- 1Department of Cardiology, Inselspital, Bern University Hospital, University of Bern, Bern, Switzerland
- 2Department of Cardiology, Bispebjerg Frederiksberg University Hospital, Copenhagen, Denmark
- 3Diagram B.V., Zwolle, Netherlands
- 4Isala Heart Centre, Zwolle, Netherlands
- 5Department of Cardiology, Hospital Clínico Universitario de Santiago, University of Santiago de Compostela, Santiago de Compostela, Spain
- 6Department of Cardiac Rehabilitation, Assistance Publique Hopitaux de Paris, Paris, France
- 7Department of Cardiology, Parma University Hospital, Parma, Italy
- 8Klinikum Ludwigshafen and Institut für Herzinfarktforschung Ludwigshafen, Ludwigshafen, Germany
- 9Department of Cardiology, Radboud University, Nijmegen, Netherlands
- 10Department of Cardiology, Maastricht University Medical Center, Maastricht, Netherlands
- 11Department of Cardiology, Zuyderland Medical Center, Heerlen, Netherlands
Background: Inefficient ventilation is an established prognostic marker in patients with heart failure. It is not known whether inefficient ventilation is also linked to poor prognosis in patients with left ventricular dysfunction (LVD) but without overt heart failure.
Objectives: To investigate whether inefficient ventilation in elderly patients with LVD is more common than in patients without LVD, whether it improves with exercise-based cardiac rehabilitation (exCR), and whether it is associated with major adverse cardiovascular events (MACE).
Methods: In this large multicentre observational longitudinal study, patients aged ≥65 years with acute or chronic coronary syndromes (ACS, CCS) without cardiac surgery who participated in a study on the effectiveness of exCR in seven European countries were included. Cardiopulmonary exercise testing (CPET) was performed before, at the termination of exCR, and at 12 months follow-up. Ventilation (VE), breathing frequency (BF), tidal volume (VT), and end-expiratory carbon dioxide pressure (PETCO2) were measured at rest, at the first ventilatory threshold, and at peak exercise. Ventilatory parameters were compared between patients with and without LVD (based on cardio-echography) and related to MACE at 12 month follow-up.
Results: In 818 patients, age was 72.5 ± 5.4 years, 21.9% were women, 79.8% had ACS, and 151 (18%) had LVD. Compared to noLVD, in LVD resting VE was increased by 8%, resting BF by 6%, peak VE, peak VT, and peak PETCO2 reduced by 6%, 8%, and 5%, respectively, and VE/VCO2 slope increased by 11%. From before to after exCR, resting VE decreased and peak PETCO2 increased significantly more in patients with compared to without LVD. In LVD, higher resting BF, higher nadir VE/VCO2, and lower peak PETCO2 at baseline were associated with MACE.
Conclusions: Similarly to patients with HF, in elderly patients with ischemic LVD, inefficient resting and exercise ventilation was associated with worse outcomes, and ExCR alleviated abnormal breathing patterns and gas exchange parameters.
Introduction
Ischemic heart disease is the most prevalent risk factor for left ventricular dysfunction (LVD) and chronic heart failure (HF), both for women and men (1). Impaired pulmonary gas exchange, quantified as an increased ventilation (VE) to carbon dioxide exhalation (VCO2) slope during exercise, and low end-tidal pressure of carbon dioxide (PETCO2) are landmarks of heart failure patients (2, 3). These parameters received attention when several studies found higher VE/VCO2 slopes and lower PETCO2 to be associated with poorer prognosis (4–6). The components of the VE/VCO2 slope are the arterial CO2 partial pressure (PaCO2), which in CHF is affected mainly by ventilation, and the pulmonary dead space to tidal volume ration (VD/VT) mainly by pulmonary perfusion abnormalities (3, 7). Exercise hyperventilation is a hallmark of a failing heart (2). Several mechanisms have been proposed as mediators of this excessive ventilatory response, including: (1) alveolar ventilation-perfusion mismatching, (2) increased humoral stimuli (e.g., lactate and H+) due to skeletal muscle hypoperfusion and deconditioning, (3) juxta-capillary receptor stimulation consequent to pulmonary vascular congestion and/or hypertension, (4) augmented central and peripheral chemosensitivity, and (5) an inordinately high degree of afferent neural traffic originating from within the locomotor muscles (i.e., the ergoreflex or “skeletal muscle” hypothesis). Hyperventilation is well known to stimulate sympathetic nervous activity (8). Chronic sympathetic nervous hyperactivity, in turn, may decrease the aerobic capacity of skeletal muscles by reducing capillarisation (9) and red blood cell flux (10), which lead to a shift in muscle fibre type towards a lower content on type I fibres (11). The ensuing anaerobic muscle metabolism leads to increased muscle fatiguability (12) and acidosis already at low levels of exercise, which trigger exaggerated responses in ventilation (13). While exaggerated ventilation has been found to be a strong predictor of mortality and adverse cardiac events in patients with established heart failure, this has not been assessed in patients with LVD or with ischemic heart disease (IHD). There is a continuum from patients with IHD to LVD and HF (14), with asymptomatic LVD being twice as common as HF (15). However, inefficient ventilation has not been assessed as a prognostic marker in patients with IHD or LVD.
Comprehensive exercise-based cardiac rehabilitation (exCR) has the potential to improve cardiorespiratory fitness (CRF) and quality of life (16), cardiovascular risk factors (17), and reduce hospitalisations and cardiovascular mortality (17, 18). In HF patients with reduced ejection fraction, studies assessing the effects of exercise have focussed largely on circulatory parameters (19), peak oxygen uptake (VO2) and muscle strength (20). Some studies have also reported changes in ventilatory efficiency in response to exercise during exCR (21, 22). However, data in elderly patients with LVD are sparse and no data exists on how exCR influences breathing patterns at rest.
The purpose of the present study in elderly patients with and without ischemic LVD was to (1) describe breathing patterns and pulmonary gas exchange parameters at rest and during exercise; (2) assess the association of exCR with a change of these parameters; and (3) assess the prognostic value of these parameters on major adverse cardiac events (MACE). We hypothesised that ventilation would be less efficient in patients with compared to those without LVD, and that, similarly to patients with HF, exCR would improve ventilatory efficiency, and that ventilatory inefficiency would be associated with MACE.
Methods
The EU-CaRE observational longitudinal study was a European project focusing on the effectiveness and sustainability of exCR programs in the elderly (65 years or above). EU-CaRE was conducted from 2016 to 2019 and involved eight participating CR sites in seven countries, with five offering ambulatory CR of 6–12 weeks duration (Denmark, Italy, the Netherlands, Spain, and Switzerland) and two offering stationary CR of 3–4 weeks duration (France, Germany) (23).
Study population, assessments, and intervention
Inclusion and exclusion criteria of the study population and outcome data, such as peak oxygen uptake, smoking, body mass index, diet, physical activity, serum lipids, psychological distress, and medication have been reported previously (24–28). Briefly, the inclusion criteria were patients ≥65 years old with coronary heart disease (including also valve surgery patients who were not considered for the present study). For the present study, only data from patients with acute or chronic coronary syndromes (ACS/CCS) with or without percutaneous coronary intervention (PCI) were included. Exclusion criteria were valve and cardiovascular surgery. Patients after open-chest surgery were excluded because they were found to have much greater recoveries in power output, peak oxygen consumption, and ventilatory efficiency than patients after PCI. Further, patients with open heart surgery had a lower percentage of MACE than patients after PCI (27). Patients were assessed at baseline before commencing exCR (T0), after completing the exCR program (T1), and at 1-year follow-up (T2). Detailed information about the exCR programs of the different centres was given elsewhere (23) and training intensity of the endurance training sessions of each centre have also been analysed previously (26). They were grouped according to left ventricular ejection fraction (LVEF) determined by the patient's most recent echocardiography into patients with LVD (LVEF < 45%), vs. those without LVD (noLVD, LVEF ≥ 45%). At the time when this study was designed, this cut-off was one of the more widely used and accepted cut-offs for systolic LVD and HF with reduced LVEF (HFrEF) (29).
The study was approved by all relevant medical ethics committees, and registered at trialregister.nl (NTR5306). The participants gave written informed consent before they were included in the study.
Data collection of predictors and outcomes
Recorded information included demographics, index event, socioeconomic factors, medical history including co-mobidities and cardiovascular risk factors, and clinical information such as weight, blood pressure (BP), resting heart rate (HR), medication, SF36 quality of life questionnaire, and patient-reported physical activity as number of days per week with at least moderate physical activity of minimally 30 min. Details on the collected data have been provided elsewhere (24, 30, 31).
LVEF was determined by standard two-dimensional transthoracic echocardiography performed by the different hospital echocardiography systems. LV end-diastolic (LVEDV), and LV end-systolic volumes (LVESV) were calculated using the biplane method of disks summation (modified Simpson's rule) (32).
CRF and breathing parameters were assessed by cardiopulmonary exercise testing (CPET). After reporting to the laboratory, patients rested supine for 10–15 min during which a resting 12-lead electrocardiogram (ECG) was performed. Then patients stood up and performed a resting spirometry with the determination of forced vital capacity (FVC) and forced expiratory volume during the first second (FEV1). After this, patients mounted the cycle ergometers and were fitted with a facemask. Then they remained sitting quietly for 3 min during which heart rate, blood pressure, and breathing parameters were measured. As an average of the last minute of the 3-min resting period, while sitting on the ergometer, the following parameters were determined: minute ventilation (VE), breathing frequency (BF), tidal volume (VT), partial pressure of carbon dioxide (PETCO2), and heart rate. After a 3-min warm-up at 5 Watt an individual ramp was chosen to achieve a test duration of 8–12 min until exhaustion and kept constant in follow-up tests. Data were analysed at the CPET core lab in Bern as previously described (27, 31). During the ramp, the VE/VCO2 slope, and the nadir of the VE/VCO2 ratio were determined. Oxygen consumption (VO2), VE, BF, VT, PETCO2, and heart rate were determined at the first ventilatory threshold as previously described (27). Peak values of the same parameters were determined as the highest value of a 30 s moving average. Ventilatory parameters were excluded when the respiratory quotient was below 0.7 at resting and below 0.8 at peak exercise due to the suspected presence of mask leakage.
MACE was defined as the combined incidence of all-cause mortality, ACS, cardiac-related emergency visit, hospitalisation for cardiac reasons, near sudden cardiac death, and cardiac intervention. MACE was recorded by monthly telephone calls and assessed individually by an independent Clinical Event Committee (27).
Statistical analysis
All statistics were performed with R (Version 4.1.0, R Core Team, 2021). Changes in ventilatory and circulatory parameters were calculated between T0 and T1. Mixed linear models were performed for ventilatory parameters VE, BF, VT, and PETCO2 at rest and peak exercise, as well as VE/VCO2 slope and peak VO2 relative to body weight and tested for interaction effects between time points T0, T1, and T2 and group (with and without LVD). All models were adjusted for age, sex, and body mass index (BMI), and patients nested within centres were entered as random factors (intercepts). We chose mixed linear models because they can handle the presence of missing data and allow assessment of longitudinal data and adjustment for confounders, such as centres, age, sex, and BMI.
The association of ventilatory parameters at rest and during exercise with MACE was also assessed with mixed linear models for patients with and without LVD and with and without MACE. For all models, the alpha was set at 0.05.
Results
Of 1,633 enrolled EU-CaRE patients, 986 patients had an ACS or CCS with percutaneous coronary intervention or no revascularisation. Of these, 79.8% had an ACS and 90.2% a PCI as an indication for exCR. LVEF was known in 912 patients. Of these, valid CPET at T0 was available in 867 patients of whom 707 had no LVD and 166 had LVD. Amongst these, data on breathing patterns were available from at least one time point in 151 with and 667 patients without LVD (Figure 1). The baseline data of the two patient groups are shown in Table 1. Patients with LVD were older, were less often women, and had lower BMI and FVC relative to body surface area. An equal prevalence of 86% of patients with New York Heart Association (NYHA) functional class I existed in patients with and without LVD, and NYHA III class was present in 2% and 3% of patients with and without LVD, respectively. Patients with LVD had significantly lower physical component scores of the SF36 indicating poorer health status. Patients with LVD had a higher percentage of pre-existing chronic heart failure and anaemia, and a higher prescription rate of beta-blockers and ACE inhibitors or ARBs.
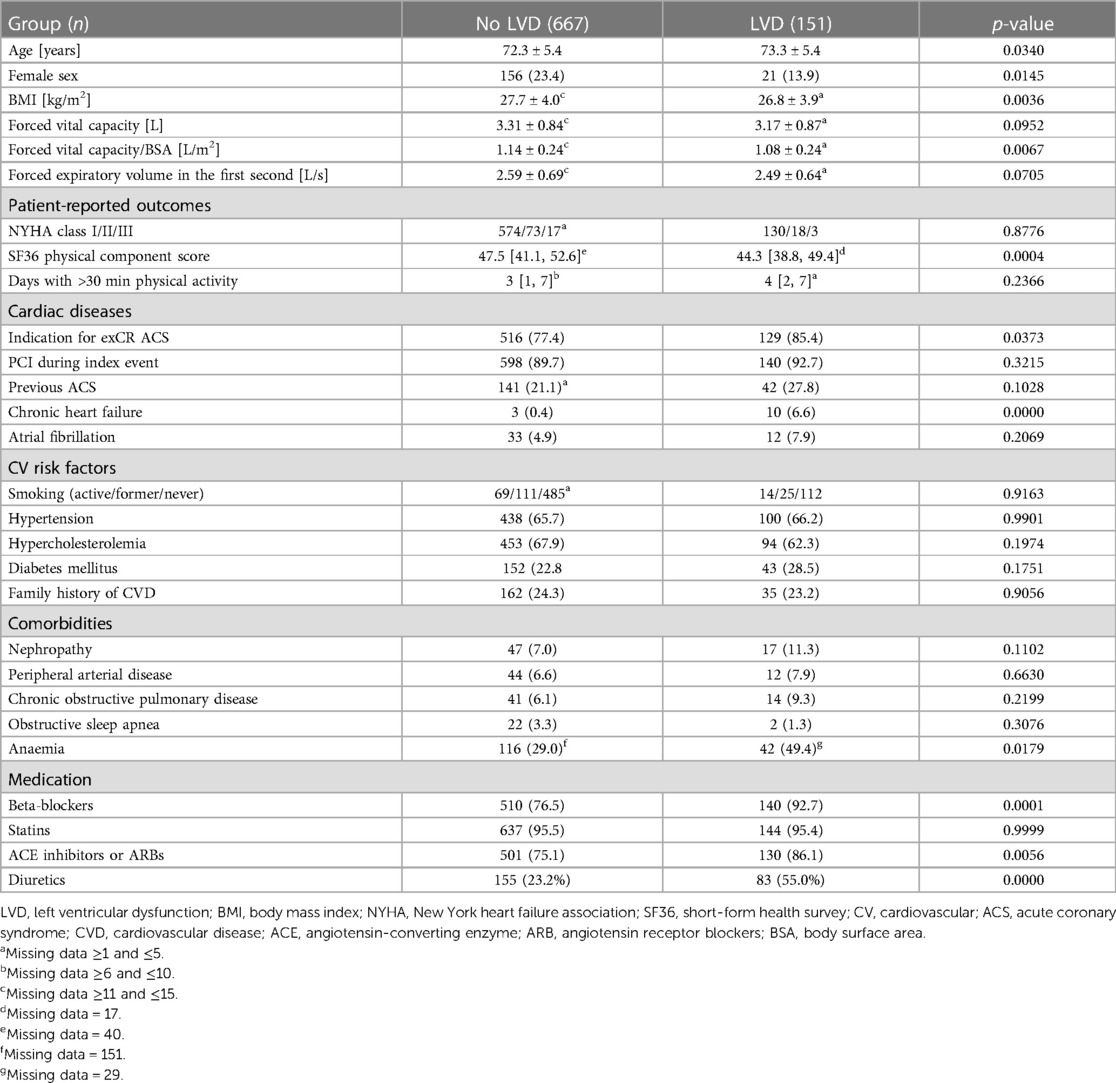
Table 1. Baseline characteristics of patients without vs. with LVD. Parameters are indicated as mean ± SD, n (%), or median [1st, 3rd quartile]. P-values are derived from unpaired T-tests, Wilcoxon tests, Chi-square or Fisher’s exact test, as appropriate.
With regard to ventilatory parameters, two centres did not record data on BF and VT, therefore, these parameters were missing in 5 and 51 patients with and without LVD, respectively. Due to an insufficient ventilation monitoring duration (≤1 min) during the 3-min resting phase, VE and PETCO2 at rest were missing in 9 and 68 patients with and without LV dysfunction at T0, respectively, in 6 and 82 at T1, respectively, and in 4 and 46 at T2, respectively. The first ventilatory threshold could not be determined in 38 and 130 patients with and without LV dysfunction, respectively, at T0, in 20 and 71 at T1, respectively, and in 16 and 61 at T2, respectively. The largest relative adjusted (for sex, age, and BMI) differences of the LVD group compared to the noLVD group at baseline were found for VO2 peak (−12.6%), VE/VCO2 slope (11.0%), nadir VE/VCO2 (8.8%), and resting VE (8.1%) (Table 2).
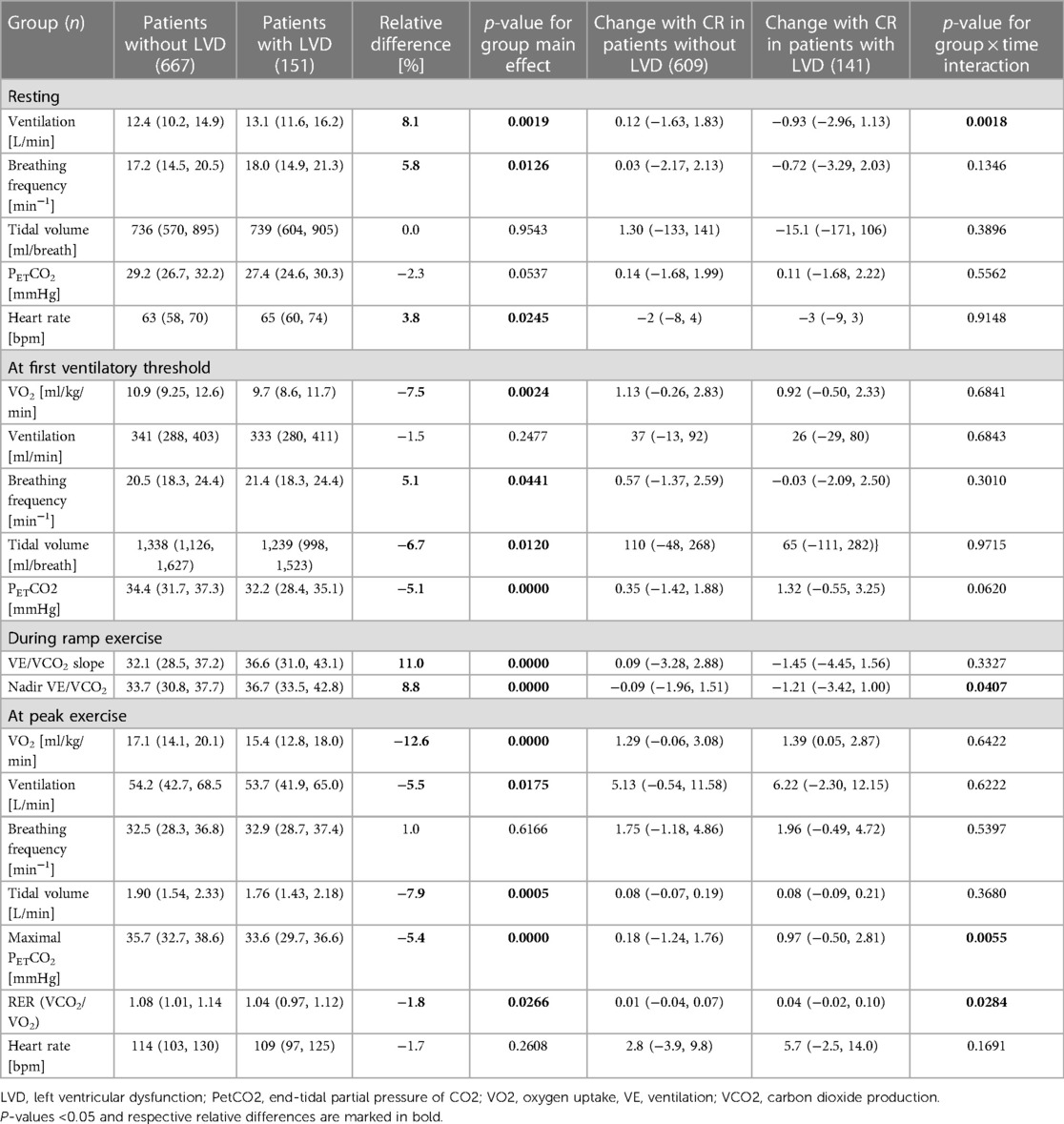
Table 2. Ventilatory and circulatory parameters at rest, during ramp exercise (at first ventilatory threshold), and at peak exercise for patients without vs. patients with LVD before the start of exCR as well as change until completion of CR. Shown are medians and first and third quartiles in round brackets. The relative difference between groups is derived from group effect from adjusted models and is relative to values from groups without LVD. P-values are derived from mixed linear models adjusted for age, sex, and body mass index and with patients nested within centres as random intercepts.
Regarding the changes in ventilatory parameters from before to after CR, patients with LVD had significantly greater reductions in resting VE (Table 2). They also had greater reductions in nadir VE/VCO2, and a greater increase in PETCO2 and RER at peak exercise.
Results from the mixed linear models including data also for the 1-year follow-up measurement as well as confounding factors age, sex, and BMI showed a significantly greater reduction of VE at rest in the group of patients with LVD not only from before to after exCR but also to 1-year follow-up (Figure 2, Supplementary Table S1). BF at rest was higher in patients with LVD at all time points (Figure 2, Supplementary Table S1), and resting PETCO2 increased significantly at 1-year follow-up in both groups (Figure 3, Supplementary Table S1). At peak exercise, VE was reduced in the patients with LVD due to a significantly lower VT in the patients with LVD but increased with exCR and to 1-year follow-up in both groups (Figure 2, Supplementary Table S2). Resting PETCO2 was lower by trend (p = 0.0537) in patients with LVD and significantly lower at peak exercise at all time points (Figure 3, Supplementary Tables S1 and S2). Accordingly, VE/VCO2 slope was increased in patients with LVD at all time points (Figure 3, Supplementary Table S3). VO2 relative to body weight was greatly reduced in patients with LVD at peak exercise (Figure 3, Supplementary Table S3) at all time points. Peak VO2 increased in both groups from before to after exCR and VE/VCO2 slope decreased over time. The physical component score was consistently lower by 3 points in patients with LVD but significant consistent improvements in SF36 PCS between time points were comparable in both groups with nearly 3 points between T0 and T2 (Supplementary Table S3).
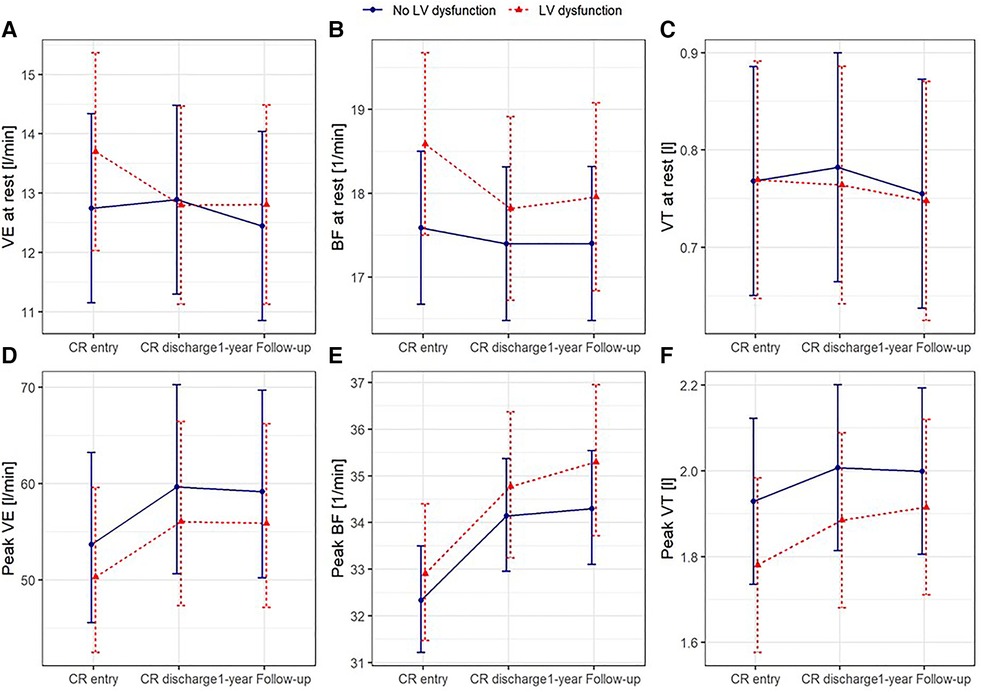
Figure 2. Predicted means with 95% confidence intervals based on the mixed linear models for ventilation (A), breathing frequency (B), and tidal volume (C) at rest and at peak exercise (D–F) adjusted for age, sex, and body mass index, with patients nested within centres as random intercepts.
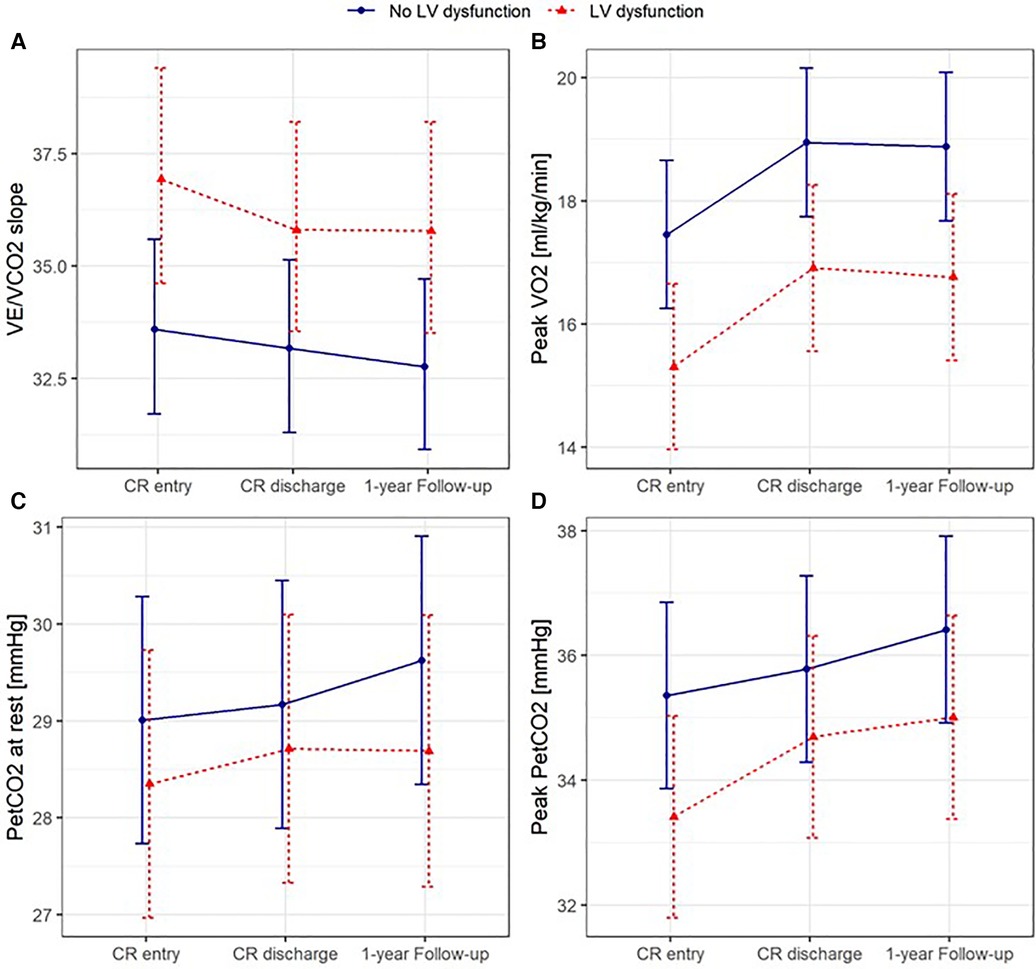
Figure 3. Predicted means with 95% confidence intervals based on the mixed linear models for VE/VCO2 slope (A), peak VO2 (B), and end-tidal carbon dioxide partial pressure at rest (C) and at peak exercise (D) adjusted for age, sex, and body mass index, with patients nested within centres as random intercepts.
Approximately 20% of patients with and without LVD had a MACE within 1-year follow-up with no difference between groups (p-value for Chi-square test 0.069). Nadir of VE/VCO2 significantly discriminated patients with and without MACE in patients with and without LVD, while VE/VCO2 slope and peak VO2 discriminated for MACE only in patients without LVD, and peak PETCO2 and resting BF discriminated for MACE only in patients with LVD (Table 3).
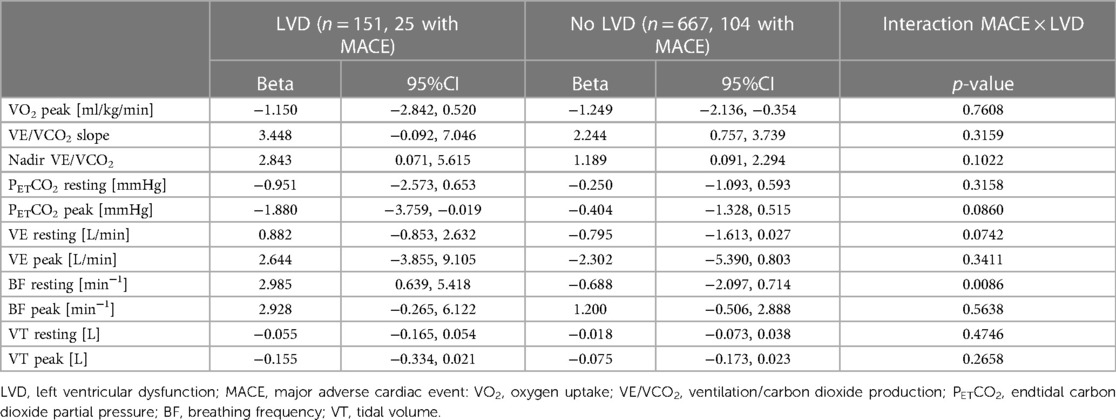
Table 3. Association of MACE with CPET parameter. Shown are estimates and 95% CI for MACE from the mixed linear models with the respective CPET parameter as the dependent variable and covariates age and sex, and with site as random factor. Models were performed for patients with and without LVD and for the total population to test for group interaction.
Discussion
This study provides four clinically relevant and novel aspects in a representative European cohort of elderly patients with coronary artery disease undergoing exCR: (1) Nearly one-fifth of patients had asymptomatic LVD at the start of exCR; (2) LVD was associated with elevated ventilation at rest and impaired pulmonary gas exchange during exercise; (3) exCR was associated with an improvement of ventilation at rest and gas exchange parameters during exercise, but ventilatory efficiency and PETCO2 remained significantly reduced in patients with LVD compared to patients without LVD in the 1-year follow-up; and (4) BF at rest, ventilatory efficiency and PETCO2 during exercise were associated with worse outcome in patients with LVD.
This is the first large study demonstrating increased resting ventilation and impaired pulmonary gas exchange during exercise in patients with LVD without major signs and symptoms of congestive heart failure. NYHA functional class did not differ between patients with and without LVD and 86% of patients in both groups were in NYHA functional class I. Furthermore, self-reported physical activity did not differ between the groups. Although the median physical component score of the SF36 questionnaire was 3 points lower in patients with LVD compared to patients without LVD, the values of 44.3 at T0 and 47.5 at T2 were clearly above the median of 35.3 found in patients with chronic heart failure (33).
We suggest that signs of an exaggerated ventilatory drive are already apparent in patients with LVD at rest (higher resting VE and BF), during exercise (increased VE/VCO2 slope and nadir VE/VCO2), and at peak exercise (lower VO2, VT, and PETCO2). Reductions of the exaggerated ventilatory drive seen with exCR were similarly beneficial in patients with and without LVD. The decrease in nadir VE/VCO2 was significantly greater in patients with LVD than in those without LVD, with a similar magnitude of the difference between changes in VE/VCO2 slope, albeit not reaching statistical significance. This is in accordance with some previous studies that found ventilatory efficiency to improve most in patients with the most severe ventilatory inefficiency at baseline. For example, in a study with 131 patients after acute myocardial infarction without HF participating in exCR, VE/VCO2 slope improved most in patients with VE/VCO2 slope > 32 (34), which was also confirmed by a study comparing the effect of aerobic training to a combination of aerobic and strength training (35). Similarly, a study in patients with HFrEF by Servantes et al. found large decreases in VE/VCO2 slopes with mean pre-exercise values of 36 (36). In part, a larger decrease in patients with higher starting values may be due to a phenomenon referred to as “regression to the mean” (37). In a study including 123 CAD patients, patients in the group with peak VO2 < 17.5 ml/kg/min also had the highest nadir VE/VCO2 (38). This group also had the highest improvement in peak VO2 and nadir VE/VCO2 after exCR. Likewise, in 37 CAD patients completing exCR, PETCO2 at the first and second ventilatory threshold and peak exercise were improved after the programme. On the contrary, Fu et al. did not demonstrate an improvement of the VE/VCO2 slope with exercise training in patients with HFrEF and a VE/VCO2 slope of 35 at baseline (21).
At peak exercise, peak ventilation was 3 L/min (6%) lower in patients with compared to patients without LVD, while peak VO2 was reduced by 2.2 ml/kg/min (12%). The disproportionate reduction in gas exchange was likely to be due to the significantly smaller peak VT (by 150 ml or 8%) in patients with LVD at an insignificantly increased peak BF, resulting in a larger anatomical dead space. This is in line with observation in patients with HFrEF (39), and the emerging evidence that HF patients adopt a “rapid shallow breathing pattern” to avoid the adverse effects of large intrathoracic pressure swings on cardiac pre- and afterload (2). Importantly, our study extends the evidence on abnormal breathing patterns and hyperventilation in patients with HFrEF to patients with LVD. The significantly higher resting HR in patients with LVD compared to patients without LVD is compatible with sympathetic hyperactivity (2, 40). This finding suggests that several proposed mechanisms for exaggerated ventilation in HFrEF (e.g., augmented peripheral and central chemosensitivity and an inordinately high degree of afferent neural traffic originating from within the locomotor muscles) may already be present in patients with asymptomatic LVD starting the vicious cycle that may progress LVD to HFrEF (2). Interestingly, the models corrected for age, sex, and BMI showed a significant positive relationship of BMI with PETCO2 at rest and at AT, and a significant inverse relationship with nadir VE/VCO2. This raises the question of whether the obesity paradox not only applies to patients with HF but already to elderly patients with (and without) LVD (41).
The observed normalisation of the excessive ventilation of patients with LVD after exCR may partly be ascribed to the beneficial effects of exercise training on ergoreflex sensitivity, and/or chemoreceptor activation (40). Importantly, guideline-directed medical and device therapies for HFrEF improve chemo- and baroreceptor function insufficiently, highlighting the importance of exercise in this population (42).
While the VE/VCO2 slope is an established prognostic parameter in patients with HFrEF, only a few studies have reported the physiologically inversely related parameter PETCO2 at rest and at peak exercise (43, 44). Matsumoto and colleagues have found the severity of HF in 112 patients with cardiac disease to be negatively associated with PETCO2 at rest and at peak exercise (43). They found PETCO2 at peak exercise to be negatively associated with VE/VCO2 slope and VD/VT, but not with PaCO2. Arena and colleagues found a resting PETCO2 of <33 mmHg to discriminate significantly for cardiac events at 1-year follow-up and to significantly add discriminative power to VE/VCO2 slope (44). Similarly, Schäper and colleagues found resting PETCO2 ≤ 31 mmHg to be associated with mortality (45). Our study demonstrated consistent findings on the prognostic value of pulmonary gas exchange parameters in patients with LVD. The nadir VE/VCO2 and peak PETCO2 were associated with MACE. Moreover, as a novel finding, BF at rest was associated with MACE. The discriminative value of resting ventilation parameters for MACE deserves further investigation as both resting PETCO2 and BF have been found to be predictive for cardiovascular complications also in lung resection patients (46). Interestingly, NYHA class was not associated with MACE.
The important role of low PETCO2 in patients with HFrEF and periodic breathing has been pointed out by a study by Apostolo and colleagues who restored a normal breathing pattern with inhalation of 2% CO2 in 95% of their study patients (47). Likewise, inhalation of CO2 has been shown to stabilise breathing in patients with periodic breathing and Cheyne-Stokes respiration during sleep (48–50). Breathing instabilities are recognised markers for poor prognosis in patients with HFrEF and highlight the central role of breathing patterns in the progression of HF (51). Nevertheless, few therapies have been developed to address breathing patterns. A few small studies with encouraging results have employed slow breathing training in patients with heart failure (52, 53).
Strengths of this study are the large sample size of a representative European cohort of elderly patients with coronary artery disease undergoing exCR, and the longitudinal as well as comprehensive assessment of ventilatory parameters at rest, during ramp exercise, and at peak exercise. Furthermore, this is the first documentation of improved breathing patterns and pulmonary gas exchange both at rest and with exercise after completion of exCR in both patients with and without LVD.
One limitation of this study are its observational design which does not allow the identification of cause-effect relationships. This explorative study is also limited by multiple testing. Therefore, confidence intervals and p-values have to be interpreted accordingly. Unfortunately, we did not have data on beta-blocker classes. Previous studies showed that carvedilol, an unselective ß1- and ß2-blocker, was associated with a lower VE/VCO2 slope and nadir VE/VCO2 (54, 55). However, the standard treatment of ACS and CCS includes cardioselective beta-blockers like metoprolol. We can therefore only speculate that only a small proportion of the 93% of our patients have received an unselective beta-blocker. Further, ACE inhibitors have been shown to improve gas transfer and ventilation perfusion matching (56). Nevertheless, the higher percentage of ACE in patients with LVD could not completely offset their less efficient ventilation.
Logistic regressions for the identification of CPET variables significantly improving MACE prediction were performed for the whole EU-CaRE cohort (including patients with open heart surgery and valve replacement) recently (27). Due to the small sample size of our subgroup of patients with LVD, we did not perform logistic regression models in the present study.
Conclusion
Patients with LVD had an exaggerated BF at rest and impaired pulmonary gas exchange during exercise. Abnormal breathing patterns may be an early and clinically relevant sign of LVD and linked to increased ergoreflex sensitivity and/or abnormal chemosensitivity. ExCR may contribute to improvements in breathing patterns and pulmonary gas exchange in this population. However, interventions aimed at specifically improving altered breathing patterns at rest and during exercise may have additive value and should be investigated.
Data availability statement
The datasets presented in this article are not readily available because some centres have not requested permission to make data publicly accessible. Requests to access the datasets should be directed to arnoud.vant.hof@mumc.nl.
Ethics statement
The study was approved by all relevant medical ethics committees. All included participants provided their written informed consent to participate.
Author contributions
PE: is responsible for the content of the manuscript. Study conception and design: EdK, AV, EP, AvV, WB, MI, CG, DA, UZ, MW. Acquisition of data: PE, TM, LP, EdK, EP, MI, CG, DA, UZ, EM, MW. Data analysis: PE, TM, EP, EK. Drafting of the manuscript: PE, MW. Revising and approving of the manuscript: PE, TM, LP, EdK, EP, EK, MI, CG, DA, UZ, EM, MW, AV, AvV, WB. All authors contributed to the article and approved the submitted version.
Funding
The study was funded by the European Union's Horizon 2020 research and innovation program under grant agreement number 634439 and by the Swiss State Secretariat for Education, Research, and Innovation for the Swiss consortium partner.
Acknowledgments
We would like to thank Judith Peterhans for her valuable contributions to study logistics and data collection.
Conflict of interest
AV reports grants from Medtronic, grants and personal fees from Astra Zeneca, outside the submitted work, UZ reports grants and personal fees from Astra Zeneca, Bayer, BMS, Novartis, and MSD, and personal fees from Boehringer Ingelheim, Daiichi Sankyo, Eli Lilly, Trommsdorf, and Amgen, all outside the submitted work.
The remaining authors declare that the research was conducted in the absence of any commercial or financial relationships that could be construed as a potential conflict of interest.
Publisher's note
All claims expressed in this article are solely those of the authors and do not necessarily represent those of their affiliated organizations, or those of the publisher, the editors and the reviewers. Any product that may be evaluated in this article, or claim that may be made by its manufacturer, is not guaranteed or endorsed by the publisher.
Supplementary material
The Supplementary Material for this article can be found online at: https://www.frontiersin.org/articles/10.3389/fcvm.2023.1219589/full#supplementary-material
References
1. Lawson CA, Zaccardi F, Squire I, Okhai H, Davies M, Huang W, et al. Risk factors for heart failure: 20-year population-based trends by sex, socioeconomic Status, and ethnicity. Circ Heart Fail. (2020) 13(2):e006472. doi: 10.1161/CIRCHEARTFAILURE.119.006472
2. Cross TJ, Kim CH, Johnson BD, Lalande S. The interactions between respiratory and cardiovascular systems in systolic heart failure. J Appl Physiol (1985). (2020) 128(1):214–24. doi: 10.1152/japplphysiol.00113.2019
3. Malhotra R, Bakken K, D'Elia E, Lewis GD. Cardiopulmonary exercise testing in heart failure. JACC Heart Fail. (2016) 4(8):607–16. doi: 10.1016/j.jchf.2016.03.022
4. Ponikowski P, Francis DP, Piepoli MF, Davies LC, Chua TP, Davos CH, et al. Enhanced ventilatory response to exercise in patients with chronic heart failure and preserved exercise tolerance: marker of abnormal cardiorespiratory reflex control and predictor of poor prognosis. Circulation. (2001) 103(7):967–72. doi: 10.1161/01.CIR.103.7.967
5. Myers J, Arena R, Oliveira RB, Bensimhon D, Hsu L, Chase P, et al. The lowest VE/VCO2 ratio during exercise as a predictor of outcomes in patients with heart failure. J Card Fail. (2009) 15(9):756–62. doi: 10.1016/j.cardfail.2009.05.012
6. Nadruz W Jr., West E, Sengelov M, Santos M, Groarke JD, Forman DE, et al. Prognostic value of cardiopulmonary exercise testing in heart failure with reduced, midrange, and preserved ejection fraction. J Am Heart Assoc. (2017) 6(11). doi: 10.1161/JAHA.117.006000
7. Johnson RL J. Gas exchange efficiency in congestive heart failure. Circulation. (2000) 101(24):2774–6. doi: 10.1161/01.CIR.101.24.2774
8. Coats AJ, Clark AL, Piepoli M, Volterrani M, Poole-Wilson PA. Symptoms and quality of life in heart failure: the muscle hypothesis. Br Heart J. (1994) 72(2 Suppl):S36–9. doi: 10.1136/hrt.72.2_Suppl.S36
9. Duscha BD, Kraus WE, Keteyian SJ, Sullivan MJ, Green HJ, Schachat FH, et al. Capillary density of skeletal muscle: a contributing mechanism for exercise intolerance in class II-III chronic heart failure independent of other peripheral alterations. J Am Coll Cardiol. (1999) 33(7):1956–63. doi: 10.1016/S0735-1097(99)00101-1
10. Hirai DM, Musch TI, Poole DC. Exercise training in chronic heart failure: improving skeletal muscle O2 transport and utilization. Am J Physiol Heart Circ Physiol. (2015) 309(9):H1419–39. doi: 10.1152/ajpheart.00469.2015
11. Sullivan MJ, Duscha BD, Klitgaard H, Kraus WE, Cobb FR, Saltin B. Altered expression of myosin heavy chain in human skeletal muscle in chronic heart failure. Med Sci Sports Exerc. (1997) 29(7):860–6. doi: 10.1097/00005768-199707000-00004
12. Schulze PC, Linke A, Schoene N, Winkler SM, Adams V, Conradi S, et al. Functional and morphological skeletal muscle abnormalities correlate with reduced electromyographic activity in chronic heart failure. Eur J Cardiovasc Prev Rehabil. (2004) 11(2):155–61. doi: 10.1097/01.hjr.0000124327.85096.a5
13. Piepoli M, Clark AL, Volterrani M, Adamopoulos S, Sleight P, Coats AJ. Contribution of muscle afferents to the hemodynamic, autonomic, and ventilatory responses to exercise in patients with chronic heart failure: effects of physical training. Circulation. (1996) 93(5):940–52. doi: 10.1161/01.CIR.93.5.940
14. Baliga RR, Young JB. Reducing the burden of stage B heart failure will require connecting the dots between “knowns” and “known unknowns”. Heart Fail Clin. (2012) 8(1):xi–v. doi: 10.1016/j.hfc.2011.10.001
15. McDonagh TA, Morrison CE, Lawrence A, Ford I, Tunstall-Pedoe H, McMurray JJ, et al. Symptomatic and asymptomatic left-ventricular systolic dysfunction in an urban population. Lancet. (1997) 350(9081):829–33. doi: 10.1016/S0140-6736(97)03033-X
16. Mitchell BL, Lock MJ, Davison K, Parfitt G, Buckley JP, Eston RG. What is the effect of aerobic exercise intensity on cardiorespiratory fitness in those undergoing cardiac rehabilitation? A systematic review with meta-analysis. Br J Sports Med. (2019) 53(21):1341–51. doi: 10.1136/bjsports-2018-099153
17. Doimo S, Fabris E, Piepoli M, Barbati G, Antonini-Canterin F, Bernardi G, et al. Impact of ambulatory cardiac rehabilitation on cardiovascular outcomes: a long-term follow-up study. Eur Heart J. (2019) 40(8):678–85. doi: 10.1093/eurheartj/ehy417
18. Anderson L, Oldridge N, Thompson DR, Zwisler AD, Rees K, Martin N, et al. Exercise-based cardiac rehabilitation for coronary heart disease: cochrane systematic review and meta-analysis. J Am Coll Cardiol. (2016) 67(1):1–12. doi: 10.1016/j.jacc.2015.10.044
19. Haykowsky MJ, Liang Y, Pechter D, Jones LW, McAlister FA, Clark AM. A meta-analysis of the effect of exercise training on left ventricular remodeling in heart failure patients: the benefit depends on the type of training performed. J Am Coll Cardiol. (2007) 49(24):2329–36. doi: 10.1016/j.jacc.2007.02.055
20. Ruku DM, Tran Thi TH, Chen HM. Effect of center-based or home-based resistance training on muscle strength and VO(2) peak in patients with HFrEF: a systematic review and meta-analysis. Enferm Clin (Engl Ed). (2021):S1130-8621(21)00040-1. doi: 10.1016/j.enfcli.2021.01.011
21. Fu TC, Yang NI, Wang CH, Cherng WJ, Chou SL, Pan TL, et al. Aerobic interval training elicits different hemodynamic adaptations between heart failure patients with preserved and reduced ejection fraction. Am J Phys Med Rehabil. (2016) 95(1):15–27. doi: 10.1097/PHM.0000000000000312
22. Coats AJ, Adamopoulos S, Radaelli A, McCance A, Meyer TE, Bernardi L, et al. Controlled trial of physical training in chronic heart failure. Exercise performance, hemodynamics, ventilation, and autonomic function. Circulation. (1992) 85(6):2119–31. doi: 10.1161/01.CIR.85.6.2119
23. Prescott E, Meindersma EP, van der Velde AE, Gonzalez-Juanatey JR, Iliou MC, Ardissino D, et al. A European study on effectiveness and sustainability of current cardiac rehabilitation programmes in the elderly: design of the EU-CaRE randomised controlled trial. Eur J Prev Cardiol. (2016) 23(2 suppl):27–40. doi: 10.1177/2047487316670063
24. Prescott E, Eser P, Mikkelsen N, Holdgaard A, Marcin T, Wilhelm M, et al. Cardiac rehabilitation of elderly patients in eight rehabilitation units in Western Europe: outcome data from the EU-CaRE multi-centre observational study. Eur J Prev Cardiol. (2020) 27(16):1716–29. doi: 10.1177/2047487320903869
25. Eser P, Marcin T, Prescott E, Prins LF, Kolkman E, Bruins W, et al. Clinical outcomes after cardiac rehabilitation in elderly patients with and without diabetes mellitus: the EU-CaRE multicenter cohort study. Cardiovasc Diabetol. (2020) 19(1):37. doi: 10.1186/s12933-020-01013-8
26. Marcin T, Eser P, Prescott E, Prins LF, Kolkman E, Bruins W, et al. Training intensity and improvements in exercise capacity in elderly patients undergoing European cardiac rehabilitation—the EU-CaRE multicenter cohort study. PLoS One. (2020) 15(11):e0242503. doi: 10.1371/journal.pone.0242503
27. Marcin T, Eser P, Prescott E, Prins LF, Kolkman E, Bruins W, et al. Changes and prognostic value of cardiopulmonary exercise testing parameters in elderly patients undergoing cardiac rehabilitation: the EU-CaRE observational study. PLoS One. (2021) 16(8):e0255477. doi: 10.1371/journal.pone.0255477
28. Eser P, Marcin T, Prescott E, Prins LF, Kolkman E, Bruins W, et al. Predictors for one-year outcomes of cardiorespiratory fitness and cardiovascular risk factor control after cardiac rehabilitation in elderly patients: the EU-CaRE study. PLoS One. (2021) 16(8):e0255472. doi: 10.1371/journal.pone.0255472
29. Kelly JP, Mentz RJ, Mebazaa A, Voors AA, Butler J, Roessig L, et al. Patient selection in heart failure with preserved ejection fraction clinical trials. J Am Coll Cardiol. (2015) 65(16):1668–82. doi: 10.1016/j.jacc.2015.03.043
30. Prescott E, Mikkelsen N, Holdgaard A, Eser P, Marcin T, Wilhelm M, et al. Cardiac rehabilitation in the elderly patient in eight rehabilitation units in Western Europe: baseline data from the EU-CaRE multicentre observational study. Eur J Prev Cardiol. (2019) 26(10):1052–63. doi: 10.1177/2047487319839819
31. Marcin T, Eser P, Prescott E, Mikkelsen N, Prins LF, Kolkman EK, et al. Predictors of pre-rehabilitation exercise capacity in elderly European cardiac patients—the EU-CaRE study. Eur J Prev Cardiol. (2020) 27(16):1702–12. doi: 10.1177/2047487319894676
32. Lang RM, Badano LP, Mor-Avi V, Afilalo J, Armstrong A, Ernande L, et al. Recommendations for cardiac chamber quantification by echocardiography in adults: an update from the American Society of Echocardiography and the European Association of Cardiovascular Imaging. Eur Heart J Cardiovasc Imaging. (2015) 16(3):233–70.25712077
33. Huber A, Oldridge N, Höfer S. International SF-36 reference values in patients with ischemic heart disease. Qual Life Res. (2016) 25(11):2787–98. doi: 10.1007/s11136-016-1316-4
34. Satoh T, Okano Y, Takaki H, Matsumoto T, Yasumura Y, Aihara N, et al. Excessive ventilation after acute myocardial infarction and its improvement in 4 months. Jpn Circ J. (2001) 65(5):399–403. doi: 10.1253/jcj.65.399
35. Laoutaris ID, Adamopoulos S, Manginas A, Panagiotakos DB, Kallistratos MS, Doulaptsis C, et al. Benefits of combined aerobic/resistance/inspiratory training in patients with chronic heart failure. A complete exercise model? A prospective randomised study. Int J Cardiol. (2013) 167(5):1967–72. doi: 10.1016/j.ijcard.2012.05.019
36. Servantes DM, Pelcerman A, Salvetti XM, Salles AF, de Albuquerque PF, de Salles FC, et al. Effects of home-based exercise training for patients with chronic heart failure and sleep apnoea: a randomized comparison of two different programmes. Clin Rehabil. (2012) 26(1):45–57. doi: 10.1177/0269215511403941
37. Stigler SM. Regression towards the mean, historically considered. Stat Methods Med Res. (1997) 6(2):103–14. doi: 10.1177/096228029700600202
38. Prado DM, Rocco EA, Silva AG, Silva PF, Lazzari JM, Assumpção GL, et al. The influence of aerobic fitness status on ventilatory efficiency in patients with coronary artery disease. Clinics. (2015) 70(1):46–51. doi: 10.6061/clinics/2015(01)09
39. Witte KK, Thackray SD, Nikitin NP, Cleland JG, Clark AL. Pattern of ventilation during exercise in chronic heart failure. Heart. (2003) 89(6):610–4. doi: 10.1136/heart.89.6.610
40. Aimo A, Saccaro LF, Borrelli C, Fabiani I, Gentile F, Passino C, et al. The ergoreflex: how the skeletal muscle modulates ventilation and cardiovascular function in health and disease. Eur J Heart Fail. (2021) 23(9):1458–67. doi: 10.1002/ejhf.2298
41. Lavie CJ, De Schutter A, Alpert MA, Mehra MR, Milani RV, Ventura HO. Obesity paradox, cachexia, frailty, and heart failure. Heart Fail Clin. (2014) 10(2):319–26. doi: 10.1016/j.hfc.2013.12.002
42. Giannoni A, Gentile F, Buoncristiani F, Borrelli C, Sciarrone P, Spiesshoefer J, et al. Chemoreflex and baroreflex sensitivity hold a strong prognostic value in chronic heart failure. JACC Heart Fail. (2022) 10(9):662–76. doi: 10.1016/j.jchf.2022.02.006
43. Matsumoto A, Itoh H, Eto Y, Kobayashi T, Kato M, Omata M, et al. End-tidal CO2 pressure decreases during exercise in cardiac patients: association with severity of heart failure and cardiac output reserve. J Am Coll Cardiol. (2000) 36(1):242–9. doi: 10.1016/S0735-1097(00)00702-6
44. Arena R, Peberdy MA, Myers J, Guazzi M, Tevald M. Prognostic value of resting end-tidal carbon dioxide in patients with heart failure. Int J Cardiol. (2006) 109(3):351–8. doi: 10.1016/j.ijcard.2005.06.032
45. Schäper C, Gläser S, Wolff B, Koch B, Vietzke G, Felix SB, et al. Resting alveolar gas tensions as a mortality prognosticator in chronic heart failure. Transplant Proc. (2010) 42(7):2681–6. doi: 10.1016/j.transproceed.2010.05.166
46. Mazur A, Brat K, Homolka P, Merta Z, Svoboda M, Bratova M, et al. Ventilatory efficiency is superior to peak oxygen uptake for prediction of lung resection cardiovascular complications. PLoS One. (2022) 17(8):e0272984. doi: 10.1371/journal.pone.0272984
47. Apostolo A, Agostoni P, Contini M, Antonioli L, Swenson ER. Acetazolamide And inhaled carbon dioxide reduce periodic breathing during exercise in patients with chronic heart failure. J Card Fail. (2014) 20(4):278–88. doi: 10.1016/j.cardfail.2014.01.007
48. Lorenzi-Filho G, Rankin F, Bies I, Douglas Bradley T. Effects of inhaled carbon dioxide and oxygen on cheyne-stokes respiration in patients with heart failure. Am J Respir Crit Care Med. (1999) 159(5 Pt 1):1490–8. doi: 10.1164/ajrccm.159.5.9810040
49. Steens RD, Millar TW, Su X, Biberdorf D, Buckle P, Ahmed M, et al. Effect of inhaled 3% CO2 on cheyne-stokes respiration in congestive heart failure. Sleep. (1994) 17(1):61–8. doi: 10.1093/sleep/17.1.61
50. Giannoni A, Baruah R, Willson K, Mebrate Y, Mayet J, Emdin M, et al. Real-time dynamic carbon dioxide administration: a novel treatment strategy for stabilization of periodic breathing with potential application to central sleep apnea. J Am Coll Cardiol. (2010) 56(22):1832–7. doi: 10.1016/j.jacc.2010.05.053
51. Giannoni A, Emdin M, Bramanti F, Iudice G, Francis DP, Barsotti A, et al. Combined increased chemosensitivity to hypoxia and hypercapnia as a prognosticator in heart failure. J Am Coll Cardiol. (2009) 53(21):1975–80. doi: 10.1016/j.jacc.2009.02.030
52. Parati G, Malfatto G, Boarin S, Branzi G, Caldara G, Giglio A, et al. Device-guided paced breathing in the home setting: effects on exercise capacity, pulmonary and ventricular function in patients with chronic heart failure: a pilot study. Circ Heart Fail. (2008) 1(3):178–83. doi: 10.1161/CIRCHEARTFAILURE.108.772640
53. Lachowska K, Bellwon J, Narkiewicz K, Gruchała M, Hering D. Long-term effects of device-guided slow breathing in stable heart failure patients with reduced ejection fraction. Clin Res Cardiol. (2019) 108(1):48–60. doi: 10.1007/s00392-018-1310-7
54. Agostoni P, Apostolo A, Cattadori G, Salvioni E, Berna G, Antonioli L, et al. Effects of beta-blockers on ventilation efficiency in heart failure. Am Heart J. (2010) 159(6):1067–73. doi: 10.1016/j.ahj.2010.03.034
55. Contini M, Apostolo A, Cattadori G, Paolillo S, Iorio A, Bertella E, et al. Multiparametric comparison of CARvedilol, vs. NEbivolol, vs. BIsoprolol in moderate heart failure: the CARNEBI trial. Int J Cardiol. (2013) 168(3):2134–40. doi: 10.1016/j.ijcard.2013.01.277
Keywords: coronary artery disease, respiration, exercise training, breathing frequency, cardiopulmonary exercise testing (CPET), heart failure
Citation: Eser P, Marcin T, Prescott E, Prins LF, Kolkman E, Bruins W, van der Velde AE, Gil CP, Iliou M-C, Ardissino D, Zeymer U, Meindersma EP, Van’t Hof AWJ, de Kluiver EP and Wilhelm M (2023) Breathing pattern and pulmonary gas exchange in elderly patients with and without left ventricular dysfunction—modification with exercise-based cardiac rehabilitation and prognostic value. Front. Cardiovasc. Med. 10:1219589. doi: 10.3389/fcvm.2023.1219589
Received: 10 May 2023; Accepted: 1 August 2023;
Published: 1 September 2023.
Edited by:
Elisabetta Salvioni, Monzino Cardiology Center (IRCCS), ItalyReviewed by:
Paola Gargiulo, University of Naples Federico II, ItalyMaria Inês Pires, Centro Hospitalar de Tondela-Viseu, Portugal
Cássia Goulart, Federal University of São Carlos, Brazil
© 2023 Eser, Marcin, Prescott, Prins, Kolkman, Bruins, van der Velde, Gil, Iliou, Ardissino, Zeymer, Meindersma, Van't Hof, de Kluiver and Wilhelm. This is an open-access article distributed under the terms of the Creative Commons Attribution License (CC BY). The use, distribution or reproduction in other forums is permitted, provided the original author(s) and the copyright owner(s) are credited and that the original publication in this journal is cited, in accordance with accepted academic practice. No use, distribution or reproduction is permitted which does not comply with these terms.
*Correspondence: Matthias Wilhelm matthias.wilhelm@insel.ch
†Present addresses: Prisca Eser and Matthias Wilhelm, Rehabilitation & Sports Medicine, Inselspital, Bern University Hospital, University of Bern, Switzerland. Thimo Marcin, Research Department, Berner Reha Zentrum, Heiligenschwendi, Switzerland
Abbreviations ACS, acute coronary syndrome; BF, breathing frequency; CCS, chronic coronary syndrome; CPET, cardiopulmonary exercise testing; ECG, echocardiogram; exCR, exercise-based cardiac rehabilitation; FEV1, forced expiratory volume during the first second; FVC, forced vital capacity; HF, heart failure; HFrEF, heart failure with reduced ejection fraction; LVEF, left ventricular ejection fraction; LVD, left ventricular dysfunction; MACE, major adverse cardiac event; PaCO2, arterial partial pressure of carbon dioxide; PETCO2, end-expiratory partial pressure of carbon dioxide; VCO2, carbon dioxide production; VO2, oxygen consumption; VE, ventilation; VT, tidal volume.