- 1Department of Cardiology, New Cross Hospital, The Royal Wolverhampton NHS Trust, Wolverhampton, United Kingdom
- 2The Institute of Cardiovascular Sciences, College of Medical and Dental Sciences, University of Birmingham, Edgbaston, Birmingham, United Kingdom
- 3Department of Cardiology, New Cross Hospital, Wolverhampton, United Kingdom
- 4Department of Molecular and Clinical Medicine, Faculty of Science and Engineering, Research Institute of Healthcare Science, University of Wolverhampton, Wolverhampton, United Kingdom
Coronary physiological measurements have transformed the treatment of coronary artery disease (CAD), with increasing evidence supporting the use of pressure wire guided revascularisation. Advances in microvascular assessment have enabled clinicians to discern angina aetiology even in patients without obstructive epicardial coronary artery disease, paving the way for more effective tailored therapy. In this article, the authors will examine pressure wire indices, their role in influencing clinical outcomes and future directions.
Introduction
Currently more than ten different modalities exist for the assessment of coronary physiology. Despite the wealth of evidence and technology available, however, most lesions are still treated based on visual assessment. In a survey from 2014, 71% of cases were managed following angiography alone whereas fractional flow reserve (FFR) was utilised in 21% (1). Despite changes in guidelines, seven years from the 2014 survey, functional assessment increased to merely 31% (2). In this review we will examine the different physiological assessment modalities, their impact on planning and decision making in contemporary percutaneous coronary intervention (PCI and their impact on clinical outcomes. In addition we discuss new developments and future directions in this important area.
Modalities of coronary physiology
Pressure wire coronary physiology
Hyperaemic pressure ratio
Fractional flow reserve (FFR) is the most well-known and established method for the assessment of coronary physiology; it is considered widely to be the gold standard. FFR is defined as the mean ratio of distal coronary pressure to aortic pressure during maximal hyperaemia (typically induced by adenosine see Figure 1). In essence, FFR represents the percentage by which an epicardial coronary stenosis impairs myocardial flow.
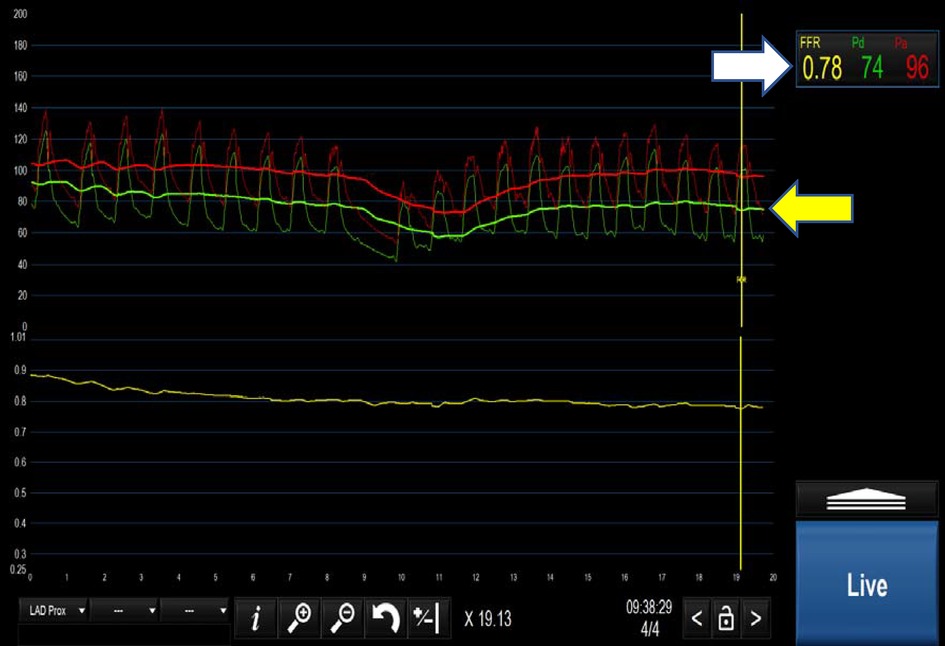
Figure 1. Positive FFR <0.80 (indicated by white arrow). Yellow arrow demonstrates the separation of the distal coronary pressure (green waveform) from the aortic pressure (red waveform) during hyperaemia induced by intravenous adenosine.
The assessment of multiple lesions in one artery, or simply tandem lesions is complex, and many pressure wire systems try to address this difficult area by allowing for a “pull back” recording, either during maximal hyperaemia or during measurement of the instantaneous free wave ratio (IFR). The interaction of the impact of tandem lesions of the fluid dynamics within the artery do make these assessments somewhat difficult to interpret and caution is usually applied to the results in clinical practice.
The clinical significance of FFR was initially validated against non-invasive tests in 1996 and a binary “cut-off” value of 0.75 (17) was postulated. Later, the landmark FAME I trial demonstrated clear superiority of FFR-guided PCI (using an FFR threshold of <0.80, to include a 0.05 “grey area”) compared to angiography-guided PCI in the composite end-points of death, myocardial infarction (MI) and repeat revascularisation at 1-year for patients with stable angina (18). It was also more cost effective without prolonging the procedure time (19). At 5-year follow up, this difference persisted, although it was no longer statically significant (20). Subsequently, the FAME II trial demonstrated FFR-guided PCI was superior to medical therapy, although outcomes were primarily driven by urgent revascularisation rather than death or MI (21, 22). This was further confirmed after 5 years of follow-up (23).
There is growing evidence for utilising FFR in patients with acute coronary syndrome (ACS). Several studies have demonstrated FFR can accurately determine the functional significance of non-culprit coronary lesions in ACS patients with improved clinical outcomes (24–27) In the COMPARE-Acute and DANAMI-3-PRIMULTI trials, patients with FFR-guided complete revascularisation had significantly reduced composite end-outcomes, primarily driven by reduced repeat revascularisation (26, 27). In patients with STEMI and multi vessel disease, the functional assessment of non cultprit lesions in the context of a STEMI has been questioned due to the microvasculature status in remote myocardial territories, which could effect the reliability of iFR and FFR (28). As mentioned above, numerous trials like the DANAMI-3-PRIMULTI anCOMPARE-Acute trials addressed this question and generally favored the use of coronary physiology in revascularization of the non culprit vessel (26, 27). Furthermore, in the COMPARE-Acute trial, the functional assessment was performed during the primary pci, whereas in the DINAMI-3-PRIMULTI, it was performed as a staged procedure prior to discharge. Interstingly, both demonstated the superiority of FFR-guided complete revascularization. The question then arises weather these patients should have hyperaemic or non hypeaemic assessment. The WAVE study compared iFR with FFR assessment of non culprit arteries during primary pci and demonstrated similar diagnostic yields (29).
More recently, the COMPLETE trial demonstrated significant reduction in MI and cardiovascular death with complete revascularisation in STEMI patients compared to culprit-only treatment based on angiography alone, rather than physiology (30, 31). Similarly, the FLOWER-MI trial found no difference between FFR-guided complete revascularisation compared to an angiography-guided strategy. However, this trial was under-powered due to low event rates and had wide confidence intervals for the hazard ratio of the primary endpoint (31). It is worth noting that FFR is a measurement of pressure and a surrogate for flow based on experimental conditions (30), which may contribute to suboptimal results of FFR in the post-MI setting.
A subset of patients with FFR negative lesions may be harbouring vulnerable plaques at high risk of future events [for example due to the presence of thin cap fibroatheromas (TCFA)]. This insight primarily comes from two observational studies: COMBINE OCT-FFR (32) (examining diabetic patients with predominantly stable coronary artery disease) and PECTUS-Obs (33) (examining MI patients). These studies demonstrate that up to a quarter of FFR negative lesions can be classified as having TCFA, which is associated with significantly increased major adverse clinical events. However, there is currently a lack of randomized control trials inestigating the clinical effectiveness of routine evaluation of vulnerable plaques.
Non-hyperaemic pressure ratios (NHPR)
The major perceived drawback of the hyperaemic pressure ratio method is the need to administer intravenous adenosine, or other hyparaemic agents. Adenosine causes microvascular hyperaemia to maintain constant microvascular resistance. In this state, the pressure across an epicardial lesion is proportional to the flow, without the influence of any microvascular dysfunction. Despite its very short half-life, adenosine is often not well tolerated. In order to avoid this problem, the concept of the measurement of non-hyperaemic pressure ratios (NHPR) was developed. These measurements derive from the ratio of distal coronary pressure (Pd) to aortic pressure (Pa) over periods in the cardiac cycle where microvascular resistance is in a steady-state or at the lowest value. A number of indices have been proposed which include measurement of mean Pd/Pa in a specific point in diastole (instantaneous wave-free period), over the entire diastolic period (diastolic hyperaemia-free ratio), or as a single lowest value over the entire cardiac cycle (resting full-cycle ratio).
Instantaneous wave-free ratio (iFR) is the first and the most-well established NHPR and was first introduced in 2012 (Figure 2) (34). The concept of iFR has been further validated against FFR (34, 35) and a cut-off of 0.89 was determined to be closely matched to the FFR threshold of 0.80 (35). Subsequently, two large randomised controlled trials (see Table 1) compared iFR and FFR with clinical outcomes as end-points and showed iFR-guided PCI was non-inferior to FFR-guided PCI (51, 52). In response, the 2018 European Society of Cardiology (ESC) guidelines on myocardial revascularisation were updated with a class 1a recommendation for both FFR and iFR in the assessment of intermediate grade stenoses when evidence of ischaemia is not available (53). Interestingly, although around 20% of cases showed discrepancy between FFR and iFR for borderline lesions at the respective thresholds of 0.8 and 0.89, this did not translate to difference in clinical outcomes (54). Any trial looking to definitively address this question was estimated to require 290,000 patients (55). In our view, when conducting invasive physiological assessment, there is no convincing difference between iFR and FFR: using either method is superior to angiography alone for the assessment of intermediate lesions.
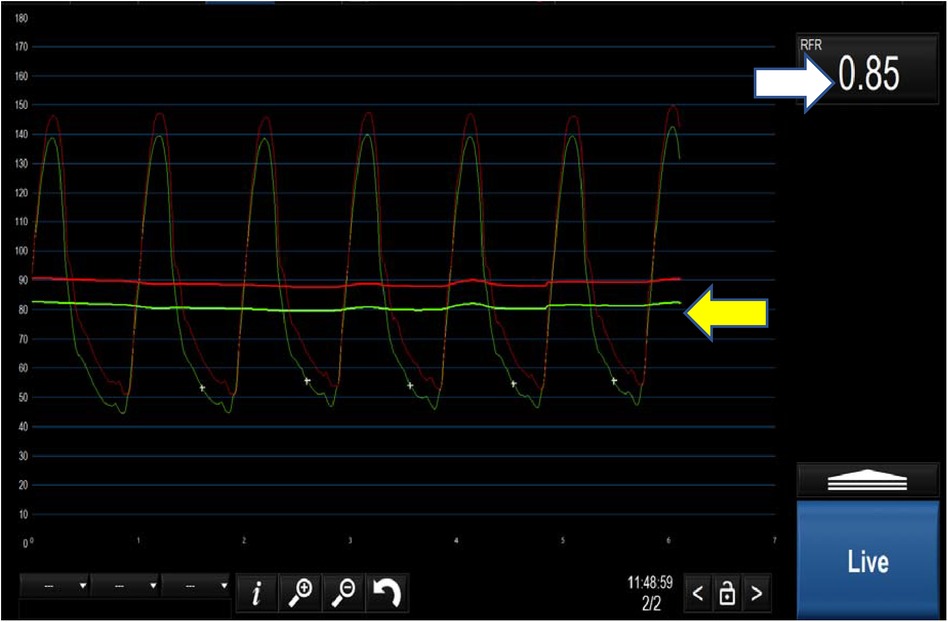
Figure 2. Positive iFR <0.89 (white arrow). The yellow arrow demonstrates the separation of the distal coronary pressure (green waveform) from the aortic pressure (red waveform) at rest.
Both hyperaemic and non hyperaemic pressure ratios can be used in the post PCI setting but this is not routinely practiced. The evidence in this arena is rather equivocal. Although trials like the REPEAT-FFR showed that suboptimal physiological outcome (FFR < 0.90) was associated with a higher incidence of MACE at 1 year, other trials like FFR-SEARCH proved that post PCI FFR did not correlate with clinical events at 30 days (56, 57). However, the follow up period in FFR-SEARCH was considered too short and typically, clinically significant ISR would take months to manifest.
Other NHPR
Since the introduction of iFR, other NHPR have become available including Diastolic hyperaemia-Free Ratio (DFR) (Boston Scientific, MA) and Resting Full-cycle Ratio (RFR) (Abbott, IL). These are all proprietary and can only be used with the hardware and software provided by the vendors. They also have limited validation data and no randomised controlled trial (RCT) data to evaluate the clinical outcomes compared to established PCI strategies (see Table 1). They, however, generally do correspond well to iFR (58) with the VALIDATE-RFR retrospective study of 651 iFR waveforms finding that RFR correlated highly with iFR (R2 = 0.985). Statistical equivalence testing within a 1% margin of error confirmed RFR and iFR were diagnostically equivalent (mean difference −0.002, 95% CI: = 0.009–0.006, p = 0.03) (59). It is widely accepted in the interventional cardiology community that these NHPRs are a reasonable substitute for FFR and iFR in most cases.
Angiography-derived FFR
Given the success of CT derived FFR, there is increasing interest in the development of novel non-invasive methods to assess FFR. Currently, 3 technologies are commercially available to assess FFR from coronary angiography alone: QFR (Medis Medical imaging systems, NE), FFRangio (Cathworks IS), vFFR (Pie Medical, NE).
Quantitative flow ratio (QFR) has the most clinical data including large multi-centre trials and one RCT (60–63) (see Table 1). In the recent FAVOR III trial, QFR-guided PCI had significantly reduced 1-year MACE compared to angiography-guided PCI driven primarily by reduced MI and repeat revascularisation (63). There are less data for FFRangio and vFFR: FFRangio was recently validated in the prospective FAST-FFR study (64) whilst vFFR was validated retrospectively in a cohort study (65) (see Table 1). In a systematic review and Bayesian meta-analysis, angiography-derived FFR compared well to pressure wire FFR and no difference was demonstrated between online and off-line methods of analysis (66). One of the main advantages of angiography-derived FFR is the ability for rapid online and offline analysis. In the FAVOR II study, the median time for QFR computation was only 5 min, compared to 7 min for pressure wire FFR (62). Further randomised control trials are required before angiography-derived FFR can be considered as an alternative to pressure wire or CT-derived FFR.
Computer tomography (CT) derived FFR
Most interventional cardiologists are familiar with the pressure wire study of coronary physiological indices such as FFR and instantaneous wave-free ratio (iFR). More recently, developments in computerised tomography (CT) have allowed the non-invasive estimation of FFR using fluid dynamics modelling. The most well-known and widely used CT derived FFR, i.e., FFRCT (Heartflow, CA), is based on 3-dimensional coronary artery reconstruction and fluid dynamics. FFRCT has been validated against and shown to be comparable to pressure wire FFR in three large prospective trials (3–5) (see Table 1) and further confirmed in a recent meta-analysis (6). In the PLATFORM and FORECAST trials, FFRCT was shown to be feasible, safe and associated with lower rate of invasive coronary angiography with normal coronary arteries (7, 8). Furthermore, a negative FFRCT (≥0.80) result is associated with good clinical outcomes at 12 months (9, 10) and 4.7 years from the follow up data of the NXT trial (11). This is currently being further evaluated in the ongoing randomised controlled PRECISE trial (NCT03702244). More recently, in the SYNTAX II trial, FFRCT was demonstrated to be feasible in three vessel coronary disease and provided comparable results to pressure wire FFR (12). Based on these finding, SYNTAX III trial showed planning of revascularisation for patients with left main stem or three-vessel coronary disease based on CT and FFRCT was feasible and correlated highly with decisions made from invasive coronary angiography (13). Growing evidence supporting FFRCT for the evaluation and diagnosis of patients with chest pain is reflected in the guidelines. The National Institute for Health and Care Excellence (NICE) issued initial guidance for FFRCT in 2017 as the most cost-effective recommended option, and this was updated in 2021 with expected savings of £9.4 million through avoidance of invasive tests and treatments (14).
In the American Heart Association/American College of Cardiology (AHA/ACC) guideline updated in 2021, CT coronary angiography (CTCA) is the recommended investigation for intermediate risk patients (1a recommendation) and FFRCT is recommended for those with coronary stenosis of 40%–90% (2a recommendation) (15). A low risk anatomy is defined as either normal or stentosis <30%, for which FFRCT is not required and optimal medical therpapy (OMT) would suffice as treatment. A high risk anatomy is defined as left main stem (LMS) disease of >50% stenosis, >70% stenosis in the LAD or three vessel stenosis for all of which and invasic coronary angiogram would be indicated, hence FFRCT may be unnecessary. However, in this cohort, FFRCT may be useful in patients with three vessel disease who are not fit for surgery and may benefit from PCI. This would also be applicable in patients with significant (>70%) single vessel disease and would aid in deciding between OMT and PCI. As mentioned above as well as reflective of the current guidelines, FFRCT is most uself in the intermediate risk category (30%–69% stenosis) as it would help determine OMT vs. invasive coronary assessment and PCI.
A recent multicentre audit of clinical data with cost analysis of the use and efficacy of FFRCT suggested that it had a low positive predictive value and costs £2,102 per patient compared with an average of £1,411 for stress imaging, making its use more expensive than conventional stress imaging modalities (16).
There are also other important limitations to consider for FFRCT. Sub-optimal imaging is a major limiting factor and often caused by factors such as breathing, fast or irregular heart rate. Even with improvement in technology, extensive coronary calcification remains a significant challenge. It is well known that coronary CT angiography (CTA) has low specificity and limited accuracy in the setting of increased calcification owing to calcium blooming and overestimation of luminal stenosis. FFRCT values can potentially be affected as a result of calcium compromising the identification of vessel boundaries for modeling. Interstingly in a subset anlaysis of the NXT study and other machine learning techniques have demonstrated that discrimination of lesion spepcifc ischaemia as well as diagnostic accuracy and specificity of FFRCT were higher at every level of calcium when compared to CTA alone (5). Hence, it may be valuable to perform FFRCT in patients with extensive calcification particularly in planning revascularization of complex calcified lesions. FFRCT lacks validation in revascularized vessels, microvascular dysfunction, spontaneous coronary artery dissection (SCAD), acute plaque rupture, coronary artery bypass grafts and congenital heart disease including coronary anomalies. Lastly, FFRCT can only be performed in a central core lab (Heartflow, Redwood City, CA) which necessitates transfer of patient data and delay in analysis.
Other methods of CT derived FFR have been developed which allow real-time on-site analysis. Taking into account the limited available evidence, they all have acceptable accuracy, but they are not widely or commercially available currently.
Microvascular disease & physiology
Many patients with stable angina undergoing coronary angiography are found to have myocardial ischaemia with non-obstructive coronary arteries (INOCA), the most common cause of which is coronary microvascular dysfunction (CMD).
The coronary microcirculation is a complex and structured system of small vessels (calibre <400 μm) which adapt their function in order to sustain the myocardium's physiological demands (67). Increasing evidence over the last few decades has shown that structural and functional abnormalities of the coronary microvasculature are highly prevalent and are associated with adverse clinical outcomes (68, 69).
Coronary Vasomotion Disorders International Study Group (COVADIS) formulated criteria (70) for the diagnosis of microvascular angina including:
(1) Angina secondary to myocardial ischemia
(2) Invasive or non-invasive evidence of unobstructed coronary arteries (<50% diameter reduction or FFR < 0.8)
(3) Objective evidence of myocardial ischemia (ETT, stress perfusion scan or RWMA on echo)
(4) Evidence of coronary microvascular function
Microvascular vasospasm fulfils the COVADIS criteria if the vasoreactivity test reproduces the usual anginal symptoms associated with ischemic ECG changes in the absence of significant epicardial spasm (67, 70).
The pathophysiology of CMD entails enhanced microvascular coronary vasoconstrictive reactivity, increased coronary microvascular resistance and impaired vasodilator capacity (68). Various pathophysiological mechanisms have been proposed. Impaired endothelium-dependent vasodilation affects the arterioles as a consequence of progressive endothelial dysfunction which leads to a reduction of nitric oxide production and release, hence resulting in insufficient nitric oxide mediated vasodilation (67). This is thought to be a consequence of loss of balance between myosin light chain kinase and phosphatase activity resulting in excess vascular smooth muscle contraction (71). Additionally, other factors like endothelial dysfunction, vascular smooth muscle hyper-reactivity, triggers like inflammation, oxidative stress and genetic factors are considered likely culprits of epicardial as well as microvascular spasm (67). Prognosis could be worse if there is associated unequivocal myocardial ischemia, and quality of life may be impaired due to recurrent angina, hospitalisation, and coronary angiography (72). A majority of patients with unobstructed coronary arteries have microvascular coronary abnormalities which can be safely investigated during coronary angiography. Invasive angiography can consequently provide vital diagnostic information using physiologic indices that can influence treatment and outcome (73).
The Coronary Microvascular Angina (CorMicA) trial assessed whether an interventional diagnostic procedure (IDP) linked to stratified medical therapy improved outcomes and quality of life in patients with INOCA (74). IDP entailed assessment of coronary vascular function and included guide wire-based assessment of coronary flow reserve (CFR), index of microcirculatory resistance (IMR), FFR and vasoreactivity (microvascular spasm) testing with acetylcholine. The authors conducted a randomised controlled blind clinical trial in which patients with INOCA were randomised to the intervention group or the control group (74). The primary endpoint was the mean difference in the severity of angina at six months which was assessed by the Seattle Angina Questionnaire summary score (74). CorMica ultimately confirmed that standard coronary angiography often failed to identify patients with microvascular disease (74). Conversely, IDP supplemented with optimal medical therapy improved angina in patients with INOCA without any major differences in major adverse cardiac events at six months (74).
Microvascular assessment (CFR & IMR)
Coronary flow reserve and index of microcirculatory resistance are invasively calculated from two temperature sensors located on the proximal and distal portions of the intracoronary pressure wire, using thermodilution (75). Other non-invasive modalities such as transthoracic doppler echo, positron emission tomography and stress cardiac magnetic resonance (see Table 1) can also be used to determine CFR (67). The thermodilution method has, however, been proven to correlate well with true microcirculatory flow and resistance, and hence produces accurate and reliable CFR and IMR assessment (76).
The catheter is firstly flushed with 5 ml saline before a volume of 3 ml of ambient temperature saline is rapidly injected into the coronary artery. As it passes the proximal and distal sensors of the pressure wire, temperature changes are detected. The time interval of the passage of saline between the proximal and distal sensor is used to determine the coronary flow [mean transit time (Tmn)]. This process is done at rest as well as on induced hyperaemia.
Hyperaemia is induced with an intravenous infusion of adenosine at a dose of 140 mg/kg/min (77). Adenosine-mediated coronary microvascular tone and reactive hyperaemia occur through activation of adenosine receptors (especially A2AR) on endothelial as well as smooth muscle cells which results in coronary vasodilation (77). Other agents like intracoronary papaverine can be used at a dose of 12–16 mg for the left coronary artery and 8–12 mg for the right coronary artery. Intracoronary papaverine was previously the gold standard, but concerns have been raised due to its potential adverse effects of QTc prolongation and ventricular arrhythmias (78). Furthermore, the duration of action of papaverine is too short for steady state hyperaemic pressure recordings in comparison to intravenous adenosine which produces excellent steady state phasic intracoronary pressure recordings within one minute of initiation (78, 79). Adenosine is therefore the more ideal vasodilator of choice in epicardial and microvascular coronary physiology assessment.
CFR is calculated by dividing the rest Tmn by the hyperaemic Tmn. A CFR < 2.5 indicates impaired flow, at either the coronary or microvascular level. Based on Ohm's law, vascular resistance (R) is the pressure difference across the myocardium (ΔP = Pd-Pv) divided by the coronary flow rate (Q) which is inversely related to the Tmn (1/Tmn). Therefore R can be calculated as (Pd-Pv) × Tmn. Index of coronary microvascular resistance (IMR) is calculated with thermodilution as the product of the distal coronary pressures (Pd) and the Tmn during maximal hyperaemia (67). An IMR > 25 indicates high microvascular resistance and, indirectly, coronary microvascular dysfunction. Importantly, this measure is independent of the degree of coronary obstruction (67). A combination of a CFR < 2.5 and an IMR > 25 is diagnostic of microvascular dysfunction in patients with INOCA (80) (Table 2 and Figure 4). ESC guidelines on the management of chronic coronary syndromes (2019) recommend CFR and/or IMR should be considered in patients with persistent symptoms but have coronary arteries that are either angiographically normal or have moderate stenosis with preserved iFR and FFR (class 2a, level of evidence B). Similarly, the AHA/ACC guidelines for the evaluation and diagnosis of chest pain (2021) recommend consideration of invasive microvascular assessment in patients with persistent stable chest pain and INOCA (15) (class 2a, level of evidence B).
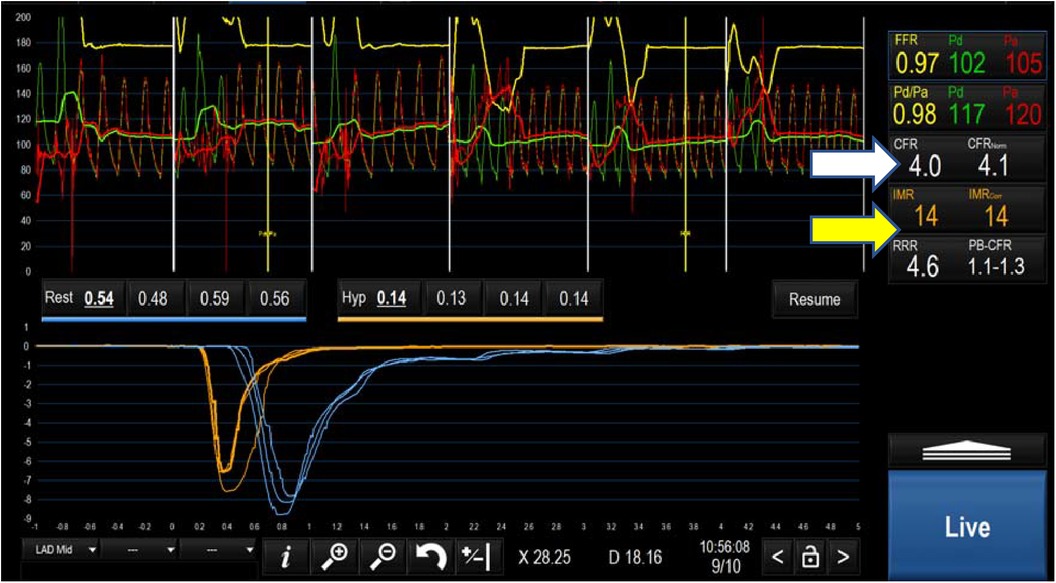
Figure 3. Negative microvascular study demonstrating a CFR of 4 (white arrow) and an IMR of 14 (yellow arrow) ruling out microvascular dysfunction.
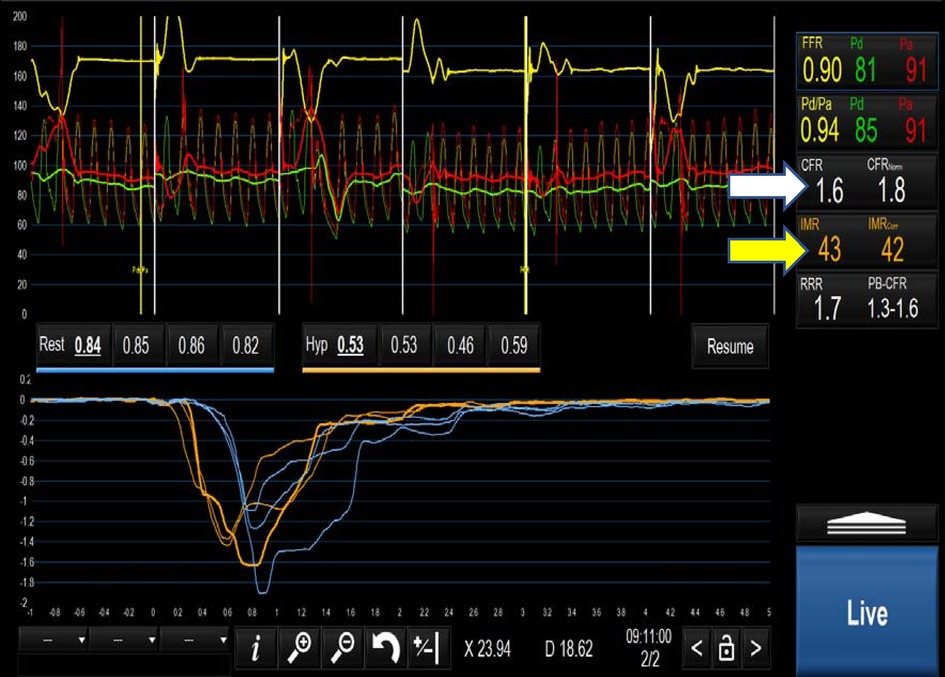
Figure 4. Positive microvascular study demonstrating a CFR of 1.6(white arrow) and an IMR of 43 (yellow arrow) fulfilling the criteria for MVD i.e. CFR < 2.5 and IMR > 25.
Vasoreactivity testing
When microvascular spasm is suspected, usually a provocation test is performed by administering intracoronary acetylcholine (Ach) (67, 81). High doses of intracoronary acetylcholine are administered, acting on both the epicardial coronary arteries as well as the coronary microvasculature, which unmasks any underlying vasomotor abnormalities hence resulting in vasoconstriction rather than vasodilation (67, 71). As per the COVADIS criteria, a positive microvascular vasoreactivity test would reproduce ischemic symptoms as well as ECG changes in the absence of significant epicardial spasm (<90% coronary diameter reduction) (70). If however, there is >90% coronary diameter reduction, a diagnosis of coronary vasospasm is evident.
Acetylcholine 20–100 mcg is usually administered in a stepwise manner into the left coronary artery (LCA) and 20–50 mcg into the right coronary artery (RCA) over a period of 20 s with a 3–5 min interval between each injection. In view of the potential adverse consequences of acetylcholine, such as ST elevation myocardial infarction, chest pain, bradyarrhythmias and ventricular tachycardia and fibrillation (82, 83), this test is not universally offered and our centre does not routinely practice vasoreactivity testing. Consideration should be given to transferring the patient for assessment at a specialist unit. Other agents that can be used are ergonovine (ER), neuropeptide Y and dopamine. However the vast majority of data supports the clinical use of predominantly Ach as well as ER (71).
Treatment strategies
The treatment of microvascular dysfunction is dependent on the cause. If the aetiology is impaired microcirculatory conductance, (CFR < 2.5, IMR > 2.5), empirical anti-anginal therapy is recommended acknowledging limited trial data (Figure 5). Current European Society of Cardiology (ESC) guidelines recommend beta-blockers as the first line therapy in addition to an ACE-inhibitor and statin. Weight loss and lifestyle changes should also be recommended. Short acting nitrate agents may help to relieve symptoms during an attack and if effective, adjunctive long-acting nitrates may also be given. In cases of microvascular dysfunction caused by a microvascular vasomotor disorder, calcium channel blockers and long-acting nitrates are the preferred treatment (84).
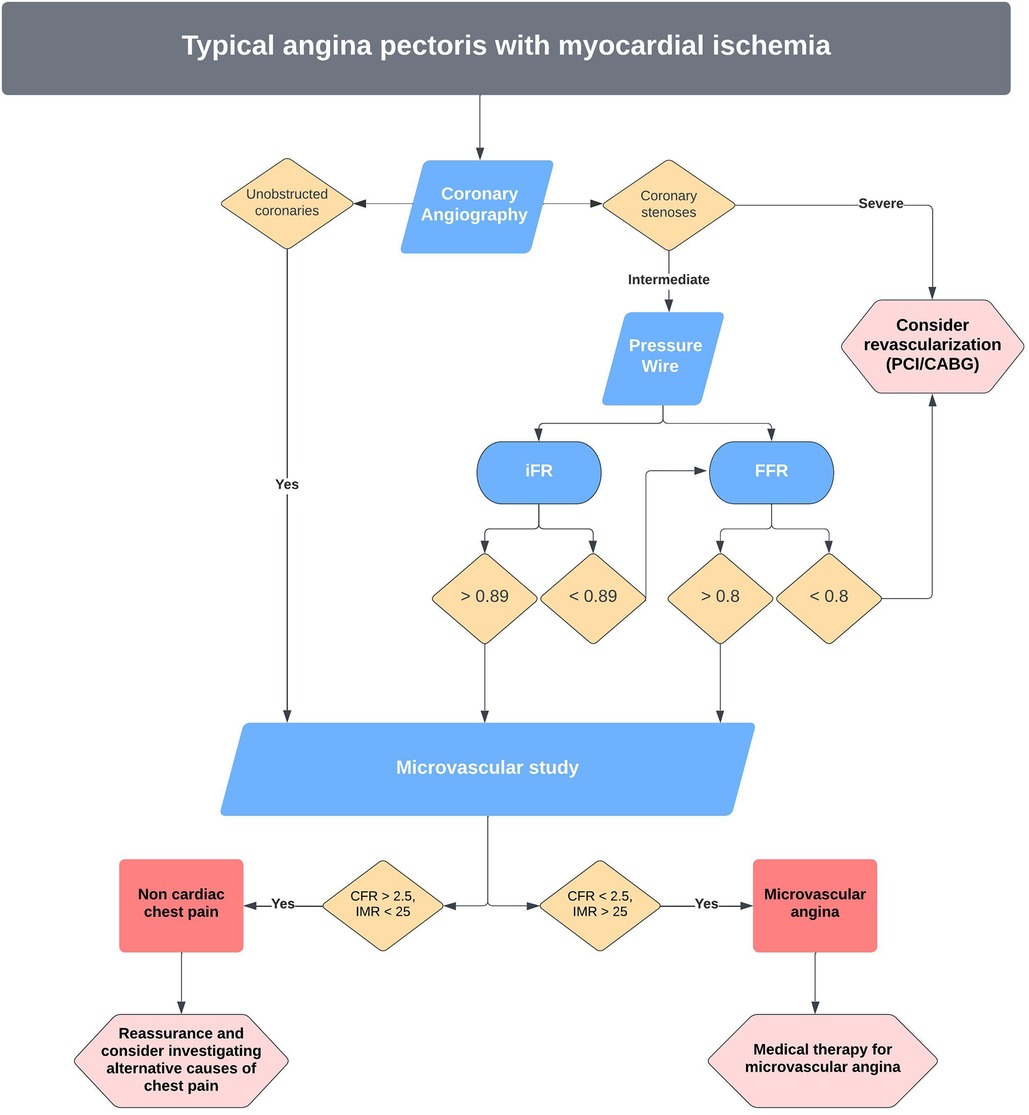
Figure 5. Proposed pathway for invasive management of angina and microvascular dysfunction at our centre.
Conclusion
Invasive coronary physiology assessment is proving to be a valuable adjunct to coronary angiography in aiding the diagnosis of physiologically significant epicardial and microvascular coronary artery disease. While FFR (and increasingly NHPR) assessment of intermediate epicardial stenoses has become widely adopted, routine coronary physiology evaluation in INOCA patients is not commonplace, despite the low risk and straightforward methodology. This is partly due to the additional training, procedural time and cost involved. However, these disadvantages are likely to be offset by the ability to offer definitive diagnoses and appropriate treatments, thereby reducing recurrent hospital admissions and repeated investigations.
Author contributions
ZA: Resources, Visualization, Writing – original draft, Writing – review & editing. HS: Writing – review & editing. LF: Resources, Supervision, Writing – original draft, Writing – review & editing. JC: Resources, Supervision, Writing – review & editing.
Conflict of interest
The authors declare that the research was conducted in the absence of any commercial or financial relationships that could be construed as a potential conflict of interest.
Publisher's note
All claims expressed in this article are solely those of the authors and do not necessarily represent those of their affiliated organizations, or those of the publisher, the editors and the reviewers. Any product that may be evaluated in this article, or claim that may be made by its manufacturer, is not guaranteed or endorsed by the publisher.
References
1. Toth GG, Toth B, Johnson NP, De Vroey F, Di Serafino L, Pyxaras S, et al. Revascularization decisions in patients with stable angina and intermediate lesions: results of the international survey on interventional strategy. Circ Cardiovasc Interv. (2014) 7(6):751–9. doi: 10.1161/CIRCINTERVENTIONS.114.001608
2. Toth GG, Johnson NP, Wijns W, Toth B, Achim A, Fournier S, et al. Revascularization decisions in patients with chronic coronary syndromes: results of the second International Survey on Interventional Strategy (ISIS-2). Int J Cardiol. (2021) 336:38–44. doi: 10.1016/J.IJCARD.2021.05.005
3. Koo BK, Erglis A, Doh JH, Daniels DV, Jegere S, Kim HS, et al. Diagnosis of ischemia-causing coronary stenoses by noninvasive fractional flow reserve computed from coronary computed tomographic angiograms. Results from the prospective multicenter DISCOVER-FLOW (Diagnosis of Ischemia-Causing Stenoses Obtained Via Noninvasive Fractional Flow Reserve) study. J Am Coll Cardiol. (2011) 58(19):1989–97. doi: 10.1016/J.JACC.2011.06.066
4. Min JK, Leipsic J, Pencina MJ, Berman DS, Koo BK, van Mieghem C, et al. Diagnostic accuracy of fractional flow reserve from anatomic CT angiography. JAMA. (2012) 308(12):1237–45. doi: 10.1001/2012.JAMA.11274
5. Nørgaard BL, Leipsic J, Gaur S, Seneviratne S, Ko BS, Ito H, et al. Diagnostic performance of noninvasive fractional flow reserve derived from coronary computed tomography angiography in suspected coronary artery disease: the NXT trial (Analysis of Coronary Blood Flow Using CT Angiography: next Steps). J Am Coll Cardiol. (2014) 63(12):1145–55. doi: 10.1016/J.JACC.2013.11.043
6. Agasthi P, Kanmanthareddy A, Khalil C, Egbuche O, Yarlagadda V, Sachdeva R, et al. Comparison of computed tomography derived fractional flow reserve to invasive fractional flow reserve in diagnosis of functional coronary stenosis: a meta-analysis. Sci Rep. (2018) 8(1):11535. doi: 10.1038/S41598-018-29910-9
7. Curzen N, Nicholas Z, Stuart B, Wilding S, Hill K, Shambrook J, et al. Fractional flow reserve derived from computed tomography coronary angiography in the assessment and management of stable chest pain: the FORECAST randomized trial. Eur Heart J. (2021) 42(37):3844–52. doi: 10.1093/EURHEARTJ/EHAB444
8. Douglas PS, Pontone G, Hlatky MA, Patel MR, Norgaard BL, Byrne RA, et al. Clinical outcomes of fractional flow reserve by computed tomographic angiography-guided diagnostic strategies vs. usual care in patients with suspected coronary artery disease: the prospective longitudinal trial of FFR(CT): outcome and resource impacts study. Eur Heart J. (2015) 36(47):3359–67. doi: 10.1093/EURHEARTJ/EHV444
9. Nørgaard BL, Gaur S, Fairbairn TA, Douglas PS, Jensen JM, Patel MR, et al. Prognostic value of coronary computed tomography angiographic derived fractional flow reserve: a systematic review and meta-analysis. Heart. (2022) 108(3):194–202. doi: 10.1136/HEARTJNL-2021-319773
10. Patel MR, Nørgaard BL, Fairbairn TA, Nieman K, Akasaka T, Berman DS, et al. 1-year impact on medical practice and clinical outcomes of FFRCT: the ADVANCE registry. JACC Cardiovasc Imaging. (2020) 13(1 Pt 1):97–105. doi: 10.1016/J.JCMG.2019.03.003
11. Ihdayhid AR, Norgaard BL, Gaur S, Leipsic J, Nerlekar N, Osawa K, et al. Prognostic value and risk continuum of noninvasive fractional flow reserve derived from coronary CT angiography. Radiology. (2019) 292(2):343–51. doi: 10.1148/RADIOL.2019182264
12. Collet C, Miyazaki Y, Ryan N, Asano T, Tenekecioglu E, Sonck J, et al. Fractional flow reserve derived from computed tomographic angiography in patients with multivessel CAD. J Am Coll Cardiol. (2018) 71(24):2756–69. doi: 10.1016/J.JACC.2018.02.053
13. Collet C, Onuma Y, Andreini D, Sonck J, Pompilio G, Mushtaq S, et al. Coronary computed tomography angiography for heart team decision-making in multivessel coronary artery disease. Eur Heart J. (2018) 39(41):3689–98. doi: 10.1093/EURHEARTJ/EHY581
14. Overview | HeartFlow FFRCT for estimating fractional flow reserve from coronary CT angiography | Guidance | NICE.
15. Gulati M, Levy PD, Mukherjee D, Amsterdam E, Bhatt DL, Birtcher KK, et al. 2021 AHA/ACC/ASE/CHEST/SAEM/SCCT/SCMR guideline for the evaluation and diagnosis of chest pain: executive summary: a report of the American College of Cardiology/American Heart Association Joint Committee on clinical practice guidelines. Circulation. (2021) 144(22):E336–67. doi: 10.1161/CIR.0000000000001030
16. Mittal TK, Hothi SS, Venugopal V, Taleyratne J, O’Brien D, Adnan K, et al. The use and efficacy of FFR-CT: real-world multicenter audit of clinical data with cost analysis. JACC Cardiovasc Imaging. Published online March 9. (2023) 16(8):1056–65. doi: 10.1016/J.JCMG.2023.02.005
17. Pijls NHJ, de Bruyne B, Peels K, van der Voort PH, Bonnier HJRM, Bartunek J, et al. Measurement of fractional flow reserve to assess the functional severity of coronary-artery stenoses. N Engl J Med. (1996) 334(26):1703–8. doi: 10.1056/NEJM199606273342604
18. Patricio L, Tonino PAL, De Bruyne B, Pijls NHJ, Siebert U, Ikeno F, et al. Fractional flow reserve versus angiography for guiding percutaneous coronary intervention. N Engl J Med. (2009) 360(3):229–30. doi: 10.1056/NEJMOA0807611
19. Fearon WF, Bornschein B, Tonino PAL, Gothe RM, De Bruyne B, Pijls NHJ, et al. Economic evaluation of fractional flow reserve-guided percutaneous coronary intervention in patients with multivessel disease. Circulation. (2010) 122(24):2545–50. doi: 10.1161/CIRCULATIONAHA.109.925396
20. Van Nunen LX, Zimmermann FM, Tonino PAL, Barbato E, Baumbach A, Engstrøm T, et al. Fractional flow reserve versus angiography for guidance of PCI in patients with multivessel coronary artery disease (FAME): 5-year follow-up of a randomised controlled trial. Lancet. (2015) 386(10006):1853–60. doi: 10.1016/S0140-6736(15)00057-4
21. De Bruyne B, Pijls NHJ, Kalesan B, Barbato E, Tonino PAL, Piroth Z, et al. Fractional flow reserve-guided PCI versus medical therapy in stable coronary disease. N Engl J Med. (2012) 367(11):991–1001. doi: 10.1056/NEJMOA1205361
22. De Bruyne B, Fearon WF, Pijls NHJ, Barbato E, Tonino P, Piroth Z, et al. Fractional flow reserve-guided PCI for stable coronary artery disease. N Engl J Med. (2014) 371(13):1208–17. doi: 10.1056/NEJMOA1408758
23. Xaplanteris P, Fournier S, Pijls NHJ, Fearon WF, Barbato E, Tonino PAL, et al. Five-year outcomes with PCI guided by fractional flow reserve. N Engl J Med. (2018) 379(3):250–9. doi: 10.1056/NEJMOA1803538
24. Sels JWEM, Tonino PAL, Siebert U, Fearon WF, Van’T Veer M, De Bruyne B, et al. Fractional flow reserve in unstable angina and non-ST-segment elevation myocardial infarction experience from the FAME (Fractional flow reserve versus Angiography for Multivessel Evaluation) study. JACC Cardiovasc Interv. (2011) 4(11):1183–9. doi: 10.1016/J.JCIN.2011.08.008
25. Ntalianis A, Sels JW, Davidavicius G, Tanaka N, Muller O, Trana C, et al. Fractional flow reserve for the assessment of nonculprit coronary artery stenoses in patients with acute myocardial infarction. JACC Cardiovasc Interv. (2010) 3(12):1274–81. doi: 10.1016/J.JCIN.2010.08.025
26. Smits PC, Abdel-Wahab M, Neumann FJ, Boxma-de Klerk BM, Lunde K, Schotborgh CE, et al. Fractional flow reserve-guided multivessel angioplasty in myocardial infarction. N Engl J Med. (2017) 376(13):1234–44. doi: 10.1056/NEJMOA1701067
27. Engstrøm T, Kelbæk H, Helqvist S, Høfsten DE, Kløvgaard L, Holmvang L, et al. Complete revascularisation versus treatment of the culprit lesion only in patients with ST-segment elevation myocardial infarction and multivessel disease (DANAMI-3—pRIMULTI): an open-label, randomised controlled trial. Lancet. (2015) 386(9994):665–71. doi: 10.1016/S0140-6736(15)60648-1
28. Scarsini R, Terentes-Printzios D, De Maria GL, Ribichini F, Banning A. Why, when and how should clinicians use physiology in patients with acute coronary syndromes? Interv Cardiol Rev. (2020) 15(1):e05. doi: 10.15420/ICR.2019.26
29. Musto C, De Felice F, Rigattieri S, Chin D, Marra A, Nazzaro MS, et al. Instantaneous wave-free ratio and fractional flow reserve for the assessment of nonculprit lesions during the index procedure in patients with ST-segment elevation myocardial infarction: the WAVE study. Am Heart J. (2017) 193:63–9. doi: 10.1016/J.AHJ.2017.07.017
30. Mehta SR, Wood DA, Storey RF, Mehran R, Bainey KR, Nguyen H, et al. Complete Revascularization with multivessel PCI for myocardial infarction. N Engl J Med. (2019) 381(15):1411–21. doi: 10.1056/NEJMOA1907775
31. Puymirat E, Cayla G, Simon T, Steg PG, Montalescot G, Durand-Zaleski I, et al. Multivessel PCI guided by FFR or angiography for myocardial infarction. N Engl J Med. (2021) 385(4):297–308. doi: 10.1056/NEJMOA2104650
32. Kedhi E, Berta B, Roleder T, Hermanides RS, Fabris E, IJsselmuiden AJJ, et al. Thin-cap fibroatheroma predicts clinical events in diabetic patients with normal fractional flow reserve: the COMBINE OCT-FFR trial. Eur Heart J. (2021) 42(45):4671–9. doi: 10.1093/EURHEARTJ/EHAB433
33. Mol JQ, Belkacemi A, Volleberg RHJA, Meuwissen M, Protopopov AV, Laanmets P, et al. Identification of anatomic risk factors for acute coronary events by optical coherence tomography in patients with myocardial infarction and residual nonflow limiting lesions: rationale and design of the PECTUS-obs study. BMJ Open. (2021) 11(7). doi: 10.1136/BMJOPEN-2021-048994
34. Sen S, Escaned J, Malik IS, Mikhail GW, Foale RA, Mila R, et al. Development and validation of a new adenosine-independent index of stenosis severity from coronary wave-intensity analysis: results of the ADVISE (ADenosine Vasodilator Independent Stenosis Evaluation) study. J Am Coll Cardiol. (2012) 59(15):1392–402. doi: 10.1016/J.JACC.2011.11.003
35. Petraco R, Escaned J, Sen S, Nijjer S, Asrress KN, Echavarria-Pinto M, et al. Classification performance of instantaneous wave-free ratio (iFR) and fractional flow reserve in a clinical population of intermediate coronary stenoses: results of the ADVISE registry. EuroIntervention. (2013) 9(1):91–101. doi: 10.4244/EIJV9I1A14
36. Danad I, Raijmakers PG, Driessen RS, Leipsic J, Raju R, Naoum C, et al. Comparison of coronary CT angiography, SPECT, PET, and hybrid imaging for diagnosis of ischemic heart disease determined by fractional flow reserve. JAMA Cardiol. (2017) 2(10):1100–7. doi: 10.1001/JAMACARDIO.2017.2471
37. Neglia D, Rovai D, Caselli C, Pietila M, Teresinska A, Aguadé-Bruix S, et al. Detection of significant coronary artery disease by noninvasive anatomical and functional imaging. Circ Cardiovasc Imaging. (2015) 8(3):e002179. doi: 10.1161/CIRCIMAGING.114.002179
38. Takx RA, Blomberg BA, El Aidi H, Habets J, de Jong PA, Nagel E, et al. Diagnostic accuracy of stress myocardial perfusion imaging compared to invasive coronary angiography with fractional flow reserve meta-analysis. Circ Cardiovasc Imaging. (2015) 8(1):e002666. doi: 10.1161/CIRCIMAGING.114.002666
39. Hussain ST, Paul M, Plein S, McCann GP, Shah AM, Marber MS, et al. Design and rationale of the MR-INFORM study: stress perfusion cardiovascular magnetic resonance imaging to guide the management of patients with stable coronary artery disease. J Cardiovasc Magn Reson. (2012) 14(1):65. doi: 10.1186/1532-429X-14-65
40. Ripley DP, Motwani M, Brown JM, Nixon J, Everett CC, Bijsterveld P, et al. Individual component analysis of the multi-parametric cardiovascular magnetic resonance protocol in the CE-MARC trial. J Cardiovasc Magn Reson. (2015) 17(1):59. doi: 10.1186/S12968-015-0169-2
41. Lin A, Van Diemen PA, Motwani M, McElhinney P, Otaki Y, Han D, et al. Machine learning from quantitative coronary computed tomography angiography predicts fractional flow reserve-defined ischemia and impaired myocardial blood flow. Circ Cardiovasc Imaging. (2022) 15(10):710–20. doi: 10.1161/CIRCIMAGING.122.014369
42. Bedetti G, Pasanisi EM, Tintori G, Fonseca L, Tresoldi S, Minneci C, et al. Stress echo in chest pain unit: the SPEED trial. Int J Cardiol. (2005) 102(3):461–7. doi: 10.1016/J.IJCARD.2004.05.058
43. Woodward W, Dockerill C, McCourt A, Upton R, O’Driscoll J, Balkhausen K, et al. Real-world performance and accuracy of stress echocardiography: the EVAREST observational multi-centre study. Eur Heart J Cardiovasc Imaging. (2022) 23(5):689–98. doi: 10.1093/EHJCI/JEAB092
44. Berry C, McClure JD, Oldroyd KG. Coronary revascularization guided by instantaneous wave-free ratio compared to fractional flow reserve: pooled 5-year mortality in the DEFINE-FLAIR and iFR-SWEDEHEART trials. Eur Heart J. (2023) 44(41):4388–90. doi: 10.1093/EURHEARTJ/EHAD552
45. Sen S, Asrress KN, Nijjer S, Petraco R, Malik IS, Foale RA, et al. Diagnostic classification of the instantaneous wave-free ratio is equivalent to fractional flow reserve and is not improved with adenosine administration. Results of CLARIFY (Classification Accuracy of Pressure-Only Ratios Against Indices Using Flow Study). J Am Coll Cardiol. (2013) 61(13):1409–20. doi: 10.1016/J.JACC.2013.01.034
46. Scoccia A, Byrne RA, Banning AP, Landmesser U, Van Belle E, Amat-Santos IJ, et al. Fractional flow reserve or 3D-quantitative-coronary-angiography based vessel-FFR guided revascularization. Rationale and study design of the prospective randomized fast III trial. Am Heart J. (2023) 260:1–8. doi: 10.1016/J.AHJ.2023.02.003
47. Andreini D, Takahashi K, Mushtaq S, Conte E, Modolo R, Sonck J, et al. Impact of coronary calcification assessed by coronary CT angiography on treatment decision in patients with three-vessel CAD: insights from SYNTAX III trial. Interact Cardiovasc Thorac Surg. (2022) 34(2):176–84. doi: 10.1093/ICVTS/IVAB249
48. Min JK, Berman DS, Budoff MJ, Jaffer FA, Leipsic J, Leon MB, et al. Rationale and design of the DeFACTO (Determination of Fractional Flow Reserve by Anatomic Computed Tomographic AngiOgraphy) study. J Cardiovasc Comput Tomogr. (2011) 5(5):301–9. doi: 10.1016/J.JCCT.2011.08.003
49. Ahn JM, Park DW, Shin ES, Koo BK, Nam CW, Doh JH, et al. Fractional Flow Reserve and Cardiac Events in Coronary Artery Disease: data From a Prospective IRIS-FFR Registry (Interventional Cardiology Research Incooperation Society Fractional Flow Reserve). Circulation. (2017) 135(23):2241–51. doi: 10.1161/CIRCULATIONAHA.116.024433
50. Zimmermann FM, Ferrara A, Johnson NP, Van Nunen LX, Escaned J, Albertsson P, et al. Deferral vs. performance of percutaneous coronary intervention of functionally non-significant coronary stenosis: 15-year follow-up of the DEFER trial. Eur Heart J. (2015) 36(45):3182–8. doi: 10.1093/EURHEARTJ/EHV452
51. Davies JE, Sen S, Dehbi HM, Al-Lamee R, Petraco R, Nijjer SS, et al. Use of the instantaneous wave-free ratio or fractional flow reserve in PCI. N Engl J Med. (2017) 376(19):1824–34. doi: 10.1056/NEJMOA1700445
52. Götberg M, Christiansen EH, Gudmundsdottir IJ, Sandhall L, Danielewicz M, Jakobsen L, et al. Instantaneous wave-free ratio versus fractional flow reserve to guide PCI. N Engl J Med. (2017) 376(19):1813–23. doi: 10.1056/NEJMOA1616540
53. Neumann FJ, Sousa-Uva M, Ahlsson A, Alfonso F, Banning AP, Benedetto U, et al. 2018 ESC/EACTS guidelines on myocardial revascularization. Eur Heart J. (2019) 40(2):87–165. doi: 10.1093/EURHEARTJ/EHY394
54. Lee JM, Rhee TM, Choi KH, Park J, Hwang D, Kim J, et al. Clinical outcome of lesions with discordant results among different invasive physiologic indices—resting distal coronary to aortic pressure ratio, resting full-cycle ratio, diastolic pressure ratio, instantaneous wave-free ratio, and fractional flow reserve. Circ J. (2019) 83(11):2210–21. doi: 10.1253/CIRCJ.CJ-19-0230
55. Seto AH. Instantaneous wave-free ratio outcomes and the epistemology of ischemia. JACC Cardiovasc Interv. (2017) 10(24):2511–3. doi: 10.1016/J.JCIN.2017.08.007
56. Impact of post-percutaneous coronary intervention fractional flow reserve measurement on procedural management and clinical outcomes: the REPEAT-FFR study—PubMed. Available at: https://pubmed.ncbi.nlm.nih.gov/31199348/ (Accessed October 10, 2023).
57. van Bommel RJ, Masdjedi K, Diletti R, Lemmert ME, van Zandvoort L, Wilschut J, et al. Routine fractional flow reserve measurement after percutaneous coronary intervention. Circ Cardiovasc Interv. (2019) 12(5):e007428. doi: 10.1161/CIRCINTERVENTIONS.118.007428
58. van’t Veer M, Pijls NHJ, Hennigan B, Watkins S, Ali ZA, De Bruyne B, et al. Comparison of different diastolic resting indexes to iFR: are they all equal? J Am Coll Cardiol. (2017) 70(25):3088–96. doi: 10.1016/J.JACC.2017.10.066
59. Svanerud J, Ahn JM, Jeremias A, Van ’T Veer M, Gore A, Maehara A, et al. Validation of a novel non-hyperaemic index of coronary artery stenosis severity: the resting full-cycle ratio (VALIDATE RFR) study. EuroIntervention. (2018) 14(7):806–14. doi: 10.4244/EIJ-D-18-00342
60. Tu S, Westra J, Yang J, von Birgelen C, Ferrara A, Pellicano M, et al. Diagnostic accuracy of fast computational approaches to derive fractional flow reserve from diagnostic coronary angiography: the international multicenter FAVOR pilot study. JACC Cardiovasc Interv. (2016) 9(19):2024–35. doi: 10.1016/J.JCIN.2016.07.013
61. Xu B, Tu S, Qiao S, Qu X, Chen Y, Yang J, et al. Diagnostic accuracy of angiography-based quantitative flow ratio measurements for online assessment of coronary stenosis. J Am Coll Cardiol. (2017) 70(25):3077–87. doi: 10.1016/J.JACC.2017.10.035
62. Westra J, Andersen BK, Campo G, Matsuo H, Koltowski L, Eftekhari A, et al. Diagnostic performance of in-procedure angiography-derived quantitative flow reserve compared to pressure-derived fractional flow reserve: the FAVOR II Europe-Japan study. J Am Heart Assoc. (2018) 7(14):e009603. doi: 10.1161/JAHA.118.009603
63. Xu B, Tu S, Song L, Jin Z, Yu B, Fu G, et al. Angiographic quantitative flow ratio-guided coronary intervention (FAVOR III China): a multicentre, randomised, sham-controlled trial. Lancet. (2021) 398(10317):2149–59. doi: 10.1016/S0140-6736(21)02248-0
64. Fearon WF, Achenbach S, Engstrom T, Assali A, Shlofmitz R, Jeremias A, et al. Accuracy of fractional flow reserve derived from coronary angiography. Circulation. (2019) 139(4):477–84. doi: 10.1161/CIRCULATIONAHA.118.037350
65. Masdjedi K, van Zandvoort LJC, Balbi MM, Gijsen FJH, Ligthart JMR, Rutten MCM, et al. Validation of a three-dimensional quantitative coronary angiography-based software to calculate fractional flow reserve: the FAST study. EuroIntervention. (2020) 16(7):591–9. doi: 10.4244/EIJ-D-19-00466
66. Collet C, Onuma Y, Sonck J, Asano T, Vandeloo B, Kornowski R, et al. Diagnostic performance of angiography-derived fractional flow reserve: a systematic review and Bayesian meta-analysis. Eur Heart J. (2018) 39(35):3314–21. doi: 10.1093/EURHEARTJ/EHY445
67. Mangiacapra F, Viscusi MM, Verolino G, Paolucci L, Nusca A, Melfi R, et al. Invasive Assessment of Coronary Microvascular Function. J Clin Med. (2022) 11(1):228. doi: 10.3390/JCM11010228
68. Godo S, Takahashi J, Yasuda S, Shimokawa H. Role of inflammation in coronary epicardial and microvascular dysfunction. European Cardiology Review. (2021) 16(5):1625–37. doi: 10.15420/ECR.2020.47
69. Sara JD, Widmer RJ, Matsuzawa Y, Lennon RJ, Lerman LO, Lerman A. Prevalence of coronary microvascular dysfunction among patients with chest pain and nonobstructive coronary artery disease. JACC Cardiovasc Interv. (2015) 8(11):1445–53. doi: 10.1016/J.JCIN.2015.06.017
70. Ong P, Camici PG, Beltrame JF, Crea F, Shimokawa H, Sechtem U, et al. International standardization of diagnostic criteria for microvascular angina. Int J Cardiol. (2018) 250:16–20. doi: 10.1016/J.IJCARD.2017.08.068
71. Zaya M, Mehta PK, Bairey Merz CN. Provocative testing for coronary reactivity and spasm. J Am Coll Cardiol. (2014) 63(2):103. doi: 10.1016/J.JACC.2013.10.038
72. Radico F, Zimarino M, Fulgenzi F, Ricci F, Di Nicola M, Jespersen L, et al. Determinants of long-term clinical outcomes in patients with angina but without obstructive coronary artery disease: a systematic review and meta-analysis. Eur Heart J. (2018) 39(23):2135–46. doi: 10.1093/EURHEARTJ/EHY185
73. Lee BK, Lim HS, Fearon WF, Yong AS, Yamada R, Tanaka S, et al. Invasive evaluation of patients with angina in the absence of obstructive coronary artery disease. Circulation. (2015) 131(12):1054–60. doi: 10.1161/CIRCULATIONAHA.114.012636
74. Ford TJ, Stanley B, Good R, Rocchiccioli P, McEntegart M, Watkins S, et al. Stratified medical therapy using invasive coronary function testing in angina: the CorMicA trial. J Am Coll Cardiol. (2018) 72(23 Pt A):2841–55. doi: 10.1016/J.JACC.2018.09.006
75. De Bruyne B, Pijls NHJ, Smith L, Wievegg M, Heyndrickx GR. Coronary thermodilution to assess flow reserve. Circulation. (2001) 104(17):2003–6. doi: 10.1161/HC4201.099223
76. Barbato E, Aarnoudse W, Aengevaeren WR, Werner G, Klauss V, Bojara W, et al. Validation of coronary flow reserve measurements by thermodilution in clinical practice. Eur Heart J. (2004) 25(3):219–23. doi: 10.1016/J.EHJ.2003.11.009
77. Zhang Y, Wernly B, Cao X, Mustafa SJ, Tang Y, Zhou Z. Adenosine and adenosine receptor-mediated action in coronary microcirculation. Basic Res Cardiol. (2021) 116(1):22. doi: 10.1007/S00395-021-00859-7
78. Mizukami T, Sonck J, Gallinoro E, Kodeboina M, Canvedra A, Nagumo S, et al. Duration of hyperemia with intracoronary administration of papaverine. J Am Heart Assoc. (2021) 10(3):1–3. doi: 10.1161/JAHA.120.018562
79. Jeremias A, Whitbourn RJ, Filardo SD, Fitzgerald PJ, Cohen DJ, Tuzcu EM, et al. Adequacy of intracoronary versus intravenous adenosine-induced maximal coronary hyperemia for fractional flow reserve measurements. Am Heart J. (2000) 140(4):651–7. doi: 10.1067/mhj.2000.109920
80. Ford TJ, Stanley B, Sidik N, Good R, Rocchiccioli P, McEntegart M, et al. 1-year outcomes of angina management guided by invasive coronary function testing (CorMicA). JACC Cardiovasc Interv. (2020) 13(1):33–45. doi: 10.1016/J.JCIN.2019.11.001
81. Montone RA, Niccoli G, Fracassi F, Russo M, Gurgoglione F, Cammà G, et al. Patients with acute myocardial infarction and non-obstructive coronary arteries: safety and prognostic relevance of invasive coronary provocative tests. Eur Heart J. (2018) 39(2):91–8. doi: 10.1093/EURHEARTJ/EHX667
82. Sueda S, Kurokawa K, Kurokawa T, Sakaue T, Ikeda S. Clinical outcomes and provoked epicardial spasm phenotypes via intracoronary acetylcholine testing in 680 patients with angina and nonobstructive coronary arteries. Life. (2022) 12(10):1465. doi: 10.3390/LIFE12101465/S1
83. Kaski JC. Provocative tests for coronary artery spasm in MINOCA: necessary and safe? Eur Heart J. (2018) 39(2):99–101. doi: 10.1093/EURHEARTJ/EHX737
84. Neumann FJ, Sechtem U, Banning AP, Bonaros N, Bueno H, Bugiardini R, et al. 2019 ESC guidelines for the diagnosis and management of chronic coronary syndromesThe Task Force for the diagnosis and management of chronic coronary syndromes of the European Society of Cardiology (ESC). Eur Heart J. (2020) 41(3):407–77. doi: 10.1093/EURHEARTJ/EHZ425
Keywords: coronary physiology, microvascular disease, angina, coronary angiography, coronary artery disease
Citation: Alisiddiq Z, Sharma H, Cotton J and Fan L (2023) Intra-coronary physiology in contemporary percutaneous coronary intervention and anginal therapy with a focus on microvascular disease. Front. Cardiovasc. Med. 10:1255643. doi: 10.3389/fcvm.2023.1255643
Received: 9 July 2023; Accepted: 2 November 2023;
Published: 29 November 2023.
Edited by:
Panagiotis Xaplanteris, Université Libre de Bruxelles, BelgiumReviewed by:
Vincenzo Sucato, University of Palermo, ItalyZoltan Ruzsa, University of Szeged, Hungary
Flavio Giuseppe Biccirè, Sapienza University of Rome, Italy
© 2023 Alisiddiq, Sharma, Cotton and Fan. This is an open-access article distributed under the terms of the Creative Commons Attribution License (CC BY). The use, distribution or reproduction in other forums is permitted, provided the original author(s) and the copyright owner(s) are credited and that the original publication in this journal is cited, in accordance with accepted academic practice. No use, distribution or reproduction is permitted which does not comply with these terms.
*Correspondence: Lampson Fan lampson.fan1@nhs.net