- 1Guang’anmen Hospital, China Academy of Chinese Medical Sciences, Beijing, China
- 2Beijing Association of the Integrating of Traditional and Westem Medicine, Beijing, China
- 3Eye Hospital, China Academy of Chinese Medical Sciences, Beijing, China
- 4Wangjing Hospital, China Academy of Chinese Medical Sciences, Beijing, China
- 5Graduate School of Beijing University of Chinese Medicine, Beijing, China
Background: Recently, attention has been paid to the protective properties of active ingredients in Salvia miltiorrhiza (AISM) against organ toxicity induced by chemotherapy drugs. Purpose of the present systematic review is to evaluate the chemoprotective effects and mechanisms of AISM on in vitro and in vivo models of doxorubicin-induced cardiotoxicity (DIC).
Methods: According to the PRISMA guideline, the current systematic review was conducted in the Web of Science, PubMed, Embase, and the Cochrane Library to collect all relevant in vitro and in vivo studies on “the role of AISM on DIC” published up until May 2023. The SYRCLE's tool was used to identify potential risk of bias.
Results: Twenty-two eligible articles were included in this systematic review. Eleven types of active ingredients in Salvia miltiorrhiza were used for DIC, which have the following effects: improvement of physical signs and biochemical indicators, reduction of cardiac function damage caused by DIC, protection of heart tissue structure, enhancement of myocardial cell viability, prevention of cardiomyocyte apoptosis, increase of the chemosensitivity of cancer cells to Doxorubicin, etc. The cardioprotective mechanism of AISM involves inhibiting apoptosis, attenuating oxidative stress, suppressing endoplasmic reticulum (ER) stress, decreasing inflammation, improving mitochondrial structure and function, affecting cellular autophagy and calcium homeostasis. The quality scores of included studies ranged from 4 to 7 points (a total of 10 points), according to SYRCLE's risk of bias tool.
Conclusion: This systematic review demonstrated that AISM have chemoprotective effects on DIC in vivo and in vitro models through several main mechanisms such as anti-apoptosis, antioxidant effects, anti-ER stress, and anti-inflammatory.
1. Introduction
Cancer is the main cause of death and an important obstacle to extending life expectancy in countries around the world (1). Worldwide, an estimated 19.3 million new cancer cases and almost 10.0 million cancer deaths occurred in 2020. The global cancer burden is expected to be 28.4 million cases in 2040, a 47% rise from 2020. Overall, the burden of cancer incidence and mortality is rapidly growing worldwide (2). The conventional therapies for cancer are surgery, radiotherapy, and chemotherapy (3). Although chemotherapy is effectively used for systemic treatment of different cancers, its clinical application is limited by some shortcomings, such as the lack of tumor selectivity, which leads to serious toxic side effects on normal tissues and organs (4–7).
Adriamycin (Doxorubicin, DOX) is an anthracycline antibiotic, produced by Streptomyces peucetius bacteria and was first used as a cytotoxic medication in 1969 (8). Due to its excellent anti-tumor properties, it has always been used as one of the most commonly used and effective anti-tumor drugs alone or in combination with other drugs (9). When DOX is given as a single drug or in combination with other anti-tumor drugs, the most common tumor reactions include breast cancer and esophageal cancer; osteosarcoma, Kaposi's sarcoma, and soft tissue sarcoma; And Hodgkin's lymphoma and non-Hodgkin's lymphoma. Other cancers that have poor response to doxorubicin but can still be treated with the drug due to its overall benefits include gastric cancer, liver cancer, bile duct cancer, pancreatic cancer and endometrial cancer (10). DOX has pleiotropic anticancer activity, including its contribution to DNA damage, reactive oxygen species (ROS) production, apoptosis, senescence, autophagy, ferroptosis, and pyroptosis induction, as well as its immunomodulatory role (11). However, the harmful effects of DOX are not unique to cancer cells, as it affects both healthy and cancer cells, leading to multiple organ damage (12). Dose dependent cardiotoxicity is considered the most relevant side effect of DOX. DOX at doses of 500–550 mg/m2 causes approximately 4% of patients to develop cardiomyopathy, while at doses of 551–600 mg/m2, it is approximately 18%. Over 600 mg/m2 causes 36% of patients to develop cardiomyopathy (13). This cardiac toxicity can be classified as acute (dose dependent) or chronic (cumulative) (14, 15). Acute cardiac toxicity is characterized by chest pain, tachycardia, and other electrocardiogram changes (16). Chronic cardiotoxicity is permanent and irreversible (17). The most serious chronic side effect of the heart is dilated cardiomyopathy, which may develop 10–15 years after treatment and lead to congestive heart failure directly related to the cumulative dose of DOX (18, 19). In addition to the heart, DOX also induced changes in organs such as the liver, kidney, testicle, and brain (20–23). Given the dual nature of DOX, it is recommended to use chemical protectants during doxorubicin treatment, which may reduce adverse reactions and improve patient survival.
In the past few decades, the use of herbs and natural products or their derivatives to reduce adverse reactions caused by chemotherapy or increase the sensitivity of cancer cells to chemotherapy drugs has attracted widespread attention. Salvia miltiorrhiza (Danshen, Simplified Chinese: 丹参) is a common medicinal herb, first recorded in the oldest medical monograph in China, Shennong's Classic of Materia Medica (Shennong Bencao Jing). Its roots have high medicinal value in traditional Chinese medicine, and has been used as a medicated diet in Asia for thousands of years (24). As one of the most commonly used traditional drugs, Danshen has been used to treat various diseases, including cardiovascular disease, cerebrovascular disease, neurodegenerative disease, diabetes, etc (25–30). Until now, more than 200 natural compounds extracted from Salvia miltiorrhiza have been identified, collectively known as active ingredients in Salvia miltiorrhiza (AISM), mainly including lipophilic diterpenoids, such as tanshinone I (Tan I), tanshinone IIA (Tan IIA), tanshinone IIB (Tan IIB), cryptotanshinone (CPT), dihydrotanshinone I (DHT), etc., water-soluble phenolic acids, such as danshensu (DSS), salvianolic acid A and B (Sai A and Sai B), protocatechuic aldehyde, etc., and other constituents, which have exhibited various pharmacological activities, such as anti-inflammation, anti-oxidation, anti-atherogenesis, and anti-diabetes (31). Importantly, these compounds have the ability to kill tumor cells and make tumor cells more sensitive to treatment methods such as chemotherapy and radiation therapy (32). Zhang W et al. reported that Tan IIA significantly inhibited the proliferation of several types of tumors, blocked the cell cycle, induced apoptosis and autophagic death, in addition to inhibiting cell migration and invasion (33). Cai Zhang et al. found that salvianolic acid increased the accumulation of doxorubicin in brain tumors through caveolae endocytosis (34). In addition, their role as chemosensitizers has also been verified in cancer cells such as breast cancer cells and ovarian cancer cells (35, 36). Recently, attention has been paid to the other biological activities of Salvia miltiorrhiza, including its protective properties against organ toxicity induced by chemotherapy drugs. Li K et al. reported that Tan IIA enhanced Dox's chemotherapeutic effect on breast cancer, while reducing its side effects, including weight loss, bone marrow suppression, cardiotoxicity and nephrotoxicity (37). Wenjing Ma et al. found that salvianolic acid C effectively reduced the risk of drug-induced immune thrombocytopenia and venous thromboembolism induced by Dox and the repercussions of amplified platelet-cancer interaction in the tumor microenvironment (38). Other studies also found that Tan IIA and Sai A have antagonism on DOX induced nephrotoxicity (39, 40).
Now, focusing on the heart, the current study aimed to evaluate the chemoprotective effects of active ingredients in Salvia miltiorrhiza on in vitro and in vivo models of doxorubicin-induced cardiotoxicity through a systematic review of the literature, to provide more possible options for developing ideal cardioprotective agents.
2. Methods
The Preferred Reporting Items for PRISMA was used to design the current systematic review (41). At each stage of the study, including study search and selection, data extraction, and risk of bias assessment, two independent researchers participated.
2.1. Searching strategy
Searches were conducted on the Web of Science, PubMed, Embase, and the Cochrane Library. Two authors independently searched all original articles that had been published up until May, 2023. Due to a linguistic constraint for the selection, only articles in the English language were taken into account. The following diseases and treatments were identified using a combination of MeSH and free text terms:
i) Salvia miltiorrhiza or Danshen extract or tanshinone or Cryptotanshinone or Danshensu or Salvianolic acid
and
ii) Doxorubicin
All the articles from these searches were exported to EndNote X8, duplicate records, reviews, and conference abstracts were deleted. First, articles were screened by reading their titles and abstracts; those that were unrelated or lacking full text were then disqualified. The remaining articles were then assessed using the inclusion and exclusion criteria after being read in their entirety.
2.2. Inclusion and exclusion criteria
The inclusion criteria were taken into account: (1) in vitro and in vivo studies; (2) Studies that focused on active ingredients in Salvia miltiorrhiza vs. doxorubicin-induced cardiotoxicity; (3) Original data that is independent and full-text searchable.
The exclusion criteria were taken into account: (1) Studies focused on the active ingredients extracted from non-Salvia miltiorrhiza plants; (2) Studies focused on organ toxicity other than cardiotoxicity; (3) Research on combination with other drugs; (4) Lack of control.
2.3. Data extraction and management
A Microsoft Excel sheet was used by two researchers to separately collect data. When a consensus could not be reached about a discrepancy, the third reviewer was consulted. The following details were taken out of each study: (1) author, year of publication; (2) models (in vivo, or/and in vitro); (3) doxorubicin dosage, usage, and administration route; (4) outcomes of doxorubicin on cardiac cells/tissue; (5) types of Salvia miltiorrhiza extract, dosage, usage, and administration route; (6) outcomes after active ingredients in Salvia miltiorrhiza coadministration; (7) the major findings of each article.
2.4. Methodological quality appraisal for in vivo studies
The methodological quality of in vivo studies was assessed using the SYRCLE's risk of bias (RoB) tool (42). It consists of ten items within six main domains. For the judgment of bias, the response options were “YES” to indicate a low risk of prejudice, “NO” to indicate a high risk of bias, or “NC” (NOT CLEAR) to indicate an undetermined level of bias due to insufficient data. The items judged as “YES” were scored one point, and the scores of 10 items were added together for the quality score of each study.
3. Results
3.1. Study inclusion
437 articles in total, of which 95 appeared in Web of Science, 74 in PubMed, 64 in Embase, and 204 in Scopus (Nothing was found in the Cochrane Library), were extracted from the original retrieval. After then, search filters were used to exclude 278 items (187 duplicates, 85 reviews, and 6 conference abstracts). By reading the titles and abstracts, 42 studies focused on other diseases, 5 other types of studies, and 76 other irrelevant studies were excluded. Thus, 36 articles were read in their full text, 14 of these articles were found to have failed at least one criterion (other compounds, other organ toxicity) and were eliminated after analysis. Finally, 22 articles were included in the systematic review (43–64). The process and results were summarized in Figure 1.
3.2. Study characteristics
In the current systematic review, 22 studies were finally included, they contained in vivo (5 studies), in vitro (6 studies) experiments, and 11 studies done both. Doxorubicin was used to induce cardiac toxicity. The most commonly used concentration for in vitro experiments was 1 µm (11 studies), with a minimum of 0.5 µm and a maximum of 200 µm. The dosages of doxorubicin used for in vivo studies in different animals are as follows: 3–18 mg/kg i.p. or 4–20 mg/kg i.v. for mice; 1.25–3 mg/kg i.p. for rats. Eleven types of active ingredients in Salvia miltiorrhiza were used for doxorubicin-induced cardiotoxicity (DIC), including Tanshinone I (1 studiy; 5, 10 mg/kg, p.o. for in vivo and 10µm for mice), Dihydrotanshinone I (1 studiy; 20 mg/kg p.o. for mice and 10 µm for in vitro), Tanshinone IIA (6 studies; 2.5–30 mg/kg i.p. or 10 mg/kg p.o. for mice and 0.1–40 µm for in vitro), Tanshinone IIA sodium sulphonate (TSNIIA-SS; 2 studies; 30 mg/kg i.p. for mice and 1.6 µm-0.5 mm for in vitro), Salvianolic acids (SA; 1 studiy; 40 mg/kg, i.p. for mice), Salvianolic acid A (2 studies; 50 mg/kg i.p. for mice and 2 µm-1 mm for in vitro), Salvianolic acid B (2 studies; 2 mg/kg i.p. for mice or 0.25–1 mg/kg i.v. for rats and 20 µg/ml for in vitro), Cryptotanshinone (3 studies; 50 mg/kg, p.o. for rats and 2–25 µm for in vitro), Danshensu (1 studiy; 50, 100 mg/kg, i.p. for mice), Diethyl Blechnic (DB; 1 studiy; 5–20 μm for in vitro), Salvia miltiorrhiza aqueous extract (SMAE; 2 studies; 20–100 mg/kg, p.o. for rats and 0.3125–10 mg/ml for in vitro). Some of compounds's chemical structures were shown in Figure 2. The details of the study characteristics and the effects of active ingredients in Salvia miltiorrhiza on doxorubicin-induced cardiotoxicity were shown in Table 1.
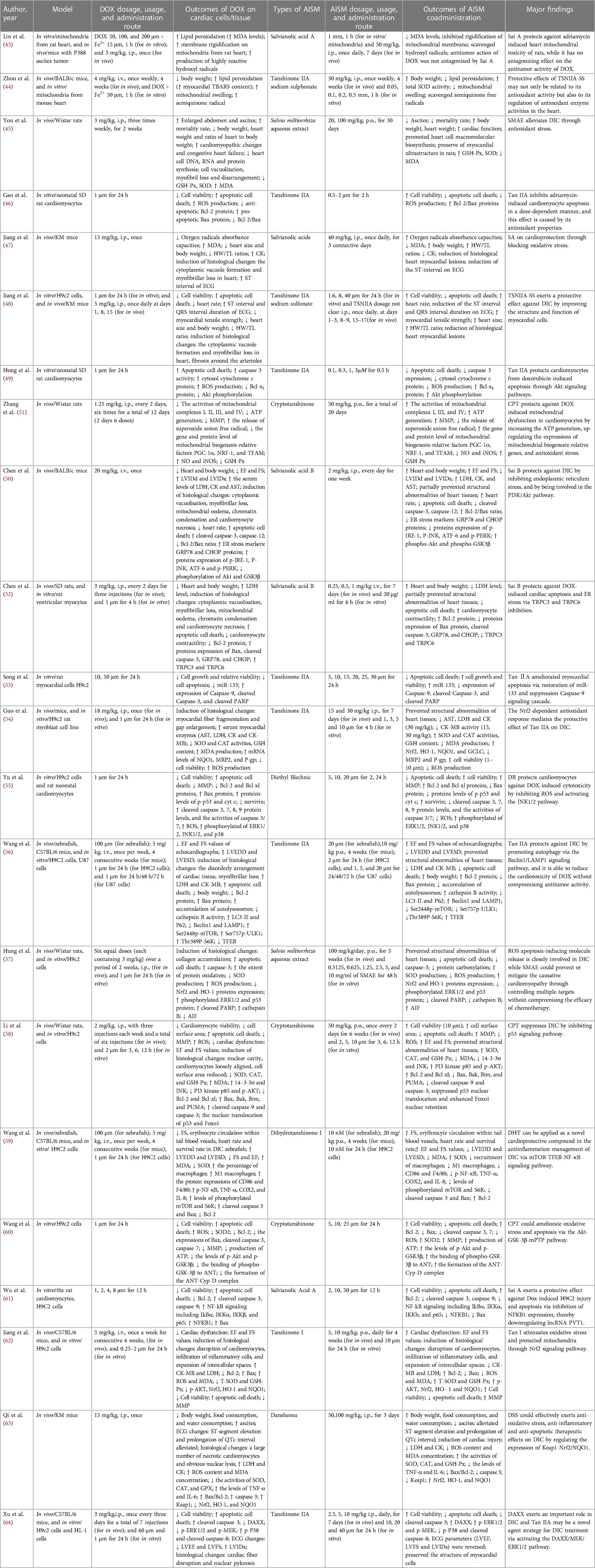
Table 1. Chemoprotective effects of active ingredients in Salvia miltiorrhiza on doxorubicin-induced cardiotoxicity.
In the review, the SYRCLE checklist was used to evaluate the risk of bias for 16 included in vivo studies. The quality scores ranged from 4 to 7 points. Baseline characteristics, performance bias, blinding items of detection bias, attrition, reporting, and other sources all had low risks of bias. However, there were also many high-risk items that attention should be paid to the detailed reporting of random sequence generation, allocation concealment, random outcome assessment and the use of blinding in the future, which will improve the reliability and rigor of the studies. The bias risk of in vivo studies was summarized in Table 2.
3.3. The role of active ingredients in Salvia miltiorrhiza on doxorubicin induced cardiotoxicity
3.3.1. Effect on physical signs change
Seven studies have reported the effect of AISM on doxorubicin induced changes in animal signs, including body weight, heart weight, ascites, heart rates, food and water consumption, mortality rate, etc. The results of this study showed that compared with the control group, the body weight and heart weight of mice/rats in the DOX group decreased (44, 45, 47, 48, 52, 63). We observed a decrease in the ratio of animal heart to body weight and the ratio of heart weight to tibial length after treatment with DOX (45, 47, 48, 50). In addition, compared with untreated rats, rats treated with doxorubicin showed a significant accumulation of ascites (45, 63), and the mortality rate was significantly higher than other rats (45, 63). Compared with the group treated with doxorubicin alone, the combination of AISM and doxorubicin significantly increased the body weight, heart weight, heart-to-body weight ratio, and heart weight to tibia length ratio of mice/rats, as well as food consumption and water consumption. In addition, co-treatment with AISM significantly reduced the increase in ascites value in animals treated with doxorubicin and reduced mortality.
3.3.2. Effect on biochemical markers
Seven studies reported the changes in biochemical markers after DOX. Serum myocardial enzymes are the important indexes that reflect the extent of myocardial injury. Creatine kinase (CK) is an important clinical marker of cardiac injury. Lactate dehydrogenase (LDH) and creatine kinase-muscle/brain (CK-MB) are located in the cytoplasm of cardiomyocytes under normal conditions and the release of LDH and CK-MB into the blood is a diagnostic indicator of heart failure. DOX significantly increased the activity of all enzymes compared with controls, indicating cardiotoxicity. Among these compounds, Tan I reduced serum levels of CK-MB and LDH (63), Tan IIA significantly reduced the levels of serum myocardial enzymes, including aspartate transaminase (AST), LDH, CK and CK-MB (54, 56). Baohong Jiang et al. illustrated that SA significantly decreased the level of CK (47). Sai B has been reported to reduce the levels of LDH, CK and AST in mice (50), as well as the level of LDH in rats (52). In addition, DSS was also found to reduce CK and LDH levels, and exhibited a dose-dependent trend (63).
3.3.3. Effect on cardiac function
Ten studies reported that AISM maintains heart function from DOX cardiotoxicity damage. Jyh Sheng You et al. found that SMAE treatment alleviated doxorubicin induced cardiomyopathy and congestive heart failure, improved cardiac function (45). The electrocardiogram showed that the QTc interval of animals in the DOX group was 2.3 folds higher than that of the normal group, accompanied by significant ST-segment elevation. DSS treatment effectively alleviated the prolongation of QTc interval (63). Echocardiography showed that DOX reduced the ejection fraction (EF) and shortening fraction (FS), increased the left ventricular end-diastolic dimension (LVEDD), left ventricular end-systolic dimension (LVESD), left ventricular internal diameter at diastolic phase (LVIDd), and left ventricle internal diameter in systolic phase (LVIDs) values, indicating severe damage to mouse cardiac function. However, salvianolic acid has been shown to partially reverse these functional changes (47, 50). Similarly, DHT I was proved to increase FS value and promote tail venous blood flow in zebrafish model. In mice, DHT I reduced LVEDD and LVESD values. The significant increase in FS and EF values represented a strengthening in cardiac contractility, indicating that DHT I treatment can improve left ventricular function (59). And, Tan I was also observed to increase EF and FS values in a dose-dependent manner (63). TSNIIA-SS significantly reversed the prolongation of ST and QRS intervals induced by DOX (48). After treatment with Tan IIA, EF, FS, and LVIDs values increased, while LVEDD and LVESD levels significantly decreased (56, 64). Hemodynamic examination showed that DOX induced a left shift of the pressure-volume (PV) loop. The EF and FS of rats treated with simultaneous oral administration of CPT increased, and the PV loop shifted to the right along the horizontal axis, indicating that CPT treatment alleviated dox-induced cardiac dysfunction in rats (58).
3.3.4. Effect on cardiac histology
The morphological and histological changes in the heart have been described in detail in 7 studies. Jyh-Sheng You et al. observed that under the microscope, the myocardial structure of rats in the DOX treatment group showed cell vacuolization, myofibril loss and disorder, while the myocardial ultrastructure of rats in the SMAE + DOX group was basically well preserved (45). Baohong Jiang et al. further evaluated DOX induced cardiac toxicity using H&E staining (47). The hearts of the control group showed normal cell distribution and normal myocardial morphology, while the hearts of DOX treated animals showed cytoplasmic vacuoles and myofibril loss, which were typical manifestations of cardiomyopathy induced by DOX. The myocardial lesions in animals treated with salvianolic acid were significantly reduced. Using the transmission electron microscope, Rongchang Chen et al. observed the obvious abnormalities such as cytoplasmic vacuolization, myofibril loss, mitochondrial edema, chromatin condensation and myocardial necrosis in the heart tissue of DOX treated mice (50). The pretreatment with Sai B partially prevented DOX induced cardiac tissue structural abnormalities (52). DIC model group showed pathological changes such as myocardial cell structure disorder, myofibril loss, karyopyknosis and plasma dissolving myocardial cells, while Tan IIA pretreatment also partially alleviated these injuries (54, 56, 64). Another study found that the heart size of DOX treated mice was smaller, the ventricular cavity was dilated smaller, the formation of cytoplasmic vesicles and the loss of myofibril. The pathological changes in the hearts and myocardial cells of animals treated with TSNIIA-SS were significantly delayed (48). Le Li et al. reported that treatment with CPT reversed the decrease in surface area of myocardial cells induced by DOX and the increase in collagen deposition in the heart (58). Xiaoping Wang et al. found that DOX caused damage to myocardial cells, infiltration of inflammatory cells, and expansion of intercellular spaces (59). Tan I protected their structure and alleviated inflammatory cell infiltration and cell damage caused by DOX (63). In addition, DOX caused the destruction of cardiac myocytes and the disturbance of myofibril. However, the structure of most cells remained normal after DSS treatment, with clear horizontal lines, and only a few necrotic cardiac myocytes existed (63).
3.3.5. Effect on myocardial cells
3.3.5.1 Cell viability and survival
Sixteen studies provided the effect of AISM on cardiac cell viability and survival after treatment with DOX. Qianqian Jiang et al. reported that DOX dose-dependent reduction in cell viability, while Tan I pretreatment increased cell viability in a dose-dependent manner (62). At a concentration of 10 µm, Tan I had the most significant protective effect on DIC. Under the treatment of 10 µm and 25 µm concentrations of DHT I, the cell apoptosis rate was reduced by 22.4% and 19.4%, respectively (59).
Tan IIA has a significant protective effect on DOX induced cardiomyocyte apoptosis and cell viability (46, 48, 49, 53, 54, 56, 64). Its individual treatment does not affect normal cell survival. On the contrary, the use of Tan IIA on DOX treated cells can prevent cell death. Jie Gao et al. demonstrated that Tan IIA (0.5, 1, 2 mol/L) inhibited DOX (1 mol/L) induced cell death in a dose-dependent manner (46). In MTT detection, the survival rates of myocardial cells increased to 85.6%, 89.1%, and 95.7%, respectively. Hoechst staining showed that Tan Ⅱ A significantly reduced the number of apoptotic cells in typical nuclear fragmentation induced by DOX, which were 18.0%, 13.8% and 6.4%. When flow cytometry was used to quantify apoptosis, the percentage of cardiomyocyte apoptosis decreased, to 15.6%, 13.3% and 10.0%, respectively.
Sai A and B exhibited significant protective effects on DOX induced cardiomyocyte apoptosis. 10 and 50 μm Sai A restored the vitality of H9C2 cells, and the apoptosis rate of H9C2 cells treated with DOX and Sai A was lower (61). Sai B pretreatment also reduced the proportion of TUNEL positive cells (50, 52). TUNEL detection and Hoechst staining both showed that CPT significantly reduced the apoptosis rate of cardiac myocytes induced by dox in rats, and CPT alone did not cause collagen deposition and apoptosis in the rat heart (58, 60). The same effect was observed in the SAME (57). In addition, Diethyll Blechnic pretreatment prevented dox-induced cell death in a concentration dependent manner in primary cultured mouse cardiomyocytes (55).
3.3.5.2. Mitochondrial structure and function
Six studies focused on the effect of AISM on mitochondrial function after DIC. Sai A (43) and TSNIIA-SS (44) showed protective effects on cardiac mitochondrial damage induced by DOX. DOX induced rigidification of mitochondrial membrane, mitochondria were swollen, the addition of Sai A and TSNIIA-SS could significantly inhibit these changes. This indicated that these compounds have a protective effect on the integrity and function of mitochondrial membranes. CPT (51, 60) exerted myocardial protective effects by restoring mitochondrial dysfunction caused by DOX. DOX led to a decrease in the activity of mitochondrial complexes and inhibition of ATP generation, while CPT enhanced the activity of complexes and promoted ATP generation. CPT also increased the mitochondrial membrane potential (MMP), promoted the structural repair and functional recovery of mitochondrial membrane. Meanwhile, CPT partially protected and promoted mitochondrial biogenesis by regulating the expression of factors related to mitochondrial biogenesis. Two other compounds, DB (55) and Tan I (63), also showed protective effects on mitochondrial abnormalities induced by DOX. Pretreatment of DB attenuated the decrease of mitochondrial membrane potential induced by doxorubicin, which confirmed its protective effect. Tan I inhibited DOX induced cardiotoxicity and alleviated DOX induced damage to mouse heart mitochondria by regulating nuclear factor (erythroid-derived 2)-like 2 (Nrf2) signaling pathway.
3.3.6. Other benefits
The combination of AISM and DOX has demonstrated potential for anti-tumor and cardiac protection in different experimental models, and may play a role by regulating multiple signaling pathways and protein expression. Tong Jun Lin et al. demonstrated that Sai A alone did not exhibit anti-tumor activity (43). However, when combined with DOX, Sai A will not antagonize its anti-tumor effect, and even SMAE can enhance DOX's inhibitory effect on breast cancer cells (57). Tan IIA has also been found to reduce the toxicity of DOX to the heart without affecting its anti-tumor effect. It increased the chemosensitivity of cancer cells to DOX by inhibiting the expression of multiple drug resistance protein 1 and multiple drug resistance related protein 1 (64). Tan IIA was observed to restore autophagic flux and improve the cell viability of DOX-stimulated H9C2 cells via increasing autophagosome formation and autolysosome degradation, the efficacy of improving autophagic flux was shown to be mediated by the Beclin1/lysosomal-associated membrane proteins-1 (LAMP1) pathway (56). In addition, Sal B alleviated DOX induced cardiomyocyte dysfunction and intracellular calcium disorder, and reduced intracellular calcium overload and endoplasmic reticulum stress by downregulating the levels of transient receptor potential canonical (TRPC) 3 and TRPC6 (52). A significant decrease in tensile strength was also observed in DOX mice, while TSNIIA-SS treatment partially reversed the decrease in tensile strength (48).
3.4. Possible mechanisms
3.4.1. Cells apoptosis and endoplasmic reticulum stress
Fourteen studies reported that the cardioprotective effect of AISM is related to apoptosis related pathways. After DOX treatment, the B-cell lymphoma 2/Bcl-2-associated × protein (Bcl-2/Bax) ratio in myocardial cells decreased, which promoted the process of cell apoptosis. However, pretreatment with Tan IIA reversed the DOX induced effect by inhibiting Bax expression, upregulating Bcl-2 levels, and restoring the Bcl-2/Bax ratio to normal (46, 56) Tan IIA also promoted the expression of B-cell lymphoma-extra-large (Bcl-xl), inhibited the increase of caspase-3 activity induced by DOX, reduced the release of cytochrome c, and downregulated the expression of cleaved Poly (ADP-ribose) polymerase (PARP). At the same time, protein kinase B (Akt) signaling pathway was also involved in the effect of Tan IIA on DOX induced cardiomyocyte apoptosis (49). The study also found that Tan IIA upregulated the expression of miR-133 and reduced cell apoptosis by inhibiting the expression of caspase-9 and related downstream signaling molecules (53). In addition, Tan IIA intervention increased the expression of phosphorylated extracellular signal-regulated protein kinase 1/2(p-ERK1/2), phosphorylated mitogen-activated protein kinase (p-MEK), and death domain-associated protein (DAXX) in myocardial cells, and decreased the expression of cleaved caspase-3, cleaved caspase-8, and p-P38, indicating that Tan IIA alleviated dox-induced cardiac cell apoptosis by activating the DAXX/MEK/ERK1/2 pathway (64).
Sal A treatment protected Dox induced cardiomyocyte apoptosis by inhibiting the activation of the nuclear factor-κB (NF-κB) signaling pathway and the expression of nuclear factor kappa B subunit 1 (NFKB1) (61). Sal B was also observed to have a similar effect. In the DOX group, the levels of caspase-3 and caspase-12 significantly increased, and the Bcl-2/Bax ratio decreased, but these changes were reversed by Sal B pretreatment. Glucose-regulated protein 78 (GRP78) and CCAAT-enhancer-binding protein homologous protein (CHOP) are markers of endoplasmic reticulum stress, and Sal B significantly reduces the expression of GRP78 and CHOP (50, 52). While, DOX treatment increased the expression level of endoplasmic reticulum related apoptosis proteins, including phosphorylated inositol requiring enzyme 1 (p-IRE-1), phosphorylated c-Jun N-terminal kinase (p-JNK), activating transcription factor-6 (ATF-6) and phosphorylated PKR-like ER kinase (p-PERK), while Sal B pretreatment inhibited these protein levels. Phosphatidylinositol 3-kinase (PI3K)/Akt is a survival regulation pathway, which saves cardiac systolic dysfunction by inhibiting endoplasmic reticulum stress. Sal B partially attenuated DOX induced endoplasmic reticulum stress by activating the PI3K/Akt signaling pathway, thus playing an anti-apoptotic role.
In another study, CPT treatment upregulated PI3 kinase p85 and p-AKT, inhibiting the expression of 14-3-3σ and p-JNK (58). CPT also regulated the levels of Bcl-2, Bax, Cleared caspase 3, and caspase 7, thereby alleviating DOX induced cardiomyocyte apoptosis (60). Moreover, the expression of cleared caspase 3 was increased in the left ventricle of mice treated with DOX. DHT I and DSS treatments both inhibited the expression of cleared caspase 3 and regulated the levels of Bcl-2 and Bax. Further research has shown that the anti-apoptotic effect of DHT I was partially mediated through the mammalian target of rapamycin (mTOR) pathway (59, 63). SMAE also blocked DOX induced cell apoptosis response by regulating ERK1/2 and p53 signaling pathways (57).
In addition, DB pretreatment increased the expression levels of Bcl-2, Bcl-xl, and survivin, and decreased the expression levels of Bax, p-p53, cytochrome c, and lysozyme 3, 7, 8, and 9. The protective effect mediated by it increases with the expression of c-Jun N-terminal kinase 1/2 (JNK1/2). Therefore, DB protects DOX induced cardiomyocyte apoptosis by activating the JNK1/2 pathway (55).
3.4.2. Oxidant stress
Fifteen studies suggested that AISM exerted cardiac protective effect in different DIC models by decreasing oxidative stress. Tan IIA has significant antioxidant activity, which reduced DOX induced peroxide production in myocardial cells, inhibited the release of superoxide anion free radicals, and alleviated oxidative stress damage to mitochondria (44, 46, 49, 54). In the DIC model, Tan IIA pretreatment inhibited the production of ROS in a dose-dependent manner, reduced the production of malondialdehyde (MDA), and increased the activities of superoxide dismutase (SOD), catalase (CAT), and glutathione (GSH). Tan IIA pretreatment also induced the nuclear accumulation of Nrf2 and its downstream genes heme oxygenase-1 (HO-1), NAD(P)H dehydrogenase (quinone) 1 (NQO1), and glutamate-cysteine ligase catalytic subunit (GCLC) in both the mice cardiac tissues and H9c2 cells. This indicates that Nrf2-dependent antioxidant response mediates the protective effect of Tan IIA on DIC. Similarly, DSS can reduce the production of ROS, increase the levels of antioxidant enzymes such as SOD, CAT, and glutathione peroxidase (GSH-Px), and exert its effect by inhibiting the activation of the Keap1-Nrf2/NQO1 signaling pathway (63). Tan I has also been shown to enhance the expression of antioxidant enzymes such as HO-1 and NQO1 by activating the Nrf2 signaling pathway (63).
Tong Jun Lin et al. found that in the presence of ferrous ions, DOX stimulated the lipid peroxidation of mitochondria (43). The addition of Sai A could inhibit the formation of MDA in heart mitochondria induced by DOX, and in a dose-dependent manner eliminate the hydroxyl radical produced by DOX. Similar to DB (55), salvianolic acid exerts its antioxidant effect by inhibiting the accumulation of ROS (47). In addition, SMAE (45, 57), CPT (51, 58, 60), and DHT I (59) also inhibited DOX induced increase in myocardial MDA levels. The first two effectively eliminated ROS production and increased the content of antioxidant enzymes such as CAT, SOD, and GSH-Px, thereby protecting the heart from ROS damage.
3.4.3. Inflammation
Two literature studies investigated the mechanism of action of DHT I and DSS in the treatment of cardiac inflammation. Xiaoping Wang et al. (59) found that DHT I exerts anti-inflammatory effects through multiple pathways. Firstly, DHT I treatment inhibited the recruitment of macrophages and the activation of M1 type macrophages, thereby reducing inflammatory responses. At the same time, it inhibited the activation of the NF-κB signaling pathway and reduced the activation of NF-κB and the expression of downstream inflammatory genes. Further experiments have confirmed that DHT I exerts its anti-inflammatory effect by regulating the mTOR TFEB-NF-κB signaling pathway, inhibiting the phosphorylation levels of mTOR and S6K, and promoting nuclear recruitment of transcription factor EB (TFEB). Jia-Ying Qi et al. found that DSS reduced the levels of TNF-α and IL-6 in heart tissue, reducing the cardiac inflammatory response caused by DOX. Based on these results, DHT I and DSS have shown potential anti-inflammatory effects in the treatment of cardiac inflammation, providing an important research basis for the development of new treatment pathways (63).
4. Discussion
4.1. Summary of evidence
Adriamycin is a widely used antineoplastic agent. However, the clinical use of adriamycin is limited by its unique cardiotoxicity. The ideal solution is to use natural chemical protectants during DOX treatment to reduce adverse reactions and improve patients' survival rates. Our study summarizes the protective effect of active ingredients in Salvia miltiorrhiza, including Tan I, DHT I, Tan IIA, TSNIIA-SS, SA, Sai B, CPT, DSS, DB, and SAME, which were described in the literature as cardioprotective agents. The functions include: improvement of physical signs and biochemical indicators, protection of cardiac function damage caused by DOX, alleviation of the development of cardiac toxicity, reduction of myocardial lesions and protection of heart tissue structure, enhancement of myocardial cell viability, prevention of cardiomyocyte apoptosis, increase of the chemosensitivity of cancer cells to DOX, etc. They also exerted myocardial protective effects by protecting the integrity and function of mitochondrial membranes, promoting mitochondrial biogenesis. The cardioprotective effect of AISM involves a variety of mechanisms that are related to inhibiting apoptosis, decreasing inflammation, attenuating oxidative stress, suppressing endoplasmic reticulum stress, affecting cellular autophagy and calcium homeostasis (Figure 3). These results provide sufficient evidence for further clinical studies.
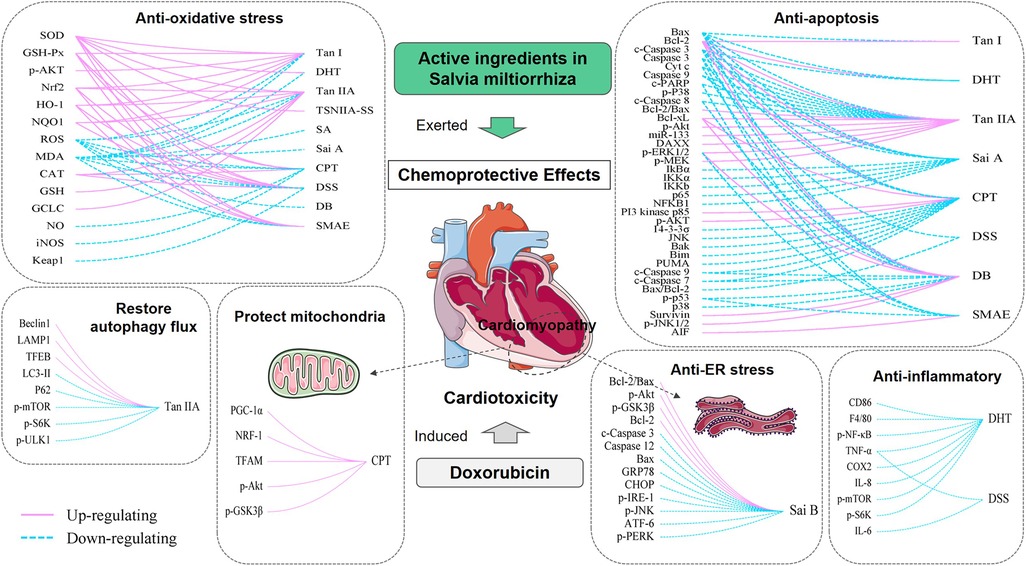
Figure 3. Mechanisms involved in AISM chemoprotective effects in DIC. Each box represents a function of AISM. On the right side of the curve are different types of AISM, and on the left side are the factors that are regulated by them. The solid pink line represents upregulation, and the dashed blue line represents downregulation. By regulating these factors, AISM can exert antioxidant stress, antiapoptotic, anti-endoplasmic reticulum stress, anti-inflammatory role, affect cell autophagy, and improve mitochondrial function, thereby exerting a cardioprotective effect on DIC. Tan I, Tanshinone I; DHT, Dihydrotanshinone I; Tan IIA, Tanshinone IIA; TSNIIA-SS, Tanshinone IIA sodium sulphonate; SA, Salvianolic acids; Sai A, Salvianolic acid A; Sai B, Salvianolic acid B; CPT, Cryptotanshinone; DSS, Danshensu; DB, Diethyl Blechnic; SMAE, Salvia miltiorrhiza aqueous extract.
4.2. Implications for future directions and clinical practice
We reviewed the literature on the cardioprotective effects of AISM on DOX induced cardiotoxicity, providing clinicians with options to address cardiotoxicity through different avenues. Some extracts were more concerned by researchers than other phytochemistry substances, such as tanshinones, salvianolic acids, etc. Many of these compounds have multiple mechanisms of action, including antioxidant stress, anti-apoptotic activity, as well as anti-inflammatory effects. However, considering the dosage range and treatment time of AISM and DOX, as well as the frequency of administration and the starting time, the diversity of research designs makes it difficult to compare different studies and draw reliable conclusions. These limitations also hinder the comparison of AISM with each other to select a compound with higher potential. In addition, most studies mainly focused on DOX induced cardiotoxicity, with only a few studies targeting organs such as the liver, kidneys, brain, or testicles that are also affected by DOX. Therefore, future research should aim to clarify and demonstrate the administration plans of different compounds, as well as clarify the toxicity of DOX and the effects of Salvia miltiorrhiza compounds on these organs (65).
At present, the evidence on the cardioprotective effect of Salvia miltiorrhiza compounds is mainly limited to preclinical trials. Unfortunately, our literature search did not find any clinical trials in this regard. The reasons for the lack of clinical trials are multiple, like other phytochemistry substances, which may include several aspects: lack of evidence on the bioavailability of these compounds in humans; Most of these studies were conducted on cells or animal models lacking cancer cells; It is unclear whether these compounds affect the anti-tumor activity of doxorubicin. A common concern is whether the use of antioxidants will interfere with the effects of chemotherapy drugs by preventing ROS damage to cancer cells (66). An ideal cardioprotective agent should not only prevent cardiotoxicity, but also should not interfere with the required action of DOX. Therefore, it is recommended that future research focus on developing synthetic derivatives of these compounds to enhance their bioavailability in human tissues; Future research should be recommended to use cells or animal models containing cancer to test the possible interactions between these compounds and DOX anti-tumor activity; Design high-quality clinical trials to validate the findings observed in preclinical models (67).
Although Salvia miltiorrhiza compounds have significant protective effects, their adverse pharmacokinetic/pharmacodynamic characteristics limit their application. The clinical application of tanshinone IIA in anti-cancer treatment is hindered by its low water solubility, low cell uptake, short half-life, and first-pass metabolism (68–70). Therefore, in the future, attention needs to be focused on optimizing delivery strategies for these chemical protectants to overcome these limitations. Currently, some research results have been reported. Guanxing Sun et al. constructed a drug delivery system for the co-delivery of DOX and TAN. Lipid nanoparticles loaded with DOX and TAN (N-DOX/TAN) were prepared by emulsification and solvent-diffusion methods. Prostate-specific membrane antigen (PSMA) targeted nanoparticles loaded with DOX and TAN were synthesized by conjugating a PSMA targeted ligand to N-DOX/TAN. Through in vitro and in vivo experiments, it has been found that the novel nanomedicine offers great promise for the dual drug delivery to prostate cancer cells, showing the potential of synergistic combination therapy for prostate cancer (71). These new delivery systems have been reported to improve the bioavailability of AISM, promote their protective effects on the heart and kidneys, and more importantly, maintain the anticancer and anti-tumor efficacy of DOX (72–75). It should be noted that the data represented in the current system review is based on in vitro and in vivo models. Therefore, in the future, it is recommended to use Salvia miltiorrhiza compounds as chemical protectants in combination with doxorubicin for cancer patients, which requires further research, as sometimes the results of in vitro and in vivo models and clinical studies may differ.
4.3. Limitations
Some limitations should be addressed. Firstly, studies evaluated in this systematic review were inconsistent in some important aspects, including the types of AISM, durations, dosages, and routes of administration of AISM and DOX, which reinforced the heterogeneity of the studies. Thus, meta-analyses were not performed for the accessed data. Secondly, according to the SYRCLE's tool, many of the studies suffer from risk of bias. Most studies only mentioned “randomization”, but did not introduce specific approaches. All of the studies received an “NC” rating for the allocation of concealment items and the random outcome assessment. Thirdly, the review was limited to studies published in English only, so there are language and regional differences, literature published in other languages may be missed.
5. Conclusion
The findings showed that doxorubicin chemotherapeutic agent can induce the changes in biochemistry and histology of the cardiac cells/tissue. However, using active ingredients in Salvia miltiorrhiza alleviate the doxorubicin-induced adverse effects, does not affect or even enhance the anticancer effect of DOX. Mechanically, active ingredients in Salvia miltiorrhiza exert their chemoprotective effects through several main mechanisms of antiapoptosis, antioxidant, anti-ER stress, and anti-inflammatory.
Data availability statement
The original contributions presented in the study are included in the article/Supplementary Material, further inquiries can be directed to the corresponding author.
Author contributions
QW: Conceptualization, Writing – original draft. JL: Investigation, Software, Writing – original draft. XC: Data curation, Writing – original draft. XJ: Methodology, Writing – review & editing. CZ: Methodology, Writing – review & editing. FL: Formal Analysis, Writing – review & editing. XZ: Supervision, Writing – review & editing. YL: Formal Analysis, Writing – review & editing. QS: Project administration, Writing – review & editing. BP: Funding acquisition, Resources, Visualization, Writing – review & editing.
Funding
The author(s) declare financial support was received for the research, authorship, and/or publication of this article.
This work was supported by the Capital's Funds for Health Improvement and Research (No. CFH 2022-2-4415); and the Scientific and Technological Innovation Project of China Academy of Chinese Medical Sciences (No. CI2021A01805).
Conflict of interest
The authors declare that the research was conducted in the absence of any commercial or financial relationships that could be construed as a potential conflict of interest.
The reviewer FY declared a shared parent affiliation with the authors QW, JL, XC, XJ, CZ, FL, YL, QS, BP to the handling editor at time of review.
Publisher's note
All claims expressed in this article are solely those of the authors and do not necessarily represent those of their affiliated organizations, or those of the publisher, the editors and the reviewers. Any product that may be evaluated in this article, or claim that may be made by its manufacturer, is not guaranteed or endorsed by the publisher.
Supplementary material
The Supplementary Material for this article can be found online at: https://www.frontiersin.org/articles/10.3389/fcvm.2023.1267525/full#supplementary-material
References
1. Bray F, Laversanne M, Weiderpass E, Soerjomataram I. The ever-increasing importance of cancer as a leading cause of premature death worldwide. Cancer. (2021) 127(16):3029–30. doi: 10.1002/cncr.33587
2. Sung H, Ferlay J, Siegel RL, Laversanne M, Soerjomataram I, Jemal A, et al. Global cancer statistics 2020: GLOBOCAN estimates of incidence and mortality worldwide for 36 cancers in 185 countries. CA Cancer J Clin. (2021) 71(3):209–49. doi: 10.3322/caac.21660
3. Hu LF, Lan HR, Li XM, Jin KT. A systematic review of the potential chemoprotective effects of resveratrol on doxorubicin-induced cardiotoxicity: focus on the antioxidant, antiapoptotic, and anti-inflammatory activities. Oxid Med Cell Longev. (2021) 2021:2951697. doi: 10.1155/2021/2951697
4. Vizel M, Oster MW. Ocular side effects of cancer chemotherapy. Cancer. (1982) 49(10):1999–2002. doi: 10.1002/1097-0142(19820515)49:10%3C1999::aid-cncr2820491009%3E3.0.co;2-b
5. Verstappen CC, Heimans JJ, Hoekman K, Postma TJ. Neurotoxic complications of chemotherapy in patients with cancer: clinical signs and optimal management. Drugs. (2003) 63(15):1549–63. doi: 10.2165/00003495-200363150-00003
6. He Q, Shi J. MSN anti-cancer nanomedicines: chemotherapy enhancement, overcoming of drug resistance, and metastasis inhibition. Adv Mater. (2014) 26(3):391–411. doi: 10.1002/adma.201303123
7. Ren X, Boriero D, Chaiswing L, Bondada S, St Clair DK, Butterfield DA. Plausible biochemical mechanisms of chemotherapy-induced cognitive impairment (“chemobrain”), a condition that significantly impairs the quality of life of many cancer survivors. Biochim Biophys Acta Mol Basis Dis. (2019) 1865(6):1088–97. doi: 10.1016/j.bbadis.2019.02.007
8. Arcamone F, Cassinelli G, Fantini G, Grein A, Orezzi P, Pol C, et al. Adriamycin, 14-hydroxydaunomycin, a new antitumor antibiotic from S. peucetius var. caesius. Biotechnol Bioeng. (1969) 11(6):1101–10. doi: 10.1002/bit.260110607
9. Carvalho C, Santos RX, Cardoso S, Correia S, Oliveira PJ, Santos MS, et al. Doxorubicin: the good, the bad and the ugly effect. Curr Med Chem. (2009) 16(25):3267–85. doi: 10.2174/092986709788803312
10. Singal PK, Iliskovic N. Doxorubicin-induced cardiomyopathy. N Engl J Med. (1998) 339(13):900–5. doi: 10.1056/nejm199809243391307
11. Kciuk M, Gielecińska A, Mujwar S, Kołat D, Kałuzińska-Kołat Ż, Celik I, et al. Doxorubicin-An agent with multiple mechanisms of anticancer activity. Cells. (2023) 12(4):659. doi: 10.3390/cells12040659
12. Varela-López A, Battino M, Navarro-Hortal MD, Giampieri F, Forbes-Hernández TY, Romero-Márquez JM, et al. An update on the mechanisms related to cell death and toxicity of doxorubicin and the protective role of nutrients. Food Chem Toxicol. (2019) 134:110834. doi: 10.1016/j.fct.2019.110834
13. Lefrak EA, Pitha J, Rosenheim S, Gottlieb JA. A clinicopathologic analysis of adriamycin cardiotoxicity. Cancer. (1973) 32(2):302–14. doi: 10.1002/1097-0142(197308)32:2%3C302::aid-cncr2820320205%3E3.0.co;2-2
14. Granados-Principal S, Quiles JL, Ramirez-Tortosa CL, Sanchez-Rovira P, Ramirez-Tortosa MC. New advances in molecular mechanisms and the prevention of adriamycin toxicity by antioxidant nutrients. Food Chem Toxicol. (2010) 48(6):1425–38. doi: 10.1016/j.fct.2010.04.007
15. Shabalala S, Muller CJF, Louw J, Johnson R. Polyphenols, autophagy and doxorubicin-induced cardiotoxicity. Life Sci. (2017) 180:160–70. doi: 10.1016/j.lfs.2017.05.003
16. Wenningmann N, Knapp M, Ande A, Vaidya TR, Ait-Oudhia S. Insights into doxorubicin-induced cardiotoxicity: molecular mechanisms, preventive strategies, and early monitoring. Mol Pharmacol. (2019) 96(2):219–32. doi: 10.1124/mol.119.115725
17. Bernstein D, Fajardo G, Zhao M, Urashima T, Powers J, Berry G, et al. Differential cardioprotective/cardiotoxic effects mediated by beta-adrenergic receptor subtypes. Am J Physiol Heart Circ Physiol. (2005) 289(6):H2441–2449. doi: 10.1152/ajpheart.00005.2005
18. Thorn CF, Oshiro C, Marsh S, Hernandez-Boussard T, McLeod H, Klein TE, et al. Doxorubicin pathways: pharmacodynamics and adverse effects. Pharmacogenet Genomics. (2011) 21(7):440–6. doi: 10.1097/FPC.0b013e32833ffb56
19. Kumar S, Marfatia R, Tannenbaum S, Yang C, Avelar E. Doxorubicin-induced cardiomyopathy 17 years after chemotherapy. Tex Heart Inst J. (2012) 39(3):424–7.22719160
20. Yeh YC, Lai HC, Ting CT, Lee WL, Wang LC, Wang KY, et al. Protection by doxycycline against doxorubicin-induced oxidative stress and apoptosis in mouse testes. Biochem Pharmacol. (2007) 74(7):969–80. doi: 10.1016/j.bcp.2007.06.031
21. Kuzu M, Kandemir FM, Yildirim S, Kucukler S, Caglayan C, Turk E. Morin attenuates doxorubicin-induced heart and brain damage by reducing oxidative stress, inflammation and apoptosis. Biomed Pharmacother. (2018) 106:443–53. doi: 10.1016/j.biopha.2018.06.161
22. Prasanna PL, Renu K, Valsala Gopalakrishnan A. New molecular and biochemical insights of doxorubicin-induced hepatotoxicity. Life Sci. (2020) 250:117599. doi: 10.1016/j.lfs.2020.117599
23. Hussain MA, Abogresha NM, AbdelKader G, Hassan R, Abdelaziz EZ, Greish SM. Antioxidant and anti-inflammatory effects of crocin ameliorate doxorubicin-induced nephrotoxicity in rats. Oxid Med Cell Longev. (2021) 2021:8841726. doi: 10.1155/2021/8841726
24. Li ZM, Xu SW, Liu PQ. Salvia miltiorrhiza burge (danshen): a golden herbal medicine in cardiovascular therapeutics. Acta Pharmacol Sin. (2018) 39(5):802–24. doi: 10.1038/aps.2017.193
25. Wu B, Liu M, Zhang S. Dan shen agents for acute ischaemic stroke. Cochrane Database Syst Rev. (2007) (2):Cd004295. doi: 10.1002/14651858.CD004295.pub3
26. Zhang W, Zheng L, Zhang Z, Hai CX. Protective effect of a water-soluble polysaccharide from Salvia miltiorrhiza bunge on insulin resistance in rats. Carbohydr Polym. (2012) 89(3):890–8. doi: 10.1016/j.carbpol.2012.04.027
27. Chang CC, Chang YC, Hu WL, Hung YC. Oxidative stress and Salvia miltiorrhiza in aging-associated cardiovascular diseases. Oxid Med Cell Longev. (2016) 2016:4797102. doi: 10.1155/2016/4797102
28. Subedi L, Gaire BP. Tanshinone IIA: a phytochemical as a promising drug candidate for neurodegenerative diseases. Pharmacol Res. (2021) 169:105661. doi: 10.1016/j.phrs.2021.105661
29. Yang Y, Song J, Liu N, Wei G, Liu S, Zhang S, et al. Salvianolic acid A relieves cognitive disorder after chronic cerebral ischemia: involvement of Drd2/cryab/NF-κB pathway. Pharmacol Res. (2022) 175:105989. doi: 10.1016/j.phrs.2021.105989
30. Yang Y, Shao M, Cheng W, Yao J, Ma L, Wang Y, et al. A pharmacological review of tanshinones, naturally occurring monomers from Salvia miltiorrhiza for the treatment of cardiovascular diseases. Oxid Med Cell Longev. (2023) 2023:3801908. doi: 10.1155/2023/3801908
31. M.E. XD, Cao YF, Che YY, Li J, Shang ZP, Zhao WJ, et al. Danshen: a phytochemical and pharmacological overview. Chin J Nat Med. (2019) 17(1):59–80. doi: 10.1016/s1875-5364(19)30010-x
32. Xu Z, Chen L, Xiao Z, Zhu Y, Jiang H, Jin Y, et al. Potentiation of the anticancer effect of doxorubicinin drug-resistant gastric cancer cells by tanshinone IIA. Phytomedicine. (2018) 51:58–67. doi: 10.1016/j.phymed.2018.05.012
33. Zhang W, Liu C, Li J, Lu Y, Li H, Zhuang J, et al. Tanshinone IIA: new perspective on the anti-tumor mechanism of A traditional natural medicine. Am J Chin Med. (2022) 50(1):209–39. doi: 10.1142/s0192415x22500070
34. Zhang C, Pan Y, Cai R, Guo S, Zhang X, Xue Y, et al. Salvianolic acid A increases the accumulation of doxorubicin in brain tumors through caveolae endocytosis. Neuropharmacology. (2020) 167:107980. doi: 10.1016/j.neuropharm.2020.107980
35. Jiang G, Liu J, Ren B, Zhang L, Owusu L, Liu L, et al. Anti-tumor and chemosensitization effects of cryptotanshinone extracted from Salvia miltiorrhiza bge. On ovarian cancer cells in vitro. J Ethnopharmacol. (2017) 205:33–40. doi: 10.1016/j.jep.2017.04.026
36. Li K, Lai H. Tanshinone IIA enhances the chemosensitivity of breast cancer cells to doxorubicin through down-regulating the expression of MDR-related ABC transporters. Biomed Pharmacother. (2017) 96:371–7. doi: 10.1016/j.biopha.2017.10.016
37. Li K, Liu W, Zhao Q, Wu C, Fan C, Lai H, et al. Combination of tanshinone IIA and doxorubicin possesses synergism and attenuation effects on doxorubicin in the treatment of breast cancer. Phytother Res. (2019) 33(6):1658–69. doi: 10.1002/ptr.6353
38. Ma W, Rousseau Z, Slavkovic S, Shen C, Yousef GM, Ni H. Doxorubicin-induced platelet activation and clearance relieved by salvianolic acid compound: novel mechanism and potential therapy for chemotherapy-associated thrombosis and thrombocytopenia. Pharmaceuticals (Basel). (2022) 15(12):1444. doi: 10.3390/ph15121444
39. Liu X, Wang Y, Ma C, Zhang L, Wu W, Guan S, et al. Proteomic assessment of tanshinone IIA sodium sulfonate on doxorubicin induced nephropathy. Am J Chin Med. (2011) 39(2):395–409. doi: 10.1142/s0192415x11008907
40. Fan HY, Yang MY, Qi D, Zhang ZK, Zhu L, Shang-Guan XX, et al. Salvianolic acid A as a multifunctional agent ameliorates doxorubicin-induced nephropathy in rats. Sci Rep. (2015) 5:12273. doi: 10.1038/srep12273
41. Page MJ, Moher D, Bossuyt PM, Boutron I, Hoffmann TC, Mulrow CD, et al. PRISMA 2020 explanation and elaboration: updated guidance and exemplars for reporting systematic reviews. Br Med J. (2021) 372:n160. doi: 10.1136/bmj.n160
42. Hooijmans CR, Rovers MM, de Vries RB, Leenaars M, Ritskes-Hoitinga M, Langendam MW. SYRCLE’s risk of bias tool for animal studies. BMC Med Res Methodol. (2014) 14:43. doi: 10.1186/1471-2288-14-43
43. Lin TJ, Liu GT, Liu Y, Xu GZ. Protection by salvianolic acid A against adriamycin toxicity on rat heart mitochondria. Free Radic Biol Med. (1992) 12(5):347–51. doi: 10.1016/0891-5849(92)90083-s
44. Zhou GY, Zhao BL, Hou JW, Ma GE, Xin WJ. Protective effects of sodium tanshinone IIA sulphonate against adriamycin-induced lipid peroxidation in mice hearts in vivo and in vitro. Pharmacol Res. (1999) 40(6):487–91. doi: 10.1006/phrs.1999.0545
45. You JS, Pan TL, Lee YS. Protective effects of danshen (Salvia miltiorrhiza) on adriamycin-induced cardiac and hepatic toxicity in rats. Phytother Res. (2007) 21(12):1146–52. doi: 10.1002/ptr.2225
46. Gao J, Yang G, Pi R, Li R, Wang P, Zhang H, et al. Tanshinone IIA protects neonatal rat cardiomyocytes from adriamycin-induced apoptosis. Transl Res. (2008) 151(2):79–87. doi: 10.1016/j.trsl.2007.11.005
47. Jiang B, Zhang L, Li M, Wu W, Yang M, Wang J, et al. Salvianolic acids prevent acute doxorubicin cardiotoxicity in mice through suppression of oxidative stress. Food Chem Toxicol. (2008) 46(5):1510–5. doi: 10.1016/j.fct.2007.12.020
48. Jiang B, Zhang L, Wang Y, Li M, Wu W, Guan S, et al. Tanshinone IIA sodium sulfonate protects against cardiotoxicity induced by doxorubicin in vitro and in vivo. Food Chem Toxicol. (2009) 47(7):1538–44. doi: 10.1016/j.fct.2009.03.038
49. Hong HJ, Liu JC, Chen PY, Chen JJ, Chan P, Cheng TH. Tanshinone IIA prevents doxorubicin-induced cardiomyocyte apoptosis through akt-dependent pathway. Int J Cardiol. (2012) 157(2):174–9. doi: 10.1016/j.ijcard.2010.12.012
50. Chen R, Sun G, Yang L, Wang J, Sun X. Salvianolic acid B protects against doxorubicin induced cardiac dysfunction via inhibition of ER stress mediated cardiomyocyte apoptosis. Toxicol Res (Camb). (2016) 5(5):1335–45. doi: 10.1039/c6tx00111d
51. Zhang Y, Chen L, Li F, Wang H, Yao Y, Shu J, et al. Cryptotanshinone protects against adriamycin-induced mitochondrial dysfunction in cardiomyocytes. Pharm Biol. (2016) 54(2):237–42. doi: 10.3109/13880209.2015.1029052
52. Chen RC, Sun GB, Ye JX, Wang J, Zhang MD, Sun XB. Salvianolic acid B attenuates doxorubicin-induced ER stress by inhibiting TRPC3 and TRPC6 mediated ca(2+) overload in rat cardiomyocytes. Toxicol Lett. (2017) 276:21–30. doi: 10.1016/j.toxlet.2017.04.010
53. Song T, Yao Y, Wang T, Huang H, Xia H. Tanshinone IIA ameliorates apoptosis of myocardiocytes by up-regulation of miR-133 and suppression of caspase-9. Eur J Pharmacol. (2017) 815:343–50. doi: 10.1016/j.ejphar.2017.08.041
54. Guo Z, Yan M, Chen L, Fang P, Li Z, Wan Z, et al. Nrf2-dependent antioxidant response mediated the protective effect of tanshinone IIA on doxorubicin-induced cardiotoxicity. Exp Ther Med. (2018) 16(4):3333–44. doi: 10.3892/etm.2018.6614
55. Yu J, Gao H, Wu C, Xu QM, Lu JJ, Chen X. Diethyl blechnic, a novel natural product isolated from Salvia miltiorrhiza bunge, inhibits doxorubicin-induced apoptosis by inhibiting ROS and activating JNK1/2. Int J Mol Sci. (2018) 19(6):1809. doi: 10.3390/ijms19061809
56. Wang X, Li C, Wang Q, Li W, Guo D, Zhang X, et al. Tanshinone IIA restores dynamic balance of autophagosome/autolysosome in doxorubicin-induced cardiotoxicity via targeting Beclin1/LAMP1. Cancers (Basel). (2019) 11(7):910. doi: 10.3390/cancers11070910
57. Hung YC, Wang PW, Lin TY, Yang PM, You JS, Pan TL. Functional redox proteomics reveal that Salvia miltiorrhiza aqueous extract alleviates adriamycin-induced cardiomyopathy via inhibiting ROS-dependent apoptosis. Oxid Med Cell Longev. (2020) 2020:5136934. doi: 10.1155/2020/5136934
58. Li L, Wu B, Zhao Q, Li J, Han Y, Fan X, et al. Attenuation of doxorubicin-induced cardiotoxicity by cryptotanshinone detected through association analysis of transcriptomic profiling and KEGG pathway. Aging (Albany NY). (2020) 12(10):9585–603. doi: 10.18632/aging.103228
59. Wang X, Wang Q, Li W, Zhang Q, Jiang Y, Guo D, et al. TFEB-NF-κB inflammatory signaling axis: a novel therapeutic pathway of dihydrotanshinone I in doxorubicin-induced cardiotoxicity. J Exp Clin Cancer Res. (2020) 39(1):93. doi: 10.1186/s13046-020-01595-x
60. Wang X, Sun Q, Jiang Q, Jiang Y, Zhang Y, Cao J, et al. Cryptotanshinone ameliorates doxorubicin-induced cardiotoxicity by targeting akt-GSK-3β-mPTP pathway in vitro. Molecules. (2021) 26:5. doi: 10.3390/molecules26051460
61. Wu Y, Xiu W, Wu Y. Salvianolic acid A protects H9C2 cardiomyocytes from doxorubicin-induced damage by inhibiting NFKB1 expression thereby downregulating long-noncoding RNA (lncRNA) plasmacytoma variant translocation 1 (PVT1). Med Sci Monit. (2021) 27:e929824. doi: 10.12659/msm.929824
62. Jiang Q, Chen X, Tian X, Zhang J, Xue S, Jiang Y, et al. Tanshinone I inhibits doxorubicin-induced cardiotoxicity by regulating Nrf2 signaling pathway. Phytomedicine. (2022) 106:154439. doi: 10.1016/j.phymed.2022.154439
63. Qi JY, Yang YK, Jiang C, Zhao Y, Wu YC, Han X, et al. Exploring the mechanism of danshensu in the treatment of doxorubicin-induced cardiotoxicity based on network pharmacology and experimental evaluation. Front Cardiovasc Med. (2022) 9:827975. doi: 10.3389/fcvm.2022.827975
64. Xu L, He D, Wu Y, Shen L, Wang Y, Xu Y. Tanshinone IIA inhibits cardiomyocyte apoptosis and rescues cardiac function during doxorubicin-induced cardiotoxicity by activating the DAXX/MEK/ERK1/2 pathway. Phytomedicine. (2022) 107:154471. doi: 10.1016/j.phymed.2022.154471
65. Navarro-Hortal MD, Varela-López A, Romero-Márquez JM, Rivas-García L, Speranza L, Battino M, et al. Role of flavonoids against adriamycin toxicity. Food Chem Toxicol. (2020) 146:111820. doi: 10.1016/j.fct.2020.111820
66. Akbas HS, Timur M, Ozben T. Concurrent use of antioxidants in cancer therapy: an update. Expert Rev Clin Immunol. (2006) 2(6):931–9. doi: 10.1586/1744666x.2.6.931
67. Abushouk AI, Ismail A, Salem AMA, Afifi AM, Abdel-Daim MM. Cardioprotective mechanisms of phytochemicals against doxorubicin-induced cardiotoxicity. Biomed Pharmacother. (2017) 90:935–46. doi: 10.1016/j.biopha.2017.04.033
68. Chen X, Zhou ZW, Xue CC, Li XX, Zhou SF. Role of P-glycoprotein in restricting the brain penetration of tanshinone IIA, a major active constituent from the root of Salvia miltiorrhiza bunge, across the blood-brain barrier. Xenobiotica. (2007) 37(6):635–78. doi: 10.1080/00498250701411258
69. Yu XY, Lin SG, Zhou ZW, Chen X, Liang J, Liu PQ, et al. Role of P-glycoprotein in the intestinal absorption of tanshinone IIA, a major active ingredient in the root of Salvia miltiorrhiza bunge. Curr Drug Metab. (2007) 8(4):325–40. doi: 10.2174/138920007780655450
70. Zhang Y, Jiang P, Ye M, Kim SH, Jiang C, Lü J. Tanshinones: sources, pharmacokinetics and anti-cancer activities. Int J Mol Sci. (2012) 13(10):13621–66. doi: 10.3390/ijms131013621
71. Sun G, Sun K, Sun J. Combination prostate cancer therapy: prostate-specific membranes antigen targeted, pH-sensitive nanoparticles loaded with doxorubicin and tanshinone. Drug Deliv. (2021) 28(1):1132–40. doi: 10.1080/10717544.2021.1931559
72. Zhang J, Li Y, Fang X, Zhou D, Wang Y, Chen M. TPGS-g-PLGA/Pluronic F68 mixed micelles for tanshinone IIA delivery in cancer therapy. Int J Pharm. (2014) 476(1-2):185–98. doi: 10.1016/j.ijpharm.2014.09.017
73. Chen F, Zhang J, He Y, Fang X, Wang Y, Chen M. Glycyrrhetinic acid-decorated and reduction-sensitive micelles to enhance the bioavailability and anti-hepatocellular carcinoma efficacy of tanshinone IIA. Biomater Sci. (2016) 4(1):167–82. doi: 10.1039/c5bm00224a
74. Zhang X, Zong W, Cheng W, Han X. Codelivery of doxorubicin and sodium tanshinone IIA sulfonate using multicompartmentalized vesosomes to enhance synergism and prevent doxorubicin-induced cardiomyocyte apoptosis. J Mater Chem B. (2018) 6(32):5243–7. doi: 10.1039/c8tb01136b
75. Zhang B, Zhang Y, Dang W, Xing B, Yu C, Guo P, et al. The anti-tumor and renoprotection study of E-[c(RGDfK)(2)]/folic acid co-modified nanostructured lipid carrier loaded with doxorubicin hydrochloride/salvianolic acid A. J Nanobiotechnol. (2022) 20(1):425. doi: 10.1186/s12951-022-01628-x
Glossary
AIF, apoptosis-inducing factor; Akt, protein kinase B; ANT, adenine nucleotide translocator; AST, aspartate transaminase; ATF-6, activating transcription factor-6; Bak, Bcl-2- antagonist/killer; Bax, Bcl-2-associated × protein; Bcl-2, B-cell lymphoma 2; Bcl-xL, B-cell lymphoma-extra-large; Bim, Bcl-2 interacting mediator of cell death; CAT, catalase; CHOP, CCAAT-enhancer-binding protein homologous protein; CK, creatine kinase; CK-MB, creatine kinase-muscle/brain; COX2, Cyclooxygenase-2; CPT, cryptotanshinone; Cyp-D, cyclophilin D; Cyt c, cytochrome c; DAXX, death domain-associated protein; DHT, dihydrotanshinone; DIC, doxorubicin-induced cardiotoxicity; DOX, Doxorubicin; DSS, danshensu; ECG, electrocardiography; EF, ejection fraction; ERK1/2, external-signal regulated kinase1/2; FS, fractional shortening; GCLC, glutamate-cysteine ligase catalytic subunit; GRP78, glucose-regulated protein 78; GSH, glutathione; GSH-Px, GPX, glutathione peroxidase; GSK3β, glycogen synthase kinase 3β; HO-1, heme oxygenase-1; HW/TL, heart weight/tibia length; IkBα, NF-kappa-B inhibitor alpha; IKKα, IkB kinase-α; IKKβ, IkB kinase-β; IL-8, interleukin 8; iNOS, inducible nitric oxide synthase; JNK, p-c-Jun N-terminal kinase; JNK1/2, c-Jun N-terminal kinase 1/2; Keap1, Kelch-like ECH-associated protein 1; LAMP1, lysosomal-associated membrane proteins-1; LC3-II, light chain 3-II; LDH, lactate dehydrogenase; LVEDD, left ventricular end-diastolic dimension; LVEF, left ventricular ejection fraction; LVESD, left ventricular end-systolic dimension; LVFS, left ventricular fractional shortening; LVIDd, left ventricular internal diameter at diastolic phase; LVIDs, left ventricle internal diameter in systolic phase; MDA, malondialdehyde; MMP, mitochondrial membrane potential; MRP2, multidrug resistance-associated protein 2; mTOR, mammalian target of rapamycin; NFKB1, nuclear factor kappa B subunit 1; NO, oxidative stress-relative enzymes nitric oxide; NQO1, NAD(P)H dehydrogenase (quinone) 1; NRF-1, nuclear respiratory factor-1; Nrf2, nuclear factor (erythroid-derived 2)-like 2; PARP, Poly (ADP-ribose) polymerase; PGC-1α, Peroxisome proliferator-activated receptor γ coactivator-1α; P-gp, P-glycoprotein; PI3K, phosphatidylinositol 3-kinase; p-IRE-1, phosphorylated inositol requiring enzyme 1; P-JNK, phosphorylated c-Jun N-terminal kinase; p-MEK, phosphorylated mitogen-activated protein kinase; p-NF-κB, phosphorylated nuclear factor-κB; p-PERK, phosphorylated PKR-like ER kinase; PUMA, P53 up-regulated modulator of apoptosis; PV, pressure-volume; ROS, reactive oxygen species; Sai A, Sai B, salvianolic acid A and B; AISM, active ingredients in Salvia miltiorrhiza; SOD, superoxide dismutase; Tan I, tanshinone I; Tan IIA, tanshinone IIA; Tan IIB, tanshinone IIB; TBARS, TBA reactive substrate; TFAM, Transcription Factor A, Mitochondrial; TFEB, transcription factor EB; TNF-α, tumor necrosis factor-α; TRPC, transient receptor potential canonical; T-SOD, total superoxide dismutase; ULK1, UNC-51-like kinase 1
Keywords: Salvia miltiorrhiza, doxorubicin, Adriamycin, cardiotoxicity, cardioprotection
Citation: Wang Q, Li J, Chu X, Jiang X, Zhang C, Liu F, Zhang X, Li Y, Shen Q and Pang B (2023) Potential chemoprotective effects of active ingredients in Salvia miltiorrhiza on doxorubicin-induced cardiotoxicity: a systematic review of in vitro and in vivo studies. Front. Cardiovasc. Med. 10:1267525. doi: 10.3389/fcvm.2023.1267525
Received: 26 July 2023; Accepted: 3 October 2023;
Published: 17 October 2023.
Edited by:
Yu-Qing Zhang, McMaster University, CanadaReviewed by:
Fei Cai, Hubei University of Science and Technology, ChinaFan Yang, China Academy of Chinese Medical Sciences, China
© 2023 Wang, Li, Chu, Jiang, Zhang, Liu, Zhang, Li, Shen and Pang. This is an open-access article distributed under the terms of the Creative Commons Attribution License (CC BY). The use, distribution or reproduction in other forums is permitted, provided the original author(s) and the copyright owner(s) are credited and that the original publication in this journal is cited, in accordance with accepted academic practice. No use, distribution or reproduction is permitted which does not comply with these terms.
*Correspondence: Bo Pang drpangbo@gmail.com
†These authors have contributed equally to this work and share first authorship