- 1Department of Cardiology, Careggi University Hospital, Florence, Italy
- 2Department of Internal Medicine, ASST Sette Laghi, Varese, Italy
- 3Cardiology, ASST Spedali Civili and Department of Medical and Surgical Specialties, Radiological Science and Public Health, University of Brescia, Brescia, Italy
Acute heart failure (AHF) represents a common clinical scenario that requires prompt evaluation and therapy and that is characterized by a high risk of mortality or subsequent rehospitalizations. The pathophysiology leading to AHF decompensation is still not fully understood. Significant activation of inflammatory pathways has been identified in patients with AHF, particularly in its most severe forms, and it has been hypothesized that systemic inflammation has a role in AHF pathogenesis. Several inflammatory mediators and cytokines, such as high sensitivity C-reactive protein, tumor necrosis factor-α, interleukin-6, interleukin-1, soluble suppression of tumorigenicity 2 and galectin-3, have been shown to play a role in the pathogenesis, development and worsening of this condition with an independent prediction of adverse outcomes. This manuscript reviews the prevalence and prognostic value of systemic inflammation in AHF, as well as the potential role of anti-inflammatory therapies, focusing on available evidence from clinical trials and ongoing studies.
Introduction
Acute heart failure (AHF) encompasses a broad spectrum of disease states and is characterized by heterogeneous clinical presentations (1–4). It refers to the onset of symptoms and/or signs of heart failure (HF) leading to an unplanned hospital admission and requiring urgent evaluation and therapy (5). Four major clinical presentations can be described with possible overlaps: acute decompensated heart failure (ADHF), acute pulmonary oedema, isolated right ventricular failure and cardiogenic shock (6). Finally, one-third of AHF presents as new-onset (de novo) HF (7). Although AHF is a common disease, with HF-related hospitalizations representing 1%–2% of all Emergency Department (ED) admissions in Europe (8), the pathophysiologic mechanisms underlying acute HF decompensations remain partially unexplained and the management unsatisfactory (9). AHF remains associated with increased mortality both in-hospital and to a larger extent post-discharge (10). The risk of death or rehospitalization is maximal in the few weeks after discharge, the so-called vulnerable phase, and decreases exponentially thereafter though remaining increased compared to that of the patients who remain ambulatory (11–13). In-hospital mortality ranges from 4% to 10% with rates of death or readmission higher than 45% after one year from hospital discharge (5). No evidence-based therapy is able to determine a substantial prognostic improvement during AHF hospitalization (2), although recent trials have demonstrated the benefit of early implementation of guideline-directed medical therapies on outcomes after discharge (14–17).
Systemic inflammation has been frequently identified in patients with both acute and chronic HF, with significantly enhanced neurohormonal and inflammatory activation especially in AHF (9, 18–20), but without a definitive pathophysiological link between inflammation and AHF development (21, 22). There is evidence that inflammation in ADHF may contribute to changes in vascular resistance, fluid redistribution and pulmonary congestion, diastolic dysfunction and systemic hypoperfusion (23, 24). Inflammatory activation also promotes a proapoptotic and prothrombotic milieu (25).
Several inflammatory mediators and cytokines are involved in AHF pathophysiology including high sensitivity C-reactive protein (hsCRP), tumor necrosis factor-alpha (TNF-α), interleukin-6 (IL-6), interleukin-1 (IL-1), soluble suppression of tumorigenesis-2 (sST2) and galectin-3. They have been associated to disease development and progression, representing independent predictors of poor mid-term and long-term outcomes in patients with recent ADHF (26–29). Unfortunately, it has been challenging to translate these prognostic findings into “prognostically-relevant” therapies (30). In fact, the goal of using anti-inflammatory drugs as disease-modifying therapy in AHF has not yet been achieved, with conflicting results from the most important clinical trials conducted in this direction, likely reflecting our poor understanding of the inflammatory networks underlying the heterogenous HF syndrome (31).
In our review, we discuss the role of inflammatory biomarkers in AHF, their prognostic value and the potential utility of targeting precise phenotypes to improve outcomes. We also summarize the results of most important clinical trials of therapies targeting inflammatory processes in the AHF setting, and explore future directions in this field.
Prevalence and pathophysiology of inflammation in AHF
Numerous studies have confirmed the presence of a sterile inflammation state (without concomitant infections) in ADHF, with high levels of several inflammatory cytokines at hospital admission detected in some patients with AHF. The intensity of systemic inflammation, as identified by different biomarkers, varies in different studies and according to different AHF presentation.
In AHF, the inflammatory response may arise both from antigenic stimulation during infection (e.g., viral myocarditis) or as a result of hemodynamic stress (32, 33). Systemic congestion and/or peripheral hypoperfusion cause neurohormonal activation, inflammation and oxidative stress which, in turn, damage endothelial glycocalix and consequently impair endothelial function and fluid homeostasis, promote a prothrombotic and proapoptotic environment, thus leading to a vicious pathogenetic circle (25, 34). Inflammatory activation seem to persist beyond the acute event and may contribute to high rehospitalization rates of heart failure (35).
The acute phase protein hsCRP is produced by hepatocytes in response to inflammation and is the most commonly measured inflammatory biomarker because simple to assay and relatively stable in peripheral blood (36). In the Acute Study of Clinical Effectiveness of Nesiritide in Decompensated Heart Failure (ASCEND-HF) trial, a median hsCRP concentration of 12.6 mg/L was observed at hospital admission (Table 1), highlighting the exacerbation of an inflammatory state related to AHF (37). In the prospective analysis conducted by Matsumoto et al. to evaluate the association between CRP levels at hospital admission and long-term mortality in 527 patients with ADHF, the quartile with the lowest baseline hsCRP value was numerically the least representative (<0.3 mg/dl, n = 114) (38). In the prospective multicenter Acute decompensated heart failure syndrome (ATTEND) registry, an overall median hsCRP level at admission of 5.8 mg/L was observed, with the first tertile including patients with lowest hsCRP levels at baseline (<2.9 mg/L) as the least numerous subgroup (39). In a recent sub-analysis of the Epidemiology of Acute Heart Failure in the Emergency Departments (EAHFE) registry including 1,109 patients with CRP > 5 mg/L, the median CRP value was of 36.7 mg/L (40).
Moving to other biomarkers beyond CRP, some studies evaluated IL-6 and IL-1 as markers of inflammation. IL-6 promotes a shift from a neutrophilic to a mononuclear cell infiltrate and is the primary cytokine that mediates the transition from acute to chronic inflammation (41). It acts downstream of IL-1 and represents the primary stimulus for the liver production of CRP (42). IL-6 increases cardiomyocyte stiffness reducing titin phosphorylation (43). Additionally, IL-6 also impairs natriuresis (by stimulating ENaC in the distal renal tubule) and urinary IL-6 concentrations are associated with worsening renal function and diuretic resistance. IL-6, like TNF-alpha, can also produce myocardial dysfunction; IL-6 signaling plays a role in harmful effects in the myocardium as cardiac myocyte loss and contributes to the progression of compensatory LV hypertrophy to heart failure, trigger a series of pathological responses, such as oxidative stress, endothelial dysfunction, induction of myocyte apoptosis with adverse remodelling which ultimately leads to cardiomyocyte dysfunction (44). In a secondary analysis of the large prospective multicenter Basics in Acute Shortness of Breath EvaLuation (BASEL-V) study, a novel IL-6 immunoassay has been used to quantify systemic inflammation in AHF. The vast majority (84%) of patient with AHF had elevated IL-6 concentrations (>4.45 ng/L), with less than one third with other causes of dyspnea (45). In ASCEND-HF, the median baseline IL-6 value was 14.1 pg/ml (IQR 8.1–26.4 pg/ml) and 293 out of 883 patients recruited had baseline IL-6≥20.4 pg/ml (46). In a retrospective analysis of the PROTECT cohort the levels of both plasma IL-6 and BNP determined by high-sensitivity single molecule counting technology (Singulex Inc.). Patients with higher baseline IL-6 levels were older, had lower estimated glomerular filtration rates and higher BNP levels (47).
IL-1 is an apical inflammatory cytokine that is moderately elevated in most HF forms and is markedly elevated in ADHF, as measured by CRP and IL-6 that are surrogate biomarkers of IL-1 activity. Furthermore, sST2, encoded by gene ST2 located on human chromosome 2q12 (48), is a protein member of the IL-1 receptor family released under conditions of myocardial and vascular strain and plays an essential role in mediating myocardial remodeling and fibrosis (49). The ST2 protein is found both as a trans-membrane and a soluble form in serum. The trans-membrane form of ST2 plays a role in modulating responses of T helper type 2 cells, whereas the soluble form of ST2 is up-regulated in growth-stimulated fibroblasts. Despite the potential role played by ST2 in inflammation, significant parallels between ST2 and natriuretic peptides exist: the ST2 gene is markedly up-regulated in states of myocyte stretch, similar to the induction of the BNP gene (50).
To evaluate the efficacy of new therapies against the inflammatory IL-1 pathway such as anakinra, CRP (or hsCRP) and/or plasma IL-6 levels have been used as IL-1 surrogate biomarkers. In the Recently Decompensated Heart Failure Anakinra Response Trial (REDHART) left ventricular ejection fraction (LVEF) < 50% and CRP > 2 mg/L, a good correlation was observed between CRP levels at 12 weeks and changes in peak Vo2 (R = −0.57; P = 0.001) (51). In a small randomized pilot study conducted by Van Tassell et al. and evaluating the usefulness of anakinra in reducing systemic inflammation among 30 patients with ADHF and hsCRP ≥ 5 mg/L, hsCPR plasma levels were considered as a good marker to express IL-1 inflammatory pathway activation (52). Moreover a study conducted by Pascal-Figual et al. evaluated the relationship between IL-1β and sST2 in ADHF, as well as the prognostic role of elevated IL-1β and sST2 concentrations (53).
Taken together, these studies suggest that signs of systemic inflammation are frequently observed among patients with AHF, potentially with higher levels in patients with more severe clinical presentation (i.e., cardiogenic shock) as compared to those with less severe phenotypes.
Another important biomarker that correlate with inflammation and cardiac fibrosis with adverse remodeling is galectin-3, a soluble β-galactoside-binding protein secreted by activated macrophages, whose main action is to bind to and activate the fibroblasts that form collagen (54). Driven by the growing interest in this topic, new potential markers of inflammation have been related to AHF. Myeloperoxidase (MPO) is released from activated neutrophils, monocytes and endothelial cells in response to oxidative stress, especially after myocardial infarction, while growth differentiation factor 15 (GDF-15) regulates inflammatory and apoptotic pathways by inhibiting macrophage activation in a large number of pathological conditions, including AHF (55, 56).
Recently, carbohydrate antigen 125 (CA125), a large glycoprotein synthesized by mesothelial cells, has been validated as a reliable indicator of congestion and inflammation in patients with AHF.
Furthermore, another aspect deserving attention is the potential bidirectional relationship between HF decompensation and inflammation. A robust interconnection exists between inflammation, HF evolution and hemodynamic impairment (reduced cardiac output, elevated filling pressures) (57). This synergistic interplay assumes heightened significance during the acute stages of the disease. Recent studies demonstrated how conventional HF medications can exert a substantial “pleiotropic” effect in mitigating inflammation. The study of Mapelli et al. shows how sacubitril/valsartan have effects on HF biomarkers (in particular decreasing NT-proBNP and ST2 levels), indicating its ability to modulate the underlying pathophysiological processes associated with HF (58). The impact of sacubitril/valsartan has been also examined on circulating microRNAs (miRNAs) in patients with HF: miRNAs are small RNA molecules that play a crucial role in regulating gene expression and have been implicated in various cardiovascular diseases, including HF. The results of the study indicate that sacubitril/valsartan treatment leads to changes in the levels of circulating miRNAs that are known to be involved in the pathophysiological processes of HF, including also inflammation (59).
As demonstrated in the study of Campodonico et al., another drug used in AHF, levosimendan, has positive effects on inflammation: the results of the study demonstrate that acute hemodynamic improvement with levosimendan leads to changes in surfactant proteins in patients with advanced chronic HF. Specifically, there are alterations in the levels of certain surfactant proteins, indicating a potential impact on lung function. These findings suggest that the beneficial effects of levosimendan in HF may extend beyond the cardiovascular system and affect pulmonary function as well (60).
Prognostic impact of inflammation in AHF
The prognostic role of inflammatory markers in both chronic and acute HF is well known (26, 61). Therefore, several studies have been conducted with the aim of evaluating whether the decreasing levels of these markers, in response to anti-inflammatory therapies, correlated with better outcomes.
The potential prognostic role and predictive value of hsCRP have been evaluated (62, 63). In patients with AHF, high levels of CRP at hospital admission have been associated with higher short-term cardiac and non-cardiac mortality, as demonstrated by the ASCEND-HF trial, the ATTEND study and the China Patient-centered Evaluative Assessment of Cardiac Events (PEACE 5p-HF) study (Table 1). In a biomarker study of the ASCEND-HF trial, higher baseline hsCRP levels were associated with longer hospital stay [0.57 days per log2 hsCRP in adjusted models; 95% confidence interval (CI) 0.33–0.81; P < 0.001], but were not associated with the composite short-term endpoint of death or worsening HF during index admission, 30-day death or HF readmission, or 180-day mortality. In both HFrEF and HFpEF subgroups, levels and changes of hsCRP were not differentially associated with outcomes. However, persistently elevated or increasing hsCRP levels at day 30 after admission were predictive of 180-day mortality (37).
In the ATTEND study, significant increases in all-cause, cardiac, and non-cardiac death were observed from the lowest to highest tertiles of CRP levels measured on admission (log-rank P < 0.001, P < 0.001, and P < 0.001, respectively). Among patients in the third, second and first tertile, the incidence rates of all-cause death was 34.1% (95% CI 31.5–36.7), 22.9% (95% CI 20.6–25.2) and 17.0% (95% CI 15.0–19.1), respectively. Furthermore, there were significant interactions of CRP levels with mortality when the patients were stratified by LVEF, with risk of cardiac mortality significantly greater in the subgroup of patients with reduced ejection fraction (40%) than in those with preserved ejection fraction (>40%, P = 0.047 for interaction) (39, 64). In PEACE 5p-HF, patients with persistently high and very high trajectories had a significantly higher risk of all-cause and cardiovascular mortality than those with the persistently low trajectory. Of note, CRP appears to have no role in the prognostic evaluation of AHF patients in the context of a concomitant infectious state. Non-infected patients with CRP > 12.3 mg/L had a doubled risk of death or readmission for HF, independent of other well-established predictors of prognosis. All-cause death or readmission for HF worsening occurred in 40% of the patients whose CRP value increased compared with 26.4% of those whose CRP decreased (P = 0.23). Evaluating the entire non infected sample, a lower CRP (<6.7 mg/L) was associated with better hospitalization-free survival, reinforcing the idea that stronger inflammatory activation carries a worse prognosis (65).
IL-6 elevations in both plasma and urine were associated with features of cardio-renal syndrome with decreased glomerular filtration rate, decreased diuretic responsiveness, and enhanced neurohormonal activation (66). Of note, in a recent analysis of BASEL V, IL-6 concentrations were a strong independent predictor of 1-year all-cause mortality among patients with AHF, in particular in patients without clinically overt infection at presentation. The observed 1-year all-cause mortality and HF rehospitalization rate were significantly higher in patients with elevated IL-6 levels compared to those with normal levels (P < 0.001 and P = 0.002, respectively). Furthermore IL-6 concentrations differed substantially among different AHF phenotypes with the highest concentrations observed in cardiogenic shock and the lowest in hypertensive HF or worsening HF, showing a correlation between the most severe AHF phenotype and a most extensive systemic inflammation. The addition of IL-6 also improved the predictive value of the BIOSTAT-CHF risk model for all-cause death (45). In an ASCEND-HF substudy, high baseline IL-6 values were associated with 30-day and 180-day mortality in patients with AHF. Moreover IL-6 levels closely correlated with NT-proBNP in this population (46). Furthermore, the temporal evolution patterns and the time-course of changes in the levels of IL-6 had additive prognostic value independent of BNP changes in patients with AHF, correlated with HF severity and predicted worsening HF (47, 67, 68). Finally, Miettinen et al. demonstred how IL-6 is linked to other pro-inflammatory cytokines, and elevated circulating levels of IL-6 and TNF-α were strongly associated with increased 1-year mortality in patients with ADHF, with poor outcome for ADHF patients with upper tertile levels of IL-6 and TNF-α (69).
In ADHF, IL-1β concentration at clinical presentation was associated with prior HF hospitalizations, higher NT-proBNP values and functional impairment. As continuous variables, IL-1β and sST2 concentrations were associated with higher all-cause mortality, with significantly higher IL-1β levels in patients who died during the first year after hospitalization (53). If the sST2 values currently do not have diagnostic role, they instead have a strong prognostic value in ADHF, as demonstrated by the PRIDE study: in a stratified analysis of only those patients with A HF and available ST2 results, ST2 > 0.20 ng/ml was an indipendent predictor of death, with a subsequently graded relationship between ST2 concentrations and mortality. Subjects above the ST2 median had 11-fold greater odds for death compared with those below the median (70).
Galectin-3 leads to progressive cardiac fibrosis, linking local inflammation and myocardial remodeling. It has been demonstrated that galectin-3 is a useful marker patients with AHF in predicting 60-day and long-term mortality. In an analysis conducted by van Kimmenade et al., an elevated level of galectin-3 was the best independent predictor of 60-day mortality or the combination of death/recurrent HF within 60 days, superior to NT-proBNP. Median concentrations of galectin-3 were significantly higher among those subjects dying by 60 days of follow-up than in those surviving (71).
V. Shah et al. have demonstrated a good correlation between galectin-3 concentrations and echocardiographic markers of ventricular diastolic and sistolic function. Furthermore, in dyspnoeic patients with ADHF, galectin-3 remained a significant predictor of 4-year mortality independent of echocardiographic markers of risk. Patients with galectin-3 levels above the median value had a 63% mortality; patients less than the median value had a 37% mortality (P = 0.003) (72).
Furthermore, high MPO and high GDF-15 concentrations were independently associated with worse outcomes in AHF, including higher mortality. MPO concentrations, despite their poor diagnostic accuracy for AHF, have shown an important prognostic role as indipendent predictors of 1-year mortality in AHF. In patients with AHF, higher mortality was observed when MPO concentrations at presentation were in the second (99–190 pmol/L, 37% mortality) and third (>190 pmol/L, 39% mortality) tertiles of MPO compared with the first tertile (≤99 pmol/L, 28% mortality, P = 0.058) (73). Results from the RELAX-AHF study showed that higher levels of GDF-15 at baseline and larger increases at day 2 and day 14 were associated with significantly increased risks of the combined endpoint of 60-day HF rehospitalizations or CV death (74).
In a multi-marker prognostic analysis in AHF, higher GDF-15 concentrations were associated with worse prognosis in AHF independently of BNP, in both patients with HFrEF and HFpEF. Patients discharged with both BNP and GDF-15 above the mean had a multivariate adjusted HR of 2-year death of 4.33 (95% CI 2.07–90.6, P < 0.001) when compared with the reference category (both BNP and GDF-15 below the mean) (75).
CA125, a complex glycoprotein encoded by the MUC16 gene in humans, has been recently studied in cardiovascular diseases, particularly in cases of decompensated HF and during the transition to clinical stability. Much evidence has recently supported the positive correlation of plasma levels of CA125 with congestion and inflammation. The reasons for elevated CA125 levels in decompensated HF are not well understood, but it appears that both hemodynamic and inflammatory factors play a role (31). One proposed mechanism is the activation of mesothelial cells in response to increased pressure, mechanical stress, and cytokine activation, leading to CA125 synthesis. Inflammatory cytokines like IL-1, TNF-α, and lipopolysaccharide have been shown to enhance CA125 secretion (76). In HF, there is evidence linking higher CA125 levels to congestion and inflammation which are strictly interconnected, and long-term venous congestion may trigger the inflammatory system, resulting in cytokine-driven CA125 synthesis and release by mesothelial cells, even when there is no visible serosal effusion (77).
As demonstrated in the study of Kouris et al., elevated CA125 levels were significantly associated with the severity of congestion in HF patients (72). Around two-thirds of patients with AHF were found to have elevated CA125 levels. Another study by Miñana et al. investigated the factors influencing the plasma levels of CA125 in patients with AHF, identifying a strong association between elevated CA125 levels and the severity of congestion in AHF patients. Moreover, the authors identified specific factors, such as clinical parameters of congestion and the severity of tricuspid regurgitation, that were significantly correlated with variations in CA125 concentrations (73). Finally, Llàcer et al. compared the effectiveness of two biomarkers, CA125 and NT-proBNP, in evaluating congestion in patients with AHF, showing that both CA125 and NT-proBNP were useful indicators of congestion in AHF, with elevated levels of both biomarkers being associated with increased severity of congestion (74). Of note, CA125 exhibited a stronger correlation with congestion compared to NT-proBNP. The study also found that the combination of these biomarkers improved the accuracy of evaluating congestion levels in AHF patients, allowing for a more precise and reliable diagnosis and potentially aiding in better treatment decisions. These findings suggest that CA125 could be used as a valuable biomarker for assessing the severity and prognosis of AHF, providing clinicians with useful information for patient management and treatment strategies.
Therefore, available evidence suggests that different biomarkers involved in inflammatory pathways (CRP, IL-6, IL-1 axis, Galectin-3, MPO, GDF-15 and CA-125) have a strong prognostic impact in patients with AHF, and their increased circulating levels secondary to enhanced systemic inflammation are associated with worse outcomes and may represent interesting therapeutic targets (Figure 1).
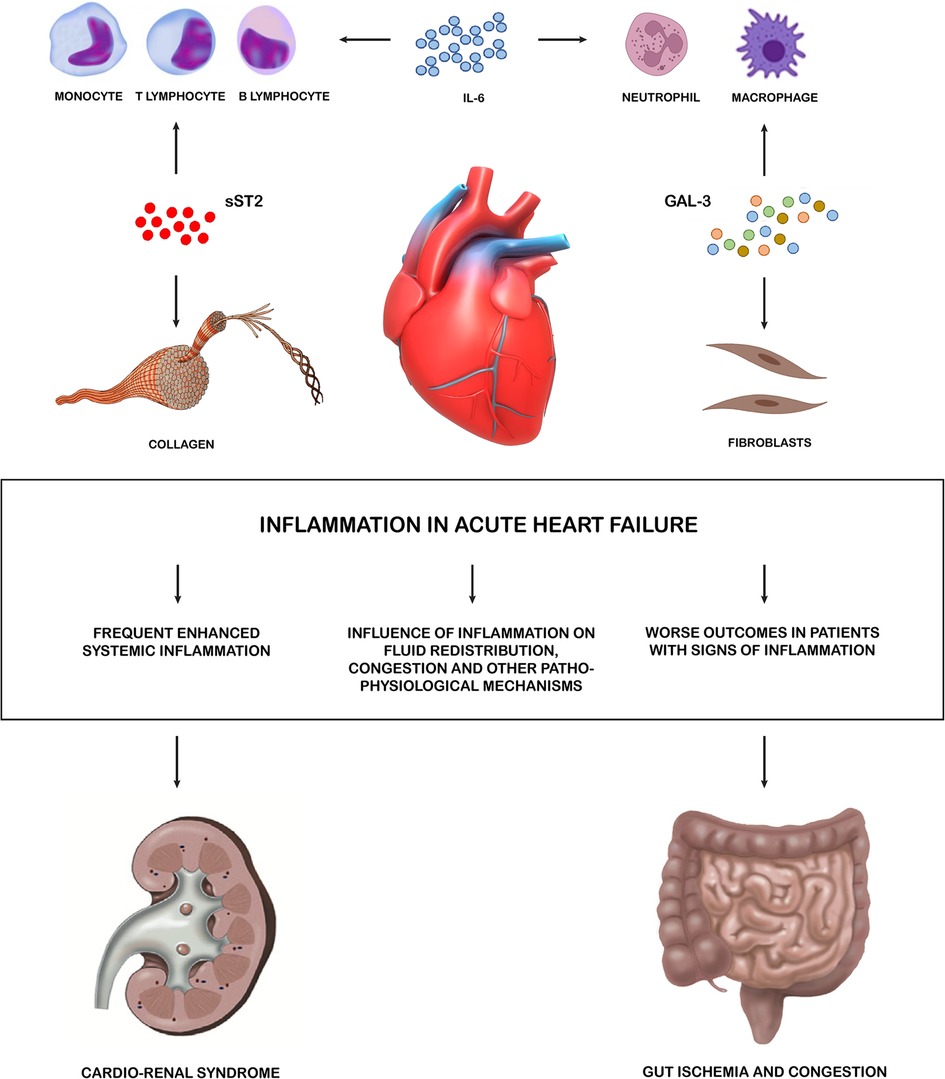
Figure 1. Inflammation in acute heart failure. Systemic inflammation has been proved to be frequently enhanced during AHF, with high levels of different inflammatory biomarkers identified in relevant subset of patients. Such biomarkers have also been involved in key pathophysiological pathways leading to HF decompensation. These pro-inflammatory cytokines allow the crosstalk between the heart and multiple peripheral organ systems. The main effectors of inflammations are the lymphatic tissue cells which, through cytokines, also activate fibroblasts and lead to the formation of collagen. Kidney and gut are targets of these negative effects. sST2, soluble suppression of tumorigenesis-2; GAL 3, galectin 3; AHF, acute heart failure.
Anti-inflammatory therapies in AHF
Over the past decades, several anti-inflammatory therapies have been investigated for their potential efficacy in treating HF, including colchicine, methotrexate, xanthine oxidase inhibitors, monoclonal antibodies targeting inflammatory cytokines, and corticosteroids. However, the majority of these studies have focused on the role of anti-inflammatory drugs in chronic HF, while evidence is scarce about their use in patients with AHF (Table 2). Different immune mechanisms might be involved in the acute and chronic phases of HF. Innate immune responses might be more relevant targets in de novo HF, whereas adaptive immune responses might be more prominent in chronic or acutely decompensated HF settings (78).
To date, only a few studies evaluated the effect of anti-inflammatory therapy in the setting of AHF. Monoclonal antibodies targeting inflammatory cytokines, such as canakinumab and anakinra, have shown promising results in reducing inflammation yet not definitive results in improving outcomes among patients with AHF. A pilot randomized trial enrolled 30 patients with ADHF, LVEF < 40% and elevated hsCRP levels (≥5 mg/L) that were randomized 1:1 to either anakinra 100 mg twice daily for 3 days followed by once daily for 11 days or matching placebo. Anakinra reduced CRP at 72 h by 61% versus baseline, compared with a 6% reduction among patients receiving placebo (p = 0.004). The study was designed to evaluated only changes in inflammatory markers and not functional or clinical outcomes (52). In the REDHART trial, 60 patients with LVEF <50%, recent hospitalization for decompensated HF and elevated CRP levels (>2 mg/L), within 14 days of hospital discharge, were randomized 1:1:1 to daily subcutaneous injections with anakinra 100 mg for 2 weeks, 12 weeks, or placebo. The aim of the study was to evaluate whether IL-1 blockade with anakinra would improve aerobic exercise capacity in patients with recently decompensated systolic HF. The evidence of enhanced systemic inflammation, in particular of the IL-1 inflammatory pathway, was demonstrated by elevated CRP plasma levels (>2 mg/L, measured by high-sensitivity assay). CRP at 12 weeks was reduced by a median of 66% in the 12-week anakinra group (P = 0.011), whereas by 12 weeks the CRP levels in the anakinra 2-week group were no longer significantly different from baseline, with a good correlation between CRP levels at 12 weeks and changes in peak Vo2 (R = −0.57; P = 0.001). No change in peak VO2 occurred at 2 weeks in patients treated with anakinra, although an improvement was seen in those who continued anakinra for 12 weeks (51).
Is well known that colchicine may reduce myocardial stiffness and cardiac hypertrophy also through various anti-inflammatory actions, such as inhibiting the activation of the nucleotide-binding domain-like receptor protein 3 inflammasome, which leads to the maturation of IL-1β and IL-18. It also hampers the movement of neutrophils by blocking tubulin polymerization and microtubule formation, reduces IL-1 production in activated neutrophils, and decreases the expression of TNF-α receptors in macrophages and endothelial cells (79). In a retrospective study evaluating the role of colchicine to treat acute gout flares in patients with ADHF, colchicine use was associated with a lower rate of in-hospital all-cause and cardiovascular mortality, but patients who received colchicine had longer hospital stays. There was no significant difference in 30-day readmission rates between patients receiving and not receiving colchicine (80). Regarding steroid therapy in AHF, some trials have been conducted evaluating its inflammatory role with discordant results so far. A single-arm, observational study by Zhang et al. investigated the potential benefit of adding prednisone to usual care treatment in patients with refractory decompensated congestive HF. The study enrolled 35 patients with refractory decompensated HF who received prednisone in addition to usual care. The primary endpoints of the study were the effects on daily urine volume, patient- and physician-assessed dyspnoea and global clinical status, and changes in renal function. The results of the study showed that treatment with prednisone, on top of usual care, induced potent diuresis and was associated with an improvement in congestive symptoms, clinical status and renal function (81). In the Cardiac Outcome Prevention Effectiveness of Glucocorticoids in Acute Decompensated Heart Failure (COPE-ADHF) trial, 102 patients with ADHF were randomized to receive corticosteroids (single dose of 20-mg dexamethasone followed by 1 mg/kg prednisone daily with a maximum dose of 60 mg daily for 7 days) plus diuretic therapy or standard diuretic therapy alone. The primary endpoint of the study was a change from baseline in serum creatinine at day 7 and cardiovascular death within 30 days. There was a remarkable reduction in creatinine after 7 days of treatment and a reduction in 30-day cardiovascular death in the glucocorticoid group (82). Recently, the large observational CORTicosterioids in Acute Heart Failure (CORT-AHF) study confirmed the safety of new onset of systemic corticosteroids in AHF, reporting no evidence of harm among 11,356 patients with AHF enrolled in EAHFE registry. This was confirmed both in patients with or without concomitant chronic obstructive pulmonary disease (COPD) (83).
Finally, levosimendan was associated to a significant decrease in circulating pro-inflammatory cytokines and also a reduction in soluble apoptosis mediators demonstrating that this medication has a positive effect on reducing inflammation and cell death processes in patients with decompensated advanced HF (84).
Therefore, although preliminary evidence suggests the safety of anti-inflammatory therapy in the AHF setting, especially for systemic corticosteroids, no definitive proof of efficacy has been demonstrated in patients with AHF and concomitant systemic inflammation.
Ongoing studies and future perspectives
Some studies testing anti-inflammatory therapy in AHF are ongoing. Beyond the randomized Dexamethasone Versus Prednisone in Heart Failure Patients, Hospitalized With Exacerbation of Chronic Obstructive Pulmonary Disease trial (NCT02237820), that is focused on HF patients hospitalized with exacerbation of COPD, other studies are specifically focusing on the impact on anti-inflammatory strategies in AHF.
The Recently Decompensated Heart failure Anakinra Response 2 Trial (REDHART2) planned to enrol 102 patients with recently decompensated HF (before hospital discharge), LVEF ≤ 40% and hsCRP >2 mg/L, that are randomized 2:1 to anakinra 100 mg for 24 weeks or placebo (NCT03797001). The primary objective is to determine whether sustained anakinra treatment determines an improvement in aerobic exercise capacity, with peak VO2 change at 24 weeks as primary endpoint.
The ongoing Randomized Double-blind Trial to Study the Benefit of Colchicine in Patients With Acutely Decompensated Heart Failure (COLICA) study is randomizing 278 patients with ADHF and either reduced or preserved LVEF to colchicine 0.5 mg or placebo, initiated within the first 24 h of hospitalisation and administered for 8 weeks (NCT04705987). The primary objective of the study is the reduction of NT-proBNP after 2 months of treatment. Of note, evidence of systemic inflammation is not needed to be enrolled in the COLICA trial.
The Effect of Short-Term Prednisone Therapy on C-Reactive Protein Change in Emergency Department Patients With Acute Heart Failure and Elevated Inflammatory Marker (CORTAHF) trial is including 120 patients with AHF evaluated at the ED, randomized 1:1 to prednisone 40 mg for 7 days plus standard therapy or standard therapy alone (NCT05668676). Of note, prednisone therapy is initiated in the ED and continued up to 7 days. The primary endpoint is change in CRP from baseline to day 7, and other clinical and functional outcomes will be evaluated as secondary endpoints.
The multicenter, randomized, open-label, controlled study to evaluate the efficacy and safety of corticoSTEROids added to standard therapy in patients with Acute Heart Failure (STERO-AHF) pilot trial will enrol 120 patients hospitalized for AHF, irrespective of LVEF, with documented diuretic resistance (according to current guidelines) (5) and with evidence of sustained systemic inflammation (CRP ≥ 20 mg/L) (NCT05809011). Patients will be randomized 1:1 to or standard-of-care plus corticosteroid therapy for up to 7 days (single-bolus intravenous dexamethasone 20 mg on day 1 followed by oral prednisone 1 mg/kg daily—maximum 60 mg daily—from day 2 to day 7) or standard-of-care alone. The two primary endpoints are diuretic response, defined as absolute body weight change from baseline to day 8 (or to discharge or to the occurrence of death) per 40 mg total dose of administered intravenous furosemide or equivalent, and early clinical benefit, defined as a hierarchical composite of all-cause death, worsening HF, or change in patient-reported dyspnea (quantified by the visual analogue scale) from baseline to day 8 (or to discharge or to the occurrence of death).
Hopefully, these ongoing studies will help defining the role of pharmacological or interventional anti-inflammatory therapies in patients with AHF episodes.
Conclusions
Different inflammatory pathways can be implicated in different HF stages, also including the specific setting of AHF. Inflammation can be both a cause and consequence of HF decompensation and seems to play a pathogenetic and prognostic role, with several inflammatory mediators and cytokines up-regulated and associated with worse prognosis in patients with AHF. Despite numerous studies validating the association between specific inflammatory biomarkers and AHF, results from clinical trials have proven conflicting results, with some recent trials showing encouraging preliminary findings, but without definitive and convincing evidence supporting the use of anti-inflammatory therapies in this setting, yet. Managing inflammation is still a clinical challenge in AHF, and ongoing studies will help defining the role of different anti-inflammatory pharmacological approaches (corticosteroids, colchicine, anti-IL-1 therapy) in improving AHF outcomes.
Author contributions
All authors listed have made a substantial, direct, and intellectual contribution to the work and approved it for publication.
Conflict of interest
MP has received personal fees from Abbot Vascular, AstraZeneca, Boehringer Ingelheim and Vifor Pharma. MA reports speaker fees from Abbott Vascular and Medtronic. MM has received consulting honoraria as a member of trial committees or advisory boards for Abbott Vascular, Actelion, Amgen, Bayer, Edwards Therapeutics, Servier, Vifor Pharma and Windtree Therapeutics.
The remaining authors declare that the research was conducted in the absence of any commercial or financial relationships that could be construed as a potential conflict of interest.
The reviewer MM declared a past co-authorship with the authors MM, CML, RMI, DT to the handling editor.
Publisher's note
All claims expressed in this article are solely those of the authors and do not necessarily represent those of their affiliated organizations, or those of the publisher, the editors and the reviewers. Any product that may be evaluated in this article, or claim that may be made by its manufacturer, is not guaranteed or endorsed by the publisher.
References
1. Shoaib A, Farag M, Nolan J, Rigby A, Patwala A, Rashid M, et al. Mode of presentation and mortality amongst patients hospitalized with heart failure? A report from the first euro heart failure survey. Clin Res Cardiol Off J Ger Card Soc. (2019) 108(5):510–9. doi: 10.1007/s00392-018-1380-6
2. Tomasoni D, Lombardi CM, Sbolli M, Cotter G, Metra M. Acute heart failure: more questions than answers. Prog Cardiovasc Dis. (2020) 63(5):599–606. doi: 10.1016/j.pcad.2020.04.007
3. Gupta AK, Tomasoni D, Sidhu K, Metra M, Ezekowitz JA. Evidence-based management of acute heart failure. Can J Cardiol. (2021) 37(4):621–31. doi: 10.1016/j.cjca.2021.01.002
4. Rosano GMC, Vitale C, Adamo M, Metra M. Roadmap for the management of heart failure patients during the vulnerable phase after heart failure hospitalizations: how to implement excellence in clinical practice. J Cardiovasc Med Hagerstown Md. (2022) 23(3):149–56. doi: 10.2459/JCM.0000000000001221
5. McDonagh TA, Metra M, Adamo M, Gardner RS, Baumbach A, Bohm M, et al. 2021 ESC guidelines for the diagnosis and treatment of acute and chronic heart failure: developed by the task force for the diagnosis and treatment of acute and chronic heart failure of the European Society of Cardiology (ESC). with the special contribution of the Heart Failure Association (HFA) of the ESC. Eur J Heart Fail. (2022) 24(1):4–131. doi: 10.1002/ejhf.2333
6. Chiocel O, Mebazaa A, Harjola VP, Coats AJ, Piepoli MF, Crespo-Leiro MG, et al. Clinical phenotypes and outcome of patients hospitalized for acute heart failure: the ESC heart failure long-term registry. Eur J Heart Fail. (2017) 19(10):1242–54. doi: 10.1002/ejhf.890
7. Nieminem MS, Brutsaert D, Dickstein K, Drexler H, Follath F, Harjola VP, et al. Euroheart failure survey II (EHFS II): a survey on hospitalized acute heart failure patients: description of population. Eur Heart J. (2006) 27(22):2725–36. doi: 10.1093/eurheartj/ehl193
8. Rosano GMC, Seferovic P, Savarese G, Spoletini I, Lopatin Y, Gustafsson F, et al. Impact analysis of heart failure across European countries: an ESC-HFA position paper. ESC Heart Fail. (2022) 9(5):2767–78. doi: 10.1002/ehf2.14076
9. Davison BA, Senger S, Sama IE, Koch GG, Mebazaa A, Dickstein K, et al. Is acute heart failure a distinctive disorder? An analysis from BIOSTAT-CHF. Eur J Heart Fail. (2021) 23(1):43–57. doi: 10.1002/ejhf.2077
10. Hariharaputhiran S, Peng Y, Ngo L, Ali A, Hossain S, Visvanathan R, et al. Long-term survival and life expectancy following an acute heart failure hospitalization in Australia and New Zealand. Eur J Heart Fail. (2022) 24(9):1519–28. doi: 10.1002/ejhf.2595
11. Kimmoun A, Takagi K, Gall E, Ishihara S, Hammoum P, El Bèze N, et al. Temporal trends in mortality and readmission after acute heart failure: a systematic review and meta-regression in the past four decades. Eur J Heart Fail. (2021) 23(3):420–31. doi: 10.1002/ejhf.2103
12. Logeart D, Berthelot E, Bihry N, Eschalier R, Salvat M, Garcon P, et al. Early and short-term intensive management after discharge for patients hospitalized with acute heart failure: a randomized study (ECAD-HF). Eur J Heart Fail. (2022) 24(1):219–26. doi: 10.1002/ejhf.2357
13. Solomon SD, Dobson J, Pocock S, Skali H, McMurray JJV, Granger CB, et al. Influence of nonfatal hospitalization for heart failure on subsequent mortality in patients with chronic heart failure. Circulation. (2007) 116(13):1482–7. doi: 10.1161/CIRCULATIONAHA.107.696906
14. Mebazaa A, Davison B, Chioncel O, Cohen-Solal A, Diaz R, Filippatos G, et al. Safety, tolerability and efficacy of up-titration of guideline-directed medical therapies for acute heart failure (STRONG-HF): a multinational, open-label, randomised, trial. Lancet Lond Engl. (2022) 400(10367):1938–52. doi: 10.1016/S0140-6736(22)02076-1
15. Ponikowski P, Kirwan BA, Anker SD, McDonagh T, Dorobantu M, Drozdz J, et al. Ferric carboxymaltose for iron deficiency at discharge after acute heart failure: a multicentre, double-blind, randomised, controlled trial. Lancet Lond Engl. (2020) 396(10266):1895–904. doi: 10.1016/S0140-6736(20)32339-4
16. Voors AA, Angermann CE, Teerlink JR, Collins SP, Kosiborod M, Biegus J, et al. The SGLT2 inhibitor empagliflozin in patients hospitalized for acute heart failure: a multinational randomized trial. Nat Med. (2022) 28(3):568–74. doi: 10.1038/s41591-021-01659-1
17. Bhatt DL, Szarek M, Steg PG, Cannon CP, Leiter LA, McGuire DK, et al. Sotagliflozin in patients with diabetes and recent worsening heart failure. N Engl J Med. (2021) 384(2):117–28. doi: 10.1056/NEJMoa2030183
18. Mohebi R, Liu Y, van Kimmenade R, Gaggin HK, Murphy SP, Januzzi JL. Inflammation across universal definition of heart failure stages: the CASABLANCA study. Eur J Heart Fail. (2023) 25(2):152–60. doi: 10.1002/ejhf.2742
19. Reina-Couto M, Pereira-Terra P, Quelhas-Santos J, Silva-Pereira C, Albino-Teixeira A, Sousa T. Inflammation in human heart failure: major mediators and therapeutic targets. Front Physiol. (2021) 12:746494. doi: 10.3389/fphys.2021.746494
20. Adamo L, Rocha-Resende C, Prabhu SD, Mann DL. Reappraising the role of inflammation in heart failure. Nat Rev Cardiol. (2020) 17(5):269–85. doi: 10.1038/s41569-019-0315-x
21. Remmelzwaal S, van Oort S, Handoko ML, van Empel V, Heymans SRB, Beulens JWJ. Inflammation and heart failure: a two-sample mendelian randomization study. J Cardiovasc Med Hagerstown Md. (2022) 23(11):728–35. doi: 10.2459/JCM.0000000000001373
22. Georgiopoulos G, Delialis D, Aimo A. Inflammation in heart failure: causal determinant or bystander? J Cardiovasc Med Hagerstown Md. (2022) 23(11):736–7. doi: 10.2459/JCM.0000000000001396
23. Deswal A, Petersen NJ, Feldman AM, Young JB, White BG, Mann DL. Cytokines and cytokine receptors in advanced heart failure: an analysis of the cytokine database from the vesnarinone trial (VEST). Circulation. (2001) 103(16):2055–9. doi: 10.1161/01.cir.103.16.2055
24. Kataja A, Tarvasmaki T, Lassus J, Sionis A, Mebazaa A, Pulkki K, et al. Kinetics of procalcitonin, C-reactive protein and interleukin-6 in cardiogenic shock—insights from the CardShock study. Int J Cardiol. (2021) 322:191–6. doi: 10.1016/j.ijcard.2020.08.069
25. Mentz RJ, O’Connor CM. Pathophysiology and clinical evaluation of acute heart failure. Nat Rev Cardiol. (2016) 13(1):28–35. doi: 10.1038/nrcardio.2015.134
26. Zhu X, Cheang I, Xu F, Gao R, Liao S, Yao W, et al. Long-term prognostic value of inflammatory biomarkers for patients with acute heart failure: construction of an inflammatory prognostic scoring system. Front Immunol. (2022) 13:1005697. doi: 10.3389/fimmu.2022.1005697
27. Aimo A, Vergaro G, Ripoli A, Bayes-Genis A, Pascual Figal DA, De Boer RA, et al. Meta-analysis of soluble suppression of tumorigenicity-2 and prognosis in acute heart failure. JACC Heart Fail. (2017) 5(4):287–96. doi: 10.1016/j.jchf.2016.12.016
28. Mueller C, Laule-Kilian K, Christ A, Brunner-La Rocca HP, Perruchoud AP. Inflammation and long-term mortality in acute congestive heart failure. Am Heart J. (2006) 151(4):845–50. doi: 10.1016/j.ahj.2005.06.046
29. Njoroge JN, Teerlink JR. Pathophysiology and therapeutic approaches to acute decompensated heart failure. Circ Res. (2021) 128(10):1468–86. doi: 10.1161/CIRCRESAHA.121.318186
30. Panahi M, Papanikolaou A, Torabi A, Zhang JG, Khan H, Vazir A, et al. Immunomodulatory interventions in myocardial infarction and heart failure: a systematic review of clinical trials and meta-analysis of IL-1 inhibition. Cardiovasc Res. (2018) 114(11):1445–61. doi: 10.1093/cvr/cvy145
31. Murphy SP, Kakkar R, McCarthy CP, Januzzi JL. Inflammation in heart failure: JACC state-of-the-art review. J Am Coll Cardiol. (2020) 75(11):1324–40. doi: 10.1016/j.jacc.2020.01.014
32. Chen D, Assad-Kottner C, Orrego C, Torre-Amione G. Cytokines and acute heart failure. Crit Care Med. (2008) 36(1 Suppl):S9–16. doi: 10.1097/01.CCM.0000297160.48694.90
33. Virzì GM, Breglia A, Brocca A, De Cal M, Bolin C, Vescovo G, et al. Levels of proinflammatory cytokines, oxidative stress, and tissue damage markers in patients with acute heart failure with and without cardiorenal syndrome type 1. Cardiorenal Med. (2018) 8(4):321–31. doi: 10.1159/000492602
34. Harjola VP, Mullens W, Banaszewski M, Bauersachs J, Brunner-La Rocca HP, Chioncel O, et al. Organ dysfunction, injury and failure in acute heart failure: from pathophysiology to diagnosis and management. A review on behalf of the acute heart failure committee of the Heart Failure Association (HFA) of the European Society of Cardiology (ESC). Eur J Heart Fail. (2017) 19(7):821–36. doi: 10.1002/ejhf.872
35. Cotter G, Felker GM, Adams KF, Milo-Cotter O, O’Connor CM. The pathophysiology of acute heart failure–is it all about fluid accumulation? Am Heart J. (2008) 155(1):9–18. doi: 10.1016/j.ahj.2006.02.038
36. Pugliese NR, Pellicori P, Filidei F, et al. Inflammatory pathways in heart failure with preserved left ventricular ejection fraction: implications for future interventions. Cardiovasc Res. (2023) 118(18):3536–55. doi: 10.1093/cvr/cvac133
37. Kalogeropoulos AP, Tang WHW, Hsu A, Felker GM, Hernandez AF, Troughton RW, et al. High-sensitivity C-reactive protein in acute heart failure: insights from the ASCEND-HF trial. J Card Fail. (2014) 20(5):319–26. doi: 10.1016/j.cardfail.2014.02.002
38. Matsumoto H, Kasai T, Sato A, Ishiwata S, Yatsu S, Shitara J, et al. Association between C-reactive protein levels at hospital admission and long-term mortality in patients with acute decompensated heart failure. Heart Vessels. (2019) 34(12):1961–8. doi: 10.1007/s00380-019-01435-9
39. Minami Y, Kajimoto K, Sato N, Hagiwara N, Takano T, Study Investigators ATTEND. C-reactive protein level on admission and time to and cause of death in patients hospitalized for acute heart failure. Eur Heart J Qual Care Clin Outcomes. (2017) 3(2):148–56. doi: 10.1093/ehjqcco/qcw054
40. Miró Ò, Takagi K, Davison BA, Edwards C, Freund Y, Jacob J, et al. Effect of systemic corticosteroid therapy for acute heart failure patients with elevated C-reactive protein. ESC Heart Fail. (2022) 9(4):2225–32. doi: 10.1002/ehf2.13926
41. Gabay C. Interleukin-6 and chronic inflammation. Arthritis Res Ther. (2006) 8(Suppl 2):S3. doi: 10.1186/ar1917
42. Ridker PM. From C-reactive protein to interleukin-6 to interleukin-1: moving upstream to identify novel targets for atheroprotection. Circ Res. (2016) 118(1):145–56. doi: 10.1161/CIRCRESAHA.115.306656
43. Van Linthout S, Tschöpe C. Inflammation—cause or consequence of heart failure or both? Curr Heart Fail Rep. (2017) 14(4):251–65. doi: 10.1007/s11897-017-0337-9
44. Eskandari V, Amirzargar AA, Mahmoudi MJ, Rahnemoon Z, Rahmani F, Sadati S, et al. Gene expression and levels of IL-6 and TNFα in PBMCs correlate with severity and functional class in patients with chronic heart failure. Ir J Med Sci. (2018) 187(2):359–68. doi: 10.1007/s11845-017-1680-2
45. Michou E, Wussler D, Belkin M, Simmen C, Strebel I, Nowak A, et al. Quantifying inflammation using interleukin-6 for improved phenotyping and risk stratification in acute heart failure. Eur J Heart Fail. (2023) 25(2):174–84. doi: 10.1002/ejhf.2767
46. Perez AL, Grodin JL, Chaikijurajai T, O'Connor C, Starling RC, Wilson Tang WH, et al. Interleukin-6 and outcomes in acute heart failure: an ASCEND-HF substudy. J Card Fail. (2021) 27(6):670–6. doi: 10.1016/j.cardfail.2021.01.006
47. Markousis-Mavrogenis G, Tromp J, Mentz RJ, O'Connor CM, Metra M, Ponikowski P, et al. The additive prognostic value of serial plasma interleukin-6 levels over changes in brain natriuretic peptide in patients with acute heart failure. J Card Fail. (2021) 27(7):808–11. doi: 10.1016/j.cardfail.2021.01.008
48. Aimo A, Januzzi JL, Bayes-Genis A, Vergaro G, Sciarrone P, Passino C, et al. Clinical and prognostic significance of sST2 in heart failure. J Am Coll Cardiol. (2019) 74(17):2193–203. doi: 10.1016/j.jacc.2019.08.1039
49. Coglianese EE, Larson MG, Vasan RS, Ho JE, Ghorbani A, McCabe EL, et al. Distribution and clinical correlates of the interleukin receptor family member soluble ST2 in the framingham heart study. Clin Chem. (2012) 58(12):1673–81. doi: 10.1373/clinchem.2012.192153
50. Weinberg EO, Shimpo M, De Keulenaer GW, MacGillivray C, Tominaga SI, Solomon SD, et al. Expression and regulation of ST2, an interleukin-1 receptor family member, in cardiomyocytes and myocardial infarction. Circulation. (2002) 106(23):2961–6. doi: 10.1161/01.cir.0000038705.69871.d9
51. Van Tassell BW, Canada J, Carbone S, Trankle C, Buckley L, Oddie Erdle C, et al. Interleukin-1 blockade in recently decompensated systolic heart failure: results from REDHART (recently decompensated heart failure anakinra response trial). Circ Heart Fail. (2017) 10(11):e004373. doi: 10.1161/CIRCHEARTFAILURE.117.004373
52. Van Tassell BW, Abouzaki NA, Oddi Erdle C, Carbone S, Trankle C, Melchior R, et al. Interleukin-1 blockade in acute decompensated heart failure: a randomized, double-blinded, placebo-controlled pilot study. J Cardiovasc Pharmacol. (2016) 67(6):544–51. doi: 10.1097/FJC.0000000000000378
53. Pascual-Figal DA, Bayes-Genis A, Asensio-Lopez MC, Hernandez-Vincente A, Garrido-Bravo I, Pastor-Perez F, et al. The interleukin-1 axis and risk of death in patients with acutely decompensated heart failure. J Am Coll Cardiol. (2019) 73(9):1016–25. doi: 10.1016/j.jacc.2018.11.054
54. De Boer RA, Yu L, Van Veldhuisen DJ. Galectin-3 in cardiac remodeling and heart failure. Curr Heart Fail Rep. (2010) 7(1):1–8. doi: 10.1007/s11897-010-0004-x
55. Ali M, Pulli B, Courties G, Tricot B, Sebas M, Iwamoto Y, et al. Myeloperoxidase inhibition improves ventricular function and remodeling after experimental myocardial infarction. JACC Basic Transl Sci. (2016) 1(7):633–43. doi: 10.1016/j.jacbts.2016.09.004
56. Lourenço P, Cunha FM, Ferreira-Coimbra J, Barroso I, Guimarães J, Bettencourt P. Dynamics of growth differentiation factor 15 in acute heart failure. ESC Heart Fail. (2021) 8(4):2527–34. doi: 10.1002/ehf2.13377
57. Aimo A, Castiglione V, Borrelli C, Saccaro LF, Franzini M, Masi S, et al. Oxidative stress and inflammation in the evolution of heart failure: from pathophysiology to therapeutic strategies. Eur J Prev Cardiol. (2020) 27(5):494–510. doi: 10.1177/2047487319870344
58. Mapelli M, Mattavelli I, Salvioni E, Banfi C, Ghilardi S, De Martino F, et al. Impact of sacubitril/valsartan on surfactant binding proteins, central sleep apneas, lung function tests and heart failure biomarkers: hemodynamic or pleiotropism? Front Cardiovasc Med. (2022) 9:971108. doi: 10.3389/fcvm.2022.971108
59. Brioschi M, D'Alessandra Y, Mapelli M, Mattavelli I, Salvioni E, Eligini S, et al. Impact of sacubitril/valsartan on circulating microRNA in patients with heart failure. Biomedicines. (2023) 11(4):1037. doi: 10.3390/biomedicines11041037
60. Campodonico J, Mapelli M, Spadafora E, Ghilardi S, Agostoni P, Banfi C, et al. Surfactant proteins changes after acute hemodynamic improvement in patients with advanced chronic heart failure treated with levosimendan. Respir Physiol Neurobiol. (2018) 252-253:47–51. doi: 10.1016/j.resp.2018.03.007
61. Aimo A, Bayes-Genis A. Biomarkers of inflammation in heart failure: from risk prediction to possible treatment targets. Eur J Heart Fail. (2023) 25(2):161–2. doi: 10.1002/ejhf.2771
62. van Wezenbeek J, Canada JM, Ravindra K, Carbone S, Trankle CR, Kadariya D, et al. C-reactive protein and N-terminal pro-brain natriuretic peptide levels correlate with impaired cardiorespiratory fitness in patients with heart failure across a wide range of ejection fraction. Front Cardiovasc Med. (2018) 5:178. doi: 10.3389/fcvm.2018.00178
63. Lakhani I, Wong MV, Hung JKF, Gong M, Waleed KB, Xia Y, et al. Diagnostic and prognostic value of serum C-reactive protein in heart failure with preserved ejection fraction: a systematic review and meta-analysis. Heart Fail Rev. (2021) 26(5):1141–50. doi: 10.1007/s10741-020-09927-x
64. He G, Ji R, Huo X, Su X, Ge J, Li W, et al. Long-term trajectories of high-sensitivity C-reactive protein level among patients with acute heart failure. J Inflamm Res. (2023) 16:359–71. doi: 10.2147/JIR.S387534
65. Lourenço P, Paulo Araújo J, Paulo C, Mascarenhas J, Frioes F, Azevedo A, et al. Higher C-reactive protein predicts worse prognosis in acute heart failure only in noninfected patients. Clin Cardiol. (2010) 33(11):708–14. doi: 10.1002/clc.20812
66. Hanberg JS, Rao VS, Ahmad T, Chunara Z, Mahoney D, Jackson K, et al. Inflammation and cardio-renal interactions in heart failure: a potential role for interleukin-6. Eur J Heart Fail. (2018) 20(5):933–4. doi: 10.1002/ejhf.963
67. Suzuki H, Sato R, Sato T, Shoji M, Iso Y, Kondo T, et al. Time-course of changes in the levels of interleukin 6 in acutely decompensated heart failure. Int J Cardiol. (2005) 100(3):415–20. doi: 10.1016/j.ijcard.2004.08.041
68. Gwechenberger M, Hülsmann M, Berger R, Graf S, Springer C, Stanek B, et al. Interleukin-6 and B-type natriuretic peptide are independent predictors for worsening of heart failure in patients with progressive congestive heart failure. J Heart Lung Transplant Off Publ Int Soc Heart Transplant. (2004) 23(7):839–44. doi: 10.1016/j.healun.2003.07.023
69. Miettinen KH, Lassus J, Harjola VP, Siirila-Waris K, Melin J, Punnonen KR, et al. Prognostic role of pro- and anti-inflammatory cytokines and their polymorphisms in acute decompensated heart failure. Eur J Heart Fail. (2008) 10(4):396–403. doi: 10.1016/j.ejheart.2008.02.008
70. Januzzi JL, Peacock WF, Maisel AS, Chae CU, Jesse RL, Baggish AL, et al. Measurement of the interleukin family member ST2 in patients with acute dyspnea: results from the PRIDE (pro-brain natriuretic peptide investigation of dyspnea in the emergency department) study. J Am Coll Cardiol. (2007) 50(7):607–13. doi: 10.1016/j.jacc.2007.05.014
71. van Kimmenade RR, Januzzi JL, Ellinor PT, Sharma UC, Bakker JA, Low AF, et al. Utility of amino-terminal pro-brain natriuretic peptide, galectin-3, and apelin for the evaluation of patients with acute heart failure. J Am Coll Cardiol. (2006) 48(6):1217–24. doi: 10.1016/j.jacc.2006.03.061
72. Shah RV, Chen-Tournoux AA, Picard MH, van Kimmenade RRJ, Januzzi JL. Galectin-3, cardiac structure and function, and long-term mortality in patients with acutely decompensated heart failure. Eur J Heart Fail. (2010) 12(8):826–32. doi: 10.1093/eurjhf/hfq091
73. Reichlin T, Socrates T, Egli P, Potocki M, Breidthardt T, Arenja N, et al. Use of myeloperoxidase for risk stratification in acute heart failure. Clin Chem. (2010) 56(6):944–51. doi: 10.1373/clinchem.2009.142257
74. Cotter G, Voors AA, Prescott MF, Felker GM, Filippatos G, Greenberg BH, et al. Growth differentiation factor 15 (GDF-15) in patients admitted for acute heart failure: results from the RELAX-AHF study. Eur J Heart Fail. (2015) 17(11):1133–43. doi: 10.1002/ejhf.331
75. Bettencourt P, Ferreira-Coimbra J, Rodrigues P, Marques P, Moreira H, Joao Pinto M, et al. Towards a multi-marker prognostic strategy in acute heart failure: a role for GDF-15. ESC Heart Fail. (2018) 5(6):1017–22. doi: 10.1002/ehf2.12301
76. Zeillemaker AM, Verbrugh HA, van Papendrecht AA H, Leguit P. CA 125 secretion by peritoneal mesothelial cells. J Clin Pathol. (1994) 47(3):263–5. doi: 10.1136/jcp.47.3.263
77. Colombo PC, Onat D, Harxhi A, Demmer RT, Hayashi Y, Jelic S, et al. Peripheral venous congestion causes inflammation, neurohormonal, and endothelial cell activation. Eur Heart J. (2014) 35(7):448–54. doi: 10.1093/eurheartj/eht456
78. Markousis-Mavrogenis G, Baumhove L, Al-Mubarak AA, Aboumsallem JP, Bomer N, Voors AA, et al. Immunomodulation and immunopharmacology in heart failure. Nat Rev Cardiol. (2023). Published online September 14. doi: 10.1038/s41569-023-00919-6
79. Deftereos S, Giannopoulos G, Papoutsidakis N, Panagopoulou V, Kossyvakis C, Raisakis K, et al. Colchicine and the heart: pushing the envelope. J Am Coll Cardiol. (2013) 62(20):1817–25. doi: 10.1016/j.jacc.2013.08.726
80. Roth ME, Chinn ME, Dunn SP, Bilchick KC, Mazimba S. Association of colchicine use for acute gout with clinical outcomes in acute decompensated heart failure. Clin Cardiol. (2022) 45(7):733–41. doi: 10.1002/clc.23830
81. Zhang H, Liu C, Ji Z, Liu G, Zhao Q, Ao YG, et al. Prednisone adding to usual care treatment for refractory decompensated congestive heart failure. Int Heart J. (2008) 49(5):587–95. doi: 10.1536/ihj.49.587
82. Liu C, Liu K, COPE-ADHF Study Group. Cardiac outcome prevention effectiveness of glucocorticoids in acute decompensated heart failure: cOPE-ADHF study. J Cardiovasc Pharmacol. (2014) 63(4):333–8. doi: 10.1097/FJC.0000000000000048
83. Miró Ò, Takagi K, Gayat E, Llorens P, Martin-Sanchez FJ, Jacob J, et al. CORT-AHF Study: effect on outcomes of systemic corticosteroid therapy during early management acute heart failure. JACC Heart Fail. (2019) 7(10):834–45. doi: 10.1016/j.jchf.2019.04.022
84. Parissis JT, Adamopoulos S, Antoniades C, Kostakis G, Rigas A, Kyrzopoulos S, et al. Effects of levosimendan on circulating pro-inflammatory cytokines and soluble apoptosis mediators in patients with decompensated advanced heart failure. Am J Cardiol. (2004) 93(10):1309–12. doi: 10.1016/j.amjcard.2004.01.073
Keywords: heart failure, acute heart failure (AHF), inflammation, systemic inflammation, anti-inflammatory therapy
Citation: Garofalo M, Corso R, Tomasoni D, Adamo M, Lombardi CM, Inciardi RM, Gussago C, Di Mario C, Metra M and Pagnesi M (2023) Inflammation in acute heart failure. Front. Cardiovasc. Med. 10:1235178. doi: 10.3389/fcvm.2023.1235178
Received: 5 June 2023; Accepted: 30 October 2023;
Published: 17 November 2023.
Edited by:
Jeroen Dauw, AZ Sint-Lucas, BelgiumReviewed by:
Vincenzo Castiglione, Sant'Anna School of Advanced Studies, ItalyMassimo Mapelli, Monzino Cardiology Center (IRCCS), Italy
Lukas Baumhove, University Medical Center Groningen, Netherlands
© 2023 Garofalo, Corso, Tomasoni, Adamo, Lombardi, Inciardi, Gussago, Di Mario, Metra and Pagnesi. This is an open-access article distributed under the terms of the Creative Commons Attribution License (CC BY). The use, distribution or reproduction in other forums is permitted, provided the original author(s) and the copyright owner(s) are credited and that the original publication in this journal is cited, in accordance with accepted academic practice. No use, distribution or reproduction is permitted which does not comply with these terms.
*Correspondence: Marco Metra marco.metra@unibs.it Matteo Pagnesi m.pagnesi@gmail.com
†These authors have contributed equally to this work