- 1Department of Cardiology, The Third People’s Hospital of Chengdu, Chengdu, China
- 2Affiliated Hospital of Southwest Jiaotong University, College of Medicine, Southwest Jiaotong University, Chengdu, China
- 3Cardiovascular Disease Research Institute of Chengdu, Chengdu, China
It has been shown that patients with cancer have a longer expected life duration, benefiting from advanced medical therapy. Meanwhile, the risk of suffering from cardiovascular disease (CVD) has been increasing with ageing. A growing number of studies have elucidated the association between cancer and CVD. Cancer, atrial fibrillation (AF) and coronary artery disease share some common factors and interact with each other, such as obesity, aging, diabetes, and inflammation, but the potential specific mechanism is still unclear. In addition, cancer-specific and therapy-related factors may increase the risk of embolism and bleeding in patients with cancer than in general population. However, current available embolic and bleeding risk scores applied in patients with CVD may not be applicable for risk assessment in cancer patients, which would be difficult for clinicians to select an appropriate antithrombotic regimen and ensure the balance between bleeding and embolism. Moreover, different types of cancer have distinct risks, which may increase the complexity of antithrombotic therapy. In this review, we review the literature related to cancer, AF, and acute coronary syndrome, focusing on the epidemiological status, physiological mechanism, embolism and bleeding risks, and strategies of antithrombotic therapy.
Introduction
Cardiovascular disease and cancer have been considered to be the two leading causes of death in developed countries (1, 2). For decades, with continuous advances in cancer screening, diagnosis and therapies, the number of cancer patients and survivors has increased steadily (2, 3). Due to the increase of life expectancy in cancer patients and the impact of cancer therapies, the risk of cancer patients complicated with coronary arterial disease (CAD) and AF is also increasing. Although risk assessment of embolism and bleeding in patients with AF and ACS is relatively perfect, including embolism and bleeding risk scores (CHA2DS2-VASc score and HAS-BLED score) and relevant guidelines (4, 5), the factor of cancer is not included in these scores. Cancer-specific and therapy-related risk factors may lead to an increased risk of embolism. Moreover, patients with cancer have a higher risk of bleeding than those without cancer (6, 7). Thus, the balance of thrombotic and bleeding risks has become a thorny issue in the antithrombotic therapy of cancer patients with AF and acute coronary syndrome (ACS). Additionally, most cardiovascular randomized controlled trials exclude cancer patients, and there are few relevant guidelines to guide antithrombotic therapy, which is a gap in clinical practice. Therefore, this paper reviews the prevalence, potential pathological mechanism and interaction of AF and ACS and current evidence of antithrombotic therapy in patients with cancer.
Epidemiology
Since the 1990s, cancer-related mortality has steadily declined, which has led to a steady increase of cancer survivors (2, 8, 9). As the second leading cause of death after CVD, cancer has been the leading cause of death in high-income countries (1). In 2020, it is estimated that 19.3 million new cancer cases were diagnosed, in which breast cancer was become the most common diagnosed cancer (11.7%) compared with lung cancer (11.4%), followed by colorectal cancer (10.0%), prostate cancer (7.3%) and gastric cancer (5.6%) (2).
AF is the most common cardiac arrhythmia and affected nearly 59,700 thousand people worldwide until 2019 (9). The lifetime risk of AF is estimated to be one in four in both men and women at age 40 years and older (10). Patients with cancer may have a higher risk of AF than those without cancer. In a prospective study including 1,045 patients (11), the incidence of AF in cancer patients was higher than that in noncancer patients during the mean follow-up time of 16.3 years (HR 2.47; 95%CI: 1.57–3.88). Recently, an American nationwide epidemiological study on 85,423 patients with breast cancer showed that 9,425 patients had AF prior to the diagnosis of breast cancer, and 2,993 patients had new-onset AF within one year after the diagnosis of breast cancer [incidence 3.3%, 95% CI: 3.0%–3.5%, at 1 year; higher rate in the first 60 days (0.6%/month)] (12). ACS is regarded as a cardiovascular complication that requires special attention in cancer patients. Data from the national inpatient sample (NIS) database of 6,563,255 patients with acute myocardial infarction (AMI) between 2004 and 2014 showed that the incidence of cancer was 9%. Among them, prostate cancer, breast cancer, colon cancer and lung cancer are most closely linked with AMI (13). In a prospective, multicenter special project university medical ACS cohort, the incidence of cancer was 7.74% in 2,132 ACS patients (14).
The epidemiological data are relatively limited in cancer patients with AF and ACS. In the analysis of the ENGAGE AF-TIMI 48 Trial (15), a significant relationship between malignancy and CAD was observed. (p = 0.017). In line with this, the baseline of the ORBIT-AF registry shows that the incidence of prior myocardial infarction in cancer patients is 2.7% higher than that in noncancer patients (p = 0.02) (16). In another observational study (17), a difference was found in the prevalence of ACS in AF patients with and without cancer (p < 0.001). In short, it is undeniable that cancer patients have a higher risk of CVD than noncancer patients (18).
Pathophysiology in cancer, AF and ACS
Cross talk between cancer and platelets
Cancer and platelets can interact with each other, which can be simply understood as tumor cell induced platelet activation and aggregation and activated platelets participate in every step of cancer development by promoting tumor growth, angiogenesis, metastasis, and cancer-related thrombosis (19). Circulating tumor cells (CTC) can induce platelet activation and aggregation (tumor cell induced platelet aggregation, TCIPA), leading to tumor thrombi formation (20, 21). In addition, the association between tumor cells and platelet activation and aggregation may also be related to tissue factors (19, 21). In fact, as early as the 19th century, studies have reported a correlation between thrombocytosis and poor prognosis in cancer patients (22). Platelets can promote tumor angiogenesis and vascular remodeling. Platelets contain various angiogenic factors, which can affect angiogenesis and indirectly promote angiogenesis, such as vascular endothelial growth factor (VEGF), fibroblast growth factor, platelet-derived growth factor (PDGF), insulin-like growth factors (IGF-I), tissue growth factor β (TGF-β), platelet factor 4 (PF4) and so on (23). However, studies have found that PF4 has an inhibitory effect on tumor angiogenesis (24). Platelets also play an important role in the invasion and metastasis of tumor cells. Activated platelets can encapsulate CTC through integrin, fibrin, and P-selectin (25, 26). Subsequently, activated platelets secrete adherents and bind to the surface of CTC, protecting them from shear stress and immune cell attacks. In addition, the adhesion molecules expressed by platelets can also help CTC form active and persistent adhesion with the endothelium, promoting extravasation and seeding of metastatic lesions (27).
Cancer and thrombosis
The increased risk of thrombosis in cancer patients may be explained by Virchow's triad, including blood stasis, endothelial injury or vessel walls injury, and hypercoagulability. In cancer patients, each of the three components Virchow's triad that are prone to thrombosis have abnormalities, thus meeting the requirements for prethrombosis or hypercoagulability (28). Mechanical compression and inactivity of tumors may be potential factors which lead to blood stasis and thromboembolism. Long bed rest after cancer treatment surgery is associated with venous thromboembolism (29). The use of anticancer drugs, inflammation and the production of neutrophil extracellular traps may be associated with endothelial damage and activation in cancer patients (30). In addition, potential mechanisms of hypercoagulability in cancer patients may include expression of tissue factors, release of prethrombotic substances, and upregulation of heparinase (30, 31).
Pathogenesis of AF and cancer
The potential mechanism between AF and cancer may be explained by the following assumptions: (1) Cancer-related systemic inflammation may lead to electrical and structural remodeling of the atrium, which causes AF. AF may induce new inflammation, leading to new AF-the so-called “AF causes AF” (32–35). (2) Some factors of cancer, including pain, infection, metabolic abnormalities, emotion, and physical pressure, may cause an imbalance in the autonomic nervous system, which causes AF (36). (3) The occurrence of AF is also associated with cancer therapies that may be linked to mitochondrial dysfunction, oxidative stress, inflammation, left ventricular dysfunction and necrosis (37). A study showed that doxorubicin can induce Ca/calmodulin-dependent protein kinase II (CaMKII)-mediated Ca2+ leakage in the sarcoplasmic reticulum to disrupt intracellular Ca2+ homeostasis. Increased sarcoplasmic reticulum calcium leakage is also a potential mechanism for inducing AF (38). In addition, the risk of AF may be increased by ibrutinib (39, 40), potentially through inhibition of cardiac PI3K-AKT signaling and production of reactive oxygen species (ROS).
Anticancer therapy-related coronary artery disease
Cancer therapy-related modalities (e.g., chemotherapy, radiotherapy, immunosuppressive therapy, and targeted therapy) are associated with an increased risk of CAD.
Platinum may induce cardiotoxicity due to endothelial injury, platelet aggregation, and thrombosis (41, 42). The mechanism of cardiotoxicity induced by fluorouracil is unknown. However, coronary thrombosis, coronary vasospasm, vascular endothelial injury, direct cardiotoxicity, and oxidative stress have been proposed (43–45).
Targeted drugs may increase the risk of CAD by damaging the vascular endothelium, arterial thrombosis, and vasospasm (46). Vascular endothelial growth factor (VEGF) can promote cell proliferation, endothelial cell migration and angiogenesis. Some anti-VEGF drugs, such as bevacizumab, sorafenib, and sunitinib, may reduce endothelial cell function, contributing to the increased risk of endothelial cell injury and thrombotic events (47). Sorafenib can also cause CAD by inducing vasospasm (48).
Radiotherapy may cause vascular endothelial damage by activating lysosomal enzymes in the intima and media of blood vessels, which can promote the formation of cholesterol plaques after a few days of radiotherapy (49, 50). In addition, radiotherapy may also cause fibrosis of the intima, media, and adventitia of blood vessels, thus accelerating the development of arteriosclerosis (51, 52).
The potential common mechanism among cancer, AF and CAD
Cancer, AF and CAD share common risk factors (53), including smoking, obesity, diabetes, hypertension and lack of exercise. Obesity is regarded as the main risk factor for CVD (54). A study showed that 20% of all cancer patients may be related to weight gain and obesity (55). Insulin resistance, dyslipidemia and inflammation commonly occur in obese patients and can lead to CVD and cancer (56–59). Diabetes not only increases the risk of CVD by 2–5-fold (60) but can also increase the risk of cancer, especially colorectal cancer (61). Diabetes mellitus may induce cancer through a variety of factors, including excessive reactive oxygen species (ROS) formation, oxidative stress, chronic inflammation, and mitochondrial dysfunction (62). Smoking is closely related to the development of CVD. Tobacco ingredients, including carbon monoxide and oxidants, may increase oxidative stress and reduce the level of nitric oxide that causes endothelial damage, inflammation, and insulin resistance to affect atherosclerosis (63–65). Smoking can also induce cancer through ROS and oxidative damage (66).
Inflammation exists in many diseases, including CVD and cancer (67–69). In 1863, Virchow found white blood cells in tumor tissue and proposed the hypothesis that cancer may originate from chronic inflammation (70). Cancer, AF, and CAD may be linked to inflammation (32, 67, 68). The NLRP3 (Nacht, LRR, and PYD domain containing protein 3) inflammasome can be activated by AF and mediate the release of IL-1B, which leads to the fibrosis of cardiomyocytes, accelerating atrial remodeling and causing the generation of new AF (71, 72). Animal models have also demonstrated that the NLRP3 inflammasome and IL-1 promote arteriosclerosis and increase arterial thrombosis (73–75). IL-1 can promote tumor proliferation and increase the invasiveness of cancer cells in chronic inflammation. IL-6 is secreted by activated monocyte macrophages. In the atrium, there was a significant positive correlation between IL-6 levels and extracellular matrix volume in patients with AF (76). The increase in the interstitial extracellular matrix is associated with atrial remodeling, which may maintain AF (77). Meanwhile, IL-6 has also been confirmed to be associated with vascular endothelial injury and atherosclerosis (78, 79).
Risk of embolism and bleeding in cancer patients comorbid with AF and ACS
Thromboembolism
Cancer was not included in the embolism risk score, including the CHA2DS2-VASc score (80) (congestive heart failure, hypertension, 75 years old or older, diabetes, stroke/transient ischemic attack/thromboembolism, vascular disease, 65–74 years old, sex) and ATRIA score (81) (previous ischemic stroke, age, female, diabetes, congestive heart failure, hypertension, proteinuria, eGFR < 45 or ESRD). A study comparing the usefulness of the CHADS2 or CHA2DS2-VASc score in AF patients diagnosed with cancer showed that the CHADS2 score is more predictive of increased stroke risk in AF patients with cancer than the CHA2DS2-VASc score, which is also the largest study to analyze stroke risk in AF patients with cancer (82).
The risk of embolism in AF patients with cancer is not certain. On the one hand, cancer may not increase the risk of embolism in AF patients. Data from the ARISTOTLE trial (83) showed that no significant difference was observed in the risk of stroke/systemic embolism (SE) in AF patients with or without cancer, however, the superior efficacy and safety of apixaban vs. warfarin were consistent. A similar result was obtained from the ROCKET-AF trial: it seemed that cancer status had little association with stroke in AF patients (76). However, cancer increases the risk of embolism in AF patients. In a prospective study with nonvalvular AF patients (84), the incidence of thromboembolic events (ischemic stroke/transient ischemic attack and SE) in cancer patients was higher than that in noncancer patients (adjusted HR 2.58, 95% CI 1.08–6.16, p = 0.033). Similar to a French observational, retrospective cohort study including 2,435,541 AF patients (85), pancreatic cancer and breast cancer patients had a higher risk of thromboembolism than noncancer patients [IRR (1.2; 95%CI, 1.0–1.4) p = 0.02; (1.1; 95%CI, 1.0–1.2) p = 0.0002, respectively].
Cancer patients are more likely to have a higher risk of recurrent myocardial infarction after percutaneous coronary intervention (PCI). Data from the US National readmission database showed that the 90-day readmission rate for acute myocardial infarction (AMI) after PCI was significantly increased in active cancer (86), including nonmetastatic cancer (OR 1.28, 95%CI: 1.20–1.37, p < 0.001) and metastatic cancer (OR 1.63, 95%CI: 1.38–1.93, p < 0.001). In the subgroup analysis, AMI readmission rates within 90 days were higher in patients with active cancer (12.1% for lung cancer, 10.8% for colon cancer, 7.5% for breast cancer, 7.0% for prostate cancer, and 9.1% for all cancers) than in patients without cancer (5.6%) (p < 0.05). Malignancy was the strongest predictor of stent thrombosis in ACS patients undergoing PCI (87). Another study exploring the risk of thromboembolism after PCI in cancer patients also showed that during the 5-year follow-up period (88), cancer patients had a higher incidence of MI after PCI than noncancer patients (16.1% vs. 8.0%; HR 2.10; 95% CI: 1.49–2.96; p < 0.001). Other scores for evaluating ACS prognosis also do not include cancer (89).
It is not self-evident that the risk of embolism in AF patients with cancer may be different from what we imagine. The risk of thromboembolism may be higher in specific cancers (e.g., lung cancer, pancreatic cancer, breast cancer). In addition, although our commonly used AF score does not include cancer, it still has some predictive power.
Bleeding
Cancer is also not included in the common bleeding scores, including the HAS-BLED score (90), ORBIT (91) and ATRIA (81) bleeding risk score. Although cancer is considered an independent predictor of major bleeding in the complete ORBIT bleeding model, cancer is not included in the final 5-factor score (91). An observational retrospective cohort study, including 399,344 patients with AF and cancer comparing the role of HAS-BLED, ORBIT and ATRIA bleeding risk scores, showed that three scores were significantly linked to major bleeding, GI bleeding, and intracranial hemorrhage (ICH) (92). Moreover, the HAS-BLED score performed better than other scores in ICH prediction, while the ORBIT score showed the best prediction for major bleeding and GI bleeding (p < 0.001 for all AUC comparisons) (92). In a Swedish national study to validate the PRECISE-DAPT score, the PRECISE-DAPT score could predict major bleeding in the cancer group but with only poor or moderate discriminative capability (c-statistic 0.59; 95%CI, 0.53–0.66) (93). A retrospective registry-based cohort study of Spain including 1,137 patients with AF and cancer compared the predictive efficacy of HAS-BLED score, ATRIA score, and HEMORR2HAGES score on bleeding risk. The result showed that all scores were poor in patients with cancer (c-statistic <0.6 and Brier score >0.1). HAS-BLED score (c-statistic 0.56 and Brier score 0.17) has better predictive performance, compared to ATRIA score (c-statistic 0.55 and Brier score 0.17) and HEMORR2HAGES score (c-statistic 0.54 and Brier score 0.17) (94). Available bleeding risk scores are shown in Table 1.
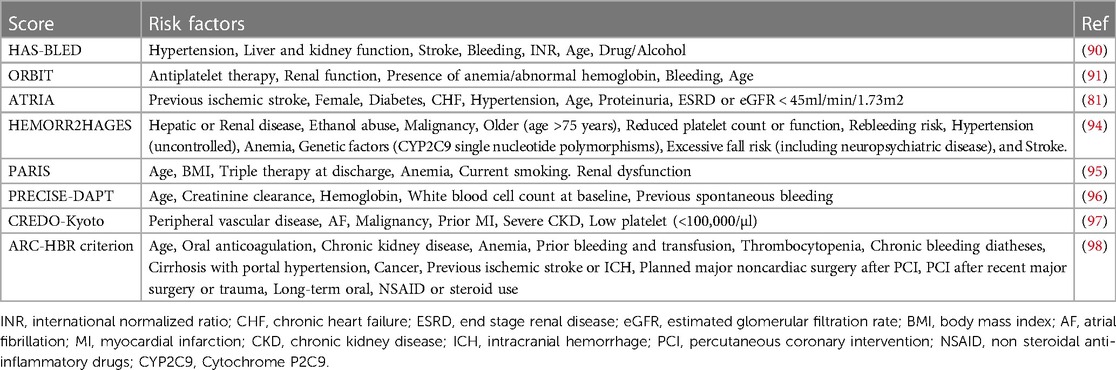
Table 1. Current available bleeding risk scores for patients with cardiovascular disease under antithrombotic therapy.
It has been reported that the occurrence of cancer can increase the risk of bleeding in AF patients (15, 16). In a large cohort of AF patients, the authors found that the risk of ICH increased significantly in patients with prostate cancer (adjusted HR 1.31; 95%CI: 1.06–1.62), and the risk of gastrointestinal (GI) bleeding was higher in patients with colorectal, prostate, ovarian, pancreatic and metastatic cancer as well as myeloma after stopping anticoagulation (99). Similarly, a prospective study also showed that AF patients with cancer had a statistically significant increase in the risk of major bleeding (MB) (adjusted HR 2.02; 95%CI: 1.25–3.27) (84). Surprisingly, Ording et al. found that total cancer status did seem to have no significant association with bleeding risk in AF and cancer patients, whether receiving VKA or NOAC treatment (100). In line with this, a nonsignificant association was observed between bleeding and active cancer as well as remote cancer in the ARISTOTLE Trial (83).
An observational compared the bleeding risk in gastrointestinal cancer (GICA) patients who accepted apixaban or edoxaban anticoagulation, but the cumulative incidence of total bleeding, MB, and clinically relevant non-major bleeding (CRNMB) did not significantly differ between apixaban and rivaroxaban groups (101). However, in patients with GI cancers, especially upper GI cancers, meta-regression analysis revealed that DOACs were associated with higher rates of CRNMB events compared with dalteparin (102). In patients with primary brain tumors or secondary brain metastases, several retrospective studies showed that DOACs do not increased the risk of MB compared with LMWH, but DOACs were associated with lower risk of intracranial hemorrhage (103–106). When choosing LMWH, VKA, or DOACs among cancer patients with cancer-associated thrombosis (CAT), it is recommended to use LMWH instead of DOACs in patients with gastrointestinal or urogenital malignancies, while patients with solid tumors can use DOACs (107, 108).
The evidence of choosing anticoagulants among specific cancer patients with AF is limited. Most studies are observational. A large subgroup analysis of ARISTOPHANES trial retrospectively compared the MB risk of different anticoagulants in AF patients with breast cancer, gastrointestinal cancer, genitourinary cancer, hematologic cancer, and lung cancer. The study showed apixaban had a lower MB risk than warfarin in patients with breast cancer, gastrointestinal cancer, and genitourinary cancer, rivaroxaban and warfarin had a similar MB risk, apixaban had a lower MB risk than rivaroxaban only in patients with breast cancer (109). A small observational study explored the safety of DOACs (apixaban, rivaroxaban, and dabigatran) in patients with breast cancer and AF. The results showed that only rivaroxaban group had 3 cases of major bleeding events and 2 cases of clinically relevant non major bleeding (110). And an observational multicentre study from the AMBER-AF registry showed the MB risk did not entail differences (HR 1.53, 95%CI 0.93–2.53) in patients with AF and breast cancer treated with DOACs or warfarin (111). In a large-scale observational study involving 16,096 patients with AF and active cancer from the United States, stratified analysis showed no statistically significant differences in bleeding risk between warfarin and rivaroxaban as well as dabigatran in breast cancer, colon cancer, lung cancer, and prostate cancer (112). Evidence from a Danish nationwide cohort study suggested a similar 1-year risk of bleeding associated with DOAC compared with VKA among patients with AF and GI cancer (HR 1.12, 95%CI 0.71–1.76) (113). And, another Danish nationwide cohort study also found the 1-year risk of bleeding (hematuria and MB) was comparable in patients with AF and history of urologic cancer (114).
Cancer patients have a higher risk of bleeding after PCI. In a multicenter, observational study of ACS patients recruited for PCI (115), multiple regression analysis showed that the presence of cancer was the strongest independent predictor of bleeding (HR 1.5, 95%CI 1.1–2.1, p = 0.015). Data from the Mayo Clinic Cath's lab PCI registry database showed that cancer patients had a 2.8% higher bleeding rate than noncancer patients after PCI over an overall 5-year follow-up (HR 1.73; 95%CI: 1.06–2.83; p = 0.03) (88). In addition, Jessica et al. (116) explored some complications after PCI in four major cancers (prostate cancer, lung cancer, breast cancer, and colon cancer) and found that lung cancer, colon cancer, and prostate cancer were associated with an increased risk of bleeding ((OR 1.79, 95%CI 1.56–2.05), (OR 3.65, 95%CI 3.07–4.35), (OR 1.41, 95%CI 1.20–1.65), respectively). In another retrospective study to explore the clinical results for cancer patients after PCI (117), Kanenawa et al. used the PARIS bleeding score (95), PRECISE-DAPT score (96), CREDO-Kyoto risk score (97), and ARC-HBR criterion (98) to evaluate the risk of bleeding in cancer patients after PCI. All scores showed that cancer patients had a higher bleeding risk than noncancer patients (p < 0.001). After further exploratory analysis, patients that undergone aggressive cancer therapies such as surgery, radiation, chemotherapy or immunotherapy were associated with a greater risk of major bleeding (117).
Antithrombotic therapy in cancer patients comorbid with AF and ACS
Anticoagulation in patients with AF and cancer
Warfarin reduced the risk of stroke by 64% and all-cause death by 26% in patients with nonvalvular AF compared with the placebo group (118). Warfarin is also the only safe anticoagulant in AF patients with rheumatic mitral valve disease or artificial heart valves. However, the use of warfarin requires regular monitoring of the international normalized ratio (INR). The advent of nonvitamin K antagonist oral anticoagulants (NOACs) provides a new choice for AF patients. NOACs have better compliance, effectiveness, and safety, without the need for routine monitoring of coagulation function and INR (119, 120). In four well-known RCTs (RE-LY, ARISTOTLE, ROCKET AF, ENGAGE AF-TIMI), NOACs were not inferior to warfarin in the prevention of stroke or systemic embolism (121–124). In addition, a meta-analysis showed that compared to warfarin, NOACs reduce the risk of stroke by 19% and intracranial hemorrhage (ICH) by 52%, with a similar risk of major bleeding, but increase the risk of gastrointestinal bleeding by 25% (119).
The use of anticoagulants to prevent stroke in AF and cancer patients has been an ongoing challenge. Most cardiologists (63%) regard NOACs as the first choice for anticoagulation (125). A retrospective cohort study of the United States analyzing the use of OACs in patients with cancer and nonvalvular AF found that the use of NOACs increased from 21.8% to 76.2%, whereas the use of warfarin decreased from 78.2% to 23.8% from 2011 to 2016 (126). NOACs appear to be superior to warfarin in AF and cancer. A meta-analysis of three RCTs (ROCKET AF, ARISTOTLE, ENGAGE AF-TIMI 48) showed that NOACs and warfarin had no significant difference in the risk of stroke/SE (RR 0.76; 95%CI: 0.52–1.10), but NOACs significantly reduced the risk of major bleeding (RR 0.79; 95%CI: 0.63–0.99) (127). In line with this, Shah et al. (112) also found that NOACs had a similar risk of ischemic stroke to warfarin in terms of efficacy, and rivaroxaban and dabigatran had a similar risk of severe bleeding to warfarin (HR 1.09 95%CI: 0.79–1.50 P = 0.59; HR 0.96 95%CI: 0.72–1.27 P = 0.75, respectively) in terms of safety, whereas apixaban had a significantly lower risk of major bleeding than warfarin (HR 0.37 95%CI: 0.17–0.79) (112). However, data from the AMBER-AF registry showed that the incidence of stroke and bleeding did not entail differences in those receiving NOAC and warfarin (adjusted HR of stroke (0.91; 95% CI: 0.42–1.99) or severe bleedings (1.53; 95% CI: 0.93–2.53)) in AF patients with breast cancer (111).
Many antitumor drugs inhibit and compete with the cytochrome P-4503A4 enzyme (CYP3A4) or permeability glycoprotein transporter (P-gp). Thus, the simultaneous use of antitumor drugs and NOACs may cause drug‒drug interactions, which may enhance anticoagulation of NOACs and thereby increase the risk of major bleeding (128–130). Meanwhile, the 2018 European Heart Rhythm Association Practical Guide also indicated that strong inhibitors of CYP3A4 or P-gp should not be used with NOACs in AF patients (128). However, a national retrospective study showed that only 18% of patients with AF and cancer had major bleeding when NOACs were combined with antitumor drugs with inhibitory or competitive effects on CYP3A4 or P-gp activity, which may be explained by the use of low-dose NOACs and cancer-related hypercoagulability (131). The Table 2 showed some common anticancer drugs that can induce or inhibit CYP3A4 or P-gp substrate (128).
Thrombocytopenia (TP) is relatively common in cancer patients. It has been reported that approximately 10% of cancer patients have less than 10 × 109/L because of cancer and its therapies (132, 133). TP in patients with malignancy is associated with an increased risk of bleeding and ischemic complications (134). In the case of stable TP > 50 × 109/L, the European Hematology Association (EHA) recommends that full-dose NOACs should be superior to warfarin or low-molecular-weight heparin (LMWH) in nonvalvular AF patients with cancer (135). In AF patients with cancer and platelet counts 25–50 × 109/L, a 50% reduction in the LMWH dose may be safe (135, 136). Platelet count <25 × 109/L may have individualized treatment (136, 137). In conclusion, NOACs may be considered the first choice of anticoagulation for AF and cancer patients (138).
Antithrombotic therapy in patients with cancer and ACS
In the 2017 ESC Guideline on dual antiplatelet therapy (DAPT) in coronary arterial disease, DAPT with aspirin and P2Y12 inhibitor is recommended for 12 months in all ACS patients without high bleeding risk (HBR) but for 6 months in ACS patients with percutaneous coronary intervention (PCI) and HBR and for at least one month in ACS patients with medical therapy alone and HBR (139).
Cancer increases the risk of thrombotic and bleeding events in ACS patients. A retrospective study analyzed 456 patients with acute myocardial infarction (AMI) and active cancer, and only 211 (46.3%) patients used aspirin (140). Additionally, Yusuf et al. (140) analyzed the reasons why cancer patients with AMI did not use aspirin, most of whom had TP (73%). Thirty-nine percent of patients with cancer and thrombocytopenia have been diagnosed with ACS (141). Aspirin can improve the survival of cancer patients with ACS and thrombocytopenia (142, 143). The 7-day survival rate of patients who did not receive aspirin was 6%, while that of patients who received aspirin was 90% (142). The Society for Cardiovascular Angiography and Interventions (SCAI) Expert Consensus Statement recommends that aspirin may be used when platelet counts are >10 × 109/L; DAPT with clopidogrel may be used when platelet counts are 30–50 × 109/L, and prasugrel, ticagrelor and IIB-IIIA inhibitors should not be used in patients with platelet counts <50,000/ml (144). Moreover, if platelet counts are <50 × 109/L, the duration of DAPT may be restricted to 2 weeks after percutaneous coronary angioplasty (PTCA) alone, 4 weeks after bare-metal stents, and 6 months after second- or third-generation drug-eluting stents if optimal stent expansion is confirmed by intravascular ultrasound or optical coherence tomography (144). Aspirin and clopidogrel should be first recommended for ACS patients recently diagnosed with cancer (<12 months) (145, 146). Ticagrelor and prasugrel should not normally be used because of the high risk of bleeding and limited data about their efficacy and safety in patients with active cancer (145). In addition, clopidogrel may occur drug-drug interactions with anticancer drugs through CYP450 (146). To reduce the risk of bleeding, the duration and intensity of DAPT should be minimized (147). In addition, the 2017 ESC Guideline DAPT also recommended that bleeding risk is a major factor affecting the duration of DAPT (139). Thus, the approach of shortening DAPT duration (1–3 months) followed by single antiplatelet therapy is an absorbing option for cancer patients with high bleeding risk (145–148).
Antithrombotic strategy in cancer patients with ACS and AF
In AF patients with ACS with or without PCI, a combined antithrombotic regimen of antiplatelet and anticoagulant therapy may be needed. Guidelines recommend choosing either dual antithrombotic therapy [DAT: (N)OAC + P2Y12 inhibitor] or triple antithrombotic therapy [TAT: (N)OAC + P2Y12 inhibitor + aspirin] for AF and ACS patients (4, 5, 139, 146, 149). Many RCTs [WOEST (150), ISAR-TRIPLE (151), PIONEER AF-PCI (152), RE-DUAL PCI (153), AUGUSTUS (154), ENTRUST-AF PCI (155)] have confirmed that DAT is noninferior to or even superior to TAT in safety (bleeding risk), while it is similar to TAT in efficacy (stroke, direct thrombosis, cardiovascular events). Similar results were also obtained in four meta-analyses of the above RCTs (156–159). However, these benefits may be accompanied by an increased risk of ischemia (mainly stent thrombosis and recurrent myocardial infarction). Thus, current guidelines probably recommend that AF patients with ACS/PCI be treated with short-term TAT (one week to one month) and then followed by DAT up to 12 months (4, 5, 139, 149). NOACs are recommended for anticoagulants, and clopidogrel is recommended for P2Y12 inhibitors considering that prasugrel and ticagrelor may increase the risk of major bleeding and are the choice of most patients in most experiments (160). In addition, for AF and ACS patients with medical treatment alone, the guidelines recommended DAT treatment for six months followed by NOAC monotherapy as a default strategy (149).
Cancer is regarded as the strongest independent predictor of bleeding (4, 115, 161). Based on the fact that active malignancy is considered one of the main criteria of ARC-HBR (Academic Research Consortium-High Bleeding Risk), 2020 ESC NSTE-ACS Guidelines recommend that AF and cancer patients with ACS should be treated with TAT for a week and then DAT up to 6 months, followed by NOAC alone (4). The 2020 ESC guidelines for the diagnosis and management of AF patients recommend that if the risk of stent thrombosis is low or the risk of bleeding is higher than the risk of stent thrombosis, it is recommended to stop aspirin early (one week) (149). Additionally, 2022 ESC Guidelines on cardio-oncology recommend that NOACs and single antiplatelet therapies (preferably clopidogrel) are the default strategies after short-term triple antithrombotic therapy (up to one week in the hospital) for AF and cancer patients with ACS (147). Given antitumor therapy, complications, more underlying diseases and cancer-related factors such as cancer itself, cancer type, stage, metastasis and activity (161), the choice of the antithrombotic scheme should be individualized, especially the antithrombotic time, in cancer patients with AF and ACS. The risk of blood clots and bleeding in cancer patients should be assessed individually in the decision-making process for anticoagulants.
Currently, the choice of the antithrombotic regimen may be based on the default strategy recommended by the guidelines for AF and ACS patients with cancer (Figure 1), and the best treatment regimen should be determined by individualized and comprehensive assessment of the risk of bleeding and thrombosis (4, 5, 149). The comprehensive evaluation method for bleeding and embolism in AF and ACS patients with cancer is shown in Table 3. Additionally, the dose of anticoagulant may be reduced due to the patient's age, renal function and weight (162).
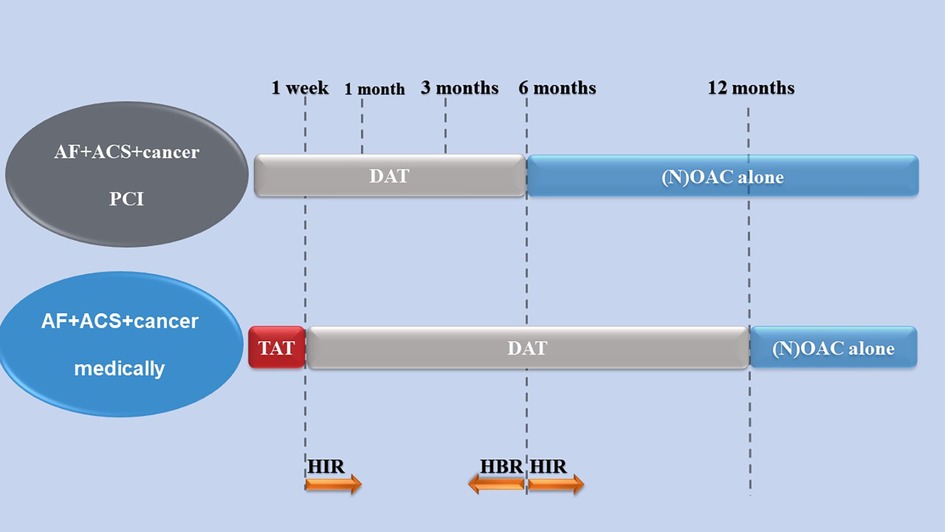
Figure 1. An antithrombotic strategy in patients with cancer comorbid with AF and ACS (4, 147, 149). Arrows indicate the increase or decrease in antithrombotic time. TAT, (N)OAC + P2Y12 inhibitor+aspirin; OAC, oral anticoagulants; NOAC, nonvitamin K oral anticoagulant; DAT, (N)OAC + P2Y12 inhibitor; AF, atrial fibrillation; ACS, acute coronary syndrome; HIR, high ischemic risk; HBR, high bleeding risk.
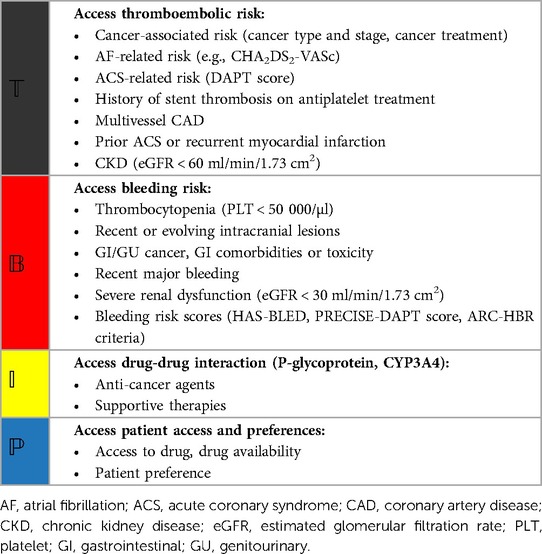
Table 3. A potential method to evaluate the risk of ischemia and bleeding in patients with AF and ACS comorbid with cancer (139, 147, 149, 162).
Challenges
Currently, antithrombotic treatment for cancer patients comorbid with CVD, especially AF and ACS, is a challenging issue for clinician, as cancer increases the uncertainty of risk of bleeding and embolism. It is difficult to find the balance between safety and efficacy in the process of antithrombotic therapy. The predictive efficacy of common cardiovascular scores used to assess the risk of bleeding and embolism in cancer patients is relatively low, which makes it difficult for clinical doctors to accurately estimate the risk of bleeding and embolism. In addition, when clinician formulate antithrombotic treatment plans, they need to deal with specific types of cancer, such as gastric cancer. However, most current research on anti-thrombotic therapy for cancer and CVDs only analyzes all types of tumors, which also adds challenges for clinical doctors to make specific decisions. And there is no large-scale RCTs to study anti-thrombotic therapy in cancer comorbid CVD. Most studies are post hoc analysis of RCTs (Figure 2). Furthermore, among specific types of cancer, the risk of stent thrombosis in patients with AF undergoing PCI surgery is also difficult to predict, which may increase the risk of recurrent myocardial infarction. In summary, antithrombotic therapy requires individualization in cancer patients with comorbid AF and ACS.
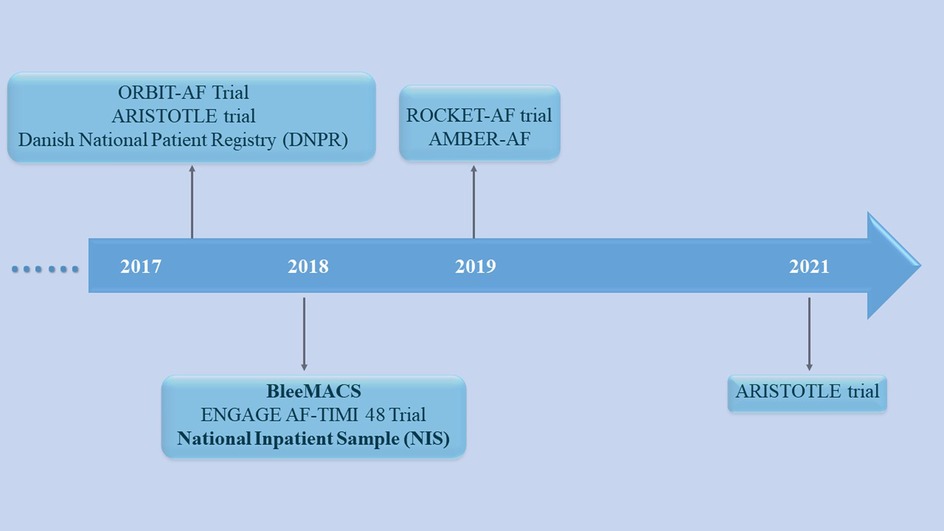
Figure 2. The important mentioned studies for cancer with atrial fibrillation and/or acute coronary syndrome in the review.
Conclusion
In cancer patients with comorbid AF and ACS, the selection of a proper antithrombotic strategy is still a challenge for physicians. Most current cardiovascular randomized controlled trials (RCTs) have excluded patients with cancer, resulting in insufficient available evidence in cancer patients with CVD. In addition, scores commonly used to assess bleeding and ischemia risk do not include cancer patients, such as the CHA2DS2-VASc score, HAS-BLED score, and PRECISE-DAPT score, which may underestimate the risk of bleeding and ischemia in cancer patients. Therefore, individualized antithrombotic therapy is inevitable for AF and ACS patients with cancer. The following problems may need to be solved urgently: (1) develop an embolism and bleeding risk score suitable for patients with CVD and cancer; and (2) implement RCTs to compare the effectiveness of current antithrombotic regimens in patients with cancer and explore the proper antithrombotic strategy in cancer patients comorbid with AF and ACS.
Author contributions
TW: Writing – original draft, Writing – review & editing. XL: Writing – review & editing. YZhu: Writing – review & editing. YZha: Writing – review & editing. JL: Writing – review & editing. ZZ: Writing – review & editing. GH: Conceptualization, Methodology, Supervision, Writing – review & editing. JX: Conceptualization, Methodology, Supervision, Writing – review & editing.
Funding
The author(s) declare financial support was received for the research, authorship, and/or publication of this article.
This study was supported by Sichuan Health Commission (Contracts No. 23LCYJ044), the Fundamental Research Funds for the Central Universities (Contracts No. 2682022ZTPY029, 2682021ZTPY026), Department of Human Resources and Social Security of Sichuan Province (Contracts No. 2021-11), Chengdu Municipal Health Commission (Contracts Nos. 2021200, 2022392).
Conflict of interest
The authors declare that the research was conducted in the absence of any commercial or financial relationships that could be construed as a potential conflict of interest.
Publisher's note
All claims expressed in this article are solely those of the authors and do not necessarily represent those of their affiliated organizations, or those of the publisher, the editors and the reviewers. Any product that may be evaluated in this article, or claim that may be made by its manufacturer, is not guaranteed or endorsed by the publisher.
References
1. Dagenais GR, Leong DP, Rangarajan S, Lanas F, Lopez-Jaramillo P, Gupta R, et al. Variations in common diseases, hospital admissions, and deaths in middle-aged adults in 21 countries from five continents (PURE): a prospective cohort study. Lancet. (2020) 395(10226):785–94. doi: 10.1016/s0140-6736(19)32007-0
2. Sung H, Ferlay J, Siegel RL, Laversanne M, Soerjomataram I, Jemal A, et al. Global cancer statistics 2020: GLOBOCAN estimates of incidence and mortality worldwide for 36 cancers in 185 countries. CA Cancer J Clin. (2021) 71(3):209–49. doi: 10.3322/caac.21660
3. Allemani C, Matsuda T, Di Carlo V, Harewood R, Matz M, Nikšić M, et al. Global surveillance of trends in cancer survival 2000–14 (CONCORD-3): analysis of individual records for 37 513 025 patients diagnosed with one of 18 cancers from 322 population-based registries in 71 countries. Lancet. (2018) 391(10125):1023–75. doi: 10.1016/s0140-6736(17)33326-3
4. Collet JP, Thiele H, Barbato E, Barthelemy O, Bauersachs J, Bhatt DL, et al. 2020 ESC guidelines for the management of acute coronary syndromes in patients presenting without persistent ST-segment elevation. Eur Heart J. (2021) 42(14):1289–367. doi: 10.1093/eurheartj/ehaa575
5. January CT, Wann LS, Calkins H, Chen LY, Cigarroa JE, Cleveland JC Jr, et al. 2019 AHA/ACC/HRS focused update of the 2014 AHA/ACC/HRS guideline for the management of patients with atrial fibrillation: a report of the American college of cardiology/American heart association task force on clinical practice guidelines and the heart rhythm society. J Am Coll Cardiol. (2019) 74(1):104–32. doi: 10.1016/j.jacc.2019.01.011
6. Khorana AA, Francis CW, Culakova E, Lyman GH. Risk factors for chemotherapy-associated venous thromboembolism in a prospective observational study. Cancer. (2005) 104(12):2822–9. doi: 10.1002/cncr.21496
7. Kamphuisen PW, Beyer-Westendorf J. Bleeding complications during anticoagulant treatment in patients with cancer. Thromb Res. (2014) 133(Suppl 2):S49–55. doi: 10.1016/S0049-3848(14)50009-6
8. Herrmann J, Lerman A, Sandhu NP, Villarraga HR, Mulvagh SL, Kohli M. Evaluation and management of patients with heart disease and cancer: cardio-oncology. Mayo Clin Proc. (2014) 89(9):1287–306. doi: 10.1016/j.mayocp.2014.05.013
9. Roth GA, Mensah GA, Johnson CO, Addolorato G, Ammirati E, Baddour LM, et al. Global burden of cardiovascular diseases and risk factors, 1990–2019: update from the GBD 2019 study. J Am Coll Cardiol. (2020) 76(25):2982–3021. doi: 10.1016/j.jacc.2020.11.010
10. Lloyd-Jones DM, Wang TJ, Leip EP, Larson MG, Levy D, Vasan RS, et al. Lifetime risk for development of atrial fibrillation. Circulation. (2004) 110(9):1042–6. doi: 10.1161/01.Cir.0000140263.20897.42
11. Kattelus H, Kesaniemi YA, Huikuri H, Ukkola O. Cancer increases the risk of atrial fibrillation during long-term follow-up (OPERA study). PLoS One. (2018) 13(10):e0205454. doi: 10.1371/journal.pone.0205454
12. Guha A, Fradley MG, Dent SF, Weintraub NL, Lustberg MB, Alonso A, et al. Incidence, risk factors, and mortality of atrial fibrillation in breast cancer: a SEER-medicare analysis. Eur Heart J. (2022) 43(4):300–12. doi: 10.1093/eurheartj/ehab745
13. Bharadwaj A, Potts J, Mohamed MO, Parwani P, Swamy P, Lopez-Mattei JC, et al. Acute myocardial infarction treatments and outcomes in 6.5 million patients with a current or historical diagnosis of cancer in the USA. Eur Heart J. (2020) 41(23):2183–93. doi: 10.1093/eurheartj/ehz851
14. Yousif N, Niederseer D, Davies A, El Issa M, Sidia B, Noor HA, et al. Impact of malignancy on clinical outcomes in patients with acute coronary syndromes. Int J Cardiol. (2021) 328:8–13. doi: 10.1016/j.ijcard.2020.12.010
15. Fanola CL, Ruff CT, Murphy SA, Jin J, Duggal A, Babilonia NA, et al. Efficacy and safety of edoxaban in patients with active malignancy and atrial fibrillation: analysis of the ENGAGE AF—tIMI 48 trial. J Am Heart Assoc. (2018) 7(16):e008987. doi: 10.1161/JAHA.118.008987
16. Melloni C, Shrader P, Carver J, Piccini JP, Thomas L, Fonarow GC, et al. Management and outcomes of patients with atrial fibrillation and a history of cancer: the ORBIT-AF registry. Eur Heart J Qual Care Clin Outcomes. (2017) 3(3):192–7. doi: 10.1093/ehjqcco/qcx004
17. Nakatsuma K, Shiomi H, Morimoto T, Watanabe H, Nakagawa Y, Furukawa Y, et al. Influence of a history of cancer on long-term cardiovascular outcomes after coronary stent implantation (an observation from coronary revascularization demonstrating outcome study-Kyoto registry cohort-2). Eur Heart J Qual Care Clin Outcomes. (2018) 4(3):200–7. doi: 10.1093/ehjqcco/qcy014
18. Armenian SH, Xu L, Ky B, Sun C, Farol LT, Pal SK, et al. Cardiovascular disease among survivors of adult-onset cancer: a community-based retrospective cohort study. J Clin Oncol. (2016) 34(10):1122–30. doi: 10.1200/JCO.2015.64.0409
19. Santoro C, Capone V, Canonico ME, Gargiulo G, Esposito R, Sanna GD, et al. Single, dual, and triple antithrombotic therapy in cancer patients with coronary artery disease: searching for evidence and personalized approaches. Semin Thromb Hemost. (2021) 47(8):950–61. doi: 10.1055/s-0041-1726298
20. Menter DG, Hatfield JS, Harkins C, Sloane BF, Taylor JD, Crissman JD, et al. Tumor cell-platelet interactions in vitro and their relationship to in vivo arrest of hematogenously circulating tumor cells. Clin Exp Metastasis. (1987) 5(1):65–78. doi: 10.1007/BF00116627
21. Shi Q, Ji T, Tang X, Guo W. The role of tumor-platelet interplay and micro tumor thrombi during hematogenous tumor metastasis. Cell Oncol (Dordr). (2023) 46(3):521–32. doi: 10.1007/s13402-023-00773-1
22. Ikeda M, Furukawa H, Imamura H, Shimizu J, Ishida H, Masutani S, et al. Poor prognosis associated with thrombocytosis in patients with gastric cancer. Ann Surg Oncol. (2002) 9(3):287–91. doi: 10.1007/BF02573067
23. Battinelli EM, Markens BA, Italiano JE Jr. Release of angiogenesis regulatory proteins from platelet alpha granules: modulation of physiologic and pathologic angiogenesis. Blood. (2011) 118(5):1359–69. doi: 10.1182/blood-2011-02-334524
24. Struyf S, Burdick MD, Peeters E, Van den Broeck K, Dillen C, Proost P, et al. Platelet factor-4 variant chemokine CXCL4L1 inhibits melanoma and lung carcinoma growth and metastasis by preventing angiogenesis. Cancer Res. (2007) 67(12):5940–8. doi: 10.1158/0008-5472.CAN-06-4682
25. Harjunpaa H, Llort Asens M, Guenther C, Fagerholm SC. Cell adhesion molecules and their roles and regulation in the immune and tumor microenvironment. Front Immunol. (2019) 10:1078. doi: 10.3389/fimmu.2019.01078
26. Bachmann M, Kukkurainen S, Hytonen VP, Wehrle-Haller B. Cell adhesion by integrins. Physiol Rev. (2019) 99(4):1655–99. doi: 10.1152/physrev.00036.2018
27. Zhou L, Zhang Z, Tian Y, Li Z, Liu Z, Zhu S. The critical role of platelet in cancer progression and metastasis. Eur J Med Res. (2023) 28(1):385. doi: 10.1186/s40001-023-01342-w
28. Lip GY, Chin BS, Blann AD. Cancer and the prothrombotic state. Lancet Oncol. (2002) 3(1):27–34. doi: 10.1016/s1470-2045(01)00619-2
29. Agnelli G, Bolis G, Capussotti L, Scarpa RM, Tonelli F, Bonizzoni E, et al. A clinical outcome-based prospective study on venous thromboembolism after cancer surgery: the @RISTOS project. Ann Surg. (2006) 243(1):89–95. doi: 10.1097/01.sla.0000193959.44677.48
30. Leiva O, Newcomb R, Connors JM, Al-Samkari H. Cancer and thrombosis: new insights to an old problem. J Med Vasc. (2020) 45(6S):6S8–6S16. doi: 10.1016/S2542-4513(20)30514-9
31. Nasser NJ, Fox J, Agbarya A. Potential mechanisms of cancer-related hypercoagulability. Cancers (Basel). (2020) 12(3):566. doi: 10.3390/cancers12030566
32. Hu YF, Chen YJ, Lin YJ, Chen SA. Inflammation and the pathogenesis of atrial fibrillation. Nat Rev Cardiol. (2015) 12(4):230–43. doi: 10.1038/nrcardio.2015.2
33. Nattel S, Harada M. Atrial remodeling and atrial fibrillation: recent advances and translational perspectives. J Am Coll Cardiol. (2014) 63(22):2335–45. doi: 10.1016/j.jacc.2014.02.555
34. Guzzetti S, Costantino G, Fundaro C. Systemic inflammation, atrial fibrillation, and cancer. Circulation. (2002) 106(9):e40; author reply e. doi: 10.1161/01.cir.0000028399.42411.13
35. Huang G, Xu JB, Liu JX, He Y, Nie XL, Li Q, et al. Angiotensin-converting enzyme inhibitors and angiotensin receptor blockers decrease the incidence of atrial fibrillation: a meta-analysis. Eur J Clin Invest. (2011) 41(7):719–33. doi: 10.1111/j.1365-2362.2010.02460.x
36. Chen PS, Chen LS, Fishbein MC, Lin SF, Nattel S. Role of the autonomic nervous system in atrial fibrillation: pathophysiology and therapy. Circ Res. (2014) 114(9):1500–15. doi: 10.1161/CIRCRESAHA.114.303772
37. Cheng WL, Kao YH, Chen SA, Chen YJ. Pathophysiology of cancer therapy-provoked atrial fibrillation. Int J Cardiol. (2016) 219:186–94. doi: 10.1016/j.ijcard.2016.06.009
38. Neef S, Steffens A, Pellicena P, Mustroph J, Lebek S, Ort KR, et al. Improvement of cardiomyocyte function by a novel pyrimidine-based CaMKII-inhibitor. J Mol Cell Cardiol. (2018) 115:73–81. doi: 10.1016/j.yjmcc.2017.12.015
39. McMullen JR, Boey EJH, Ooi JYY, Seymour JF, Keating MJ, Tam CS. Ibrutinib increases the risk of atrial fibrillation, potentially through inhibition of cardiac PI3K-Akt signaling. Blood. (2014) 124(25):3829–30. doi: 10.1182/blood-2014-10-604272
40. Yang X, An N, Zhong C, Guan M, Jiang Y, Li X, et al. Enhanced cardiomyocyte reactive oxygen species signaling promotes ibrutinib-induced atrial fibrillation. Redox Biol. (2020) 30:101432. doi: 10.1016/j.redox.2020.101432
41. Jafri M, Protheroe A. Cisplatin-associated thrombosis. Anticancer Drugs. (2008) 19(9):927–9. doi: 10.1097/CAD.0b013e3283100e9c
42. Ito D, Shiraishi J, Nakamura T, Maruyama N, Iwamura Y, Hashimoto S, et al. Primary percutaneous coronary intervention and intravascular ultrasound imaging for coronary thrombosis after cisplatin-based chemotherapy. Heart Vessels. (2012) 27(6):634–8. doi: 10.1007/s00380-011-0222-5
43. Kosmas C, Kallistratos MS, Kopterides P, Syrios J, Skopelitis H, Mylonakis N, et al. Cardiotoxicity of fluoropyrimidines in different schedules of administration: a prospective study. J Cancer Res Clin Oncol. (2008) 134(1):75–82. doi: 10.1007/s00432-007-0250-9
44. Chong JH, Ghosh AK. Coronary artery vasospasm induced by 5-fluorouracil: proposed mechanisms, existing management options and future directions. Interv Cardiol. (2019) 14(2):89–94. doi: 10.15420/icr.2019.12
45. Polk A, Vistisen K, Vaage-Nilsen M, Nielsen DL. A systematic review of the pathophysiology of 5-fluorouracil-induced cardiotoxicity. BMC Pharmacol Toxicol. (2014) 15:47. doi: 10.1186/2050-6511-15-47
46. Moslehi JJ. Cardiovascular toxic effects of targeted cancer therapies. N Engl J Med. (2016) 375(15):1457–67. doi: 10.1056/NEJMra1100265
47. Yeh ET, Bickford CL. Cardiovascular complications of cancer therapy: incidence, pathogenesis, diagnosis, and management. J Am Coll Cardiol. (2009) 53(24):2231–47. doi: 10.1016/j.jacc.2009.02.050
48. Pantaleo MA, Mandrioli A, Saponara M, Nannini M, Erente G, Lolli C, et al. Development of coronary artery stenosis in a patient with metastatic renal cell carcinoma treated with sorafenib. BMC Cancer. (2012) 12:231. doi: 10.1186/1471-2407-12-231
49. Lee MS, Finch W, Mahmud E. Cardiovascular complications of radiotherapy. Am J Cardiol. (2013) 112(10):1688–96. doi: 10.1016/j.amjcard.2013.07.031
50. Han XJ, Li JQ, Khannanova Z, Li Y. Optimal management of coronary artery disease in cancer patients. Chronic Dis Transl Med. (2019) 5(4):221–33. doi: 10.1016/j.cdtm.2019.12.007
51. Brosius FC 3rd, Waller BF, Roberts WC. Radiation heart disease. Analysis of 16 young (aged 15 to 33 years) necropsy patients who received over 3,500 rads to the heart. Am J Med. (1981) 70(3):519–30. doi: 10.1016/0002-9343(81)90574-x
52. Veinot JP, Edwards WD. Pathology of radiation-induced heart disease: a surgical and autopsy study of 27 cases. Hum Pathol. (1996) 27(8):766–73. doi: 10.1016/s0046-8177(96)90447-5
53. Lau ES, Paniagua SM, Liu E, Jovani M, Li SX, Takvorian K, et al. Cardiovascular risk factors are associated with future cancer. JACC CardioOncol. (2021) 3(1):48–58. doi: 10.1016/j.jaccao.2020.12.003
54. Andersson C, Nayor M, Tsao CW, Levy D, Vasan RS. Framingham heart study: JACC focus seminar, 1/8. J Am Coll Cardiol. (2021) 77(21):2680–92. doi: 10.1016/j.jacc.2021.01.059
55. Wolin KY, Carson K, Colditz GA. Obesity and cancer. Oncologist. (2010) 15(6):556–65. doi: 10.1634/theoncologist.2009-0285
56. Avgerinos KI, Spyrou N, Mantzoros CS, Dalamaga M. Obesity and cancer risk: emerging biological mechanisms and perspectives. Metab Clin Exp. (2019) 92:121–35. doi: 10.1016/j.metabol.2018.11.001
57. Iyengar NM, Gucalp A, Dannenberg AJ, Hudis CA. Obesity and cancer mechanisms: tumor microenvironment and inflammation. J Clin Oncol. (2016) 34(35):4270–6. doi: 10.1200/JCO.2016.67.4283
58. Koliaki C, Liatis S, Kokkinos A. Obesity and cardiovascular disease: revisiting an old relationship. Metab Clin Exp. (2019) 92:98–107. doi: 10.1016/j.metabol.2018.10.011
59. Van Gaal LF, Mertens IL, De Block CE. Mechanisms linking obesity with cardiovascular disease. Nature. (2006) 444(7121):875–80. doi: 10.1038/nature05487
60. Kannel WB, McGee DL. Diabetes and cardiovascular disease. The framingham study. JAMA. (1979) 241(19):2035–8. doi: 10.1001/jama.241.19.2035
61. Yuhara H, Steinmaus C, Cohen SE, Corley DA, Tei Y, Buffler PA. Is diabetes mellitus an independent risk factor for colon cancer and rectal cancer? Am J Gastroenterol. (2011) 106(11):1911–21; quiz 22. doi: 10.1038/ajg.2011.301
62. Srivastava SP, Goodwin JE. Cancer biology and prevention in diabetes. Cells. (2020) 9(6):1380. doi: 10.3390/cells9061380
63. Messner B, Bernhard D. Smoking and cardiovascular disease: mechanisms of endothelial dysfunction and early atherogenesis. Arterioscler Thromb Vasc Biol. (2014) 34(3):509–15. doi: 10.1161/ATVBAHA.113.300156
64. Ambrose JA, Barua RS. The pathophysiology of cigarette smoking and cardiovascular disease: an update. J Am Coll Cardiol. (2004) 43(10):1731–7. doi: 10.1016/j.jacc.2003.12.047
65. Golbidi S, Edvinsson L, Laher I. Smoking and endothelial dysfunction. Curr Vasc Pharmacol. (2020) 18(1):1–11. doi: 10.2174/1573403X14666180913120015
66. Caliri AW, Tommasi S, Besaratinia A. Relationships among smoking, oxidative stress, inflammation, macromolecular damage, and cancer. Mutat Res Rev Mutat Res. (2021) 787:108365. doi: 10.1016/j.mrrev.2021.108365
67. Alfaddagh A, Martin SS, Leucker TM, Michos ED, Blaha MJ, Lowenstein CJ, et al. Inflammation and cardiovascular disease: from mechanisms to therapeutics. Am J Prev Cardiol. (2020) 4:100130. doi: 10.1016/j.ajpc.2020.100130
68. Coussens LM, Werb Z. Inflammation and cancer. Nature. (2002) 420(6917):860–7. doi: 10.1038/nature01322
69. Huang G, Zhong XN, Zhong B, Chen YQ, Liu ZZ, Su L, et al. Significance of white blood cell count and its subtypes in patients with acute coronary syndrome. Eur J Clin Invest. (2009) 39(5):348–58. doi: 10.1111/j.1365-2362.2009.02107.x
70. Balkwill F, Mantovani A. Inflammation and cancer: back to virchow? Lancet. (2001) 357(9255):539–45. doi: 10.1016/S0140-6736(00)04046-0
71. Yao C, Veleva T, Scott L Jr, Cao S, Li L, Chen G, et al. Enhanced cardiomyocyte NLRP3 inflammasome signaling promotes atrial fibrillation. Circulation. (2018) 138(20):2227–42. doi: 10.1161/CIRCULATIONAHA.118.035202
72. Gungor B, Ekmekci A, Arman A, Ozcan KS, Ucer E, Alper AT, et al. Assessment of interleukin-1 gene cluster polymorphisms in lone atrial fibrillation: new insight into the role of inflammation in atrial fibrillation. Pace. (2013) 36(10):1220–7. doi: 10.1111/pace.12182
73. Westerterp M, Fotakis P, Ouimet M, Bochem AE, Zhang H, Molusky MM, et al. Cholesterol efflux pathways suppress inflammasome activation, NETosis, and atherogenesis. Circulation. (2018) 138(9):898–912. doi: 10.1161/CIRCULATIONAHA.117.032636
74. Liberale L, Holy EW, Akhmedov A, Bonetti NR, Nietlispach F, Matter CM, et al. Interleukin-1beta mediates arterial thrombus formation via NET-associated tissue factor. J Clin Med. (2019) 8(12):2072. doi: 10.3390/jcm8122072
75. Ridker PM. From C-reactive protein to interleukin-6 to interleukin-1: moving upstream to identify novel targets for atheroprotection. Circ Res. (2016) 118(1):145–56. doi: 10.1161/CIRCRESAHA.115.306656
76. Chen ST, Hellkamp AS, Becker RC, Berkowitz SD, Breithardt G, Fox KAA, et al. Efficacy and safety of rivaroxaban vs. warfarin in patients with non-valvular atrial fibrillation and a history of cancer: observations from ROCKET AF. Eur Heart J Qual Care Clin Outcomes. (2019) 5(2):145–52. doi: 10.1093/ehjqcco/qcy040
77. Nattel S. Molecular and cellular mechanisms of atrial fibrosis in atrial fibrillation. JACC Clin Electrophysiol. (2017) 3(5):425–35. doi: 10.1016/j.jacep.2017.03.002
78. Esteve E, Castro A, Lopez-Bermejo A, Vendrell J, Ricart W, Fernandez-Real JM. Serum interleukin-6 correlates with endothelial dysfunction in healthy men independently of insulin sensitivity. Diabetes Care. (2007) 30(4):939–45. doi: 10.2337/dc06-1793
79. Lee WY, Allison MA, Kim DJ, Song CH, Barrett-Connor E. Association of interleukin-6 and C-reactive protein with subclinical carotid atherosclerosis (the rancho bernardo study). Am J Cardiol. (2007) 99(1):99–102. doi: 10.1016/j.amjcard.2006.07.070
80. Lip GY, Nieuwlaat R, Pisters R, Lane DA, Crijns HJ. Refining clinical risk stratification for predicting stroke and thromboembolism in atrial fibrillation using a novel risk factor-based approach: the euro heart survey on atrial fibrillation. Chest. (2010) 137(2):263–72. doi: 10.1378/chest.09-1584
81. Singer DE, Chang Y, Borowsky LH, Fang MC, Pomernacki NK, Udaltsova N, et al. A new risk scheme to predict ischemic stroke and other thromboembolism in atrial fibrillation: the ATRIA study stroke risk score. J Am Heart Assoc. (2013) 2(3):e000250. doi: 10.1161/JAHA.113.000250
82. Patell R, Gutierrez A, Rybicki L, Khorana AA. Usefulness of CHADS2 and CHA2DS2-VASc scores for stroke prediction in patients with cancer and atrial fibrillation. Am J Cardiol. (2017) 120(12):2182–6. doi: 10.1016/j.amjcard.2017.08.038
83. Melloni C, Dunning A, Granger CB, Thomas L, Khouri MG, Garcia DA, et al. Efficacy and safety of apixaban versus warfarin in patients with atrial fibrillation and a history of cancer: insights from the ARISTOTLE trial. Am J Med. (2017) 130(12):1440–8.e1. doi: 10.1016/j.amjmed.2017.06.026
84. Vedovati MC, Giustozzi M, Verdecchia P, Pierpaoli L, Conti S, Verso M, et al. Patients with cancer and atrial fibrillation treated with doacs: a prospective cohort study. Int J Cardiol. (2018) 269:152–7. doi: 10.1016/j.ijcard.2018.07.138
85. Pastori D, Marang A, Bisson A, Menichelli D, Herbert J, Lip GYH, et al. Thromboembolism, mortality, and bleeding in 2,435,541 atrial fibrillation patients with and without cancer: a nationwide cohort study. Cancer. (2021) 127(12):2122–9. doi: 10.1002/cncr.33470
86. Kwok CS, Wong CW, Kontopantelis E, Barac A, Brown SA, Velagapudi P, et al. Percutaneous coronary intervention in patients with cancer and readmissions within 90 days for acute myocardial infarction and bleeding in the USA. Eur Heart J. (2021) 42(10):1019–34. doi: 10.1093/eurheartj/ehaa1032
87. van Werkum JW, Heestermans AA, Zomer AC, Kelder JC, Suttorp MJ, Rensing BJ, et al. Predictors of coronary stent thrombosis: the Dutch stent thrombosis registry. J Am Coll Cardiol. (2009) 53(16):1399–409. doi: 10.1016/j.jacc.2008.12.055
88. Guo W, Fan X, Lewis BR, Johnson MP, Rihal CS, Lerman A, et al. Cancer patients have a higher risk of thrombotic and ischemic events after percutaneous coronary intervention. JACC Cardiovasc Interv. (2021) 14(10):1094–105. doi: 10.1016/j.jcin.2021.03.049
89. Huang G, Zhao JL, Du H, Lan XB, Yin YH. Coronary score adds prognostic information for patients with acute coronary syndrome. Circ J. (2010) 74(3):490–5. doi: 10.1253/circj.cj-09-0637
90. Pisters R, Lane DA, Nieuwlaat R, de Vos CB, Crijns HJ, Lip GY. A novel user-friendly score (HAS-BLED) to assess 1-year risk of major bleeding in patients with atrial fibrillation: the euro heart survey. Chest. (2010) 138(5):1093–100. doi: 10.1378/chest.10-0134
91. O’Brien EC, Simon DN, Thomas LE, Hylek EM, Gersh BJ, Ansell JE, et al. The ORBIT bleeding score: a simple bedside score to assess bleeding risk in atrial fibrillation. Eur Heart J. (2015) 36(46):3258–64. doi: 10.1093/eurheartj/ehv476
92. Pastori D, Marang A, Bisson A, Herbert J, Lip GYH, Fauchier L. Performance of the HAS-BLED, ORBIT, and ATRIA bleeding risk scores on a cohort of 399 344 hospitalized patients with atrial fibrillation and cancer: data from the French national hospital discharge database. J Am Heart Assoc. (2022) 11(23):e026388. doi: 10.1161/JAHA.121.026388
93. Wester A, Mohammad MA, Olivecrona G, Holmqvist J, Yndigegn T, Koul S. Validation of the 4-item PRECISE-DAPT score: a SWEDEHEART study. J Am Heart Assoc. (2021) 10(20):e020974. doi: 10.1161/JAHA.121.020974
94. Raposeiras Roubin S, Abu Assi E, Munoz Pousa I, Dominguez Erquicia P, Melendo Viu M, Gonzalez Bermudez I, et al. Incidence and predictors of bleeding in patients with cancer and atrial fibrillation. Am J Cardiol. (2022) 167:139–46. doi: 10.1016/j.amjcard.2021.11.053
95. Baber U, Mehran R, Giustino G, Cohen DJ, Henry TD, Sartori S, et al. Coronary thrombosis and major bleeding after PCI with drug-eluting stents: risk scores from PARIS. J Am Coll Cardiol. (2016) 67(19):2224–34. doi: 10.1016/j.jacc.2016.02.064
96. Costa F, van Klaveren D, James S, Heg D, Raber L, Feres F, et al. Derivation and validation of the predicting bleeding complications in patients undergoing stent implantation and subsequent dual antiplatelet therapy (PRECISE-DAPT) score: a pooled analysis of individual-patient datasets from clinical trials. Lancet. (2017) 389(10073):1025–34. doi: 10.1016/S0140-6736(17)30397-5
97. Natsuaki M, Morimoto T, Yamaji K, Watanabe H, Yoshikawa Y, Shiomi H, et al. Prediction of thrombotic and bleeding events after percutaneous coronary intervention: CREDO-Kyoto thrombotic and bleeding risk scores. J Am Heart Assoc. (2018) 7(11):e008708. doi: 10.1161/JAHA.118.008708
98. Urban P, Mehran R, Colleran R, Angiolillo DJ, Byrne RA, Capodanno D, et al. Defining high bleeding risk in patients undergoing percutaneous coronary intervention: a consensus document from the academic research consortium for high bleeding risk. Eur Heart J. (2019) 40(31):2632–53. doi: 10.1093/eurheartj/ehz372
99. Aspberg S, Yu L, Gigante B, Smedby KE, Singer DE. Risk of ischemic stroke and major bleeding in patients with atrial fibrillation and cancer. J Stroke Cerebrovasc Dis. (2020) 29(3):104560. doi: 10.1016/j.jstrokecerebrovasdis.2019.104560
100. Ording AG, Horvath-Puho E, Adelborg K, Pedersen L, Prandoni P, Sorensen HT. Thromboembolic and bleeding complications during oral anticoagulation therapy in cancer patients with atrial fibrillation: a Danish nationwide population-based cohort study. Cancer Med. (2017) 6(6):1165–72. doi: 10.1002/cam4.1054
101. Ullah F, Song J, Rojas Hernandez CM, Kroll MH, Escalante CP, Toale KM. Safety and effectiveness of direct oral anticoagulants for the treatment of gastrointestinal cancer-associated venous thromboembolism. Oncologist. (2023) 28(11):e1005–16. doi: 10.1093/oncolo/oyad148
102. Sabatino J, De Rosa S, Polimeni A, Sorrentino S, Indolfi C. Direct oral anticoagulants in patients with active cancer: a systematic review and meta-analysis. JACC CardioOncol. (2020) 2(3):428–40. doi: 10.1016/j.jaccao.2020.06.001
103. Carney BJ, Uhlmann EJ, Puligandla M, Mantia C, Weber GM, Neuberg DS, et al. Intracranial hemorrhage with direct oral anticoagulants in patients with brain tumors. J Thromb Haemost. (2019) 17(1):72–6. doi: 10.1111/jth.14336
104. Leader A, Hamulyak EN, Carney BJ, Avrahami M, Knip JJ, Rozenblatt S, et al. Intracranial hemorrhage with direct oral anticoagulants in patients with brain metastases. Blood Adv. (2020) 4(24):6291–7. doi: 10.1182/bloodadvances.2020003238
105. Lee A, Oley F Jr, Lo M, Fong R, McGann M, Saunders I, et al. Direct oral anticoagulants or low-molecular-weight heparins for venous thromboembolism in patients with brain tumors. Thromb Res. (2021) 208:148–55. doi: 10.1016/j.thromres.2021.10.023
106. Swartz AW, Drappatz J. Safety of direct oral anticoagulants in central nervous system malignancies. Oncologist. (2021) 26(5):427–32. doi: 10.1002/onco.13698
107. Kakkos SK, Gohel M, Baekgaard N, Bauersachs R, Bellmunt-Montoya S, Black SA, et al. Editor’s choice—European society for vascular surgery (ESVS) 2021 clinical practice guidelines on the management of venous thrombosis. Eur J Vasc Endovasc Surg. (2021) 61(1):9–82. doi: 10.1016/j.ejvs.2020.09.023
108. Ning H, Yang N, Ding Y, Chen H, Wang L, Han Y, et al. Efficacy and safety of direct oral anticoagulants for the treatment of cancer-associated venous thromboembolism: a systematic review and Bayesian network meta-analysis. Med Clin (Barc). (2023) 160(6):245–52. doi: 10.1016/j.medcli.2022.06.022
109. Deitelzweig S, Keshishian AV, Zhang Y, Kang A, Dhamane AD, Luo X, et al. Effectiveness and safety of oral anticoagulants among nonvalvular atrial fibrillation patients with active cancer. JACC CardioOncol. (2021) 3(3):411–24. doi: 10.1016/j.jaccao.2021.06.004
110. Pacholczak-Madej R, Bazan-Socha S, Zareba L, Undas A, Dropinski J. Direct oral anticoagulants in the prevention of stroke in breast cancer patients with atrial fibrillation during adjuvant endocrine therapy: a cohort study. Int J Cardiol. (2021) 324:78–83. doi: 10.1016/j.ijcard.2020.09.037
111. Pardo Sanz A, Rincon LM, Guedes Ramallo P, Belarte Tornero LC, de Lara Delgado G, Tamayo Obregon A, et al. Current status of anticoagulation in patients with breast cancer and atrial fibrillation. Breast. (2019) 46:163–9. doi: 10.1016/j.breast.2019.05.017
112. Shah S, Norby FL, Datta YH, Lutsey PL, MacLehose RF, Chen LY, et al. Comparative effectiveness of direct oral anticoagulants and warfarin in patients with cancer and atrial fibrillation. Blood Adv. (2018) 2(3):200–9. doi: 10.1182/bloodadvances.2017010694
113. Ording AG, Sogaard M, Skjoth F, Grove EL, Lip GYH, Larsen TB, et al. Bleeding complications in patients with gastrointestinal cancer and atrial fibrillation treated with oral anticoagulants. Cancer Med. (2021) 10(13):4405–14. doi: 10.1002/cam4.4012
114. Ording AG, Sogaard M, Skjoth F, Grove EL, Lip GYH, Larsen TB, et al. Hematuria in anticoagulated patients with atrial fibrillation and urologic cancer. Res Pract Thromb Haemost. (2022) 6(1):e12629. doi: 10.1002/rth2.12629
115. Iannaccone M, D’Ascenzo F, Vadala P, Wilton SB, Noussan P, Colombo F, et al. Prevalence and outcome of patients with cancer and acute coronary syndrome undergoing percutaneous coronary intervention: a BleeMACS substudy. Eur Heart J Acute Cardiovasc Care. (2018) 7(7):631–8. doi: 10.1177/2048872617706501
116. Potts JE, Iliescu CA, Lopez Mattei JC, Martinez SC, Holmvang L, Ludman P, et al. Percutaneous coronary intervention in cancer patients: a report of the prevalence and outcomes in the United States. Eur Heart J. (2019) 40(22):1790–800. doi: 10.1093/eurheartj/ehy769
117. Kanenawa K, Yamaji K, Morinaga T, Hiromasa T, Hayashi M, Hiramori S, et al. Clinical outcomes after percutaneous coronary intervention in patients with cancer. Circ J. (2021) 85(6):837–46. doi: 10.1253/circj.CJ-20-1119
118. Hart RG, Pearce LA, Aguilar MI. Meta-analysis: antithrombotic therapy to prevent stroke in patients who have nonvalvular atrial fibrillation. Ann Intern Med. (2007) 146(12):857–67. doi: 10.7326/0003-4819-146-12-200706190-00007
119. Ruff CT, Giugliano RP, Braunwald E, Hoffman EB, Deenadayalu N, Ezekowitz MD, et al. Comparison of the efficacy and safety of new oral anticoagulants with warfarin in patients with atrial fibrillation: a meta-analysis of randomised trials. Lancet. (2014) 383(9921):955–62. doi: 10.1016/S0140-6736(13)62343-0
120. Obamiro KO, Chalmers L, Bereznicki LR. A summary of the literature evaluating adherence and persistence with oral anticoagulants in atrial fibrillation. Am J Cardiovasc Drugs. (2016) 16(5):349–63. doi: 10.1007/s40256-016-0171-6
121. Connolly SJ, Ezekowitz MD, Yusuf S, Eikelboom J, Oldgren J, Parekh A, et al. Dabigatran versus warfarin in patients with atrial fibrillation. N Engl J Med. (2009) 361(12):1139–51. doi: 10.1056/NEJMoa0905561
122. Granger CB, Alexander JH, McMurray JJ, Lopes RD, Hylek EM, Hanna M, et al. Apixaban versus warfarin in patients with atrial fibrillation. N Engl J Med. (2011) 365(11):981–92. doi: 10.1056/NEJMoa1107039
123. Rosenstein R, Parra D. Rivaroxaban versus warfarin in nonvalvular atrial fibrillation. N Engl J Med. (2011) 365(24):2334; author reply 5. doi: 10.1056/NEJMc1112233
124. Giugliano RP, Ruff CT, Braunwald E, Murphy SA, Wiviott SD, Halperin JL, et al. Edoxaban versus warfarin in patients with atrial fibrillation. N Engl J Med. (2013) 369(22):2093–104. doi: 10.1056/NEJMoa1310907
125. Penela D, Berruezo A, Cappato R. What do we really know about anticoagulation in patients with cancer and atrial fibrillation? Eur J Prev Cardiol. (2021) 28(6):606–7. doi: 10.1093/eurjpc/zwaa106
126. Ardeshirrouhanifard S, An HJ, Goyal RK, Raji MA, Segal JB, Alexander GC, et al. Use of oral anticoagulants among individuals with cancer and atrial fibrillation in the United States, 2010-2016. Pharmacotherapy. (2022) 42(5):375–86. doi: 10.1002/phar.2679
127. Casula M, Fortuni F, Fabris F, Leonardi S, Gnecchi M, Sanzo A, et al. Direct oral Xa inhibitors versus warfarin in patients with cancer and atrial fibrillation: a meta-analysis. J Cardiovasc Med (Hagerstown). (2020) 21(8):570–6. doi: 10.2459/JCM.0000000000001041
128. Steffel J, Verhamme P, Potpara TS, Albaladejo P, Antz M, Desteghe L, et al. The 2018 European heart rhythm association practical guide on the use of non-vitamin K antagonist oral anticoagulants in patients with atrial fibrillation. Eur Heart J. (2018) 39(16):1330–93. doi: 10.1093/eurheartj/ehy136
129. Wiggins BS, Dixon DL, Neyens RR, Page RL 2nd, Gluckman TJ. Select drug-drug interactions with direct oral anticoagulants: JACC review topic of the week. J Am Coll Cardiol. (2020) 75(11):1341–50. doi: 10.1016/j.jacc.2019.12.068
130. Khan Y, Zaidi SO, Razak BS, Zaki M, Malik BH. Use of new oral anticoagulants / direct oral anticoagulants in malignant patients. Cureus. (2020) 12(2):e7007. doi: 10.7759/cureus.7007
131. Wang CL, Wu VC, Tu HT, Huang YT, Chen SW, Chu PH, et al. Risk of major bleeding associated with concomitant use of anticancer drugs and direct oral anticoagulant in patients with cancer and atrial fibrillation. J Thromb Thrombolysis. (2022) 53(3):633–45. doi: 10.1007/s11239-021-02570-9
132. Liebman HA. Thrombocytopenia in cancer patients. Thromb Res. (2014) 133(Suppl 2):S63–9. doi: 10.1016/S0049-3848(14)50011-4
133. Nalysnyk L, Ranganathan G, Wu Y, Aravind S. Burden of thrombocytopenia in cancer patients undergoing chemotherapy: a retrospective database analysis. Blood. (2008) 112(11):4545. doi: 10.1182/blood.V112.11.4545.4545
134. Yusuf SW, Iliescu C, Bathina JD, Daher IN, Durand JB. Antiplatelet therapy and percutaneous coronary intervention in patients with acute coronary syndrome and thrombocytopenia. Tex Heart Inst J. (2010) 37(3):336–40. PMID: 20548817
135. Falanga A, Leader A, Ambaglio C, Bagoly Z, Castaman G, Elalamy I, et al. EHA Guidelines on management of antithrombotic treatments in thrombocytopenic patients with cancer. Hemasphere. (2022) 6(8):e750. doi: 10.1097/HS9.0000000000000750
136. Tufano A. Optimizing antithrombotic therapy for atrial fibrillation in cancer. Thromb Res. (2022) 213(Suppl 1):S103–S6. doi: 10.1016/j.thromres.2022.01.001
137. Leader A, Gurevich-Shapiro A, Spectre G. Anticoagulant and antiplatelet treatment in cancer patients with thrombocytopenia. Thromb Res. (2020) 191(Suppl 1):S68–S73. doi: 10.1016/S0049-3848(20)30400-X
138. Delluc A, Wang TF, Yap ES, Ay C, Schaefer J, Carrier M, et al. Anticoagulation of cancer patients with non-valvular atrial fibrillation receiving chemotherapy: guidance from the SSC of the ISTH. J Thromb Haemostasis. (2019) 17(8):1247–52. doi: 10.1111/jth.14478
139. Valgimigli M, Bueno H, Byrne RA, Collet JP, Costa F, Jeppsson A, et al. 2017 ESC focused update on dual antiplatelet therapy in coronary artery disease developed in collaboration with EACTS: the task force for dual antiplatelet therapy in coronary artery disease of the European society of cardiology (ESC) and of the European association for cardio-thoracic surgery (EACTS). Eur Heart J. (2018) 39(3):213–60. doi: 10.1093/eurheartj/ehx419
140. Yusuf SW, Daraban N, Abbasi N, Lei X, Durand JB, Daher IN. Treatment and outcomes of acute coronary syndrome in the cancer population. Clin Cardiol. (2012) 35(7):443–50. doi: 10.1002/clc.22007
141. Radmilovic J, Di Vilio A, D'Andrea A, Pastore F, Forni A, Desiderio A, et al. The pharmacological approach to oncologic patients with acute coronary syndrome. J Clin Med. (2020) 9(12):3926. doi: 10.3390/jcm9123926
142. Sarkiss MG, Yusuf SW, Warneke CL, Botz G, Lakkis N, Hirch-Ginsburg C, et al. Impact of aspirin therapy in cancer patients with thrombocytopenia and acute coronary syndromes. Cancer. (2007) 109(3):621–7. doi: 10.1002/cncr.22434
143. Feher A, Kampaktsis PN, Parameswaran R, Stein EM, Steingart R, Gupta D. Aspirin is associated with improved survival in severely thrombocytopenic cancer patients with acute myocardial infarction. Oncologist. (2017) 22(2):213–21. doi: 10.1634/theoncologist.2016-0110
144. Iliescu C, Grines CL, Herrmann J, Yang EH, Cilingiroglu M, Charitakis K, et al. SCAI Expert consensus statement: evaluation, management, and special considerations of cardio-oncology patients in the cardiac catheterization laboratory (endorsed by the cardiological society of India, and sociedad latino Americana de cardiologia intervencionista). Catheter Cardiovasc Interv. (2016) 87(5):895–9. doi: 10.1002/ccd.26375
145. Gevaert SA, Halvorsen S, Sinnaeve PR, Sambola A, Gulati G, Lancellotti P, et al. Evaluation and management of cancer patients presenting with acute cardiovascular disease: a consensus document of the acute CardioVascular care (ACVC) association and the ESC council of cardio-oncology-part 1: acute coronary syndromes and acute pericardial diseases. Eur Heart J-Acute Ca. (2021) 10(8):947–59. doi: 10.1093/ehjacc/zuab056
146. Byrne RA, Rossello X, Coughlan JJ, Barbato E, Berry C, Chieffo A, et al. 2023 ESC guidelines for the management of acute coronary syndromes. Eur Heart J. (2023) 44(38):3720–826. doi: 10.1093/eurheartj/ehad191
147. Lyon AR, Lopez-Fernandez T, Couch LS, Asteggiano R, Aznar MC, Bergler-Klein J, et al. 2022 ESC guidelines on cardio-oncology developed in collaboration with the European hematology association (EHA), the European society for therapeutic radiology and oncology (ESTRO) and the international cardio-oncology society (IC-OS). Eur Heart J. (2022) 43(41):4229–361. doi: 10.1093/eurheartj/ehac244
148. Shobayo F, Bajwa M, Koutroumpakis E, Hassan SA, Palaskas NL, Iliescu C, et al. Acute coronary syndrome in patients with cancer. Expert Rev Cardiovasc Ther. (2022) 20(4):275–90. doi: 10.1080/14779072.2022.2063840
149. Hindricks G, Potpara T, Dagres N, Arbelo E, Bax JJ, Blomstrom-Lundqvist C, et al. 2020 ESC guidelines for the diagnosis and management of atrial fibrillation developed in collaboration with the European association for cardio-thoracic surgery (EACTS): the task force for the diagnosis and management of atrial fibrillation of the European society of cardiology (ESC) developed with the special contribution of the European heart rhythm association (EHRA) of the ESC. Eur Heart J. (2021) 42(5):373–498. doi: 10.1093/eurheartj/ehaa612
150. Dewilde WJM, Oirbans T, Verheugt FWA, Kelder JC, De Smet BJGL, Herrman JP, et al. Use of clopidogrel with or without aspirin in patients taking oral anticoagulant therapy and undergoing percutaneous coronary intervention: an open-label, randomised, controlled trial. Lancet. (2013) 381(9872):1107–15. doi: 10.1016/S0140-6736(12)62177-1
151. Fiedler KA, Maeng M, Mehilli J, Schulz-Schupke S, Byrne RA, Sibbing D, et al. Duration of triple therapy in patients requiring oral anticoagulation after drug-eluting stent implantation: the ISAR-TRIPLE trial. J Am Coll Cardiol. (2015) 65(16):1619–29. doi: 10.1016/j.jacc.2015.02.050
152. Gibson CM, Mehran R, Bode C, Halperin J, Verheugt FW, Wildgoose P, et al. Prevention of bleeding in patients with atrial fibrillation undergoing PCI. N Engl J Med. (2016) 375(25):2423–34. doi: 10.1056/NEJMoa1611594
153. Cannon CP, Bhatt DL, Oldgren J, Lip GYH, Ellis SG, Kimura T, et al. Dual antithrombotic therapy with dabigatran after PCI in atrial fibrillation. N Engl J Med. (2017) 377(16):1513–24. doi: 10.1056/NEJMoa1708454
154. Lopes RD, Heizer G, Aronson R, Vora AN, Massaro T, Mehran R, et al. Antithrombotic therapy after acute coronary syndrome or PCI in atrial fibrillation. N Engl J Med. (2019) 380(16):1509–24. doi: 10.1056/NEJMoa1817083
155. Vranckx P, Lewalter T, Valgimigli M, Tijssen JG, Reimitz PE, Eckardt L, et al. Evaluation of the safety and efficacy of an edoxaban-based antithrombotic regimen in patients with atrial fibrillation following successful percutaneous coronary intervention (PCI) with stent placement: rationale and design of the ENTRUST-AF PCI trial. Am Heart J. (2018) 196:105–12. doi: 10.1016/j.ahj.2017.10.009
156. Gargiulo G, Goette A, Tijssen J, Eckardt L, Lewalter T, Vranckx P, et al. Safety and efficacy outcomes of double vs. Triple antithrombotic therapy in patients with atrial fibrillation following percutaneous coronary intervention: a systematic review and meta-analysis of non-vitamin K antagonist oral anticoagulant-based randomized clinical trials. Eur Heart J. (2019) 40(46):3757–67. doi: 10.1093/eurheartj/ehz732
157. Potpara TS, Mujovic N, Proietti M, Dagres N, Hindricks G, Collet JP, et al. Revisiting the effects of omitting aspirin in combined antithrombotic therapies for atrial fibrillation and acute coronary syndromes or percutaneous coronary interventions: meta-analysis of pooled data from the PIONEER AF-PCI, RE-DUAL PCI, and AUGUSTUS trials. Europace. (2020) 22(1):33–46. doi: 10.1093/europace/euz259
158. Lopes RD, Hong H, Harskamp RE, Bhatt DL, Mehran R, Cannon CP, et al. Safety and efficacy of antithrombotic strategies in patients with atrial fibrillation undergoing percutaneous coronary intervention: a network meta-analysis of randomized controlled trials. JAMA Cardiol. (2019) 4(8):747–55. doi: 10.1001/jamacardio.2019.1880
159. Golwala HB, Cannon CP, Steg PG, Doros G, Qamar A, Ellis SG, et al. Safety and efficacy of dual vs. Triple antithrombotic therapy in patients with atrial fibrillation following percutaneous coronary intervention: a systematic review and meta-analysis of randomized clinical trials. Eur Heart J. (2018) 39(19):1726–35a. doi: 10.1093/eurheartj/ehy162
160. Verlinden NJ, Coons JC, Iasella CJ, Kane-Gill SL. Triple antithrombotic therapy with aspirin, P2Y12 inhibitor, and warfarin after percutaneous coronary intervention: an evaluation of prasugrel or ticagrelor versus clopidogrel. J Cardiovasc Pharmacol Ther. (2017) 22(6):546–51. doi: 10.1177/1074248417698042
161. Mano TB, Timoteo AT, Rosa SA, Belo A, Ferreira RC, Investigators PR. Cancer patients with acute coronary syndrome have non-superior bleeding risk compared to patients with similar characteristics -- a propensity score analysis from the ProACS registry. Rev Port Cardiol. (2022) 41(7):573–82. doi: 10.1016/j.repc.2021.04.010
Keywords: cancer, atrial fibrillation, acute coronary syndrome, coronary artery disease, antithrombotic therapy
Citation: Wang T, Liu X, Zhu Y, Zhang Y, Zhang Z, Huang G and Xu J (2023) Antithrombotic strategy in cancer patients comorbid with acute coronary syndrome and atrial fibrillation. Front. Cardiovasc. Med. 10:1325488. doi: 10.3389/fcvm.2023.1325488
Received: 21 October 2023; Accepted: 27 November 2023;
Published: 12 December 2023.
Edited by:
Grégoire Rangé, Les Hôpitaux de Chartres, FranceReviewed by:
Iris Parrini, Hospital Mauritian Turin, ItalyMario Enrico Canonico, University of Colorado, United States
© 2023 Wang, Liu, Zhu, Zhang, Zhang, Huang and Xu. This is an open-access article distributed under the terms of the Creative Commons Attribution License (CC BY). The use, distribution or reproduction in other forums is permitted, provided the original author(s) and the copyright owner(s) are credited and that the original publication in this journal is cited, in accordance with accepted academic practice. No use, distribution or reproduction is permitted which does not comply with these terms.
*Correspondence: Gang Huang b2lnZzE5ODJAZ21haWwuY29t; aHVhbmdnYW5nQHN3anR1LmVkdS5jbg== Junbo Xu eHVqdW5ibzIwMDBAc2luYS5jb20=