV, and global longitudinal strain being discriminatory variables on baseline scans (p = 0.01, 0.02, and 0.04, respectively). T1- or T2-analysis revealed no changes, remaining within normal limits.
Conclusion: Symptomatic load as well as blood pressures decreased on follow-up. CMR did not detect significant changes in tissue parameters; however, volumetric, specifically LV-SV, and deformation indexes improved during mid-term follow-up.
After the Coronavirus disease 2019 (COVID-19) pandemic impacted healthcare systems worldwide, the next challenge for physicians emerged in the form of the Post-COVID-19 syndrome (PCS) (1, 2). This term was introduced to categorize and characterize patients with prolonged complaints, defined by symptoms lasting for more than 12 weeks after an acute COVID-19 infection (1). The symptoms include fatigue, dyspnea, exercise intolerance, palpitations, and chest pain among other neurologic manifestations such as concentration difficulties and memory impairments (3). Outpatient cardiologists are often tasked to rule out an underlying cardiac pathology as the sequalae of an acute COVID-19 infection might include myocarditis, arrhythmias, myocardial infarctions, and heart failure (4). Basic work-up includes an electrocardiogram, laboratory assessments in the form of troponin and N-terminal pro b-type natriuretic peptide (NT-pro-BNP) levels as well as transthoracic echocardiography (2, 5). When abnormalities on this basic work-up testing are found, the next step is often a referral for cardiovascular magnetic resonance (CMR) as a recent study reported an increased frequency of myocardial structural impairments in this patient subgroup (6). Even in the absence of abnormalities, on basic work-up patients are often referred for further CMR diagnostics owing to ongoing symptoms (7). Current expert consensus recommends CMR to be carried out after a COVID-19 infection with ongoing symptoms as this modality can accurately assess biventricular function and further provide tissue quantification to detect focal changes, such as scars or fibrosis on late gadolinium enhancement (LGE), diffuse fibrosis on T1 mapping, and detection of active myocardial inflammation in the form of T2-weighted imaging or quantitatively by T2 mapping (7–9). Rates of abnormal findings on CMR vary depending on the severity of the initial infection as well as cardiovascular comorbidities and risks (10–12). In outpatient groups, myocardial alterations are either absent or subtle (13, 14). Reports vary in the prevalence of non-ischemic and ischemic injuries as well as the extent of ongoing myocardial inflammation (15). It is important to note that left ventricular (LV) function is often preserved and only indexes of myocardial deformation are impaired (14). There is a lack of evidence regarding patients with symptoms and mild myocardial involvement on baseline CMR. In addition, the exact relevance of these myocardial alterations on baseline is not fully understood and therefore remains to pose a diagnostic and therapeutic challenge. Recently an emphasis has been put on biventricular functional and volumetric parameters, which might potentially be impaired in patients with PCS (16). Subtle and mild alterations may often be missed and detailed follow-ups (FU) are needed. This study aimed to describe the trajectories of myocardial alterations in patients with PCS and slight alterations on baseline CMR by mid-term FU, focusing on functional, volumetric, and deformation indexes.
For this exploratory study all patients referred for an outpatient FU CMR exam between August 2020 and May 2023 with ongoing symptoms after COVID-19 infection and mild alterations on baseline CMR scans were included and retrospectively analyzed. Alterations on baseline scans included reduced right ventricular (RV) (RV ejection fraction <42%) and/or LV function (LV ejection fraction <55%) and/or native T1 times >1.037 ms and/or T2 times >54 ms and/or focal fibrosis on LGE imaging. All cutoffs were defined based on established in-house post-processing and analysis with limits derived from healthy cohorts (17–19). The time of the acute event was defined by the first positive polymerase chain reaction test. Patients were excluded from final analysis if severe systemic illnesses, severe acute COVID-19 infection (including hospitalization for the acute infection), or previous chemotherapy were known. Finally, if arrhythmias during the scan impaired image acquisition or the examination was incomplete, patients were excluded. To compile basic characteristics, including medical history, drugs taken as well as previous imaging and laboratory assessment, documents from the outpatient providers as well as the local hospital records were considered.
This study complies with the Declaration of Helsinki and was approved by the institutional ethics committee. The requirement for written informed consent was waived due to the retrospective study design (EA1/042/22). The baseline results were presented in a previous publication (14).
All CMR exams were acquired on a 1.5 T Scanner (AvantoFit, Siemens, Erlangen, Germany) with ECG-gating and a 32-channel phased-array surface coil. For biventricular function assessment, balanced steady-state free precession cine images were acquired in four long-axis views including a four-, two-, three-chamber view as well as an RV view and one short-axis (SAX) stack, covering the entire ventricle without a gap. Parametric T2 and T1 mapping were acquired in multiple SAX slices covering the entire ventricle. T2-mapping acquisition was based on a motion-corrected balanced steady-state free precession sequence. Native T1 mapping was based on a motion-corrected modified Look-Locker inversion recovery technique using a 5-3-3 scheme. Synthetic extracellular volume was calculated from T1 mapping pre- and post-contrast media application based on a prototype sequence in basal and mid-ventricular slices. LGE imaging was acquired by a phase-sensitive inversion recovery sequence, 10–15 min after the application of 0.2 mmol/kg of contrast media (Gadoteridol, Prohance, Bracco Imaging, Konstanz, Germany). LGE images were acquired in the same axis as the cine images. FU scans were carried out using the same protocol with the exception of a subgroup of patients not receiving contrast media (N = 27). The details about the sequence parameters are given in the Supplemental Material E1.
Two readers [one with 7 years of experience in CMR (YB) and one resident in-training with 3 years of experience (JG)] performed image analysis using CVI42 (version 5.13.0, Circle Cardiovascular Imaging, Calgary, Canada). LV and RV function assessments were executed on cine SAX images following current recommendations regarding the papillary muscle mass attributed to the total LV mass (20). Right atrial function was assessed on the cine four chamber view. Left atrial function was assessed biplanar using the two- and four- chamber views. Strain analysis was carried out with retrospective feature tracking on cine images as reported recently (21). Global values for longitudinal, radial, and circumferential strain are reported. Quantitative mapping analysis was carried out with endo- and epicardial border delineations in each slice to obtain global values. Slice locations were allocated in the respective segment and level by delineating the extent of the LV. Slices with visible LV-outflow tracts were excluded. Similarly, apical slices with no blood pool or thin myocardial walls were excluded. A qualitative survey ensured the exclusion of segments with artifacts as well as focal fibrosis detected by LGE. Focal scars were assessed visually by LGE analysis by both readers independently concerning presence and location of scars. In case of uncertainties, a consensus read was performed.
Continuous variables are expressed as median and interquartile range (IQR) or mean and standard deviation (SD) as appropriate. Categorical variables are given as absolute frequencies and percentages. Normal distribution was assessed by the Shapiro–Wilk test. Baseline and FU scans were compared using either the t-test or the Wilcoxon rang test for continuous variables and the χ2 or Fisher's exact test for categorical variables. A post-hoc group analysis based on the percent change of LV stroke volume (LV-SV) (increase >10% vs. decrease >10% vs. change <10%) was carried out with either ANOVA or the Kruskal–Wallis test as global tests and followed-up in the cases with significant findings with pairwise testing. As the analysis was regarded as exploratory in nature, no corrections for multiple tests were carried out. A p-value <0.05 was defined as being statistically significant whereas a p-value <0.10 was considered as a trend. Statistical calculations were performed using the software SPSS Statistics (Version 27.0.0, IBM, Armonk, NY, USA).
In total, 43 patients [median age (IQR) 46 (37–56) years, 33 women, height 167 cm (163–180 cm), weight 70.0 kg (63.0–82.5 kg), body mass index 25.0 (22.1–27.0)] received FUs. Baseline CMRs were carried out at an average of 155 (70–239) days after acute infection with FUs having occurred at an average of 347 (167–651) days after initial diagnosis and 155 (56–440) days after baseline CMR. Alterations on baseline scans were as follows: 5 patients displayed reduced LV function, 1 displayed reduced RV function, 14 showed T1 times >cutoff, 4 showed T2 times >cutoff, and 20 had focal scars. On FU scans, patients displayed lower systolic [baseline 123 mmHg (118–130) vs. FU 110 mmHg (100–120); p < 0.001] and diastolic blood pressure [baseline 74 mmHg (67–83) vs. FU 68 mmHg (62–76); p = 0.01] with no significant difference in heart rate between scans [baseline 74 beats per minute (72–81) vs. FU 68 beats per minute (67–82); p = 0.57]. Overall comorbidity burden was low with arterial hypertension [9/43 (21%)] and hyperlipidemia [4/43 (9%)] being the most frequent conditions. The remaining comorbidities included diabetes mellitus [2/43 (5%)] and non-COVID-19-related lung diseases [3/43 (7%)]. No patient had a history of coronary artery disease, myocardial infarction, heart failure with reduced ejection fraction, valvular heart disease, or arrhythmias. At baseline, fatigue [27/43 (63%)] and dyspnea [31/43 (72%)] were reported as the leading symptoms. The overall symptom load declined between baseline and FU scans [total symptomatic patients at baseline 43/43 (100%) vs. FU 21/43 (49%), p < 0.001] as well as the symptoms themselves (p for all symptoms < 0.03). This, however, was not accompanied by a change in drug regimen. Detailed drug treatment and symptoms at baseline and FU are presented in Table 1.
Given the retrospective nature of the study, only a minority of patients reported laboratory results at baseline (N = 23) with even less at FU (N = 5). At baseline, one patient had a NT-pro-BNP above the laboratory cutoff (>125 ng/L) with the rest not revealing any abnormalities [NT-pro-BNP: 70 ng/L (39–108); Troponin T high sensitivity 3 ng/L (3–7)]. Baseline ECGs revealed a first-degree atrioventricular (AV) block in one patient. Additional ambulatory long-term ECG recordings (between 24 and 48 h) at baseline were available in 18 patients. One patient had a short non-sustained ventricular tachycardia. Other recordings did not show any abnormalities.
Statistically significant improvement of function parameters was noted for LV-SV [baseline 83.3 (72.7–95.0) ml vs. FU 84.0 (77.0–100.3) ml; p = 0.045] with positive trends for LV ejection fraction and end-diastolic volume. Of the five patients with reduced LV ejection fraction on baseline exams, two showed a normalized LV ejection fraction on FU, whereas three remained below the cutoff of 55%, despite an overall improvement in LV ejection fraction. Overall RV function and volume remained stable without trends across the examined time frame. However, one patient with a reduced RV ejection fraction on baseline continued to have an impairment on FU. Atrial function did not differ between scans, and LV strain analysis revealed improvements for global radial [25.3% (23.4%–27.9%) vs. 27.4% (24.4%–33.1%); p < 0.001] and circumferential strains [−16.5% (−17.5% to −15.6%) vs. −17.2% (−19.5% to −16.1%); p < 0.001] (Table 2 and Figure 1).
Figure 1. Boxplots comparing significant baseline and follow-up CMR parameters. Boxplots representing the median (solid inside the box), interquartile range (box), and 1.5 × interquartile range (whiskers) for left ventricular stroke volume, global radial strain, and circumferential strain (left to right) for baseline (blue) and follow-up (orange) scans. Every value below or above 1.5 × interquartile range is marked as an outlier.
Tissue characterization by CMR showed no changes between baseline and FU for T1 and T2 mapping. Although none of the four patients with initially elevated T2 times showed these elevations on FU, no statistic trends were detectable. On baseline scans, 20 patients had positive LGE (1 in an ischemic pattern and 19 in a non-ischemic pattern). In a subgroup with contrast media application (16 patients) on FU, LGE as well as extracellular volume assessment were carried out. Extracellular volume showed no change over the time period studied, nevertheless, focal fibrosis on LGE showed a trend toward regression [baseline 8/16 (50%) vs. FU 3/16 (19%); p = 0.06]. The patient with the non-sustained ventricular tachycardia had no LGE.
In total, 17 patients had a change >10% of LV-SV on FU scans (5 with a decrease, 12 with an increase). Comparing the baseline variables between the subgroups, LV-SV, RV-SV, and global longitudinal strain were significantly different (Figure 2). Interestingly, patients with an improvement in LV-SV >10% on FU CMR had the lowest global longitudinal strain on baseline scans [patients with a change <10% in LV-SV −18.8% (−19.9% to −17.7%) vs. decrease >10% in LV-SV −19.3% (−21.1% to −16.5%) vs. increase >10% in LV-SV −16.8% (−18.6% to −15.2%); p = 0.04] (Table 3).
Figure 2. Trajectories for individual patients based on left ventricular stroke volume changes. (A) represents trajectories for LV-SV for each individual patient between baseline and follow-up scan. (B) depicts baseline values for left ventricular stroke volume, (C) for right ventricular strain, and (D) for global longitudinal strain. The boxplots represent the median (solid inside the box), interquartile range (box), and 1.5 × interquartile range (whiskers). Every value below or above 1.5 × interquartile range is marked as an outlier. Black, mean change; gray, change <10%; red, decrease >10%; and green, increase >10%.
For the 20 patients with and the 23 without LGE findings on baseline, FU analysis revealed improvement in global circumferential and radial strains (all p < 0.002). In the LGE negative (−) group, this was accompanied by an improvement in LV ejection fraction [baseline 64.0% (58.2%–65.5%) vs. FU 64.8% (63.1%–68.1%); p = 0.04] as well as a slight lower myocardial mass [baseline 72.5 g (59.9–78.8 g) vs. FU 70.8 g (63.8–84.4 g); p = 0.02]. Both baseline and FU values were within normal ranges. Myocardial tissue analysis by T1 and T2 mapping did not disclose any changes between baseline and FU scans for LGE positive (+) and (−) cohorts (Table 4). Figure 3 shows individual examples of patients with and without LGE and their individual trajectories.
Figure 3. Examples of functional and tissue parameter changes in patients with and without LGE. Provided are examples for a patient with late gadolinium enhancement (LGE) (left columns) and one without (right columns). Images for ventricular function based on cine images, T1 and T2 mapping as well as post-processed cine images for global radial strain are presented (from top to bottom). These images are exemplary and do not represent changes in left ventricular stroke volume, the main finding of the study. Orange images point at subepicardial LGE. Red arrows point at a pericardial effusion.
In total, 22 patients were asymptomatic on FU compared with the 21 symptomatic patients on FU. The asymptomatic patients had lower left atrial function indexes (left atrial size in cm2 p = 0.049 and left atrial end-diastolic volume index divided by body surface area p = 0.01) and larger RV end-diastolic volume (p = 0.049) at baseline (Supplementary Table E1). On FU, only left atrial ejection fraction was shown to reveal lower values in asymptomatic patients (p = 0.01). Tissue characterization showed lower T2 values on FU between the two cohorts [T2 asymptomatic on FU 50.5 ms (49.2–51.6 ms) vs. symptomatic at FU 49.2 ms (48.4–50.8 ms); p = 0.04] (Supplementary Table E2). Comparing baseline and FU results for symptomatic patients at FU, LV-SV (p = 0.03) as well as global radial (p = 0.01) and circumferential strain (p = 0.02) showed improvement (Supplementary Table E2). Correspondingly, radial (p = 0.001) and circumferential strains (p = 0.002) improved in asymptomatic patients at FU as well (Supplementary Table E3).
The principal results of this mid-term FU of symptomatic PCS patients in an outpatient setting are as follows: First, the majority of functional and tissue parameters remained stable even after a year. Second, the symptoms declined during mid-term FU. Third, LV-SV as well as myocardial deformation indexes, especially radial and circumferential strains, might potentially reflect a reverse remodeling. And lastly, this change is accompanied by a decrease in arterial blood pressure.
Cardiac post-acute sequalae of COVID-19 are manifold and include subacute myocarditis and chronic inflammation, ischemic tissue injury as well as new-onset arrhythmias and strokes (4, 10, 15). The exact pathophysiology is not entirely understood; however, a possible combination of mechanisms could play a role, such as direct viral toxicity, autoimmune responses, and inflammatory cascades. Hospitalized patients with COVID-19 have a high prevalence of non-ischemic and ischemic injuries, especially patients with elevated troponins (15). Therefore, elevated troponins might be associated with adverse cardiovascular outcomes (22). In contrast to this, outpatient cases without abnormalities on basic testing are less researched and followed-up. Hanneman et al. recently presented echocardiographic- and CMR-based data in symptomatic patients at 3–6 and 12–18 months FU after mild COVID-19 infection (23). The authors did not find structural or functional differences between the COVID-19 cohort and healthy controls; however, elevated cardiac T1 times were associated with symptoms at FU (23). A prospective trial by Puntmann et al. including 346 patients with COVID-19 infection reported baseline and FU scan results at a median of 109 days (IQR, 77–177 days) and 329 days (IQR, 274–383 days), respectively (10). Myocardial tissue characterization revealed overall lower T1 and T2 times as well as lower RV ejection fractions at FU. This change was concomitant with lower systolic blood pressure. Remarkably, patients with ongoing symptoms at FU had higher T2 times than asymptomatic patients. In contrast to this study, we report lower T2 times in symptomatic patients; however, all median mapping values in the current study are within normal ranges. As the observed differences are falling well into an intraobserver range, it is unlikely that the lower T2 values reflect a pathologic process (24). In contrast to the discrepancies regarding the T2 findings in the aforementioned study, our findings are in line regarding the lower systolic blood pressure. Unfortunately, SV was not reported by Puntmann et al. (10).
Following the importance of hospitalized vs. non-hospitalized patient groups, one should consider the timing of the CMR scan after the acute infection (4, 14). In a study by Zhang et al., 39 outpatients were scanned 26 days after an acute infection (25). In addition, CMR scans before the infection were available for an intraindividual comparison. The authors report no change in function, volume, and tissue properties. Although this study reports the acute phase, it underlines the mild effect of COVID-19 on the myocardium. Kravchenko et al. report a comparison of PCS patients, scanned at a median of 103 days after the infection, and a healthy cohort, with no significant differences regarding function and tissue characterization (13). Although no FU was carried out in this study, the overall results regarding tissue characteristics are in line with our findings.
Given the limited data on CMR studies, one should also look into other imaging modalities. A current echocardiography study by Olszanecka et al. reported FU data for 229 patients with mild to severe COVID-19 infection at 3, 6, and 12 months (26). They found a significant improvement in LV-SV as well as a reduction in LV mass accompanied by a lower systolic blood pressure at FU. These findings are in line with ours, promoting the importance of hemodynamic variables such as blood pressure. In contrast to these results, Young et al. carried out pre- and post-COVID-19 infection transthoracic echocardiography studies in 259 patients, reporting no statistically significant changes in the entire cohort except an improvement in LV ejection fraction after the infection (27). It should be noted that blood pressures did not change throughout the study, with systolic blood pressures being 130 and 132 mmHg at baseline and FU, respectively. This underlines the importance of hemodynamic factors.
Infections with COVID-19 might either worsen pre-existing arterial hypertension or even induce new-onset arterial hypertension based on interactions with the angiotensinogen II receptor (28, 29). It seems that blood pressure changes are related to autonomic dysfunction in these patients (30–32). The association between arterial blood pressure dysregulation has not only been proven for COVID-19 but also other viral infections (33, 34). The increased afterload might obstruct proper diastolic functioning, reducing LV-SV. An impaired ability to increase LV-SV during exercise has been established as a pathophysiologic link to dyspnea especially in heart failure with preserved ejection fraction (35, 36). The overlap extends to the observation that acute COVID-19 shows an association with this entity (37). Conversely, low LV-SV might even be able to better predict onset of heart failure than pure assessment of ejection fraction and therefore holds promising value especially in longitudinal FU (38, 39). Changes however might be small and within low-normal ranges, needing precise imaging tools, such as CMR, to be picked up (16). Whether this association transmits to PCS has yet to be established. It seems that risk factors, such as female gender, obesity, and arterial hypertension, predispose to PCS as well as heart failure with preserved ejection fraction (40, 41). Whether the underlying pathophysiologic mechanism, such as microvascular inflammation or autoimmune imbalances (42), autonomic dysfunction, commonly in the form of the postural orthostatic tachycardia syndrome in patients with PCS, is associated with impaired regulation of LV-SV, promoting the importance of this volumetric parameter (43).
The presented study results should be viewed under potential confounders that might as well impact the myocardium after an acute COVID-19 infection. These include potential alterations in lung function, especially in regard to pulmonary embolism, as well as after COVID-19 vaccine (14, 44). Although the effects of vaccines on the myocardium are often described as mild, some studies report tissue alterations in the form of scars or elevated T1 times (45). Lastly, the symptomatic overlap to a chronic fatigue syndrome (CFS) should be mentioned, which could be present in our patient cohort as well, given the common overlap between PCS and CFS (3, 46). These limitations warrant future prospective multi-center studies investigating the pathophysiologic links. Indices of mechanical ventricular function, for example circumferential, radial, and longitudinal strains, should be incorporated into such efforts as they might be valuable indicators for a recovery of the myocardium as shown across all subgroup comparisons in this study. In the LGE negative (−) subgroup, this was accompanied by a median reduction in LV mass, potentially influencing the deformation. Similar findings with a shorter FU were published recently based on CMR data (47). The authors reported lower strain rates at baseline; this finding is backed-up by recent evidence from echocardiography, suggesting that these changes already take place in patients shortly after an acute COVID-19 infection (48). Overall and in accordance with our findings, strain indexes seem to improve on FU (49). The same holds true for COVID-19 vaccination-associated changes (50). Certainly, the end-goal should be to couple volumetric and deformation indexes to treatment strategies in randomized controlled trials, such as the current ongoing MYOFLAME-19 study (NCT05619653) (51).
As the detected changes are rather small, direct implications for clinical practice are hard to infer from this analysis. However, a major point to be made is the association of dysregulated blood pressure and PCS (30). Evidence is accumulating that COVID-19 can trigger dysregulation of blood pressure responses, especially shortly after an acute infection (31). Focus on patient management should be on identification of co-existing diseases, such as arterial hypertension, diabetes mellitus, hyperlipidemia as well as heart failure with preserved ejection fraction. FU should be carried out in symptomatic patients, optimally with the same imaging technique and at the same site to precisely compare and track changes over time (16).
The major limitation concerning this study is the retrospective nature of the analysis. As all patients were referred from outpatient providers, not all medical records were available, particularly laboratory and ancillary tests. The referral by outpatient providers might potentially introduce a referral bias as well as lack of data for patient characterization. Furthermore, not all patients underwent an FU scan with contrast media application, which was decided by the physician performing the exam. Lastly, the change in blood pressure over the time course of the study is a confounder for the LV-SV and strain results and our analysis has to be viewed under this circumstance.
Mid-term FU in patients with PCS reveals a reduction in symptomatic load accompanied by improved LV-SV as well as global LV deformation indexes. Blood pressure dysregulation, especially after the acute infection, is an important hemodynamic factor, which needs to be taken into consideration while assessing patients with PCS. Further multi-center research is needed on medical patient management as well as the interaction between autonomic dysfunction and PCS.
The datasets analyzed during the current study are not publicly available due to German laws but are available from the corresponding author on reasonable request.
The studies involving humans were approved by Ethikkommission der Charité—Universitätsmedizin Berlin. The studies were conducted in accordance with the local legislation and institutional requirements. The ethics committee/institutional review board waived the requirement of written informed consent for participation from the participants or the participants’ legal guardians/next of kin because of the retrospective study design (EA1/042/22).
JG: Conceptualization, Data curation, Formal Analysis, Funding acquisition, Investigation, Methodology, Project administration, Resources, Software, Supervision, Validation, Visualization, Writing – original draft, Writing – review & editing. LG: Data curation, Investigation, Software, Visualization, Writing – review & editing. PvD: Data curation, Formal Analysis, Investigation, Software, Validation, Writing – review & editing. YB: Data curation, Investigation, Methodology, Software, Visualization, Writing – review & editing. EB: Data curation, Formal Analysis, Methodology, Supervision, Writing – review & editing. JS-M: Conceptualization, Data curation, Formal Analysis, Funding acquisition, Investigation, Methodology, Project administration, Resources, Software, Supervision, Validation, Visualization, Writing – original draft, Writing – review & editing.
The authors declare that financial support was received for the research, authorship, and/or publication of this article.
JG has received funding from the European Union’s Horizon 2020 research and innovation program (Grant No. 18HLT05 QUIERO, 2020). JS-M holds institutional grants from the Charité Medical University. The group holds research grants from Siemens Healthineers.
We gratefully thank our CMR technicians Kerstin Kretschel, Denise Kleindienst, and Martina Kohla for technical assistance as well as our study nurses Annette Köhler and Elke Nickel-Szczech. The whole Working Group CMR was involved, and we are thankful for the input on different levels. The prototype sequence was provided by Kelvin Chow (Siemens Healthineers). We thank Carsten Schwenke for his support in matters of study statistics. We also thank Natalie Kaban for proofreading the final manuscript.
The authors declare that the research was conducted in the absence of any commercial or financial relationships that could be construed as a potential conflict of interest.
All claims expressed in this article are solely those of the authors and do not necessarily represent those of their affiliated organizations, or those of the publisher, the editors and the reviewers. Any product that may be evaluated in this article, or claim that may be made by its manufacturer, is not guaranteed or endorsed by the publisher.
The Supplementary Material for this article can be found online at: https://www.frontiersin.org/articles/10.3389/fcvm.2024.1357349/full#supplementary-material
1. Nalbandian A, Sehgal K, Gupta A, Madhavan MV, McGroder C, Stevens JS, et al. Post-acute COVID-19 syndrome. Nat Med. (2021) 27:601–15. doi: 10.1038/s41591-021-01283-z
2. Raman B, Bluemke DA, Lüscher TF, Neubauer S. Long COVID: post-acute sequelae of COVID-19 with a cardiovascular focus. Eur Heart J. (2022) 43:1157–72. doi: 10.1093/eurheartj/ehac031
3. Legler F, Meyer-Arndt L, Mödl L, Kedor C, Freitag H, Stein E, et al. Long-term symptom severity and clinical biomarkers in post-COVID-19/chronic fatigue syndrome: results from a prospective observational cohort. EClinicalMedicine. (2023) 63:102146. doi: 10.1016/j.eclinm.2023.102146
4. Xie Y, Xu E, Bowe B, Al-Aly Z. Long-term cardiovascular outcomes of COVID-19. Nat Med. (2022) 28:583–90. doi: 10.1038/s41591-022-01689-3
5. Task Force for the Management of COVID-19 of the European Society of Cardiology. European Society of Cardiology guidance for the diagnosis and management of cardiovascular disease during the COVID-19 pandemic: part 1—epidemiology, pathophysiology, and diagnosis. Eur Heart J. (2022) 43:1033–58. doi: 10.1093/eurheartj/ehab696
6. Vidula MK, Rajewska-Tabor J, Cao JJ, Kang Y, Craft J, Mei W, et al. Myocardial injury on CMR in patients with COVID-19 and suspected cardiac involvement. JACC Cardiovasc Imaging. (2023) 16:609–24. doi: 10.1016/j.jcmg.2022.10.021
7. Ferreira VM, Plein S, Wong TC, Tao Q, Raisi-Estabragh Z, Jain SS, et al. Cardiovascular magnetic resonance for evaluation of cardiac involvement in COVID-19: recommendations by the society for cardiovascular magnetic resonance. J Cardiovasc Magn Reson. (2023) 25:21. doi: 10.1186/s12968-023-00933-0
8. Petersen SE, Friedrich MG, Leiner T, Elias MD, Ferreira VM, Fenski M, et al. Cardiovascular magnetic resonance for patients with COVID-19. JACC Cardiovasc Imaging. (2022) 15(4):685–99. doi: 10.1016/j.jcmg.2021.08.021
9. Ferreira VM, Schulz-Menger J, Holmvang G, Kramer CM, Carbone I, Sechtem U, et al. Cardiovascular magnetic resonance in nonischemic myocardial inflammation: expert recommendations. J Am Coll Cardiol. (2018) 72:3158–76. doi: 10.1016/j.jacc.2018.09.072
10. Puntmann VO, Martin S, Shchendrygina A, Hoffmann J, Ka MM, Giokoglu E, et al. Long-term cardiac pathology in individuals with mild initial COVID-19 illness. Nat Med. (2022) 28(10):2117–23. doi: 10.1038/s41591-022-02000-0
11. Ammirati E, Lupi L, Palazzini M, Hendren NS, Grodin JL, Cannistraci CV, et al. Prevalence, characteristics, and outcomes of COVID-19-associated acute myocarditis. Circulation. (2022) 145:1123–39. doi: 10.1161/CIRCULATIONAHA.121.056817
12. Kotecha T, Knight DS, Razvi Y, Kumar K, Vimalesvaran K, Thornton G, et al. Patterns of myocardial injury in recovered troponin-positive COVID-19 patients assessed by cardiovascular magnetic resonance. Eur Heart J. (2021) 42:1866–78. doi: 10.1093/eurheartj/ehab075
13. Kravchenko D, Isaak A, Zimmer S, Mesropyan N, Reinert M, Faron A, et al. Cardiac MRI in patients with prolonged cardiorespiratory symptoms after mild to moderate COVID-19. Radiology. (2021) 301:E419–25. doi: 10.1148/radiol.2021211162
14. Gröschel J, Bhoyroo Y, Blaszczyk E, Trauzeddel RF, Viezzer D, Saad H, et al. Different impacts on the heart after COVID-19 infection and vaccination: insights from cardiovascular magnetic resonance. Front Cardiovasc Med. (2022) 9:916922. doi: 10.3389/fcvm.2022.916922
15. Artico J, Shiwani H, Moon JC, Gorecka M, McCann GP, Roditi G, et al. Myocardial involvement after hospitalization for COVID-19 complicated by troponin elevation: a prospective, multicenter, observational study. Circulation. (2023) 147:364–74. doi: 10.1161/CIRCULATIONAHA.122.060632
16. Puntmann VO, Shchendrygina A, Bolanos CR, Madjiguène Ka M, Valbuena S, Rolf A, et al. Cardiac involvement due to COVID-19: insights from imaging and histopathology. Eur Cardiol. (2023) 18:e58. doi: 10.15420/ecr.2023.02
17. Gröschel J, Trauzeddel R-F, Müller M, von Knobelsdorff-Brenkenhoff F, Viezzer D, Hadler T, et al. Multi-site comparison of parametric T1 and T2 mapping: healthy travelling volunteers in the Berlin research network for cardiovascular magnetic resonance (BER-CMR). J Cardiovasc Magn Reson. (2023) 25:47. doi: 10.1186/s12968-023-00954-9
18. Schmacht L, Traber J, Grieben U, Utz W, Dieringer MA, Kellman P, et al. Cardiac involvement in myotonic dystrophy type 2 patients with preserved ejection fraction: detection by cardiovascular magnetic resonance. Circ Cardiovasc Imaging. (2016) 9:e004615. doi: 10.1161/CIRCIMAGING.115.004615
19. von Knobelsdorff-Brenkenhoff F, Schüler J, Dogangüzel S, Dieringer MA, Rudolph A, Greiser A, et al. Detection and monitoring of acute myocarditis applying quantitative cardiovascular magnetic resonance. Circ Cardiovasc Imaging. (2017) 10(2):e005242. doi: 10.1161/CIRCIMAGING.116.005242
20. Schulz-Menger J, Bluemke DA, Bremerich J, Flamm SD, Fogel MA, Friedrich MG, et al. Standardized image interpretation and post-processing in cardiovascular magnetic resonance—2020 update. J Cardiovasc Magn Reson. (2020) 22:19. doi: 10.1186/s12968-020-00610-6
21. Gröschel J, Kuhnt J, Viezzer D, Hadler T, Hormes S, Barckow P, et al. Comparison of manual and artificial intelligence based quantification of myocardial strain by feature tracking-a cardiovascular MR study in health and disease. Eur Radiol. (2024) 34(2):1003–15. doi: 10.1007/s00330-023-10127-y
22. Lala A, Johnson KW, Januzzi JL, Russak AJ, Paranjpe I, Richter F, et al. Prevalence and impact of myocardial injury in patients hospitalized with COVID-19 infection. J Am Coll Cardiol. (2020) 76:533–46. doi: 10.1016/j.jacc.2020.06.007
23. Hanneman K, Houbois C, Kei T, Gustafson D, Thampinathan B, Sooriyakanthan M, et al. Multimodality cardiac imaging, cardiac symptoms, and clinical outcomes in patients who recovered from mild COVID-19. Radiology. (2023) 308:e230767. doi: 10.1148/radiol.230767
24. Zange L, Muehlberg F, Blaszczyk E, Schwenke S, Traber J, Funk S, et al. Quantification in cardiovascular magnetic resonance: agreement of software from three different vendors on assessment of left ventricular function, 2D flow and parametric mapping. J Cardiovasc Magn Reson. (2019) 21:12. doi: 10.1186/s12968-019-0522-y
25. Zhang J, Luo S, Cai J, Kong X, Zhang L, Qi L, et al. Multiparametric cardiovascular magnetic resonance in nonhospitalized COVID-19 infection subjects: an intraindividual comparison study. J Thorac Imaging. (2024) 39(2):86–92. doi: 10.1097/RTI.0000000000000774
26. Olszanecka A, Wojciechowska W, Bednarek A, Kusak P, Wizner B, Terlecki M, et al. Serial echocardiographic evaluation of COVID-19 patients without prior history of structural heart disease: a 1-year follow-up CRACoV-HHS study. Front Cardiovasc Med. (2023) 10:1230669. doi: 10.3389/fcvm.2023.1230669
27. Young KA, Krishna H, Jain V, Hamza I, Scott CG, Pellikka PA, et al. Serial left and right ventricular strain analysis in patients recovered from COVID-19. J Am Soc Echocardiogr. (2022) 35:1055–63. doi: 10.1016/j.echo.2022.06.007
28. Wrona M, Skrypnik D. New-onset diabetes mellitus, hypertension, dyslipidaemia as sequelae of COVID-19 infection—systematic review. Int J Environ Res Public Health. (2022) 19:13280. doi: 10.3390/ijerph192013280
29. Gallo G, Calvez V, Savoia C. Hypertension and COVID-19: current evidence and perspectives. High Blood Press Cardiovasc Prev. (2022) 29:115–23. doi: 10.1007/s40292-022-00506-9
30. Fedorowski A, Fanciulli A, Raj SR, Sheldon R, Shibao CA, Sutton R. Cardiovascular autonomic dysfunction in post-COVID-19 syndrome: a major health-care burden. Nat Rev Cardiol. (2024). doi: 10.1038/s41569-023-00962-3. [Epub ahead of print].
31. Nandadeva D, Skow RJ, Grotle A-K, Stephens BY, Young BE, Fadel PJ. Impact of COVID-19 on ambulatory blood pressure in young adults: a cross-sectional analysis investigating time since diagnosis. J Appl Physiol (1985). (2022) 133:183–90. doi: 10.1152/japplphysiol.00216.2022
32. da Silva FS, Bonifácio LP, Bellissimo-Rodrigues F, Joaquim LF, Martins Dias DP, Dias Romano MM, et al. Investigating autonomic nervous system dysfunction among patients with post-COVID condition and prolonged cardiovascular symptoms. Front Med. (2023) 10:1216452. doi: 10.3389/fmed.2023.1216452
33. Schillaci G, Maggi P, Madeddu G, Pucci G, Mazzotta E, Penco G, et al. Symmetric ambulatory arterial stiffness index and 24-h pulse pressure in HIV infection: results of a nationwide cross-sectional study. J Hypertens. (2013) 31:560–7; discussion 567. doi: 10.1097/HJH.0b013e32835ca949
34. Cheng J, Ke Q, Jin Z, Wang H, Kocher O, Morgan JP, et al. Cytomegalovirus infection causes an increase of arterial blood pressure. PLoS Pathog. (2009) 5:e1000427. doi: 10.1371/journal.ppat.1000427
35. Wolsk E, Kaye DM, Komtebedde J, Shah SJ, Borlaug BA, Burkhoff D, et al. Determinants and consequences of heart rate and stroke volume response to exercise in patients with heart failure and preserved ejection fraction. Eur J Heart Fail. (2021) 23:754–64. doi: 10.1002/ejhf.2146
36. Rosch S, Kresoja K-P, Besler C, Fengler K, Schöber AR, von Roeder M, et al. Characteristics of heart failure with preserved ejection fraction across the range of left ventricular ejection fraction. Circulation. (2022) 146:506–18. doi: 10.1161/CIRCULATIONAHA.122.059280
37. Hadzibegovic S, Lena A, Churchill TW, Ho JE, Potthoff S, Denecke C, et al. Heart failure with preserved ejection fraction according to the HFA-PEFF score in COVID-19 patients: clinical correlates and echocardiographic findings. Eur J Heart Fail. (2021) 23:1891–902. doi: 10.1002/ejhf.2210
38. De Marco M, Gerdts E, Mancusi C, Roman MJ, Lønnebakken MT, Lee ET, et al. Influence of left ventricular stroke volume on incident heart failure in a population with preserved ejection fraction (from the strong heart study). Am J Cardiol. (2017) 119:1047–52. doi: 10.1016/j.amjcard.2016.12.011
39. Kerstens TP, Weerts J, van Dijk APJ, Weijers G, Knackstedt C, Eijsvogels TMH, et al. Association of left ventricular strain-volume loop characteristics with adverse events in patients with heart failure with preserved ejection fraction. Eur Heart J Cardiovasc Imaging. (2023) 24:1168–76. doi: 10.1093/ehjci/jead117
40. Subramanian A, Nirantharakumar K, Hughes S, Myles P, Williams T, Gokhale KM, et al. Symptoms and risk factors for long COVID in non-hospitalized adults. Nat Med. (2022) 28:1706–14. doi: 10.1038/s41591-022-01909-w
41. Heidenreich PA, Bozkurt B, Aguilar D, Allen LA, Byun JJ, Colvin MM, et al. 2022 AHA/ACC/HFSA guideline for the management of heart failure: a report of the American College of Cardiology/American Heart Association Joint Committee on Clinical Practice Guidelines. Circulation. (2022) 145:e895–1032. doi: 10.1161/CIR.0000000000001063
42. Gevaert AB, Kataria R, Zannad F, Sauer AJ, Damman K, Sharma K, et al. Heart failure with preserved ejection fraction: recent concepts in diagnosis, mechanisms and management. Heart Br Card Soc. (2022) 108:1342–50. doi: 10.1136/heartjnl-2021-319605
43. Masuki S, Eisenach JH, Schrage WG, Johnson CP, Dietz NM, Wilkins BW, et al. Reduced stroke volume during exercise in postural tachycardia syndrome. J Appl Physiol (1985). (2007) 103:1128–35. doi: 10.1152/japplphysiol.00175.2007
44. Suh YJ, Hong H, Ohana M, Bompard F, Revel M-P, Valle C, et al. Pulmonary embolism and deep vein thrombosis in COVID-19: a systematic review and meta-analysis. Radiology. (2021) 298:E70–80. doi: 10.1148/radiol.2020203557
45. Shiyovich A, Witberg G, Aviv Y, Eisen A, Orvin K, Wiessman M, et al. Myocarditis following COVID-19 vaccination: magnetic resonance imaging study. Eur Heart J Cardiovasc Imaging. (2022) 23(8):1075–82. doi: 10.1093/ehjci/jeab230
46. Haffke M, Freitag H, Rudolf G, Seifert M, Doehner W, Scherbakov N, et al. Endothelial dysfunction and altered endothelial biomarkers in patients with post-COVID-19 syndrome and chronic fatigue syndrome (ME/CFS). J Transl Med. (2022) 20:138. doi: 10.1186/s12967-022-03346-2
47. Mojica-Pisciotti ML, Panovský R, Holeček T, Opatřil L, Feitová V. Lower ventricular and atrial strain in patients who recovered from COVID-19 assessed by cardiovascular magnetic resonance feature tracking. Front Cardiovasc Med. (2023) 10:1293105. doi: 10.3389/fcvm.2023.1293105
48. Honchar O, Ashcheulova T. Short-term echocardiographic follow-up after hospitalization for COVID-19: a focus on early post-acute changes. Front Cardiovasc Med. (2023) 10:1250656. doi: 10.3389/fcvm.2023.1250656
49. Schellenberg J, Matits L, Bizjak DA, Kersten J, Kirsten J, Vollrath S, et al. Assessment of myocardial function and cardiac performance using left ventricular global longitudinal strain in athletes after COVID-19: a follow-up study. Front Cardiovasc Med. (2023) 10:1240278. doi: 10.3389/fcvm.2023.1240278
50. Kravchenko D, Isaak A, Mesropyan N, Bischoff LM, Pieper CC, Attenberger U, et al. Cardiac magnetic resonance follow-up of COVID-19 vaccine associated acute myocarditis. Front Cardiovasc Med. (2022) 9:1049256. doi: 10.3389/fcvm.2022.1049256
51. Puentmann V. Randomised placebo controlled clinical trial of efficacy of MYOcardial protection in patients with postacute inFLAMmatory cardiac involvEment Due to COVID-19. [Clinical trial registration]. clinicaltrials.gov (2023). Available online at: https://clinicaltrials.gov/study/NCT05619653 (accessed January 1, 2024).
Keywords: cardiovascular magnetic resonance, COVID-19, post-COVID-19 syndrome, mapping, late gadolinium enhancement, stroke volume
Citation: Gröschel J, Grassow L, van Dijck P, Bhoyroo Y, Blaszczyk E and Schulz-Menger J (2024) Trajectories of functional and structural myocardial parameters in post-COVID-19 syndrome—insights from mid-term follow-up by cardiovascular magnetic resonance. Front. Cardiovasc. Med. 11:1357349. doi: 10.3389/fcvm.2024.1357349
Received: 17 December 2023; Accepted: 18 March 2024;
Published: 2 April 2024.
Edited by:
Grigorios Korosoglou, GRN Klinik Weinheim, GermanyReviewed by:
Anastasios Nikolaos Panagopoulos, University of Nebraska Medical Center, United States© 2024 Gröschel, Grassow, van Dijck, Bhoyroo, Blaszczyk and Schulz-Menger. This is an open-access article distributed under the terms of the Creative Commons Attribution License (CC BY). The use, distribution or reproduction in other forums is permitted, provided the original author(s) and the copyright owner(s) are credited and that the original publication in this journal is cited, in accordance with accepted academic practice. No use, distribution or reproduction is permitted which does not comply with these terms.
*Correspondence: Jeanette Schulz-Menger amVhbmV0dGUuc2NodWx6LW1lbmdlckBjaGFyaXRlLmRl
Abbreviations PCS, post-COVID-19 syndrome; CMR, cardiovascular magnetic resonance; LGE, late gadolinium enhancement; LV, left ventricle; FU, follow-up; RV, right ventricle; SAX, short axis; IQR, interquartile range; SD, standard deviation; LV-SV, left ventricular stroke volume.
Disclaimer: All claims expressed in this article are solely those of the authors and do not necessarily represent those of their affiliated organizations, or those of the publisher, the editors and the reviewers. Any product that may be evaluated in this article or claim that may be made by its manufacturer is not guaranteed or endorsed by the publisher.
Research integrity at Frontiers
Learn more about the work of our research integrity team to safeguard the quality of each article we publish.