- 1Division of Cardiology, Cardiovascular and Thoracic Department, “Città della Salute e della Scienza” Hospital, Turin, Italy
- 2Department of Medical Sciences, University of Turin, Turin, Italy
- 3Division of Cardiology, Santa Croce e Carle Hospital, Cuneo, Italy
- 4Department of Cardiology, Civic Hospital of Chivasso, Chivasso, Italy
Background: Recognizing etiology is essential for treatment and secondary prevention of cerebral ischemic events. A magnetic resonance imaging (MRI) pattern suggestive of an embolic etiology has been described but, to date, there are no uniformly accepted criteria.
Aim: The purpose of the study is to describe MRI features of ischemic cerebral lesions occurring after transcatheter ablation of atrial fibrillation (AF).
Methods: A systematic review and meta-analysis of studies performing brain imaging investigations before and after AF transcatheter ablation was performed. The incidence of cerebral ischemic lesions after AF transcatheter ablation was the primary endpoint. The co-primary endpoints were the prevalence of the different neuroimaging features regarding the embolic cerebral ischemic lesions.
Results: A total of 25 studies, encompassing 3,304 patients, were included in the final analysis. The incidence of ischemic cerebral lesions following AF transcatheter ablation was 17.2% [95% confidence interval (CI) 12.2%–23.8%], of which a minimal fraction was symptomatic [0.60% (95% CI 0.09%–3.9%)]. Only 1.6% of the lesions (95% CI 0.9%–3.0%) had a diameter >10 mm, and in 20.5% of the cases the lesions were multiple (95% CI 17.1%–24.4%). Brain lesions were equally distributed across the two hemispheres and the different lobes; cortical location was more frequent [64.0% (95% CI 42.9%–80.8%)] while the middle cerebral artery territory was the most involved 37.0% (95% CI 27.3–48.0).
Conclusions: The prevailing MRI pattern comprises a predominance of small (<10 mm) cortical lesions, more prevalent in the territory of the middle cerebral artery.
Introduction
Stroke is a leading cause of mortality and long-term disability (1); recognizing the underlying cause of stroke is relevant for treatment, prognosis, and secondary prevention (2). However, about 25% of strokes are defined “cryptogenic” because the etiology remains unknown in spite of exhaustive investigations (3). Hart et al. recently proposed to call these types of lesions “embolic strokes of undetermined source” (ESUS) (4), considering that the vast majority recognizes an embolic etiology. Detecting a potential mechanism would, indeed, be relevant, when therapeutic options aiming at preventing recurrences are available, such as the initiation of oral anticoagulation in case of atrial fibrillation (AF) or percutaneous closure in case of suspected paradoxical embolism through a patent foramen oval (PFO).
With the development of computerized tomography (CT) and magnetic resonance imaging (MRI) techniques, a specific imaging pattern of brain lesions for every etiologic type of acute stroke has been hypothesized. A number of studies have concluded that in cardioembolic strokes, lesions are mostly multiple and cortical in location (5–7), but a systematic description of the neuroimaging features associated with a cardioembolic genesis of ischemic strokes is presently lacking. In the past two decades, silent cerebral lesions have been described at MRI following AF transcatheter ablation (8). These lesions have been carefully identified by comparing imaging before and after the procedure with MRI diffusion-weighted imaging (DWI) sequences, the most sensitive technique for the detection of acute cerebral ischemia (9).
Because during an uncomplicated transcatheter AF ablation the patient does not experience hemodynamic compromise, we hypothesize that the pathophysiological mechanism underlying brain lesions is embolic, and not related to hypoperfusive genesis. The major mechanisms involved include endothelium damage at transseptal puncture, conventional clotting (e.g., from the groin) and crossed emboli in the iatrogenic interatrial septum defect, and thermal thrombus formation in the left atrium (charring and gas embolism). In addition, during a transcatheter ablation the introduction of bulky devices, such as multielectrode catheters, and balloons carries a risk of air embolism (10).
In the present study we conducted a systematic review and meta-analysis to describe the neuroimaging features of newly formed cardioembolic lesions after AF transcatheter ablation.
Methods
This work was conducted following the Preferred Reporting Items for Systematic reviews and Meta-Analyses (PRISMA) guidelines (11).
Search strategy
Pertinent articles were searched in MEDLINE/PubMed with MeSH strategy, using the following terms: ((cerebral lesion* OR stroke OR silent cerebral lesion OR SCI embolism) AND cerebral MRI AND (Atrial fibrillation ablation OR Fib ablation OR AF ablation)). The search was ended on 30 January 2023.
Study selection and data extraction
Two independent reviewers (EB, MB) screened the retrieved citations through title and/or abstract. When potentially pertinent, the studies were appraised as complete reports according to the following inclusion/exclusion criteria:
• They reported the absolute number of new ischemic cerebral lesions that occurred after AF transcatheter ablation; this implies that the included studies were designed to perform cerebral MRI scan prior to and immediately after the ablation procedure.
• They reported at least one of the evaluated characteristics of the ischemic cerebral lesions (please refer to the following section regarding the specific study endpoints for detailed description of evaluated features).
Exclusion criteria were non-human setting; duplicate reporting (in which case, the manuscript reporting the largest sample of patients was selected).
Two independent, unblinded reviewers (EB and MB) abstracted the following data on prespecified forms: authors, journal, year of publication, baseline clinical and interventional features, cerebral MRI protocol, and neuroimaging features. Data collection was conducted by mutual agreement and all potential disagreement was resolved by a third reviewer (AS).
Study endpoints
The incidence of new cerebral ischemic lesions after AF transcatheter ablation was the primary endpoint. The co-primary endpoints were the prevalence of the following neuroimaging features regarding new cerebral ischemic lesions:
• Lesion dimension: size > 10 mm;
• Multiple lesions;
• Left vs. right hemisphere location;
• Cortical, subcortical (white matter and/or basal ganglia), or cerebellar location;
• Involved anatomical lobe: frontal, parietal, occipital, temporal;
• Involved vascular territory: anterior cerebral artery (ACA), middle cerebral artery (MCA), posterior cerebral artery (PCA), and border zone (BZ).
The secondary endpoint was the incidence of symptomatic cerebral ischemic events (stroke/transient ischemic attack).
Statistical analysis
The baseline characteristics of the pooled study populations were reported as median values and their interquartile ranges (IQRs). The meta-analysis of the proportions (crude incidence of new cerebral ischemic lesions and prevalence of the prespecified neuroimaging features) was performed using a generalized linear mixed models (12) under a random-effect framework and the results were reported together with the corresponding 95% confidence interval (CI). Cochrane I2 test was used to investigate heterogeneity, with I2 values of 25%, 50%, and 75% representing, respectively, mild, moderate, and extensive heterogeneity. Statistical analyses were performed with R version 4.0.0 (R Foundation for Statistical Computing, Vienna, Austria) and p-values less than 0.05 were considered statistically significant.
Results
The initial search retrieved 125 studies. Among these, three studies were removed because of duplication, and one study was not assessed for eligibility because of the unavailability of the English translation. Given that 39 studies were not pertinent to the topic of the meta-analyses, 82 studies were assessed for eligibility, and 25 eventually included (13–37). Figure 1 reports the detailed PRISMA flowchart of the selection process. The resulting meta-analytic population encompassed 3,304 patients who had undergone AF transcatheter ablation and pre- and post-procedure cerebral MRI scans to detect new ischemic lesions. More details regarding the included studies, in particular concerning the adopted cerebral MRI protocol, the ablation technique, and the energy source are reported in Table 1. The AF type was paroxysmal in the majority of the patients (69%, IQR 59%–97%). The median age was 61 (IQR 58–63) years with a nearly 2:1 male-to-female ratio (males 68%, IQR 63–75).
The incidence of new ischemic cerebral lesions in patients that had undergone AF transcatheter ablation was 17.2% (95% CI 12.2%–23.8%; I2: 93%) (Figure 2A). Among these lesions, only a minimal proportion had ischemic symptoms [0.60% (95% CI 0.09%–3.9%; I2: 56%)] (Figure 2B).
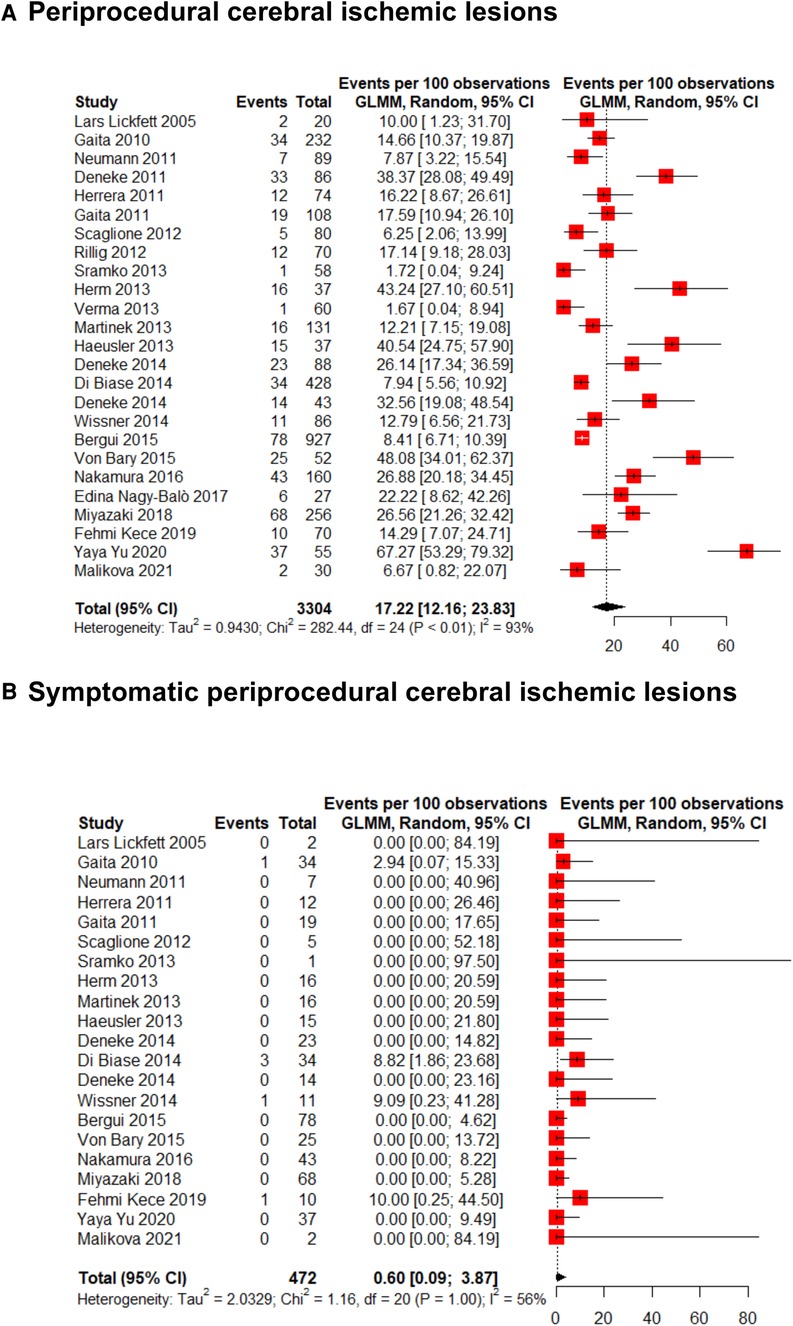
Figure 2. Forest plot for (A) overall and (B) symptomatic periprocedural cerebral ischemic lesions incidence.
Concerning the neuroimaging features of the new cerebral ischemic lesions, the size, reported in 16 studies (evaluating 778 lesions), had a diameter of above 10 mm in 1.6% (95% CI 0.9%–3.0%; I2: 10%) of the cases (Figure 3A). Multiple lesions, described in 13 studies (464 lesions), were reported in 20.5% (95% CI 17.1%–24.4%; I2: 0%) of the scans (Figure 3B). The pooled prevalence of left hemisphere location, reported in 14 studies (667 lesions), was 42.3% (95% CI 31.7%–53.7%; I2: 83%) (Figure 3C). The cortical location (reported in 13 studies, 661 lesions) showed the highest pooled prevalence [64.0% (95% CI 42.9%–80.8%; I2: 94%); Figure 3D] while lesions were, instead, subcortical (5 studies, 266 lesions) or cerebellar (12 studies, 627 lesions) in 25.5% (95% CI 7.1%–60.7%; I2: 91%; Supplementary Figure S1) and 15.2% (95% CI 9.7%–23.1%; I2: 75%; Supplementary Figure S2) of the cases, respectively. The pooled prevalence of frontal lobe location (13 studies, 627 lesions) was 19.7% (95% CI 14.3%–26.5%; I2: 63%; Figure 3E), while parietal (12 studies, 595 lesions), occipital (12 studies, 593 lesions), and temporal (10 studies, 537 lesions) lobes were involved in 17.1% (95% CI 9.3%–29.5%; I2: 87%; Supplementary Figure S3), 12.1% (95% CI 8.9%–16.1%; I2: 29%; Supplementary Figure S4), and 8.6% (95% CI 3.5%–19.8%; I2: 79%; Supplementary Figure S5) of the scans, respectively. The vascular territory of the MCA was the most commonly involved (3 studies, 81 lesions), reported in 37.0% (95% CI 27.3%–48.0%; I2: 0%; Figure 3F) of the cases. ACA (3 studies, 242 lesions), PCA (3 studies, 242 lesions), and border zone (3 studies, 108 lesions) territories were involved in 28.5% (95% CI 23.2%–34.5%; I2: 0%; Supplementary Figure S6), 28.1% (95% CI 22.8%–34.1%; I2: 0%; Supplementary Figure S7), and 22.9% (95% CI 8.5%–48.8%; I2: 81%; Supplementary Figure S8) of the scans, respectively.
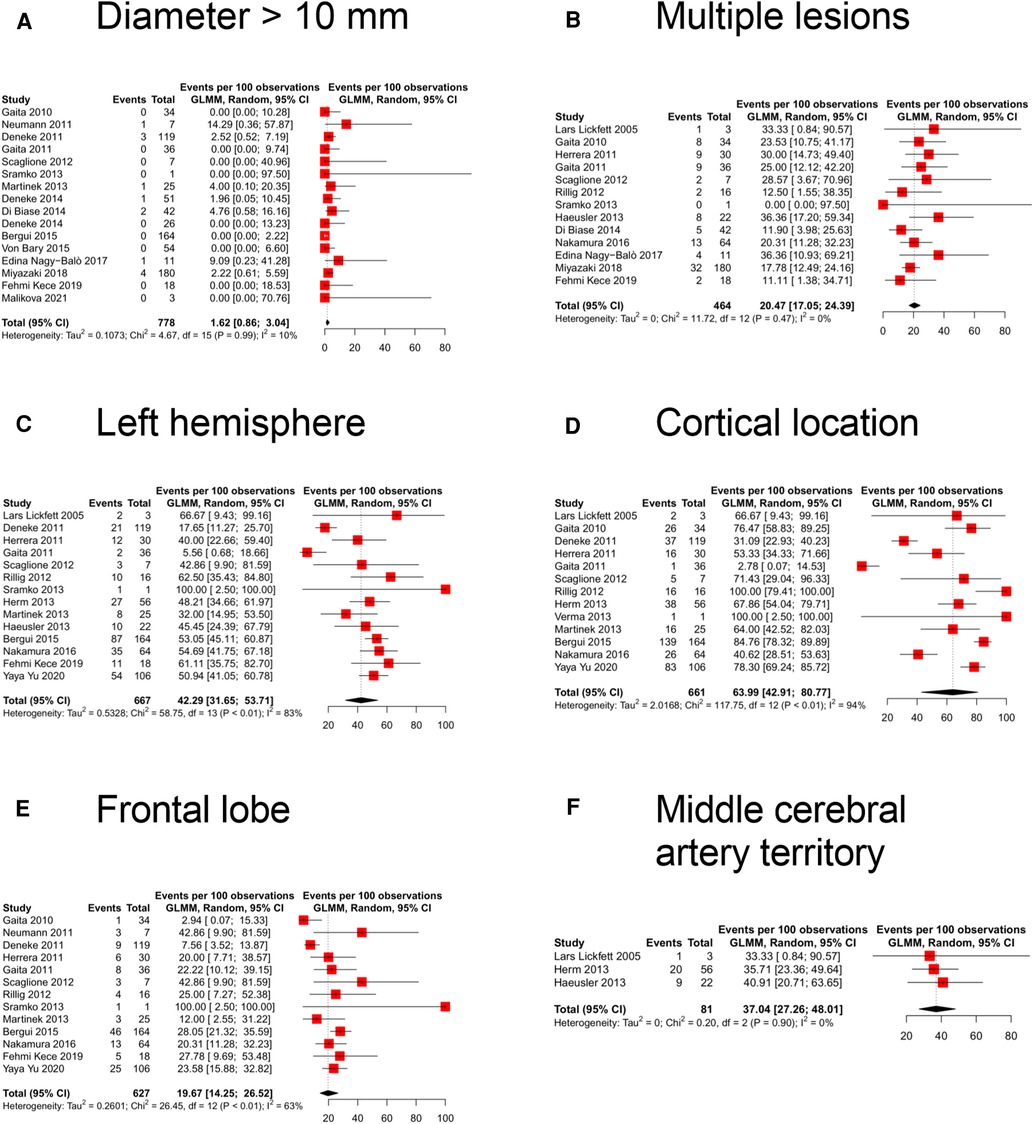
Figure 3. Forest plot of the different neuroimaging features: (A) diameter more than 10 mm, (B) multiple lesions, (C) left hemisphere location, (D) cortical location, (E) frontal lobe location, and (F) middle cerebral artery territory location.
Discussion
The main findings of the present analysis are as follows (Figure 4—Graphical Abstract):
• Among the proportion of patients presenting a cerebral ischemic lesion at cerebral MRI after AF transcatheter ablation (17%), 0.6% are symptomatic from a neurological standpoint.
• These cardioembolic cerebral lesions are generally balanced between the right and the left sides and are ubiquitously detected in all cerebral lobes; the lesions are typically small (diameter less than 10 mm), generally single (multiple only in about 20% of the cases), and they preferentially affect the cerebral cortex (in nearly two-third of the cases) of the MCA vascular territory.
Determining the underlying cause of an acute stroke is important not only to guide patient's immediate management but also to prevent new events, given that stroke recurrence is strongly related to its specific etiology (38–40). Imaging has a primary role in early diagnosis of strokes: in fact, some patterns of brain infarction may suggest a specific cause. In particular, regarding ESUS, early identification of a potential cause underlying the ischemic event might be of paramount importance, since it could expedite the clinical decision process leading to the adoption of therapeutic strategies, such as initiation of oral anticoagulation in case of an AF-related genesis. Moreover, considering that patients with AF frequently present asymptomatic cerebral lesions (41), keeping in mind that AF-related subclinical lesions might be due to several mechanisms (42–47), recognizing a certified neuroimaging pattern suggestive of subclinical AF-related lesions might even help prevent the occurrence of clinically relevant events.
Despite these potential benefits, a systematic description of the neuroimaging features associated with a cardioembolic genesis of ischemic strokes is presently lacking. For this purpose, AF transcatheter ablation can be regarded as an in vivo model of cardioembolic lesions, used to derive a neuroimaging “fingerprint” of typical cardioembolic lesions. In fact, during an uncomplicated transcatheter AF ablation the patient does not experience hemodynamic compromise, making a hypoperfusive genesis (due to transient reduction of cardiac output) of new cerebral ischemic lesions not plausible. Other cardioembolic models, such as cardiac surgery [during which hypotensive episodes might occur (48)] or transcatheter aortic valve replacement [the rapid ventricular pacing performed during valve deployment temporarily reduces cardiac output (49)], certainly do not share this feature.
The present analysis suggests that typical cardioembolic lesions, such as occurring during an AF ablation procedure, tend to be located at a cortical level and, particularly, in the vascular territory of the middle cerebral artery. Cerebral lesions related to a hypoperfusive genesis, instead, more commonly are subcortical and determine watershed infarcts (50–52). Watershed infarcts occur at the border between cerebral vascular territories where the tissue is furthest from arterial supply and thus most vulnerable to hypotension and hypoperfusion, or might exacerbate embolism-related damage (e.g., delayed embolism “washout,” impaired perfusion of ischemic penumbra) (53). In any case, this neuroimaging “fingerprint” differs quite clearly from that of cerebral lesions related to a cardioembolic genesis.
Limitations
The analysis is limited by the inherent limitations of a meta-analysis. In particular, although all studies performed diffusion-weighted imaging sequences to detect new cerebral lesions, hidden technicalities across the different studies cannot be excluded. Moreover, the present results might apply prevalently to a population of paroxysmal AF patients undergoing catheter ablation. In addition, although we selected a cut-off value of 10 mm to distinguish between smaller and larger lesions, we cannot exclude that different cut-offs (e.g., 3 or 5 mm) might be more appropriate and provide different trends. Finally, we cannot directly exclude that the populations included in studies from the same Research Groups might present partial overlaps.
Conclusions
In conclusion, by thoroughly assessing incidence and neuroimaging features of cerebral ischemic lesions following AF transcatheter ablation, it emerges that in predominance they consist of small (<10 mm) cortical lesions, almost ubiquitous in all cerebral lobes and in both hemispheres, prevalent in the territory of the middle cerebral artery.
The present analysis supports the existence of an, at least, suspicious neuroimaging “fingerprint” of cardioembolic brain lesions. If confirmed in specifically designed studies, specific neuroimaging features in de novo cerebral lesions would rapidly prompt a tailored clinical management, shortening time to diagnosis of the underlying etiology, and, potentially, preventing recurrences.
Data availability statement
The raw data supporting the conclusions of this article will be made available by the authors, without undue reservation.
Ethics statement
Ethical approval was not required for the study involving humans in accordance with the local legislation and institutional requirements. Written informed consent to participate in this study was not required from the participants or the participants’ legal guardians/next of kin in accordance with the national legislation and the institutional requirements.
Author contributions
AS: Conceptualization, Data curation, Investigation, Methodology, Writing – original draft, Formal Analysis, Supervision. EB: Data curation, Methodology, Writing – original draft. MB: Data curation, Methodology, Writing – original draft. IF: Conceptualization, Data curation, Methodology, Writing – original draft. CR: Data curation, Methodology, Writing – original draft. FO: Conceptualization, Data curation, Methodology, Supervision, Writing – original draft. GDF: Data curation, Methodology, Supervision, Writing – original draft. MA: Conceptualization, Data curation, Investigation, Methodology, Supervision, Writing – original draft.
Funding
The authors declare that no financial support was received for the research, authorship, and/or publication of this article.
Conflict of interest
The authors declare that the research was conducted in the absence of any commercial or financial relationships that could be construed as a potential conflict of interest.
The authors declared that they were an editorial board member of Frontiers, at the time of submission. This had no impact on the peer review process and the final decision.
Publisher's note
All claims expressed in this article are solely those of the authors and do not necessarily represent those of their affiliated organizations, or those of the publisher, the editors and the reviewers. Any product that may be evaluated in this article, or claim that may be made by its manufacturer, is not guaranteed or endorsed by the publisher.
Supplementary material
The Supplementary Material for this article can be found online at: https://www.frontiersin.org/articles/10.3389/fcvm.2024.1327567/full#supplementary-material
References
1. Tsao CW, Aday AW, Almarzooq ZI, Anderson CAM, Arora P, Avery CL, et al. Heart disease and stroke statistics—2023 update: a report from the American Heart Association. Circulation. (2023) 147(8):e93–e621. doi: 10.1161/CIR.0000000000001123
2. Kolominsky-Rabas PL, Weber M, Gefeller O, Neundoerfer B, Heuschmann PU. Epidemiology of ischemic stroke subtypes according to TOAST criteria: incidence, recurrence, and long-term survival in ischemic stroke subtypes: a population-based study. Stroke. (2001) 32:2735–40. doi: 10.1161/hs1201.100209
3. Jones HR, Caplan LR, Come PC, Swinton NW, Breslin DJ. Cerebral emboli of paradoxical origin. Ann Neurol. (1983) 13:314–9. doi: 10.1002/ana.410130315
4. Hart RG, Diener HC, Coutts SB, Easton JD, Granger CB, O’Donnell MJ, et al. Embolic strokes of undetermined source: the case for a new clinical construct. Lancet Neurol. (2014) 13:429–38. doi: 10.1016/S1474-4422(13)70310-7
5. Roh JK, Kang DW, Lee SH, Yoon BW, Chang KH. Significance of acute multiple brain infarction on diffusion-weighted imaging. Stroke. (2000) 31:688–94. doi: 10.1161/01.STR.31.3.688
6. Wessels T, Wessels C, Ellsiepen A, Reuter I, Trittmacher S, Stolz E, et al. Contribution of diffusion-weighted imaging in determination of stroke etiology. Am J Neuroradiol. (2006) 27:35–9.16418352
7. Doufekias E, Segal AZ, Kizer JR. Cardiogenic and aortogenic brain embolism. J Am Coll Cardiol. (2008) 51:1049–59. doi: 10.1016/j.jacc.2007.11.053
8. Deneke T, Jais P, Scaglione M, Schmitt R, Di Biase L, Christopoulos G, et al. Silent cerebral events/lesions related to atrial fibrillation ablation: a clinical review. J Cardiovasc Electrophysiol. (2015) 26(4):455–63. doi: 10.1111/jce.12608
9. Kang DW, Chalela JA, Ezzeddine MA, Warach S. Association of ischemic lesion patterns on early diffusion-weighted imaging with TOAST stroke subtypes. Arch Neurol. (2003) 60:1730–4. doi: 10.1001/archneur.60.12.1730
10. Anselmino M, Gaita F. Unresolved issues in transcatheter atrial fibrillation ablation: silent cerebrovascular ischemias. J Cardiovasc Electrophysiol. (2013) 24(2):129–31. doi: 10.1111/jce.12018
11. Moher D, Liberati A, Tetzlaff J, Altman DG, Antes G, Atkins D, et al. Preferred reporting items for systematic reviews and meta-analyses: the PRISMA statement. PLoS Med. (2009) 6(7):e1000097. doi: 10.1371/journal.pmed.1000097
12. Lifeng Lin HC. Meta-analysis of proportions using generalized linear mixed models. Epidemiology. (2020) 31(5):713–7. doi: 10.1097/EDE.0000000000001232
13. Lickfett L, Hackenbroch M, Lewalter T, Selbach S, Schwab JO, Yang A, et al. Cerebral diffusion-weighted magnetic resonance imaging: a tool to monitor the thrombogenicity of left atrial catheter ablation. J Cardiovasc Electrophysiol. (2006) 17(1):1–7. doi: 10.1111/j.1540-8167.2005.00279.x
14. Gaita F, Caponi D, Pianelli M, Scaglione M, Toso E, Cesarani F, et al. Radiofrequency catheter ablation of atrial fibrillation: a cause of silent thromboembolism? Circulation. (2010) 122(17):1667–73. doi: 10.1161/circulationaha.110.937953
15. Verma A, Debruyne P, Nardi S, Deneke T, Degreef Y, Spitzer S, et al. Evaluation and reduction of asymptomatic cerebral embolism in ablation of atrial fibrillation, but high prevalence of chronic silent infarction: results of the evaluation of reduction of asymptomatic cerebral embolism trial. Circ Arrhythmia Electrophysiol. (2013) 6(5):835–42. doi: 10.1161/CIRCEP.113.000612
16. Martinek M, Sigmund E, Lemes C, Derndorfer M, Aichinger J, Winter S, et al. Asymptomatic cerebral lesions during pulmonary vein isolation under uninterrupted oral anticoagulation. Europace. (2013) 15(3):325–31. doi: 10.1093/europace/eus329
17. Haeusler KG, Koch L, Herm J, Kopp UA, Heuschmann PU, Endres M, et al. 3 Tesla MRI-detected brain lesions after pulmonary vein isolation for atrial fibrillation: results of the MACPAF study. J Cardiovasc Electrophysiol. (2013) 24(1):14–21. doi: 10.1111/j.1540-8167.2012.02420.x
18. Deneke T, Nentwich K, Schmitt R, Christhopoulos G, Krug J, Di Biase L, et al. Exchanging catheters over a single transseptal sheath during left atrial ablation is associated with a higher risk for silent cerebral events. Indian Pacing Electrophysiol J. (2014) 14(5):240–9. doi: 10.1016/S0972-6292(16)30795-1
19. Di Biase L, Gaita F, Toso E, Santangeli P, Mohanty P, Rutledge N, et al. Does periprocedural anticoagulation management of atrial fibrillation affect the prevalence of silent thromboembolic lesion detected by diffusion cerebral magnetic resonance imaging in patients undergoing radiofrequency atrial fibrillation ablation with open irrigated catheters? Results from a prospective multicenter study. Hear Rhythm. (2014) 11(5):791–8. doi: 10.1016/j.hrthm.2014.03.003
20. Deneke T, Schade A, Müller P, Schmitt R, Christopoulos G, Krug J, et al. Acute safety and efficacy of a novel multipolar irrigated radiofrequency ablation catheter for pulmonary vein isolation. J Cardiovasc Electrophysiol. (2014) 25(4):339–45. doi: 10.1111/jce.12316
21. Wissner E, Metzner A, Neuzil P, Petru J, Skoda J, Sediva L, et al. Asymptomatic brain lesions following laserballoon-based pulmonary vein isolation. Europace. (2014) 16(2):214–9. doi: 10.1093/europace/eut250
22. von Bary C, Deneke T, Arentz T, Schade A, Lehrmann H, Eissnert C, et al. Silent cerebral events as a result of left atrial catheter ablation do not cause neuropsychological sequelae—a MRI-controlled multicenter study. J Interv Card Electrophysiol. (2015) 43(3):217–26. doi: 10.1007/s10840-015-0004-6
23. Bergui M, Castagno D, D’Agata F, Cicerale A, Anselmino M, Maria Ferrio F, et al. Selective vulnerability of cortical border zone to microembolic infarct. Stroke. (2015) 46(7):1864–9. doi: 10.1161/STROKEAHA.114.008194
24. Neumann T, Kuniss M, Conradi G, Janin S, Berkowitsch A, Wojcik M, et al. MEDAFI-trial (micro-embolization during ablation of atrial fibrillation): comparison of pulmonary vein isolation using cryoballoon technique vs. radiofrequency energy. Europace. (2011) 13(1):37–44. doi: 10.1093/europace/euq303
25. Nakamura T, Okishige K, Kanazawa T, Yamashita M, Kawaguchi N, Kato N, et al. Incidence of silent cerebral infarctions after catheter ablation of atrial fibrillation utilizing the second-generation cryoballoon. Europace. (2017) 19(10):1681–8. doi: 10.1093/europace/euw191
26. Nagy-Baló E, Martirosyan M, Sándorfi G, Hajas O, Lánczi L, Berényi E, et al. Cerebral micro-embolization during pulmonary vein isolation: relation to post-ablation silent cerebral ischemia. Cardiol J. (2017) 24(3):234–41. doi: 10.5603/CJ.a2017.0030
27. Miyazaki S, Kajiyama T, Yamao K, Hada M, Yamaguchi M, Nakamura H, et al. Silent cerebral events/lesions after second-generation cryoballoon ablation: how can we reduce the risk of silent strokes? Hear Rhythm. (2019) 16(1):41–8. doi: 10.1016/j.hrthm.2018.07.011
28. Keçe F, Bruggemans EF, de Riva M, Alizadeh Dehnavi R, Wijnmaalen AP, Meulman TJ, et al. Incidence and clinical significance of cerebral embolism during atrial fibrillation ablation with duty-cycled phased-radiofrequency versus cooled-radiofrequency: a randomized controlled trial. JACC Clin Electrophysiol. (2019) 5(3):318–26. doi: 10.1016/j.jacep.2018.11.008
29. Yu Y, Wang X, Li X, Zhou X, Liao S, Yang W, et al. Higher incidence of asymptomatic cerebral emboli after atrial fibrillation ablation found with high-resolution diffusion-weighted magnetic resonance imaging. Circ Arrhythmia Electrophysiol. (2020) 13(1):e007548. doi: 10.1161/CIRCEP.119.007548
30. Malikova H, Kremenova K, Budera P, Herman D, Weichet J, Lukavsky J, et al. Silent strokes after thoracoscopic epicardial ablation and catheter ablation for atrial fibrillation: not all lesions are permanent on follow-up magnetic resonance imaging. Quant Imaging Med Surg. (2021) 11(7):3219–33. doi: 10.21037/qims-21-35
31. Gaita F, Leclercq JF, Schumacher B, Scaglione M, Toso E, Halimi F, et al. Incidence of silent cerebral thromboembolic lesions after atrial fibrillation ablation may change according to technology used: comparison of irrigated radiofrequency, multipolar nonirrigated catheter and cryoballoon. J Cardiovasc Electrophysiol. (2011) 22(9):961–8. doi: 10.1111/j.1540-8167.2011.02050.x
32. Deneke T, Shin DI, Balta O, Bnz K, Fassbender F, Mgge A, et al. Postablation asymptomatic cerebral lesions: long-term follow-up using magnetic resonance imaging. Hear Rhythm. (2011) 8(11):1705–11. doi: 10.1016/j.hrthm.2011.06.030
33. Siklódy CH, Deneke T, Hocini M, Lehrmann H, Shin DI, Miyazaki S, et al. Incidence of asymptomatic intracranial embolic events after pulmonary vein isolation: comparison of different atrial fibrillation ablation technologies in a multicenter study. J Am Coll Cardiol. (2011) 58(7):681–8. doi: 10.1016/j.jacc.2011.04.010
34. Scaglione M, Blandino A, Raimondo C, Caponi D, Di Donna P, Toso E, et al. Impact of ablation catheter irrigation design on silent cerebral embolism after radiofrequency catheter ablation of atrial fibrillation: results from a pilot study. J Cardiovasc Electrophysiol. (2012) 23(8):801–5. doi: 10.1111/j.1540-8167.2012.02298.x
35. Rillig A, Meyerfeldt U, Tilz RR, Talazko J, Arya A, Zvereva V, et al. Incidence and long-term follow-up of silent cerebral lesions after pulmonary vein isolation using a remote robotic navigation system as compared with manual ablation. Circ Arrhythmia Electrophysiol. (2012) 5(1):15–21. doi: 10.1161/CIRCEP.111.967497
36. Sramko M, Peichl P, Wichterle D, Tintera J, Maxian R, Weichet J, et al. A novel biomarker-based approach for the detection of asymptomatic brain injury during catheter ablation of atrial fibrillation. J Cardiovasc Electrophysiol. (2014) 25(4):349–54. doi: 10.1111/jce.12325
37. Herm J, Fiebach JB, Koch L, Kopp UA, Kunze C, Wollboldt C, et al. Neuropsychological effects of MRI-detected brain lesions after left atrial catheter ablation for atrial fibrillation: long-term results of the MACPAF study. Circ Arrhythmia Electrophysiol. (2013) 6(5):843–50. doi: 10.1161/CIRCEP.113.000174
38. Petty GW, Brown RD, Whisnant JP, Sicks JRD, O’Fallon WM, Wiebers DO. Ischemic stroke subtypes: a population-based study of functional outcome, survival, and recurrence. Stroke. (2000) 31(5):1062–8. doi: 10.1161/01.STR.31.5.1062
39. Kolmos M, Christoffersen L, Kruuse C. Recurrent ischemic stroke—a systematic review and meta-analysis. J Stroke Cerebrovasc Dis. (2021) 30(8):105935. doi: 10.1016/j.jstrokecerebrovasdis.2021.105935
40. Adams HP, Bendixen BH, Kappelle LJ, Biller J, Love BB, Gordon DL, et al. Classification of subtype of acute ischemic stroke. Definitions for use in a multicenter clinical trial. TOAST. Trial of org 10172 in acute stroke treatment. Stroke. (1993) 24(1):35–41. doi: 10.1161/01.str.24.1.35
41. Conen D, Rodondi N, Müller A, Beer JH, Ammann P, Moschovitis G, et al. Relationships of overt and silent brain lesions with cognitive function in patients with atrial fibrillation. J Am Coll Cardiol. (2019) 73(9):989–99. doi: 10.1016/j.jacc.2018.12.039
42. Saglietto A, Matta M, Gaita F, Jacobs V, Bunch TJ, Anselmino M. Stroke-independent contribution of atrial fibrillation to dementia: a meta-analysis. Open Hear. (2019) 6(1):e000984. doi: 10.1136/openhrt-2018-000984
43. Anselmino M, Scarsoglio S, Saglietto A, Gaita F, Ridolfi L. Transient cerebral hypoperfusion and hypertensive events during atrial fibrillation: a plausible mechanism for cognitive impairment. Sci Rep. (2016) 6:28635. doi: 10.1038/srep28635
44. Saglietto A, Scarsoglio S, Ridolfi L, Gaita F, Anselmino M. Higher ventricular rate during atrial fibrillation relates to increased cerebral hypoperfusions and hypertensive events. Sci Rep. (2019) 9(1):3779. doi: 10.1038/s41598-019-40445-5
45. Saglietto A, Scarsoglio S, Canova D, Roatta S, Gianotto N, Piccotti A, et al. Increased beat-to-beat variability of cerebral microcirculatory perfusion during atrial fibrillation: a near-infrared spectroscopy study. Europace. (2021) 23(8):1219–26. doi: 10.1093/europace/euab070
46. Scarsoglio S, Saglietto A, Tripoli F, Zwanenburg JJM, Biessels GJ, De Ferrari GM, et al. Cerebral hemodynamics during atrial fibrillation: computational fluid dynamics analysis of lenticulostriate arteries using 7 T high-resolution magnetic resonance imaging. Phys Fluids. (2022) 34(12):121909. doi: 10.1063/5.0129899
47. Saglietto A, Scarsoglio S, Tripoli F, Zwanenburg J, Biessels GJ, De Ferrari GM, et al. Atrial fibrillation hemodynamic effects on lenticulostriate arteries identified at 7-Tesla cerebral magnetic resonance imaging. Clin Transl Med. (2023) 13(9):e1367. doi: 10.1002/ctm2.1367
48. Hogue CW, Gottesman RF, Stearns J. Mechanisms of cerebral injury from cardiac surgery. Crit Care Clin. (2008) 24(1):83–98. doi: 10.1016/j.ccc.2007.09.004
49. Fanning JP, Walters DL, Wesley AJ, Anstey C, Huth S, Bellapart J, et al. Intraoperative cerebral perfusion disturbances during transcatheter aortic valve replacement. Ann Thorac Surg. (2017) 104:1564–8. doi: 10.1016/j.athoracsur.2017.04.053
50. Vanninen R, Äikiä M, Könönen M, Partanen K, Tulla H, Hartikainen P, et al. Subclinical cerebral complications after coronary artery bypass grafting: prospective analysis with magnetic resonance imaging, quantitative electroencephalography, and neuropsychological assessment. Arch Neurol. (1998) 55(5):618–27. doi: 10.1001/archneur.55.5.618
51. Knipp SC, Matatko N, Schlamann M, Wilhelm H, Thielmann M, Forsting M, et al. Small ischemic brain lesions after cardiac valve replacement detected by diffusion-weighted magnetic resonance imaging: relation to neurocognitive function. Eur J Cardio Thoracic Surg. (2005) 28:88–96. doi: 10.1016/j.ejcts.2005.02.043
52. Floyd TF, Shah PN, Price CC, Harris F, Ratcliffe SJ, Acker MA, et al. Clinically silent cerebral ischemic events after cardiac surgery: their incidence, regional vascular occurrence, and procedural dependence. Ann Thorac Surg. (2006) 81:2160–6. doi: 10.1016/j.athoracsur.2006.01.080
Keywords: stroke, imaging, cardioembolic, MRI pattern, atrial fibrillation, ablation
Citation: Saglietto A, Bertello E, Barra M, Ferraro I, Rovera C, Orzan F, De Ferrari GM and Anselmino M (2024) MRI pattern characterization of cerebral cardioembolic lesions following atrial fibrillation ablation. Front. Cardiovasc. Med. 11:1327567. doi: 10.3389/fcvm.2024.1327567
Received: 25 October 2023; Accepted: 9 January 2024;
Published: 24 January 2024.
Edited by:
Richard Hauer, University Medical Center Utrecht, NetherlandsReviewed by:
Florian Straube, Heart Center Munich-Bogenhausen, GermanyBirgitta Velthuis, University Medical Center Utrecht, Netherlands
© 2024 Saglietto, Bertello, Barra, Ferraro, Rovera, Orzan, De Ferrari and Anselmino. This is an open-access article distributed under the terms of the Creative Commons Attribution License (CC BY). The use, distribution or reproduction in other forums is permitted, provided the original author(s) and the copyright owner(s) are credited and that the original publication in this journal is cited, in accordance with accepted academic practice. No use, distribution or reproduction is permitted which does not comply with these terms.
*Correspondence: Andrea Saglietto andrea.saglietto@live.com