- 1Department of Nuclear Medicine, The Third Affiliated Hospital of Soochow University, Changzhou, China
- 2Institute of Clinical Translation of Nuclear Medicine and Molecular Imaging, Soochow University, Changzhou, China
- 3Department of Nuclear Medicine, The first afflicted hospital of Ningbo University, Ningbo, China
- 4Department of Cardiology, The Third Affiliated Hospital of Soochow University, Changzhou, China
Background: Most coronary artery disease (CAD) patients with a normal left ventricular ejection fraction (LVEF) experience a poor prognosis. Single-photon emission computerized tomography (SPECT)–myocardial perfusion imaging (MPI), a routine examination, is useful in assessing risk and predicting major adverse cardiovascular events (MACEs) in populations with suspected or known CAD. SPECT/CT is a “one-stop shop” examination, which, through non-contrast CT, can produce attenuation correction for MPI and obtain information on coronary artery calcium (CAC) and epicardial fat volume (EFV) simultaneously. This study aims to investigate the predictive and incremental value of EFV to MPI for MACE in Chinese populations with suspected or known CAD with a normal LVEF.
Methods and results: We retrospectively studied 290 suspected or known CAD inpatients with a normal LVEF who underwent SPECT/CT between February 2014 and December 2017. Abnormal MPI was defined as a summed stress score ≥4 or summed difference score ≥2. EFV and CAC were calculated using non-contrast CT. The end date of follow-ups was in February 2022. The follow-up information was obtained from the clinical case notes of the patients or reviews of telephone calls. MACE was defined as cardiac death, late coronary revascularization ≥3 months after MPI, non-fatal myocardial infarction, angina-related rehospitalization, heart failure, and stroke. During the 76-month follow-up, the event rate was 32.0% (93/290). Univariate and multivariate Cox regression analyses concluded that high EFV (>108.3 cm3) [hazard ratio (HR): 3.3, 95% CI: 2.1–5.2, P < 0.000] and abnormal MPI (HR: 1.8, 95% CI: 1.1–2.8, P = 0.010) were independent risk factors for MACE. The event-free survival of patients with high EFV was significantly lower than that of the low EFV group (log-rank test P < 0.001). In the subgroup with normal MPI, high EFV was associated with reduced event-free survival (log-rank P < 0.01), with a higher annualized event rate (8.3% vs. 1.9%). Adding high EFV to MPI could predict MACEs more effectively, with a higher concordance index (0.56–0.69, P < 0.01), higher global chi square (7.2–41.4, P < 0.01), positive integrated discrimination improvement (0.10, P < 0.01), and net reclassification index (0.37, P < 0.01).
Conclusions: In Chinese populations with suspected or known CAD with normal LVEF, high EFV was an independent risk factor for MACE after adjusting for traditional risk factors, CAC and MPI. In subgroups with normal MPI, EFV could also improve risk stratification. Adding EFV to MPI had an incremental value for predicting MACE.
Introduction
Coronary artery disease (CAD), the most common cause of death worldwide, has affected over 11 million patients in China. Left ventricular ejection fraction (LVEF) is a common parameter in assessing left ventricular global contraction function in clinical practice. The prognostic importance of LVEF has been demonstrated, and patients with low LVEF have increased mortality rates compared with those with normal LVEF (1). However, 314 patients died in a cohort study of 3,816 CAD patients with normal LVEF, and the risk stratification of CAD patients with normal LVEF has been poorly studied (2). Previous studies showed that single-photon emission computerized tomography (SPECT)-myocardial perfusion imaging (MPI) can effectively predict major adverse cardiovascular events (MACEs) by evaluating myocardial ischemia (3). However, SPECT-MPI has several disadvantages and a high false negative rate (4, 5). Even if the results of the MPI are normal, there is still a potential risk of cardiac death, myocardial infarction (MI), or late coronary revascularization (6, 7). Therefore, improving the risk stratification of populations with suspected or known CAD with normal LVEF and the prediction of MPI for MACE is necessary. As a “one-stop shop” examination, SPECT/CT can not only perform MPI but also improve the diagnosis of MPI by attenuation correction through non-contrast CT. In addition, it quantitatively assesses the coronary artery calcium score (CACS) and epicardial fat volume (EFV) simultaneously. CACS has been reported to be associated with MACE, which is controversial (8), and there are racial differences in CACS, with the lowest levels found in Asians (9).
Epicardial adipose tissue (EAT), which can be quantitatively measured using EFV, is a localized fat depot between the serous epicardium and pericardial fibers with paracrine and autocrine functions (10–13). By secreting various inflammatory factors, EAT can promote the occurrence and development of CAD, which may be an important predictor for MACE (14). Our previous study (15) demonstrated that the EFV was significantly associated with obstructive CAD in the Chinese population. In addition, a large population study (16) which included 5,743 Chinese patients showed that EFV improved the prediction of CAD, compared with clinical risk factors and CACS. There were significant racial differences in EFV (17, 18), and it was reported that EFV had an increased value in predicting MACE in western populations (8). The relationship between EFV and MACE and whether EFV can provide an incremental prognostic value to MPI in Chinese populations with suspected or known CAD with normal LVEF is still unknown.
This study aims to investigate the relationship between EFV and MACE and determine whether EFV could provide an incremental value above SPECT-MPI in predicting MACE in Chinese populations with suspected or known CAD with normal LVEF.
Method
Study population
The study was retrospective, and we consecutively enrolled 535 suspected and known CAD patients who underwent SPECT/CT to evaluate myocardial ischemia between February 2014 and December 2017 at the Third Affiliated Hospital of Soochow University. SPECT/CT is a “one-stop shop” examination, which can not only perform MPI but also produce attenuation correction and acquire CAC and EFV simultaneously through a non-contrast CT scan. Initially, 165 outpatients were excluded, followed by 40 inpatients that underwent rest-only SPECT-MPI. A total of 10 patients had incomplete clinical data or information, and 16 patients had inadequate image quality of non-contrast CT scans. Finally, the analysis included 290 inpatients. Figure 1 shows the flow chart. Patients with a history of MI, percutaneous coronary intervention (PCI), or coronary artery bypass grafting (CABG) were defined as known CAD (19).
Clinical data
Body mass index (BMI) was calculated as weight (kg) divided by height squared (m2). Hypertension was defined as systolic blood pressure ≥140 mmHg, diastolic blood pressure ≥90 mmHg, or use of antihypertensive medication. Hypertension was defined as having a history of hypertension or receiving antihypertensive therapy. Diabetes mellitus was defined by random blood glucose ≥11.1 mmol/L or fasting blood glucose ≥7 mmol/L or 2-h postprandial blood glucose (oral glucose tolerance test) ≥11.1 mmol/L or HbA1c ≥6.5%. Data for LVEF were obtained by echocardiography before SPECT-CT. LVEF ≥ 50% was defined as normal; LVEF<50% was defined as abnormal. Hyperlipidemia was defined as a history of hyperlipidemia, total cholesterol >5.2 mmol/L, low-density lipoprotein cholesterol >3.36 mmol/L, or use of lipid-lowering medications. Current smokers were defined as smoking one cigarette per day for at least half a year.
SPECT/CT imaging acquisition
SPECT-MPI was performed according to indication and a 2-day standard imaging protocol. Medications such as nitrates and beta-blockers were not allowed before testing. Approximately 69.6% of the patients underwent stress testing with exercise stress MPI based on the Bruce protocol, and 30.4% received pharmacological stress MPI with adenosine intravenously (i.v.) infused at 0.14 mg/kg/min for 6 min. Any products containing methylxanthines, including caffeinated coffee, tea, or other caffeinated beverages, caffeinated medicines, and theophylline, were avoided before using adenosine intravenously for at least 12 h. MPI acquisitions were initiated 60–90 min after intravenous injection of 99mTc-MIBI (radiochemical purity 95%, injected dose of 740–925 MBq). MPI was performed using a dual-head camera at a 90° angle (SPECT/CT Symbia T16, Siemens Medical Systems, Erlangen, Germany). This setup included a high-resolution low-energy parallel hole collimator and a 180° orbit (from the right anterior oblique of 45° to the left posterior oblique of 45°). MPI was produced using a matrix of 128 × 128, magnification of 1.45, and a 20% window centered on the 140 keV peak energy. We used the filtered back projection method (Butterworth filter with cutoff frequency of 0.35 and order of 5) to reconstruct MPI and acquire horizontal long axis, vertical long axis, and short axis images. A non-contrast CT chest scan was acquired for attenuation correction after MPI. EFV and CACS were obtained through the non-contrast chest CT scan after rest MPI. The following scan parameters were then executed: 60%–80% RR, tube voltage: 130 kV, tube current: 100 mAs, and thickness: 3 mm. Each scan extended from the plane underneath the tracheal carina to the place 1–2 cm below the heart diaphragmatic surface, which is almost 20 cm.
Imaging data processing and analysis
Two experienced nuclear medicine physicians, unaware of the clinical information of the patients, independently performed the imaging. In cases where the two nuclear medicine physicians had differing opinions, a third expert was consulted to establish consensus results. Myocardial perfusion images were divided into 17 segments, and a 5-point continuous scoring system was used (0: normal, 1: mild abnormality, 2: moderate abnormality, 3: severe abnormality, and 4: segmental loss of uptake). The summed stress score (SSS) and summed rest score (SRS) were calculated from all abnormal segments. The summed difference score (SDS) was determined by subtracting SRS from SSS. Abnormal MPI was defined as SSS ≥4 or SDS ≥2 (20).
CAC
CAC was identified as the dense area in the coronary arteries that exceeded the 130 HU threshold measured using Agatston automated analysis software. For patients who previously underwent coronary artery bypass surgery, the Agatston score was calculated using only the native vessel. Segments previously implanted with coronary stents were excluded from CAC calculations (21).
EFV
EFV is identified as any adipose tissue located within the pericardial sac. The specific methods used are as follows: initially, the upper limit of the slice is determined by the bifurcation of the pulmonary artery, while the lower limit of the slice is defined as the last slice that contains any part of the heart. The pericardial contour was then manually traced using the reconstructed axial slices. The sum of all pixels within the −190 to −30 HU window of the region of interest was defined as a fat voxel. EFV was calculated by multiplying the sum of the cross-sectional areas of the fat by the slice thickness (22). The whole process was performed using software (Syngo, Siemens Medical Solutions). The intra-class correlation coefficient for EFV was 0.940 (95% CI: 0.855–0.976, P < 0.001).
Radiation exposure
The standard effective radiation dose of 99mTc-MIBI imaging (including rest and stress imaging) was 9–12 mSv. The dose of the non-contrast CT scan (voltage, 130 kV; tube current, 100 mA) used to assess CACS and EFV was 1–2 mSv.
Patient follow-up and MACE
The follow-up information was obtained from patient clinical case notes or telephone reviews. All endpoints were determined by consensus between two blind reviewers. The follow-up deadline was February 2022. MACE was defined as cardiac death, late coronary revascularization ≥3 months after MPI (23), non-fatal MI, angina-related rehospitalization, heart failure, and stroke. Cardiac death was defined as death due to acute MI, ventricular arrhythmia, or cardiogenic shock. Late coronary revascularization ≥3 months after SPECT-MPI included CABG and PCI. Those with early coronary revascularizations within 3 months from SPECT-MPI were excluded from the MACE since these procedures were likely to be triggered by the SPECT-MPI test result itself. Non-fatal MI was diagnosed based on symptoms, enzymes (creatine kinase levels, troponin T or I), and ECG signs (23). Angina-related rehospitalization was defined as chest pain or chest pain equivalent with dynamic ECG changes such as ST depression or T-wave inversion without abnormal cardiac biomarkers and characterized by rest symptoms, new-onset angina (less than 2 months), or an increased duration or severity of earlier stable angina symptoms. The diagnosis of heart failure was confirmed following the established criteria (24). Stroke was identified as an ischemic cerebral infarction induced by a thrombotic or embolic occlusion of any major intracranial artery (25).
Statistical analysis
Continuous variables are expressed as mean ± standard deviation, and categorical variables are expressed as numbers and percentages. Comparisons were analyzed for significant differences using the t-test, Mann–Whitney U-test, or chi-squared test. Time-dependent receiver-operating characteristic (ROC) (survival ROC) curves were applied to assess the EFV prognostic power, and the optimal cutoff value of EFV for predicting MACE was 108.3 cm3, which was used to split the data into two categories. The Kaplan–Meier (K–M) method was used to analyze the event-free survival rate over time, and the differences between survival curves were compared using the log-rank test. The point-biserial correlation coefficient was used in correlation analysis. A collinearity analysis was performed to exclude all indicators with a variance inflation factor of >5. Cox proportional hazards regression models were used to identify predictors of MACE, and hazard ratios (HR) with 95% confidence intervals (CI) were calculated. Univariable and multivariable Cox regression models were created to assess the association between EFV and MACE. The global chi-squared test, C-index, net reclassification index (NRI), and integrated discrimination improvement (IDI) were used to analyze the incremental prognostic values of EFV to MPI. All statistical analyses were performed using a commercially available software package (R).
Result
Study population
This analysis included 290 inpatients (mean age: 67.62 ± 9.52 years, male: 57.9%). Table 1 summarizes their detailed characteristics. The average value of LVEF was 62.7%. The maximum value of EFV was 247.89 cm3, and the minimum value of EFV was 37.3 cm3. Mean ± standard deviation of EFV was 114.56 ± 36.63 cm3. The median (Q1, Q3) was 107.94 (88.69, 137.73) cm3. Abnormal MPI occurred in 77 (26.5%) patients, and MACEs occurred in 93 patients (32.0%), including seven cardiac deaths, 15 non-fatal MIs, 10 revascularizations, 48 angina-related rehospitalizations, two heart failures, and 11 strokes.
Differences between the MACE group and without MACE group
Among patients who experienced MACE, male proportion, hypertension, diabetes mellitus (DM), hyperlipidemia, current smoker, high EFV (EFV >108.3 cm3), CAC, and abnormal MPI were more common than in patients without MACE (P for all <0.05) (Table 1).
The relationship between EFV and MACE
In the K–M survival curve analysis, the event-free survival rate of patients with high EFV was significantly lower than that of patients with low EFV, and there was a significant difference between the curves based on the log-rank test (P < 0.001) (Figure 2C). Subgroup analysis showed that patients with high EFV were more likely to experience MACE, and this trend was consistent in the suspected and known CAD group (both log-rank P-values <0.001, Figures 2A, B). In the univariate Cox proportional hazards regression analysis, age (HR: 1.0, 95% CI: 1.0–1.0), male proportion (HR: 1.8, 95% CI: 1.2–2.8), high EFV (HR: 3.1, 95% CI: 2.1–4.7), CAC (HR: 2.2, 95% CI: 1.5–3.4), and abnormal MPI (HR: 1.8, 95% CI: 1.2–2.7) were associated with MACE (P for all <0.05). In univariate and multivariate Cox proportional hazards regression analyses employing traditional risk factors (age, male gender, BMI, current smoking status, presence of hypertension, DM, and hyperlipidemia), CAC, high EFV, and abnormal MPI, only high EFV (HR: 3.3, 95% CI: 2.1–5.2, P < 0.001) and abnormal MPI (HR: 1.8, 95% CI: 1.1–2.8, P = 0.010) were significantly associated with MACE (Table 2). Patients with abnormal MPI exhibited lower event-free survival rates in the K–M survival curve compared with those with normal MPI, and these differences were significant based on the log-rank test (P < 0.001) (Supplementary Figure 1).
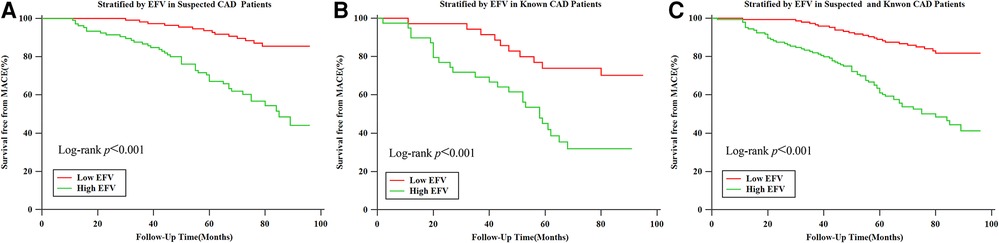
Figure 2. Kaplan–Meier survival curves for patients with low (≤108.3 cm3) and high (>108.3 cm3) EFV. (A) Kaplan–Meier survival curves for patients with low (≤108.3 cm3) and high (>108.3 cm3) EFV in suspected CAD group populations. (B) Kaplan–Meier survival curves for patients with low (≤108.3 cm3) and high (>108.3 cm3) EFV in known CAD populations. (C) Kaplan–Meier survival curves for patients with low (≤108.3 cm3) and high (>108.3 cm3) EFV in suspected and known CAD populations.
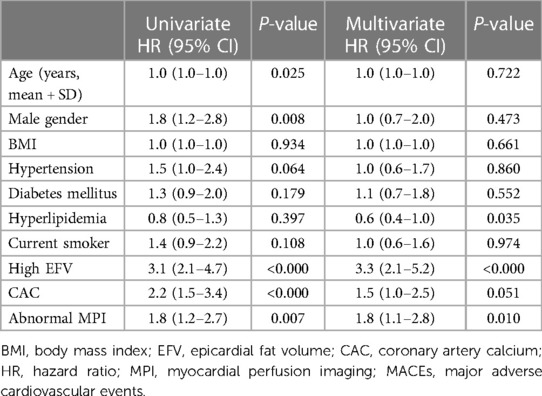
Table 2. Univariable and multivariable Cox proportional hazard regression analyses for predicting MACE.
Incremental predictive value of EFV to MPI for MACE
To further assess the predictive value of EFV as an adjunct to MPI for MACE, we calculated the global chi-squared score, concordance index, IDI, and NRI (Figure 3). It has shown that after adding EFV to MPI, the global chi-squared score increased significantly from 7.2 to 41.4 (P < 0.01), and the concordance index increased from 0.59 to 0.69 (P < 0.01). A positive IDI of 0.10 and positive NRI of 0.37 (both P < 0.01) were noted.
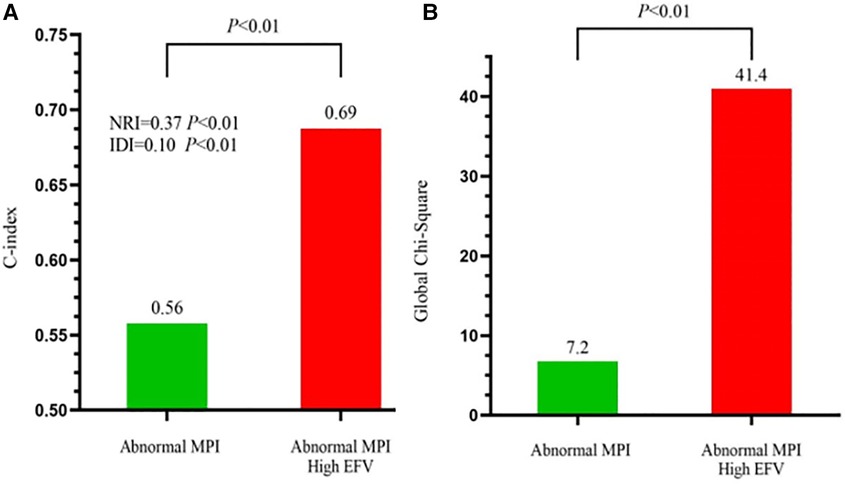
Figure 3. Incremental predictive value of EFV to MPI for MACE. (A) Receiver-operating characteristic curve analysis to evaluate the incremental prognostic value of EFV >108.3 cm3 over abnormal MPI. C-index, concordance index. (B) Global chi-squared test analysis to evaluate the incremental prognostic value of EFV >108.3 cm3 over abnormal MPI.
Risk stratification analysis
Figure 4A shows the annualized event rates among four subgroups stratified by MPI (normal and abnormal) and EAT volume (cutoff value: 108.3 cm3), which were 1.9%, 8.3%, 4.5%, and 14.1% in normal MPI and low EFV, normal MPI and high EFV, abnormal MPI and low EFV, and abnormal MPI and high EFV, respectively. In the normal MPI subgroup, K–M survival curves reveal a higher cumulative event rate for patients with high EFV (log-rank P < 0.001). High EFV was associated with a higher HR for MACE (HR: 4.24, 95% CI: 2.51–7.17, P = 0.001) (Figure 4B). Figure 5 is the case example.
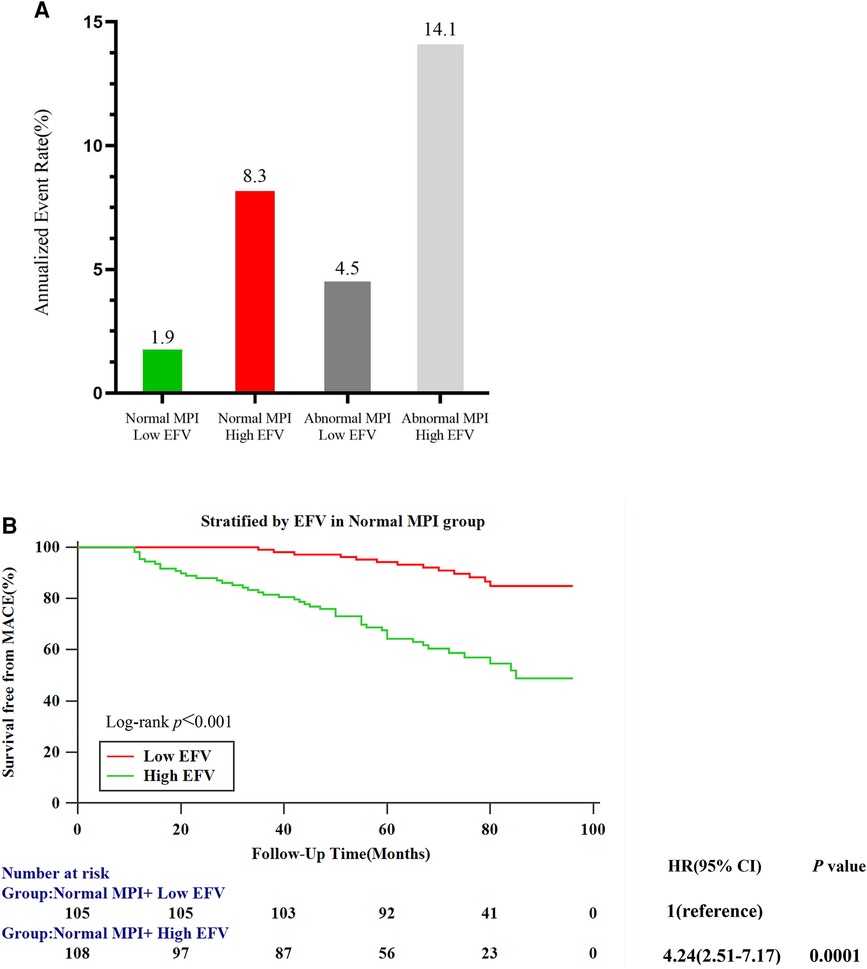
Figure 4. Risk stratification analysis. (A) Annualized event rates for MACEs by the combination of MPI and EFV. (B) Kaplan–Meier survival curves for patients with low (≤108.3 cm3) and high (>108.3 cm3) EFV in the normal MPI subgroup.
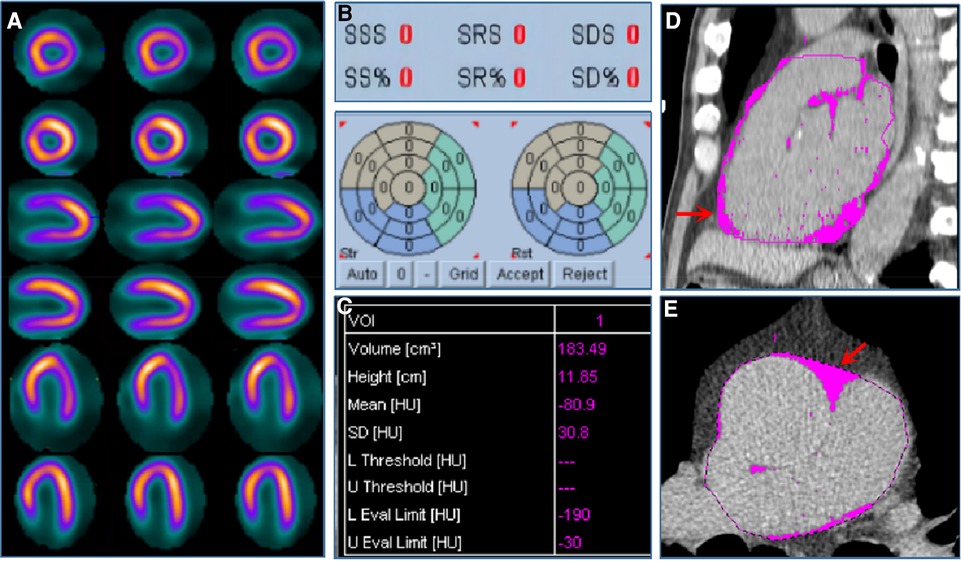
Figure 5. Case example. A 57-year-old male patient was admitted to the hospital with atypical chest pain for half a month. The patient was diagnosed with suspected CAD and received stress–rest MPI. (A) shows stress (the odd row) and rest (the even row) MPI of the short axis, vertical long axis, and horizontal long axis images, respectively, indicating the normal MPI with SDS equaled to 0 (B). (C) shows EFV was 183.49 cm3. (D,E) show EAT in pink of coronal and sagittal views from non-contrast CT scan.
Discussion
In this study, we examined the association of EFV with MACE in a Chinese population with suspected and known CAD with normal LVEF. K–M curve analysis based on the log-rank test indicated that patients with high EFV have a higher risk of MACE. Through Cox univariate and multivariate regression analyses, we observed that high EFV was an independent risk factor for MACE after adjusting for traditional risk factors, CAC, and abnormal MPI. Adding EFV to MPI had a significantly incremental value for predicting MACE. Among patients with normal MPI, the high EFV group had a higher risk of MACE.
CAD is the major cause of mortality in the Chinese population and is a serious threat to the national health. It is well known that patients with reduced LVEF have a poor prognosis. However, in the early compensatory period, LVEF is usually normal, even though myocardial impairment or ischemia may already exist (26). A study has shown that 8.2% of patients also experienced death in CAD patients with normal LVEF (2), and the risk stratification of CAD patients with normal LVEF has been poorly studied. Effective risk stratification of suspected or known CAD patients with normal LVEF is important in improving the prognosis. As a classic examination, MPI is a commonly used imaging tool for the non-invasive diagnosis of myocardial ischemia and risk stratification. In the present study, we concluded that abnormal MPI was significantly associated with MACE after performing Cox regression univariate and multivariate analyses. However, MPI has low spatial resolution, and balanced ischemia can lead to false negatives, which may affect risk stratification and clinical decision-making. Previous studies found that CAC, obtained from the one-stop shop SPECT/CT, was an independent predictor of MACE, and it can supply incremental value to MPI for predicting events (8). In this study, CAC was significantly associated with MACE in univariate analysis. However, statistical significance was lost after performing a multivariate analysis adjusting for clinical risk factors, possibly due to the racial differences and patients enrolled. A multiethnic study of atherosclerosis showed significant racial differences in CACS, and the Chinese population had the lowest CACS for both men and women (9). Similar to our result, after adjusting for traditional risk factors, CAC could not effectively predict MACE in Asian participants (27).
EAT, an ectopic adipose tissue directly surrounding the coronary arteries, can secrete various cytokines and adipokines through paracrine and endocrine pathways, damaging the myocardium and coronary arteries (28). EAT is not evenly distributed around the heart. It is most commonly found around the right ventricle, the right atrioventricular groove, and the apex of the heart. In contrast, it is least commonly found around the left anterior descending artery and the left atrioventricular groove. EAT can be quantitatively evaluated by EFV, which can be measured from the same CT image used to measure CACS. This approach has been given more and more attention recently. Previous studies have shown that elevated EFV may be associated with early atherosclerosis, and patients with abnormal EFV may be in a subclinical atherosclerotic state (29). In addition, EFV is important in affecting vascular function, especially in response to plaque changes and in influencing microvessels (30). The association between EFV and MACE has not been elucidated yet. It was demonstrated that high EFV may be an independent predictor of future coronary events in patients without proven CAD (23, 31). In addition, Mahabadi et al. (32) concluded that EFV is associated with fatal and non-fatal coronary events in the general population independent of traditional cardiovascular risk factors and provided additional information above the CACS. On the contrary, Possner et al. (33) found that EFV was associated with MACE and may improve risk stratification beyond clinical cardiovascular risk factors in populations with suspected and known CAD. However, when CACS and/or SPECT-MPI results were available, EFV did not provide any additional clinically relevant prognostic value. It is worth noting that their study population consists of Caucasians, and they did not consider racial differences. The distribution of EFV has obvious racial and geographical differences (34, 35), and EFV was significantly higher in Asians compared with Caucasians. Previous large cohort studies have confirmed that EFV was associated with obstructive CAD in the Chinese population (16). In addition, our previous results support the notion that the accumulation of EAT plays a critical role in the development of CAD, but the prognostic value of EFV for Chinese patients with suspected and known CAD with normal LVEF has not been extensively studied. In the present study, we adopted 108.3 cm3 as the cutoff value of EFV based on the time-dependent ROC (survival ROC) curves. We demonstrated that patients with high EFV have a higher risk of MACE, and EFV was an independent prognostic factor of MACE in the Chinese population with suspected and known CAD with normal LVEF after adjusting for traditional risk factors, CAC, and abnormal MPI. Our results showed that among patients with normal MPI, those in the high EFV group had a higher risk of MACEs.
This study followed up suspected and known CAD subjects with normal LVEF for approximately 6 years and showed that adding EAT to MPI had a significantly incremental value for predicting MACE. To our knowledge, this is the first study to demonstrate that EFV can provide an incremental value for MPI in predicting the prognosis in the Chinese population with suspected and known CAD with normal LVEF. The most provocative finding is that the high EFV group has significantly worse long-term outcomes, even in a normal SPECT group. EFV represents a novel therapeutic target for the treatment of CAD. Statins have been shown to induce a reduction of EFV and a direct anti-inflammatory effect on EAT (36). High EFV leads to a poor prognosis in Chinese populations with suspected or known CAD with normal LVEF, which may further improve their risk stratification. Through treatment and with the reduction of EFV, it had the potential to improve outcomes, which warrants additional investigation.
Limitation
Several limitations were found in this study. First, this study is based on a single-center retrospective study, and there may be bias in the included population. Second, this study did not measure the perivascular Fat Attenuation Index (FAI), and we will quantitatively measure the FAI in the future to evaluate whether FAI could add a prognostic value beyond that provided by EFV, MPI, and CACS. Third, we did not consider the prognostic impact of drug use such as statins and aspirin. Further prospective observational studies are needed to evaluate the effect of statins on improving prognosis by reducing EFV. Fourth, all subjects were of the Chinese population with suspected and known CAD with normal LVEF, and the findings cannot be extrapolated to other populations. Further investigations are required to clarify the validity of our findings in patients with reduced LVEF. In addition, only a few patients (n = 8) underwent reduced LVEF during the follow-up, which is insufficient for statistical analysis. Follow-up studies are warranted to clarify the relationship between changes in LVEF and primary endpoint further. Finally, we did not distinguish fat types such as brown fat and white fat. Inflammatory white adipose tissue and anti-inflammatory brown adipose tissue may have different effects on MACEs (37, 38).
Conclusion
In Chinese populations with suspected or known CAD with normal LVEF, EFV was the independent risk factor for MACE after adjusting for traditional risk factors, CAC, and MPI. In subgroups with normal MPI, EFV could also improve risk stratification. Adding EFV to MPI had an incremental value for predicting MACE.
Data availability statement
The raw data supporting the conclusions of this article will be made available by the authors, without undue reservation.
Author contributions
LY: Writing – Original draft. WY: Writing – Original draft. PW: Data curation, Writing – Original draft. JWW: Writing – Review & editing, Investigation, Methodology. XS: Data curation, Writing – Review & editing. FZ: Data curation, Writing – Original draft. XY: Writing – Review & editing. YC: Writing – Review & editing, formal analysis. QL: Writing – Review & editing, Data curation. DJ: Writing – Review & editing, Methodology. YFW: Investigation, Writing – Review & editing. QJ: Writing – Review & editing, Investigation. JFW: Supervision, Writing – Review & editing. YW: Conceptualization, Supervision, Writing – Review & editing.
Funding
This research was supported by National Natural Science Foundation of China (82272031, 81871381, PI: YW), Key Research and Development Program of Jiangsu Province (Social Development) (Grant NO. BE2021638), Top Talent of Changzhou “The 14th Five-Year Plan” High-Level Health Talents Training Project [(2022)260], Changzhou Clinical Medical Center [(2022)261], Science and technology project for youth talents of Changzhou Health Committee (QN202212, PI: WY), Young Talent Development Plan of Changzhou Health Commission (CZQM2023008, PI: WY.
Conflict of interest
The authors declare that the research was conducted in the absence of any commercial or financial relationships that could be construed as a potential conflict of interest.
Publisher's note
All claims expressed in this article are solely those of the authors and do not necessarily represent those of their affiliated organizations, or those of the publisher, the editors and the reviewers. Any product that may be evaluated in this article, or claim that may be made by its manufacturer, is not guaranteed or endorsed by the publisher.
Supplementary material
The Supplementary Material for this article can be found online at: https://www.frontiersin.org/articles/10.3389/fcvm.2023.1261215/full#supplementary-material
Supplementary Figure 1
Kaplan–Meier survival curves by MPI for the prediction of MACEs in suspected and known CAD.
Abbreviations
CAD, coronary artery disease; LVEF, left ventricular ejection fraction; SPECT, single-photon emission computerized tomography; MPI, myocardial perfusion imaging; MACE, major adverse cardiovascular events; CACS, coronary artery calcium score; EAT, epicardial adipose tissue; EFV, epicardial fat volume; CABG, coronary artery bypass grafting; BMI, body mass index; SSS, summed stress score; SRS, summed rest score; SDS, summed difference score; PCI, percutaneous coronary intervention.
References
1. Pocock SJ, Ariti CA, McMurray Jl, Maggioni A, Kober L, Squire IB, et al. Predicting survival in heart failure: a risk score based on 39 372 patients from 30 studies. Eur Heart J. (2013) 34(19):1404–13. doi: 10.1093/eurheartj/ehs337
2. Siontis GC, Branca M, Serruys P, Silber S, Räber L, Pilgrim T, et al. Impact of left ventricular function on clinical outcomes among patients with coronary artery disease. Eur J Prev Cardiol. (2019) 26(12):1273–84. doi: 10.1177/2047487319841939
3. Hachamovitch R, Hayes SW, Friedman JD, Cohen I, Berman DS Stress myocardial perfusion single-photon emission computed tomography is clinically effective and cost effective in risk stratification of patients with a high likelihood of coronary artery disease (CAD) but no known CAD. J Am Coll Cardiol. (2004) 43(2):200–8. doi: 10.1016/j.jacc.2003.07.043
4. Bateman TM. Advantages and disadvantages of PET and SPECT in a busy clinical practice. J Nucl Cardiol. (2012) 19(Suppl 1):S3–11. doi: 10.1007/s12350-011-9490-9
5. Sirajuddin A, Mirmomen SM, Kligerman SJ, Groves DW, Burke AP, Kureshi F, et al. Ischemic heart disease: noninvasive imaging techniques and findings. Radiographics. (2021) 41(4):990–1021. doi: 10.1148/rg.2021200125
6. Go V, Bhatt MR, Hendel RC. The diagnostic and prognostic value of ECG-gated SPECT myocardial perfusion imaging. J Nucl Med. (2004) 45(5):912–21.15136643
7. Kassab K, Hussain K, Torres A, et al. The diagnostic and prognostic value of near-normal perfusion or borderline ischemia on stress myocardial perfusion imaging. J Nucl Cardiol. (2022) 29(2):826–35. doi: 10.1007/s12350-020-02375-y
8. Kassab K, Hussain K, Torres A, Iskander F, Iskander M, Khan R, et al. Long-term prognostic value of coronary artery calcium scanning, coronary computed tomographic angiography and stress myocardial perfusion imaging in patients with suspected coronary artery disease. J Nucl Cardiol. (2018) 25(3):833–41. doi: 10.1007/s12350-016-0657-2
9. McClelland RL, Chung H, Detrano R, Post W, Kronmal RA Distribution of coronary artery calcium by race, gender, and age: results from the Multi-Ethnic Study of Atherosclerosis (MESA). Circulation. (2006) 113(1):30–7. doi: 10.1161/CIRCULATIONAHA.105.580696
10. Arita Y, Kihara S, Ouchi N, Maeda K, Kuriyama H, Okamoto Y, et al. Adipocyte-derived plasma protein adiponectin acts as a platelet-derived growth factor-BB-binding protein and regulates growth factor-induced common postreceptor signal in vascular smooth muscle cell. Circulation. (2002) 105(24):2893–8. doi: 10.1161/01.CIR.0000018622.84402.FF
11. Hirata Y, Tabata M, Kurobe H, Motoki T, Akaike M, Nishio C, et al. Coronary atherosclerosis is associated with macrophage polarization in epicardial adipose tissue. J Am Coll Cardiol. (2011) 58(3):248–55. doi: 10.1016/j.jacc.2011.01.048
12. Iacobellis G Epicardial adipose tissue in contemporary cardiology. Nat Rev Cardiol. (2022) 19(9):593–606. doi: 10.1038/s41569-022-00679-9
13. Mazurek T, Zhang L, Zalewski A, Mannion JD, Diehl JT, Arafat H, et al. Human epicardial adipose tissue is a source of inflammatory mediators. Circulation. (2003) 108(20):2460–6. doi: 10.1161/01.CIR.0000099542.57313.C5
14. Sinha SK, Thakur R, Jha Ml, Goel A, Kumar V, Kumar A, et al. Epicardial adipose tissue thickness and its association with the presence and severity of coronary artery disease in clinical setting: a cross-sectional observational study. J Clin Med Res. (2016) 8(5):410–9. doi: 10.14740/jocmr2468w
15. Yu W, Zhang F, Liu B, Wang J, Shao X, Yang M, et al. Incremental value of epicardial fat volume to coronary artery calcium score and traditional risk factors for predicting myocardial ischemia in patients with suspected coronary artery disease. J Nucl Cardiol. (2021) 29(4):1583–92. doi: 10.1007/s12350-021-02538-5
16. Zhou l, Chen Y, Zhang Y, Wang H, Tan Y, Liu Y, et al. Epicardial fat volume improves the prediction of obstructive coronary artery disease above traditional risk factors and coronary calcium score. Circ Cardiovasc Imaging. (2019) 12(1):e008002. doi: 10.1161/CIRCIMAGING.118.008002
17. Moharram MA, Aitken-Buck HM, Reijers R, Hout IV, Williams MJ, Jones P, et al. Correlation between epicardial adipose tissue and body mass index in New Zealand ethnic populations. N Z Med J. (2020) 133(1516):22–32.32525859
18. Sun DF, Kangaharan N, Costello B, Nicholls SJ, Emdin CA, Tse R, et al. Epicardial and subcutaneous adipose tissue in indigenous and non-indigenous individuals: implications for cardiometabolic diseases. Obes Res Clin Pract. (2020) 14(1):99–102. doi: 10.1016/j.orcp.2019.12.001
19. Trpkoy C, Savtchenko A, Liang Z, Feng P, Southern DA, Wilton SB, et al. Visually estimated coronary artery calcium score improves SPECT-MPI risk stratification. Int J Cardiol Heart Vasc. (2021) 35:100827. doi: 10.1016/j.ijcha35:100827.19;35:100827
20. Xu Y, Fish M, Gerlach J, Lemley M, Berman DS, Germano G, et al. Combined quantitative analysis of attenuation corrected and non-corrected myocardial perfusion SPECT: method development and clinical validation. J Nucl Cardiol. (2010) 17(4):591–9. doi: 10.1007/s12350-010-9220-8
21. Mahaisavariya P, Detrano R, Kang X, Garner D, Vo A, Georgiou D, et al. Quantitation of in vitro coronary artery calcium using ultrafast computed tomography. Cathet Cardiovasc Diagn. (1994) 32(4):387–93. doi: 10.1002/ccd.1810320421
22. Huang G, Wang D, Zeb I, Budoff MJ, Harman SM, Miller V, et al. Intra-thoracic fat, cardiometabolic risk factors, and subclinical cardiovascular disease in healthy, recently menopausal women screened for the Kronos Early Estrogen Prevention Study (KEEPS). Atherosclerosis. (2012) 221(1):198–205. doi: 10.1016/j.atherosclerosis.2011.12.004
23. Kunita E, Yamamoto H, Kitagawa T, Ohashi N, Oka T, Utsunomiya H, et al. Prognostic value of coronary artery calcium and epicardial adipose tissue assessed by non-contrast cardiac computed tomography. Atherosclerosis. (2014) 233(2):447–53. doi: 10.1016/j.atherosclerosis.2014.01.038
24. Ponikowski P, Voors AA, Anker SD, Bueno H, Cleland JGF, Coats AJS, et al. 2016 ESC guidelines for the diagnosis and treatment of acute and chronic heart failure: the task force for the diagnosis and treatment of acute and chronic heart failure of the European Society of Cardiology (ESC). Developed with the special contribution of the Heart Failure Association (HFA) of the ESC. Eur J Heart Fail. (2016) 18(8):891–975. doi: 10.1002/ejhf.592
25. Liu L, Dai N, Yin G, Zhang W, Mohammed A, Xu S, et al. Prognostic value of combined coronary angiography-derived IMR and myocardial perfusion imaging by CZT SPECT in INOCA. J Nucl Cardiol. (2022) 30(2):684–701. doi: 10.1007/s12350-022-03038-w
26. Murphy SP, Ibrahim NE, Januzzi JL Jr. Heart failure with reduced ejection fraction: a review. JAMA. (2020) 324(5):488–504. doi: 10.1001/jama.2020.10262
27. Yamamoto H, Kitagawa T, Kihara Y. Clinical implications of the coronary artery calcium score in Japanese patients. J Atheroscler Thromb. (2014) 21(11):1101–8. doi: 10.5551/jat.26427
28. Baker AR, Harte AL, Howell N, Pritlove DC, Ranasinghe AM, Silva NFD, et al. Epicardial adipose tissue as a source of nuclear factor-kappaB and c-Jun N-terminal kinase mediated inflammation in patients with coronary artery disease. J Clin Endocrinol Metab. (2009) 94(1):261–7. doi: 10.1210/jc.2007-2579
29. Baker AR, Harte AL, Howell N, Pritlove DC, Ranasinghe AM, Silva NFD, et al. Epicardial adipose tissue density and volume are related to subclinical atherosclerosis, inflammation and major adverse cardiac events in asymptomatic subjects. J Cardiovasc Comput Tomogr. (2018) 12(1):67–73. doi: 10.1016/j.jcct.2017.11.007
30. Nappi C, Ponsiglione A, Acampa W, Gaudieri V, Zampella E, Assante R, et al. Relationship between epicardial adipose tissue and coronary vascular function in patients with suspected coronary artery disease and normal myocardial perfusion imaging. Eur Heart J Cardiovasc Imaging. (2019) 20(12):1379–87. doi: 10.1093/ehjci/jez182
31. Payne GA, Kohr MC, Tune JD. Epicardial perivascular adipose tissue as a therapeutic target in obesity-related coronary artery disease. Br J Pharmacol. (2012) 165(3):659–69. doi: 10.1111/j.1476-5381.2011.01370.x
32. Mahabadi AA, Berg MH, Lehmann N, Kälsch H, Bauer M, Kara K, et al. Association of epicardial fat with cardiovascular risk factors and incident myocardial infarction in the general population: the Heinz Nixdorf Recall Study. J Am Coll Cardiol. (2013) 61(13):1388–95. doi: 10.1016/j.jacc.2012.11.062
33. Possner M, Liga R, Gaisl T, Vontobel J, Clerc OF, Mikulicic F, et al. Quantification of epicardial and intrathoracic fat volume does not provide an added prognostic value as an adjunct to coronary artery calcium score and myocardial perfusion single-photon emission computed tomography. Eur Heart J Cardiovasc Imaging. (2016) 17(8):885–91. doi: 10.1093/ehjci/jev209
34. Adams DB, Narayan O, Munnur RK, Cameron JD, Wong DT, Talman AH, et al. Ethnic differences in coronary plaque and epicardial fat volume quantified using computed tomography. Int J Cardiovasc Imaging. (2017) 33(2):241–9. doi: 10.1007/s10554-016-0982-1
35. Tanami Y, Jinzaki M, Kishi S, Matheson M, Vavere AL, Rochitte CE, et al. Lack of association between epicardial fat volume and extent of coronary artery calcification, severity of coronary artery disease, or presence of myocardial perfusion abnormalities in a diverse, symptomatic patient population: results from the CORE320 multicenter study. Circ Cardiovasc Imaging. (2015) 8(3):e002676. doi: 10.1161/CIRCIMAGING.114.002676
36. Raggi P, Gadiyaram V, Zhang C, Chen Z, Lopaschuk G, Stillman AE, et al. Statins reduce epicardial adipose tissue attenuation independent of lipid lowering: a potential pleiotropic effect. J Am Heart Assoc. (2019) 8(12):e013104. doi: 10.1161/JAHA.119.013104
37. Franssens BT, Nathoe HM, Visseren FL, Graaf Y, Leiner T, Group SS, et al. Relation of epicardial adipose tissue radiodensity to coronary artery calcium on cardiac computed tomography in patients at high risk for cardiovascular disease. Am J Cardiol. (2017) 119(9):1359–65. doi: 10.1016/j.amjcard.2017.01.031
Keywords: EFV, coronary artery calcium, non-contrast CT, myocardial perfusion imaging, major adverse cardiovascular events (MACEs)
Citation: Yang L, Yu W, Wan P, Wang J, Shao X, Zhang F, Yang X, Chen Y, Li Q, Jiang D, Wang Y, Jiang Q, Wang J and Wang Y (2023) Epicardial fat volume, an independent risk factor for major adverse cardiovascular events, had an incremental prognostic value to myocardial perfusion imaging in Chinese populations with suspected or known coronary artery disease with a normal left ventricular ejection fraction. Front. Cardiovasc. Med. 10:1261215. doi: 10.3389/fcvm.2023.1261215
Received: 19 July 2023; Accepted: 8 September 2023;
Published: 2 October 2023.
Edited by:
Jonathan Vigne, Centre Hospitalier Universitaire de Caen, FranceReviewed by:
Bibhu Mohanty, University of South Florida, United StatesFabian Islas, Complutense University of Madrid, Spain
© 2023 Yang, Yu, Wan, Wang, Shao, Zhang, Yang, Chen, Li, Jiang, Wang, Jiang, Wang and Wang. This is an open-access article distributed under the terms of the Creative Commons Attribution License (CC BY). The use, distribution or reproduction in other forums is permitted, provided the original author(s) and the copyright owner(s) are credited and that the original publication in this journal is cited, in accordance with accepted academic practice. No use, distribution or reproduction is permitted which does not comply with these terms.
*Correspondence: Yuetao Wang eXVldGFvLXdAMTYzLmNvbQ== Jianfeng Wang d2pmODQwNjIzQDE2My5jb20=
†These authors share first authorship
‡ORCID Yuetao Wang orcid.org/0000-0003-2859-8625