- 1Department of Pediatric and Adolescent Endocrinology, Chair of Pediatrics, Pediatric Institute, Jagiellonian University Medical College in Kraków, Kraków, Poland
- 2Interclinical Center for the Treatment of Childhood Obesity, University Children’s Hospital of Kraków, Kraków, Poland
- 3Pediatric Department, Consorcio Hospital General, University of Valencia, Valencia, Spain
- 4CIBER Fisiopatología Obesidad y Nutrición (CIBEROBN), Instituto de Salud Carlos III, Madrid, Spain
- 5INCLIVA Biomedical Research Institute, Hospital Clínico, University of Valencia, Valencia, Spain
- 6Department of Pediatrics, Gastroenterology and Nutrition, Pediatric Institute, Jagiellonian University Medical College in Kraków, Kraków, Poland
- 7Department of Pediatrics, Gastroenterology, Allergology and Pediatric Nutrition, Medical University of Gdansk, Kraków, Poland
- 8Unit of Molecular Biology and Nutrigenomics, School of Pharmacy, University of Camerino, Camerino, Italy
- 9Faculty of Health Science, Libertas International University, Zagreb, Croatia
- 10Division of Pediatric Nephrology, Center for Pediatrics and Adolescent Medicine, Heidelberg University, Heidelberg, Germany
- 11Department of Clinical Psychology, University Hospital of Bellvitge-IDIBELL, Barcelona, Spain
- 12Clinical Psychology Unit, University Hospital of Bellvitge, Barcelona, Spain
- 13Psychoneurobiology of Eating and Addictive Behaviours Group, Bellvitge Biomedical Research Institute (IDIBELL), Barcelona, Spain
- 14Department of Medical Sciences, Faculty of Sport and Physical Education, University of Niš, Niš, Serbia
- 15Children’s Hospital, Hannover Medical School, Hannover, Germany
- 16Department of Quantitative Methods for Economics and Management, University of Las Palmas de Gran Canaria, Las Palmas de Gran Canaria, Spain
- 17Department of Clinical Sciences, School of Medicine and Health Sciences, University of Barcelona, Barcelona, Spain
- 18Department of Pediatrics, Pediatric Endocrinology and Diabetes, Medical Faculty, University of Rzeszów, Rzeszów, Poland
- 19Department of Medical and Surgical Sciences, University of Bologna, Bologna, Italy
- 20Department of Pediatric Nephrology and Hypertension, Chair of Pediatrics, Pediatric Institute, Jagiellonian University Medical College, Kraków, Poland
Childhood obesity has become a worldwide epidemic in the 21st century. Its treatment is challenging and often ineffective, among others due to complex, often not obvious causes. Awareness of the existence and meaning of psychosocial and environmental risk factors seems to be an essential element in the prevention and treatment of obesity and its complications, especially arterial hypertension. In this review, we will discuss the role of that risk factors linking obesity and increased cardiovascular disorders including the role of nutritional factors (including the role of unhealthy diet, inadequate hydration), unhealthy behaviors (e.g. smoking, alcohol and drugs, sedentary behavior, low physical activity, disrupted circadian rhythms, sleep disorders, screen exposure), unfavorable social factors (such as dysfunctional family, bullying, chronic stress, mood disorders, depression, urbanization, noise, and environmental pollution), and finally differences in cardiovascular risk in girls and boys.
1. Introduction
Childhood obesity has become one of the most serious global health crises of this century. According to the most actual definition, obesity is chronic, progressive, relapsing, and treatable multi-factorial, neurobehavioral disease, wherein an increase in body fat promotes adipose tissue dysfunction and abnormal fat mass physical forces, resulting in adverse metabolic, biomechanical, and psychosocial health consequences (1, 2). It is one of the most important reasons for increasing cardiovascular risk in young adults nowadays. Obesity-related hypertension is the dominant form of abnormal, elevated blood pressure (BP) in adolescents. Numerous studies have shown the existence of a tracking phenomenon, in which pathological processes begin as a result of various risk factors and lead to permanent changes and cardiovascular complications through endothelial damage, vascular and myocardial remodeling, and atherosclerotic processes (3). Therefore, targeting obesity reduction solely at children with obesity or overweight may not substantially reduce the overall burden of obesity-related disease in adulthood. Obesity should be considered a multifactorial disease, resulting from a dynamic interplay between genetics, physiology, behavior, psychosocial factors and environmental factors. All these factors accumulate over time since conception and can lead to the deterioration in energy homeostasis throughout life. Most of the reviews published so far focus on factors directly related to the person suffering from obesity: genes, metabolic disorders, and mental factors. The purpose of this study is to provide an up-to-date review of research on external factors—related to the patient's environment. Increased evidence points to their importance. The paper presents a narrative review of the literature on these issues.
2. Nutrition related factors
2.1. Unhealthy diet
An unhealthy diet in children and adolescents has been associated with overweight and obesity. A major contributor to obesity and hypertension is the high consumption of processed and fast foods high in sodium, saturated- and trans-fats, sugars and calories. Also, beverage like soda, fruit juices, and sports drinks stand for a high font of simple carbohydrates. Song et al., show in their meta-analysis that the prevalence of childhood hypertension correlates with higher body mass index (BMI) (4). In children aged 6–12 years triglyceride levels (≥150 mg/dl), and low-density lipoprotein cholesterol level (≥110 mg/dl) have been positively associated with hypertension, while dietary intake of fiber, vitamin C and copper were negatively related to hypertension (5). Meta-analysis shows a positive association for the risk of hypertension for 100 g/day of red meat or 50 g/day of processed meat (6). Preventive strategies to contrast hypertension include the correct consumption of vegetables and fruits which contain important bioactive molecules, vitamins and fiber useful to modulate properly gut microbiome and help to maintain lower BP. An adequate intake of whole grains is another source of insoluble fibre that can help reduce the risk of developing hypertension (7). A daily consume of 30 g/day of fiber in patients with hypertension (stage 1; 18–65 years old) lower heart rate, contributing to the control of hypertension (8). A meta-analysis on the impact of dietary fiber consumption on hypertension, shows that fiber decreases BP, low-density lipoprotein, plasma cholesterol level and improves blood glucose level (9). The intake of various types of vegetables (3 portions/day) and the correct quantity of fruit (2 portions/day) is associated with short chain fatty acids (SCFA) production from fiber by gut microbiome; SCFA can mediate anti-inflammatory response, regulate lipid and carbohydrate metabolism. Moreover, the bioactive compounds contained in plant-based food can modulate properly genes driving antioxidant, anti-inflammatory and detoxifying responses. Furthermore, green vegetables are key font of folic acid, that together with B2, B6 and B12 (from animal food), choline and betaine, guarantee the substrates and co-factors required by one-carbon cycle, which produces the universal methyl group donor the S-adenosyl-methionine. This pathway permits to convert homocysteine to methionine and guarantees the required methyl groups for the maintenance of cell epigenetic signature. Low-fat dairy products, protein from plant-based food (i.e., legumes and cereals) or from selected animal products (i.e., small fish and white meat) can contribute to maintain a healthy lipid profile. Moreover a positive impact on kidney function (8% lower risk) was measured in individuals consuming omega-3 polyunsaturated fatty acids from seafoods (10). In conclusion the correct lifestyle (food intake and physical activity) can prevent obesity and hypertension in children and adolescents; education needs to start from both parents and children. It is necessary to address the required evidence about healthy (i.e., whole cereals, vegetables, fruit, fish, water) and unhealthy (i.e., processed food, red and processed meat, beverages) food and beverage to stakeholders in order to turn prevention into a strategy for combating obesity and high BP in children and young people.
2.2. Inadequate hydration
Even small chronic dehydration can have an impact on both physical health (kidney stones, constipation, urinary tract infections) and mental health. This condition increases fatigue and reduces mental performance by reducing memory, attention, and response time. According to studies, children drink too little water, contrary to the EFSA recommendations (see Table 1).
As water turnover increases with the BMI, children with obesity need more water. Studies have shown that children with obesity are more likely to dehydrate (19, 20). Children with obesity drink less liquids, have lower total body water percentages, and have higher urine density, while children with normal weight are less dehydrated (21). Additionally, dehydration may be aggravated due to the increased sodium intake in children with obesity. Studies in the pediatric population show an increase in sodium intake with an increase in BMI Z-score. Sodium intake, based on 24-h urinary excretion, has been associated with obesity in several European studies, with those with the highest sodium excretion having a higher BMI compared to those with the lowest excretion. A Polish study found that high sodium levels in overweight children and excessive salt consumption, as well as low potassium consumption, are significant predictors of dehydration regardless of total water consumption (TWI) (22). In this study, the systolic BP was also directly associated with 24-h urinary sodium excretion. Therefore, nutritional education of children with obesity should focus on increased consumption of products rich in potassium, mainly vegetables and fruits, in order to compensate for the effect of increased sodium intake. Children should be also educated on how much and what liquids they should choose to drink.
2.3. Smoking, alcohol and drugs
According to the Organization for Economic Co-operation and Development (OECD) report on adolescent smoking and alcohol consumption published in 2021, the average prevalence of smoking among adolescents in all OECD countries was 16.4%. On average, 21.5% of adolescents aged 15 years had been drunk at least twice in their lifetime, with prevalence only slightly higher among boys (22.6%) than girls (20.3%), and 7% of 15-year-olds reported cannabis smoking within the last months (23). The adverse effects of tobacco smoking on adult cardiovascular health are well described. However, these negative effects are not limited to nicotine use in adolescence and adulthood, but also to fetal nicotine exposure during pregnancy or passive smoking in infancy and childhood (24–28). In a meta-analysis of 17 studies on the effects of maternal smoking during pregnancy, children with intrauterine nicotine exposure had an increased risk of obesity at an average age of nine years compared with children of nonsmoking mothers (29). In another meta-analysis, based on results of more than 84,000 children reported in 14 observational studies, children whose mothers smoked during pregnancy were at 50% elevated risk for overweight, compared with children whose mothers did not smoke (30). It is suggested that maternal smoking during pregnancy may lead to nicotine-induced fetal growth retardation and faster postnatal weight gain, both of which are associated with an increased risk of obesity later in life (30). In addition, maternal tobacco smoking may increase the child's BP by inducing endothelial and vascular dysfunction and alterations of renal morphology and function (31). Maternal smoking has also been shown to be associated with reprogramming of BP control mechanisms in the child (32). Both active and passive smoking have been associated with higher systolic BP levels in children and adolescents (26). Smoking exposure even at low levels and intensity of alcohol use were associated individually and together with increased arterial stiffness (33). Starting to smoke in adolescence is associated with an increased risk of nicotine dependence, both in childhood and thereafter. And although e-cigarettes seem to be a less harmful form of nicotine delivery, they appear to increase the risk of nicotine dependency later in life (34). Recently, it has also been shown that smoking and alcohol consumption are independent risk factors influencing childhood obesity (35). Beliefs that smoking protects against obesity may be over-simplistic; especially among younger and heavier smokers. Among smokers, the risk of obesity increased with the amount smoked and former heavy smokers were more likely to be obese than former light smokers (adjusted OR 1.60) (36). In a meta-analysis of 21 studies, active smoking increased the risk of childhood obesity by 17%. In a bidirectional analysis of the correlation between BMI and smoking, a higher BMI did not predict an increased risk for cigarette smoking, while cigarette smoking predicted a higher BMI, and higher frequency of cigarette smoking in adolescence predicted chronic obesity in young adulthood (37–39). Given the demonstrated correlation between e-cigarette use and cigarette smoking among adolescents, the association between obesity and cigarette smoking could also apply to e-cigarette use (40). In contrast to the effect of smoking on BMI, based on a meta-analysis of 13 studies, drinking alcohol had no significant effect on the risk of childhood obesity (35). However, alcohol energy (7 kcal/g) can be a contributing factor to weight gain. Therefore, alcohol consumption might increase the risk of obesity in some individuals. Smoking promotes damage to the cardiovascular system in several ways. Tobacco smoke is a complex mixture of more than 5,000 substances, including numerous carcinogenic and toxic substances. These can interact with each other and reinforce effects. Nicotine constricts blood vessels by releasing catecholamines, thereby acutely increasing BP. Several different constituents of tobacco smoke damage the endothelium, induce dyslipidemia, have prothrombotic effects, and cause chronic inflammation. Active smokers have increased arterial stiffness compared with nonsmokers, promoting chronic hypertension. The deleterious association between tobacco exposure and endothelial function is observed even in young children without other risk factors (41). The tobacco consumption during adolescence is the greatest risk factor for cardiovascular disorders and events in adulthood (42).
Acute alcohol consumption results in a short-lasting decrease in BP (43). In contrast, chronic alcohol consumption has a dose-dependent, linear association with hypertension that has been shown to be consistent across sex, age and ethnicity, and is independent of BMI, smoking habits, and physical activity level (44–47).
Smoked cannabis is one of the most commonly used drugs among young people. Acute cannabis smoking has been associated with an increased risk of heart attacks and ischemic strokes. However, it is still uncertain whether individuals who smoke cannabis exclusively are at increased risk for accelerated atherosclerosis, as has been found in tobacco smokers. The stimulants methamphetamines and cocaine increase the risk of stroke and heart attack and accelerate atherosclerosis (48).
3. Sedentary behavior, low physical activity
3.1. Definitions
A wealth of evidence supports the importance for children and adolescents to meet physical activity guidelines, which include 60 min of moderate to vigorous physical activity (MVPA) daily (49). However, research on the associations between physical activity (PA) and health for the potentially remaining 23 h per day has traditionally been less numerous but is growing rapidly. Sedentary behavior is particularly important, defined as any waking behavior characterized by energy expenditure ≤1.5 MET while sitting or reclining, as defined by the Sedentary Behavior Research Network (SBRN) in 2012, in an effort to have a consensus definition (50). However, there are some terms such as physical inactivity, screen time, stationary behavior etc. that can lead to confusion. They have been cleared on a recent document published by the same network in 2017 (51). Definitions are included in Table 2. Another point of discussion was if the general definition of sedentary behavior could also be applied to children, considering their higher basal metabolic rate. There was broad support for using also in preschool children and youth the threshold of ≤1.5 METs to define sedentary lifestyle provided that a standard higher than 3.5 ml/kg/min is used to define MET, following the recommendations of the compendium of energy expenditures for youth (52–54).
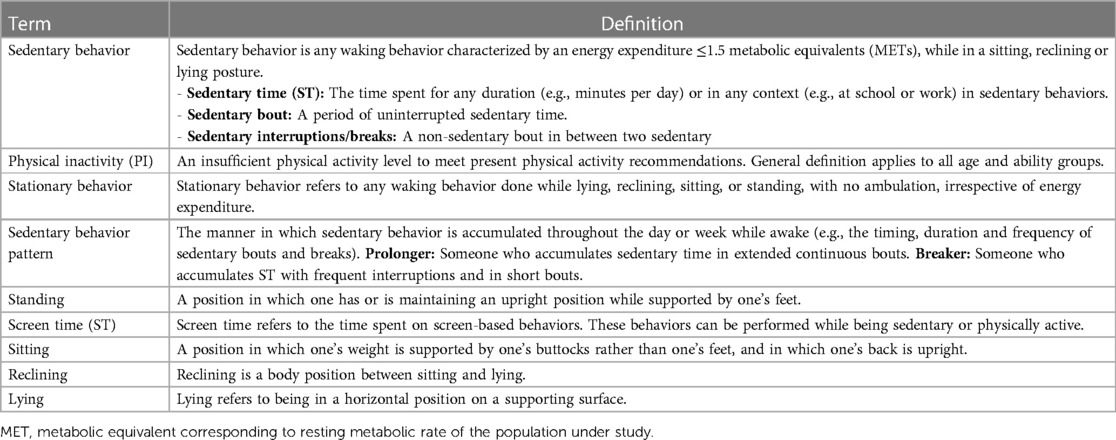
Table 2. Final definitions of key terms from the sedentary behavior research network (SBRN) terminology consensus project (51).
3.2. Epidemiology of sedentary behavior in children and adolescents
Focusing on insufficient PA, in 2010 worldwide, its prevalence in children aged 11–17 years was 78.4% for boys and 84.4% for girls (55). The same research group reported global trends of non-objectively measured PA from 2001 to 2016, based on 298 surveys from 146 countries, including 1.6 million participants (56). In 2016, 81% of the participants between 11 and 17 years old, presented insufficient PA. Between 2001 and 2016, the prevalence decreased by 2.5% (significant change) for boys, while there was no significant change for girls. This makes the difference in PI between sexes increase to 7.1% (4.7% in low-middle-income countries and 11.8% in high-income countries). No differences were found in PA according to the income of the countries in which they live. The gold standard measurement to determine sedentary time (ST) is accelerometry. The International Children's Accelerometry Database collects standardized PA data measured by the actigraph accelerometer. In 2020 this group published the pooled results of eight studies including longitudinal accelerometer data of 5,991 children (aged 4–15 years), with a mean follow-up of 2.7 years (57). The authors found that the average total ST (from 7am to midnight) of the participants ranged from 243 to 375 min/day at baseline for boys and from 250 to 393 min/day for girls, with significant but small in size differences among sexes. In both sexes, the average ST far exceeds the maximum of 120 min recommended by the WHO. The results of the multilevel analyses indicated that total ST increased on average by 21.4 min/day for each additional year of follow-up, being this increase high during childhood and moderate during the transition from childhood to adolescence. And, are there differences in ST between normal weight and children and adolescents with obesity? This question was answered by this systematic review including 14,739 PA-accelerometer-measured participants (3,526 with obesity) (58). Researchers did not find marked differences in ST between obese and non-obese peers, although the amount of moderate-to-vigorous intensity physical activity (MVPA) was superior in the most ST and also by screen time of the included studies in normal-weight participants. Contrary to what common sense would lead us to believe, the evidence supports that unfortunately, among children and adolescents, sedentary behavior is the norm, not the exception. Overall, emerging evidence in the wake of the COVID-19 pandemic indicates that the number of children and adolescents meeting guidelines for physical activity and sedentary behavior is declining (59, 60). The COVID-19 pandemic has led to the implementation of policies that mandate various restrictions on daily life, including social distancing, the closure of public services and schools, and movement limitations. The coexistence of childhood obesity and COVID-19 and changes in the bioecological environment have put children and adolescents at increased risk for developing obesity and exacerbating the severity of this disorder (61). The need for social isolation had an effect of causing or worsening obesity and its comorbidities (62).
3.3. Effect of sedentary behaviour on health
In children and adolescents sedentary behavior is linked to an increased risk to develop obesity, increased cardiovascular risk, decreased fitness, behavioral problems, and lower self-esteem (63). Among the mechanisms proposed to explain these associations, are the decreased energy expenditure caused by SED and also by screen time in relation to the increase in the consumption of unhealthy foods (64). Evidence about the direct unfavorable effects on metabolic health is scarce and partly it seems to be mediated mainly by screen-time-unhealthy-eating (65). In both cross-sectional and longitudinal studies, a relationship has been found between excessive inactivity time and higher risk to develop HTN. Recent studies in adults based on objective measures of physical activity indicate that sedentary behavior is associated with an increased risk of obesity, CVD, decreased CRF and all causes of mortality even in subjects who comply with physical activity recommendations. Only, high levels of moderate-intensity physical activity (i.e., about 60–75 min per day) seem to eliminate the increased risk of death associated with high sitting time (66). Further analysis of the data indicates that this is due in part to the negative effect of prolonged uninterrupted ST time bouts (low number and duration of breaks in ST time). Recent studies in adults have shown that these prolonged periods have deleterious effects on health, independent of those produced by physical inactivity (67) because in addition, they affect vascular function, altering systemic blood flow (68) they favor an increase in BP they have been related to the activation of the sympathetic system (69) they raise postprandial glycemia and insulin resistance by reducing consumption by inactive muscles (70) they alter the regulation of cerebral blood flow (71) they favor an increase in systemic inflammation (67, 72) and, finally, they could even reduce the beneficial effect of the practice of physical activity (73). Among ST behaviors in children and adolescents, recreational screen time (63, 74) and prolonged uninterrupted ST bouts (75) have shown associations with adverse health outcomes. This association was directly correlated to the duration of ST bouts and indirectly with the mean duration of sedentary breaks. In the case of young children, this negative effect of prolonged periods of inactivity has not been demonstrated fully, because the natural behavior of children is to spontaneously interrupt periods of inactivity. In children (5–12 years old) total MVPA is important for lowering cardiometabolic risk in children, while both total and uninterrupted sedentary time seem of less importance (76, 77). But the social trend of continuous use of screens or prolonged periods of sitting during classes could also have an impact on child´s health. At school, with age, the number of hours spent at school and the number of hours needed for homework and self-study also increases. For this reason, the amount of forced time spent sitting increases during adolescence. In addition, these prolonged periods of inactivity have been identified in higher-risk populations such as children and adolescents with obesity or adolescents with intensive screen use (more on weekends) but more research is needed on this topic.
Most of these deleterious effects can be avoided by interrupting these prolonged periods of inactivity. Therefore, recommendations are increasingly being made not only to increase PA by decreasing ST and reaching the PA goals but also to interrupt prolonged periods of inactivity with small PA “snacks” (78, 79). Strategies such as “sitting less and moving more” or “physical activity snacks” (79) could be adapted to pediatric populations pursuing a goal: replacing sedentary behavior with any intensity of physical activity (that is, movement) that will have health benefits, but with greater benefits seen when sedentary behavior is replaced with moderate-vigorous- intensity physical activity (80, 81). This type of intervention would be even more important in the case of children and adolescents with obesity for whom introducing and maintaining MVPA is complicated. A school and/or family-level intervention focused on reducing ST (and prolonged periods) through short periods of intense PA could be a successful alternative. The effect of this type of intervention to reduce sedentary behaviour on blood pressure levels in children and adolescents has not been rigorously evaluated. We can only say that there is good evidence that PA of at least moderate to vigorous intensity has a beneficial effect on blood pressure control in youth with hypertension.
3.4. Screen exposure
Screen time has become a ubiquitous part of modern childhood. Many children are spending hours each day in front of screens watching TV, using a smartphone, computer or playing video games. The American Academy of Pediatrics and the World Health Organization (WHO) have recommended avoiding screen use completely in children under 18 to 24 months and limiting screen time to 1 h a day in children aged 2–5 years (49). While there is no agreement on the amount of leisure screen time that is considered to be harmful for older children, it is generally suggested that this time should be limited to two hours per day. Multiple studies have shown a positive association between screen time and both hypertension and obesity in children (82, 83). The connection between increased screen time and obesity and higher BP levels could be due to various factors. Excessive screen time leads to less physical activity, and very often to eating large amounts of food, usually unhealthy snacks and sugary drinks which can promote weight gain (84). The marketing of unhealthy foods and drinks through screen-based media may also be contributing to the development of obesity. Furthermore, screen time can lead to disrupted sleep patterns, which can have negative effects on both hypertension and obesity (82). In addition, the habit of excessive TV viewing in younger children is tracking to later childhood and adolescence and thus exposes the child to a long period of unhealthy, sedentary behavior (85). Prolonged TV viewing and total screen time during leisure time in adolescence are associated with unfavorable levels of several cardiovascular risk factors in young adulthood. Nagata et al. conducted a study with the aim of determining the potential links between screen time in childhood and cardiometabolic risk in adulthood over a 24-year period. Their findings indicated that greater screen time during adolescence was associated with an increased likelihood of certain cardiometabolic disease indicators in adulthood (86). Hagjoo et al. in a meta-analysis of a large sample size (n = 112,489) also reported that high screen time is associated with a 1.27 times higher chance of overweight/obesity in adolescents, with TV watching being the most influential factor (87). Zulfiqar et al. reported a positive association between obesity and TV watching, but not with video games (88). According to Lopez Gonzalez's research, in a population-based cross-sectional study that evaluated 1,463 children and adolescents, high screen time was identified as obesogenic (89). Stabouli et al. found that screen time is linked to hypertension and obesity in children. Each additional hour of weekly screen time increased the likelihood of hypertension by 1.18 times in adolescents, even after adjusting for other risk factors (90).
4. Disrupted circadian rhythms
4.1. The circadian rhythms
The circadian rhythms are driven by a central clock located in suprachiasmatic nucleus and various peripheral clocks located in different tissues and organs of the body (91). The process of metabolism is also under circadian regulation. Disturbances of synchronization between the internal clock and environmental timers result in disruption of the circadian rhythms that seriously impact metabolic homeostasis leading to changed eating behavior, altered glucose and lipid metabolism, and weight gain. This in turn augments the risk of having various cardio-metabolic disorders such as obesity, diabetes, metabolic syndrome, and cardiovascular disease. Blood pressure follows a circadian rhythm, it increases on waking in the morning and decreases during sleeping at night (92). Disruption of the circadian BP rhythm has been reported to be associated with worsened cardiovascular and renal outcomes. Growing evidence from animal models has found that the circadian rhythms of BP can be distinctly altered by genetic manipulation of the core clock genes (93). An explicit mechanism has not been explained between chronic kidney disease and disruption of circadian BP rhythm. It has been shown, that an excessive circadian variation in BP is also associated with an increased risk of nephropathy. Probably due to this data, the application of anti-hypertensive drugs according to this information should be change for rational and effective administration time.
4.2. Sleep disorders
The most overt circadian rhythm is the sleep–wake cycle. Healthy night sleep is necessary for the full functioning of the brain, memory, regulation of metabolic processes, maturation and growth. Sufficient sleep is essential for maintaining healthy physical, mental, and emotional functioning. It has been shown by The National Health and Nutrition Examination Survey (NHANES) that there is a link between too few hours of daily sleep at night and a higher prevalence of obesity (94). The relationship between sleep disorders and increased cardiovascular risk in adults is clear. The literature on cardiovascular consequences of sleep disorders in children is not as robust, but there is some evidence of BP disturbances in children with sleep deprivation and obstructive sleep apnea (95). An inadequate number of hours of sleep or its disturbed course cause adverse effects on mood and behavior control, overexcitement in the cognitive-emotional and behavioral spheres, as well as in the autonomic or central nervous systems, and hyperactivation of hypotalamic-pituitary-adrenal axis (96). This is manifested in an increase in heart rate and a decrease in heart rate variability during the day, an increase in body temperature, and an increase in the ACTH and cortisol secretion (97). The same mechanisms contribute to the development of arterial hypertension. The prevalence of arterial hypertension in children with sleep-disordered breathing is 14%, which is almost three times more than in the general population (98). On the other side, it has been demonstrated by Hinkle et al, that 23% of children with elevated BP snored, and in those that underwent polysomnography, 80% were diagnosed with obstructive sleep apnea (99). In another study, it was shown that children with apnea/hypopnea index (AHI) > 5 had higher prevalence of systolic hypertension (57%) when compared with non-snorers or children with AHI < 5 (100). Children who sleep less than the age-adjusted amount of sleep (9–11 h for children at the age of 6–12, 8–10 h for older adolescents) are also more likely to have high BP (101). As it has been revealed less than a third of adolescents in the USA obtain the recommended amount of sleep for age on school nights (102). During adolescence the circadian timing system undergoes a phase delay. For this reason, the time to fall asleep in the evening and the time to wake up naturally in the morning are naturally delayed. This, together with the need to adapt to the rhythm imposed by social functioning and school, can reduce the number of hours of sleep. In addition, in the evening and night using the screens, social-network engagement become more available as youngsters pass through adolescence (103).
5. Family and social relations
5.1. Unfavorable family interactions
Frequent and unfavorable interactions within family units are associated with a higher risk for chronic illnesses, such as cardiovascular disease (104). The family environment and negative childhood events significantly influence the development of cardiovascular disease (105). Dysfunctional family and negative family dynamics play a significant role in the development of hypertension (106). Previous 23-year longitudinal research found that negative experiences predict high BP (107). Additionally, lower BP in children aged 15 was predicted by parental sensitivity (i.e., observer assessments of emotional support during a difficult task) in the early years (108). Moreover, family connection dynamics and poor sleep quality both raise the risk of hypertension (109).
5.2. Bullying
Bullying at school between classmates is a globally acknowledged problem (110). Bullying is understood as repeated physical or verbal abuse where the aggressor is more dominant than the victim (111). Obesity in children and adolescents is linked to worse quality of life and more stigma or unfair treatment. Decreased school motivation, sadness, depression, psychosomatic issues, loss of sleep, and even suicide are possible outcomes for bullied individuals (112). A meta-analysis indicates that both youths with obesity or overweight experience significantly more bullying than normal-weight youths (113). To our knowledge, no research has directly identified bullying at school as a risk factor for hypertension in children and adolescents. However, several studies have found a link between experiencing bullying and reporting poor general health and also with exhibiting unhealthy dietary habits (114–123).
5.3. Chronic stress, mood disorders, depression
The American Heart Association published a scientific statement in 2015 that recognized mood disorders, such as major depressive disorder or bipolar disorder, as moderate-risk conditions for early cardiovascular disease among youth (124). Since then, several cross sectional and longitudinal studies endorsed this statement, reporting evidence of the association between mood dysregulation and medical conditions, such as obesity or hypertension, in children and adolescents. A study conducted in Turkey, the European country with the highest rates of overweight and obesity, found increased eating behaviours associated with negative feelings in preadolescents, possibly as a coping strategy, that can result in weight gain (125, 126). Regarding the direct relationship of mood disorders with obesity and hypertension in children and adolescents, Medrano et al. reported results about cardiovascular risk factors in youth with overweight and obesity (127). In this population, they found that mood disorders were associated with elevated adiposity levels and higher rates of hypertension, although those with mood disorders were also older. Moreover, another cross-sectional study found that adolescents and young adults with bipolar disorder showed twice the prevalence of metabolic syndrome than the general population (128). Also, in relation to cardiovascular diseases (CVD), Waloszek et al. found that adolescents with depressive symptoms had poorer vascular functioning and higher risk of CVD than adolescents without depressive symptoms, and Korczak et al. reported that children and adolescents with depression presented a higher rates of early CVD among family members (129, 130). Longitudinal evidence has also been reported in this regard. A prospective cohort study with adolescents found a relationship between high levels of depression, obesity and poor dietary and physical activity behaviours, reinforcing the idea of a negative feedback loop between depressive symptoms, poor diet, low physical activity and obesity (131). However, another prospective study reported that female children with higher negative affect showed decreased physical activity in the longitudinal follow-up, but male children showed the inverse effect, with increased physical activity associated with higher negative affect suggesting sex differences in the relationship of these factors. Chronic emotional stress is another relevant factor that has also been associated with the cardiovascular health of young people as it may result into the development of obesity and/or hypertension suggesting sex differences about the relationship of these factors (132–136). A longitudinal study reported that adverse experiences, that can result in chronic stress, were associated with the development of obesity during childhood, even when these events occurred at very early stages of the development (137). In the same line, another longitudinal study also assessed the relationship between child adverse events and BMI and found a relationship between adverse events during childhood or adolescence and increased adult BMI (138). This would suggest that the negative impact of the stress response to adverse events might take some time to express in the BMI. However, other recent prospective results suggest that obesity may be driving stress related biological factors, rather than preceding them (139). Socioeconomic adversities could also produce elevated chronic stress during childhood and adolescence and, therefore, be a risk factor to develop obesity during childhood (134). However, Olstad et al. found no relation between hair cortisol levels and BMI in children with low socioeconomic status, suggesting that obesity development would be more related to psych behavioural factors (140). However, another study found that racial discrimination and low household education were associated relationship with BMI in children and adolescents, but not the perceived stress (141). Both mood and chronic stress seem to have an impact on cardiovascular health of children and adolescents. They may produce a direct effect by disturbing the physiological and psychological functioning, and also an effect via unhealthy behavior such as poor sleep patterns, low physical activity, unhealthy eating or consumption of alcohol and tobacco (142).
5.5. Social environment
The social environment is also extremely important in the prevention, treatment of obesity and the prevention of its complications. Appropriate social conditions and support for children from risk groups are of great importance. Building public–private partnerships, engaging all entities to cooperate, in particular nongovernmental organizations promoting a healthy lifestyle, consumers, organizations, and private sector entities, including the food industry and media—joint activities to promote healthy behavior (143).
6. Urbanization, noise, and environmental pollution
Changes related to the development of civilization may be important factors in increasing the frequency of non-communicable diseases in pediatric population, including arterial hypertension. Emerging risk factors include pollution (air, water, noise, and light), urbanization, and a loss of green space. This effect may be direct or indirect due to higher prevalence of obesity and overweight. Studies of recent years, mainly from Asian or African countries, clearly show that the progressing urbanization process, sedentary lifestyle, and poor dietary habits are a few new factors that might contribute to a higher prevalence of these problems not only in adults, but also in children (144). Recent findings demonstrate also that such endocrine-disrupting chemicals, termed “obesogens”, can promote adipogenesis and cause weight gain. This includes compounds to which the human population is exposed in daily life through their use in pesticides/herbicides, industrial and household products, plastics, detergents, flame retardants and as ingredients in personal care products (145). A study conducted in Ghana found that BP levels were lower in the rural population than in the semi-urban and urban children (146). Research conducted in China confirmed that in comparison with urban children, rural children had a lower prevalence of arterial hypertension (especially isolated diastolic hypertension) (147). Finally, as shown in a recently published meta-analysis by Meena et al. arterial hypertension in India is diagnosed in 7% of urban children 7% (95% CI: 6%–9%), compared to 5% in rural counterparts 5% (95% CI: 3%–6%) (144). Urbanization is undoubtedly associated with increased exposure to noise, mainly from transport, and air pollution. The existing literature of both mechanistic and epidemiological design strongly points towards a relationship between exposure to transportation noise and elevated BP in adults (148). Nevertheless, the association between transportation noise and BP in children has also been investigated. In a meta-analysis by Dzhambov et al. authors reported that a 5 dB rise in road traffic noise at kindergarten/school was associated with a 0.48 mmHg higher systolic BP [95% CI (−0.87–1.83)] and a 0.22 mmHg higher diastolic BP [95% CI (−0.64–1.07)] (149). Many epidemiological studies indicate that nocturnal noise exposure (especially aircraft or railway noise) may be more relevant for cardiovascular health than daytime noise exposure (150). The effect of noise on increasing BP is multidirectional. Starting from sleep, communication and daily activities annoyance. That chronic annoyance causes stress characterized by increased levels of stress hormones such as cortisol and catecholamines, which causes permanent changes leading to an increase in BP (151). Although limited data suggest air pollution exposures may contribute to pediatric high BP, the results of the recently published CANDLE study clearly confirm such a relationship. Moreover, as shown by the authors even prenatal air pollution exposure, particularly in the second trimester, is associated with elevated early childhood BP (152). Short and long-term exposure to air pollution provokes oxidative stress, systemic inflammation, and autonomic nervous system imbalance that subsequently induce endothelial dysfunction and vasoconstriction leading to increased BP (153).
7. Differences in cardiovascular risk in girls and boys
Sex differences in BP in children and adolescents are well known (154). In general, girls exhibit lower BP compared to boys. BP rises with age, with this increase not attributed to the process of aging itself, but rather linked to the increase in body size (155). During adolescence the differences in BP between girls and boys become more prominent (156). BP difference between the sexes is primarily influenced by lower stroke volume as well as lower total peripheral resistance. There is a positive correlation between intra-abdominal fat and BP, albeit less significant in girls than in boys. Additionally, factors such as fat-free mass, fat mass, and insulin resistance are also positively associated with BP, with similar patterns observed in both girls and boys (156). These associations, combined with the sex-specific variations in body composition and metabolism, contribute to the observed BP differences between the sexes. In pre-pubertal girls (aged 6–11 years) BP seems to be associated also with age independent of body size and adiposity; this association is not observed in boys (157). Among 6 to 11-year-old children, the prevalence of hypertension is higher in girls compared to boys with a higher prevalence of elevated diastolic BP in girls (158). A retrospective study assessing 74,233 children showed male sex to be associated with high BP and a possible association between material and social deprivation and high BP, particularly in boys (159). A longitudinal study on adolescents (aged 12–18 years) found that boys had a greater likelihood of developing high systolic BP compared to girls (160). These differences regarding BP and hypertension have to be in context with the known differences in obesity. Girls tend to have lower rates of obesity than boys depending on the country (161). Boys typically have more visceral fat, while girls tend to accumulate more subcutaneous fat (162). Despite this, a study showed significant associations of visceral fat with cholesterol subclasses were only in girls (163). Another study reported that girls with higher BMI have a greater left ventricular muscle mass (LVMI) compared to boys with comparable BMI (164). Additionally, in youth, masked hypertension is a precursor of sustained hypertension. The risk of developing sustained hypertension is higher in boys than it is for girls (165). These recent reports challenge the general view that cardiovascular risk is greater in boys, but point towards a greater vulnerability in girls under specific circumstances. More research is needed to address this issue.
7. Closing remarks
Obesity is a relevant risk factor whose prevalence is progressively increasing and involves childhood and adolescence with a negative impact for future generations. The problem of obesity in children is that of the associated hypertension and other risk factors for cardiovascular and metabolic disease that have been repeatedly described in cross-sectional and longitudinal epidemiology. The increase in body weight is just the quantifiable evidence of an underlying involvement of cardiometabolic system progressively leading prospectively to an impaired quality of life and general health. This pathophysiological interaction introduces the concept that the management of obesity in childhood should take into consideration a well-designed strategy aimed at controlling body weight but also any additional risk factor involved in cardiovascular and metabolic abnormalities, in particular hypertension. Dietary measures are the cornerstone of prevention through a correct and well-adjusted nutritional approach including healthy foods, proper hydration, and the prevention of dangerous lifestyle habits (smoking, alcohol, drugs). One of the main causes of obesity/hypertension in children is the progressive decrease of physical activity that has been progressively overcome by a remarkable “static” activity based on the extensive use of interacting devices that is clearly expressed by the new measure of physical inactivity that is the duration of “screen time” that can be particularly dangerous when combined with alterations of circadian rhythms. An increase in the exposure to screen-based interfaces particularly during the night or early morning can be responsible for a remarkable disruption of the individual neuro-humoral response that can have a negative impact on energetic metabolism and autonomic response leading to those significant changes in BP organ damage that are frequently observed in overweight adolescent subjects. In particular, the increase in the isolation behaviors either physical (self-restriction) or functional (extensive use of ear sets of any kind) can significantly affect both exercise capacity and mental attitude toward reciprocal negative relationship resulting in a positive energy balance that can promote childhood obesity predisposing to cardio-metabolic disease in adult life. As expected, a cornerstone in the prevention of obesity and its consequences in terms of cardiovascular and metabolic disease is the quality of the “environment” surrounding children and adolescents during their daily life. The most important is the “human” environment that involves both the family and the peers (friends, classmate, teammate, etc). than can directly or indirectly contribute to food disorders but also to the abnormalities of BP and metabolic control with a negative impact on cardiometabolic profile. An improvement in the functionality of family group and a constructive influence by the many daily contacts may largely prevent dangerous behaviors and promote an easy lifestyle with some advantages in terms of weight control As far as the” natural” environment, many recent studies have emphasized the negative impact of air and acoustic pollution on cardiovascular health with a significant increase in the rate of myocardial infarction and stroke in the adult population. Both these risk factors can increase the sympathetic stimulation, the endothelial damage, and the insulin sensitivity with an unfavorable influence on the interaction between environmental risk factors, overweight, and BP. All these specific conditions, combined with the sex-specific variations in body composition and metabolism, may support the observed differences in BP and cardiovascular risk between the sexes. The evidence reported in this paper challenges the general view that cardiovascular risk is greater in boys but point towards a greater vulnerability in girls under specific circumstances and this is something that is needed to be addressed more deeply in the future.
8. Conclusion
The problem of obesity and hypertension is progressively growing increasing the risk of cardiovascular disease in the next adult generation. The early identification of the all possible and emerging risk factors in childhood is a cornerstone for present and future approach to cardiovascular prevention in the general population.
Author contributions
MW: Conceptualization, Formal Analysis, Investigation, Methodology, Project administration, Resources, Supervision, Writing – original draft, Writing – review & editing. JÁ: Conceptualization, Investigation, Methodology, Project administration, Resources, Writing – original draft, Writing – review & editing. AK: Investigation, Methodology, Writing – original draft, Writing – review & editing. MB: Visualization, Writing – original draft, Writing – review & editing. RG: Writing – original draft, Writing – review & editing. VH: Writing – original draft, Writing – review & editing. EW: Conceptualization, Funding acquisition, Project administration, Resources, Supervision, Validation, Writing – original draft, Writing – review & editing. IL: Writing – original draft, Writing – review & editing. DR: Writing – original draft, Writing – review & editing. AnM: Writing – original draft, Writing – review & editing. BG: Writing – original draft, Writing – review & editing. FF: Writing – original draft, Writing – review & editing. ArM: Writing – original draft, Writing – review & editing. EL: Funding acquisition, Project administration, Writing – original draft, Writing – review & editing. CB: Writing – original draft, Writing – review & editing. DD: Funding acquisition, Investigation, Methodology, Project administration, Resources, Supervision, Validation, Writing – original draft, Writing – review & editing.
Funding
The author(s) declare financial support was received for the research, authorship, and/or publication of this article.
This publication is based on the work of the COST Action HyperChildNET (CA19115), with the support of COST (European Cooperation in Science and Technology) and the funding of the European Union.
Conflict of interest
The authors declare that the research was conducted in the absence of any commercial or financial relationships that could be construed as a potential conflict of interest.
The authors declared that they were an editorial board member of Frontiers, at the time of submission. This had no impact on the peer review process and the final decision.
Publisher's note
All claims expressed in this article are solely those of the authors and do not necessarily represent those of their affiliated organizations, or those of the publisher, the editors and the reviewers. Any product that may be evaluated in this article, or claim that may be made by its manufacturer, is not guaranteed or endorsed by the publisher.
References
1. Hampl SE, Hassink SG, Skinner AC, Armstrong SC, Barlow SE, Bolling CF, et al. Clinical practice guideline for the evaluation and treatment of children and adolescents with obesity. Pediatrics. (2023) 151(2):e2022060640. doi: 10.1542/peds.2022-060640
2. Cuda S, Censani M. Progress in pediatric obesity: new and advanced therapies. Curr Opin Pediatr. (2022) 34(4):407–13. doi: 10.1097/MOP.0000000000001150
3. Drozdz D, Alvarez-Pitti J, Wójcik M, Borghi C, Gabbianelli R, Mazur A, et al. Obesity and cardiometabolic risk factors: from childhood to adulthood. Nutrients. (2021) 13(11):4176. doi: 10.3390/nu13114176
4. Song P, Zhang Y, Yu J, Zha M, Zhu Y, Rahimi K, et al. Global prevalence of hypertension in children. JAMA Pediatr. (2019) 173(12):1154. doi: 10.1001/jamapediatrics.2019.3310
5. Tong J, An X, Zhao L, Qu P, Tang X, Chen M, et al. Combining multiaspect factors to predict the risk of childhood hypertension incidence. J Clin Hypertens. (2022) 24(8):1015–25. doi: 10.1111/jch.14544
6. Schwingshackl L, Schwedhelm C, Hoffmann G, Knüppel S, Iqbal K, Andriolo V, et al. Food groups and risk of hypertension: a systematic review and dose-response meta-analysis of prospective studies. Adv Nutr. (2017) 8(6):793–803. doi: 10.3945/an.117.017178
7. Ye Z, Wu Q, Yang S, Zhang Y, Zhou C, Liu M, et al. Variety and quantity of dietary insoluble fiber intake from different sources and risk of new-onset hypertension. BMC Med. (2023) 21(1):61. doi: 10.1186/s12916-023-02752-7
8. Ju Y, Zhang C, Zhang Z, Zhu H, Liu Y, Liu T, et al. Effect of dietary fiber (oat bran) supplement in heart rate lowering in patients with hypertension: a randomized DASH-diet-controlled clinical trial. Nutrients. (2022) 14(15):3148. doi: 10.3390/nu14153148
9. Reynolds AN, Akerman A, Kumar S, Diep Pham HT, Coffey S, Mann J. Dietary fibre in hypertension and cardiovascular disease management: systematic review and meta-analyses. BMC Med. (2022) 20(1):139. doi: 10.1186/s12916-022-02328-x
10. Ong KL, Marklund M, Huang L, Rye KA, Hui N, Pan XF, et al. Association of omega 3 polyunsaturated fatty acids with incident chronic kidney disease: pooled analysis of 19 cohorts. Br Med J. (2023):e072909. doi: 10.1136/bmj-2022-072909
11. Bonnet F, Lepicard EM, Cathrin L, Letellier C, Constant F, Hawili N, et al. French children start their school day with a hydration deficit. Ann Nutr Metab. (2012) 60(4):257–63. doi: 10.1159/000337939
12. Bougatsas D, Arnaoutis G, Panagiotakos DB, Seal AD, Johnson EC, Bottin JH, et al. Fluid consumption pattern and hydration among 8–14 years-old children. Eur J Clin Nutr. (2018) 72(3):420–7. doi: 10.1038/s41430-017-0012-y
13. Kavouras SA, Bougatsas D, Johnson EC, Arnaoutis G, Tsipouridi S, Panagiotakos DB. Water intake and urinary hydration biomarkers in children. Eur J Clin Nutr. (2017) 71(4):530–5. doi: 10.1038/ejcn.2016.218
14. Kozioł-Kozakowska A, Piórecka B, Suder A, Jagielski P. Body composition and a school day hydration state among Polish children—a cross-sectional study. Int J Environ Res Public Health. (2020) 17(19):7181. doi: 10.3390/ijerph17197181
15. Michels N, Van den Bussche K, Vande Walle J, De Henauw S. Belgian Primary school children’s hydration status at school and its personal determinants. Eur J Nutr. (2017) 56(2):793–805. doi: 10.1007/s00394-015-1126-4
16. Padrao P, Neto M, Pinto M, Oliveira AC, Moreira A, Moreira P. Urinary hydration biomarkers and dietary intake in children. Nutr Hosp. (2016) 33(Suppl 3):314. doi: 10.20960/nh.314
17. Stahl A, Kroke A, Bolzenius K, Manz F. Relation between hydration status in children and their dietary profile—results from the DONALD study. Eur J Clin Nutr. (2007) 61(12):1386–92. doi: 10.1038/sj.ejcn.1602663
18. Stookey JD, Brass B, Holliday A, Arieff A. What is the cell hydration status of healthy children in the USA? Preliminary data on urine osmolality and water intake. Public Health Nutr. (2012) 15(11):2148–56. doi: 10.1017/S1368980011003648
19. Maffeis C, Tommasi M, Tomasselli F, Spinelli J, Fornari E, Scattolo N, et al. Fluid intake and hydration status in obese vs normal weight children. Eur J Clin Nutr. (2016) 70(5):560–5. doi: 10.1038/ejcn.2015.170
20. Kozioł-Kozakowska A, Wójcik M, Stochel-Gaudyn A, Szczudlik E, Suder A, Piórecka B. The severity of obesity promotes greater dehydration in children: preliminary results. Nutrients. (2022) 14(23):5150. doi: 10.3390/nu14235150
21. Celik A, Cebeci AN. Evaluation of hydration status of children with obesity—a pilot study. J Pediatr Endocrinol Metab. (2021) 34(3):295–300. doi: 10.1515/jpem-2020-0495
22. Piórecka B, Jamka-Kasprzyk M, Niedźwiadek A, Jagielski P, Jurczak A. Fluid intake and the occurrence of erosive tooth wear in a group of healthy and disabled children from the MaAopolska region (Poland). Int J Environ Res Public Health. (2023) 20(5):4585. doi: 10.3390/ijerph20054585
23. OECD (2021) Smoking and alcohol consumption among adolescents. Health at a Glance 2021: OECD Indicators, Paris.
24. Ivorra C, Fraga MF, Bayón GF, Fernández AF, Garcia-Vicent C, Chaves FJ, et al. DNA Methylation patterns in newborns exposed to tobacco in utero. J Transl Med. (2015) 13(1):25. doi: 10.1186/s12967-015-0384-5
25. Ivorra C, García-Vicent C, Ponce F, Ortega-Evangelio G, Fernández-Formoso JA, Lurbe E. High cotinine levels are persistent during the first days of life in newborn second hand smokers. Drug Alcohol Depend. (2014) 134:275–9. doi: 10.1016/j.drugalcdep.2013.10.017
26. Aryanpur M, Yousefifard M, Oraii A, Heydari G, Kazempour-Dizaji M, Sharifi H, et al. Effect of passive exposure to cigarette smoke on blood pressure in children and adolescents: a meta-analysis of epidemiologic studies. BMC Pediatr. (2019) 19(1):161. doi: 10.1186/s12887-019-1506-7
27. Banderali G, Martelli A, Landi M, Moretti F, Betti F, Radaelli G, et al. Short and long term health effects of parental tobacco smoking during pregnancy and lactation: a descriptive review. J Transl Med. (2015) 13(1):327. doi: 10.1186/s12967-015-0690-y
28. Lauria L, Lamberti A, Grandolfo M. Smoking behaviour before, during, and after pregnancy: the effect of breastfeeding. The Scientific World Journal. (2012) 2012:1–9. doi: 10.1100/2012/154910
29. Ino T. Maternal smoking during pregnancy and offspring obesity: meta-analysis. Pediatr Int. (2010) 52(1):94–9. doi: 10.1111/j.1442-200X.2009.02883.x
30. Oken E, Levitan EB, Gillman MW. Maternal smoking during pregnancy and child overweight: systematic review and meta-analysis. Int J Obes. (2008) 32(2):201–10. doi: 10.1038/sj.ijo.0803760
31. Bruin JE, Gerstein HC, Holloway AC. Long-term consequences of fetal and neonatal nicotine exposure: a critical review. Toxicol Sci. (2010) 116(2):364–74. doi: 10.1093/toxsci/kfq103
32. Cohen G, Jeffery H, Lagercrantz H, Katz-Salamon M. Long-Term reprogramming of cardiovascular function in infants of active smokers. Hypertension. (2010) 55(3):722–8. doi: 10.1161/HYPERTENSIONAHA.109.142695
33. Charakida M, Georgiopoulos G, Dangardt F, Chiesa ST, Hughes AD, Rapala A, et al. Early vascular damage from smoking and alcohol in teenage years: the ALSPAC study. Eur Heart J. (2019) 40(4):345–53. doi: 10.1093/eurheartj/ehy524
34. Peterson LA, Hecht SS. Tobacco, e-cigarettes, and child health. Curr Opin Pediatr. (2017) 29(2):225–30. doi: 10.1097/MOP.0000000000000456
35. Poorolajal J, Sahraei F, Mohamdadi Y, Doosti-Irani A, Moradi L. Behavioral factors influencing childhood obesity: a systematic review and meta-analysis. Obes Res Clin Pract. (2020) 14(2):109–18. doi: 10.1016/j.orcp.2020.03.002
36. Dare S, Mackay DF, Pell JP. Relationship between smoking and obesity: a cross-sectional study of 499,504 middle-aged adults in the UK general population. PLoS One. (2015) 10(4):e0123579. doi: 10.1371/journal.pone.0123579
37. Huang DYC, Lanza HI, Wright-Volel K, Anglin MD. Developmental trajectories of childhood obesity and risk behaviors in adolescence. J Adolesc. (2013) 36(1):139–48. doi: 10.1016/j.adolescence.2012.10.005
38. Lanza HI. Weighing the risk: developmental pathways and processes underlying obesity to substance use in adolescence. J Res Adolesc. (2022) 32(1):337–54. doi: 10.1111/jora.12610
39. Pasch KE, Velazquez CE, Cance JD, Moe SG, Lytle LA. Youth substance use and body composition: does risk in one area predict risk in the other? J Youth Adolesc. (2012) 41(1):14–26. doi: 10.1007/s10964-011-9706-y
40. Soneji S, Barrington-Trimis JL, Wills TA, Leventhal AM, Unger JB, Gibson LA, et al. Association between initial use of e-cigarettes and subsequent cigarette smoking among adolescents and young adults. JAMA Pediatr. (2017) 171(8):788. doi: 10.1001/jamapediatrics.2017.1488
41. Kallio K, Jokinen E, Raitakari OT, Hämäläinen M, Siltala M, Volanen I, et al. Tobacco smoke exposure is associated with attenuated endothelial function in 11-year-old healthy children. Circulation. (2007) 115(25):3205–12. doi: 10.1161/CIRCULATIONAHA.106.674804
42. Jacobs DR, Woo JG, Sinaiko AR, Daniels SR, Ikonen J, Juonala M, et al. Childhood cardiovascular risk factors and adult cardiovascular events. N Engl J Med. (2022) 386(20):1877–88. doi: 10.1056/NEJMoa2109191
43. Tasnim S, Tang C, Musini VM, Wright JM. Effect of alcohol on blood pressure. Cochrane Database Syst Rev. (2020) 7(7):CD012787. doi: 10.1002/14651858.CD012787.pub2
44. Fuchs FD, Fuchs SC. The effect of alcohol on blood pressure and hypertension. Curr Hypertens Rep. (2021) 23(10):42. doi: 10.1007/s11906-021-01160-7
45. Husain K, Ansari RA, Ferder L. Alcohol-induced hypertension: mechanism and prevention. World J Cardiol. (2014) 6(5):245. doi: 10.4330/wjc.v6.i5.245
46. Lucas DL, Brown RA, Wassef M, Giles TD. Alcohol and the cardiovascular system. J Am Coll Cardiol. (2005) 45(12):1916–24. doi: 10.1016/j.jacc.2005.02.075
47. Piano MR. Alcohol’s effects on the cardiovascular system. Alcohol Res. (2017) 38(2):219–41.28988575
48. Middlekauff HR, Cooper ZD, Strauss SB. Drugs of misuse: focus on vascular dysfunction. Can J Cardiol. (2022) 38(9):1364–77. doi: 10.1016/j.cjca.2022.04.011
49. Guidelines on Physical Activity, Sedentary Behaviour and Sleep for Children under 5 Years of Age. WHO Guidelines Approved by the Guidelines Review Committee. Geneva 2019. (2019).
50. Tremblay M. Letter to the editor: standardized use of the terms “sedentary” and “sedentary behaviours.”. Appl Physiol Nutr Metab. (2012) 37:540–2. doi: 10.1139/h2012-024
51. Tremblay MS, Aubert S, Barnes JD, Saunders TJ, Carson V, Latimer-Cheung AE, et al. Sedentary behavior research network (SBRN)—terminology consensus project process and outcome. Int J Behav Nutr Phys Act. (2017) 14(1):75. doi: 10.1186/s12966-017-0525-8
52. Saint-Maurice PF, Kim Y, Welk GJ, Gaesser GA. Kids are not little adults: what MET threshold captures sedentary behavior in children? Eur J Appl Physiol. (2016) 116(1):29–38. doi: 10.1007/s00421-015-3238-1
53. Lau M, Wang L, Acra S, Buchowski MS. Energy expenditure of common sedentary activities in youth. J Phys Act Health. (2016) 13(s1):S17–20. doi: 10.1123/jpah.2015-0727
54. Butte NF, Watson KB, Ridley K, Zakeri IF, Mcmurray RG, Pfeiffer KA, et al. A youth compendium of physical activities. Med Sci Sports Exerc. (2018) 50(2):246–56. doi: 10.1249/MSS.0000000000001430
55. Sallis JF, Bull F, Guthold R, Heath GW, Inoue S, Kelly P, et al. Progress in physical activity over the Olympic quadrennium. Lancet. (2016) 388(10051):1325–36. doi: 10.1016/S0140-6736(16)30581-5
56. Guthold R, Stevens GA, Riley LM, Bull FC. Global trends in insufficient physical activity among adolescents: a pooled analysis of 298 population-based surveys with 1·6 million participants. Lancet Child Adolesc Health. (2020) 4(1):23–35. doi: 10.1016/S2352-4642(19)30323-2
57. van Ekris E, Wijndaele K, Altenburg TM, Atkin AJ, Twisk J, Andersen LB, et al. Tracking of total sedentary time and sedentary patterns in youth: a pooled analysis using the international children’s accelerometry database (ICAD). Int J Behav Nutr Phys Act. (2020) 17(1):65. doi: 10.1186/s12966-020-00960-5
58. Elmesmari R, Martin A, Reilly JJ, Paton JY. Comparison of accelerometer measured levels of physical activity and sedentary time between obese and non-obese children and adolescents: a systematic review. BMC Pediatr. (2018) 18(1):106. doi: 10.1186/s12887-018-1031-0
59. Helgadóttir B, Fröberg A, Kjellenberg K, Ekblom Ö, Nyberg G. COVID-19 induced changes in physical activity patterns, screen time and sleep among Swedish adolescents—a cohort study. BMC Public Health. (2023) 23(1):380. doi: 10.1186/s12889-023-15282-x
60. Okely AD, Trost SG, Steele JR, Cliff DP, Mickle K. Adherence to physical activity and electronic media guidelines in Australian pre-school children. J Paediatr Child Health. (2009) 45(1–2):5–8. doi: 10.1111/j.1440-1754.2008.01445.x
61. Calcaterra V, Verduci E, Vandoni M, Rossi V, Di Profio E, Carnevale Pellino V, et al. Telehealth: a useful tool for the management of nutrition and exercise programs in pediatric obesity in the COVID-19 era. Nutrients. (2021) 13(11):3689. doi: 10.3390/nu13113689
62. Nogueira-de-Almeida CA, Del Ciampo LA, Ferraz IS, Del Ciampo IRL, Contini AA, da V Ued F. COVID-19 and obesity in childhood and adolescence: a clinical review. J Pediatr (Rio J). (2020) 96(5):546–58. doi: 10.1016/j.jped.2020.07.001
63. Carson V, Hunter S, Kuzik N, Gray CE, Poitras VJ, Chaput JP, et al. Systematic review of sedentary behaviour and health indicators in school-aged children and youth: an update. Appl Physiol Nutr Metab. (2016) 41(6 Suppl 3):S240–65. doi: 10.1139/apnm-2015-0630
64. Thivel D, Tremblay MS, Katzmarzyk PT, Fogelholm M, Hu G, Maher C, et al. Associations between meeting combinations of 24-hour movement recommendations and dietary patterns of children: a 12-country study. Prev Med (Baltim). (2019) 118:159–65. doi: 10.1016/j.ypmed.2018.10.025
65. Saunders TJ, Chaput JP, Tremblay MS. Sedentary behaviour as an emerging risk factor for cardiometabolic diseases in children and youth. Can J Diabetes. 2014;38(1):53–61. doi: 10.1016/j.jcjd.2013.08.266
66. Ekelund U, Steene-Johannessen J, Brown WJ, Fagerland MW, Owen N, Powell KE, et al. Does physical activity attenuate, or even eliminate, the detrimental association of sitting time with mortality? A Harmonised Meta-Analysis of Data from More than 1 million men and women. Lancet. (2016) 388(10051):1302–10. doi: 10.1016/S0140-6736(16)30370-1
67. Dunstan DW, Dogra S, Carter SE, Owen N. Sit less and move more for cardiovascular health: emerging insights and opportunities. Nat Rev Cardiol. (2021) 18(9):637–48. doi: 10.1038/s41569-021-00547-y
68. Paterson C, Fryer S, Zieff G, Stone K, Credeur DP, Barone Gibbs B, et al. The effects of acute exposure to prolonged sitting, with and without interruption, on vascular function among adults: a meta-analysis. Sports Med. (2020) 50(11):1929–42. doi: 10.1007/s40279-020-01325-5
69. Dempsey PC, Larsen RN, Dunstan DW, Owen N, Kingwell BA. Sitting less and moving more. Hypertension. (2018) 72(5):1037–46. doi: 10.1161/HYPERTENSIONAHA.118.11190
70. Bergouignan A, Latouche C, Heywood S, Grace MS, Reddy-Luthmoodoo M, Natoli AK, et al. Frequent interruptions of sedentary time modulates contraction- and insulin-stimulated glucose uptake pathways in muscle: ancillary analysis from randomized clinical trials. Sci Rep. (2016) 6(1):32044. doi: 10.1038/srep32044
71. Carter SE, Draijer R, Holder SM, Brown L, Thijssen DHJ, Hopkins ND. Regular walking breaks prevent the decline in cerebral blood flow associated with prolonged sitting. J Appl Physiol. (2018) 125(3):790–8. doi: 10.1152/japplphysiol.00310.2018
72. Dogra S, Wolf M, Jeffrey MP, Foley RCA, Logan-Sprenger H, Jones-Taggart H, et al. Disrupting prolonged sitting reduces IL-8 and lower leg swell in active young adults. BMC Sports Sci Med Rehabil. (2019) 11(1):23. doi: 10.1186/s13102-019-0138-4
73. Akins JD, Crawford CK, Burton HM, Wolfe AS, Vardarli E, Coyle EF. Inactivity induces resistance to the metabolic benefits following acute exercise. J Appl Physiol. 2019;126(4):1088–94. doi: 10.1152/japplphysiol.00968.2018
74. LeBlanc AG, Katzmarzyk PT, Barreira T V., Broyles ST, Chaput JP, Church TS, et al. Correlates of total sedentary time and screen time in 9–11 Year-Old children around the world: the international study of childhood obesity, lifestyle and the environment. PLoS One. (2015) 10(6):e0129622. doi: 10.1371/journal.pone.0129622
75. Bailey DP, Charman SJ, Ploetz T, Savory LA, Kerr CJ. Associations between prolonged sedentary time and breaks in sedentary time with cardiometabolic risk in 10–14-year-old children: the HAPPY study. J Sports Sci. (2017) 35(22):2164–71. doi: 10.1080/02640414.2016.1260150
76. Chinapaw M, Klakk H, Møller NC, Andersen LB, Altenburg T, Wedderkopp N. Total volume versus bouts: prospective relationship of physical activity and sedentary time with cardiometabolic risk in children. Int J Obes. (2018) 42(10):1733–42. doi: 10.1038/s41366-018-0063-8
77. Hjorth MF, Chaput JP, Damsgaard CT, Dalskov SM, Andersen R, Astrup A, et al. Low physical activity level and short sleep duration are associated with an increased cardio-metabolic risk profile: a longitudinal study in 8-11 year old danish children. PLoS One. (2014) 9(8):e104677. doi: 10.1371/journal.pone.0104677
78. Huang CH, Yen M. [Applying an exercise snack-based health promotion strategy]. Hu Li Za Zhi. (2023) 70(2):78–83.
79. Islam H, Gibala MJ, Little JP. Exercise snacks: a novel strategy to improve cardiometabolic health. Exerc Sport Sci Rev. (2022) 50(1):31–7. doi: 10.1249/JES.0000000000000275
80. Stamatakis E, Huang BH, Maher C, Thøgersen-Ntoumani C, Stathi A, Dempsey PC, et al. Untapping the health enhancing potential of vigorous intermittent lifestyle physical activity (VILPA): rationale, scoping review, and a 4-pillar research framework. Sports Med. (2021) 51(1):1–10. doi: 10.1007/s40279-020-01368-8
81. Daley AJ, Griffin RA, Moakes CA, Sanders JP, Skrybant M, Ives N, et al. SnacktivityTM to promote physical activity and reduce future risk of disease in the population: protocol for a feasibility randomised controlled trial and nested qualitative study. Pilot Feasibility Stud. (2023) 9(1):45. doi: 10.1186/s40814-023-01272-8
82. de Jong E, Visscher TLS, HiraSing RA, Heymans MW, Seidell JC, Renders CM. Association between TV viewing, computer use and overweight, determinants and competing activities of screen time in 4- to 13-year-old children. Int J Obes. (2013) 37(1):47–53. doi: 10.1038/ijo.2011.244
83. Ramírez-Coronel AA, Abdu WJ, Alshahrani SH, Treve M, Jalil AT, Alkhayyat AS, et al. Childhood obesity risk increases with increased screen time: a systematic review and dose–response meta-analysis. J Health Popul Nutr. (2023) 42(1):5. doi: 10.1186/s41043-022-00344-4
84. Berentzen NE, Smit HA, van Rossem L, Gehring U, Kerkhof M, Postma DS, et al. Screen time, adiposity and cardiometabolic markers: mediation by physical activity, not snacking, among 11-year-old children. Int J Obes. (2014) 38(10):1317–23. doi: 10.1038/ijo.2014.110
85. De Decker E, De Craemer M, De Bourdeaudhuij I, Wijndaele K, Duvinage K, Androutsos O, et al. Influencing factors of sedentary behavior in European preschool settings: an exploration through focus groups with teachers. J School Health. (2013) 83(9):654–61. doi: 10.1111/josh.12078
86. Nagata JM, Lee CM, Lin F, Ganson KT, Pettee Gabriel K, Testa A, et al. Screen time from adolescence to adulthood and cardiometabolic disease: a prospective cohort study. J Gen Intern Med. (2023) 38(8):1821–7. doi: 10.1007/s11606-022-07984-6
87. Haghjoo P, Siri G, Soleimani E, Farhangi MA, Alesaeidi S. Screen time increases overweight and obesity risk among adolescents: a systematic review and dose-response meta-analysis. BMC Primary Care. (2022) 23(1):161. doi: 10.1186/s12875-022-01761-4
88. Zulfiqar T, Strazdins L, Dinh H, Banwell C, D’Este C. Drivers of overweight/obesity in 4–11 year old children of Australians and immigrants; evidence from growing up in Australia. J Immigr Minor Health. (2019) 21(4):737–50. doi: 10.1007/s10903-018-0841-3
89. Lopez-Gonzalez D, Partida-Gaytán A, Wells JC, Reyes-Delpech P, Avila-Rosano F, Ortiz-Obregon M, et al. Obesogenic lifestyle and its influence on adiposity in children and adolescents, evidence from Mexico. Nutrients. (2020) 12(3):819. doi: 10.3390/nu12030819
90. Stabouli S, Antza C, Vareta G, Efstratiadou M, Sideras L, Kotsis V. “Screen time and blood pressure in children and adolescents: the role of obesity.” Biomed J Sci Tech Res. (2022) 45(5):36941–8. doi: 10.26717/BJSTR.2022.45.007278
91. Zisapel N. New perspectives on the role of melatonin in human sleep, circadian rhythms and their regulation. Br J Pharmacol. (2018) 175(16):3190–9. doi: 10.1111/bph.14116
92. Costello HM, Gumz ML. Circadian rhythm, clock genes, and hypertension: recent advances in hypertension. Hypertension. (2021) 78(5):1185–96. doi: 10.1161/HYPERTENSIONAHA.121.14519
93. Malik A, Kondratov R V., Jamasbi RJ, Geusz ME. Circadian clock genes are essential for normal adult neurogenesis, differentiation, and fate determination. PLoS One. (2015) 10(10):e0139655. doi: 10.1371/journal.pone.0139655
94. Gangwisch JE, Malaspina D, Boden-Albala B, Heymsfield SB. Inadequate sleep as a risk factor for obesity: analyses of the NHANES I. Sleep. (2005) 28(10):1289–96. doi: 10.1093/sleep/28.10.1289
95. DelRosso LM, Mogavero MP, Ferri R. Effect of sleep disorders on blood pressure and hypertension in children. Curr Hypertens Rep. (2020) 22(11):88. doi: 10.1007/s11906-020-01100-x
96. Riemann D, Nissen C, Palagini L, Otte A, Perlis ML, Spiegelhalder K. The neurobiology, investigation, and treatment of chronic insomnia. Lancet Neurol. (2015) 14(5):547–58. doi: 10.1016/S1474-4422(15)00021-6
97. Isayeva G, Shalimova A, Buriakovska O. The impact of sleep disorders in the formation of hypertension. Arterial Hypertension. (2022) 26(4):170–9. doi: 10.5603/AH.a2022.0014
98. Li AM, Au CT, Sung RYT, Ho C, Ng PC, Fok TF, et al. Ambulatory blood pressure in children with obstructive sleep apnoea: a community based study. Thorax. 2008;63(9):803–9. doi: 10.1136/thx.2007.091132
99. Hinkle J, Connolly H V., Adams HR, Lande MB. Severe obstructive sleep apnea in children with elevated blood pressure. J Am Soc Hypertens. (2018) 12(3):204–10. doi: 10.1016/j.jash.2017.12.010
100. Brooks DM, Kelly A, Sorkin JD, Koren D, Chng SY, Gallagher PR, et al. The relationship between sleep-disordered breathing, blood pressure, and urinary cortisol and catecholamines in children. J Clin Sleep Med. (2020) 16(6):907–16. doi: 10.5664/jcsm.8360
101. Fobian AD, Elliott L, Louie T. A systematic review of sleep, hypertension, and cardiovascular risk in children and adolescents. Curr Hypertens Rep. 2018;20(5):42. doi: 10.1007/s11906-018-0841-7
102. Wheaton AG, Olsen EO, Miller GF, Croft JB. Sleep duration and injury-related risk behaviors among high school students — united States, 2007–2013. MMWR Morb Mortal Wkly Rep. (2016) 65(13):337–41. doi: 10.15585/mmwr.mm6513a1
103. Carskadon MA. Sleep in adolescents: the perfect storm. Pediatr Clin North Am. (2011) 58(3):637–47. doi: 10.1016/j.pcl.2011.03.003
104. Vedanthan R, Bansilal S, Soto AV, Kovacic JC, Latina J, Jaslow R, et al. Family-Based approaches to cardiovascular health promotion. J Am Coll Cardiol. (2016) 67(14):1725–37. doi: 10.1016/j.jacc.2016.01.036
105. Chen E, Brody GH, Miller GE. Childhood close family relationships and health. American Psychologist. (2017) 72(6):555–66. doi: 10.1037/amp0000067
106. Su S, Jimenez MP, Roberts CTF, Loucks EB. The role of adverse childhood experiences in cardiovascular disease risk: a review with emphasis on plausible mechanisms. Curr Cardiol Rep. (2015) 17(10):88. doi: 10.1007/s11886-015-0645-1
107. Su S, Wang X, Pollock JS, Treiber FA, Xu X, Snieder H, et al. Adverse childhood experiences and blood pressure trajectories from childhood to young adulthood. Circulation. (2015) 131(19):1674–81. doi: 10.1161/CIRCULATIONAHA.114.013104
108. Boyer BP, Nelson JA. Longitudinal associations of childhood parenting and adolescent health: the mediating influence of social competence. Child Dev. (2015) 86(3):828–43. doi: 10.1111/cdev.12347
109. Gunn HE, Eberhardt KR. Family dynamics in sleep health and hypertension. Curr Hypertens Rep. (2019) 21(5):39. doi: 10.1007/s11906-019-0944-9
110. Nansel TR, Craig W, Overpeck MD, Saluja G, Ruan WJ. Cross-national consistency in the relationship between bullying behaviors and psychosocial adjustment. Arch Pediatr Adolesc Med. (2004) 158(8):730. doi: 10.1001/archpedi.158.8.730
111. Olweus D. Bullying at school: basic facts and effects of a school based intervention program. J Child Psychol Psychiatry. (1994) 35(7):1171–90. doi: 10.1111/j.1469-7610.1994.tb01229.x
112. Gini G, Pozzoli T. Association between bullying and psychosomatic problems: a meta-analysis. Pediatrics. (2009) 123(3):1059–65. doi: 10.1542/peds.2008-1215
113. van Geel M, Vedder P, Tanilon J. Are overweight and obese youths more often bullied by their peers? A meta-analysis on the relation between weight status and bullying. Int J Obes. (2014) 38(10):1263–7. doi: 10.1038/ijo.2014.117
114. Albaladejo-Blázquez N, Ferrer-Cascales R, Ruiz-Robledillo N, Sánchez-Sansegundo M, Clement-Carbonell V, Zaragoza-Martí A. Poor dietary habits in bullied adolescents: the moderating effects of diet on depression. Int J Environ Res Public Health. (2018) 15(8):1569. doi: 10.3390/ijerph15081569
115. Armitage R. Bullying in children: impact on child health. BMJ Paediatr Open. (2021) 5(1):e000939. doi: 10.1136/bmjpo-2020-000939
116. Callaghan M, Kelly C, Molcho M. Bullying and bystander behaviour and health outcomes among adolescents in Ireland. J Epidemiol Community Health (1978). (2019) 73(5):416–21. doi: 10.1136/jech-2018-211350
117. Chester KL, Spencer NH, Whiting L, Brooks FM. Association between experiencing relational bullying and adolescent health-related quality of life. J School Health. (2017) 87(11):865–72. doi: 10.1111/josh.12558
118. Dubey VP, Kievišienė J, Rauckiene-Michealsson A, Norkiene S, Razbadauskas A, Agostinis-Sobrinho C. Bullying and health related quality of life among adolescents—a systematic review. Children. (2022) 9(6):766. doi: 10.3390/children9060766
119. Gobina I, Zaborskis A, Pudule I, Kalnins I, Villerusa A. Bullying and subjective health among adolescents at schools in Latvia and Lithuania. Int J Public Health. (2008) 53(5):272–6. doi: 10.1007/s00038-008-7041-1
120. González-Cabrera J, Machimbarrena JM, Ortega-Barón J, Álvarez-Bardón A. Joint association of bullying and cyberbullying in health-related quality of life in a sample of adolescents. Qual Life Res. (2020) 29(4):941–52. doi: 10.1007/s11136-019-02353-z
121. Hansson E, Garmy P, Vilhjálmsson R, Kristjánsdóttir G. Bullying, health complaints, and self-rated health among school-aged children and adolescents. J Int Med Res. (2020) 48(2):030006051989535. doi: 10.1177/0300060519895355
122. Jahn A, Rysgaard TK, Andersen JH, Winding TN. Adverse childhood experiences and future self-rated health: a prospective cohort study. BMC Public Health. (2021) 21(1):895. doi: 10.1186/s12889-021-10941-3
123. Ottova-Jordan V, Smith ORF, Augustine L, Gobina I, Rathmann K, Torsheim T, et al. Trends in health complaints from 2002 to 2010 in 34 countries and their association with health behaviours and social context factors at individual and macro-level. Eur J Public Health. (2015) 25(suppl 2):83–9. doi: 10.1093/eurpub/ckv033
124. Goldstein BI, Carnethon MR, Matthews KA, McIntyre RS, Miller GE, Raghuveer G, et al. Major depressive disorder and bipolar disorder predispose youth to accelerated atherosclerosis and early cardiovascular disease. Circulation. (2015) 132(10):965–86. doi: 10.1161/CIR.0000000000000229
125. World Health Organization. WHO European regional obesity report 2022. World Health Organization. Regional Office for Europe. (2022).
126. Koç ET, Çalişkan Z, Erdem E. Factors affecting obesity in preadolescents: the case of cappadocia. Cukurova Med J. (2021) 46(3):1175–83. doi: 10.17826/cumj.920570
127. Medrano L, Amatya K, Vizthum D, Fadrowski JJ, Brady TM. Association of mood disorders with cardiovascular disease risk factors in overweight and obese youth with elevated blood pressure. J Clin Hypertens. (2018) 20(9):1268–75. doi: 10.1111/jch.13348
128. Li C, Birmaher B, Rooks B, Gill MK, Hower H, Axelson DA, et al. High prevalence of metabolic syndrome among adolescents and young adults with bipolar disorder. J Clin Psychiatry. (2019) 80(4):18m12422. doi: 10.4088/JCP.18m12422
129. Waloszek JM, Byrne ML, Woods MJ, Nicholas CL, Bei B, Murray G, et al. Early physiological markers of cardiovascular risk in community based adolescents with a depressive disorder. J Affect Disord. (2015) 175:403–10. doi: 10.1016/j.jad.2015.01.008
130. Korczak DJ, Cleverley K, Birken CS, Pignatiello T, Mahmud FH, McCrindle BW. Cardiovascular disease risk factors among children and adolescents with depression. Front Psychiatry. (2021) 12:702737. doi: 10.3389/fpsyt.2021.702737
131. Hoare E, Millar L, Fuller-Tyszkiewicz M, Skouteris H, Nichols M, Malakellis M, et al. Depressive symptomatology, weight status and obesogenic risk among Australian adolescents: a prospective cohort study. BMJ Open. (2016) 6(3):e010072. doi: 10.1136/bmjopen-2015-010072
132. Korczak DJ, Madigan S, Colasanto M, Szatmari P, Chen Y, Maguire J, et al. The longitudinal association between temperament and physical activity in young children. Prev Med (Baltim). (2018) 111:342–7. doi: 10.1016/j.ypmed.2017.11.021
133. Crestani CC. Adolescent vulnerability to cardiovascular consequences of chronic emotional stress: review and perspectives for future research. Neurosci Biobehav Rev. (2017) 74:466–75. doi: 10.1016/j.neubiorev.2016.03.027
134. Hemmingsson E. Early childhood obesity risk factors: socioeconomic adversity, family dysfunction, offspring distress, and junk food self-medication. Curr Obes Rep. (2018) 7(2):204–9. doi: 10.1007/s13679-018-0310-2
135. Hemmingsson E, Johansson K, Reynisdottir S. Effects of childhood abuse on adult obesity: a systematic review and meta-analysis. Obes Rev. (2014) 15(11):882–93. doi: 10.1111/obr.12216
136. Bautista LE, Bajwa PK, Shafer MM, Malecki KMC, McWilliams CA, Palloni A. The relationship between chronic stress, hair cortisol and hypertension. Int J Cardiol Hypertens. (2019) 2:100012. doi: 10.1016/j.ijchy.2019.100012
137. McKelvey LM, Saccente JE, Swindle TM. Adverse childhood experiences in infancy and toddlerhood predict obesity and health outcomes in middle childhood. Childhood Obesity. (2019) 15(3):206–15. doi: 10.1089/chi.2018.0225
138. Elsenburg LK, Smidt N, Liefbroer AC. The longitudinal relation between accumulation of adverse life events and body mass Index from early adolescence to young adulthood. Psychosom Med. (2017) 79(3):365–73. doi: 10.1097/PSY.0000000000000401
139. Doom JR, Lumeng JC, Sturza J, Kaciroti N, Vazquez DM, Miller AL. Longitudinal associations between overweight/obesity and stress biology in low-income children. Int J Obes. (2020) 44(3):646–55. doi: 10.1038/s41366-019-0447-4
140. Olstad DL, Ball K, Wright C, Abbott G, Brown E, Turner AI. Hair cortisol levels, perceived stress and body mass index in women and children living in socioeconomically disadvantaged neighborhoods: the READI study. Stress. (2016) 19(2):158–67. doi: 10.3109/10253890.2016.1160282
141. Nelson DS, Gerras JM, McGlumphy KC, Shaver ER, Gill AK, Kanneganti K, et al. Racial discrimination and low household education predict higher body mass index in African American youth. Childhood Obesity. (2018) 14(2):114–21. doi: 10.1089/chi.2017.0218
142. Suglia SF, Koenen KC, Boynton-Jarrett R, Chan PS, Clark CJ, Danese A, et al. Childhood and adolescent adversity and cardiometabolic outcomes: a scientific statement from the American Heart Association. Circulation. (2018) 137(5):e15–28. doi: 10.1161/CIR.0000000000000536
143. Mazur A, Zachurzok A, Baran J, Dereń K, Łuszczki E, Weres A, et al. Childhood obesity: position statement of Polish society of pediatrics, Polish society for pediatric obesity, Polish society of pediatric endocrinology and diabetes, the college of family physicians in Poland and Polish Association for study on obesity. Nutrients. (2022) 14(18):3806. doi: 10.3390/nu14183806
144. Meena J, Singh M, Agarwal A, Chauhan A, Jaiswal N. Prevalence of hypertension among children and adolescents in India: a systematic review and meta-analysis. Indian J Pediatr. (2021) 88(11):1107–14. doi: 10.1007/s12098-021-03686-9
145. Darbre PD. Endocrine disruptors and obesity. Curr Obes Rep. (2017) 6(1):18–27. doi: 10.1007/s13679-017-0240-4
146. Agyemang C, Redekop WK, Owusu-Dabo E, Bruijnzeels MA. Blood pressure patterns in rural, semi-urban and urban children in the ashanti region of Ghana, West Africa. BMC Public Health. (2005) 5(1):114. doi: 10.1186/1471-2458-5-114
147. Ye X, Yi Q, Shao J, Zhang Y, Zha M, Yang Q, et al. Trends in prevalence of hypertension and hypertension phenotypes among Chinese children and adolescents over two decades (1991–2015). Front Cardiovasc Med. (2021) 8:627741. doi: 10.3389/fcvm.2021.627741
148. Münzel T, Sørensen M. Noise pollution and arterial hypertension. Eur Cardiol Rev. (2017) 12(1):26. doi: 10.15420/ecr.2016:31:2
149. Dzhambov AM, Dimitrova DD. Children’s blood pressure and its association with road traffic noise exposure—a systematic review with meta-analysis. Environ Res. (2017) 152:244–55. doi: 10.1016/j.envres.2016.10.024
150. Dratva J, Phuleria HC, Foraster M, Gaspoz JM, Keidel D, Künzli N, et al. Transportation noise and blood pressure in a population-based sample of adults. Environ Health Perspect. (2012) 120(1):50–5. doi: 10.1289/ehp.1103448
151. Munzel T, Gori T, Babisch W, Basner M. Cardiovascular effects of environmental noise exposure. Eur Heart J. (2014) 35(13):829–36. doi: 10.1093/eurheartj/ehu030
152. Ni Y, Szpiro AA, Young MT, Loftus CT, Bush NR, LeWinn KZ, et al. Associations of Pre- and postnatal air pollution exposures with child blood pressure and modification by maternal nutrition: a prospective study in the CANDLE cohort. Environ Health Perspect. (2021) 129(4):47004. doi: 10.1289/EHP7486
153. Sanidas E, Papadopoulos DP, Grassos H, Velliou M, Tsioufis K, Barbetseas J, et al. Air pollution and arterial hypertension. A new risk factor is in the air. J Am Soc Hypertens. (2017) 11(11):709–15. doi: 10.1016/j.jash.2017.09.008
154. Wójcik M, Starzyk JB, Drożdż M, Drożdż D. Effects of puberty on blood pressure trajectories — underlying processes. Curr Hypertens Rep. (2023) 25(7):117–25. doi: 10.1007/s11906-023-01241-9
155. National High Blood Pressure Education Program Working Group on High Blood Pressure in Children and Adolescents. The fourth report on the diagnosis, evaluation, and treatment of high blood pressure in children and adolescents. Pediatrics. (2004) 114(2 Suppl 4th Report):555–76.15286277
156. Syme C, Abrahamowicz M, Leonard GT, Perron M, Richer L, Veillette S, et al. Sex differences in blood pressure and its relationship to body composition and metabolism in adolescence. Arch Pediatr Adolesc Med. (2009) 163(9):818. doi: 10.1001/archpediatrics.2009.92
157. Barba G, Casullo C, Dello Russo M, Russo P, Nappo A, Lauria F, et al. Gender-Related differences in the relationships between blood pressure, age, and body size in prepubertal children. Am J Hypertens. (2008) 21(9):1007–10. doi: 10.1038/ajh.2008.228
158. Genovesi S, Giussani M, Pieruzzi F, Vigorita F, Arcovio C, Cavuto S, et al. Results of blood pressure screening in a population of school-aged children in the province of Milan: role of overweight. J Hypertens. (2005) 23(3):493–7. doi: 10.1097/01.hjh.0000160203.35910.9f
159. Telencoe S, Singer A, Kosowan L, Dart AB. An analysis of sex differences and socioeconomic deprivation among Canadian children with high blood pressure: a retrospective, cross-sectional study. Pediatr Nephrol. (2023) 38(7):2137–45. doi: 10.1007/s00467-022-05841-6
160. Dasgupta K, O’Loughlin J, Chen S, Karp I, Paradis G, Tremblay J, et al. Emergence of sex differences in prevalence of high systolic blood pressure. Circulation. (2006) 114(24):2663–70. doi: 10.1161/CIRCULATIONAHA.106.624536
161. Shah B, Tombeau Cost K, Fuller A, Birken CS, Anderson LN. Sex and gender differences in childhood obesity: contributing to the research agenda. BMJ Nutr Prev Health. (2020) 3(2):387–90. doi: 10.1136/bmjnph-2020-000074
162. Gavin KM, Bessesen DH. Sex differences in adipose tissue function. Endocrinol Metab Clin North Am. (2020) 49(2):215–28. doi: 10.1016/j.ecl.2020.02.008
163. Hatch-Stein JA, Kelly A, Gidding SS, Zemel BS, Magge SN. Sex differences in the associations of visceral adiposity, homeostatic model assessment of insulin resistance, and body mass index with lipoprotein subclass analysis in obese adolescents. J Clin Lipidol. (2016) 10(4):757–66. doi: 10.1016/j.jacl.2016.02.002
164. von der Born J, Baberowski S, Memaran N, Grams L, Homeyer D, Borchert-Mörlins B, et al. Impact of sex and obesity on echocardiographic parameters in children and adolescents. Pediatr Cardiol. (2022) 43(7):1502–16. doi: 10.1007/s00246-022-02876-2
Keywords: children, obesity, hypertension, psychosocial factors, environmental factors
Citation: Wójcik M, Alvarez-Pitti J, Kozioł-Kozakowska A, Brzeziński M, Gabbianelli R, Herceg-Čavrak V, Wühl E, Lucas I, Radovanović D, Melk A, González Lopez-Valcarcel B, Fernández-Aranda F, Mazur A, Lurbe E, Borghi C and Drożdż D (2023) Psychosocial and environmental risk factors of obesity and hypertension in children and adolescents—a literature overview. Front. Cardiovasc. Med. 10:1268364. doi: 10.3389/fcvm.2023.1268364
Received: 4 October 2023; Accepted: 24 October 2023;
Published: 20 November 2023.
Edited by:
Tlili Barhoumi, King Abdullah International Medical Research Center (KAIMRC), Saudi ArabiaReviewed by:
Mirjam Močnik, University Clinical Centre Maribor, SloveniaFrida Dangardt, University of Gothenburg, Sweden
© 2023 Wójcik, Alvarez-Pitti, Kozioł-Kozakowska, Brzeziński, Gabbianelli, Herceg-Čavrak, Wühl, Lucas, Radovanović, Melk, González Lopez-Valcarcel, Fernández-Aranda, Mazur, Lurbe, Borghi and Drożdż. This is an open-access article distributed under the terms of the Creative Commons Attribution License (CC BY). The use, distribution or reproduction in other forums is permitted, provided the original author(s) and the copyright owner(s) are credited and that the original publication in this journal is cited, in accordance with accepted academic practice. No use, distribution or reproduction is permitted which does not comply with these terms.
*Correspondence: Małgorzata Wójcik bWFsZ29yemF0YS53b2pjaWtAdWouZWR1LnBs; d29qY2lrLmdvc2lhQGdtYWlsLmNvbQ==