- 1Department of Cardiovascular Disease, Inova Schar Heart and Vascular, Inova Fairfax Medical Campus, Falls Church, VA, United States
- 2Department of Cardiovascular Disease, Virginia Heart, Falls Church, VA, United States
- 3Department of Medicine, University of Texas Southwestern Medical Center, Dallas, TX, United States
Cardiogenic shock (CS) is a time-sensitive and hemodynamically complex syndrome with a broad spectrum of etiologies and clinical presentations. Despite contemporary therapies, CS continues to maintain high morbidity and mortality ranging from 35 to 50%. More recently, burgeoning observational research in this field aimed at enhancing the early recognition and characterization of the shock state through standardized team-based protocols, comprehensive hemodynamic profiling, and tailored and selective utilization of temporary mechanical circulatory support devices has been associated with improved outcomes. In this narrative review, we discuss the pathophysiology of CS, novel phenotypes, evolving definitions and staging systems, currently available pharmacologic and device-based therapies, standardized, team-based management protocols, and regionalized systems-of-care aimed at improving shock outcomes. We also explore opportunities for fertile investigation through randomized and non-randomized studies to address the prevailing knowledge gaps that will be critical to improving long-term outcomes.
Introduction
Cardiogenic Shock (CS) is a multifactorial, hemodynamically complex syndrome characterized by profound and refractory circulatory collapse due to impaired myocardial contractility resulting in systemic hypoperfusion, metabolic acidosis, and refractory multiorgan system dysfunction (1). Despite more than two decades of advances in interventional techniques, the development of rapidly deployable temporary mechanical circulatory support (tMCS) devices, and systems-of-care strategies to treat AMI, patients with CS continue to fare poorly with 30%–50% risk of 30-day mortality and associated multiorgan dysfunction in the modern era (2–8). It was not until the landmark Should We Emergently Revascularize Occluded Arteries in Cardiogenic Shock (SHOCK) trial in 1999, however, that a strategy of early revascularization was demonstrated to improve outcomes (9, 10). While randomized controlled trials (RCTs) have failed to demonstrate clear benefit with either pharmacologic or device-based therapies, burgeoning observational research from North American CS registries have highlighted the potential merits of standardized, team-based care in improving the care of patients with CS (11–14). These studies suggest that the implementation of a multidisciplinary “Shock team,” employing early utilization of pulmonary artery catheter (PAC) hemodynamic monitoring, selective and hemodynamically tailored tMCS and comprehensive longitudinal care in an American Heart Association (AHA) Level 1 cardiac intensive care unit (CICU) may be associated with reduced in-hospital mortality. The heterogeneity of clinical presentations, complex hemodynamic perturbations, therapeutic strategies, and eventual outcomes delineates the inadequacy of a one-size-fits-all CS management algorithm. In this narrative review, we discuss the pathophysiology of CS, the phenotypes and evolving definitions to risk stratify this disease state, the gamut of pharmacologic and device-based therapies currently available, and potential treatment protocols and systems-of-care strategies to enhance outcomes in this condition. Finally, we discuss opportunities for fertile investigation to address the prevailing knowledge gaps that persist in this disease.
Epidemiology of cardiogenic shock
CS remains the most common type of shock in patients admitted to the CICU in the contemporary era (15, 16). Historically, CS was seen as a primary disorder caused by LV dysfunction resulting from AMI. However, in recent years, acute decompensation of chronic HF has been recognized as the most common underlying etiology, contributing up to >50% of the admissions for CS (15). Numerous single and multi-center studies have reported the increasing prevalence of non-AMI CS (15–19). This paradigm shift may be attributed to increased use of preventive therapies and the concomitant decline in AMI incidence, early revascularization strategies in AMI patients leading to decreased incidence of resultant CS, and improved AMI survival in patients sustaining significant irreversible ischemia, resulting in more survivors with chronic HF due to LV dysfunction (20, 21). Patients are now living longer because of declining mortality from AMI-CS (18, 19). Among 1,254,358 CS admissions from the National Inpatient Sample (NIS) from 2004 to 2018, there was a more than threefold increase in the number of hospitalizations accompanied by a substantial 25% reduction in in-hospital mortality (from 49% to 37%) during the study period (22).
Healthcare costs have burgeoned revealing the inexorable financial toll of CS on both individual patients and healthcare systems. In one retrospective observational study of the NIS population from 2000 to 2014, Vallabhajosyula et al. reported a median cost of hospitalization of $80,346 for AMI-CS and $183,767 for CS-associated multiorgan failure hospitalizations (23). A single-center retrospective study of 230 patients reported the cost of AMI-CS hospitalization was more than five times that of AMI without CS, mostly (80%) due to the use of invasive procedures and device usage in the ICU (24). The financial outlay extends beyond the index hospitalization phase, as higher CS—associated readmission rates and progressive disease result in costly follow-up care. Re-hospitalizations contribute to the already elevated cost burden, with 30-day readmission rates nearing 19% and a median cost of nearly $10,000 per readmission (25).
Pathophysiology of cardiogenic shock
The pathophysiology of CS is a complex and vicious cycle often culminating in multiorgan failure and death. Initiated by a progressive impairment in ventricular contractility, CS leads to a critical reduction in mean arterial pressure (MAP) and cardiac output (CO), resulting in systemic hypoperfusion and decreased coronary perfusion pressure with compensatory activation of baroreceptors and chemoreceptors in an attempt to maintain hemodynamics and perfusion (1, 26, 27). Arterial- and veno-constriction occur as a result of baroreceptor and chemoreceptor activation, causing an increase in vascular resistance with blood distribution away from splanchnic circulation and elevating pulmonary venous and central venous pressure (CVP); these mechanisms result in multiorgan congestion, often exacerbating preexisting volume overload seen in patients with HF, and further compromise end-organ perfusion represented by worsening lactic acidemia (28, 29). In response to tissue ischemia, a state of systemic inflammatory response syndrome (SIRS) ensues which leads to systemic vasodilation and inflammation in an already dysfunctional myocardium—propagating the progressive maladaptive spiral of CS (11, 30).
The two most commonly recognized etiologies of CS are AMI-CS and HF-CS (11). AMI-CS is typically associated with injury to >40% of the LV myocardium but can also be precipitated by mechanical complications such as ventricular septal defect and free wall or papillary muscle rupture (31). Analysis of pressure-volume loop curves in AMI-CS show a rightward and downward shift of the end-systolic pressure volume relationship suggesting a sudden reduction in LV contractility resulting in reduced stroke volume (SV), CO, and MAP and increases in pulmonary capillary wedge pressure (PCWP) and CVP (26, 32). These hemodynamic changes reflect the canonical clinical course for patients with AMI-CS, often beginning with hypotension from the acute ischemic insult leading to hypoperfusion and culminating with congestion. In contrast, HF-CS often follows a more indolent clinical course, usually presenting with congestion in acute on chronic HF-CS phenotypes leading to hypoperfusion and ending with systemic hypotension (11, 26).
Definitions and classifications of cardiogenic shock
Despite varying definitions in clinical trials, CS has been historically described as a state of systemic hypoperfusion due to impaired myocardial contractility, typically with associated hypotension (systolic blood pressure <90 mmHg for a prolonged duration usually extending >30 min) (2, 4, 9). Patients may also clinically present in a state of isolated hypoperfusion, in which they are normotensive due to compensatory system vasoconstriction, but may still have hemodynamic and biochemical evidence of malperfusion (33). This underrepresented and poorly studied patient population was first reported in the SHOCK Trial registry with a 43% in-hospital mortality (34). Recognizing the need to evolve from binary determination of CS, which does not adequately describe the spectrum and myriad of etiologies and phenotypes or the varied clinical presentations of shock, a multidisciplinary workgroup at the Society for Cardiovascular Angiography and Intervention (SCAI) established a five stage (A to E) classification system for CS in 2019, encompassing the full spectrum of the syndrome based on physical examination findings, laboratory markers, and invasive hemodynamics (35). The SCAI classification system has undergone retrospective and prospective validation in several single center and multicenter registries, with the most recent iteration published in 2022 emphasizing the presence of cardiac arrest with coma as an adverse effect modifier, dynamic baseline and maximum SCAI staging and serial re-staging, and treatment intensity to stratify risk (7, 36–38).
In the SCAI taxonomy, each stage of CS severity is defined by biochemical, physical exam, and hemodynamic findings with the development of tissue hypoperfusion, end-organ dysfunction, and the need for hemodynamic support heralding the transition from pre-shock (Stage B) to later stages (C-E) (35). In 2022, SCAI proposed the addition of a 3-axis model of CS evaluation and prognostication to SCAI staging, recognizing the dynamic nature of shock, and serving as a reminder to individualize patient care based on risk modifiers, clinical phenotype, and comorbidities (37).
More recently, the Cardiogenic Shock Working Group (CSWG) has proposed a pragmatic revision to the SCAI classification to allow for patient risk-stratification using consistent and uniform definitions (36). Utilizing data from 3,455 patients across 17 hospitals from 2016 to 2021, the SCAI-CSWG classification provides specific threshold values to define hypotension (SBP and MAP) and hypoperfusion [lactate, alanine transaminase (ALT), pH] across all stages, and incorporates other relevant variables including treatment intensity (number of vasoactive agents, inotropic therapy, and acute MCS devices) and out-of-hospital cardiac arrest (OHCA). In brief, under the newly refined classification, SCAI-CSWG Stage A is a broad representation of a myriad of hemodynamically stable patients with known cardiac diagnoses which place them at risk for CS. SCAI-CSWG Stage B patients are defined as having either isolated hypotension (SBP 60–90 mmHg or MAP 50–65 mmHg) or hypoperfusion (lactate 2–5 mmol/L or ALT 200–500 U/L) without the need for drug or device therapy. SCAI-CSWG Stage C represents the more “classic CS” patients with hypoperfusion (lactate 2–5 mmol/L or ALT 200–500 U/L) and hypotension (SBP 60–90 mmHg or MAP 50–65 mmHg) or requiring one drug or tMCS device. SCAI-CSWG Stage D patients represent those deteriorating despite initial therapies who remain hypotensive (SBP 60–90 mmHg or MAP 50–65 mmHg) with signs of worsening hypoperfusion (lactate 5–10 mmol/L or ALT >500 U/L) requiring 2–5 treatment-intensive drugs or tMCS devices or have persistent hemodynamic instability on one drug or device. Lastly, SCAI-CSWG Stage E, or extremis shock, represent patients with (1) refractory hypotension (SBP <60 mmHg or MAP <50 mmHg), (2) refractory hypoperfusion (lactate >10 mmol/L), (3) requiring >3 drugs or tMCS devices, or (4) suffering an OHCA with coma. One significant difference between the original proposed SCAI system and the new SCAI-CSWG nomenclature centers around the definition of Stage B (beginning) shock, the latter allowing for earlier recognition and more reliable capture of patients with normotensive hypoperfusion—a largely unrecognized entity increasingly identified as an independent risk factor for CS mortality (36). In this manner, the SCAI-CSWG classification provides a granular, uniform taxonomy for risk stratification to delineate the temporal progression of CS across SCAI Stages (36).
Invasive hemodynamic assessment may also be useful in the classification of CS. The original Diamond-Forrester nomenclature initially proposed binary classification of HF patients with respect to perfusion and congestion based on the initial cardiac index and pulmonary capillary wedge pressure (PCWP) assessments, respectively (39–41). Modern day hemodynamic profiling of CS has become more nuanced, further classifying CS with distinct congestive profiles including “LV-dominant”, “RV-dominant”, and “biventricular (BiV)” shock profiles (33). LV-dominant CS is characterized by reduced LV function with elevated PCWP and normal or reduced CVP, while RV-dominant CS is characterized by elevated CVP with relatively preserved LV function and normal to low PCWP and pulmonary artery pressure. Lastly, as the name suggests, BiV shock is characterized by reduced LV function with elevated right and left cardiac filling pressures (1, 35). A sub-study of SHOCK registry reported similar in-hospital mortality in patients with RV vs. LV shock (53% vs. 61%, p = 0.30) (42). However, recent literature suggests that BiV shock is not only the most commonly observed profile out of the three congestive entities but also is a significant independent predictor of mortality when compared to LV congestion profile [aOR 2.4 (95% CI 1.4–3.7)] or no congestion [aOR 2.1 (95% CI 1.1–4.0)], and not necessarily driven by RV predominant shock (43).
Acute myocardial infarction related cardiogenic shock (AMI-CS)
With an aging population and associated frailty syndromes, up to 10% of patients presenting with AMI are likely to not only have profound hemodynamic perturbations, but also complex coronary artery disease (CAD), to include multivessel disease, chronic total occlusions and calcified vasculature (2, 44, 45). In patients with pre-existing CAD, even small ischemic injuries can precipitate CS, jeopardizing up to 40% of myocardial mass (46). Initially coined a “downward spiral” by Hollenberg in 1999, the central pathophysiologic premise of AMI-CS is an ischemic insult from an acute coronary thrombosis resulting in regional myocardial necrosis, impaired cardiac output due to concomitant systolic and diastolic dysfunction, elevated intracardiac filling pressures and reflexive sympathetic activation resulting in systemic vasoconstriction and heightened afterload (47). The timing of CS onset following AMI is variable, with a post-hoc analysis of the SHOCK Trial registry reporting a median time from AMI symptoms to CS onset of 6 h, with the greatest delay in patients in which the left anterior descending artery was the culprit vessel (48). Up to 20% of patients with AMI-CS may also develop refractory and non-infectious SIRS, due to the upregulation of nitric oxide synthetase and associated cytokines (49). These patients are at increased risk for non-cardiac organ dysfunction as well as higher in-hospital and one-year mortality rates across the CS severity spectrum. Lastly, patients with AMI may develop CS due to mechanical complications, such as ventricular septal defects, papillary muscle rupture with consequent severe mitral regurgitation, and free wall rupture (50). The landmark SHOCK Trial fundamentally altered our understanding and treatment approach to AMI-CS after it demonstrated a 13% absolute reduction in all-cause mortality at one year in patients undergoing revascularization (10). The prognostic relevance of timely invasive reperfusion in this patient population was further reinforced by the findings of the Feedback Intervention and Treatment Times in ST-Elevation Myocardial Infarction (FITT-STEMI) trial which demonstrated every 10 min treatment delay was associated with 3 additional deaths per 100 patients with AMI-CS undergoing percutaneous coronary intervention (PCI) (51). The classic term “golden hour” was coined by R. Adams Cowley in 1975 where he stated, “the first hour after injury will largely determine a critically injured person’s chances for survival.” This “golden hour” in CS management must include prompt identification followed by timely revascularization, resuscitation, and admission to CICU for escalating levels of care as appropriate (52). While the term “door to balloon” (D2B) is pertinent for ST-elevation myocardial infarction (STEMI) cases, in the realm of CS, the analogous “shock to support” (S2S) and “shock to perfusion” (S2P) durations have yet to be established. These timelines reflect the window for restoring adequate blood pressure and preventing multiorgan failure, comparable to the urgency of re-establishing coronary blood flow in STEMI patients. Basir et al. demonstrated the impact of early intervention on associated survival rates. Initiating tMCS within 1.25 h of shock onset yielded a survival rate of 66% in contrast to 26% when tMCS was initiated beyond 4.25 h (53). Despite advances in interventional therapies and systems-of-care strategies for the treatment of STEMI, AMI-CS continues to pose a challenge to health systems and clinicians worldwide given its multiorgan system ramifications (54).
Emergency department care
Prompt recognition of CS by emergency medical services (EMS) personnel and emergency department providers is key to ensuring timely treatment of patients with AMI with circulatory collapse. Steps to expedite the care of AMI-CS include early 12-lead electrocardiogram acquisition, administration of vasopressors (preferably norepinephrine and avoidance of phenylephrine) to achieve MAP >65 mm Hg, mechanical ventilation, point-of-care echocardiography to assess for mechanical complications, and immediate transfer to a primary PCI-capable facility (55–58). While patients with SCAI Stages A or B AMI-CS may proceed directly to cardiac catheterization laboratories (CCL), those with SCAI stage C or D may first require adjunctive stabilization measures while also mitigating any significant delays to invasive reperfusion (59). For patients with SCAI stage E AMI-CS, particularly those without ST-segment elevation, and who may have had prolonged out-of-hospital cardiac arrest, refractory ventricular arrhythmias and severe lactic acidosis with unfavorable prognosis, careful multidisciplinary team review of expected prognosis and elucidation of prior patient wishes and goals of care is warranted, often followed by formal palliative care consultation (60, 61).
Best practices for vascular access
Transradial access has now been recognized as the default approach for coronary angiography and PCI for patients with acute and chronic coronary syndromes following clinical trial data demonstrating reductions in major bleeding and vascular complications as compared to the femoral approach (62–64). These findings have been similarly noted in patients with AMI-CS (65). It is recognized, however, that AMI-CS is an important predictor of transradial access failure, as these patients have systemic constriction and are often receiving vasoactive therapies. They may also concomitantly require large bore access to facilitate implantation of tMCS (66). Therefore, if radial access is challenging or tMCS is required, concerted efforts should be made to employ safe femoral access using contemporary multimodality imaging techniques (67). The core elements of “vascular safety bundles” include routine ultrasound and fluoroscopic guided micropuncture access, pre-and post-procedure run-off angiography, and validated hemostatic protocols (68). In select patients with peripheral vascular disease or severely constricted lower extremity vessels, further measures may be undertaken to mitigate the risk for bleeding and vascular complications. These include upstream SHiP (Single access for High Risk PCI) in patients supported with Impella CP (Abiomed, Danvers, MA) and use of distal perfusion catheters in patients requiring downstream tMCS who are at risk for acute limb ischemia (68, 69).
Antithrombotic therapy
Multiple factors pose a challenge to achieving prompt, safe, and consistent antithrombotic effects in AMI-CS including but not limited to: (1) impaired absorption of oral P2Y12 inhibitors due to microcirculatory dysfunction and opioid induced enteral dysmotility; (2) platelet dysfunction due to hypothermia during targeted temperature control (TTC), microcirculatory thrombosis, myeloid dysfunction and acute renal failure; (3) impaired cytochrome P450-dependent activation of clopidogrel due to hepatosplanchnic malperfusion; and (4) bleeding and vascular complications due to large bore access (59). To date, recommendations for antiplatelet and antithrombotic treatments in AMI-CS have been extrapolated from patients with hemodynamically stable acute coronary syndrome, given limited efficacy data in CS (70). Given the high burden of hepatic and renal failure in CS with associated risk for inconsistent pharmacokinetics and pharmacodynamics, the AHA Position Statement on CS and European guidelines recommend the use of unfractionated heparin as the anticoagulant of choice due to its rapid offset and reversibility (1, 71).
Dual antiplatelet therapy (DAPT) with aspirin and oral P2Y12 inhibition is the mainstay of contemporary antiplatelet therapy in patients with AMI. The newer generation P2Y12 inhibitors prasugrel and ticagrelor, in crushed formulations, are generally the preferred oral agents because of their rapid onset of action and favorable pharmacodynamics compared to clopidogrel (72–75). In circumstances where oral bioavailability may be limited, use of the intravenous P2Y12 inhibitor cangrelor to bridge the gap in platelet inhibition may be warranted. With the ability to achieve steady state concentration within 2 min of infusion and a half-life of less than 6 min, cangrelor has been studied in cardiac arrest patients and has been demonstrated to impart more consistent platelet inhibition without increased risk for bleeding compared to oral agents (76). The Dual Antiplatelet Therapy for Shock Patients with Acute Myocardial Infarction trial (DAPT-SHOCK-AMI) (ClinicalTrial.gov: NCR03551964) is an ongoing multicenter randomized study comparing the primary clinical and laboratory endpoints of 30-day death/myocardial infarction/stroke and ADP-induced platelet aggregation between cangrelor and ticagrelor in AMI-CS. There is limited data regarding the clinical utility of glycoprotein IIb/IIIa inhibitors in AMI-CS, with concurrent class IIa recommendation in select non-CS AMI patients with high thrombus burden, no-reflow phenomenon or abrupt periprocedural vessel closure (63, 77).
Revascularization strategy
Up to 80% of patients with AMI-CS will have multivessel CAD, an independent predictor of morbidity and mortality in this patient population (78). Despite clinical precedent favoring coronary artery bypass grafting (CABG) in patients with ischemic left ventricular dysfunction, less than 7% of shock patients undergo surgical revascularization given their elevated risk for perioperative morbidity and mortality (21, 79). Notwithstanding the merits of complete revascularization in patients with hemodynamically stable acute coronary syndromes, gaps remain in knowledge regarding the extent of revascularization strategies that most benefit patients with AMI-CS (80). To date, only one large, multicenter randomized clinical trial has addressed this issue. The Culprit Lesion Only PCI vs. Multi-vessel PCI in Cardiogenic Shock (CULPRIT-SHOCK) trial demonstrated a 17% absolute reduction in the primary endpoint of 30-day death or renal replacement therapy with culprit-vessel PCI (2). Similar findings were noted at one year, with the caveat that the culprit revascularization cohort had higher rates of heart failure rehospitalization and repeat revascularization during this intermediate time period (81). The applicability of this study’s findings to real world practice has been challenged, as fewer than one-third of all patients had tMCS and nearly one-quarter of the multivessel cohort also underwent PCI of coronary chronic total occlusions, a practice that has not been demonstrated to improve cardiac function in non-CS patient with acute coronary syndrome (82). A recent sub-study of the National Cardiogenic Shock Initiative (NCSI), for instance, showed no differences in in-hospital mortality, acute kidney injury and length of stay in patients with AMI-CS undergoing multivessel PCI when Impella was implanted prior to revascularization (83). The US and European guidelines have nevertheless downgraded the practice of ad-hoc multivessel PCI in AMI-CS to a Class III recommendation based on the CULPRIT-SHOCK findings (63, 64). Similarly, there is a paucity of data regarding coronary stent platforms in AMI-CS (84). However, iterative advances in drug elution pharmacokinetics and emerging data suggest ischemic equipoise with attenuated bleeding risk in select high-risk patients undergoing PCI; drug-eluting stents are preferred over bare-metal stents in patients undergoing PCI (85).
Heart failure related cardiogenic shock (HF-CS)
In the last decade, HF-CS has been recognized as a distinct etiology which varies from AMI-CS not only in terms of pathophysiology and clinical presentation but also with respect to acute management and long-term prognosis (17). The pathophysiology of HF—CS also varies depending on de novo vs. acute on chronic HF-CS subtypes. Chronic HF primarily manifests as congestion due to cardiac dysfunction involving increased systemic vascular resistance and redistribution of blood from the splanchnic circulation as previously discussed. These changes, including venoconstriction and elevated CVP, contribute to organ congestion, impaired renal function, and hepatic dysfunction (26). Over time, cardiovascular and muti-organ adaptations to these derangements allow patients with chronic HF to tolerate conditions that would be critically dangerous if they occurred suddenly (86). When ventricular function is severely impaired, chronic HF progresses to HF-CS, resulting in worsening hypoperfusion and subsequent acute on chronic hepatic and renal injury, lactic acidosis, reduced coronary perfusion, and further activation of baroreceptors and chemoreceptors. These factors create a vicious cycle that worsens cardiac function, and the body enters a state of systemic inflammatory response syndrome culminating in multiorgan failure and death.
Initial assessment of HF-CS should include identifying the underlying etiology of HF-CS, the severity of CS using the SCAI stage definitions, and determining the hemodynamic congestive profile. Based on the recent CSWG observational data, 90% of the patients with SCAI B deteriorated to a higher SCAI stage (i.e., C/D/E) during the course of their index hospitalization; the mean time to achieving the maximum SCAI stage was 52 h (36). Hence, early institution of PA catheter guided hemodynamic management may be useful in identifying worsening HF-CS patients sooner and potentially lead to improved outcomes (87, 88). Patients with worsening hypotension and hypoperfusion with elevated lactate levels and evidence of end-organ dysfunction should be transferred to dedicated AHA Level 1 cardiac ICUs with invasive hemodynamic monitoring to reduce the risk of progression to hemometabolic HF-CS. Zweck and colleagues described this phenotype of HF-CS patients with near complete loss of compensatory reflex mechanism to maintain CO combined with multiorgan failure as hemo-metabolic HF-CS, and this subset of patients carry the highest risk of mortality among various HF-CS phenotypes (89).
Elevated filling pressures have been recognized as a strong predictor of adverse outcomes as opposed to CO alone in the advanced HF population (90). Co-administering loop diuretics with thiazides and thiazide-like diuretics (distal sodium reabsorption blockade) or with acetazolamide (proximal sodium reabsorption blockade) can overcome diuretic resistance via sequential nephron blockade and help attain successful decongestion (euvolemia) as shown in the ADVOR (Acetazolamide in Acute Decompensated Heart Failure with Volume Overload) trial, albeit in HF patients without CS (91).
For patients experiencing clinical deterioration and failing to meet hemodynamic goals despite initial therapeutic interventions, a selective and tailored approach to MCS device selection is recommended based on the severity of HF-CS with the goal of achieving hemodynamic unloading and restoring systemic perfusion (59). The application of MCS should be contextualized within a broader strategy aimed at either bridging the patient to advanced therapies or facilitating myocardial recovery. However, it is imperative to exercise caution when considering MCS use if exit strategies are not available; palliative care consultation should be considered if not concurrently pursued. A detailed review of temporary MCS, including IABP, Impella CP and Impella 5.5, and VA ECMO is provided in the sections below.
CICU management of cardiogenic shock
Shock teams and regionalized systems of care for CS
Team-based interventions have been shown to expedite care and improve outcomes for a number of high-mortality conditions, such as trauma, cardiac arrest, sepsis and stroke (92–95). The initial experience and feasibility of a team-based approach to CS was first described at the Mayo Clinic in Arizona, where mobile ECMO teams consisting of a cardiac surgeon, perfusionists and critical care nurses were deployed to the community to rapidly resuscitate and stabilize patients in refractory circulatory collapse with VA-ECMO and to transfer them back to local tertiary care center for follow-on care (96). These patients fared better than those stabilized at the community hospital, with survival to discharge of 56% vs. 30%, respectively (96). Given these initial favorable findings and recognizing the time-sensitive clinical nature of CS, researchers issued a clarion call to action in 2015 for centers to employ multidisciplinary “shock teams” in the care of patients with refractory hemodynamic compromise (97). Since then, several dedicated single center shock registries have published their findings following implementation of institutional shock teams, demonstrating associated improvements in short-term outcomes across the severity spectrum and phenoprofiles of CS (Table 1) (11–14). Clinical researchers at Inova Health System were among the first in the country to adopt this care paradigm for patients with both AMI-CS and HF-CS, demonstrating a significant associated improvement in 30-day survival (77% in 2018 vs. 47% in 2016; p < 0.001) following implementation of a one-call shock team activation, hemodynamically driven protocols for drug and device-based selection, and institutional best practices around vascular access and closure (11). The Critical Care Cardiology Trials Network (CCCTN), a multicenter collaborative of North American Level 1 CICUs, similarly showed favorable outcomes in 10 out of 24 sites with standardized team-based approached to CS, with significant reductions in CICU mortality (23% vs. 29%; aOR 0.72; 95% CI: 0.55–0.94; p = 0.016) and enhanced utilization of PACs and advanced MCS compared to centers without dedicated shock teams (98). Based on the amalgam of this observational data, the 2022 AHA/ACC/HFSA HF guidelines have awarded a class IIa recommendation for the utilization of multidisciplinary shock teams in the triage and follow-up care of patients with CS (99).
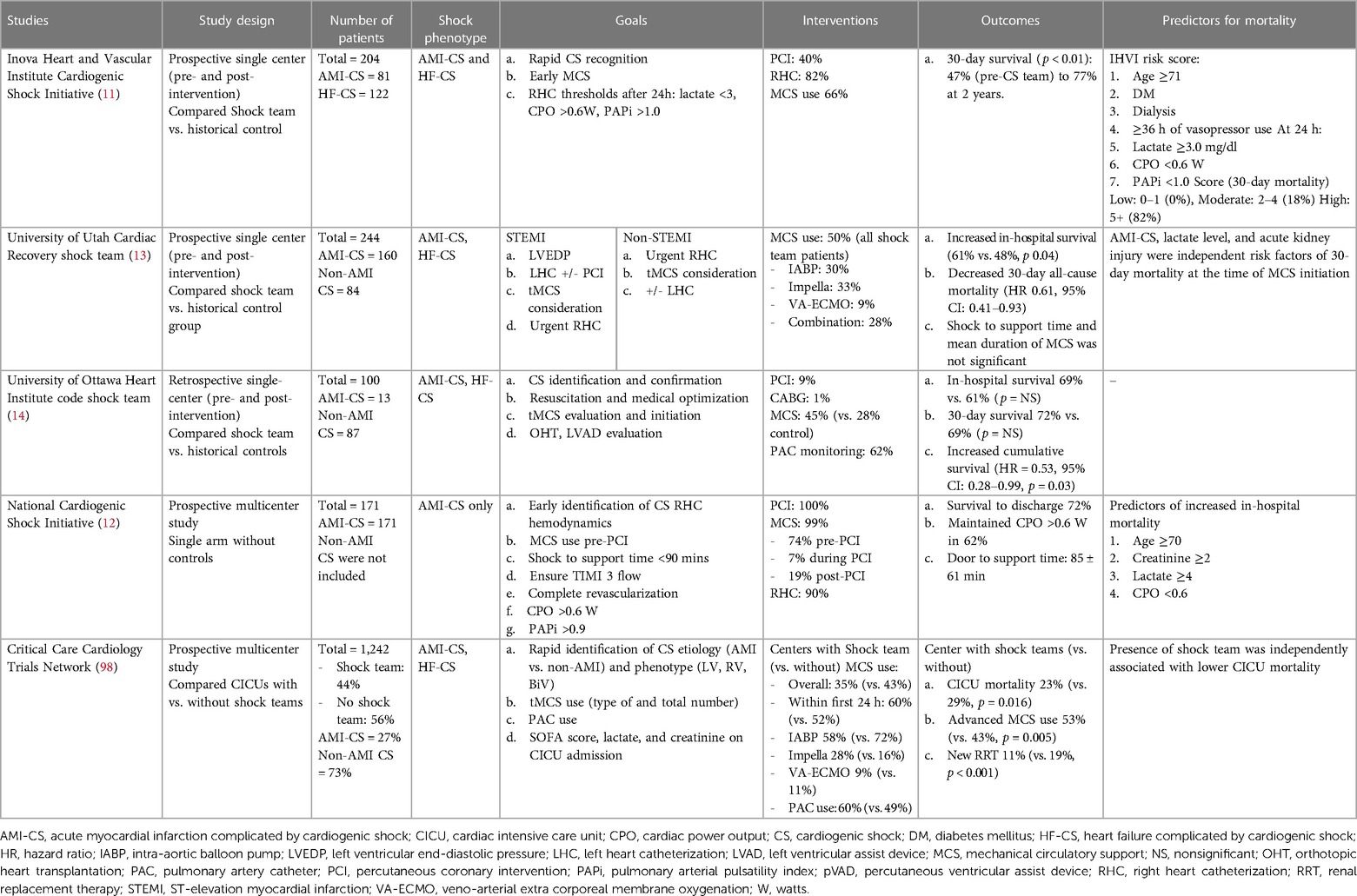
Table 1. Clinical studies evaluating outcomes in cardiogenic shock after implementation of standardized team-based approach.
Addressing regional disparities in management and outcomes remains a major knowledge gap in CS care (59, 100). The 2017 AHA scientific statement on CS endorsed a systems of care approach to management, through the development of regionalized shock networks promoting interhospital collaboration and centralized care at regional destination centers using time-sensitive transfer protocols (1). A tiered-based approach to CS care has been correspondingly described to categorize centers based on the local levels of interventional, surgical, and critical care expertise (55). In these proposed networks, level 1 or “hub” institutions are tertiary or quaternary care centers with 24/7 PCI capabilities, cardiothoracic surgery, and advanced MCS availability. These centers employ “high-intensity” CICUs which are often co-managed by critical care cardiologists and intensivists and provide multiorgan system services and durable cardiac replacement therapies. Level 2 and 3 hospitals, referred to as “spoke” centers, have more limited resources, with the former capable of providing primary PCI services and limited temporary MCS such as IABP, and the latter equipped with emergency medical departments, general medical intensive care units and advanced cardiovascular life support capabilities (Figure 1) (55). Clinical investigators at Inova Health System were among the first in the country to implement and publish an integrated shock network with 34 partnering spoke shock care centers in the Washington DC-Maryland-Virginia region. Through collaboration and integration of care protocols between providers at the spoke institutions and the local shock team at the level 1 center, no significant differences were noted between patients with CS, irrespective of whether they were initially triaged at a Level 1 or 2/3 center, with respect to in-hospital mortality, 30-day mortality, major bleeding complications, stroke, and 30-day major adverse cardiovascular and cerebrovascular complications (101). The 2022 AHA/ACC/HFSA HF guidelines provide a class IIb recommendation for the early triage of patients with CS who are refractory to initial stabilizing measures to level 1 centers with advanced MCS and critical care expertise (99). As we continue to learn about the advancements in the field of cardiogenic shock and its implications on patient management with use of tMCS devices, the original protocol for management of CS put forth by our task force at Inova has undergone several revisions and the most recent formulation stratified by AMI-CS and HF-CS are presented in Figures 2A,B, respectively. The CS team activation and coordination protocols are presented in Supplementary Figures 1 and 2.
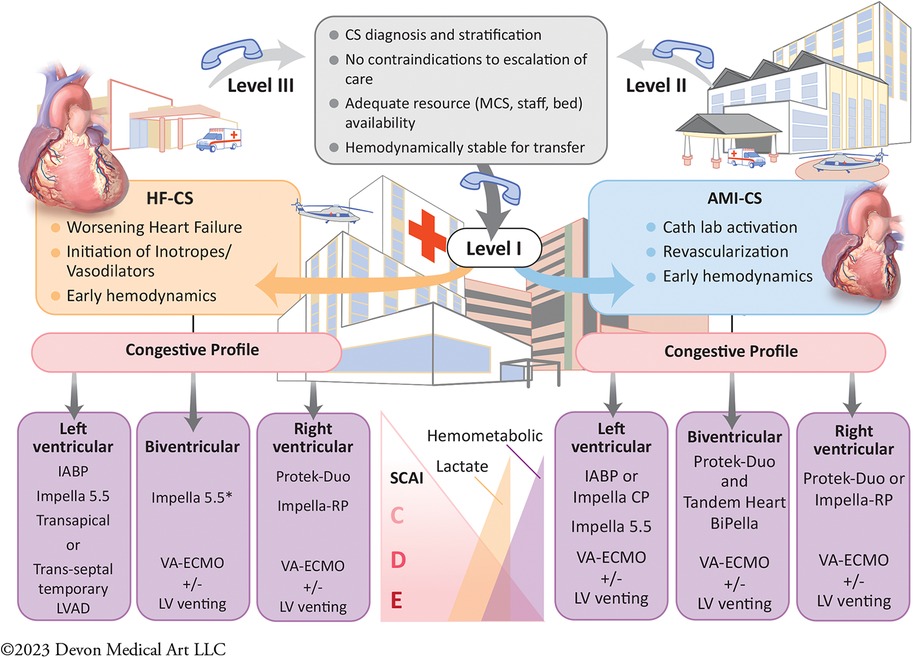
Figure 1. Central illustration. Proposed algorithm of cardiogenic shock management within a regionalized shock network by a multidisciplinary shock team. A contemporary systems of care approach for cardiogenic shock (CS) management by a multidisciplinary team in a “hub and spoke” model. This allows for timely diagnosis with early comprehensive invasive hemodynamic assessment. Early, selective, and tailored mechanical circulatory support (MCS) based on CS phenotype and congestive profiles is crucial for CS management in the modern era. This is also predicated on expedited transfer to the level 1 CS centers of excellence for team-based and comprehensive multiorgan system care. AHF, Advance Heart Failure; AMI, Acute Myocardial Infarction; CS, Cardiogenic Shock; IABP, Intra-Aortic Balloon Pump; LV, Left ventricle; LVAD, Left Ventricular Assist Device; MCS, Mechanical Circulatory Support device, SCAI, Society for Cardiovascular Angiography and Interventions; VA-ECMO, Veno-Arterial Extra Corporeal Membrane Oxygenation.
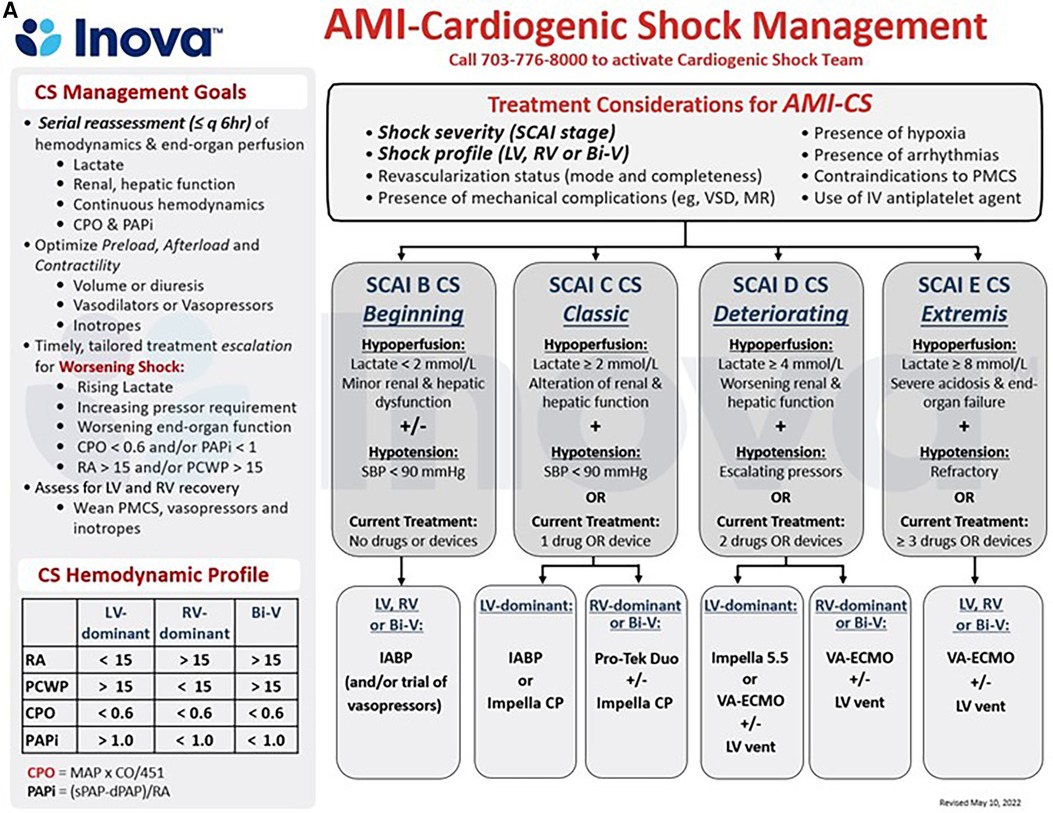
Figure 2. (A,B) schematic representation of the care pathways in the upstream and critical care management of patients with acute myocardial infarction (AMI, 4a) and acute decompensated heart failure (HF, 4b) cardiogenic shock (CS) at the INOVA schar heart and vascular institute. BiV, Biventricular; CPO, Cardiac Power Output = [mean arterial pressure x cardiac output]/451; PAPi, Pulmonary Artery Pulsatility Index = [systolic pulmonary arterial pressure—diastolic pulmonary arterial pressure]/right atrial pressure; PMCS, percutaneous Mechanical Circulatory Support; SBP, Systolic Blood Pressure, other abbreviations as in Figures 1, 2.
Vasopressors and inotropes
The acute management of patients with CS requires successful augmentation of cardiac output, typically with intravenous vasopressors and inotropes (102). These agents may enhance myocardial contractility by targeting the system of myocardial calcium fluxes, modulating adrenergic receptors or through phosphodiesterase inhibition (102). Although norepinephrine is often recommended as the first-line agent of choice for treatment of AMI-CS, data from clinical trials is limited. A subgroup analysis of the SOAP-II trial showed that norepinephrine appeared superior to dopamine based on fewer deaths in the CS subgroup (103). Epinephrine was associated with higher incidence of refractory AMI-CS than norepinephrine in the OptimaCC Study (56). Milrinone was associated with greater rates of sustained hypotension and atrial arrhythmias without any difference in cardiovascular-related hospital days as compared to placebo in the OPTIME-CHF Study (104). The DOREMI (Dobutamine Compared with Milrinone) trial, a double-blind randomized clinical study comparing dobutamine with milrinone in patients with AMI and HF-related CS, did not find any differences with regard to the composite or individual end-points of in-hospital death, resuscitated cardiac arrest, non-fatal myocardial infarction, transient ischemic attack, stroke, renal replacement therapy or need for mechanical circulatory support or heart transplant between patients randomized to milrinone or dobutamine (6). Despite frequent and prolonged administration of these drugs in the treatment of patients with CS, they all may have deleterious effects on energetic efficiency, as they may further increase myocardial oxygen demand by way of refractory ischemia, arrhythmias, and vasodilatory hypotension (102). An observational analysis from the CSWG registry demonstrated that concatenation of vasoactive therapies, independent of tMCS, was associated with worse mortality. Given that multiple vasoactive and/inotropic agents are often used contemporaneously, concerted efforts should be made to properly assess the drug(s) of choice based on their effects on right and/or left ventricular congestive profiles and to minimize the total number and duration of administration of vasopressors and inotropes (59). Clinical guidelines currently endorse a Class I recommendation for the utilization of vasopressors and/or inotropes in CS in the 2022 AHA/ACC/HFSA Guidelines for Heart Failure (105).
Invasive hemodynamic monitoring
A growing body of evidence now supports routine early invasive hemodynamic assessment with PAC use for HF-CS despite initial preliminary studies failing to show benefit (11). The Evaluation Study of Congestive Heart Failure and Pulmonary Artery Catheterization Effectiveness (ESCAPE) trial, a randomized controlled trial (RCT) of 433 patients with symptomatic, recurrent HF without CS who were assigned to PAC-guided volume optimization or clinical assessment alone failed to demonstrate an impact of PAC use on primary composite endpoint of days alive and out of the hospital at 6 months (106). Most notably, however, the ESCAPE trial did not specifically evaluate patients with CS for whom inotropic and vasopressor therapy was frequently used or for assessment of potential candidacy for MCS (107). The eligibility criteria in the ESCAPE trial also excluded patients with severe HF decompensation, significant renal dysfunction (creatinine >3.5 mg/dl), prior use of inotropic therapy, and those with pulmonary hypertension—a subset of patients who ultimately may have benefitted most from PAC-guided therapy (107). Nevertheless, the overall use of PAC among patients with HF continued to decline from 2004 to 2014 including in patients with CS (88).
Subsequently, given the controversy and limitations of ESCAPE, there have been several registries and emerging studies advocating for the incorporation of early invasive hemodynamic monitoring as a standard of care in contemporary CS management, as PAC use may lead to earlier and more accurate identification of CS phenotyping to tailor specific medical and device-based therapies (11). A contemporary analysis of 1,531,878 CS admissions from the NIS database from 2004 to 2018 observed significant regional variations in the employment of invasive hemodynamics, with the highest rate of PAC use in the Northeast United States and in urban teaching hospitals (22). Based on these findings and subsequent negative studies, societal guidelines have provided only limited recommendations regarding the use of PACs in CS, with the 2022 AHA/ACC/HFSA guidelines for the management of HF providing a Class IIa recommendation for invasive hemodynamics only in select patients with HF and worsening symptoms or signs of end organ perfusion despite optimal medical therapy and a Class IIb recommendation for its use in CS (99).
There are several potential theoretical advantages to the routine use of upfront, routine invasive hemodynamics in CS which have been postulated: (1) Timely diagnosis of CS, including assessment of RV and LV congestive profiles and elucidation of SCAI classification; (2) Optimal use of hemodynamically tailored tMCS with serial follow-up assessments to inform decision making around device escalation or de-escalation; and (3) Correlation of cardiac performance with congestion to further inform risk stratification of patients with CS and multiorgan system dysfunction (33, 36). There is an emerging body of evidence suggesting that routine PAC use may be associated with improved survival in CS, particularly in patients with tMCS (11, 12, 88, 108, 109). These observational contemporary studies have demonstrated reductions in CICU- and in-hospital mortality in patients with AMI-CS and HF-CS across all SCAI stages, particularly when complete PAC data was obtained within 6 h of hospital admission and prior to tMCS implantation (88, 108). Furthermore, routine invasive hemodynamic monitoring has been a core element in contemporary CS treatment algorithms (33). For instance, the National Cardiogenic Shock Initiative (NCIS), a single-arm, prospective, multicenter registry of AMI-CS in the United States, found that using a standardized protocol emphasizing routine invasive hemodynamic monitoring and rapid initiation of tMCS resulted in a 72% survival to discharge rate for patients presenting with AMI-CS (12). This was indeed achieved through an aggressive utilization of PAC (performed in 92% cases) and tMCS implanted in 74% prior to PCI. Likewise, recent outcome data analysis from the CSWG registry demonstrated that the mortality rates differed significantly between patient groups with no PAC profiling, incomplete PAC profiling, and complete PAC profiling (26). Patients in the complete PAC assessment group had the lowest in-hospital mortality rates across all SCAI stages [aOR 1.57 (95% CI 1.06–2.33), compared with no PAC assessment] (43). Furthermore, in an analysis of administrative data from the Nationwide Readmissions Database including over 230,000 hospitalizations for CS between 2016 and 2017, the use of PAC was associated with a significantly lower in-hospital mortality [aOR 0.69 (95% CI 0.66–0.72)] (110). More recent data from the CCCTN, a multicenter network of level I CICUs in the United States across 34 centers, again demonstrated that PAC use, even after adjustment for factors associated with their placement, was associated with lower mortality in all shock patients admitted to a CICU [OR 0.79 (95% CI 0.66–0.96); P = 0.017] (88). Currently, the latest European Society of Cardiology Guidelines for Acute and Chronic Heart Failure have recommended that invasive hemodynamic monitoring coupled with a standardized team-based approach may be associated with improved patient survival (111). An expert consensus statement from SCAI also stressed that defining CS phenotypes using invasive hemodynamics may help to guide therapy, particularly with respect to identifying patients who may require RV or BiV support (35).
Given that no RCTs have prospectively tested the utility of PAC use among patients with acute decompensated HF-CS (ADHF-CS), the Pulmonary Artery Catheter in Cardiogenic Shock (PACCS) trial (NCT05485376) is a registry-based trial designed by the CSWG and will test the hypothesis that early invasive hemodynamic assessment (within 6 h of randomization) and ongoing management with a PAC decreases the primary endpoint of in-hospital mortality compared to clinical management with delayed (beyond 48 h after randomization) or no PAC-guided assessment among patients with ADHF-CS (112). As a randomized, multicenter, adaptive design trial, PACCS intends to enroll 400 patients across several sites in the United States with a study duration of 4 years (112). Outside of hemodynamic monitoring in the inpatient setting, there remains a paucity of data testing the efficacy of remote hemodynamic monitoring in the outpatient setting to prevent adverse outcomes following CS hospitalization. The Hemodynamic monitoring to prevent Adverse events foLlowing cardiOgenic Shock (HALO Shock) trial (NCT04419480) is a prospective, unblinded study at the Inova Schar Heart and Vascular Institute that will test the hypothesis in a 1:1 randomized fashion that remote hemodynamic monitoring with CardioMEMS (Abbott) device implementation improves outpatient management including medication titration to prevent adverse outcomes following CS hospitalization (113). HALO Shock is a pilot study that has the potential to transform care for shock survivors with persistent congestion at high risk of subsequent morbidity and mortality following CS.
Temporary mechanical circulatory support devices
Temporary mechanical circulatory support (tMCS) devices are increasingly utilized in the management of CS worldwide. An analysis of 110,462 AMI-CS admissions from the NIS database from 2005 to 2014 demonstrated that tMCS was used in 55% of cases (114). Similar findings have been noted in European registries, yet with shifts toward greater employment of advanced tMCS platforms such as microaxial flow percutaneous ventricular assist devices (pVAD) and veno-arterial extracorporeal membrane oxygenation (VA-ECMO) (115). To date, RCTs evaluating pVAD and VA-ECMO in AMI-CS have not demonstrated an improvement in short-term outcomes compared to either medical therapy or conventional IABP counterpulsation (3–5). Recent large scale administrative claims data studying the use of pVADs in AMI-CS, in fact, suggest that routine employment of these devices in the management of CS patients may be associated not only with increased risk for short-term and one-year mortality, but also major bleeding complications, acute kidney injury, and stroke (66, 116, 117). As a result, the indications for tMCS in AMI-CS, timing of implantation, and how to best incorporate them into shock management protocols remains an evolving area of controversy, and clinical practice guidelines currently delineate the routine employment of these devices in AMI-CS care as a Class IIb (Level of Evidence: C) recommendation (44, 117). Mechanistically, tMCS devices may serve as bridging vehicles to myocardial recovery or cardiac replacement therapies by not only reducing intracardiac filling pressures and LV stroke work but also augmenting coronary and end-organ perfusion (59). Optimal tMCS device selection should be tailored to not only severity of the shock state, but also the respective congestive profiles and phenotypes, thus requiring a nuanced understanding of how each device platform can modulate right and left ventricular pressure-volume loop hemodynamics (26, 59). Given regional variations in the expertise and management of tMCS, centers caring for patients with these devices should employ standardized protocols not only around device insertion and removal, but also continuous monitoring in the CICU to mitigate the associated risks for bleeding, vascular complications, hemolysis, and afterload mismatch associated with these devices (68, 118). Ongoing trials evaluating the role of tMCS devices in CS are summarized in Table 2.
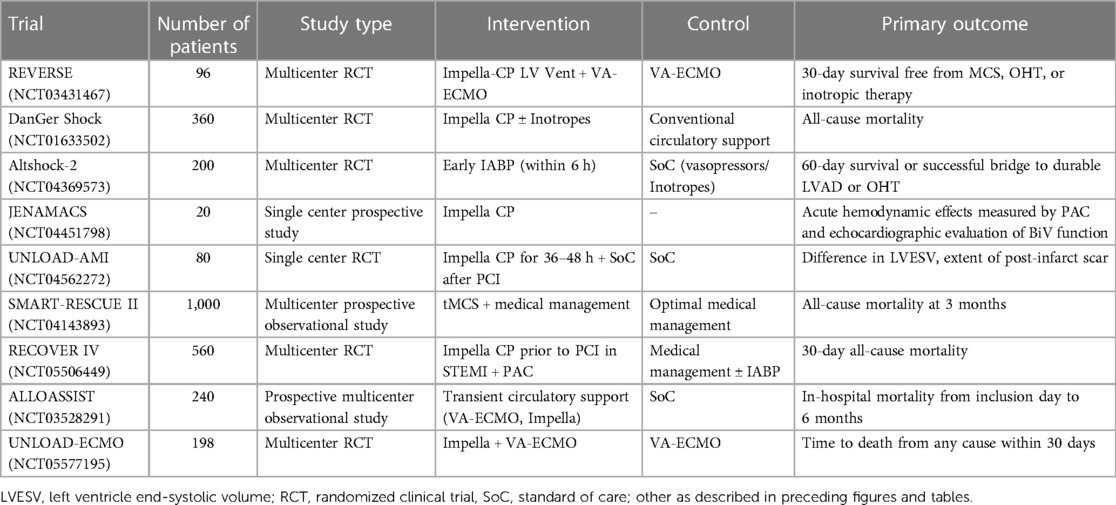
Table 2. Ongoing, select clinical trials assessing the role of mechanical circulatory support devices.
There is emerging data from several North American CS single-center registries that select utilization of tMCS devices based on time-sensitive protocols may be associated with improved survival (12, 119, 120). The 2022 AHA scientific statement on tMCS similarly endorsed an algorithmic approach to device selection and subsequent escalation and de-escalation of tMCS using a standardized framework which integrates timely, interdisciplinary input and collaboration based on serial invasive hemodynamics, clinical, and laboratory data (121). While early implantation of tMCS device to offset or reverse the ensuing end-organ dysfunction may seem attractive, the deleterious risks associated with these devices and their potential complications needs to be appreciated given the absence of any robust RCT supporting mortality benefit from instituting tMCS (Figure 3). In select patients with increasing number and doses of inotropes and/or vasopressors, one must consider escalation to tMCS strategy in order to minimize the risk of arrhythmias and increasing myocardial oxygen demand. An interdisciplinary shock team evaluation as soon as CS is identified is of utmost importance, so that appropriate and tailored MCS device can be deployed. Although no absolute level of lactate reliably differentiates between patients with and without poor prognosis, early lactate clearance within the first 6–8 h of CS onset and/or at 24 h has shown to be more prognostic in identifying treatment responders and associated with overall improved survival (32, 122). The International Society for Heart and Lung Transplantation, in collaboration with the Heart Failure Society of America, has published guidelines on employing tMCS in specific populations, including women, ACHD, the elderly and frail, as well as those who are obese. These guidelines address implementation timing, patient specific device selection, and emphasizes the importance of involving patients and their families in the decision-making process through the use of decision aids (123).
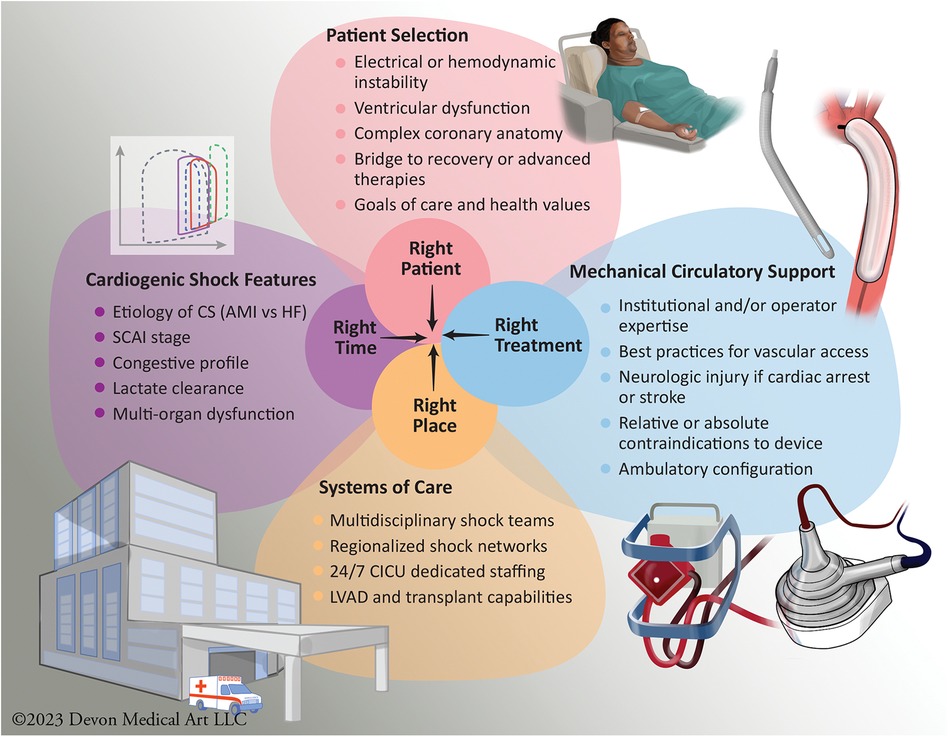
Figure 3. Optimizing patient-centric care: mechanical circulatory support considerations for appropriate use of selective and tailored approach to available devices. The figure illustrates the intricate process of achieving optimal patient-centered care in the context of MCS use. The achievement of the right patient, at the right time, with the appropriate MCS device, and in the right clinical setting who should be managed at an appropriate level of CS center in a regionalized shock network, is a complex endeavor influenced by a multitude of factors. AMI, Acute Myocardial Infarction; CICU, Cardiac Intensive Care Unit; CS, Cardiogenic Shock; HF, Heart Failure; LVAD, Left Ventricular Assist Device; SCAI, Society for Cardiovascular Angiography and Interventions.
Intra-aortic balloon pump
IABP plays a crucial role in cardiac support by employing the principle of counterpulsation. These medical devices consist of balloon-mounted catheters with a volume capacity ranging from 25 to 55 cc, strategically positioned in the descending aorta. The mechanism involves inflation during diastole, which enhances central aortic root diastolic pressure and coronary perfusion. During systole, the balloon deflates, creating a negative pressure zone, consequently reducing LV afterload. This leads to a decrease in LV cardiac work and myocardial oxygen consumption while simultaneously augmenting cardiac output by up to 1 L/min. The clinical efficacy of IABPs has been explored through randomized controlled studies, almost exclusively in cases of AMI-CS (124, 125). The IABP-SHOCK II trial randomized AMI-CS patients to IABP or standard therapy and found no significant difference in 30-day mortality, leading to the conclusion that routine IABP use did not improve survival in this patient population (124). In contrast, IABPs have been associated with improved outcomes in patients with HF-CS, albeit in nonrandomized studies, serving as a bridge to durable LVAD or orthotopic heart transplantation (HT) (126–129). Notably, there is some evidence to suggest the presence of IABP-responders and non-responders. IABP insertion has shown benefits in patients with non-ischemic cardiomyopathy and higher pulmonary artery pulsatility index (PAPi) scores, indicating its potential utility in appropriate patient selection for HF-CS (126). High SVR, low cardiac index at baseline, and diabetes mellitus have also been shown to be positive predictors of IABP response (130). The ongoing AltShock-2 trial (NCT04369573) aims to address this knowledge gap by prospectively comparing IABP to vasoactive therapy in HF-CS patients, with a primary endpoint of 60-day survival or successful bridge to OHT (131).
Percutaneous ventricular assist device (pVAD)
Impella pVADs, developed by Abiomed Inc. (Danvers, MA), are catheter-based microaxial ventricular assist devices that can deliver up to 5.5 liters of cardiac output by using the principle of an Archimedes screw, harnessing the impeller’s rotational kinetic energy to displace blood from LV to aorta, thereby reducing the LV preload and oxygen consumption while increasing mean arterial pressure and tissue perfusion (132). In the U.S., Impella devices have been FDA approved to provide temporary support for 5–7 days (or 30 days for Impella 5.5), regardless of heart rate or residual LV contractility. However, limited randomized data on the efficacy of Impella on survival in CS have led to lack of endorsement from professional society guidelines, potentially causing variations in device selection and use across centers. Studies like ISAR-SHOCK and IMPRESS-in-Severe-SHOCK have compared Impella to IABP in AMI-CS (4, 132). While these studies have shown improvements in cardiac index with Impella, especially when initiated before primary PCI, they have not demonstrated reduced 30-day mortality as compared to IABP. Additionally, a meta-analysis involving percutaneous MCS devices has reported no significant mortality difference between Impella and IABP (133). However, it highlighted a higher bleeding risk associated with tMCS (134). RECOVER IV is a prospective, multicenter, randomized controlled open-label, two-arm trial with an adaptive design evaluating whether early Impella support in STEMI patients with CS prior to percutaneous intervention as compared to a non-Impella based standard of care treatment strategy reduces all-cause mortality at 30 days. Emerging interest surrounds pre-procedural LV unloading to enhance coronary reperfusion and clinical outcomes, with ongoing randomized trials investigating this novel therapeutic approach (135, 136). Impella RP Flex is a newer device specifically designed for right ventricular (RV) support, with an inlet either in the inferior or superior vena cava and an outlet in the pulmonary artery. When combined with left-sided Impella pumps, comprehensive biventricular support may be provided although this strategy has not been formally tested in clinical trials. Impella RP, the immediate predecessor of the Impella RP Flex, has shown safety and immediate hemodynamic benefits in the RECOVER RIGHT trial (137). In summary, the Impella platform of devices offer significant hemodynamic support in CS, particularly in AMI-CS cases but without any randomized data evidence of survival benefit to date. Thus, there is a need for more comprehensive clinical data to identify the appropriate patient population for timely device selection to optimize outcomes and inform clinical practice guidelines.
TandemHeart (LivaNova, Houston, TX) is percutaneously placed via a transseptal puncture for left sided support (TH-LVAD) and with ProtekDuo, supports the right side as well (TH-RVAD) (138, 139). TH can be utilized in cases such as LV thrombus and/or significant aortic regurgitation where transvalvular options are limited. By splicing an oxygenator into the circuit, it facilitates hemodynamic stabilization, perfusion, and decongestion with oxygenation analogously to VA-ECMO. Given the transseptal approach, the placement of TH-LVAD is complicated in the emergent setting and no mortality benefit has been proven for these devices yet (140, 141).
Extracorporeal membrane oxygenation (ECMO)
ECMO is commonly used as the first line tMCS strategy in patients with acute severe or refractory cardiac and respiratory failure. The configuration of ECMO determines whether it is providing gas exchange (veno-venous or VV-ECMO) or gas exchange with hemodynamic support (VA-ECMO) (142, 143). ECMO support can be inserted percutaneously via a large bore venous cannula inserted in a central vein which drains the de-oxygenated blood, cycles through an external oxygenator and a blood pump (centrifugal or rotational), and returns the oxygenated blood to a central artery via large bore arterial cannula. To prevent limb ischemia, a distal perfusion cannula is often used, usually placed through the superficial femoral artery and sometimes through the posterior tibial artery in a retrograde fashion. Typical access sites for VA ECMO cannulation include femoral or axillary arteries and jugular or subclavian veins (144–146). A key limitation to prolonged support with VA-ECMO is increased LV afterload which will be discussed in detail in the subsequent section. Systemic anticoagulation is needed because of the large cannula size, which exposes patients to the possibility of multiple complications; overall ECMO-associated morbidity and mortality are high. A 2014 meta-analysis of almost 1,900 patients reported up to 20% rate of lower extremity ischemia, up to 50% acute renal failure needing dialysis, and up to 40% rate of major bleeding in patients supported with VA-ECMO for CS and cardiac arrest (147). The ECMO-CS trial was recently published, comparing early VA-ECMO vs. salvage VA-ECMO in 122 patients with CS SCAI stage D or E (5). The trial enrolled around two-thirds AMI-CS patients excluding patients who were comatose after cardiac arrest. Unfortunately, the trial failed to demonstrate any significant difference between the two groups in the 30-day composite primary end point of death, resuscitated cardiac arrest, or escalation of MCS (63.8% vs. 71.2% respectively; hazard ratio, 0.72; 95% CI: 0.46–1.12); 30-day mortality did not differ (50.0% vs. 47.5%) (5). The trial had comparable but high rates of serious adverse events (60.3% vs. 61.0%). In addition, the multicenter, international EURO SHOCK trial aimed to determine if early use of VA-ECMO within 6 h (± only IABP for unloading) improves 30-day mortality in patients with persistent CS 30 min after primary PCI as compared to standard of care therapy (148). The trial aimed to enroll 428 patients but was able to enlist only 35 (13.25%) patients due to recruitment challenges amidst the coronavirus disease (COVID-19) pandemic. Patients with AMI complications such as ventricular septal rupture, ischemic mitral regurgitation, or LV free-wall rupture were excluded. The trial failed to demonstrate a significant difference in all-cause mortality at 30 days between the two groups (43.8% vs. 61.1%, HR = 0.56, 95% CI: 0.21–1.45, p = 0.22). The secondary outcome of major bleeding and vascular complications were numerically higher in the VA-ECMO group. Mortality at 12-months was numerically lower but statistically not significant in the VA-ECMO group (51.8% vs. 81.5%, HR = 0.52, 95% CI = 0.21–1.26, p = 0.14) (148). Given the low recruitment and limited patient enrollment, no definitive conclusions can be drawn from this trial. Most recently, the ECLS-SHOCK (ExtraCorporeal Life Support for Acute Myocardial Infarction complicated by Cardiogenic SHOCK) trial enrolled 420 patients with AMI-CS and randomized to ECLS or usual care group and reported no significant 30-day mortality difference (47.8% vs. 49.0%, p = 0.81). ECLS was associated with increased incidence of moderate or severe bleeding (23.4% vs. 9.6%, p < 0.05) (8). In light of the aforementioned studies, the optimal role of VA ECMO in AMI-CS remains to be determined.
LV venting
During peripheral VA-ECMO, retrograde flow generated towards the aortic valve by the arterial cannula increases LV afterload (149). The increased afterload produces LV distension increasing wall stress and oxygen demand of the myocardium (149, 150). Elevated LV end-diastolic pressure, worsens subendocardial ischemia thereby further reducing LV systolic function (Figure 4). In AMI-CS, with competent mitral valve and non-compliant LV, the risk for LV distension is higher as compared to patients with HF-CS (i.e., in chronic decompensated HF, the LV is often dilated with mitral annular dilatation), in which the mitral valve serves as a potential “pop-off” valve and leads to acute pulmonary edema. In AMI-CS cases supported with VA-ECMO recovery can be delayed and often difficult owing to the above mechanisms. An artificially high afterload results in the inability of the aortic valve to open, LV blood stasis, and thrombus formation with the potential risk of embolization to systemic circulation. The effect of increasing LV end-diastolic pressure results in elevated LA pressures, which in turn increases pulmonary venous pressure, resulting in pulmonary edema, hemorrhage, and eventually systemic hypoxia (151). Multiple strategies for LV venting and/or unloading can be used and each have their own advantages and disadvantages (Table 3). Common practices include reducing the ECMO flow, increasing inotropic therapy, or left ventricular venting via a pVAD insertion, left atrial septostomy, and surgical venting (Table 3).
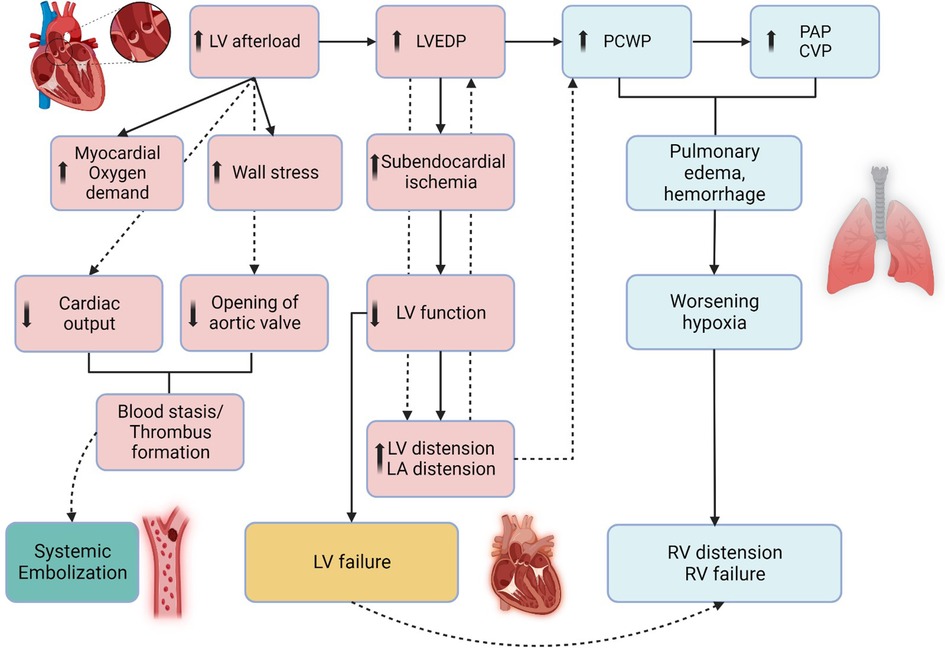
Figure 4. Pathophysiology of left ventricular distension during veno-arterial extracorporeal membrane oxygenation. The figure illustrates the pathophysiology of left ventricle (LV) distension which occurs during VA-ECMO as the outflow cannula generates retrograde flow towards the aortic valve, resulting in increased afterload. This results in high LV end-diastolic pressure (LVEDP) and increased LV end-diastolic volume (LVEDV), resulting in subendocardial ischemia, hindering LV recovery. In some cases, mitral valve may act as “pop-off” valve for the LV but leads to pulmonary edema, resulting in increased PCWP, CVP, and subsequently RV distension and RV failure. LV, CVP, Central Venous Pressure; EDP, End-Diastolic Pressure; LV, Left Ventricle; PAP, Pulmonary Artery Pressure; PCWP, Pulmonary Capillary Wedge Pressure, RV, Right Ventricle. Created with BioRender.com.
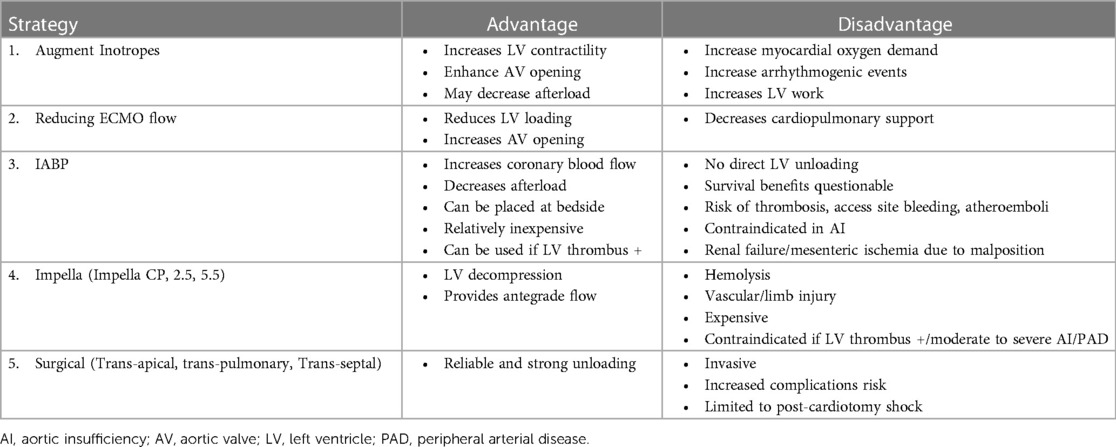
Table 3. Strategies for left ventricular unloading during veno-arterial extracorporeal membrane oxygenation.
Peripheral cannulation coupled with an LV vent using either an intra-aortic balloon pump for passive venting or Impella CP/5.5 for active unloading is a frequently employed strategy. Furthermore, surgical assist devices such as the CentriMag pump can provide full univentricular or biventricular support, approximately 5 liters per minute, for extended periods. This approach involves venting the left ventricle through an inflow cannula in the left atrium or the LV apex with an outflow cannula into the aorta (152). For right ventricular support, an inflow cannula is placed in the right atrium with an outflow cannula in the pulmonary artery. This strategy is typically reserved for patients inadequately supported by percutaneous assist devices who are awaiting heart transplantation, anticipating prolonged wait times.
Anticoagulation and anti-thrombotic therapy in temporary mechanical circulatory support devices
The use of tMCS is associated with both bleeding and thrombotic complications, which may occur simultaneously in some patients, which may pose a challenge in finding an optimal anticoagulation and anti-thrombotic regimen. In devices such as VA-ECMO, IABP, and Impella (Abiomed Inc), use of anticoagulation is mandatory to counteract activation of the coagulation cascade caused by shear force stress and the presence of a foreign material in the body, in order to prevent device-related thrombosis and embolization. This is mostly based on pathophysiological considerations as opposed to randomized clinical trial evidence, because such trials are lacking. While on the other hand, recent large retrospective studies including patients supported by microaxial flow pumps, showed higher mortality as compared to those supported by IABP, mainly due to higher rates of major bleeding complications (66, 116). Apart form use of anticoagulation, the effect of DAPT in patients with acute coronary syndrome post PCI, or those who develop acquire von Willebrand syndrome, or vascular access-site complications, the risk of bleeding increases even further. In general, unfractionated heparin (UFH) is the anticoagulant agent of choice in patients on pVAD (153, 154). In subset of patients who develop heparin-induced thrombocytopenia (HIT), use of direct thrombin inhibitors (DTIs), such as bivalirudin or argatroban, is recommended (155, 156). When used, UFH has marked variability in its anticoagulation effect in different patients and monitoring can be challenging. Also, due to presence of comorbidities and multi-organ dysfunction in these critically ill patients, aPTT may not provide an accurate assessment of its anticoagulant effect. Therefore, parallel monitoring of anti-Xa and aPTT is considered to be superior to aPTT alone because it is not affected by other confounding factors, and has been supported by studies that show mortality increases when aPTT and anti-Xa start to diverge (154, 157–159).
Long term outcomes for survivors of cardiogenic shock
Intermediate and long-term outcomes in CS patients has become an important area of investigation. The CSWG demonstrated that in-hospital mortality was significantly higher in patients with AMI-CS (39.5%) as compared to HF-CS (25.3%; P < 0.0001) despite having similar hemodynamic profiles (43). In a Nationwide Readmission Database study, there was a 16% readmission rate among 4,229 survivors of CS post-ECMO who recovered and were discharged alive (160). These patients had an in-hospital mortality rate of 10% with the most common cause of re-admission being infection followed by acute decompensated heart failure (160). Additional CS studies analyzing patients with AMI-CS and non-AMI-CS (not restricted to ECMO use), respectively, reported a higher readmission rate of 20% and 23%, respectively (161, 162). In a more recent study by our group, CS patients who survived to hospital discharge had comparable 30-day readmission rate for HF-CS and AMI-CS (19.5% vs. 24.5%; p = 0.30) (17). Our group reported similar outcomes with higher in-hospital mortality for AMI-CS when compared to HF-CS (39% vs. 24%, p < 0.001); however, in patients who survived to discharge, the one-year mortality was comparable for both groups (19.7% vs. 23.5%, p = 0.41) (17). However, limited data exists on the long-term outcomes, beyond the first year following index hospitalization of patients surviving the initial phase of CS. In the 2005 French registry of Acute ST-elevation and non-ST-elevation Myocardial Infarction (FAST-MI), 59% of patients with CS were alive at 5 years with increased risk of death in the first year after discharge (163). A recent population-based retrospective Canadian study of 9,789 AMI-CS patients showed that one-year mortality was 41% and at 5 years was around 60% (164). Among patients who survived to discharge, 48% were re-admitted to the hospital and 15% died within the first year, suggesting potential opportunities to serve CS survivors during this vulnerable phase of their illness journey (164).
Role of palliative and hospice care in CS management
Despite the wide availability of pharmacological and device-based therapies, not all CS patients may benefit from them, and the illness culminates in death for many patients (165). Palliative care services can provide social, emotional, and spiritual support to CS patients and their families and is distinguished from hospice care with its focus on controlling symptoms and improving quality of life concurrently while complementing curative therapies (1, 166, 167). Feng at al. analyzed 2017 Nationwide Readmission Database with 134,000 CS admissions with a reported mortality of 36% (168). Only 9% of CS admissions utilized palliative care services which was associated with lower 30-day readmission rate of 12% as compared to 22% in those who did not see palliative care. The hospitalization cost per patient was also lower at US $51,000 vs. $67,000, findings showing benefit not only for the individuals but also for health care systems across the US (168). Unfortunately, observational data suggest patients receiving palliative care in AMI-CS is very low at 5% in the last 15 years but there is an increasing trend toward more frequent utilization of these services (169).
Knowledge gaps and future directions for CS
There are several important knowledge gaps which provide fertile opportunities for future investigation in CS including but not limited to: electronic health alerts for early identification and recognition of CS; clinically actionable risk prediction tools; “Shock-omics” phenotyping and machine learning approaches to CS; pragmatic, registry based RCTs in CS; and longitudinal survivorship in CS.
Role for early identification
CS is a heterogeneous syndrome with protean clinical manifestations. Rapid, early identification and stratification are of utmost importance in management of CS. The CSWG observational data indicate that 90% of SCAI B and 70% of SCAI C CS patients, respectively, progress to a higher SCAI stage during the course of their index hospitalization (36). This is particularly important as SCAI stage at admission was associated with higher in-hospital mortality, which further increased after re-classifying them at 24 h in the Altshock-2 registry (SCAI B = 18%, SCAI C = 27%, SCAI D = 63%, SCAI E = 100%), and was also an independent predictor of in-hospital mortality (170). Electronic health alerts built within the electronic medical record (EMR) interface may be useful in early identification analogous to the “sepsis alert” which is based on SIRS or Sequential Organ Failure Assessment (SOFA) criteria. Application of “cardiogenic shock alert” can be considered by utilizing SCAI stages, clinical history, demographics, and hemo-metabolic markers of organ perfusion (such as lactate, renal function, transaminase levels, SBP, and/or MAP). This may potentially expedite timely diagnosis and management and transfer to a higher level of care when appropriate. However, “alert fatigue” is a common phenomenon in which clinicians often ignore EMR safety notification at a rate between 49% and 96% (171). False alerts for non-shock patients are a major cause of alert fatigue. Using Natural Language Processing (NLP) with ever-growing artificial intelligence (AI) capabilities, surveillance solutions may be able to extract information from daily documentation and refine the algorithms for “cardiogenic shock alert” systems.
Role for risk prediction in CS
Although multiple risk prediction scores have been published for AMI-CS, none are widely used in contemporary clinical practice (172). While the IABP-SHOCK II score is applicable to only AMI-CS patients, the Inova Heart and Vascular Institute (IHVI) score, CardShock risk score, and SCAI staging systems may be used more broadly in other forms of CS (Table 4) (2, 11, 19, 37, 173). Despite availability of multiple risk prediction tools, development of a unified scoring system that is capable of early prognostication to inform not only the therapeutic decisions, but also encompassing risk prediction at multiple time points and in different forms of CS has been challenging. Ideal risk scores for assessing patients with CS should be contemporary, specific to etiologies and phenotypes, and consider the evolving nature of the disease. These scores should address subphenotypes, such as acute de novo HF-CS as compared to acute-on-chronic HF cases, which may present differently. An “ideal risk score” should use readily available metrics at the initial presentation and incorporate serial data collected during the index hospitalization to refine prognostic estimates. It should also account for the patient’s response to initial treatment and potentially include novel and clinically actionable variables. For this reason, RCT populations for score derivation may not be ideal. Large, real-world multicenter CS registries may be a better source for generating risk scores. These scores should be easy to calculate at the bedside and incorporate clinical, biochemical, and hemodynamic parameters of interest. Additionally, the concept of a “futility score” is proposed to rapidly identify patients unlikely to survive, facilitating appropriate resource allocation and early engagement of palliative and hospice care (172).
Utility of machine learning and artificial intelligence approaches to CS
Machine learning approaches are rapidly emerging to elucidate the mechanisms of CS. A retrospective analysis of 1,959 patients with CS from two separate CS registries (Cardiogenic Shock Working Group and Danish Retroshock MI Registry) used machine learning approaches to identify and consecutively validate three distinct phenotypes or clusters of CS patients: “non-congested (I),” “cardiorenal (II),” and “Cardiometabolic (III).” (89) Phenotype I patients, the non-congested profile, were more likely to have lower heart rates, higher blood pressures, and lower filling pressures (right atrial pressure and pulmonary capillary wedge pressure) relative to the other phenotypes. Phenotype II patients, the cardiorenal profile, were older and more likely to have comorbidities of diabetes mellitus, chronic renal insufficiency, and hypertension. These patients were at higher odds of displaying elevated mean pulmonary artery pressures and left-sided filling pressures and consequently decreased glomerular filtration rates (GFR). This group had two- to three-fold mortality compared to phenotype I. Lastly phenotype III patients, the cardio-metabolic profile, were the sickest of all the phenotypes. This group exhibited significant elevations in biomarkers such as lactic acid and aminotransferases. Clinically, they had more profound hypotension, tachycardia, elevations in right atrial pressures, decrease in cardiac power output and cardiac index. This group had up to five-fold increase in mortality relative to phenotype I (89). Although the clustering algorithm used baseline laboratory values, the identified phenotypes showed consistent clinical differences in demographics, comorbidities, and hemodynamics. Although these findings have been validated in a CICU population, the clinical utility remains to be determined. (174), It is also unclear how these phenotypes affect prognosis and treatment decisions in contemporary clinical practice. Taken together, these findings illustrate that individualized risk stratification based on etiology and unique CS phenotype may be useful in guiding therapy and improving clinical outcomes.
Designing randomized clinical trials in cardiogenic shock
Although RCTs remain the gold standard in CS, there have been numerous challenges to randomization of care and trial enrollment in critically ill CS patients. Potential reasons for these negative results in RCTs, especially in AMI-CS, include but are not limited to: (1) selection bias and failure to account for population heterogeneity; (2) inclusion of patients who are unlikely to benefit, including many cardiac arrest patients; (3) slow enrollment and low statistical power; (4) inadequate matching to shock severity and/or severity of illness; (5) enrollment after onset of multiorgan failure and irreversible sequelae of hemometabolic shock (175, 176). Observational studies have yielded conflicting results, albeit with limitations due to residual confounding, and sometimes contrary to the results of RCTs. Conducting RCTs in patients with AMI-CS is challenging due to the need for rapid informed consent from critically ill patients or their legal representatives as well as physician biases regarding equipoise with respect to the use of MCS devices. In the United States, there is a statute for Exception from Informed Consent (EFIC) under Title 21, Code of Federal Regulations, Section 50.24 (21 CFR 50.24) which allows investigators to perform “investigations involving human subjects who have a life-threatening medical condition that necessitates urgent intervention (for which available treatments are unproven or unsatisfactory), and who, because of their condition (e.g., traumatic brain injury) cannot provide informed consent.” This has not been commonly employed in CS trials to date but will be critically examined in upcoming CS trials including Recover IV.
Notably, negative findings from RCTs may mask positive treatment effects in specific subgroups, emphasizing the importance of evaluating important interactions and heterogeneity of treatment effect in subgroups in larger RCTs (175). Large-scale multinational RCTs with sufficient statistical power and inclusion of a population that closely represents real-world CS cases are crucial. While the promise of registry-based RCTs remains to be realized, there are several opportunities to heeds the lessons of recent RCTs in designing pragmatic and adaptive studies to move the field forward (176). There is a need for robust research infrastructure, inclusion of diverse patient populations, and engagement of key stakeholders in creating prospective studies with cluster randomized or stepped-wedge trial designs.
Survivorship in cardiogenic shock
Survivors of an acute episode of CS are susceptible to the long-term ramifications of this critical illness, as compromised functional status and diminished quality of life remain pervasive (177). However, evidence suggests that despite these challenges, significant proportions of survivors maintain relatively favorable functional status and quality of life, underscoring the potential for meaningful recovery. In the SHOCK trial, 87% of patients who survived for one-year had NYHA functional class I or II (178). Another CS study estimated the total life years gained was 410 years for the 230 patients with approximately $10,000 per life year gained (24). In another series of CS patients treated with ECMO, nearly all patients managed to perform daily activities and even return to gainful employment at 1-year post-index hospitalization, attesting to the potential for successful rehabilitation (179). Recently, the IHVI Shock Registry reported a 57% survival at 1 year for HF-CS and 47% for AMI-CS based on the three year study period from 2017 to 2019 (17). Higher SCAI stage D/E and age were independently associated with 1-year mortality for both AMI-CS and HF-CS with acute decompensated heart failure as the most common cause of readmission within 30 days of discharge, irrespective of etiology. Future trials such as HALO-Shock [NCT04419480] will leverage remote hemodynamic monitoring such as Abbott’s proprietary CardioMEMS technology, an implantable pulmonary artery pressure monitoring platform, to intervene early after discharge for patients with HF-CS to assess its impact on mortality, rehospitalizations, quality of life, and biomarkers (113). Understanding and improving long term outcomes after critical illness are vitally important and efforts to promote convalescence and full recovery after critical illness, especially during the vulnerable transition phase from ICU to post discharge follow-up, should receive greater consideration (177).
Conclusion
CS is a complex, multifactorial, hemo-metabolic syndrome with increasing prevalence in the modern CICU. Despite significant advances in medical and device-based therapies, CS remains associated with high morbidity and mortality, especially with increasing incidence of HF-CS. Due to the protean clinical presentations and treatment options, managing these critically ill patients requires a comprehensive, standardized, multidisciplinary team-based approach, using a regionalized system of care. Future efforts should focus on phenotyping of CS and enhancing long-term outcomes in CS survivors, leveraging registry based RCTs and multicenter shock registries to determine the optimal timing for initiating therapeutic strategies, weaning tMCS, and bridging to destination strategies including durable LVAD, heart transplant, and native myocardial recovery.
Author contributions
AM: Writing – original draft, Writing – review & editing. IV: Writing – original draft, Writing – review & editing. AN: Writing – original draft, Writing – review & editing. WB: Writing – review & editing. VB: Writing – review & editing. LC: Writing – review & editing. AD: Writing – review & editing. MD: Writing – review & editing. SD: Writing – review & editing. MF: Writing – review & editing. II: Writing – review & editing. JK: Writing – review & editing. KK: Writing – review & editing. HM: Writing – review & editing. MP: Writing – review & editing. AR: Writing – review & editing. CR: Writing – review & editing. PS: Writing – review & editing. DT: Writing – review & editing. AT: Writing – review & editing. BT: Writing – original draft, Writing – review & editing. SS: Writing – original draft, Writing – review & editing.
Funding
The author(s) declare that financial support was received for the research, authorship, and/or publication of this article.
Grant support to PS: NIH 1K23HL143179.
Acknowledgments
The authors would like to thank Dwight and Martha Schar for their generous support of Inova Schar Heart and Vascular, and the Dudley Family for their continued contributions and support of the Inova Dudley Family Center for Cardiovascular Innovation.
We would like to express our deepest appreciation to Devon Stuart, MA, CMI who has played an instrumental role in designing and drafting several figures.
Conflict of interest
The authors declare that the research was conducted in the absence of any commercial or financial relationships that could be construed as a potential conflict of interest.
The handling editor LC declared a past co-authorship with the authors CR, SD, MP.
Publisher's note
All claims expressed in this article are solely those of the authors and do not necessarily represent those of their affiliated organizations, or those of the publisher, the editors and the reviewers. Any product that may be evaluated in this article, or claim that may be made by its manufacturer, is not guaranteed or endorsed by the publisher.
Supplementary material
The Supplementary Material for this article can be found online at: https://www.frontiersin.org/articles/10.3389/fcvm.2024.1354158/full#supplementary-material
Supplemental Figure 1
Schematic representation of multidisciplinary cardiogenic shock (CS) team activation through a 1-call “shock line” to gather physicians for multidisciplinary consultation and decision making. This “shock team” can be activated by any department not only within the hospital but also across the region, to provide appropriate management for CS. CI, cardiac index; CO, cardiac output; CPO, cardiac power output; DNR, Do Not Resuscitate order; dPAP, diastolic pulmonary arterial pressure; MAP, mean arterial pressure; PAPi, pulmonary arterial pulsatility index; PCWP, pulmonary capillary wedge pressure; pMCS, percutaneous mechanical circulatory support; sPAP, systolic pulmonary arterial pressure; other abbreviations as in Figures 1–4.
Supplemental Figure 2
Schematic representation of the care pathways in the upstream and critical care management of patients with acute myocardial infarction (AMI) and heart failure (HF) cardiogenic shock at Inova Schar Heart and Vascular Institute. CI, cardiac index; CO, cardiac output; CPO, cardiac power output; DNR, Do Not Resuscitate order; dPAP, diastolic pulmonary arterial pressure; MAP, mean arterial pressure; PAPi, pulmonary arterial pulsatility index; PCWP, pulmonary capillary wedge pressure; pMCS, percutaneous mechanical circulatory support; sPAP, systolic pulmonary arterial pressure; other abbreviations as in Figures 1–4.
References
1. van Diepen S, Katz J, Albert N, Henry T, Jacobs A, Kapur N, et al. Contemporary management of cardiogenic shock: a scientific statement from the American heart association. Circulation. (2017) 136:e232–68. doi: 10.1161/CIR.0000000000000525
2. Thiele H, Akin I, Sandri M, Fuernau G, de Waha S, Meyer-Saraei R, et al. PCI strategies in patients with acute myocardial infarction and cardiogenic shock. N Engl J Med. (2017) 377(25):2419–32. doi: 10.1056/NEJMOA1710261
3. Thiele H, Zeymer U, Neumann F, Ferenc M, Olbrich H, Hausleiter J, et al. Intraaortic balloon support for myocardial infarction with cardiogenic shock. N Engl J Med. (2012) 367(14):1287–96. doi: 10.1056/NEJMOA1208410
4. Ouweneel DM, Eriksen E, Sjauw KD, Van Dongen IM, Hirsch A, Packer EJS, et al. Percutaneous mechanical circulatory support versus intra-aortic balloon pump in cardiogenic shock after acute myocardial infarction. J Am Coll Cardiol. (2017) 69(3):278–87. doi: 10.1016/j.jacc.2016.10.022
5. Ostadal P, Rokyta R, Karasek J, Kruger A, Vondrakova D, Janotka M, et al. Extracorporeal membrane oxygenation in the therapy of cardiogenic shock: results of the ECMO-CS randomized clinical trial. Circulation. (2023) 147(6):454–64. doi: 10.1161/CIRCULATIONAHA.122.062949
6. Mathew R, Di Santo P, Jung RG, Marbach JA, Hutson J, Simard T, et al. Milrinone as compared with dobutamine in the treatment of cardiogenic shock. N Engl J Med. (2021) 385(6):516–25. doi: 10.1056/NEJMoa2026845
7. Jentzer JC, Van Diepen S, Barsness GW, Henry TD, Menon V, Rihal CS, et al. Cardiogenic shock classification to predict mortality in the cardiac intensive care unit. J Am Coll Cardiol. (2019) 74(17):2117–28. doi: 10.1016/j.jacc.2019.07.077
8. Thiele H, Zeymer U, Akin I, Behnes M, Rassaf T, Mahabadi AA, et al. Extracorporeal life support in infarct-related cardiogenic shock. N Engl J Med. (2023) 389(14):1286–97. doi: 10.1056/NEJMoa2307227
9. Hochman J, Sleeper L, Webb J, Sanborn T, White H, Talley J, et al. Early revascularization in acute myocardial infarction complicated by cardiogenic shock. SHOCK investigators. Should we emergently revascularize occluded coronaries for cardiogenic shock. N Engl J Med. (1999) 341(9):625–34. doi: 10.1056/NEJM199908263410901
10. Hochman JS, Sleeper LA, White HD, Dzavik V, Wong SC, Menon V, et al. One-year survival following early revascularization for cardiogenic shock. JAMA. (2001) 285(2):190–2. doi: 10.1001/jama.285.2.190
11. Tehrani B, Truesdell AG, Sherwood MW, Desai S, Tran HA, Epps K, et al. Standardized team-based care for cardiogenic shock. J Am Coll Cardiol. (2019) 73(13):1659–69. doi: 10.1016/J.JACC.2018.12.084
12. Basir MB, Kapur NK, Patel K, Salam MA, Schreiber T, Kaki A, et al. Improved outcomes associated with the use of shock protocols: updates from the national cardiogenic shock initiative. Catheter Cardiovasc Interv. (2019) 93(7):1173–83. doi: 10.1002/CCD.28307
13. Taleb I, Koliopoulou AG, Tandar A, McKellar SH, Tonna JE, Nativi-Nicolau J, et al. Shock team approach in refractory cardiogenic shock requiring short-term mechanical circulatory support: a proof of concept. Circulation. (2019) 140(1):98–100. doi: 10.1161/CIRCULATIONAHA.119.040654
14. Lee F, Hutson JH, Boodhwani M, McDonald B, So D, De Roock S, et al. Multidisciplinary code shock team in cardiogenic shock: a Canadian centre experience. CJC Open. (2020) 2(4):249–57. doi: 10.1016/j.cjco.2020.03.009
15. Berg D, Bohula E, Van Diepen S, Katz J, Alviar C, Baird-Zars VM, et al. Epidemiology of shock in contemporary cardiac intensive care units: data from the critical care cardiology trials network registry. Circ Cardiovasc Qual Outcomes. (2019) 12(3):e005618. doi: 10.1161/CIRCOUTCOMES.119.005618
16. Jentzer JC, Ahmed AM, Vallabhajosyula S, Burstein B, Tabi M, Barsness GW, et al. Shock in the cardiac intensive care unit: changes in epidemiology and prognosis over time. Am Heart J. (2021) 232:94–104. doi: 10.1016/j.ahj.2020.10.054
17. Sinha S, Rosner CM, Tehrani BN, Maini A, Truesdell A, Lee S, et al. Cardiogenic shock from heart failure versus acute myocardial infarction: clinical characteristics, hospital course, and 1-year outcomes. Circ Heart Fail. (2022) 15(6):e009279. doi: 10.1161/CIRCHEARTFAILURE.121.009279
18. Yandrapalli S, Sanaani A, Harikrishnan P, Aronow WS, Frishman WH, Lanier GM, et al. Cardiogenic shock during heart failure hospitalizations: age-, sex-, and race-stratified trends in incidence and outcomes. Am Heart J. (2019) 213:18–29. doi: 10.1016/j.ahj.2019.03.015
19. Harjola V, Lassus J, Sionis A, Køber L, Tarvasmäki T, Špinar J, et al. Clinical picture and risk prediction of short-term mortality in cardiogenic shock. Eur J Heart Fail. (2015) 17(9):984. doi: 10.1002/EJHF.260
20. Jeger RV, Radovanovic D, Hunziker PR, Pfisterer ME, Stauffer J-C, Erne P, et al. Ten-year trends in the incidence and treatment of cardiogenic shock. Ann Intern Med. (2008) 149(9):618–26. doi: 10.7326/0003-4819-149-9-200811040-00005
21. Kolte D, Khera S, Aronow WS, Mujib M, Palaniswamy C, Sule S, et al. Trends in incidence, management, and outcomes of cardiogenic shock complicating ST-elevation myocardial infarction in the United States. J Am Heart Assoc. (2014) 3(1):e000590. doi: 10.1161/JAHA.113.000590
22. Osman M, Syed M, Patibandla S, Sulaiman S, Kheiri B, Shah MK, et al. Fifteen-year trends in incidence of cardiogenic shock hospitalization and in-hospital mortality in the United States. J Am Heart Assoc. (2021) 10(15):e021061. doi: 10.1161/JAHA.121.021061
23. Vallabhajosyula S, Prasad A, Sandhu GS, Bell MR, Gulati R, Eleid MF, et al. Ten-year trends, predictors and outcomes of mechanical circulatory support in percutaneous coronary intervention for acute myocardial infarction with cardiogenic shock. EuroIntervention. (2021) 16(15):e1254–61. doi: 10.4244/EIJ-D-19-00226
24. Collado E, Luiso D, Ariza-Solé A, Lorente V, Sánchez-Salado JC, Moreno R, et al. Hospitalization-related economic impact of patients with cardiogenic shock in a high-complexity reference centre. Eur Heart J Acute Cardiovasc Care. (2021) 10(1):50–3. doi: 10.1093/ehjacc/zuaa003
25. Mahmoud AN, Elgendy IY, Mojadidi MK, Wayangankar SA, Bavry AA, Anderson RD, et al. Prevalence, causes, and predictors of 30-day readmissions following hospitalization with acute myocardial infarction complicated by cardiogenic shock: findings from the 2013-2014 national readmissions database. J Am Heart Assoc. (2018) 7(6):e008235. doi: 10.1161/JAHA.117.008235
26. Abraham J, Blumer V, Burkhoff D, Pahuja M, Sinha SS, Rosner C, et al. Heart failure-related cardiogenic shock: pathophysiology, evaluation and management considerations: review of heart failure-related cardiogenic shock. J Card Fail. (2021) 27(10):1126–40. doi: 10.1016/J.CARDFAIL.2021.08.010
27. Goldberg RJ, Makam RCP, Yarzebski J, McManus DD, Lessard D, Gore JM. Decade-long trends (2001–2011) in the incidence and hospital death rates associated with the in-hospital development of cardiogenic shock after acute myocardial infarction. Circ Cardiovasc Qual Outcomes. (2016) 9(2):117–25. doi: 10.1161/CIRCOUTCOMES.115.002359
28. Polsinelli VB, Sinha A, Shah SJ. Visceral congestion in heart failure: right ventricular dysfunction, splanchnic hemodynamics, and the intestinal microenvironment. Curr Heart Fail Rep. (2017) 14(6):519–28. doi: 10.1007/s11897-017-0370-8
29. Jentzer JC, Lawler PR, Van Diepen S, Henry TD, Menon V, Baran DA, et al. Systemic inflammatory response syndrome is associated with increased mortality across the Spectrum of shock severity in cardiac intensive care patients. Circ Cardiovasc Qual Outcomes. (2020) 13(12):e006956. doi: 10.1161/CIRCOUTCOMES.120.006956
30. Bohula EA, Katz JN, Van Diepen S, Alviar CL, Baird-Zars VM, Park J-G, et al. Demographics, care patterns, and outcomes of patients admitted to cardiac intensive care units: the critical care cardiology trials network prospective north American multicenter registry of cardiac critical illness. JAMA Cardiol. (2019) 4(9):928–35. doi: 10.1001/jamacardio.2019.2467
31. Alonso DR, Scheidt S, Post M, Killip T. Pathophysiology of cardiogenic shock: quantification of myocardial necrosis, clinical, pathologic and electrocardiographic correlations. Circulation. (1973) 48(3):588–96. doi: 10.1161/01.CIR.48.3.588
32. Fuernau G, Desch S, de Waha-Thiele S, Eitel I, Neumann F-J, Hennersdorf M, et al. Arterial lactate in cardiogenic shock: prognostic value of clearance versus single values. JACC Cardiovasc Interv. (2020) 13(19):2208–16. doi: 10.1016/j.jcin.2020.06.037
33. Saxena A, Garan AR, Kapur NK, O'Neill WW, Lindenfeld J, Pinney SP, et al. Value of hemodynamic monitoring in patients with cardiogenic shock undergoing mechanical circulatory support. Circulation. (2020) 141(14):1184–97. doi: 10.1161/CIRCULATIONAHA.119.043080
34. Menon V, Slater JN, White HD, Sleeper LA, Cocke T, Hochman JS. Acute myocardial infarction complicated by systemic hypoperfusion without hypotension: report of the SHOCK trial registry. Am J Med. (2000) 108(5):374–80. doi: 10.1016/s0002-9343(00)00310-7
35. Baran D, Grines C, Bailey S, Burkhoff D, Hall S, Henry T, et al. SCAI clinical expert consensus statement on the classification of cardiogenic shock. Catheter Cardiovasc Interv Off J Soc Card Angiogr Interv. (2019) 94(1):29–37. doi: 10.1002/CCD.28329
36. Kapur NK, Kanwar M, Sinha SS, Thayer KL, Garan AR, Hernandez-Montfort J, et al. Criteria for defining stages of cardiogenic shock severity. J Am Coll Cardiol. (2022) 80(3):185–98. doi: 10.1016/j.jacc.2022.04.049
37. Naidu SS, Baran DA, Jentzer JC, Hollenberg SM, Van Diepen S, Basir MB, et al. SCAI SHOCK stage classification expert consensus update: a review and incorporation of validation studies. J Soc Cardiovas Angio Interv. (2022) 79(9):933–46. doi: 10.1016/J.JSCAI.2021.100008
38. Baran DA, Long A, Badiye AP, Stelling K. Prospective validation of the SCAI shock classification: single center analysis. Catheter Cardiovasc Interv Off J Soc Card Angiogr Interv. (2020) 96(7):1339–47. doi: 10.1002/ccd.29319
39. Nohria A, Tsang SW, Fang JC, Lewis EF, Jarcho JA, Mudge GH, et al. Clinical assessment identifies hemodynamic profiles that predict outcomes in patients admitted with heart failure. J Am Coll Cardiol. (2003) 41(10):1797–804. doi: 10.1016/s0735-1097(03)00309-7
40. Forrester JS, Diamond G, Chatterjee K, Swan HJ. Medical therapy of acute myocardial infarction by application of hemodynamic subsets (first of two parts). N Engl J Med. (1976) 295(24):1356–62. doi: 10.1056/NEJM197612092952406
41. Forrester JS, Diamond G, Chatterjee K, Swan HJ. Medical therapy of acute myocardial infarction by application of hemodynamic subsets (second of two parts). N Engl J Med. (1976) 295(25):1404–13. doi: 10.1056/NEJM197612162952505
42. Jacobs AK, Leopold JA, Bates E, Mendes LA, Sleeper LA, White H, et al. Cardiogenic shock caused by right ventricular infarction: a report from the SHOCK registry. J Am Coll Cardiol. (2003) 41(8):1273–9. doi: 10.1016/s0735-1097(03)00120-7
43. Thayer KL, Zweck E, Ayouty M, Garan AR, Hernandez-Montfort J, Mahr C, et al. Invasive hemodynamic assessment and classification of in-hospital mortality risk among patients with cardiogenic shock. Circ-Heart Fail.(2020) 13(9):e007099. doi: 10.1161/CIRCHEARTFAILURE.120.007099
44. Henry TD, Tomey MI, Tamis-Holland JE, Thiele H, Rao SV, Menon V, et al. Invasive management of acute myocardial infarction complicated by cardiogenic shock: a scientific statement from the American heart association. Circulation. (2021) 143(15):e815–29. doi: 10.1161/CIR.0000000000000959
45. Claessen BE, Henriques JPS. Acute myocardial infarction, chronic total occlusion, and cardiogenic shock: the ultimate triple threat. EuroIntervention. (2018) 14(3):e252–4. doi: 10.4244/EIJV14I3A42
46. McCallister BD, Christian TF, Gersh BJ, Gibbons RJ. Prognosis of myocardial infarctions involving more than 40% of the left ventricle after acute reperfusion therapy. Circulation. (1993) 88(4):1470–5. doi: 10.1161/01.CIR.88.4.1470
47. Samsky MD, Morrow DA, Proudfoot AG, Hochman JS, Thiele H, Rao SV. Cardiogenic shock after acute myocardial infarction: a review. JAMA. (2021) 326(18):1840–50. doi: 10.1001/jama.2021.18323
48. Webb JG, Sleeper LA, Buller CE, Boland J, Palazzo A, Buller E, et al. Implications of the timing of onset of cardiogenic shock after acute myocardial infarction: a report from the SHOCK trial registry. J Am Coll Cardiol. (2000) 36(3):1084–90. doi: 10.1016/S0735-1097(00)00876-7
49. Hochman JS. Cardiogenic shock complicating acute myocardial infarction: expanding the paradigm. Circulation. (2003) 107(24):2998–3002. doi: 10.1161/01.CIR.0000075927.67673.F2
50. Damluji AA, Van Diepen S, Katz JN, Menon V, Tamis-Holland JE, Bakitas M, et al. Mechanical complications of acute myocardial infarction: a scientific statement from the American heart association. Circulation. (2021) 144(2):e16–35. doi: 10.1161/CIR.0000000000000985
51. Scholz KH, Maier SKG, Maier LS, Lengenfelder B, Jacobshagen C, Jung J, et al. Impact of treatment delay on mortality in ST-segment elevation myocardial infarction (STEMI) patients presenting with and without haemodynamic instability: results from the German prospective, multicentre FITT-STEMI trial. Eur Heart J. (2018) 39(13):1065–74. doi: 10.1093/eurheartj/ehy004
52. Mebazaa A, Tolppanen H, Mueller C, Lassus J, DiSomma S, Baksyte G, et al. Acute heart failure and cardiogenic shock: a multidisciplinary practical guidance. Intensive Care Med. (2016) 42(2):147–63. doi: 10.1007/s00134-015-4041-5
53. Basir MB, Schreiber TL, Grines CL, Dixon SR, Moses JW, Maini BS, et al. Effect of early initiation of mechanical circulatory support on survival in cardiogenic shock. Am J Cardiol. (2017) 119(6):845–51. doi: 10.1016/j.amjcard.2016.11.037
54. Jacobs AK, Ali MJ, Best PJ, Bieniarz MC, Bufalino VJ, French WJ, et al. Systems of care for ST-segment-elevation myocardial infarction: a policy statement from the American heart association. Circulation. (2021) 144(20):e310–27. doi: 10.1161/CIR.0000000000001025
55. Rab T, Ratanapo S, Kern KB, Basir MB, McDaniel M, Meraj P, et al. Cardiac shock care centers. J Am Coll Cardiol. (2018) 72(16):1972–80. doi: 10.1016/j.jacc.2018.07.074
56. Levy B, Clere-Jehl R, Legras A, Morichau-Beauchant T, Leone M, Frederique G, et al. Epinephrine versus norepinephrine for cardiogenic shock after acute myocardial infarction. J Am Coll Cardiol. (2018) 72(2):173–82. doi: 10.1016/j.jacc.2018.04.051
57. McDonagh TA, Metra M, Adamo M, Gardner RS, Baumbach A, Böhm M, et al. 2021 ESC guidelines for the diagnosis and treatment of acute and chronic heart failure. Eur Heart J. (2021) 42(36):3599–726. doi: 10.1093/eurheartj/ehab368
58. van Diepen S, Hochman JS, Stebbins A, Alviar CL, Alexander JH, Lopes RD. Association between delays in mechanical ventilation initiation and mortality in patients with refractory cardiogenic shock. JAMA Cardiol. (2020) 5(8):965–7. doi: 10.1001/jamacardio.2020.1274
59. Tehrani B, Truesdell AG, Psotka MA, Rosner C, Singh R, Sinha SS, et al. A standardized and comprehensive approach to the management of cardiogenic shock. Jacc-Heart Fail. (2020) 8(11):879–91. doi: 10.1016/J.JCHF.2020.09.005
60. Harhash AA, May TL, Hsu C-H, Agarwal S, Seder DB, Mooney MR, et al. Risk stratification among survivors of cardiac arrest considered for coronary angiography. J Am Coll Cardiol. (2021) 77(4):360–71. doi: 10.1016/j.jacc.2020.11.043
61. Rogers JG, Patel CB, Mentz RJ, Granger BB, Steinhauser KE, Fiuzat M, et al. Palliative care in heart failure: the PAL-HF randomized, controlled clinical trial. J Am Coll Cardiol. (2017) 70(3):331–41. doi: 10.1016/j.jacc.2017.05.030
62. Ferrante G, Condello F, Rao SV, Maurina M, Jolly S, Stefanini GG, et al. Distal vs conventional radial access for coronary angiography and/or intervention: a meta-analysis of randomized trials. JACC Cardiovasc Interv. (2022) 15(22):2297–311. doi: 10.1016/j.jcin.2022.09.006
63. Lawton JS, Tamis-Holland JE, Bangalore S, Bates ER, Beckie TM, Bischoff JM, et al. 2021 ACC/AHA/SCAI guideline for coronary artery revascularization: executive summary: a report of the American college of cardiology/American heart association joint committee on clinical practice guidelines. Circulation. (2022) 145(3):e4–e17. doi: 10.1161/CIR.0000000000001039
64. Neumann F-J, Sousa-Uva M, Ahlsson A, Alfonso F, Banning AP, Benedetto U, et al. 2018 ESC/EACTS guidelines on myocardial revascularization. EuroIntervention. (2019) 14(14):1435–534. doi: 10.4244/EIJY19M01_01
65. Tehrani BN, Damluji AA, Sherwood MW, Rosner C, Truesdell AG, Epps KC, et al. Transradial access in acute myocardial infarction complicated by cardiogenic shock: stratified analysis by shock severity. Catheter Cardiovasc Interv Off J Soc Card Angiogr Interv. (2021) 97(7):1354–66. doi: 10.1002/ccd.29098
66. Amin AP, Spertus JA, Curtis JP, Desai N, Masoudi FA, Bach RG, et al. The evolving landscape of impella use in the United States among patients undergoing percutaneous coronary intervention with mechanical circulatory support. Circulation. (2020) 141(4):273–84. doi: 10.1161/CIRCULATIONAHA.119.044007
67. Sandoval Y, Burke MN, Lobo AS, Lips DL, Seto AH, Chavez I, et al. Contemporary arterial access in the cardiac catheterization laboratory. JACC Cardiovasc Interv. (2017) 10(22):2233–41. doi: 10.1016/j.jcin.2017.08.058
68. Damluji AA, Tehrani B, Sinha SS, Samsky MD, Henry TD, Thiele H, et al. Position statement on vascular access safety for percutaneous devices in AMI complicated by cardiogenic shock. JACC Cardiovasc Interv. (2022) 15(20):2003–19. doi: 10.1016/j.jcin.2022.08.041
69. Wollmuth J, Korngold E, Croce K, Pinto DS. The single-access for hi-risk PCI (SHiP) technique. Catheter Cardiovasc Interv Off J Soc Card Angiogr Interv. (2020) 96(1):114–6. doi: 10.1002/ccd.28556
70. Radu RI, Ben Gal T, Abdelhamid M, Antohi E-L, Adamo M, Ambrosy AP, et al. Antithrombotic and anticoagulation therapies in cardiogenic shock: a critical review of the published literature. ESC Heart Fail. (2021) 8(6):4717–36. doi: 10.1002/ehf2.13643
71. Gorog DA, Price S, Sibbing D, Baumbach A, Capodanno D, Gigante B, et al. Antithrombotic therapy in patients with acute coronary syndrome complicated by cardiogenic shock or out-of-hospital cardiac arrest: a joint position paper from the European society of cardiology (ESC) working group on thrombosis, in association with the acute cardiovascular care association (ACCA) and European association of percutaneous cardiovascular interventions (EAPCI). Eur Heart J Cardiovasc Pharmacother. (2021) 7(2):125–40. doi: 10.1093/ehjcvp/pvaa009
72. Orban M, Mayer K, Morath T, Bernlochner I, Hadamitzky M, Braun S, et al. Prasugrel vs clopidogrel in cardiogenic shock patients undergoing primary PCI for acute myocardial infarction. Results of the ISAR-SHOCK registry. Thromb Haemost. (2014) 112(6):1190–7. doi: 10.1160/TH14-06-0489
73. Marquis-Gravel G, Zeitouni M, Kochar A, Jones WS, Sketch MH, Rao SV, et al. Technical consideration in acute myocardial infarction with cardiogenic shock: a review of antithrombotic and PCI therapies. Catheter Cardiovasc Interv Off J Soc Card Angiogr Interv. (2020) 95(5):924–31. doi: 10.1002/ccd.28455
74. Rollini F, Franchi F, Hu J, Kureti M, Aggarwal N, Durairaj A, et al. Crushed prasugrel tablets in patients with STEMI undergoing primary percutaneous coronary intervention: the CRUSH study. J Am Coll Cardiol. (2016) 67(17):1994–2004. doi: 10.1016/j.jacc.2016.02.045
75. Parodi G, Xanthopoulou I, Bellandi B, Gkizas V, Valenti R, Karanikas S, et al. Ticagrelor crushed tablets administration in STEMI patients: the MOJITO study. J Am Coll Cardiol. (2015) 65(5):511–2. doi: 10.1016/j.jacc.2014.08.056
76. Prüller F, Bis L, Milke OL, Fruhwald F, Pätzold S, Altmanninger-Sock S, et al. Cangrelor induces more potent platelet inhibition without increasing bleeding in resuscitated patients. J Clin Med. (2018) 7(11):442. doi: 10.3390/jcm7110442
77. Tousek P, Rokyta R, Tesarova J, Pudil R, Belohlavek J, Stasek J, et al. Routine upfront Abciximab versus standard periprocedural therapy in patients undergoing primary percutaneous coronary intervention for cardiogenic shock: the PRAGUE-7 study. An open randomized multicentre study. Acute Card Care. (2011) 13(3):116–22. doi: 10.3109/17482941.2011.567282
78. Webb JG, Lowe AM, Sanborn TA, White HD, Sleeper LA, Carere RG, et al. Percutaneous coronary intervention for cardiogenic shock in the SHOCK trial. J Am Coll Cardiol. (2003) 42(8):1380–6. doi: 10.1016/s0735-1097(03)01050-7
79. Acharya D, Gulack BC, Loyaga-Rendon RY, Davies JE, He X, Brennan JM, et al. Clinical characteristics and outcomes of patients with myocardial infarction and cardiogenic shock undergoing coronary artery bypass surgery: data from the society of thoracic surgeons national database. Ann Thorac Surg. (2016) 101(2):558–66. doi: 10.1016/j.athoracsur.2015.10.051
80. Mehta SR, Wood DA, Storey RF, Mehran R, Bainey KR, Nguyen H, et al. Complete revascularization with multivessel PCI for myocardial infarction. N Engl J Med. (2019) 381(15):1411–21. doi: 10.1056/NEJMoa1907775
81. Thiele H, Akin I, Sandri M, de Waha-Thiele S, Meyer-Saraei R, Fuernau G, et al. One-year outcomes after PCI strategies in cardiogenic shock. N Engl J Med. (2018) 379(18):1699–710. doi: 10.1056/NEJMoa1808788
82. Henriques JPS, Hoebers LP, Råmunddal T, Laanmets P, Eriksen E, Bax M, et al. Percutaneous intervention for concurrent chronic total occlusions in patients with STEMI: the EXPLORE trial. J Am Coll Cardiol. (2016) 68(15):1622–32. doi: 10.1016/j.jacc.2016.07.744
83. Lemor A, Basir MB, Patel K, Kolski B, Kaki A, Kapur NK, et al. Multivessel versus culprit-vessel percutaneous coronary intervention in cardiogenic shock. JACC Cardiovasc Interv. (2020) 13(10):1171–8. doi: 10.1016/j.jcin.2020.03.012
84. Chen D-Y, Mao C-T, Tsai M-L, Chen S-W, Lin Y-S, Hsieh I-C, et al. Clinical outcomes of drug-eluting stents versus bare-metal stents in patients with cardiogenic shock complicating acute myocardial infarction. Int J Cardiol. (2016) 215:98–104. doi: 10.1016/j.ijcard.2016.04.014
85. Ledwoch J, Fuernau G, Desch S, Eitel I, Jung C, De Waha S, et al. Drug-eluting stents versus bare-metal stents in acute myocardial infarction with cardiogenic shock. Heart Br Card Soc. (2017) 103(15):1177–84. doi: 10.1136/heartjnl-2016-310403
86. Stevenson LW, Tillisch JH, Hamilton M, Luu M, Chelimsky-Fallick C, Moriguchi J, et al. Importance of hemodynamic response to therapy in predicting survival with ejection fraction less than or equal to 20% secondary to ischemic or nonischemic dilated cardiomyopathy. Am J Cardiol. (1990) 66(19):1348–54. doi: 10.1016/0002-9149(90)91166-4
87. Ranka S, Mastoris I, Kapur NK, Tedford RJ, Rali A, Acharya P, et al. Right heart catheterization in cardiogenic shock is associated with improved outcomes: insights from the nationwide readmissions database. J Am Heart Assoc. (2021) 10(17):e019843. doi: 10.1161/JAHA.120.019843
88. Kadosh BS, Berg DD, Bohula EA, Park J-G, Baird-Zars VM, Alviar C, et al. Pulmonary artery catheter use and mortality in the cardiac intensive care unit. JACC Heart Fail. (2023) 11(8 Pt 1):903–14. doi: 10.1016/j.jchf.2023.04.007
89. Zweck E, Thayer KL, Helgestad OKL, Kanwar M, Ayouty M, Garan AR, et al. Phenotyping cardiogenic shock. J Am Heart Assoc. (2021) 10(14):e020085. doi: 10.1161/JAHA.120.020085
90. Cooper LB, Mentz RJ, Stevens SR, Felker GM, Lombardi C, Metra M, et al. Hemodynamic predictors of heart failure morbidity and mortality: fluid or flow? J Card Fail. (2016) 22(3):182–9. doi: 10.1016/j.cardfail.2015.11.012
91. Mullens W, Dauw J, Martens P, Verbrugge FH, Nijst P, Meekers E, et al. Acetazolamide in acute decompensated heart failure with volume overload. N Engl J Med. (2022) 387(13):1185–95. doi: 10.1056/NEJMoa2203094
92. Celso B, Tepas J, Langland-Orban B, Pracht E, Papa L, Lottenberg L, et al. A systematic review and meta-analysis comparing outcome of severely injured patients treated in trauma centers following the establishment of trauma systems. J Trauma. (2006) 60(2):371–8. doi: 10.1097/01.ta.0000197916.99629.eb
93. Maharaj R, Raffaele I, Wendon J. Rapid response systems: a systematic review and meta-analysis. Crit Care Lond Engl. (2015) 19(1):254. doi: 10.1186/s13054-015-0973-y
94. Morey JR, Oxley TJ, Wei D, Kellner CP, Dangayach NS, Stein L, et al. Mobile interventional stroke team model improves early outcomes in large vessel occlusion stroke: the NYC MIST trial. Stroke. (2020) 51(12):3495–503. doi: 10.1161/STROKEAHA.120.030248
95. Ju T, Al-Mashat M, Rivas L, Sarani B. Sepsis rapid response teams. Crit Care Clin. (2018) 34(2):253–8. doi: 10.1016/j.ccc.2017.12.004
96. Jaroszewski DE, Kleisli T, Staley L, Pierce C, Scott R, Steidley DE, et al. A traveling team concept to expedite the transfer and management of unstable patients in cardiopulmonary shock. J Heart Lung Transplant Off Publ Int Soc Heart Transplant. (2011) 30(6):618–23. doi: 10.1016/j.healun.2010.11.018
97. Doll JA, Ohman EM, Patel MR, Milano CA, Rogers JG, Wohns DH, et al. A team-based approach to patients in cardiogenic shock. Catheter Cardiovasc Interv Off J Soc Card Angiogr Interv. (2016) 88(3):424–33. doi: 10.1002/ccd.26297
98. Papolos AI, Kenigsberg BB, Berg DD, Alviar CL, Bohula E, Burke JA, et al. Management and outcomes of cardiogenic shock in cardiac ICUs with versus without shock teams. J Am Coll Cardiol. (2021) 78(13):1309–17. doi: 10.1016/j.jacc.2021.07.044
99. Heidenreich PA, Bozkurt B, Aguilar D, Allen LA, Byun JJ, Colvin MM, et al. 2022 AHA/ACC/HFSA guideline for the management of heart failure: a report of the American college of cardiology/American heart association joint committee on clinical practice guidelines. Circulation. (2022) 145(18):e895–e1032. doi: 10.1161/CIR.0000000000001063
100. Shaefi S, O'Gara B, Kociol RD, Joynt K, Mueller A, Nizamuddin J, et al. Effect of cardiogenic shock hospital volume on mortality in patients with cardiogenic shock. J Am Heart Assoc. (2015) 4(1):e001462. doi: 10.1161/JAHA.114.001462
101. Tehrani BN, Sherwood MW, Rosner C, Truesdell AG, Ben Lee S, Damluji AA, et al. A standardized and regionalized network of care for cardiogenic shock. JACC Heart Fail. (2022) 10(10):768–81. doi: 10.1016/j.jchf.2022.04.004
102. Psotka MA, Gottlieb SS, Francis GS, Allen LA, Teerlink JR, Adams KF, et al. Cardiac calcitropes, myotropes, and mitotropes: JACC review topic of the week. J Am Coll Cardiol. (2019) 73(18):2345–53. doi: 10.1016/j.jacc.2019.02.051
103. De Backer D, Biston P, Devriendt J, Madl C, Chochrad D, Aldecoa C, et al. Comparison of dopamine and norepinephrine in the treatment of shock. N Engl J Med. (2010) 362(9):779–89. doi: 10.1056/NEJMoa0907118
104. Cuffe MS, Califf RM, Adams KF, Benza R, Bourge R, Colucci WS, et al. Short-term intravenous milrinone for acute exacerbation of chronic heart failure: a randomized controlled trial. JAMA. (2002) 287(12):1541–7. doi: 10.1001/jama.287.12.1541
105. Writing Committee Members, ACC/AHA Joint Committee Members. 2022 AHA/ACC/HFSA guideline for the management of heart failure. J Card Fail. (2022) 28(5):e1–e167. doi: 10.1016/j.cardfail.2022.02.010
106. Binanay C, Califf RM, Hasselblad V, O'Connor CM, Shah MR, Sopko G, et al. Evaluation study of congestive heart failure and pulmonary artery catheterization effectiveness: the ESCAPE trial. JAMA. (2005) 294(13):1625–33. doi: 10.1001/jama.294.13.1625
107. Allen LA, Rogers JG, Warnica JW, Disalvo TG, Tasissa G, Binanay C, et al. High mortality without ESCAPE: the registry of heart failure patients receiving pulmonary artery catheters without randomization. J Card Fail. (2008) 14(8):661–9. doi: 10.1016/j.cardfail.2008.05.004
108. Garan AR, Kanwar M, Thayer KL, Whitehead E, Zweck E, Hernandez-Montfort J, et al. Complete hemodynamic profiling with pulmonary artery catheters in cardiogenic shock is associated with lower in-hospital mortality. JACC Heart Fail. (2020) 8(11):903–13. doi: 10.1016/j.jchf.2020.08.012
109. Kanwar M, Blumer V, Zhang Y, Sinha S, Garan A, Hernandez-Montfort J, et al. Pulmonary artery catheter use and risk of in-hospital death in heart failure cardiogenic shock. J Card Fail. (2023) 29(9):1234–44. doi: 10.1016/J.CARDFAIL.2023.05.001
110. Hernandez GabrielA, Lemor A, Blumer V, Rueda CA, Zalawadiya S, Stevenson LW, et al. Trends in utilization and outcomes of pulmonary artery catheterization in heart failure with and without cardiogenic shock. J Card Fail. 2019;25(5):364–71. doi: 10.1016/j.cardfail.2019.03.004
111. McDonagh TA, Metra M, Adamo M, Gardner RS, Baumbach A, Böhm M, et al. 2021 ESC guidelines for the diagnosis and treatment of acute and chronic heart failure: developed by the task force for the diagnosis and treatment of acute and chronic heart failure of the European society of cardiology (ESC) with the special contribution of the heart failure association (HFA) of the ESC. Rev Espanola Cardiol Engl Ed. (2022) 75(6):523. doi: 10.1016/j.rec.2022.05.005
112. Tufts Medical Center. The Pulmonary Artery Catheter in Cardiogenic Shock Trial. clinicaltrials.gov; (2022). Available online at: https://clinicaltrials.gov/study/NCT05485376 (Accessed August 9, 2023).
113. Inova Health Care Services. Hemodynamic Monitoring to Prevent Adverse Events foLlowing cardiOgenic Shock Trial. clinicaltrials.gov; (2023). Available online at: https://clinicaltrials.gov/study/NCT04419480 (Accessed August 9, 2023).
114. Pahuja M, Adegbala O, Mishra T, Akintoye E, Chehab O, Mony S, et al. Trends in the incidence of in-hospital mortality, cardiogenic shock, and utilization of mechanical circulatory support devices in myocarditis (analysis of national inpatient sample data, 2005–2014). J Card Fail. (2019) 25(6):457–67. doi: 10.1016/j.cardfail.2019.04.012
115. Schrage B, Becher PM, Goßling A, Savarese G, Dabboura S, Yan I, et al. Temporal trends in incidence, causes, use of mechanical circulatory support and mortality in cardiogenic shock. ESC Heart Fail. (2021) 8(2):1295–303. doi: 10.1002/ehf2.13202
116. Dhruva SS, Ross JS, Mortazavi BJ, Hurley NC, Krumholz HM, Curtis JP, et al. Association of use of an intravascular microaxial left ventricular assist device vs intra-aortic balloon pump with in-hospital mortality and Major bleeding among patients with acute myocardial infarction complicated by cardiogenic shock. JAMA. (2020) 323(8):734. doi: 10.1001/jama.2020.0254
117. Endorsed by the Latin American Society of Interventional Cardiology, PCI WRITING COMMITTEE, Levine GN, Bates ER, Blankenship JC, Bailey SR, et al. 2015 ACC/AHA/SCAI focused update on primary percutaneous coronary intervention for patients with ST-elevation myocardial infarction: an update of the 2011 ACCF/AHA/SCAI guideline for percutaneous coronary intervention and the 2013 ACCF/AHA guideline for the management of ST-elevation myocardial infarction: a report of the American college of cardiology/American heart association task force on clinical practice guidelines and the society for cardiovascular angiography and interventions. Catheter Cardiovasc Interv Off J Soc Card Angiogr Interv. (2016) 87(6):1001–19. doi: 10.1002/ccd.26325
118. Jain P, Salama M, Everett K, Reyelt L, Kapur NK. To vent or not to vent: a loaded question during venoarterial extracorporeal membrane oxygenation support for cardiogenic shock. Circ Cardiovasc Interv. (2021) 14(5):e010537. doi: 10.1161/CIRCINTERVENTIONS.121.010537
119. Møller JE, Gerke O, DanGer Shock Investigators. Danish-German cardiogenic shock trial-DanGer shock: trial design update. Am Heart J. (2023) 255:90–3. doi: 10.1016/j.ahj.2022.10.078
120. Basir MB, Pinto DS, Ziaeian B, Khandelwal A, Cowger J, Suh W, et al. Mechanical circulatory support in acute myocardial infarction and cardiogenic shock: challenges and importance of randomized control trials. Catheter Cardiovasc Interv. (2021) 98(7):1264–74. doi: 10.1002/ccd.29593
121. Geller BJ, Sinha SS, Kapur NK, Bakitas M, Balsam LB, Chikwe J, et al. Escalating and De-escalating temporary mechanical circulatory support in cardiogenic shock: a scientific statement from the American heart association. Circulation. (2022) 146(6):e50–68. doi: 10.1161/CIR.0000000000001076
122. Marbach JA, Stone S, Schwartz B, Pahuja M, Thayer KL, Faugno AJ, et al. Lactate clearance is associated with improved survival in cardiogenic shock: a systematic review and meta-analysis of prognostic factor studies. J Card Fail. (2021) 27(10):1082–9. doi: 10.1016/j.cardfail.2021.08.012
123. Bernhardt AM, Copeland H, Deswal A, Gluck J, Givertz MM, Task Force 1, et al. The international society for heart and lung transplantation/heart failure society of America guideline on acute mechanical circulatory support. J Card Fail. (2023) 29(3):304–74. doi: 10.1016/j.cardfail.2022.11.003
124. Thiele H, Zeymer U, Thelemann N, Neumann F, Hausleiter J, Abdel-Wahab M, et al. Intraaortic balloon pump in cardiogenic shock complicating acute myocardial infarction: long-term 6-year outcome of the randomized IABP-SHOCK II trial. Circulation. (2019) 139(3):395–403. doi: 10.1161/CIRCULATIONAHA.118.038201
125. Thiele H, Zeymer U, Neumann F-J, Ferenc M, Olbrich HG, Hausleiter J, et al. Intra-aortic balloon counterpulsation in acute myocardial infarction complicated by cardiogenic shock (IABP-SHOCK II): final 12 month results of a randomised, open-label trial. Lancet. (2013) 382(9905):1638-–1645. doi: 10.1016/S0140-6736(13)61783-3
126. Fried JA, Nair A, Takeda K, Clerkin K, Topkara VK, Masoumi A, et al. Clinical and hemodynamic effects of intra-aortic balloon pump therapy in chronic heart failure patients with cardiogenic shock. J Heart Lung Transplant Off Publ Int Soc Heart Transplant. (2018) 37(11):1313–21. doi: 10.1016/j.healun.2018.03.011
127. Imamura T, Juricek C, Nguyen A, Chung B, Rodgers D, Sayer G, et al. Predictors of hemodynamic improvement and stabilization following intraaortic balloon pump implantation in patients with advanced heart failure. J Invasive Cardiol. (2018) 30(2):56–61. PMID: 29335384.29335384
128. Malick W, Fried JA, Masoumi A, Nair A, Zuver A, Huang A, et al. Comparison of the hemodynamic response to intra-aortic balloon counterpulsation in patients with cardiogenic shock resulting from acute myocardial infarction versus acute decompensated heart failure. Am J Cardiol. (2019) 124(12):1947–53. doi: 10.1016/j.amjcard.2019.09.016
129. Bhimaraj A, Agrawal T, Duran A, Tamimi O, Amione-Guerra J, Trachtenberg B, et al. Percutaneous left axillary artery placement of intra-aortic balloon pump in advanced heart failure patients. JACC Heart Fail. (2020) 8(4):313–23. doi: 10.1016/j.jchf.2020.01.011
130. Castagna F, Viswanathan S, Chalhoub G, Ippolito P, Ovalle Ramos JA, Vukelic S, et al. Predicting hemodynamic changes during intra-aortic balloon pump support with a longitudinal evaluation. ASAIO J. (2023) 69(11):977–83. doi: 10.1097/MAT.0000000000002014
131. Morici N, Marini C, Sacco A, Tavazzi G, Cipriani M, Oliva F, et al. Early intra-aortic balloon pump in acute decompensated heart failure complicated by cardiogenic shock: rationale and design of the randomized altshock-2 trial. Am Heart J. (2021) 233:39–47. doi: 10.1016/j.ahj.2020.11.017
132. Seyfarth M, Sibbing D, Bauer I, Fröhlich G, Bott-Flügel L, Byrne R, et al. A randomized clinical trial to evaluate the safety and efficacy of a percutaneous left ventricular assist device versus intra-aortic balloon pumping for treatment of cardiogenic shock caused by myocardial infarction. J Am Coll Cardiol. (2008) 52(19):1584–8. doi: 10.1016/j.jacc.2008.05.065
133. Moustafa A, Khan MS, Saad M, Siddiqui S, Eltahawy E. Impella support versus intra-aortic balloon pump in acute myocardial infarction complicated by cardiogenic shock: a meta-analysis. Cardiovasc Revascularization Med Mol Interv. (2022) 34:25–31. doi: 10.1016/j.carrev.2021.01.028
134. Thiele H, Jobs A, Ouweneel D, Henriques J, Seyfarth M, Desch S, et al. Percutaneous short-term active mechanical support devices in cardiogenic shock: a systematic review and collaborative meta-analysis of randomized trials. Eur Heart J. (2017) 38(47):3523–31. doi: 10.1093/EURHEARTJ/EHX363
135. Briceno N, Annamalai SK, Reyelt L, Crowley P, Qiao X, Swain L, et al. Left ventricular unloading increases the coronary collateral flow Index before reperfusion and reduces infarct size in a swine model of acute myocardial infarction. J Am Heart Assoc. (2019) 8(22):e013586. doi: 10.1161/JAHA.119.013586
136. Kapur NK, Kim RJ, Moses JW, Stone GW, Udelson JE, Ben-Yehuda O, et al. Primary left ventricular unloading with delayed reperfusion in patients with anterior ST-elevation myocardial infarction: rationale and design of the STEMI-DTU randomized pivotal trial. Am Heart J. (2022) 254:122–32. doi: 10.1016/j.ahj.2022.08.011
137. Anderson MB, Goldstein J, Milano C, Morris LD, Kormos RL, Bhama J, et al. Benefits of a novel percutaneous ventricular assist device for right heart failure: the prospective RECOVER RIGHT study of the impella RP device. J Heart Lung Transplant Off Publ Int Soc Heart Transplant. (2015) 34(12):1549–60. doi: 10.1016/j.healun.2015.08.018
138. Kar B, Gregoric ID, Basra SS, Idelchik GM, Loyalka P. The percutaneous ventricular assist device in severe refractory cardiogenic shock. J Am Coll Cardiol. (2011) 57(6):688–96. doi: 10.1016/j.jacc.2010.08.613
139. Salna M, Garan AR, Kirtane AJ, Karmpaliotis D, Green P, Takayama H, et al. Novel percutaneous dual-lumen cannula-based right ventricular assist device provides effective support for refractory right ventricular failure after left ventricular assist device implantation. Interact Cardiovasc Thorac Surg. (2020) 30(4):499–506. doi: 10.1093/icvts/ivz322
140. Thiele H, Ohman EM, Desch S, Eitel I, De Waha S. Management of cardiogenic shock. Eur Heart J. (2015) 36(20):1223–30. doi: 10.1093/eurheartj/ehv051
141. Cheng JM, Den Uil CA, Hoeks SE, Van Der Ent M, Jewbali LSD, Van Domburg RT, et al. Percutaneous left ventricular assist devices vs. Intra-aortic balloon pump counterpulsation for treatment of cardiogenic shock: a meta-analysis of controlled trials. Eur Heart J. (2009) 30(17):2102–8. doi: 10.1093/eurheartj/ehp292
142. Rao P, Khalpey Z, Smith R, Burkhoff D, Kociol RD. Venoarterial extracorporeal membrane oxygenation for cardiogenic shock and cardiac arrest. Circ Heart Fail. (2018) 11(9):e004905. doi: 10.1161/CIRCHEARTFAILURE.118.004905
143. Keebler ME, Haddad EV, Choi CW, McGrane S, Zalawadiya S, Schlendorf KH, et al. Venoarterial extracorporeal membrane oxygenation in cardiogenic shock. JACC Heart Fail. (2018) 6(6):503–16. doi: 10.1016/j.jchf.2017.11.017
144. Takeda K, Li B, Garan AR, Topkara VK, Han J, Colombo PC, et al. Improved outcomes from extracorporeal membrane oxygenation versus ventricular assist device temporary support of primary graft dysfunction in heart transplant. J Heart Lung Transplant. (2017) 36(6):650–6. doi: 10.1016/j.healun.2016.12.006
145. Biscotti M, Bacchetta M. The “sport model”: extracorporeal membrane oxygenation using the subclavian artery. Ann Thorac Surg. (2014) 98(4):1487–9. doi: 10.1016/j.athoracsur.2014.02.069
146. Chicotka S, Rosenzweig EB, Brodie D, Bacchetta M. The “central sport model”: extracorporeal membrane oxygenation using the innominate artery for smaller patients as bridge to lung transplantation. ASAIO J Am Soc Artif Intern Organs 1992. (2017) 63(4):e39–44. doi: 10.1097/MAT.0000000000000427
147. Cheng R, Hachamovitch R, Kittleson M, Patel J, Arabia F, Moriguchi J, et al. Complications of extracorporeal membrane oxygenation for treatment of cardiogenic shock and cardiac arrest: a meta-analysis of 1,866 adult patients. Ann Thorac Surg. (2014) 97(2):610–6. doi: 10.1016/j.athoracsur.2013.09.008
148. Banning AS, Sabate M, Orban M, Gracey J, López-Sobrino T, Massberg S, et al. Venoarterial extracorporeal membrane oxygenation or standard care in patients with cardiogenic shock complicating acute myocardial infarction: the multicentre, randomised EURO SHOCK trial. EuroIntervention J Eur Collab Work Group Interv Cardiol Eur Soc Cardiol. (2023) 19(6):482–92. doi: 10.4244/EIJ-D-23-00204
149. Burkhoff D, Sayer G, Doshi D, Uriel N. Hemodynamics of mechanical circulatory support. J Am Coll Cardiol. (2015) 66(23):2663–74. doi: 10.1016/j.jacc.2015.10.017
150. Dickstein ML. The starling relationship and veno-arterial ECMO: ventricular distension explained. ASAIO J. (2018) 64(4):497. doi: 10.1097/MAT.0000000000000660
151. Truby LK, Takeda K, Mauro C, Yuzefpolskaya M, Garan AR, Kirtane AJ, et al. Incidence and implications of left ventricular distention during venoarterial extracorporeal membrane oxygenation support. ASAIO J Am Soc Artif Intern Organs 1992. (2017) 63(3):257–65. doi: 10.1097/MAT.0000000000000553
152. Singh R, Chandel A, Paras J, Lee TB, Tang DG, Shah P, et al. Transapical cannulation with a dual lumen cannula for mechanical circulatory support in cardiogenic shock. ASAIO J Am Soc Artif Intern Organs 1992. (2022) 68(11):e215–9. doi: 10.1097/MAT.0000000000001683
153. Balthazar T, Vandenbriele C, Verbrugge FH, Den Uil C, Engström A, Janssens S, et al. Managing patients with short-term mechanical circulatory support: jACC review topic of the week. J Am Coll Cardiol. (2021) 77(9):1243–56. doi: 10.1016/j.jacc.2020.12.054
154. Vandenbriele C, Arachchillage DJ, Frederiks P, Giustino G, Gorog DA, Gramegna M, et al. Anticoagulation for percutaneous ventricular assist device-supported cardiogenic shock. J Am Coll Cardiol. (2022) 79(19):1949–62. doi: 10.1016/j.jacc.2022.02.052
155. Fabrizio C, Levito MN, Rivosecchi R, Bashline M, Slocum B, Kilic A, et al. Outcomes of systemic anticoagulation with bivalirudin for impella 5.0. Int J Artif Organs. (2021) 44(10):681–6. doi: 10.1177/03913988211032238
156. Dietrich JN, Kazmi H. Bleeding risks in patients on percutaneous ventricular assist devices receiving two different dextrose concentrations of heparinized purge solution: a case series. J Pharm Pract. (2019) 32(4):464–9. doi: 10.1177/0897190018757148
157. Niebler RA, Parker H, Hoffman GM. Impact of anticoagulation and circuit technology on complications during extracorporeal membrane oxygenation. ASAIO J Am Soc Artif Intern Organs 1992. (2019) 65(3):270–6. doi: 10.1097/MAT.0000000000000811
158. Arnouk S, Altshuler D, Lewis TC, Merchan C, Smith DE, Toy B, et al. Evaluation of anti-Xa and activated partial thromboplastin time monitoring of heparin in adult patients receiving extracorporeal membrane oxygenation support. ASAIO J Am Soc Artif Intern Organs 1992. (2020) 66(3):300–6. doi: 10.1097/MAT.0000000000001004
159. Price EA, Jin J, Nguyen HM, Krishnan G, Bowen R, Zehnder JL. Discordant aPTT and anti-Xa values and outcomes in hospitalized patients treated with intravenous unfractionated heparin. Ann Pharmacother. (2013) 47(2):151–8. doi: 10.1345/aph.1R635
160. Nuqali A, Goyal A, Acharya P, Mastoris I, Dalia T, Chan W-C, et al. Thirty-day readmissions among patients with cardiogenic shock who underwent extracorporeal membrane oxygenation support in the United States: insights from the nationwide readmissions database. Am Heart J Plus Cardiol Res Pract. (2022) 13:100076. doi: 10.1016/j.ahjo.2021.100076
161. Shah M, Patil S, Patel B, Agarwal M, Davila CD, Garg L, et al. Causes and predictors of 30-day readmission in patients with acute myocardial infarction and cardiogenic shock. Circ Heart Fail. (2018) 11(4):e004310. doi: 10.1161/CIRCHEARTFAILURE.117.004310
162. Shah M, Patel B, Tripathi B, Agarwal M, Patnaik S, Ram P, et al. Hospital mortality and thirty day readmission among patients with non-acute myocardial infarction related cardiogenic shock. Int J Cardiol. (2018) 270:60–7. doi: 10.1016/j.ijcard.2018.06.036
163. Aissaoui N, Puymirat E, Simon T, Bonnefoy-Cudraz E, Angoulvant D, Schiele F, et al. Long-term outcome in early survivors of cardiogenic shock at the acute stage of myocardial infarction: a landmark analysis from the French registry of acute ST-elevation and non-ST-elevation myocardial infarction (FAST-MI) registry. Crit Care Lond Engl. (2014) 18(5):516. doi: 10.1186/s13054-014-0516-y
164. Sterling LH, Fernando SM, Talarico R, Qureshi D, Van Diepen S, Herridge MS, et al. Long-Term outcomes of cardiogenic shock complicating myocardial infarction. J Am Coll Cardiol. (2023) 82(10):985–95. doi: 10.1016/j.jacc.2023.06.026
165. Warraich HJ, Hernandez AF, Allen LA. How medicine has changed the End of life for patients with cardiovascular disease. J Am Coll Cardiol. (2017) 70(10):1276–89. doi: 10.1016/j.jacc.2017.07.735
166. Sidebottom AC, Jorgenson A, Richards H, Kirven J, Sillah A. Inpatient palliative care for patients with acute heart failure: outcomes from a randomized trial. J Palliat Med. (2015) 18(2):134–42. doi: 10.1089/jpm.2014.0192
167. Lemond L, Allen LA. Palliative care and hospice in advanced heart failure. Prog Cardiovasc Dis. (2011) 54(2):168–78. doi: 10.1016/j.pcad.2011.03.012
168. Feng Z, Fonarow GC, Ziaeian B. Palliative care services in patients admitted with cardiogenic shock in the United States: frequency and predictors of 30-day readmission. J Card Fail. (2021) 27(5):560–7. doi: 10.1016/j.cardfail.2021.01.020
169. Vallabhajosyula S, Prasad A, Dunlay SM, Murphree DH, Ingram C, Mueller PS, et al. Utilization of palliative care for cardiogenic shock complicating acute myocardial infarction: a 15-year national perspective on trends, disparities, predictors, and outcomes. J Am Heart Assoc Cardiovasc Cerebrovasc Dis. (2019) 8(15):e011954. doi: 10.1161/JAHA.119.011954
170. Morici N, Frea S, Bertaina M, Sacco A, Corrada E, Dini CS, et al. SCAI stage reclassification at 24 h predicts outcome of cardiogenic shock: insights from the altshock-2 registry. Catheter Cardiovasc Interv. (2023) 101(1):22–32. doi: 10.1002/ccd.30484
171. van der Sijs H, Aarts J, Vulto A, Berg M. Overriding of drug safety alerts in computerized physician order entry. J Am Med Inform Assoc JAMIA. (2006) 13(2):138–47. doi: 10.1197/jamia.M1809
172. Kalra S, Ranard LS, Memon S, Rao P, Garan AR, Masoumi A, et al. Risk prediction in cardiogenic shock: current state of knowledge, challenges and opportunities. J Card Fail. (2021) 27(10):1099–110. doi: 10.1016/j.cardfail.2021.08.003
173. Pöss J, Köster J, Fuernau G, Eitel I, Waha S, de Ouarrak T, et al. Risk stratification for patients in cardiogenic shock after acute myocardial infarction. J Am Coll Cardiol. (2017) 69(15):1913–20. doi: 10.1016/J.JACC.2017.02.027
174. Jentzer JC, Soussi S, Lawler PR, Kennedy JN, Kashani KB. Validation of cardiogenic shock phenotypes in a mixed cardiac intensive care unit population. Catheter Cardiovasc Interv. (2022) 99(4):1006–14. doi: 10.1002/ccd.30103
175. Jentzer JC, Naidu SS, Bhatt DL, Stone GW. Mechanical circulatory support devices in acute myocardial infarction-cardiogenic shock: current studies and future directions. J Soc Cardiovasc Angiogr Interv. (2023) 2(2):100586. doi: 10.1016/j.jscai.2023.100586
176. Sinha SS, Katz JN, Morrow DA. Cultivating the research landscape for critical care cardiology: the case for registry-based randomized controlled trials. Circulation. (2023) 147(22):1637–9. doi: 10.1161/CIRCULATIONAHA.122.060802
177. Herridge MS, Azoulay É. Outcomes after critical illness. N Engl J Med. (2023) 388(10):913–24. doi: 10.1056/NEJMra2104669
178. Sleeper LA, Ramanathan K, Picard MH, LeJemtel TH, White HD, Dzavik V, et al. Functional status and quality of life after emergency revascularization for cardiogenic shock complicating acute myocardial infarction. J Am Coll Cardiol. (2005) 46(2):266–73. doi: 10.1016/j.jacc.2005.01.061
179. Smith C, Bellomo R, Raman JS, Matalanis G, Rosalion A, Buckmaster J, et al. An extracorporeal membrane oxygenation–based approach to cardiogenic shock in an older population. Ann Thorac Surg. (2001) 71(5):1421–7. doi: 10.1016/S0003-4975(00)02504-2
180. Ceglarek U, Schellong P, Rosolowski M, Scholz M, Willenberg A, Kratzsch J, et al. The novel cystatin C, lactate, interleukin-6, and N-terminal pro-B-type natriuretic peptide (CLIP)-based mortality risk score in cardiogenic shock after acute myocardial infarction. Eur Heart J. (2021) 42(24):2344–52. doi: 10.1093/eurheartj/ehab110
Keywords: acute myocardial infarction, heart failure, cardiogenic shock, pulmonary artery catheter, shock team
Citation: Mehta A, Vavilin I, Nguyen AH, Batchelor WB, Blumer V, Cilia L, Dewanjee A, Desai M, Desai SS, Flanagan MC, Isseh IN, Kennedy JLW, Klein KM, Moukhachen H, Psotka MA, Raja A, Rosner CM, Shah P, Tang DG, Truesdell AG, Tehrani BN and Sinha SS (2024) Contemporary approach to cardiogenic shock care: a state-of-the-art review. Front. Cardiovasc. Med. 11:1354158. doi: 10.3389/fcvm.2024.1354158
Received: 12 December 2023; Accepted: 13 February 2024;
Published: 13 March 2024.
Edited by:
Lauren Cooper, Northwell Health, United StatesReviewed by:
Matthew Pierce, Northwell Health, United StatesStefania Sacchi, San Raffaele Scientific Institute (IRCCS), Italy
© 2024 Mehta, Vavilin, Nguyen, Batchelor, Blumer, Cilia, Dewanjee, Desai, Desai, Flanagan, Isseh, Kennedy, Klein, Moukhachen, Psotka, Raja, Rosner, Shah, Tang, Truesdell, Tehrani and Sinha. This is an open-access article distributed under the terms of the Creative Commons Attribution License (CC BY). The use, distribution or reproduction in other forums is permitted, provided the original author(s) and the copyright owner(s) are credited and that the original publication in this journal is cited, in accordance with accepted academic practice. No use, distribution or reproduction is permitted which does not comply with these terms.
*Correspondence: Shashank S. Sinha Shashank.Sinha@inova.org
†These authors have contributed equally to this work and share senior authorship.
Abbreviations AMI, acute myocardial infarction; CS, cardiogenic shock; HF, heart failure; tMCS, temporary mechanical circulatory support; IABP, intra-aortic balloon pump; ECMO, extra-corporeal membrane oxygenation; PAC, pulmonary artery catheter.