- 1Clinic of Vascular Surgery, Mures County Emergency Hospital, Targu Mures, Romania
- 2Department of Vascular Surgery, George Emil Palade University of Medicine, Pharmacy, Sciences and Technology of Targu Mures, Targu Mures, Romania
- 3Doctoral School of Medicine and Pharmacy, George Emil Palade University of Medicine, Pharmacy, Sciences and Technology of Targu Mures, Targu Mures, Romania
- 4Centre for Advanced Medical and Pharmaceutical Research (CCAMF), George Emil Palade University of Medicine, Pharmacy, Sciences and Technology of Targu Mures, Targu Mures, Romania
- 5Queensland Eye Institute, Woolloongabba, QLD, Australia
- 6Faculty of Medicine, George Emil Palade University of Medicine, Pharmacy, Sciences and Technology of Targu Mures, Targu Mures, Romania
- 7School of Chemistry and Physics, Queensland University of Technology, Brisbane, QLD, Australia
- 8Australian Institute of Bioengineering and Nanotechnology (AIBN), University of Queensland, St Lucia, QLD, Australia
We have reviewed the development and current status of therapies based on exposure to non-ionizing radiation (with a photon energy less than 10 eV) aimed at suppressing the venous neointimal hyperplasia, and consequentially at avoiding stenosis in arteriovenous grafts. Due to the drawbacks associated with the medical use of ionizing radiation, prominently the radiation-induced cardiovascular disease, the availability of procedures using non-ionizing radiation is becoming a noteworthy objective for the current research. Further, the focus of the review was the use of such procedures for improving the vascular access function and assuring the clinical success of arteriovenous fistulae in hemodialysis patients. Following a brief discussion of the physical principles underlying radiotherapy, the current methods based on non-ionizing radiation, either in use or under development, were described in detail. There are currently five such techniques, including photodynamic therapy (PDT), far-infrared therapy, photochemical tissue passivation (PTP), Alucent vascular scaffolding, and adventitial photocrosslinking. The last three are contingent on the mechanical stiffening achievable by the exogenous photochemical crosslinking of tissular collagen, a process that leads to the decrease of venous compliance. As there are conflicting opinions on the role of compliance mismatch between arterial and venous conduits in a graft, this aspect was also considered in our review.
Introduction
Carrel and Guthrie were the first to report the critical thickening of venous intima at anastomotic sites following the transplantation of veins into arteries (1). This process, now commonly referred to as neointimal hyperplasia (NIH), is recognized as a contributing factor to the stenosis events leading to complications and failures in interventions that involve venous conduits, such as coronary artery bypass grafting, coronary angioplasty, lower limb vein bypass, peripheral artery angioplasty, carotid endarterectomy, and arteriovenous fistula (AVF) or artificial graft for hemodialysis. The complex etiology and pathophysiology of NIH have been progressively unraveled through years of many physician-scientists' research, and excellently presented in several successive landmark reviews (2–14). In essence, the triggering event in the development of NIH is an injury to the vascular endothelium generated by turbulent vasculature hemodynamics. Following a series of other contributing events occurring over a period of post-anastomotic “arterialization”, the uncontrolled proliferation and migration of medial smooth muscle cells (SMCs) in tunica intima prevails and brings the hyperplasia process to a close, resulting in stenosis and loss of luminal patency. An abnormal arterialization is not compatible with a functional AVF, and the failure will occur rather sooner than later.
AVFs are crucial for maintaining the life and health of those afflicted by end-stage kidney disease and needing hemodialysis for survival. Failure of dialysis, leading to morbidity and mortality in such patients, is mainly caused by the vascular access dysfunction, which is a result of venous stenosis due to a complexity of pathophysiological events where NIH can play an aggravating role (15–18). The mechanism of the processes involved and their after effects on the vascular access, as well as the attempts to suppress them, are well documented (3, 4, 19–30).
The AVF, which was first introduced around 60 years ago (31, 32), has become the preferred form of vascular access for renal replacement therapies that involve dialysis (33, 34). Its functionality is traditionally assessed using the term “maturation”. According to some practical guidelines, a mature AVF must be able to deliver, ideally not later than 6 weeks after surgery, at least 300–600 ml/min blood for 3–5 h, and can be routinely cannulated with two needles. Although there is no consensus on an in-principle definition (19, 24), maturation can be reasonably qualified (32, 35, 36) as the ability of the inflow artery and the outflow vein to respond to the increased blood flow that occurs upon anastomosis of the two vessels.
Due to the low pressure in the venous system, immediately after performing AVF and exposing the venous wall to arterial pressure, the remodeling process is initiated through wall distension, endothelial injury, local ischemia, and cell apoptosis (37–39). Overtime, these will lead to generation of inflammatory process at the venous wall and NIH (38, 40). New methods, such as external stenting, have been developed and proposed to reduce NIH and local inflammation (41). However, none of these methods can be applied to AVF due to the requirement of periodic puncture of the venous component during dialysis sessions.
Amongst many therapeutic strategies against NIH-induced stenosis, the ionizing radiation has been employed due to its ability to kill cells, being administered for suppressing or reducing the proliferation of SMCs within the neointima. The method, which was pioneered (42) in hypercholesterolemic rabbit model, is classified as a sealed-source radiotherapeutic procedure, and it is known as endovascular (or intravascular) brachytherapy. Following animal experimentation, it has been applied episodically for treating stenosis associated with NIH in venous grafts including AVF, with variable outcomes (13, 24, 43–54). However, there is no clear evidence that the radiation therapies based suppressing the intimal SMCs had lasting benefits in preventing stenosis. Two forms of ionizing radiation were commonly used for the endovascular brachytherapy: particle radiation such as β radiation (beam of electrons), or electromagnetic radiation as γ-rays (photons). Radioisotopes of different half-lives were used as radiation sources, including 192Ir, 32P, 48V, 90Sr, or 90Y, and delivered in various formats such as seeds, pellets, tubes, wires, gels etc. In a different approach, the use of the ionizing region of ultraviolet (UV) radiation, UV-C, to inhibit SMC proliferation has been the subject of a U.S. patent (55), but its application has never been reported. We may conclude that no treatments based on ionizing radiation in human patients proved capable to assure the reverse of NIH in AVF.
The causal association between cardiovascular disease and exposure to ionizing radiation (either therapeutic, diagnostic or environmental), even at low dose, is of great concern (56–62), leading currently to revised principles for risk assessment and mitigation in patients undergoing radiotherapy, and to additional recommendations for cardiovascular management, protection and prevention (63, 64). This should be regarded as an argument for using the non-ionizing radiation as a substitute for the ionizing radiation, at least in vascular applications. The risk of radiation-induced cardiovascular disease remains present regardless of the location and type of therapeutic target.
A refreshment of the knowledge of the physics of non-ionizing radiation would be therefore beneficial. The radiation is energy emitted and transmitted as waves or particles through space or matter, and we are surrounded by electromagnetic, particle, acoustic, and gravitational radiations. Importantly, the first three types of radiation found applications in medicine. The radiations are commonly categorized into ionizing and non-ionizing, depending on the energy of the wave or particle, which ultimately determines the effect of irradiation onto the matter. While the particle radiations (α, β, protons, neutrons, positrons) are all of ionizing kind, the electromagnetic radiation presents a different situation. The current consensus holds that any radiation that carry an amount of energy higher than 10 eV is able to ionize atoms and molecules and cleave chemical bonds, therefore able to break down biomolecules. In the order of their increasing wavelength (i.e., decreasing energy), the electromagnetic spectrum contains the following regions: γ-rays, x-rays, ultraviolet, visible light, infrared, microwaves, and radio waves. The γ- and x-rays, with energies above 1,000 eV and much higher, can irreversibly damage cells and tissues. Ultraviolet (wavelength, 100–400 nm; photon energy, 12.4–3.1 eV) is the region where transition from ionizing to non-ionizing radiation occurs. As the 10-eV mark is situated within the ultraviolet-C region (100–280 nm; 12.4–4.43 eV), it appears that avoiding to irradiate biological tissue with UV-C would be a safe alternative. It is generally accepted that the UV-A region (315–400 nm; 3.94–3.1 eV) is biologically safe. Looking further, the photons in visible (400–700 nm; 3.1–1.7 eV) and infrared (700 –106 nm; 1.8–1.24 × 10–3 eV) regions are not energetic enough to trigger ionization, therefore they are considered safe for most medical applications.
The aim of this review is to present the procedures based on non-ionizing radiations that have ever been employed to prevent NIH-induced stenosis, focusing on their application for AVF management. There are five such procedures in practice or development, and they are presented in Table 1. With the exception of a brief mention (21), the applications of non-ionizing radiation were rather ignored in all major reviews. We also discuss the role played in the NIH process by one of the fundamental mechanical characteristics of the blood vessels, the compliance (alternative terms include flexibility and pliability), which is the opposite of stiffness and can be defined as the ability of a body (in this case, the venous wall) to exhibit deformation upon the action of external forces.
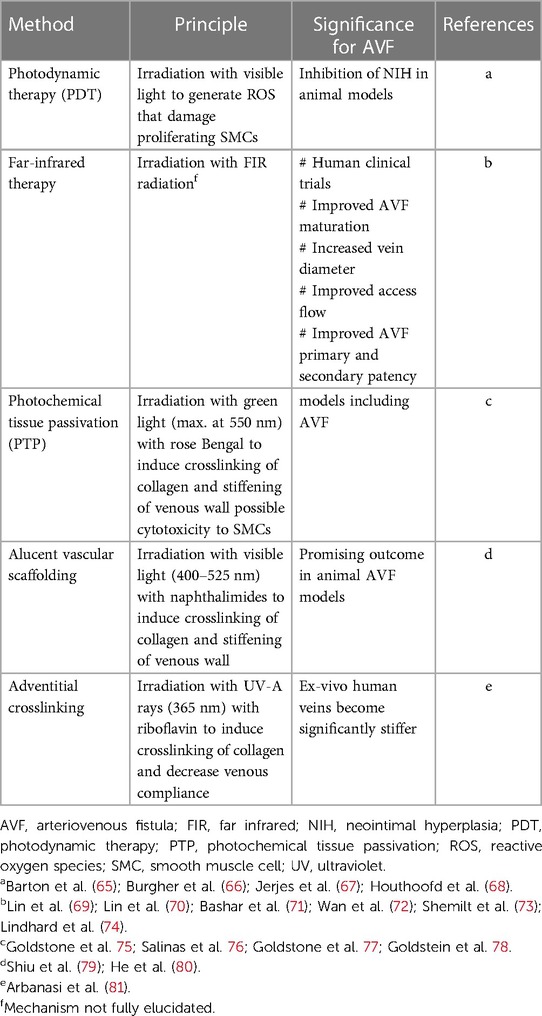
Table 1. Summary of strategies based on non-ionizing radiation to suppress venous neointimal hyperplasia.
Photodynamic therapy
A photodynamic activity in biological systems involves photochemical reactions in which oxygen is consumed to generate bioactive harmful products, a process that generally elicits cell death, the target in this case being the SMCs. In the photodynamic therapy (PDT), this photochemical process is directed to the treatment of a disease and is based on the cooperation of three essential components: a photosensitizing agent, a radiation source with a specific wavelength, and tissular oxygen. Initially developed for treating cancers, PDT is currently used in several medical applications (82–84). In brief, the process commences with the absorption by the photosensitizer (PS) molecules, located within the target tissue, of the radiation provided by the source. Upon excitation due to irradiation, PS is transformed from its ground state (the singlet, 1PS) into a short-lived excited singlet state (1PS*), which within nanoseconds will dissipate its energy excess through three alternative routes: light emission (fluorescence), heat generation, or adoption of a more stable excited state (the triplet, 3PS*) through a process called intersystem crossing. The triplet has enough long lifetime (microseconds) to transfer its excess energy to the molecular oxygen (O2) in the tissue, generating singlet oxygen (1O2), which is short-lived and has a short radius of action, but is highly reactive and can induce oxidative damage and cell death. The triplet also has enough time to react directly with the tissular biomolecules and, through transfer of electrons or hydrogen extraction, generate free radicals, which by reacting with O2 produce reactive oxygen species (ROS), mainly the superoxide (O2–·) and hydroxyl (HO·) radicals, and hydrogen peroxide (H2O2). All ROS cause severe damage to cells.
The radiation commonly used for applying PDT is within a range from the end of visible (orange/red) to the beginning of near infrared regions, i.e., ∼600–850 nm, corresponding to photon energies between ∼2.1 and 1.46 eV. Sources include dye-pumped lasers, light-emitting diodes, or conventional lamps. The latter are generally avoided because unwanted thermal side effects. There is a large variety of photosensitizers used in PDT, and new agents are in continual development (82, 85, 86), as these compounds are key factors for a successful application of PDT. They must have radiation absorption peaks within the aforementioned wavelength range, and shall possess physiochemical characteristics that facilitate their optimal distribution and efficacy in the target tissues, as well as their elimination from the body. A photosensitizer from the class of porphyrins (Photofrin II) proved to be specifically cytotoxic in vitro to human atheromatous SMCs, even without photoactivation (87) Interestingly, the in vitro photodynamic activity of this particular photosensitizer was revealed by irradiating with UV-A rays the cultured SMCs, comparatively from non-atherosclerotic arteries and from stenosing lesions (88). Only 20% of the latter remained viable following irradiation. However, ultraviolet radiation is not used currently in PDT.
The efficacy of PDT in inhibiting arterial NIH was evaluated experimentally in animal models (rabbit, dog, rat) where the initial intimal injury was created with balloon catheters (89–91). The literature reporting the application of PDT for preventing NIH and for reducing formation of atherosclerotic plaques has been recently reviewed (68). The radiation levels used in these experiments were in the wavelength range of 600–710 nm, generated by lasers at fluences up to 100 J/cm2, in the presence of a variety of photosensitizers. The general conclusion was that PDT is a promising strategy against NIH. A study has been reported (67) in human patients with congenital vascular anomalies, who were exposed to 652-nm radiation provided by a diode laser at fluences of 10–20 J/cm2 in the presence of m-tetrahydroxyphenylchlorin as a photosensitizer. After an average follow-up of 21 months, 50% of the patients displayed good response to the PDT.
Related to the vascular access in hemodialysis patients, PDT was applied to a prosthetic arteriovenous graft (AVG), where the artery and vein were connected indirectly, through a tubular graft made of polytetrafluoroethylene (PTFE, Teflon). The study (65) was performed on dogs that had femoral AVGs implanted bilaterally. Four weeks after implantation, indium chloride methylpyropheophorbide (known as PhotoPoint™ MV6401) was administered as a photosensitizer to the animals. After creating injury in the veins with balloon catheters, the anastomotic sites were irradiated with light of 590 nm wavelength at a fluence of 100 J/cm2 (source not specified). Based on the thickness reduction revealed histologically, the authors concluded that PDT effectively inhibited the formation of NIH. The same group reported a similar study (66), but using the 664-nm radiation delivered by a diode laser and a photosensitizer known as MV2101 (composition not disclosed), also with similarly positive conclusions.
As far as our literature search has extended, there was no publication reporting the use of PDT for inhibition of NIH in non-prosthetic AVF, neither in animals nor in human subjects.
Far-infrared therapy
In contrast to PDT, the use of far-infrared (FIR) radiation therapy for improving the blood flow, maturation and the patency of AVFs has been frequently reported, and was the objective of several human randomized clinical trials such as those presented in some major reviews (69–72, 74, 92, 93). It appears indeed that FIR therapy for AVF is a major application in cardiovascular medicine.
The non-ionizing far-infrared region is part of the infrared portion of electromagnetic radiation, which—in one of the many existing classifications—is contained between the wavelengths of 15 and 1,000 μm, corresponding to energies between 1.24 and 83 meV. Biologically, at such low energy, FIR is a safe radiation for medical applications. For vascular applications, emitters generating radiation within the wavelength range 3–25 μm (with a peak around 8 μm, energy 155 meV) are routinely employed. No sensitizing agents are required in this procedure. The trials reviewed in the above articles have involved thousands of hemodialysis patients (69–72, 74, 92, 93). It is important to mention that most of the FIR trials were not blinded. A range of evaluation criteria have been used, including changes in the access flow, survival number of AVF, quality of maturation, primary patency rate after one year of treatment, rate of stenosis and other AVF complications, cardiac output, assessment of inflammatory, vasoregulatory and endothelial functional factors, and changes in the content of asymmetric dimethylarginine (an inhibitor of nitric oxide synthase). However, based on changes in the content of vascular adhesion molecules, a recent clinical study (74) concluded that FIR might not have the expected vasoprotective effects, in spite of the previous favorable reports. In a prospective observational study including patients with both AVFs and AVGs (94), it was found that FIR therapy was not effective in preventing restenosis in AVFs after percutaneous translational angioplasty.
The mechanism of FIR therapeutic action is different from that of PDT and more complex. It may involve the reduction of growth rate of SMCs, but probably not by killing the cells. There are two effects of FIR, thermal and non-thermal, and they likely occur together. A number of mechanistic effects of FIR radiation have been revealed (69, 71, 73, 93, 95–97), such as vasodilation and increased access flow; angiogenesis; reduction of oxidative stress; release of anti-inflammatory factors; upregulation of endothelial nitric oxide synthase (eNOS); upregulation of heme oxygenase-1 (HO-1); inhibition of NIH. It is believed that all these effects may have a positive influence on the survival of AVFs.
Photochemical tissue passivation
The technique of photochemical tissue passivation (PTP), sometimes referred to as photochemical tissue bonding (PTB), is based on the photochemical reactions occurring when tissues are irradiated with non-ionizing visible radiation in the presence of rose Bengal. This dye is a halogenated xanthene dianion that absorbs in the green region of the electromagnetic visible spectrum, with a maximum absorption peak at 550 nm. The photochemistry behind principles of PTP is identical to those underlying PDT, however the aim of PTP is to induce the crosslinking between tissular proteins (mostly native fibrillar collagen) leading to significant enhancement of tissues' mechanical properties (e.g., stiffening) and of their enzymolytic resistance, while the cells’ life is preserved.
The methodology for exogenous crosslinking of native collagen is related to the crosslinking of engineered collagen-based materials (98–100), and has led to several therapeutic strategies for treating disorders of the connective tissue. In contrast to many photosensitizing dyes, the rose Bengal molecule manifests a preference to bind strongly to tissular collagen (101, 102). The PTP technique has been developed at Wellman Center for Photomedicine (Harvard) in Boston (102, 103). An early vascular application was reported in animal (pig, rat) arteries (104), where it provided tight seals between anastomotic surfaces.
The direct inhibition of NIH was demonstrated (75) in porcine veins of which adventitia was subjected to 550-nm radiation emitted by a diode array light source at 90–120 J/cm2, in the presence of rose Bengal. Histologically, a decrease in SMC proliferation was also noticed following PTP, which may suggest accompanying free-radical-induced cytotoxicity, like in PDT. More important, due to photochemical crosslinking of adventitial collagen, the stiffness of veins increased tenfold. The scenario proposed (75) may explain the effect of PTP. When included into the arterial environment, a vein can be injured by mechanical pressure factors such as pulsatile stretching, turbulent flow, and shear stress in the wall. Exposed to the action of these factors, the vein becomes substantially distended, which triggers endothelial injury, followed by platelet aggregation, inflammatory processes, and specific signaling cascades, leading eventually to SMC proliferation, a key element in the formation of NIH. It was assumed that by strengthening the venous adventitia, the mechanical damage is significantly restricted. Similar results were reported by the same authors in in vivo studies in rat (76) and pig models (78). The role of venous compliance/stiffness is further discussed in the last section of this review.
We are aware of only one reported study regarding the efficacy of PTP in preventing NIH formation in AVF (77). PTP was performed on the vein prior to the creation of an AVF between the femoral artery and epigastric vein in rats, by irradiating with 550-nm light (diode array source) at a fluence of 25 J/cm2 and an irradiance of 87 mW/cm2. The animals were sacrificed and assessed one month after surgery. PTP reduced venous diametric dilation by ∼70% compared to controls; it also reduced ∼4 times the juxta-anastomotic intimal area, and ∼2.5 times total intimal area. An increase in the AVF flow was recorded following PTP, but not of high statistical significance. The investigators concluded that PTP might be considered as a promising therapy for preventing AVF failure.
Alucent vascular scaffolding
This is yet another technique based on the same principle as PDT and PTP: irradiation with low-energy visible light in the presence of a photosensitizer. In fact, the only claim to the novelty of the procedure is that a new class of photosensitizers (substituted 4-amino-1,8-naphthalimides) was specifically developed for this purpose (105), although other chemical compounds were routinely available to fulfill that role. The current owner of the technology is Alucent Biomedical Inc. (Salt Lake City, UT, USA), who adopted an obvious misnomer for their proprietary product: “Natural Vascular Scaffold”. There is nothing “natural” in the concept, as the photosensitizer is a substance fully synthesized in laboratory, the radiation (wavelength 400–525 nm) is generated by manufactured instruments (xenon lamps or lasers), and luminal pre-dilation (which is crucial to the main application of the procedure) is achieved by standard balloon angioplasty. The procedure was initially intended to replace percutaneous transluminal angioplasty/stenting as a treatment for peripheral artery disease (106). It was claimed (105) that because only a limited number of crosslinks are produced in collagen, the resulting stent-like constructs will be not as rigid as the stents in current use. However, no explanation has been offered for a strategy that would be able to “limit” the extent of a photochemical crosslinking process.
The technique was applied to AVF in animal models. In such a study (79), an AVF was created between femoral vein and femoral artery in rats. After restoring blood flow, a solution of the photosensitizer (4-amino-1,8-naphthalimide) was dropped on the anastomotic site, and after 5 min the area was irradiated with 450-nm light (source not specified) for 1 min. Animals were sacrificed one month later, and tissue specimens were harvested and processed to be analyzed by histology, morphometry, immunohistochemistry, and microscopy. It was found that in the treated vessels the luminal area was larger than in controls, while the contents in IL-6 and MMP markers were significantly reduced. It was also surmised that the changes detected in the structure of native collagen might have a beneficial effect on AVF maturation. In a recent in vivo study (80), the method was applied to sheep cephalic veins using a balloon catheter coated with the photosensitizer and carrying a light fiber through which 450-nm radiation was delivered. The resulting luminal area was larger in the treated animals, where an increased number of SMCs was also observed, without noticeable NIH. In the same study, donor human saphenous and cephalic veins were subjected to the same treatment, and then to a distensibility assessment. The treated veins could tolerate up to 66% overstretch.
Adventitial photocrosslinking
Mechanical augmentation of aortic adventitia by irradiating it with UV-A rays in the presence of riboflavin has been demonstrated in ex-vivo porcine abdominal aortas (107), and proposed as a method to lower the risk of rupture of abdominal aortic aneurysms. The reinforcement effect is due to the riboflavin-photosensitized crosslinking of the adventitial collagen, and was shown to take place even if the adventitial specimens were experimentally degraded by collagenolysis (108) or elastolysis (109). There are no doubts about the chemistry underlying the method, as the radiation-induced, riboflavin-photosensitized crosslinking of tissue proteins is based on well elucidated and understood photochemical processes (102).
The method has been recently extended to the venous wall (81), with an aim at reinforcing it mechanically as a potential method to inhibit the development of NIH, of obvious relevance to the vascular access through AVFs. Whole-thickness wall specimens of human superficial femoral vein and great saphenous vein (GSV), retrieved form a patient who underwent limb amputation, were soaked in riboflavin solution and then exposed to UV-A radiation (365 nm) for 3 min at an irradiance of 50 mW/cm2. The samples were evaluated biaxially in a specialized biomechanical tester, before and after the radiative treatment. The measured Young's modulus (representing stiffness—the reciprocal of compliance) of the GSV specimens increased significantly after irradiation, by ∼120% longitudinally and ∼80% circumferentially, proving the efficacy of the method. In addition, specimens of superficial femoral artery were also included in the study (81), and the investigators found that the mechanical behavior of the irradiated vein became similar to that of the non-irradiated artery. In these experiments, the adventitia was not separated from the wall. As it is unlikely that the radiation penetrated further into the tunica media, the adventitia took up the whole radiation output. However, the mechanical effect was global, reflected in enhanced stiffness and strength of the entire venous wall. An additional complication warranting consideration of UV-A exposure as a novel therapeutic approach is the development of aneurysms in AVF, in the case where during surgery we registered an important increase in vein diameter. In instances where surgical intervention is lacking, the progression of these aneurysms may culminate in rupture, precipitating hemorrhagic shock and eventual fatality (110, 111).
Role of venous compliance
The compliance of the venous wall and the pre-operative diameter of the vein play an important role in AVF dysfunction. Numerous studies have tried to identify an optimal pre-operative venous diameter threshold to ensure AVF maturation (111–113). Thus, according to the guidelines of the European Society of Vascular and Endovascular Surgery, a minimum internal diameter for the arterial and venous component of 2 mm in the case of radiocephalic AVF (RCAVF) and a minimum of 3 mm in the case of brachiocephalic AVF (BCAVF) and brachiobasilic AVF (BBAVF) is recommended (113). Similarly Kaller et al. (111), demonstrated that a diameter greater than 2.25 mm for the radial artery and 2.55 mm for the cephalic vein is associated with a higher maturation rate in the case of RCAVF. Recently, another study (112) identified that a pre-operative diameter smaller than 2.95 mm for the artery and 2.15 mm for the vein is associated with AVF dysfunction. Moreover, other studies (114–116) have demonstrated the importance of increasing the venous diameter (intra-operatively and immediately post-operatively) in the favorable evolution of AVF. In contrast, there are several published studies (117–122) showing that by reinforcing the vein graft via an external stent/sheath made of synthetic polymers, the stiffness was enhanced, lessening the compliance mismatch, and resulting in the reduction of SMC proliferation, thus inhibiting hyperplasia.
It can be seen from the above overview (and also shown in Table 1) that three of the methods developed to inhibit NIH in venous grafts are relying on the mechanical reinforcement of the venous wall induced by photochemical crosslinking of tissular collagen, while exposed either to visible radiation, like in PTP (75–78, 104) or Alucent technique (79, 80), or to UV-A radiation, like in adventitial crosslinking method (81). The underlying hypothesis was that the reduction of vascular compliance in the venous component (equivalent to the increase of its stiffness), intended to also reduce the compliance mismatch between the two different conduits, lowers the risk of endothelial injury induced by an abnormal distension of the vein following its grafting to an artery. The role of hemodynamics in the progression of NIH and in vein-grafting outcomes has been intensively investigated over the past three decades (123, 124). It was suggested (123) that no less than 9 different mechanical effects can act upon a grafted vein conduit after being exposed to arterial pressure and flow. On the other hand, it was found (125–129) that following experimental mechanical stretching of venous conduits in vitro or in animal models, the growth factors promoting SMC proliferation were upregulated. Can an increase, achieved by exogenous means, of the venous conduit's stiffness neutralize such effects? Moreover, what shall be done about the compliance of the arterial conduit? These issues are complex and encumbered by dissenting findings or hypotheses.
Regarding the arterial component in the AVF, a review (130) showed that a stiffer arterial conduit contributes to the failure of AVF maturation, extending the conclusion to veins too with no valid reason, and recommending a general reduction of stiffness as a preventive treatment. However, other studies (131–135) failed to establish a definite role of arterial stiffness in the maturation of AVF.
Regarding the compliance of venous conduits in AVFs, two studies on human patients that included biomechanical evaluation either by plethysmography (136) or by dynamic mechanical analysis (137) have demonstrated higher failure rates with reduced venous compliance (i.e., with increased stiffness). It has also been reported that venous compliance decreases naturally with age (138) and that in hypertensive hemodialysis patients, the compliance is reduced irreversibly (139). These findings seem to cast doubt on the possibility of preventing NIH by reducing venous compliance, achievable through the cross-linking of vascular collagen. However, this issue is far from being resolved because the results of the animal model contradict (41, 140) the above-mentioned information. This obviously suggests that more research on this subject would be both relevant and beneficial. So far, FIR therapy has shown to be highly advantageous for patients with AVF, where there is no notable increase in venous diameter immediately after surgery. This therapy effectively increases the maturation and long-term patency of the AVF. As for other therapeutic strategies, while published results are promising, further studies are needed to establish the criteria for their applicability.
Conclusions
In an attempt to counter the disadvantages of therapies based on ionizing radiation, such as the risk of induced cardiovascular disease and increasingly stringent safety requirements, procedures using non-ionizing radiation are currently developed and assessed as means to inhibit neointimal hyperplasia and prevent stenosis in venous grafts. This is of crucial importance in the quest for reducing the failure rate of arteriovenous fistula in hemodialysis patients.
Author contributions
ER: Writing – review & editing. E-MA: Writing – review & editing, Writing – original draft. TC: Writing – review & editing, Writing – original draft. AM: Writing – review & editing, Writing – original draft.
Funding
The author(s) declare financial support was received for the research, authorship, and/or publication of this article.
This work was supported by the George Emil Palade University of Medicine, Pharmacy, Science, and Technology of Târgu Mureș Research Grant number 171/2/09.01.2024.
Conflict of interest
The authors declare that the research was conducted in the absence of any commercial or financial relationships that could be construed as a potential conflict of interest.
The author(s) declared that they were an editorial board member of Frontiers, at the time of submission. This had no impact on the peer review process and the final decision.
Publisher's note
All claims expressed in this article are solely those of the authors and do not necessarily represent those of their affiliated organizations, or those of the publisher, the editors and the reviewers. Any product that may be evaluated in this article, or claim that may be made by its manufacturer, is not guaranteed or endorsed by the publisher.
References
1. Carrel A, Guthrie CC. Uniterminal and biterminal venous transplantations. Surg Gynecol Obstet. (1906) 2:266–86.
2. Collins MJ, Li X, Lv W, Yang C, Protack CD, Muto A, et al. Therapeutic strategies to combat neointimal hyperplasia in vascular grafts. Expert Rev Cardiovasc. Ther. (2012) 10:635–47. doi: 10.1586/erc.12.33
3. Déglise S, Bechelli C, Allagnat F. Vascular smooth cells in intimal hyperplasia, an update. Front Physiol. (2023) 13:1081881. doi: 10.3389/fphys.2022.1081881
4. Donadoni F, Pichardo-Almarza C, Bartlett M, Dardik A, Homer-Vanniasinkam S, Diaz-Zuccarini V. Patient-specific, multi-scale modeling of neointimal hyperplasia in vein grafts. Front Physiol. (2017) 8:226. doi: 10.3389/fphys.2017.00226
5. Ebert MLA, Schmidt VF, Pfaff L, von Thaden A, Kimm MA, Wildgruber M. Animal models of neointimal hyperplasia and restenosis. Species-specific differences and implications for translational research. J Am Coll Cardiol Basic Transl Sci. (2021) 6:900–17. doi: 10.1016/j.jacbts.2021.06.006
6. Fashina O, Abbasciano RG, McQueen LW, Ladak S, George SJ, Suleiman S, et al. Large animal model of vein grafts intimal hyperplasia: a systematic review. Perfusion. (2023) 38:894–930. doi: 10.1177/02676591221091200
7. Lee T, Ul Haq N. New developments in our understanding of neointimal hyperplasia. Adv Chronic Kidney Dis. (2015) 22:431–7. doi: 10.1053/j.ackd.2015.06.010
8. Lemson MS, Tordoir JHM, Daemen MJAP, Kitslaar PJEHM. Intimal hyperplasia in vascular grafts. Eur J Vasc Endovasc Surg. (2000) 19:336–50. doi: 10.1053/ejvs.1999.1040
9. Ma S, Duan S, Liu Y, Wang H. Intimal hyperplasia of arteriovenous fistula. Ann Vasc Surg. (2022) 85:444–53. doi: 10.1016/j.avsg.2022.04.030
10. Mitra AK, Gangahar DM, Agrawal DK. Cellular, molecular and immunological mechanisms in the pathophysiology of vein graft intimal hyperplasia. Immunol Cell Biol. (2006) 84:115–24. doi: 10.1111/j.1440-1711.2005.01407.x
11. Muto A, Model L, Ziegler K, Eghbalieh SDD, Dardik A. Mechanisms of vein graft adaptation to the arterial circulation. Circ J. (2010) 74:1501–12. doi: 10.1253/circj.CJ-10-0495
12. Newby AC, Zaltsman AB. Molecular mechanisms in intimal hyperplasia. J Pathol. (2000) 190:300–9. doi: 10.1002/(SICI)1096-9896(200002)190:3%3C300::AID-PATH596%3E3.0.CO;2-I
13. Owens CD, Gasper WJ, Rahman AS, Conte MS. Vein graft failure. J Vasc Surg. (2015) 61:203–16. doi: 10.1016/j.jvs.2013.08.019
14. Wu W, Wang C, Zang H, Qi L, Azhar M, Nagarkatti M, et al. Mature vascular smooth muscle cells, but not endothelial cells, serve as the major cellular source of intimal hyperplasia in vein grafts. Arterioscler Thromb Vasc Biol. (2020) 40:1870–90. doi: 10.1161/ATVBAHA.120.314465
15. Duque JC, Tabbara M, Martinez L, Cardona J, Vazquez-Padron RI, Salman LH. Dialysis arteriovenous fistula failure and angioplasty: intimal hyperplasia and other causes of access failure. Am J Kidney Dis. (2017) 69:147–51. doi: 10.1053/j.ajkd.2016.08.025
16. Malovrh M. Non-matured arteriovenous fistulae for haemodialysis: diagnosis, endovascular and surgical treatment. Bosn J Basic Med. Sci. (2010) 10(Suppl 1):S13–7. doi: 10.17305/bjbms.2010.2640
17. Rothuizen TC, Wong CY, Quax PHA, van Zonneveld AJ, Rabelink TJ, Rotmans JI. Arteriovenous access failure: more than just intimal hyperplasia? Nephrol Dial Transplant. (2013) 28:1085–92. doi: 10.1093/ndt/gft068
18. Yen C, Tsai C, Luo Y, Yang H, Liu M, Hung P, et al. Factors affecting fistula failure in patients on chronic hemodialysis: a population-based case-control study. BMC Nephrol. (2018) 19:213. doi: 10.1186/s12882-018-1010-6
19. Barcena AJR, Perez JVD, Liu O, Mu A, Heralde FM III, Huang SY, et al. Localized perivascular therapeutic approaches to inhibit venous neointimal hyperplasia in arteriovenous fistula access for hemodialysis use. Biomolecules. (2022) 12:1367. doi: 10.3390/biom12101367
20. Beathard GA. Fistula salvage by endovascular therapy. Adv Chronic Kidney Dis. (2009) 16:339–51. doi: 10.1053/j.ackd.2009.06.001
21. Brahmbhatt A, Remuzzi A, Franzoni M, Misra S. The molecular mechanisms of hemodialysis vascular access failure. Kidney Int. (2016) 89:303–16. doi: 10.1016/j.kint.2015.12.019
23. Gorecka J, Fereydooni A, Gonzalez L, Lee SR, Liu S, Ono S, et al. Molecular targets for improving arteriovenous fistula maturation and patency. Vasc Investig Ther. (2019) 2:33–41. doi: 10.4103/VIT.VIT_9_19
24. Hu H, Patel S, Hanisch JJ, Santana JM, Hashimoto T, Bai H, et al. Future research directions to improve fistula maturation and reduce access failure. Semin Vasc Surg. (2016) 29:153–71. doi: 10.1053/j.semvascsurg.2016.08.005
25. Hu K, Guo Y, Li Y, Lu C, Cai C, Zhou S, et al. Oxidative stress: an essential factor in the process of arteriovenaous fistula failure. Front Cardiovasc Med. (2022) 9:984472. doi: 10.3389/fcvm.2022.984472
26. Lee T, Roy-Chaudhurry P. Advances and new frontiers in the pathophysiology of venous neointimal hyperplasia and dialysis access stenosis. Adv Chronic Kidney Dis. (2009) 16:329–38. doi: 10.1053/j.ackd.2009.06.009
27. Quencer KB, Arici M. Arteriovenous fistulas and their characteristic sites of stenosis. Am J Roentgenol. (2015) 205:726–34. doi: 10.2214/AJR.15.14650
28. Tanner NC, da Silva AF. Medical adjuvant treatment to improve the patency of arteriovenous fistulae and grafts: a systematic review and meta-analysis. Eur J Vasc Endovasc Surg. (2016) 52:243–52. doi: 10.1016/j.ejvs.2016.04.016
29. Yevzlin AS, Chan MR, Becker YT, Roy-Chaudhury P, Lee T, Becker BN. “Venopathy” at work: recasting neointimal hyperplasia in a new light. Transl Res. (2010) 156:216–25. doi: 10.1016/j.trsl.2010.07.004
30. Zhao J, Jourd’heuil FL, Xue M, Conti D, Lopez-Soler RI, Ginnan R, et al. Dual function for mature vascular smooth muscle cells during arteriovenous fistula remodeling. J Am Heart Assoc. (2017) 6:e004891. doi: 10.1161/JAHA.116.004891
31. Brescia MJ, Cimino JE, Appel K, Hurwich BJ. Chronic hemodialysis using venipuncture and surgically created arteriovenous fistula. N Engl J Med. (1966) 275:1089–92. doi: 10.1056/NEJM196611172752002
32. Mishler R. Autologous arteriovenous fistula creation by nephrologists. Adv Chronic Kidney Dis. (2009) 16:321–8. doi: 10.1053/j.ackd.2009.07.004
33. Arasu R, Jegatheesan D, Sivakumaran Y. Overview of hemodialysis access and assessment. Can Fam Physician. (2022) 68:577–82. doi: 10.46747/cfp.6808577
34. Neyra NR, Wazir S. The evolving panorama of vascular access in the 21st century. Front Nephrol. (2022) 2:917265. doi: 10.3389/fneph.2022.917265
35. MacRae JM, Ahmed S, Hemmelgarn B, Sun Y, Martin B-J, Roifman I, et al. Role of vascular function in predicting arteriovenous fistula outcomes: an observational pilot study. Can J Kidney Health Dis. (2015) 2:19. doi: 10.1186/s40697-015-0055-8
36. Siddiqui MA, Ashraff S, Santos D, Carline T. An overview of AVF maturation and endothelial dysfunction in an advanced renal failure. Ren Replace Ther. (2017) 3:42. doi: 10.1186/s41100-017-0123-x
37. Borin T.F., Miyakawa A.A., Cardoso L., de Figueiredo Borges L., Goncalves G.A., Krieger J.E. (2009) Apoptosis, cell proliferation and modulation of cyclin-dependent kinase inhibitor p21 (cip1) in vascular remodeling during vein arterialization in the rat. Int J Exp Pathol., 3, 328–37. doi: 10.1111/j.1365-2613.2009.00648.x
38. de Vries MR, Simons KH, Jukema JW, Braun J, Quax PH. Vein graft failure: from pathophysiology to clinical outcomes. Nat Rev Cardiol. (2016) 13:451–70. doi: 10.1038/nrcardio.2016.76
39. Lardenoye JH, de Vries MR, Lowik CW, Xu Q, Dhore CR, Cleutjens JP, et al. Accelerated atherosclerosis and calcification in vein grafts: a study in APOE*3 Leiden transgenic mice. Circ Res. (2002) 7:577–84. doi: 10.1161/01.RES.0000036901.58329.D7
40. Kaller R, Russu E, Arbanasi E-M, Muresan AV, Jakab M, Ciucanu CC, et al. Intimal CD31-positive relative surfaces are associated with systematic inflammatory markers and maturation of arteriovenous fistula in dialysis patients. J Clin Med. (2023) 12:4419. doi: 10.3390/jcm12134419
41. Yang Q, Lei D, Huang S, Yang Y, Yang Y, Ye X, et al. Effects of the different-sized external stents on vein graft intimal hyperplasia and inflammation. Ann Transl Med. (2020) 8:102. doi: 10.21037/atm.2020.01.16
42. Friedman M, Felton L, Byers S. The antiatherogenic effect of iridium192 upon the cholesterol-fed rabbit. J Clin Invest. (1964) 43:185–92. doi: 10.1172/JCI104903
43. Diehm N, Silvestro A, Do D, Greiner R, Triller J, Mahler F, et al. Endovascular brachytherapy after femoropopliteal balloon angioplasty fails to show robust clinical benefit over time. J Endovasc Ther. (2005) 12:723–30. doi: 10.1583/05-1583MR.1
44. Holmes DR, Teirstein PS, Satler L, Sketch MH, Popma JJ, Mauri L, et al. 3-Year follow-up of the SISR (sirolimus-eluting stents versus vascular brachytherapy for in-stent restenosis) trial. J Am Coll Cardiol Intv. (2008) 1:439–48. doi: 10.1016/j.jcin.2008.05.010
45. Krueger K, Bendel M, Zaehringer M, Reinicke G, Lackner K. Centered endovascular irradiation to prevent postangioplasty restenosis of arteriovenous fistula in hemodialysis patients. Results of a feasibility study. Cardiovasc Rad Med. (2004) 5:1–8. doi: 10.1016/j.carrad.2004.02.005
46. Misra S, Bonan R, Pflederer T, Roy-Chaudhury P. BRAVO I: a pilot study of vascular brachytherapy in polytetrafluoroethylene dialysis access grafts. Kidney Int. (2006) 70:2006–13. doi: 10.1038/sj.ki.5001869
47. Rodriguez VM, Grove J, Yelich S, Pearson D, Stein M, Pevec WC. Effects of brachytherapy on intimal hyperplasia in arteriovenous fistulas in a porcine model. J Vasc Interv Radiol. (2002) 13:1239–46. doi: 10.1016/S1051-0443(07)61971-X
48. Roy-Chaudhury P, Kelly BS, Melhem M, Zhang J, Li J, Desai P, et al. Vascular access in hemodialysis: issues, management, and emerging concepts. Cardiol Clin. (2005) 23:249–73. doi: 10.1016/j.ccl.2005.04.004
49. Roy-Chaudhury P, Arnold P, Seigel J, Misra S. From basic biology to randomized clinical trial: the beta radiation for arteriovenous graft outflow stenosis (BRAVO II). Semin Dial. (2013) 26:227–32. doi: 10.1111/sdi.12000
50. Sarac TP, Riggs PN, Williams JP, Feins RH, Baggs R, Rubin P, et al. The effects of low-dose radiation on neointimal hyperplasia. J Vasc Surg. (1995) 22:17–24. doi: 10.1016/S0741-5214(95)70083-8
51. Stolic R. Most important chronic complications of arteriovenous fistulas for hemodialysis. Med Princ Pract. (2013) 22:220–8. doi: 10.1159/000343669
52. Sun S, Beitler JJ, Ohki T, Calderon TM, Schechner R, Yaparpalvi R, et al. Inhibitory effect of brachytherapy on intimal hyperplasia in arteriovenous fistula. J Surg Res. (2003) 115:200–8. doi: 10.1016/S0022-4804(03)00253-1
53. Trerotola SO, Carmody TJ, Timmerman RD, Bergan KA, Dreesen RG, Frost SV, et al. Brachytherapy for the prevention of stenosis in a canine hemodialysis graft model: preliminary observations. Radiology. (1999) 212:748–54. doi: 10.1148/radiology.212.3.r99se28748
54. Waksman R, Ajani AE, White RL, Chan RC, Sattler LF, Kent KM, et al. Intravascular gamma radiation for in-stent restenosis in saphenous-vein bypass grafts. N Engl J Med. (2002) 346:1194–9. doi: 10.1056/NEJMoa012579
55. Clarke RH. Inhibition of Restenosis by Ultraviolet Radiation. U.S. Patent No. 5,053,033. Washington, DC: U.S. Patent and Trademark Office (1991).
56. Baker JE, Moulder JE, Hopewell JW. Radiation as a risk factor for cardiovascular disease. Antiox Redox Sign. (2011) 15:1945–56. doi: 10.1089/ars.2010.3742
57. Baselet B, Rombouts C, Benotmane AM, Baatout S, Aerts A. Cardiovascular diseases related to ionizing radiation: the risk of low-dose exposure (review). Int J Mol Med. (2016) 38:1623–41. doi: 10.3892/ijmm.2016.2777
58. Bhattacharya S, Asaithamby A. Ionizing radiation and heart risks. Semin Cell Dev Biol. (2016) 58:14–25. doi: 10.1016/j.semcdb.2016.01.045
59. Little MP, Lipshultz SE. Low dose radiation and circulatory diseases: a brief narrative review. Cardio-Oncology. (2015) 1:4. doi: 10.1186/s40959-015-0007-6
60. Puukila S, Lemon JA, Lees SJ, Tai TC, Boreham DR, Khaper N. Impact of ionizing radiation on the cardiovascular system: a review. Rad Res. (2017) 188:539–46. doi: 10.1667/RR14864.1
61. Sylvester CB, Abe J, Patel ZS, Grande-Allen KJ. Radiation-induced cardiovascular disease: mechanisms and importance of energy linear transfer. Front Cardiovasc Med. (2018) 5:5. doi: 10.3389/fcvm.2018.00005
62. Yan X, Sasi SP, Gee H, Lee JY, Yang Y, Mehrzad R, et al. Cardiovascular risks associated with low dose ionizing particle radiation. PLoS One. (2014) 9:e110269. doi: 10.1371/journal.pone.0110269
63. Little MP, Azizova TV, Richardson DB, Tapio S, Bernier M-O, Kreuzer M, et al. Ionising radiation and cardiovascular disease: systematic review and meta-analysis. Br Med J. (2023) 380:e072924. doi: 10.1136/bmj-2022-072924
64. Mitchell JD, Cehic DA, Morgia M, Bergom C, Toohey J, Guerrero PA, et al. Cardiovascular manifestations from therapeutic radiation. A multidisciplinary expert consensus statement from the International Cardio-Oncology Society. J Am Coll Cardiol CardioOnc. (2021) 3:360–80. doi: 10.1016/j.jaccao.2021.06.003
65. Barton J, Nielsen H, Rychnovsky S, Farooq M, Freischlag J, Grove R. Photopoint™ photodynamic therapy inhibits intimal hyperplasia in arteriovenous access grafts. Cardiovasc Rad Med. (2002) 3:147–51. doi: 10.1016/S1522-1865(03)00081-7
66. Burgher JM, Barton JM, Farooq MM, Vasek J, Scott RW, Freischlag JA, et al. Photopoint™ photodynamic therapy with local drug delivery eliminates vessel wall cells in arteriovenous graft models. Cardiovasc Rad Med. (2002) 3:163–8. doi: 10.1016/S1522-1865(03)00080-5
67. Jerjes W, Upile T, Hamdoon Z, Mosse CA, Akram S, Morley S, et al. Interstitial PDT for vascular anomalies. Lasers Surg Med. (2011) 43:357–65. doi: 10.1002/lsm.21058
68. Houthoofd S, Vuylsteke M, Mordon S, Fourneau I. Photodynamic therapy for atherosclerosis. The potential of indocyanine green. Photodiagn Photodyn Ther. (2020) 29:101568. doi: 10.1016/j.pdpdt.2019.10.003
69. Lin C, Chang C, Lai M, Chen T, Lee P, Yang W. Far-infrared therapy: a novel treatment to improve access blood flow and unassisted patency of arteriovenous fistula in hemodialysis patients. J Am Soc Nephrol. (2007) 18:985–92. doi: 10.1681/ASN.2006050534
70. Lin C, Yang W, Chen M, Liu W, Yang C, Lee P. Effect of far infrared therapy on arteriovenous fistula maturation: an open-label randomized controlled trial. Am J Kidney Dis. (2013) 62:304–11. doi: 10.1053/j.ajkd.2013.01.015
71. Bashar K, Healy D, Browne LD, Kheirelseid EAH, Walsh MT, Clarke-Moloney M, et al. Role of far infra-red therapy in dialysis arterio-venous fistula maturation and survival: systematic review and meta-analysis. PLoS One. (2014) 9:e104931. doi: 10.1371/journal.pone.0104931
72. Wan Q, Yang S, Li L, Chu F. Effects of far infrared therapy on arteriovenous fistulas in hemodialysis patients: a meta-analysis. Ren Fail. (2017) 39:613–22. doi: 10.1080/0886022X.2017.1361835
73. Shemilt R, Bagabir H, Lang C, Khan F. Potential mechanisms for the effects of far-infrared on the cardiovascular system — a review. Vasa. (2019) 48:303–12. doi: 10.1024/0301-1526/a000752
74. Lindhard K, Jensen BL, Pedersen BL, Meyer-Olesen C, Rix M, Hansen HP, et al. Far infrared treatment on the arteriovenous fistula induces changes in sVCAM and sICAM in patients on hemodialysis. Nephrol Dial Transplant. (2023) 38:1752–60. doi: 10.1093/ndt/gfad032
75. Goldstone RN, McCormack MC, Khan SI, Salinas HM, Meppelink A, Randolph MA, et al. Photochemical tissue passivation reduces vein graft intimal hyperplasia in a swine model of arteriovenous bypass grafting. J Am Heart Assoc. (2016) 5:e003856. doi: 10.1161/JAHA.116.003856
76. Salinas HM, Khan SI, McCormack MC, Fernandes JR, Gfrerer L, Watkins MT, et al. Prevention of vein graft intimal hyperplasia with photochemical tissue passivation. J Vasc Surg. (2017) 65:190–6. doi: 10.1016/j.jvs.2015.11.049
77. Goldstone RN, McCormack MC, Goldstein RL, Mallidi S, Randolph MA, Watkins MT, et al. Photochemical tissue passivation attenuates AV fistula intimal hyperplasia. Ann Surg. (2018) 267:183–8. doi: 10.1097/SLA.0000000000002046
78. Goldstein RL, McCormack MC, Mallidi S, Runyan G, Randolph MA, Austen Jr WG, et al. Photochemical tissue passivation of arteriovenous grafts prevents long-term development of intimal hyperplasia in a swine model. J Surg Res. (2020) 253:280–7. doi: 10.1016/j.jss.2020.03.006
79. Shiu Y, He Y, Tey JCS, Knysheva M, Anderson B, Kauser K. Natural vascular scaffolding treatment promotes outward remodeling during arteriovenous fistula development in rats. Front Bioeng Biotechnol. (2021) 9:622617. doi: 10.3389/fbioe.2021.622617
80. He Y, Anderson B, Hu Q, Hayes RB, Huff K, Isaacson J, et al. Photochemically aided arteriovenous fistula creation to accelerate fistula maturation. Int J Mol Sci. (2023) 24:7571. doi: 10.3390/ijms24087571
81. Arbanasi E-M, Suzuki S, Ciucanu CC, Muresan AV, Cosarca CM, Chirila TV, et al. Ex-vivo mechanical augmentation of human saphenous vein graft by UV-A irradiation in emergency vascular reconstruction—preliminary results. J Cardiovasc Emerg. (2023) 9:59–64. doi: 10.2478/jce-2023-0012
82. Correia JH, Rodrigues JA, Pimenta S, Dong T, Yang Z. Photodynamic therapy review: principles, photosensitizers, applications, and future directions. Pharmaceutics. (2021) 13:1332. doi: 10.3390/pharmaceutics13091332
83. Dougherty TJ, Gomer CJ, Henderson BW, Jori G, Kessel D, Korbelik M, et al. Photodynamic therapy. J Natl Cancer Inst. (1998) 90:889–905. doi: 10.1093/jnci/90.12.889
84. Wilson BC, Patterson MS. The physics, biophysics and technology of photodynamic therapy. Phys Med Biol. (2008) 53:R61–R109. doi: 10.1088/0031-9155/53/9/R01
85. Abrahamse H, Hamblin MR. New photosensitizers for photodynamic therapy. Biochem J. (2016) 473:347–64. doi: 10.1042/BJ20150942
86. Allison RR, Downie GH, Cuenca R, Hu X, Childs CJH, Sibata CH. Photosensitizers in clinical PDT. Photodiagn Photodyn Ther. (2004) 1:27–42. doi: 10.1016/S1572-1000(04)00007-9
87. Dartsch PC, Ischinger T, Betz E. Differential effect of photofrin II on growth of human smooth muscle cells from nonatherosclerotic arteries and atheromatous plaques in vitro. Arteriosclerosis. (1990) 10:616–24. doi: 10.1161/01.ATV.10.4.616
88. Dartsch PC, Ischinger T, Betz E. Responses of cultured smooth muscle cells from human nonatherosclerotic arteries and primary stenosing lesions after photoradiation: implications for photodynamic therapy of vascular stenosis. J Am Coll Cardiol. (1990) 15:1545–50. doi: 10.1016/0735-1097(90)92824-L
89. Adili F, van Eps RGS, Flotte TJ, LaMuraglia GM. Photodynamic therapy with local photosensitizer delivery inhibits experimental intimal hyperplasia. Lasers Surg Med. (1998) 23:263–73. doi: 10.1002/(SICI)1096-9101(1998)23:5%3C263::AID-LSM6%3E3.0.CO;2-V
90. Hsiang Y, Houston G, Crespo T, To E, Todd M, Sobeh M, et al. Preventing intimal hyperplasia with photodynamic therapy using an intravascular probe. Ann Vasc Surg. (1995) 9:80–6. doi: 10.1007/BF02015320
91. Visonà A, Angelini A, Gobbo S, Bonanome A, Thiene G, Pagnan A, et al. Local photodynamic therapy with Zn(II)-phthalocyanine in an experimental model of intimal hyperplasia. J Photochem Photobiol B: Biol. (2000) 57:94–101. doi: 10.1016/S1011-1344(00)00083-X
92. Chen C, Lee C, Chen F, Yang C, Chen T, Ou S, et al. Far-infrared therapy improves arteriovenous fistula patency and decreases plasma asymmetric dimethylarginine in patients with advanced diabetic kidney disease: a prospective randomized controlled trial. J Clin Med. (2022) 11:4168. doi: 10.3390/jcm11144168
93. Lindhard K, Rix M, Heaf JG, Hansen HP, Pedersen BL, Jensen BL, et al. Effect of far infrared therapy on arteriovenous fistula maturation, survival and stenosis in hemodialysis patients, a randomized, controlled clinical trial: the FAITH on fistula trial. BMC Nephrol. (2021) 22:283. doi: 10.1186/s12882-021-02476-x
94. Lai C-C, Fang H-C, Mar G-Y, Liou J-C, Tseng C-J, Liu C-P. Post-angioplasty far infrared radiation therapy improves 1-year angioplasty-free hemodialysis access patency of recurrent obstructive lesions. Eur J Vasc Endovasc Surg. (2013) 46:726–32. doi: 10.1016/j.ejvs.2013.09.018
95. Kipshidze N, Nikolaychik V, Muckerheidi M, Keelan MH, Chekanov V, Maternowski M, et al. Effect of short pulsed nonablative infrared laser irradiation on vascular cells in vitro and neointimal hyperplasia in a rabbit balloon injury model. Circulation. (2001) 104:1850–5. doi: 10.1161/hc3901.096101
96. Lin C, Liu X, Peyton K, Wang H, Yang W, Lin S, et al. Far infrared therapy inhibits vascular endothelial inflammation via the induction of heme oxygenase-1. Arterioscler Thromb Vasc Biol. (2008) 28:739–45. doi: 10.1161/ATVBAHA.107.160085
97. Tu Y, Chen S, Liu Y, Chen C, Hour T. Postconditioning with far-infrared irradiation increases heme oxygenas-1 expression and protects against ischemia/reperfusion injury in rat testis. Life Sci. (2013) 92:35–41. doi: 10.1016/j.lfs.2012.10.019
98. Gu L, Shan T, Ma Y, Tay FR, Niu L. Novel biomedical applications of crosslinked collagen. Trends Biotechnol. (2019) 37:464–91. doi: 10.1016/j.tibtech.2018.10.007
99. Nair M, Best SM, Cameron RE. Crosslinking collagen constructs: achieving cellular selectivity through modifications of physical and chemical properties. Appl Sci. (2020) 10:6911. doi: 10.3390/app10196911
100. Parenteau-Bareil R, Gauvin R, Berthod F. Collagen-based biomaterials for tissue engineering applications. Materials (Basel). (2010) 3:1863–87. doi: 10.3390/ma3031863
101. Alarcon EI, Poblete H, Roh H, Couture J-F, Comer J, Kochevar IE. Rose Bengal binding to collagen and tissue photobinding. ACS Omega. (2017) 2:6646–57. doi: 10.1021/acsomega.7b00675
102. Redmond RW, Kochevar IE. Medical applications of rose Bengal- and riboflavin-photosensitized protein crosslinking. Photochem Photobiol. (2019) 95:1097–115. doi: 10.1111/php.13126
103. Scott BB, Randolph MA, Guastaldi FPS, Wu RC, Redmond RW. Light-activated vascular anastomosis. Surg Innov. (2022) 30:143–9. doi: 10.1177/15533506221104382
104. O’Neill AC, Winograd JM, Zeballos JL, Johnson TS, Randolph MA, Bujold KE, et al. Microvascular anastomosis using a photochemical tissue bonding technique. Lasers Surg Med. (2007) 39:716–22. doi: 10.1002/lsm.20548
105. Munger KA, Downey TM, Haberer B, Pohlson K, Marshall LL, Utecht RE. A novel photochemical cross-linking technology to improve luminal gain, vessel compliance, and buckling post-angioplasty in porcine arteries. J Biomed Mater Res B Appl Biomater. (2016) 104:375–84. doi: 10.1002/jbm.b.33373
106. Kauser K, Warner KS, Anderson B, Keyes ED, Hayes RB, Kawamoto E, et al. Creating a natural vascular scaffold by photochemical treatment of the extracellular matrix for vascular applications. Int J Mol Sci. (2022) 23:683. doi: 10.3390/ijms23020683
107. Chirila TV, Suzuki S. Photocrosslinking of adventitial collagen in the porcine abdominal aorta: a preliminary approach to a strategy for prevention of aneurysmal rupture. Designs. (2022) 6:5. doi: 10.3390/designs6010005
108. Chirila TV, Suzuki S. Effects of ultraviolet-A radiation on enzymatically degraded tunica adventitia of the porcine abdominal aorta. Biomed Mater Dev. (2023) 1:1000–8. doi: 10.1007/s44174-023-00080-1
109. Chirila TV, Suzuki S. Ultraviolet-induced mechanical augmentation of the degraded porcine adventitia: its significance for preventing aneurysmal rupture. Global Transl. Med. (2023) 2:0897. doi: 10.36922/gtm.0897
110. Arbanasi E-M, Russu E, Muresan AV, Arbanasi E-M, Kaller R. Ulnar-basilic arteriovenous fistula with multilocular gigantic aneurysmal dilatation: a case report. Acta Marisiensis. (2021) 67:244–6. doi: 10.2478/amma-2021-0035
111. Kaller R, Muresan AV, Arbanasi E-M, Arbanasi EM, Kovacs I, Horvath E, et al. Uncommon surgical management by AVF between the great saphenous vein and anterior tibial artery for old radiocephalic AVF failure. Life. (2022) 12:529. doi: 10.3390/life12040529
112. Martinez-Mier G, Cisneros-Tinoco MA, Sanchez-Ruiz FG. Vein and artery diameter influence on arteriovenous fistula maturation and patency in a Mexican pooplation. J Vasc Access. (2023) 4:599–605. doi: 10.1177/11297298211044023
113. Schmidli J, Widmer MK, Basile C, de Donato G, Gallieni M, Gibbons CP, et al. Editor’s choice—vascular access: 2018 clinical practice guidelines of the European society for vascular surgery (ESVS). Eur J Vasc Endovasc Surg. (2018) 6:757–818. doi: 10.1016/j.ejvs.2018.02.001
114. Kim JT, Cjang WH, Oh TY, Jeong YK. Venous distensibility as a key factor in the success of arteriovenous fistulas at the wrist. Ann Vasc Surg. (2011) 8:1094–8. doi: 10.1016/j.avsg.2011.05.014
115. Malovrh M. Native arteriovenous fistula: preoperative evaluation. Am J Kidney Dis. (2002) 6:1218–25. doi: 10.1053/ajkd.2002.33394
116. Venu M, Vedamurthy Reddy P, Mallikarjuna Reddy N, Vijayabhaskar Reddy G, Ranadheer B, Sudeep B. Can Pre and postoperative vein diameter and postoperative flow velocities influence the patency of vascular access in hemodialysis patients? Indian J Vasc Endovasc Surg. (2018) 3:145–8. doi: 10.4103/ijves.ijves_26_18
117. Angelini GD, Lloyd C, Bush R, Johnson J, Newby AC. An external, oversized, porous polyester stent reduces vein graft neointima formation, cholesterol concentration, and vascular cell adhesion molecule 1 expression in cholesterol-fed pigs. J Thorac Cardiovasc. Surg. (2002) 124:950–6. doi: 10.1067/mtc.2002.127004
118. Dashwood M, Angelini G, Wan S, Yim A, Mehta D, Izzat MB, et al. Does external stenting reduce porcine vein-graft occlusion via an action on vascular nerves? J Cardiol Surg. (2002) 17:556–60. doi: 10.1046/j.1540-8191.2002.01012.x
119. Desai M, Mirzay-Razzazz J, von Delft D, Sarkar S, Hamilton G, Seifalian AM. Inhibition of neointimal formation and hyperplasia in vein grafts by external stent/sheath. Vasc Med. (2010) 15:287–97. doi: 10.1177/1358863X10366479
120. Jeremy JY, Bulbulia R, Johnson JL, Godsdon P, Vijayan V, Shukla N, et al. A bioabsorbable (polyglactin), nonrestrictive, external sheath inhibits porcine saphenous vein graft thickening. J Thorac Cardiovasc Surg. (2004) 127:1766–72. doi: 10.1016/j.jtcvs.2003.09.054
121. Liu SQ, Ruan YY, Tang D, Li YC, Goldman J, Zhong L. A possible role of initial cell death due to mechanical stretch in the regulation of subsequent cell proliferation in experimental vein grafts. Biomech Model Mechanobiol. (2002) 1:17–27. doi: 10.1007/s10237-002-0003-2
122. Vijayan V, Smith FCT, Angelini GD, Bulbulia RA, Jeremy JY. External supports and the prevention of neointimal formation in vein grafts. Eur J Vasc Surg. (2002) 24:13–22. doi: 10.1053/ejvs.2002.1676
123. Dobrin PB, Liftooy FN, Endean ED. Mechanical factors predisposing to intimal hyperplasia and medial thickening in autogenous vein grafts. Surgery. (1989) 105:393–400.2922677
124. Hammes M. Hemodynamic and biologic determinates of arteriovenous fistula outcomes in renal failure patients. Biomed Res. Int. (2015) 2015:171674. doi: 10.1155/2015/171674
125. Chen Y, Bornfeldt KE, Arner A, Jennische E, Malmqvist U, Uvelius B, et al. Increase in insulin-like growth factor I in hypertrophying smooth muscle. Am J Physiol. Endocrinol Metab. (1994) 266:E224–9. doi: 10.1152/ajpendo.1994.266.2.E224
126. Cheng J, Du J. Mechanical stretch simulates proliferation of venous smooth muscle cells through activation of the insulin-like growth factor-1 receptor. Arterioscler Thromb. Vasc Biol. (2007) 27:1744–51. doi: 10.1161/ATVBAHA.107.147371
127. Predel H-G, Yang Z, von Segesser L, Turina M, Bühler FR, Lüscher TF. Implications of pulsatile stretch on growth of saphenous vein and mammary artery smooth muscle. Lancet. (1992) 340:878–9. doi: 10.1016/0140-6736(92)93287-W
128. Standley PR, Obards TJ, Martina CL. Cyclic stretch regulates autocrine IGF-I in vascular smooth muscle cells: implications in vascular hyperplasia. Am J Physiol Endocrinol Metab. (1999) 276:E695–705. doi: 10.1152/ajpendo.1999.276.4.E697
129. Song H, Mowbray AL, Sykes MC, Jo H. Emerging role of IGF-IR in stretch-induced neointimal hyperplasia in venous grafts (editorial). Arterioscler Thromb Vasc Biol. (2007) 27:1679–81. doi: 10.1161/ATVBAHA.107.148189
130. Paulson WD. Does vascular elasticity affect arteriovenous fistula maturation? Open Urol Nephrol J. (2014) 7(Suppl 1 M4):26–32. doi: 10.2174/1874303X01407010026
131. Allon M, Greene T, Dember LM, Vita JA, Cheung AK, Hamburg NM, et al. Association between preoperative vascular function and postoperative arteriovenous fistula development. J Am Soc Nephrol. (2016) 27:3788–95. doi: 10.1681/ASN.2015020141
132. Blacher J, Guerin AP, Pannier B, Marchais S, Safar ME, London GM. Impact of aortic stiffness on survival in end-stage renal disease. Circulation. (1999) 99:2434–9. doi: 10.1161/01.CIR.99.18.2434
133. MacDonald C-J, Ross R, Houston JG. Increased brachial artery compliance after arteriovenous fistula creation as measured by shear wave elastography. J Ultrasound Med. (2020) 39:875–81. doi: 10.1002/jum.15169
134. Masengu A, Hanko JB, Maxwell AP. Arterial stiffness and arteriovenous fistula failure of maturation. J Vasc Access. (2016) 17:477–82. doi: 10.5301/jva.5000599
135. McGrogan DG, Stringer S, Cockwell P, Jesky M, Ferro C, Maxwell AP, et al. Arterial stiffness alone does not explain arteriovenous fistula outcomes. J Vasc Access. (2018) 19:63–8. doi: 10.5301/jva.5000791
136. van der Linden J, Lameris TW, van den Meiracker AH, de Smet AAEA, Blankestijn PJ, van den Dorpel MA. Forearm venous distensibility predicts successful arteriovenous fistula. Am J Kidney Dis. (2006) 47:1013–9. doi: 10.1053/j.ajkd.2006.01.033
137. Smith GE, Barnes R, Fagan M, Chetter IC. The impact of vein mechanical compliance on arteriovenous fistula outcomes. Ann Vasc Surg. (2016) 32:9–14. doi: 10.1016/j.avsg.2015.11.002
138. Lopes A, Lloret-linares C, Simoneau G, Levy B, Bergmann J-F, Mouly S. Impact of physiological aging on lower limb venous compliance. Eur Ger Med. (2013) 4:133–8. doi: 10.1016/j.eurger.2012.12.005
139. Kooman JP, Wijnen JAG, Draaijer P, van Bortel LMAB, Gladziwa U, Peltenburg HG, et al. Compliance and reactivity of the peripheral venous system in chronic intermittent hemodialysis. Kidney Int. (1992) 41:1041–8. doi: 10.1038/ki.1992.158
Keywords: venous grafts, neointimal hyperplasia, arteriovenous fistula, non-ionizing radiotherapies, collagen crosslinking, vascular compliance
Citation: Russu E, Arbanasi E-M, Chirila TV and Muresan AV (2024) Therapeutic strategies based on non-ionizing radiation to prevent venous neointimal hyperplasia: the relevance for stenosed arteriovenous fistula, and the role of vascular compliance. Front. Cardiovasc. Med. 11:1356671. doi: 10.3389/fcvm.2024.1356671
Received: 18 December 2023; Accepted: 23 January 2024;
Published: 5 February 2024.
Edited by:
Nhat Tu Le, Houston Methodist Research Institute, United StatesReviewed by:
Laisel Martinez, University of Miami, United StatesAnahita Mojiri, Houston Methodist Research Institute, United States
Surbhi Chaudhary, Cornell University, United States
© 2024 Russu, Arbanasi, Chirila and Muresan. This is an open-access article distributed under the terms of the Creative Commons Attribution License (CC BY). The use, distribution or reproduction in other forums is permitted, provided the original author(s) and the copyright owner(s) are credited and that the original publication in this journal is cited, in accordance with accepted academic practice. No use, distribution or reproduction is permitted which does not comply with these terms.
*Correspondence: Traian V. Chirila traian.chirila@qei.org.au
†These authors have contributed equally to this work and share first authorship
Abbreviations AVF, arteriovenous fistula; AVG, arteriovenous graft; FIR, far infrared; GSV, great saphenous vein; NIH, neointimal hyperplasia; PDT, photodynamic therapy; PS, photosensitizer; PTP, photochemical tissue passivation; ROS, reactive oxygen species; SMC, smooth muscle cell, UV, ultraviolet.