- Department of Radiology, University of Washington, School of Medicine, Seattle, WA, United States
Cardiac magnetic resonance (CMR) is emerging as the modality of choice to assess early cardiovascular involvement in patients with autoimmune rheumatic diseases (ARDs) that often has a silent presentation and may lead to changes in management. Besides being reproducible and accurate for functional and volumetric assessment, the strength of CMR is its unique ability to perform myocardial tissue characterization that allows the identification of inflammation, edema, and fibrosis. Several CMR biomarkers may provide prognostic information on the severity and progression of cardiovascular involvement in patients with ARDs. In addition, CMR may add value in assessing treatment response and identification of cardiotoxicity related to therapy with immunomodulators that are commonly used to treat these conditions. In this review, we aim to discuss the following objectives:
• Illustrate imaging findings of multi-parametric CMR approach in the diagnosis of cardiovascular involvement in various ARDs;
• Review the CMR signatures for risk stratification, prognostication, and guiding treatment strategies in ARDs;
• Describe the utility of routine and advanced CMR sequences in identifying cardiotoxicity related to immunomodulators and disease-modifying agents in ARDs;
• Discuss the limitations of CMR, recent advances, current research gaps, and potential future developments in the field.
1. Introduction
High burden of cardiovascular disease (CVD) and associated morbidity and mortality exists in various autoimmune rheumatic diseases (ARDs). These include rheumatoid arthritis (RA), Systemic lupus erythematosus (SLE), spondyloarthritides such as psoriatic arthritis (PsA) and ankylosing spondylitis (AS), systemic sclerosis (SSc), Sjögren's syndrome (SS), mixed connective tissue disease (MCTD), inflammatory myopathies such as dermatomyositis (DM), polymyositis (PM) and inclusion body myositis (IBM) and vasculitis of large, medium and small vessels (1–5). Life expectancy is low in patients with ARDs compared to the general population (6) mainly due to increased and often subclinical prevalence of CVD despite the introduction of new and targeted treatment strategies to reduce disease-related mortality (5, 7–9). Traditional imaging techniques are not well equipped to define the acuity of cardiac involvement in ARDs due to limited tissue characterization abilities (10–13). This particular limitation on routinely performed transthoracic echocardiography (TTE) leads to under-diagnosis of early myocardial involvement that may lead to fatal arrhythmias despite preserved LV and RV function (14).
Cardiac magnetic resonance (CMR) is a non-invasive examination with diagnostic as well as prognostic capabilities with cardiovascular involvement in patients with ARDs. The various pathophysiologic processes that can be accurately assessed on CMR include myocardial inflammation (10, 15, 16) early or late fibrosis due to inflammation (17), macro- and micro-vasculopathy (18, 19), ischemic heart disease, and myocardial infarction(18, 20, 21), rhythm and conduction disturbances, valvular diseases, pulmonary hypertension, and diastolic or systolic heart failure (3, 22). In particular, high accuracy of stress CMR is beneficial for early assessment of ischemic or hibernating myocardium resulting from epicardial coronary artery diseases in patients with ARDs (21). Techniques for vessel wall imaging can help identify arterial wall inflammation of the great vessels and overall active inflammatory burden in ARDs (20).
As per the recent clinical consensus document by the European Association of Cardiovascular Imaging (EACVI), CMR should be obtained for clinical evaluation of patients with cardiac symptoms when the echocardiogram is normal since the disease processes can be occult to echocardiogram. CMR should be considered as first line imaging modality to assess patients with SSc or SLE if there is any suspicion of cardiac involvement at the time of the diagnosis. Finally, CMR should be the next test to perform a detailed evaluation of the extent of myocardial and vascular disease burden in patients with ARD with cardiac symptoms and abnormal echocardiogram (23). CMR is the test of choice for monitoring treatment response when there is cardiac involvement and allows identification of cardiotoxicity in these patients who are often treated with long-term immunomodulatory therapies (24).
Imagers and referring providers must have a robust knowledge of the multi-parametric CMR approach for the appropriate management of ARDs. Hence, in this review, we will focus on how CMR helps in the well-rounded assessment and management of various cardiovascular manifestations in patients with ARDs.
2. Multiparametric CMR approach for diagnosing cardiac involvement in various ARDs
A comprehensive CMR examination for patients with ARDs consists of T1-weighted (T1w) and T2-weighted (T2w) inversion-recovery turbo spin-echo (black blood) images or STIR-T2 images multiplanar balanced steady-state free precision (bSSFP) bright blood cine images,, look locker and phase-sensitive inversion recovery (PSIR) sequence for early and late gadolinium enhancement (LGE), modified look locker technique for T1 and T2 parametric mapping, post contrast T1 mapping for ECV quantification. Newer techniques such as strain may be used for the early detection of regional function abnormalities. Quantitative perfusion mapping should be considered when available (25). Rich multi-parametric data provided through a single CMR examination allows for capturing the intricate details of various disease processes in ARDs.
2.1. Myocardial inflammation first-line
CMR allows the identification of myocardial edema from acute myocarditis on STIR- T2w black blood images. Native T1 and T2 parametric mapping are popular techniques for objective analysis and quantification of myocardial fibrosis and inflammation. Native T1 mapping can be elevated in the early stages of disease, when inflammation is the main abnormality, but can also be at late stages when only fibrosis is seen. Therefore, the combined assessment with both native T1 and T2 maps is quite helpful to differentiate edema from fibrosis. Extracellular volume (ECV) estimates the expansion of the extracellular space due to edema or fibrosis (26, 27). In both acute and chronic myocarditis, the most characteristic location of myocardial edema on CMR is at the subepicardial basal to mid inferolateral segment in a noncoronary distribution on T2w and LGE images (28). In our clinical experience, myocardial edema in ARD affects the myocardium globally and changes are much more subtle than in classic myocarditis.
In patients with ARDs, signs of myocardial involvement are infrequent in the clinical setting but extremely common in autopsy studies. Several studies report the presence of myocardial inflammation in up to 40%–50% of patients of RA (29) and SLE (30, 31) and in around 40% of patients in SS (32, 33), PM, and DM (34). Myocarditis is extremely rare in Sjogren's syndrome (35). In a small cohort of 20 patients with different ARDs, myocarditis was identified in most patients (80%) using CMR with a cut-off of 1.89 ± 0.25 for the signal ratio of the myocardium to skeletal muscle on T2w images, relative myocardial enhancement of 11.31 ± 11.18 on T1w images and epicardial late gadolinium enhancement. This cut-off had a higher agreement with histology and PCR (50% and 80% of positive CMR examinations) respectively (10).
In patients with SLE, disease activity has been traditionally evaluated using the European Consensus Lupus Activity Measurement (ECLAM) index (36) and the newer SLE Disease Activity Score (SLE-DAS) (37). CMR indices such as relative T2 ratio, prolonged myocardial T1 relaxation time, global relative enhancement derived from a subtracted image (38), and focal subepicardial or mid myocardial LGE in the inferolateral wall have been reported to correlate with disease activity assessed by ECLAM index (39) (Figure 1). T2 relaxation values are a sensitive indicator of myocardial disease in patients with active or subclinical SLE and may normalize with clinical improvement in myocardial disease activity (40). T2w images also help with the characterization of acute vs. chronic myocardial involvement using simple ratios such as T2 signal intensity ratio > 2 relative to skeletal muscle for acute lesions with myocardium in patients with inflammatory vasculitis (41).
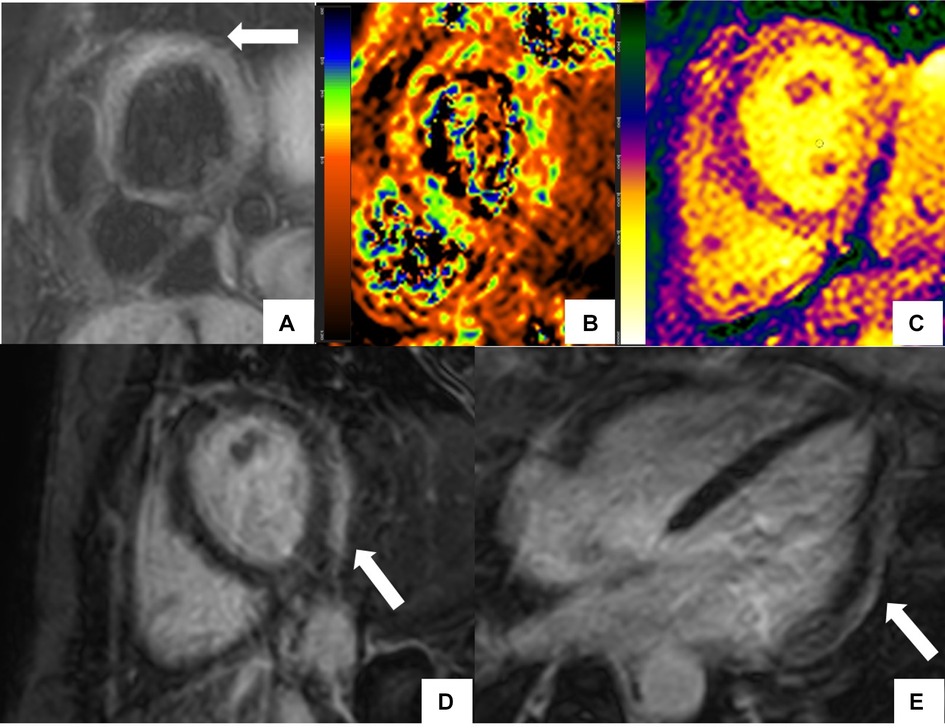
Figure 1. CMR findings in systemic lupus erythematosus (SLE) myopericarditis. 44 year old female with longstanding systemic lupus erythematosus (SLE) and overlap syndrome with premature ventricular contractions and dyspnea on exertion. Cardiac MR (CMR) showed mild biventricular systolic dysfunction with left ventricular ejection fraction (LVEF) of 48% and right ventricular ejection fraction of (RVEF) of 42%. Edema was seen on T2 black blood fat supressed short axis image (A) with elevated T2 value of 72 ms on T2 color map (B) and diffusely increased myocardial native T1 value of 1,134 ms on native T1 color map (C) On short-axis and 4-chamber late gadolinium enhancement phase-sensitive inversion recovery (LGE- PSIR) image, subepicardial hyperenhancement was seen within the entire inferolateral wall (D,E respectively). Constellation of findings were consistent with cardiac involvement in SLE with an acute inflammatory component (42). Pericardial thickening with diffuse LGE and increased T2 signal in the basal anterior wall away from LGE suggested acute on chronic pericarditis. No pericardial effusion was seen.
The updated Lake Louise criteria for myocarditis incorporates at least one T2-based criterion such as a global or regional increase of myocardial T2 relaxation time or an increased signal intensity in T2w CMR images, with at least one T1-based criterion such as increased myocardial T1, ECV, or LGE for increased specificity and diagnostic accuracy to detect acute myocardial inflammation. Its supportive criteria include CMR-based identification of pericarditis and systolic LV dysfunction in the form of global or regional wall motion abnormality on cine imaging (27). CMR has a high sensitivity of 76%, a specificity of 95.5%, and a diagnostic accuracy of up to 85% when a combined approach using T2w and early and late gadolinium enhancement is employed for the detection of myocardial inflammation (10, 41, 43). T2 mapping seems to perform better in characterizing both acute and chronic myocarditis than T1 mapping (44). Parametric T2 mapping is also independent of concomitant skeletal muscle inflammation. It provides more robust information on myocardial edema compared to T2W ratio due to reduced sensitivity to motion artifacts from motion correction and clear delineation of endocardial borders, increased objectivity from pixel-wise information of native T2 value and reduced signal intensity variability (45, 46).
2.2. Pericardial disease
Symptomatic pericarditis is the major CMR-detectable abnormality affecting around 30% of patients of SLE (30) and 40% of patients of SS (47, 48). Chronic pericarditis is seen in around 70% of patients with SS (32). Asymptomatic pericardial involvement with autopsy confirmation has been reported in up to 80% of patients with SLE (3), about 50% of patients with MTCD (49), and 30% of Primary Sjogren's syndrome (50). However, tamponade occurs in less than 2% and constrictive pericarditis is extremely rare in patients with SLE (3).
CMR helps in the assessment of pericardial thickening, adhesions, and quantification of the severity of pericardial effusion, and may show signs of constrictive pericarditis even when the pericardial is not overly thick. Enhancement of pericardium on post-contrast images is the most useful diagnostic sign for the presence of pericarditis (51) (Figure 1). T1 mapping can differentiate exudative from transudative pericardial effusion using a predetermined threshold of 3,015 ms for pericardial effusion and 3,440 ms for pleural effusions with lower values suggesting the presence of exudates (52).
Exaggerated ventricular interdependence or ventricular coupling is unique to constrictive physiology and can be obviated on real-time cine CMR as an early diastolic septal inversion or flattening at the height of rapid deep inspiration, popularly known as septal bounce (53–55). In symptomatic patients with thickened pericardium, pericardial enhancement on LGE combined with either high signal intensity on T2w and/or elevated T1/T2 mapping indicates active inflammation that may necessitate anti-inflammatory therapy rather than surgery (56). T2 mapping of the pericardium is not ready for prime time as the pericardium being too thin walled it is difficult to avoid massive partial volume artefact.
2.3. Myocardial scar characterization on late gadolinium enhancement
Patients with ARDs can develop myocardial replacement fibrosis due to infarction but also reactive fibrosis due to nonischemic insults. Ischemic cardiomyopathy is characterized by the presence of a subendocardial scar in a coronary distribution on LGE CMR. A nonischemic pattern of the scar on CMR includes diffuse subendocardial LGE in a non-coronary distribution, mid-myocardial or subepicardial pattern (Figure 2). Besides visual depiction of scar, CMR can provide scar quantification using different techniques on CMR such as full-width half maximum (FWHM), 5 standard deviations, or 6 standard deviations from remote myocardium (57). Acute and chronic myocardial processes can be characterized on CMR by combining information from LGE with T2 and T1 mapping sequences. T1/T2 mapping indices identify diffuse fibrosis and myocardial edema in patients with ARDs when significantly higher native T1 and T2 mapping values are present and they are independent of the presence of LGE (58).
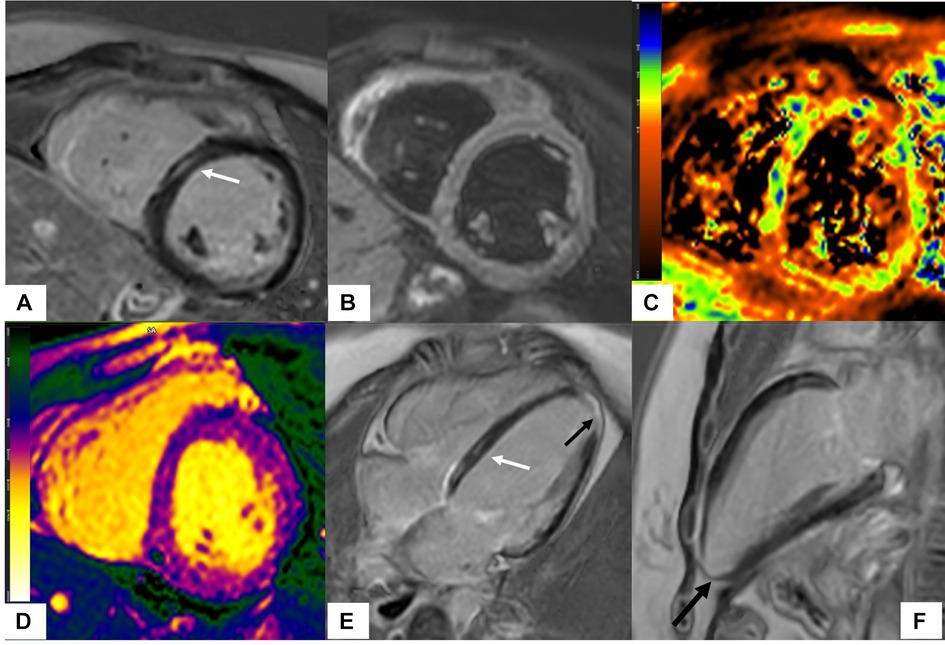
Figure 2. Scleroderma with mixed ischemic and non-ischemic cardiomyopathy on CMR. 20-year-old female with diffuse systemic sclerosis, ANA + anti Scl70 + with interstitial lung disease. Patient had occasional palpitations, ventricular tachycardia (VT) and premature ventricular contractions (PVC) burden of 13.2% on Holter monitoring. Cardiac MR (CMR) revealed normal size LV with low normal LV systolic function with left ventricular ejection fraction (LVEF) 54%, moderately dilated RV with moderately reduced right ventricular ejection fraction (RVEF) of 39%. On short-axis and 4-chamber late gadolinium enhancement phase-sensitive inversion recovery (LGE PSIR), mid myocardial late gadolinium enhancement was seen in the septum in a non-coronary distribution (A,E white arrows) without increased signal on short-axis T2w BB FS images (B), normal T2 values on T2 color map (C) and normal native T1 value on native T1 color map (D) These findings were suggestive of chronic myocardial fibrosis related to scleroderma involvement (42). Transmural LGE with akinetic apical anterior wall and apex was seen on the 4-chamber and 2-chamber views (black arrows E,F) suggestive of chronic microvascular injury and myocardial infarction in the absence of epicardial disease. Overall, findings of mixed ischemic and non-ischemic etiology were likely related to systemic sclerosis.
Imaging within the first few minutes after contrast administration helps delineate microvascular obstruction which prevents contrast delivery to the infarct core and thus results in low signal on T1w imaging (59–61).
The use of T2w CMR in conjunction with LGE can help delineate the ischemic region at risk during acute myocardial infarction, which usually extends beyond the scar. It also helps evaluate the presence of coexistent myocardial inflammation in addition to scarring in patients with ARDs (10), Churg-Strauss syndrome (62), and SLE (39, 40). Rheumatic nonischemic lesions are mainly mid-myocardial or subepicardial, independent of the coronary distribution, and common at the inferior or inferolateral wall of LV thus mimicking the image pattern of viral myocarditis (63). The presence of edema on T2w mages in rheumatic patients is indicative of the acute phase of myocardial inflammation and can be identified simultaneously or early before the appearance of LGE lesions (38, 39). In the setting of myocarditis in SLE, the “age” of injury can be estimated on CMR with the presence of T2 and early gadolinium enhancement suggesting an acute process, and their normalization but persistence of LGE suggesting sub-acute or chronic stages of myocardial inflammation (38, 64, 65). The application of LGE CMR allows the detection of myocardial necrosis as reported in patients with vasculitis (10, 66) and SLE (67, 68).
Mavrogeni et al., characterized acute and chronic lesions as T2 signal intensity (SI) ratio >2 and positive LGE and T2 SI ratio <2 and positive LGE, respectively in patients with CTDs including sarcoidosis, SS, SLE, RA, and inflammatory myopathies. The etiology of myocardial injury was defined based on the distribution of CMR findings: vasculitis was defined when LGE was diffuse and subendocardial in distribution but not following coronary distribution, myocarditis was favored when LGE was subepicardial/intramural and myocardial infarction when LGE was subendocardial/transmural following a coronary territory (16). CMR pattern of diffuse subendocardial fibrosis on LGE is compatible with diffuse subendocardial vasculitis (DSV) caused by either diffuse myocardial ischemia due to vasculitis of small epicardial vessels or by granulomatous or eosinophilic infiltration (19, 20).
2.4. Myocardial ischemia
Abnormalities of myocardial perfusion can be commonly seen in ARDs. The presence of myocardial perfusion defects can also strongly and independently predict coronary artery disease in ARDs such as SLE (69–71). Coronary vasculitis with persistent inflammation, autoimmunity, immune complex deposition, and antiphospholipid antibodies are hypothesized to cause intimal damage followed by accelerated atherosclerosis in these patients. In the absence of epicardial coronary stenosis, an abnormal coronary flow reserve causes an impaired coronary microcirculation and is associated with a negative prognosis (72). Up to 40% of women with SLE with no history of coronary artery disease showed abnormal myocardial perfusion (66–68). Coronary dissection and coronary artery aneurysms may occur in patients with SLE (73). Accelerated or premature atherosclerotic disease is a leading cause of late death in patients with SLE (74–77). In SSc, coronary involvement is not a feature but coronary flow reserve may be lower when compared to healthy controls (78). Overt perfusion defects have been identified on stress CMR in SSc patients when there is a history of digital ulceration (79) and in patients with antiphospholipid syndrome (APS) without the presence of overt cardiac symptoms (80).
There is growing evidence in favor of CMR for evaluation of coronary artery disease in young women with ARD over SPECT due to lack of ionizing radiation and higher diagnostic accuracy as per the landmark MR-IMPACT impact (81, 82) and CE-MARC study studies (83). On CMR, myocardial Ischemia is defined by the presence of myocardial perfusion defects at stress during the first pass of gadolinium, which recovers at rest (84, 85). Presence of wall motion abnormalities, abnormal wall thickening, and perfusion defects on CMR during pharmacologic stress with dobutamine has improved sensitivity (86% vs. 74%) and specificity (86% vs. 70%) for the detection of myocardial ischemia over stress echo (86–88). Such a reliable assessment of subendocardial ischemia on CMR is made possible due to a higher spatial resolution of 2–3 mm. Recent advances in the CMR perfusion technique have made it possible to achieve a spatial resolution of around 1 mm in the imaging plane, especially at 3 T scanners (89–91). Quantitative assessment of subendocardial ischemia is also feasible on CMR using myocardial perfusion reserve index (MPRI) (92) and resting myocardial blood flow in hibernating segments (93) and these approaches are found superior to SPECT when detecting significant coronary stenosis by CMR (94). Newer technique of quantitative perfusion mapping is available at dedicated centers. This utilizes respiratory motion correction to generate perfusion maps with free breathing acquisition. Pixel-wise quantification of myocardial blood flow (MBF) at rest and during vasodilator-stress is possible at a high spatial resolution of approximately 2 mm that provides objective assessment of macro and microvascular ischemia (25).
Although acute myocardial infarction (MI) is a rather unusual event in rheumatic patients, the possibility of coexisting coronary artery disease with myocardial infarction should be always kept in mind during evaluation. Myocardial infarction from coronary microvascular disease exclusively presents as subendocardial lesions (95). Acute MI usually presents with concomitant myocardial edema that can be identified on T2w sequences. The presence of myocardial hemorrhage carries a poor prognosis and can be detected using T1w, T2w, and T2*w images (96).
2.5. Macro/microvasculopathy and vascular inflammation
The silent early phases of cardiovascular involvement in patients with ARD are generally due to the presence of microvascular disease. Chronic inflammation, the duration and activity of the autoimmune disease, and immunosuppressive therapy are specific risk factors for microvascular disease. Accelerated coronary atherosclerosis at a younger age than in the general population is due to the underlying presence of CVD. Unexplained arterial thrombosis and malignant arterial hypertension may also be seen in some patients with vasculitis (97).
There is potential for CMR angiography (CMRA) as an added imaging option when an assessment of vascular involvement is desired. It can be performed using gadolinium-enhanced MR angiogram or non-gadolinium-enhanced 3-dimensional SSFP sequences, particularly useful for the coronary arteries. CMR perfusion and mapping techniques can identify microvascular disease in patients with ARD (98), but CMRA is the technique of choice for the noninvasive evaluation of coronary arteries (aneurysms, ectasia) and great vessels (inflammation, aneurysms) that are frequently involved in systemic rheumatic diseases (60). Giant coronary artery aneurysms (CAA) can form from primary vasculitis, atherosclerosis, or a combination of both to infrequently cause acute coronary syndromes (ACS) in both SLE and rheumatoid arthritis RA or Rhupus syndrome (RhS), a rare entity characterized by overlapping rheumatoid arthritis (RA) and systemic lupus erythematosus (SLE) (99). Concomitant acquisition of CMR has the potential to mitigate additional costs to evaluate coronary arteries separately using CTA and reduce the burden of subjecting patients to multiple exams.
2.6. Valvular diseases
Libman-Sacks or marantic endocarditis consists of non-infective, verrucous valvular vegetation and is the most characteristic valve lesion in SLE (Figure 3). Most frequently, this involves the mitral valve and to a lesser degree, the aortic valve. Multi-valvular involvement can also occur in SLE. Roldan et al. described valvular abnormalities in 61% of 69 cases of SLE unrelated to disease severity, disease activity, or disease duration (100). An older prospective study of 132 consecutive patients reported valvular lesions in 22.7% of the cases associated with the presence of antiphospholipid antibodies (101). In SS, tricuspid regurgitation was seen in 40%, whereas thickening of the aortic valve and mitral valve leaflets in 12% and 8% respectively (45). Although echocardiography remains the modality of choice for evaluating valvular lesions, CMR can provide a highly reproducible serial assessment of valvular disease for the characterization of verrucous vegetation. CMR allows objective and reproducible quantification of mitral or tricuspid regurgitation and aortic valve assessment in patients with ARDs with low inter-study variability and operator dependence (102).
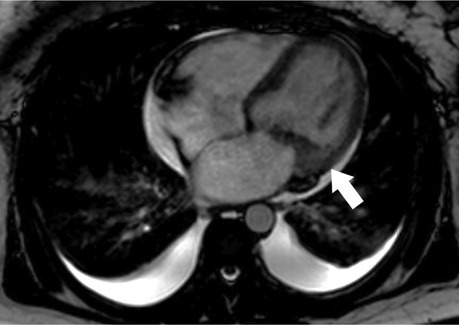
Figure 3. Libman-Sacks endocarditis on CMR. Cine 4-chamber view shows mass-like mitral valve vegetation and valve thickening (white arrow) consistent with Libman-Sacks endocarditis.
2.7. Pulmonary hypertension (PH)
Patients with ARDs often develop pulmonary hypertension during the natural course of the disease processes. Of all the ARDs, SS has the highest prevalence of pulmonary hypertension in up to half of the patients secondary to lung involvement, with a 1-year survival rate of only 50% (48, 103). The presence of PH in limited cutaneous SSc without concomitant interstitial lung disease results in an overall poor prognosis (32). Secondary pulmonary hypertension is seen in patients with Sjogren's syndrome (47) and is the leading cause of death in MTCD (104). Venous thrombo-embolic complications may cause pulmonary hypertension and right heart failure in SLE (29). The prevalence of asymptomatic pulmonary arterial hypertension in SLE was reported to be 10.8%, with a female-to-male ratio of 10:1 (105).
Recently, the Pulmonary Vascular Research Institute recommended cardiac MRI to monitor right ventricular (RV) function in patients with pulmonary hypertension (106). On CMR, the most common but non-specific sign of PH is the presence of LGE at the RV insertion points, which is not related to disease severity (Figure 4). Diagnosis of pulmonary hypertension-related heart disease can be performed with CMR, but diagnosis of pulmonary hypertension alone has been challenging. A composite CMR model of interventricular septum angle (IVS), ventricular mass index (VMI), and black blood slow flow can be used for non-invasive diagnosis of PH with improved sensitivity (93%) and specificity (79%) with high correlation to mPAP (107). The interventricular septal (IVS) angle on CMR can help differentiate pre- and postcapillary PH from isolated postcapillary PH. An elevated systolic interventricular septal angle ≥ 160° in patients with combined pre- and postcapillary pulmonary hypertension can predict the presence of left-sided heart disease (108).
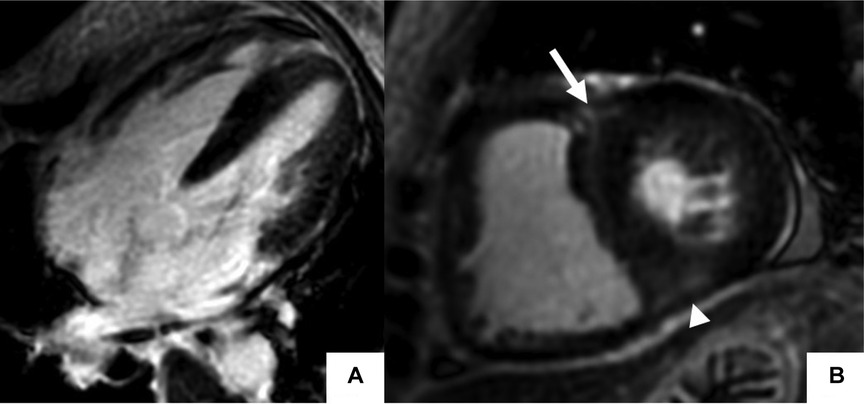
Figure 4. Late gadolinium enhancement cardiac MR (LGE-CMR) in polymyositis with pulmonary hypertension. Late gadolinium enhancement phase-sensitive inversion recovery (LGE-PSIR) images in the 4-chamber (A), and short-axis (B) showed increased RV and LV thickness with focal LGE at the superior (arrow) and inferior (arrowhead) RV insertion points in a patient with polymyositis with cardiac involvement.
2.8. Diastolic or systolic heart failure
Excellent image quality and high spatial resolution achievable by CMR allow highly reproducible measurements of ventricular volumes and function (109). In SS, right heart failure is common and mostly secondary to lung fibrosis and pulmonary hypertension (44). LV impairment is the common endpoint and imaging biomarker in ARD and acute or chronic diffuse subendocardial vasculitis (DSV) (19).
LV diastolic dysfunction is seen in SS in 29% of the patients (45), and 42% of the cases of PM and DM (110). In limited SSc, subclinical LV dysfunction as evidenced by echocardiography and perfusion scintigraphy was found in 42% (32). In Sjogren's syndrome, diastolic dysfunction was found in 50% of evaluated patients and was noted to be independent of pericardial findings (111).
3. CMR signatures for risk stratification, prognostication, and guiding treatment strategies in ARDs
The development of clinically overt cardiac signs in patients with ARDs indicates advanced cardiac disease and carries a poor prognosis (112). Identification of disease acuity and various cardiovascular diseases process such as vasculitis, myocarditis, and myocardial infarction itself influences the CV risk stratification of ARD patients (12). Every morphologic or functional change in the myocardium, detected by any diagnostic technique, should motivate the early start of ACE inhibitors and b-blocker in patients with ARDs. Conventional and biologic disease-modifying antirheumatic drugs (DMARDs) have considerably improved long-term outcomes in ARDs not only by suppressing systemic inflammation but also by lowering the CVD burden (113–115). Improvement in inflammatory joint disease using anti-TNF-α therapies has also been associated with a reduction in cardiac events, further supporting the use of these disease-modifying agents (116, 117).
There are a lot of recent publications on how CMR can add value to risk stratification and the management of patients with ARDs. For example, the presence of late gadolinium enhancement (LGE) on CMR is related to a worse cardiovascular prognosis in these patients (118). Some studies suggest that edema/fibrosis visualization with CMR may have the potential to inform cardiac and rheumatic treatment modification in ARDs with or without abnormal routine cardiac evaluation.
Currently, there are no large multicenter trials to confirm the prognostic role of CMR described in smaller clinical trials and observational studies. However, early information provided by CMR, even in patients with normal systolic function, may be useful to change risk stratification and motivate the early start of cardiac medication (119).
CMR can help in the identification of occult myocardial lesions such as myocardial edema, myocarditis, diffuse subendocardial fibrosis, and subendocardial myocardial infarction that can be potentially reversed with appropriate management in treatment naïve ARDs (24). The recent clinical consensus document by EACVI advocates for the use of CMR for evaluating the effectiveness of immunomodulatory or cardioprotective treatment in patients with ARDs (23).
3.1. RA
Identification of myocarditis on CMR in RA is associated with a lower frequency of disease relapse and heart failure (18). When present, myocarditis, in contrast to pericarditis, is an indication for treatment with corticosteroids in RA (3). Minimal disease activity and short disease duration are associated with better outcomes in RA (13). The presence of myopericarditis in CMR can precede the development of relapse and subsequent congestive heart failure in patients with RA, a poor prognostic indicator in these patients (18).
Traditional DMARDs either as monotherapy or in combination with anti-TNF-α agents are extremely effective in RA (120). CMR-guided therapy had better outcomes in terms of a decrease in pericarditis recurrence and exposure to steroids in patients treated with colchicine and nonsteroidal anti-inflammatory drugs as the first-line therapy for acute pericarditis (48). In patients with RA, improvement in CFR without significant changes in plasma ADMA levels can help to monitor the effect of anti-rheumatic therapy with DMARD or anti-TNFα treatment on endothelial dysfunction and disease activity (2). These parameters can be tracked using perfusion CMR for treatment monitoring in these patients. The administration of tocilizumab (TCZ) is associated with reduced RA disease activity and improved LV function as assessed by CMR (121). Additionally, TCZ can improve LVEF and cause a decline in LV mass index which is associated with a decrease in disease activity (122).
3.2. SLE
Identifying subclinical myocardial involvement in CMR can add prognostic value in SLE as cardiovascular complications are a leading cause of death in these patients, but clinical signs may be identified in <10% of patients (30). In autopsy studies, myocarditis was noted in up to 30%–50% of lupus patients (31, 123), so it is a common subclinical feature of the disease. Accordingly, T2-mapping techniques can frequently identify increased myocardial T2 in SLE patients with subclinical myocardial edema (124). Therefore, low-grade myocardial inflammation may be detected on CMR in SLE patients with quiescent disease and normal cardiac function, who may benefit from the initiation of immunosuppressive treatment.
Interestingly, the introduction of corticosteroids to SLE management has decreased the incidence of autopsy-identified lupus myocarditis from 50%–75% (31, 125) to about 25%–30% (123, 126). Lupus myocarditis presenting as cardiogenic shock may require mechanical support and other drugs such as rituximab, intravenous immunoglobulin azathioprine, and cyclophosphamide (127). CMR can help in assessing accurate improvement of left ventricle function and response to steroids in such patients.
Significantly higher inflammatory markers, LV mass, native T1 and T2 values, and reduced longitudinal strain has been reported in patients with SLE. Greater improvement in native T1 and T2 values in patients after intensified anti-inflammatory treatment suggests a role of native T1 and T2 as significant predictors of treatment response on follow-up CMR (128).
3.3. Scleroderma
Myocarditis is a common finding in SSc with recent-onset cardiac involvement and its early diagnosis allows timely immunosuppressive treatment leading to the prevention of cardiac damage in most cases (129) (Figure 5). Rest CMR perfusion index can be used to monitor patients with scleroderma with early myocardial perfusion defects on treatment with Nifedipine, a calcium antagonist. A significant increase in the MRI perfusion index was reported after 14 days of oral treatment with Nifedipine 60 mg/day with a mean perfusion index of 0.26 v 0.19 at baseline, p = 0.0003. Improvements were also noted in systolic and diastolic strain rates on TTE post-therapy thus implicating the utility of CMR strain in the follow-up of such patients (130).
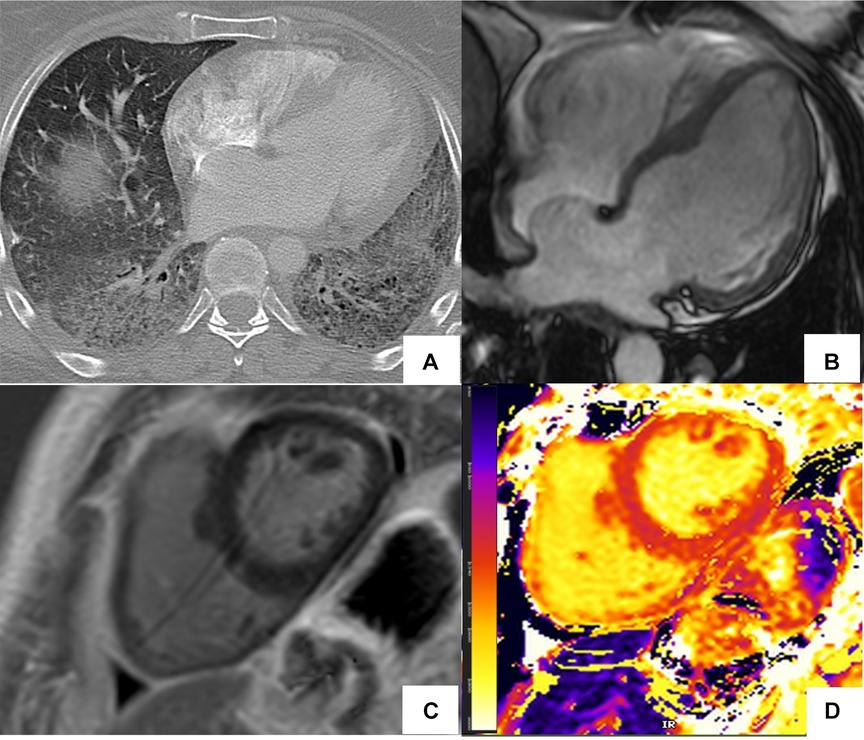
Figure 5. Abnormal cardiac MR (CMR) T1 mapping in subclinical myocardial inflammation in a patient with scleroderma. 37-year-old female with scleroderma-related interstitial lung disease (A Chest CT) and increasing dyspnea on exertion. 1.5 T cine CMR had a normal LV ejection fraction of 59% and no regional wall motion abnormality. 4ch cine showed flattening of the inter-ventricular septum consistent with known pulmonary hypertension (B) On short-axis late gadolinium enhancement phase-sensitive inversion recovery (LGE-PSIR) there was no myocardial late gadolinium enhancement, only trace pericardial effusion (C) Native T1 values were elevated to 1,250 ms on the native T1 color map (D) suggesting active myocardial inflammation.
Stress CMR can detect silent myocardial Raynaud phenomena (RP) in patients with connective tissue diseases (CTD). Myocardial perfusion reserve index (MPRI) reduction is common in both primary and secondary RP, but more severe in secondary RP irrespective of treatment with calcium channel blockers. Identification of abnormal MPRI can help with the early initiation of treatment pharmacological treatment (131).
3.4. Vasculitis
CMR can help with treatment monitoring and temporal changes in acute or chronic diffuse subendocardial vasculitis (DSV). In a small study of 20 patients with ARD, CMR detected DSV had a rapid clinical improvement after treatment modification with the resolution of CMR findings on 75% of patients. In chronic DSV, clinical improvement was seen after the initiation of ACE inhibitors but there were no changes in CMR findings of DSV on follow-up imaging. CMR also identified improvement of LV dysfunction in most acute and some chronic DSV. However, those with chronic DSV never achieved normal LV function (19). In chronic cases, identifying DSV to help with an early start of ACE inhibitors even in those with normal LVEF may help prevent overt LV dysfunction (132).
3.5. ARD-related PAH
PAH may complicate various ARDs such as SSc, SLE, MCTD, and RA. Novel targeted therapies have demonstrated a trend toward improvement of outcomes in ARD-related PAH (133–135). CMR volumetric and functional indices and native T1 mapping help with the assessment of treatment response and risk stratification when incorporated into PH risk scores, and carry prognostic value in PH. The prognostic features in patients with connective tissue disease (CTD) are found to be different from other PAH subgroups with metrics such as ventricular-vascular coupling (ratio of end-systolic to arterial elastances i.e., Ees/Ea) and RV mass being more significant than RV function and volume (136). The European Society of Cardiology and European Respiratory Society and the REVEAL (North American Registry to Evaluate Early and Long-Term PAH Disease Management) risk score calculator (REVEAL 2.0) identified CMR-based percentage-predicted right ventricular end-systolic volume index (RV ESVi) can improve risk stratification in PH when used in conjunction with current risk stratification approaches. RV ESVi threshold of 227% or a left ventricular end-diastolic volume index of 58 ml/m2 on CMR identified patients at high risk of 1-year mortality (137).
RV ejection fraction was the strongest and most well-established predictor of mortality in PAH in eight studies (539 patients) that investigated 21 different CMR findings. In addition, increased RV volumes and decreased LV end-diastolic volume at baseline have been associated with a higher mortality risk in PAH patients (138). Furthermore, because of lower measurement variability, ejection fraction derived from CMR is more cost-effective in PAH medication trials than echocardiography (139).
Other CMR features can be used for prognostic characterization in patients with PH. The myocardial strain has emerged as an early marker for biventricular dysfunction as well as a predictor for mortality (140). An elevated systolic interventricular septal angle ≥160° in patients with combined pre- and postcapillary pulmonary hypertension is predictive of left-sided disease and a poor prognostic indicator with an increased risk of death (103). Increased trabeculation at the marginal IVS on CMR is found to be associated with severe PH, reduced RV ejection fraction (RVEF), and exercise tolerance (141).
CMR can help in monitoring response to conventional and newer therapies for PH. The effect of sildenafil in addition to conventional treatment of PAH in patients with SLE and SSc has been previously evaluated with CMR. It has been shown that the combination treatment reduced the RV mass and improved cardiac function and exercise capacity in patients with PAH, WHO functional class III (142). CMR can be used to assess the response to newer combination therapy with Ambrisentan and Tadalafil by measuring improved RV contractility and function-reduced RV mass in CTD PAH such as in treatment-naive patients with SSc–PAH (143, 144).
4. CMR to identify cardiotoxicity related to immunomodulation therapies
Cardiotoxicity occurs mainly from polypharmacy, overdosage, and long pharmacotherapy with conventional disease-modifying antirheumatic drugs (DMARD) and biologic agents such as Tumor Necrosis Factor (TNF)-α blockers and IL-6 receptor Inhibitors (145–147). Acute and chronic adverse events include infusion-related hypertension, myocardial ischemia, myopericarditis, restrictive cardiomyopathy (Figure 6), and congestive heart failure (148). CMR has proven clinical utility in identifying cardiotoxicity caused by immune checkpoint inhibitors (ICI) (149). A study on 104 patients with ICI-associated myocarditis showed frequent CMR abnormalities correlating with histological findings, including LGE in areas of replacement fibrosis and T2 abnormalities in lymphocytic infiltration (150).
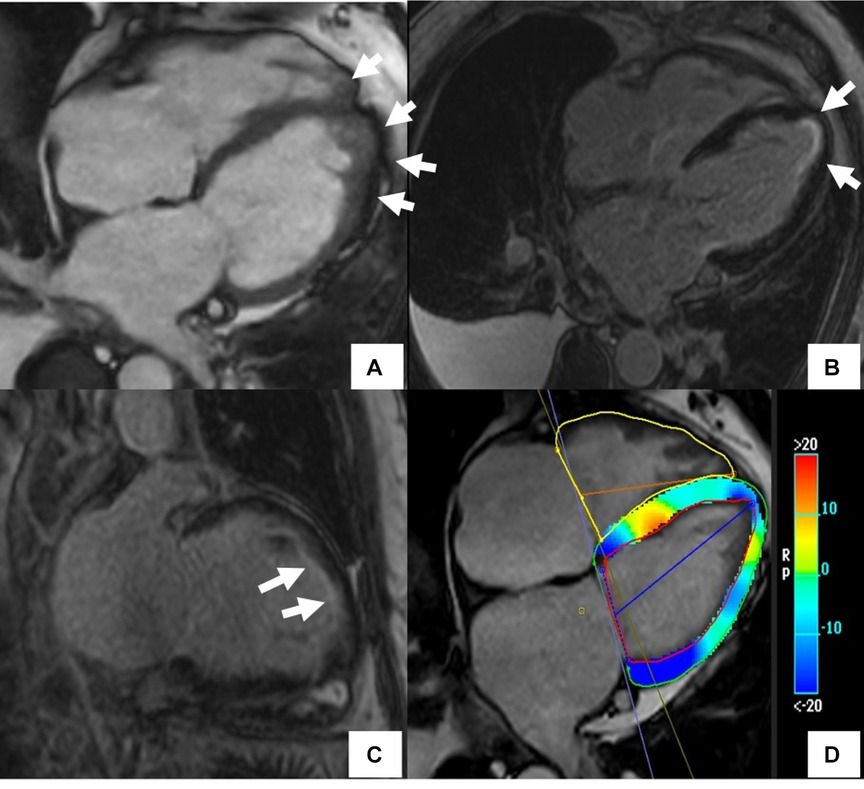
Figure 6. Cardiac MR (CMR) findings in a patient with drug-induced cardiotoxicity. The cine image in the 4-chamber plane (A) showed hyper-trabeculations in LV and RV apex (arrows). Late gadolinium enhancement phase-sensitive inversion recovery (LGE-PSIR) images showed diffuse subendocardial fibrosis (arrows) (B,C). There was also abnormal longitudinal strain relatively preserving the base on the feature tracking color map (D).
Chronic use of hydroxyl-chloroquine may result in acquired lysosomal storage disorder, leading to drug-induced cardiomyopathy characterized by concentric hypertrophy on CMR and rhythm disturbances on ECG (151). CMR can also be used to establish treatment responses in patients with extracardiac symptoms when there is a secondary improvement in cardiac imaging biomarkers (119).
5. Limitations of CMR, recent advances, current research gaps, and potential developments in the field
One of the limitations of CMR is related to the minimal risk of developing nephrogenic systemic fibrosis (NSF), particularly in patients with renal dysfunction, typically with a glomerular filtration rate of <30 ml/min/1.73 m2 and/or patients on hemodialysis (152). However the risk of NSF is very low with macrocyclic contrast agents estimated at 0.7%. There is a theoretical risk of brain accumulation of GBCA that has been discussed in the literature (153). Other limitations include a lack of cost-effectiveness analysis of CMR use in patients with ARDs. Finally, CMR is contra-indicated in patients with metal fragments in the eyes and may require special safety protocols in patients with devices that are not MR-conditional (154).
Research gaps exist in at least three levels of evidence to document the necessity of additive anti-rheumatic treatment in ARD patients with CMR evidence of myocardial inflammation; observational data derived from imaging registries with adequate phenotype, treatment, and outcome data is not available; longitudinal and long-term CMR-based observational studies monitoring ARD patients on antirheumatic medication is lacking; randomized controlled trials of antirheumatic treatment based informed by CMR findings alone, with long-term outcomes, are needed (155).
Advanced CMR applications have the potential to characterize the entire spectrum of cardiovascular involvement and provide a one-stop shop for patients with ARDs. Currently, there is limited data on the utility of advanced imaging techniques such as texture analysis, strain, and 4D flow assessment in patients with ARDs. Recent studies show the advantage of radiomics-based texture analysis of T1 and T2 mapping to define infarct-like myocarditis with high sensitivity and specificity (156). Biventricular strain is found to be significantly impaired in PH and allows early detection of right and left heart dysfunction (157) and in patients with cardiotoxicity (158) (Figure 6). 4D- flow CMR is being investigated for its ability to identify abnormal flow patterns in the main pulmonary artery (MPA) and its association with PH. A recent study showed the potential for 4D-flow CMR to estimate mean PAP (mPAP), track PVR changes, MPA wall shear stress (WSS), and quantification of the severity of tricuspid regurgitation in pulmonary arterial hypertension and PH due to left heart disease, chronic lung disease or chronic thrombo-emboli (CTE-PH) (159).
Potential areas of research identified include the need for larger multicenter observational or interventional trials to validate CMR prognostic markers in ARDs. There are also few studies with a head-to-head comparison of CMR diagnostic performance compared to routinely used cardiovascular imaging modalities or endomyocardial biopsy for diagnosis. Other areas of potential research include the assessment of the utility of CMR in monitoring response to immunomodulatory and cardioprotective medications and targeted therapies for myocardial fibrosis (160). Exploratory studies designed specifically to identify associations between CMR biomarkers and circulating levels of inflammatory biomarkers and ARD-associated autoantibodies are needed. Finally, prospective studies on the integration of CMR with electroanatomic mapping to identify sites of the arrhythmogenic substrate to guide ablations and improve outcomes are desirable (161).
Conclusion
CMR has the ability to identify cardiovascular abnormalities in patients with ARDs and can be used as a tool for the early detection of disease in asymptomatic patients. CMR is a promising method for the prognostic characterization of these patients and may be used to identify patients that could benefit from pharmacological interventions. Finally, CMR is a robust tool that could add value to monitoring treatment response and tailoring treatment strategies for patients with ARDs and cardiovascular involvement.
Author contributions
AG was instrumental in formulating the outline, she has written the manuscript under the guidance of her mentor KO; specifically, AG created the bibliography, collected the images, wrote the figure legends, collaborated with the team, and submitted the final manuscript. All authors contributed to the article and approved the submitted version.
Conflict of interest
The authors declare that the research was conducted in the absence of any commercial or financial relationships that could be construed as a potential conflict of interest.
Publisher's note
All claims expressed in this article are solely those of the authors and do not necessarily represent those of their affiliated organizations, or those of the publisher, the editors and the reviewers. Any product that may be evaluated in this article, or claim that may be made by its manufacturer, is not guaranteed or endorsed by the publisher.
References
1. Pohl D, Benseler S. Systemic inflammatory and autoimmune disorders. Handb Clin Neurol. (2013) 112:1243–52. doi: 10.1016/B978-0-444-52910-7.00047-7
2. Sitia S, Atzeni F, Sarzi-Puttini P, Di Bello V, Tomasoni L, Delfino L, et al. Cardiovascular involvement in systemic autoimmune diseases. Autoimmun Rev. (2009) 8(4):281–6. doi: 10.1016/j.autrev.2008.08.004
3. Knockaert DC. Cardiac involvement in systemic inflammatory diseases. Eur Heart J. (2007) 28(15):1797–804. doi: 10.1093/eurheartj/ehm193
4. Goldblatt F, O’Neill SG. Clinical aspects of autoimmune rheumatic diseases. Lancet. (2013) 382(9894):797–808. doi: 10.1016/S0140-6736(13)61499-3
5. Hollan I, Meroni PL, Ahearn JM, Cohen Tervaert JW, Curran S, Goodyear CS, et al. Cardiovascular disease in autoimmune rheumatic diseases. Autoimmun Rev. (2013) 12(10):1004–15. doi: 10.1016/j.autrev.2013.03.013
6. Avina-Zubieta JA, Choi HK, Sadatsafavi M, Etminan M, Esdaile JM, Lacaille D. Risk of cardiovascular mortality in patients with rheumatoid arthritis: a meta-analysis of observational studies. Arthritis Rheum. (2008) 59(12):1690–7. doi: 10.1002/art.24092
7. Sherer Y, Shoenfeld Y. Mechanisms of disease: atherosclerosis in autoimmune diseases. Nat Clin Pract Rheumatol. (2006) 2(2):99–106. doi: 10.1038/ncprheum0092
8. Kitas GD, Gabriel SE. Cardiovascular disease in rheumatoid arthritis: state of the art and future perspectives. Ann Rheum Dis. (2011) 70(1):8–14. doi: 10.1136/ard.2010.142133
9. Symmons DP, Gabriel SE. Epidemiology of CVD in rheumatic disease, with a focus on RA and SLE. Nat Rev Rheumatol. (2011) 7(7):399–408. doi: 10.1038/nrrheum.2011.75
10. Mavrogeni S, Spargias K, Markussis V, Kolovou G, Demerouti E, Papadopoulou E, et al. Myocardial inflammation in autoimmune diseases: investigation by cardiovascular magnetic resonance and endomyocardial biopsy. Inflamm Allergy Drug Targets. (2009) 8(5):390–7. doi: 10.2174/1871528110908050390
11. Mavrogeni S, Pepe A, Lombardi M. Evaluation of myocardial iron overload using cardiovascular magnetic resonance imaging. Hellenic J Cardiol. (2011) 52(5):385–90.21940285
12. Mavrogeni S, Sfikakis PP, Gialafos E, Bratis K, Karabela G, Stavropoulos E, et al. Cardiac tissue characterization and the diagnostic value of cardiovascular magnetic resonance in systemic connective tissue diseases. Arthritis Care Res (Hoboken. (2014) 66(1):104–12. doi: 10.1002/acr.22181
13. Wolfe F, Rasker JJ, Boers M, Wells GA, Michaud K. Minimal disease activity, remission, and the long-term outcomes of rheumatoid arthritis. Arthritis Rheum. (2007) 57(6):935–42. doi: 10.1002/art.22895
14. Mavrogeni SI, Sfikakis PP, Markousis-Mavrogenis G, Bournia VK, Poulos G, Koutsogeorgopoulou L, et al. Cardiovascular magnetic resonance imaging pattern in patients with autoimmune rheumatic diseases and ventricular tachycardia with preserved ejection fraction. Int J Cardiol. (2019) 284:105–9. doi: 10.1016/j.ijcard.2018.10.067
15. Mavrogeni S, Manoussakis MN. Myocarditis and subclavian stenosis in takayasu arteritis. Int J Cardiol. (2011) 148(2):223–4. doi: 10.1016/j.ijcard.2009.05.008
16. Mavrogeni S, Sfikakis PP, Karabela G, Stavropoulos E, Spiliotis G, Gialafos E, et al. Cardiovascular magnetic resonance imaging in asymptomatic patients with connective tissue disease and recent onset left bundle branch block. Int J Cardiol. (2014) 171(1):82–7. doi: 10.1016/j.ijcard.2013.11.059
17. Dimitroulas T, Giannakoulas G, Karvounis H, Garyfallos A, Settas L, Kitas GD. Micro- and macrovascular treatment targets in scleroderma heart disease. Curr Pharm Des. (2014) 20(4):536–44. doi: 10.2174/13816128113199990555
18. Mavrogeni S, Bratis K, Sfendouraki E, Papadopoulou E, Kolovou G. Myopericarditis, as the first sign of rheumatoid arthritis relapse, evaluated by cardiac magnetic resonance. Inflamm Allergy Drug Targets. (2013) 12(3):206–11. doi: 10.2174/1871528111312030008
19. Mavrogeni S, Sfikakis PP, Gialafos E, Karabela G, Stavropoulos E, Sfendouraki E, et al. Diffuse, subendocardial vasculitis. A new entity identified by cardiovascular magnetic resonance and its clinical implications. Int J Cardiol. (2013) 168(3):2971–2. doi: 10.1016/j.ijcard.2013.04.116
20. Raman SV, Aneja A, Jarjour WN. CMR In inflammatory vasculitis. J Cardiovasc Magn Reson. (2012) 14(1):82. doi: 10.1186/1532-429X-14-82
21. Mavrogeni SI, Kitas GD, Dimitroulas T, Sfikakis PP, Seo P, Gabriel S, et al. Cardiovascular magnetic resonance in rheumatology: current status and recommendations for use. Int J Cardiol. (2016) 217:135–48. doi: 10.1016/j.ijcard.2016.04.158
22. Rav-Acha M, Plot L, Peled N, Amital H. Coronary involvement in takayasu’s arteritis. Autoimmun Rev. (2007) 6(8):566–71. doi: 10.1016/j.autrev.2007.04.001
23. Mavrogeni S, Pepe A, Nijveldt R, Ntusi N, Sierra-Galan LM, Bratis K, et al. Cardiovascular magnetic resonance in autoimmune rheumatic diseases: a clinical consensus document by the European Association of Cardiovascular Imaging. Eur Heart J Cardiovasc Imaging. (2022) 23(9):e308–e22. doi: 10.1093/ehjci/jeac134
24. Mavrogeni S, Markousis-Mavrogenis G, Koutsogeorgopoulou L, Dimitroulas T, Bratis K, Kitas GD, et al. Cardiovascular magnetic resonance imaging pattern at the time of diagnosis of treatment naive patients with connective tissue diseases. Int J Cardiol. (2017) 236:151–6. doi: 10.1016/j.ijcard.2017.01.104
25. Leo I, Nakou E, Artico J, Androulakis E, Wong J, Moon JC, et al. Strengths and weaknesses of alternative noninvasive imaging approaches for microvascular ischemia. J Nucl Cardiol. (2023) 30(1):227–38. doi: 10.1007/s12350-022-03066-6
26. Friedrich MG, Sechtem U, Schulz-Menger J, Holmvang G, Alakija P, Cooper LT, et al. Cardiovascular magnetic resonance in myocarditis: a JACC white paper. J Am Coll Cardiol. (2009) 53(17):1475–87. doi: 10.1016/j.jacc.2009.02.007
27. Ferreira VM, Schulz-Menger J, Holmvang G, Kramer CM, Carbone I, Sechtem U, et al. Cardiovascular magnetic resonance in nonischemic myocardial inflammation: expert recommendations. J Am Coll Cardiol. (2018) 72(24):3158–76. doi: 10.1016/j.jacc.2018.09.072
28. Amano Y, Omori Y, Ando C, Yanagisawa F, Suzuki Y, Tang X, et al. Clinical importance of myocardial T(2) mapping and texture analysis. Magn Reson Med Sci. (2021) 20(2):139–51. doi: 10.2463/mrms.rev.2020-0007
29. del Rincon ID, Williams K, Stern MP, Freeman GL, Escalante A. High incidence of cardiovascular events in a rheumatoid arthritis cohort not explained by traditional cardiac risk factors. Arthritis Rheum. (2001) 44(12):2737–45. doi: 10.1002/1529-0131(200112)44:12%3C2737::AID-ART460%3E3.0.CO;2
30. Moder KG, Miller TD, Tazelaar HD. Cardiac involvement in systemic lupus erythematosus. Mayo Clin Proc. (1999) 74(3):275–84. doi: 10.4065/74.3.275
31. Wijetunga M, Rockson S. Myocarditis in systemic lupus erythematosus. Am J Med. (2002) 113(5):419–23. doi: 10.1016/S0002-9343(02)01223-8
32. Byers RJ, Marshall DA, Freemont AJ. Pericardial involvement in systemic sclerosis. Ann Rheum Dis. (1997) 56(6):393–4. doi: 10.1136/ard.56.6.393
33. Handa R, Gupta K, Malhotra A, Jain P, Kamath PK, Aggarwal P, et al. Cardiac involvement in limited systemic sclerosis: non-invasive assessment in asymptomatic patients. Clin Rheumatol. (1999) 18(2):136–9. doi: 10.1007/s100670050071
34. Dalakas MC, Hohlfeld R. Polymyositis and dermatomyositis. Lancet. (2003) 362(9388):971–82. doi: 10.1016/S0140-6736(03)14368-1
35. Levin MD, Zoet-Nugteren SK, Markusse HM. Myocarditis and primary sjogren’s syndrome. Lancet. (1999) 354(9173):128–9. doi: 10.1016/S0140-6736(99)02251-5
36. Vitali C, Bencivelli W, Isenberg DA, Smolen JS, Snaith ML, Sciuto M, et al. Disease activity in systemic lupus erythematosus: report of the consensus study group of the European workshop for rheumatology research. II. Identification of the variables indicative of disease activity and their use in the development of an activity score. The European Consensus Study Group for Disease Activity in SLE. Clin Exp Rheumatol. (1992) 10(5):541–7.1458710
37. Jesus D, Larosa M, Henriques C, Matos A, Zen M, Tome P, et al. Systemic lupus erythematosus disease activity score (SLE-DAS) enables accurate and user-friendly definitions of clinical remission and categories of disease activity. Ann Rheum Dis. (2021) 80(12):1568–74. doi: 10.1136/annrheumdis-2021-220363
38. Friedrich MG, Strohm O, Schulz-Menger J, Marciniak H, Luft FC, Dietz R. Contrast media-enhanced magnetic resonance imaging visualizes myocardial changes in the course of viral myocarditis. Circulation. (1998) 97(18):1802–9. doi: 10.1161/01.CIR.97.18.1802
39. Abdel-Aty H, Siegle N, Natusch A, Gromnica-Ihle E, Wassmuth R, Dietz R, et al. Myocardial tissue characterization in systemic lupus erythematosus: value of a comprehensive cardiovascular magnetic resonance approach. Lupus. (2008) 17(6):561–7. doi: 10.1177/0961203308089401
40. Singh JA, Woodard PK, Davila-Roman VG, Waggoner AD, Gutierrez FR, Zheng J, et al. Cardiac magnetic resonance imaging abnormalities in systemic lupus erythematosus: a preliminary report. Lupus. (2005) 14(2):137–44. doi: 10.1191/0961203305lu2050oa
41. Abdel-Aty H, Boye P, Zagrosek A, Wassmuth R, Kumar A, Messroghli D, et al. Diagnostic performance of cardiovascular magnetic resonance in patients with suspected acute myocarditis: comparison of different approaches. J Am Coll Cardiol. (2005) 45(11):1815–22. doi: 10.1016/j.jacc.2004.11.069
42. Cui C, Wang S, Lu M, Duan X, Wang H, Jia L, et al. Detection of recent myocardial infarction using native T1 mapping in a swine model: a validation study. Sci Rep. (2018) 8(1):7391. doi: 10.1038/s41598-018-25693-1
43. Dennert R, Crijns HJ, Heymans S. Acute viral myocarditis. Eur Heart J. (2008) 29(17):2073–82. doi: 10.1093/eurheartj/ehn296
44. Lurz P, Luecke C, Eitel I, Fohrenbach F, Frank C, Grothoff M, et al. Comprehensive cardiac magnetic resonance imaging in patients with suspected myocarditis: the MyoRacer-trial. J Am Coll Cardiol. (2016) 67(15):1800–11. doi: 10.1016/j.jacc.2016.02.013
45. Giri S, Chung YC, Merchant A, Mihai G, Rajagopalan S, Raman SV, et al. T2 quantification for improved detection of myocardial edema. J Cardiovasc Magn Reson. (2009) 11(1):56. doi: 10.1186/1532-429X-11-56
46. O’Brien AT, Gil KE, Varghese J, Simonetti OP, Zareba KM. T2 mapping in myocardial disease: a comprehensive review. J Cardiovasc Magn Reson. (2022) 24(1):33. doi: 10.1186/s12968-022-00866-0
47. Ferri C, Valentini G, Cozzi F, Sebastiani M, Michelassi C, La Montagna G, et al. Systemic sclerosis: demographic, clinical, and serologic features and survival in 1,012 Italian patients. Medicine (Baltimore). (2002) 81(2):139–53. doi: 10.1097/00005792-200203000-00004
48. Maione S, Cuomo G, Giunta A, de Horatio L T, La Montagna G, Manguso F, et al. Echocardiographic alterations in systemic sclerosis: a longitudinal study. Semin Arthritis Rheum. (2005) 34(5):721–7. doi: 10.1016/j.semarthrit.2004.11.001
49. Bezerra MC, Saraiva F Jr, Carvalho JF, Caleiro MT, Goncalves CR, Borba EF. Cardiac tamponade due to massive pericardial effusion in mixed connective tissue disease: reversal with steroid therapy. Lupus. (2004) 13(8):618–20. doi: 10.1191/0961203303lu1043xx
50. Gyongyosi M, Pokorny G, Jambrik Z, Kovacs L, Kovacs A, Makula E, et al. Cardiac manifestations in primary sjogren’s syndrome. Ann Rheum Dis. (1996) 55(7):450–4. doi: 10.1136/ard.55.7.450
51. Alraies MC, AlJaroudi W, Yarmohammadi H, Yingchoncharoen T, Schuster A, Senapati A, et al. Usefulness of cardiac magnetic resonance-guided management in patients with recurrent pericarditis. Am J Cardiol. (2015) 115(4):542–7. doi: 10.1016/j.amjcard.2014.11.041
52. Rosmini S, Seraphim A, Knott K, Brown JT, Knight DS, Zaman S, et al. Non-invasive characterization of pleural and pericardial effusions using T1 mapping by magnetic resonance imaging. Eur Heart J Cardiovasc Imaging. (2022) 23(8):1117–26. doi: 10.1093/ehjci/jeab128
53. Geske JB, Anavekar NS, Nishimura RA, Oh JK, Gersh BJ. Differentiation of constriction and restriction: complex cardiovascular hemodynamics. J Am Coll Cardiol. (2016) 68(21):2329–47. doi: 10.1016/j.jacc.2016.08.050
54. Glower DD. Sticking points in magnetic resonance diagnosis of constrictive pericarditis. J Thorac Cardiovasc Surg. (2016) 151(5):1356–7. doi: 10.1016/j.jtcvs.2015.12.042
55. Francone M, Dymarkowski S, Kalantzi M, Rademakers FE, Bogaert J. Assessment of ventricular coupling with real-time cine MRI and its value to differentiate constrictive pericarditis from restrictive cardiomyopathy. Eur Radiol. (2006) 16(4):944–51. doi: 10.1007/s00330-005-0009-0
56. Vidalakis E, Kolentinis MK, Gawor M, Vasquez M, Nagel E. CMR in pericardial diseases—an update. Curr Cardiovasc Imaging Rep. (2020) 13:1–9. doi: 10.1007/s12410-020-9535-z
57. Flett AS, Hasleton J, Cook C, Hausenloy D, Quarta G, Ariti C, et al. Evaluation of techniques for the quantification of myocardial scar of differing etiology using cardiac magnetic resonance. JACC Cardiovasc Imaging. (2011) 4(2):150–6. doi: 10.1016/j.jcmg.2010.11.015
58. Mavrogeni S, Markousis-Mavrogenis G, Koutsogeorgopoulou L, Kolovou G. Cardiovascular magnetic resonance imaging: clinical implications in the evaluation of connective tissue diseases. J Inflamm Res. (2017) 10:55–61. doi: 10.2147/JIR.S115508
59. Kim RJ, Chen EL, Lima JA, Judd RM. Myocardial Gd-DTPA kinetics determine MRI contrast enhancement and reflect the extent and severity of myocardial injury after acute reperfused infarction. Circulation. (1996) 94(12):3318–26. doi: 10.1161/01.CIR.94.12.3318
60. Wu KC. CMR Of microvascular obstruction and hemorrhage in myocardial infarction. J Cardiovasc Magn Reson. (2012) 14(1):68. doi: 10.1186/1532-429X-14-68
61. Moon BF, Iyer SK, Hwuang E, Solomon MP, Hall AT, Kumar R, et al. Iron imaging in myocardial infarction reperfusion injury. Nat Commun. (2020) 11(1):3273. doi: 10.1038/s41467-020-16923-0
62. Wassmuth R, Gobel U, Natusch A, Schneider W, Kettritz R, Dietz R, et al. Cardiovascular magnetic resonance imaging detects cardiac involvement in churg-strauss syndrome. J Card Fail. (2008) 14(10):856–60. doi: 10.1016/j.cardfail.2008.07.227
63. Mavrogeni S, Vassilopoulos D. Is there a place for cardiovascular magnetic resonance imaging in the evaluation of cardiovascular involvement in rheumatic diseases? Semin Arthritis Rheum. (2011) 41(3):488–96. doi: 10.1016/j.semarthrit.2011.04.004
64. Mahrholdt H, Goedecke C, Wagner A, Meinhardt G, Athanasiadis A, Vogelsberg H, et al. Cardiovascular magnetic resonance assessment of human myocarditis: a comparison to histology and molecular pathology. Circulation. (2004) 109(10):1250–8. doi: 10.1161/01.CIR.0000118493.13323.81
65. Assomull RG, Lyne JC, Keenan N, Gulati A, Bunce NH, Davies SW, et al. The role of cardiovascular magnetic resonance in patients presenting with chest pain, raised troponin, and unobstructed coronary arteries. Eur Heart J. (2007) 28(10):1242–9. doi: 10.1093/eurheartj/ehm113
66. Neumann T, Manger B, Schmid M, Kroegel C, Hansch A, Kaiser WA, et al. Cardiac involvement in churg-strauss syndrome: impact of endomyocarditis. Medicine (Baltimore). (2009) 88(4):236–43. doi: 10.1097/MD.0b013e3181af35a5
67. Plastiras SC, Tzelepis GE, Kelekis NL, Vlachoyiannopoulos PG. Catastrophic antiphospholipid syndrome with heart involvement: diagnostic utility of the cardiac MRI. Int J Cardiol. (2007) 116(1):e29–31. doi: 10.1016/j.ijcard.2006.08.083
68. Carrion-Barbera I, Zuccarino F, Escalante FA, Salman-Monte TC. Multiple coronary aneurysms and acute myocardial infarction in a female patient with rhupus: case report and literature review. Clin Rheumatol. (2021) 40(3):1175–84. doi: 10.1007/s10067-020-05313-y
69. Bruce IN, Burns RJ, Gladman DD, Urowitz MB. Single photon emission computed tomography dual isotope myocardial perfusion imaging in women with systemic lupus erythematosus. I. Prevalence and distribution of abnormalities. J Rheumatol. (2000) 27(10):2372–7.11036832
70. Bruce IN, Gladman DD, Ibanez D, Urowitz MB. Single photon emission computed tomography dual isotope myocardial perfusion imaging in women with systemic lupus erythematosus. II. Predictive factors for perfusion abnormalities. J Rheumatol. (2003) 30(2):288–91.12563682
71. Nikpour M, Gladman DD, Ibanez D, Bruce IN, Burns RJ, Urowitz MB. Myocardial perfusion imaging in assessing risk of coronary events in patients with systemic lupus erythematosus. J Rheumatol. (2009) 36(2):288–94. doi: 10.3899/jrheum.080776
72. Rigo F, Gherardi S, Galderisi M, Pratali L, Cortigiani L, Sicari R, et al. The prognostic impact of coronary flow-reserve assessed by Doppler echocardiography in non-ischaemic dilated cardiomyopathy. Eur Heart J. (2006) 27(11):1319–23. doi: 10.1093/eurheartj/ehi795
73. Sharma AK, Farb A, Maniar P, Ajani AE, Castagna M, Virmani R, et al. Spontaneous coronary artery dissection in a patient with systemic lupus erythematosis. Hawaii Med J. (2003) 62(11):248–53.14702766
74. Schattner A, Liang MH. The cardiovascular burden of lupus: a complex challenge. Arch Intern Med. (2003) 163(13):1507–10. doi: 10.1001/archinte.163.13.1507
75. Asanuma Y, Oeser A, Shintani AK, Turner E, Olsen N, Fazio S, et al. Premature coronary-artery atherosclerosis in systemic lupus erythematosus. N Engl J Med. (2003) 349(25):2407–15. doi: 10.1056/NEJMoa035611
76. Hahn BH. Systemic lupus erythematosus and accelerated atherosclerosis. N Engl J Med. (2003) 349(25):2379–80. doi: 10.1056/NEJMp038168
77. Roman MJ, Shanker BA, Davis A, Lockshin MD, Sammaritano L, Simantov R, et al. Prevalence and correlates of accelerated atherosclerosis in systemic lupus erythematosus. N Engl J Med. (2003) 349(25):2399–406. doi: 10.1056/NEJMoa035471
78. Montisci R, Vacca A, Garau P, Colonna P, Ruscazio M, Passiu G, et al. Detection of early impairment of coronary flow reserve in patients with systemic sclerosis. Ann Rheum Dis. (2003) 62(9):890–3. doi: 10.1136/ard.62.9.890
79. Kobayashi H, Yokoe I, Hirano M, Nakamura T, Nakajima Y, Fontaine KR, et al. Cardiac magnetic resonance imaging with pharmacological stress perfusion and delayed enhancement in asymptomatic patients with systemic sclerosis. J Rheumatol. (2009) 36(1):106–12. doi: 10.3899/jrheum.080377
80. Giacomelli R, Di Cesare E, Cipriani P, Ruscitti P, Di Sibio A, Liakouli V, et al. Pharmacological stress, rest perfusion and delayed enhancement cardiac magnetic resonance identifies very early cardiac involvement in systemic sclerosis patients of recent onset. Int J Rheum Dis. (2017) 20(9):1247–60. doi: 10.1111/1756-185X.13107
81. Schwitter J, Wacker CM, Wilke N, Al-Saadi N, Sauer E, Huettle K, et al. Superior diagnostic performance of perfusion-cardiovascular magnetic resonance versus SPECT to detect coronary artery disease: the secondary endpoints of the multicenter multivendor MR-IMPACT II (magnetic resonance imaging for myocardial perfusion assessment in coronary artery disease trial). J Cardiovasc Magn Reson. (2012) 14(1):61. doi: 10.1186/1532-429X-14-61
82. Schwitter J, Wacker CM, Wilke N, Al-Saadi N, Sauer E, Huettle K, et al. MR-IMPACT II: magnetic resonance imaging for myocardial perfusion assessment in coronary artery disease trial: perfusion-cardiac magnetic resonance vs. Single-photon emission computed tomography for the detection of coronary artery disease: a comparative multicentre, multivendor trial. Eur Heart J. (2013) 34(10):775–81. doi: 10.1093/eurheartj/ehs022
83. Greenwood JP, Motwani M, Maredia N, Brown JM, Everett CC, Nixon J, et al. Comparison of cardiovascular magnetic resonance and single-photon emission computed tomography in women with suspected coronary artery disease from the clinical evaluation of magnetic resonance imaging in coronary heart disease (CE-MARC) trial. Circulation. (2014) 129(10):1129–38. doi: 10.1161/CIRCULATIONAHA.112.000071
84. Schwitter J. Myocardial perfusion imaging by cardiac magnetic resonance. J Nucl Cardiol. (2006) 13(6):841–54. doi: 10.1016/j.nuclcard.2006.09.008
85. Schwitter J, Nanz D, Kneifel S, Bertschinger K, Buchi M, Knusel PR, et al. Assessment of myocardial perfusion in coronary artery disease by magnetic resonance: a comparison with positron emission tomography and coronary angiography. Circulation. (2001) 103(18):2230–5. doi: 10.1161/01.CIR.103.18.2230
86. Nagel E, Lehmkuhl HB, Bocksch W, Klein C, Vogel U, Frantz E, et al. Noninvasive diagnosis of ischemia-induced wall motion abnormalities with the use of high-dose dobutamine stress MRI: comparison with dobutamine stress echocardiography. Circulation. (1999) 99(6):763–70. doi: 10.1161/01.CIR.99.6.763
87. Wahl A, Paetsch I, Roethemeyer S, Klein C, Fleck E, Nagel E. High-dose dobutamine-atropine stress cardiovascular MR imaging after coronary revascularization in patients with wall motion abnormalities at rest. Radiology. (2004) 233(1):210–6. doi: 10.1148/radiol.2331030463
88. Hundley WG, Hamilton CA, Thomas MS, Herrington DM, Salido TB, Kitzman DW, et al. Utility of fast cine magnetic resonance imaging and display for the detection of myocardial ischemia in patients not well suited for second harmonic stress echocardiography. Circulation. (1999) 100(16):1697–702. doi: 10.1161/01.CIR.100.16.1697
89. Plein S, Ryf S, Schwitter J, Radjenovic A, Boesiger P, Kozerke S. Dynamic contrast-enhanced myocardial perfusion MRI accelerated with k-t sense. Magn Reson Med. (2007) 58(4):777–85. doi: 10.1002/mrm.21381
90. Gebker R, Jahnke C, Paetsch I, Kelle S, Schnackenburg B, Fleck E, et al. Diagnostic performance of myocardial perfusion MR at 3 T in patients with coronary artery disease. Radiology. (2008) 247(1):57–63. doi: 10.1148/radiol.2471070596
91. Cheng AS, Pegg TJ, Karamitsos TD, Searle N, Jerosch-Herold M, Choudhury RP, et al. Cardiovascular magnetic resonance perfusion imaging at 3-tesla for the detection of coronary artery disease: a comparison with 1.5-tesla. J Am Coll Cardiol. (2007) 49(25):2440–9. doi: 10.1016/j.jacc.2007.03.028
92. Nagel E, Klein C, Paetsch I, Hettwer S, Schnackenburg B, Wegscheider K, et al. Magnetic resonance perfusion measurements for the noninvasive detection of coronary artery disease. Circulation. (2003) 108(4):432–7. doi: 10.1161/01.CIR.0000080915.35024.A9
93. Selvanayagam JB, Jerosch-Herold M, Porto I, Sheridan D, Cheng AS, Petersen SE, et al. Resting myocardial blood flow is impaired in hibernating myocardium: a magnetic resonance study of quantitative perfusion assessment. Circulation. (2005) 112(21):3289–96. doi: 10.1161/CIRCULATIONAHA.105.549170
94. Schwitter J, Wacker CM, van Rossum AC, Lombardi M, Al-Saadi N, Ahlstrom H, et al. MR-IMPACT: comparison of perfusion-cardiac magnetic resonance with single-photon emission computed tomography for the detection of coronary artery disease in a multicentre, multivendor, randomized trial. Eur Heart J. (2008) 29(4):480–9. doi: 10.1093/eurheartj/ehm617
95. Markousis-Mavrogenis G, Bacopoulou F, Mavragani CP, Voulgari PV, Kolovou G, Kitas GD, et al. Coronary microvascular disease: the “meeting point” of cardiology, rheumatology and endocrinology. Eur J Clin Investig. (2021) 52(5):e13737. doi: 10.1111/eci.13737.
96. Pedersen SF, Thrysoe SA, Robich MP, Paaske WP, Ringgaard S, Botker HE, et al. Assessment of intramyocardial hemorrhage by T1-weighted cardiovascular magnetic resonance in reperfused acute myocardial infarction. J Cardiovasc Magn Reson. (2012) 14(1):59. doi: 10.1186/1532-429X-14-59
97. May JE, Moll S. Unexplained arterial thrombosis: approach to diagnosis and treatment. Hematology Am Soc Hematol Educ Program. (2021) 2021(1):76–84. doi: 10.1182/hematology.2021000235
98. Ordovas KG, Baldassarre LA, Bucciarelli-Ducci C, Carr J, Fernandes JL, Ferreira VM, et al. Cardiovascular magnetic resonance in women with cardiovascular disease: position statement from the society for cardiovascular magnetic resonance (SCMR). J Cardiovasc Magn Reson. (2021) 23(1):52. doi: 10.1186/s12968-021-00746-z
99. Antonini L, Le Mauff B, Marcelli C, Aouba A, de Boysson H. Rhupus: a systematic literature review. Autoimmun Rev. (2020) 19(9):102612. doi: 10.1016/j.autrev.2020.102612
100. Roldan CA, Shively BK, Crawford MH. An echocardiographic study of valvular heart disease associated with systemic lupus erythematosus. N Engl J Med. (1996) 335(19):1424–30. doi: 10.1056/NEJM199611073351903
101. Khamashta MA, Cervera R, Asherson RA, Font J, Gil A, Coltart DJ, et al. Association of antibodies against phospholipids with heart valve disease in systemic lupus erythematosus. Lancet. (1990) 335(8705):1541–4. doi: 10.1016/0140-6736(90)91373-I
102. Lopez-Mattei JC, Shah DJ. The role of cardiac magnetic resonance in valvular heart disease. Methodist Debakey Cardiovasc J. (2013) 9(3):142–8. doi: 10.14797/mdcj-9-3-142
103. Khanna D, Gladue H, Channick R, Chung L, Distler O, Furst DE, et al. Recommendations for screening and detection of connective tissue disease-associated pulmonary arterial hypertension. Arthritis Rheum. (2013) 65(12):3194–201. doi: 10.1002/art.38172
104. Kasukawa R. Mixed connective tissue disease. Intern Med. (1999) 38(5):386–93. doi: 10.2169/internalmedicine.38.386
105. Xia YK, Tu SH, Hu YH, Wang Y, Chen Z, Day HT, et al. Pulmonary hypertension in systemic lupus erythematosus: a systematic review and analysis of 642 cases in Chinese population. Rheumatol Int. (2013) 33(5):1211–7. doi: 10.1007/s00296-012-2525-y
106. Kiely DG, Levin D, Hassoun P, Ivy DD, Jone PN, Bwika J, et al. EXPRESS: statement on imaging and pulmonary hypertension from the pulmonary vascular research institute (PVRI). Pulm Circ. (2019) 9(3):2045894019841990. doi: 10.1177/2045894019841990
107. Whitfield AJ, Solanki R, Johns CS, Kiely D, Wild J, Swift AJ. MRI Prediction of precapillary pulmonary hypertension according to the sixth world symposium on pulmonary hypertension. Radiology. (2020) 294(2):482. doi: 10.1148/radiol.2019192078
108. Johns CS, Wild JM, Rajaram S, Tubman E, Capener D, Elliot C, et al. Identifying at-risk patients with combined Pre- and postcapillary pulmonary hypertension using interventricular septal angle at cardiac MRI. Radiology. (2018) 289(1):61–8. doi: 10.1148/radiol.2018180120
109. Schalla S, Nagel E, Lehmkuhl H, Klein C, Bornstedt A, Schnackenburg B, et al. Comparison of magnetic resonance real-time imaging of left ventricular function with conventional magnetic resonance imaging and echocardiography. Am J Cardiol. (2001) 87(1):95–9. doi: 10.1016/S0002-9149(00)01279-0
110. Gonzalez-Lopez L, Gamez-Nava JI, Sanchez L, Rosas E, Suarez-Almazor M, Cardona-Munoz C, et al. Cardiac manifestations in dermato-polymyositis. Clin Exp Rheumatol. (1996) 14(4):373–9.8871835
111. Garcia-Carrasco M, Ramos-Casals M, Rosas J, Pallares L, Calvo-Alen J, Cervera R, et al. Primary sjogren syndrome: clinical and immunologic disease patterns in a cohort of 400 patients. Medicine (Baltimore). (2002) 81(4):270–80. doi: 10.1097/00005792-200207000-00003
112. Al-Dhaher FF, Pope JE, Ouimet JM. Determinants of morbidity and mortality of systemic sclerosis in Canada. Semin Arthritis Rheum. (2010) 39(4):269–77. doi: 10.1016/j.semarthrit.2008.06.002
113. Choi HK, Hernan MA, Seeger JD, Robins JM, Wolfe F. Methotrexate and mortality in patients with rheumatoid arthritis: a prospective study. Lancet. (2002) 359(9313):1173–7. doi: 10.1016/S0140-6736(02)08213-2
114. Westlake SL, Colebatch AN, Baird J, Kiely P, Quinn M, Choy E, et al. The effect of methotrexate on cardiovascular disease in patients with rheumatoid arthritis: a systematic literature review. Rheumatology (Oxford). (2010) 49(2):295–307. doi: 10.1093/rheumatology/kep366
115. Guin A, Chatterjee Adhikari M, Chakraborty S, Sinhamahapatra P, Ghosh A. Effects of disease modifying anti-rheumatic drugs on subclinical atherosclerosis and endothelial dysfunction which has been detected in early rheumatoid arthritis: 1-year follow-up study. Semin Arthritis Rheum. (2013) 43(1):48–54. doi: 10.1016/j.semarthrit.2012.12.027
116. Low AS, Symmons DP, Lunt M, Mercer LK, Gale CP, Watson KD, et al. Relationship between exposure to tumour necrosis factor inhibitor therapy and incidence and severity of myocardial infarction in patients with rheumatoid arthritis. Ann Rheum Dis. (2017) 76(4):654–60. doi: 10.1136/annrheumdis-2016-209784
117. Peters MJ, van Sijl AM, Voskuyl AE, Sattar N, Smulders YM, Nurmohamed MT. The effects of tumor necrosis factor inhibitors on cardiovascular risk in rheumatoid arthritis. Curr Pharm Des. (2012) 18(11):1502–11. doi: 10.2174/138161212799504786
118. Vallianou NG, Geladari E, Panagopoulos F, Kalantzi M. Cardiac MRI in autoimmune diseases: where are we now? Curr Cardiol Rev. (2021) 17(5):e160721190002. doi: 10.2174/1573403X16666210108104236
119. Ponikowski P, Voors AA, Anker SD, Bueno H, Cleland JG, Coats AJ, et al. 2016 ESC guidelines for the diagnosis and treatment of acute and chronic heart failure: the task force for the diagnosis and treatment of acute and chronic heart failure of the European society of cardiology (ESC). developed with the special contribution of the heart failure association (HFA) of the ESC. Eur J Heart Fail. (2016) 18(8):891–975. doi: 10.1002/ejhf.592
120. Sarzi-Puttini P, Atzeni F, Shoenfeld Y, Ferraccioli G. TNF-alpha, rheumatoid arthritis, and heart failure: a rheumatological dilemma. Autoimmun Rev. (2005) 4(3):153–61. doi: 10.1016/j.autrev.2004.09.004
121. Kobayashi Y, Kobayashi H, Giles JT, Hirano M, Nakajima Y, Takei M. Association of tocilizumab treatment with changes in measures of regional left ventricular function in rheumatoid arthritis, as assessed by cardiac magnetic resonance imaging. Int J Rheum Dis. (2016) 19(11):1169–74. doi: 10.1111/1756-185X.12632
122. Kobayashi H, Kobayashi Y, Giles JT, Yoneyama K, Nakajima Y, Takei M. Tocilizumab treatment increases left ventricular ejection fraction and decreases left ventricular mass index in patients with rheumatoid arthritis without cardiac symptoms: assessed using 3.0 tesla cardiac magnetic resonance imaging. J Rheumatol. (2014) 41(10):1916–21. doi: 10.3899/jrheum.131540
123. Panchal L, Divate S, Vaideeswar P, Pandit SP. Cardiovascular involvement in systemic lupus erythematosus: an autopsy study of 27 patients in India. J Postgrad Med. (2006) 52(1):5–10. discussion.16534157
124. Zhang Y, Corona-Villalobos CP, Kiani AN, Eng J, Kamel IR, Zimmerman SL, et al. Myocardial T2 mapping by cardiovascular magnetic resonance reveals subclinical myocardial inflammation in patients with systemic lupus erythematosus. Int J Cardiovasc Imaging. (2015) 31(2):389–97. doi: 10.1007/s10554-014-0560-3
125. Griffith GC, Vural IL. Acute and subacute disseminated lupus erythematosus; a correlation of clinical and postmortem findings in eighteen cases. Circulation. (1951) 3(4):492–500. doi: 10.1161/01.CIR.3.4.492
126. Bulkley BH, Roberts WC. The heart in systemic lupus erythematosus and the changes induced in it by corticosteroid therapy. A study of 36 necropsy patients. Am J Med. (1975) 58(2):243–64. doi: 10.1016/0002-9343(75)90575-6
127. Tariq S, Garg A, Gass A, Aronow WS. Myocarditis due to systemic lupus erythematosus associated with cardiogenic shock. Arch Med Sci. (2018) 14(2):460–2. doi: 10.5114/aoms.2017.68692
128. Hinojar R, Foote L, Sangle S, Marber M, Mayr M, Carr-White G, et al. Native T1 and T2 mapping by CMR in lupus myocarditis: disease recognition and response to treatment. Int J Cardiol. (2016) 222:717–26. doi: 10.1016/j.ijcard.2016.07.182
129. Pieroni M, De Santis M, Zizzo G, Bosello S, Smaldone C, Campioni M, et al. Recognizing and treating myocarditis in recent-onset systemic sclerosis heart disease: potential utility of immunosuppressive therapy in cardiac damage progression. Semin Arthritis Rheum. (2014) 43(4):526–35. doi: 10.1016/j.semarthrit.2013.07.006
130. Vignaux O, Allanore Y, Meune C, Pascal O, Duboc D, Weber S, et al. Evaluation of the effect of nifedipine upon myocardial perfusion and contractility using cardiac magnetic resonance imaging and tissue Doppler echocardiography in systemic sclerosis. Ann Rheum Dis. (2005) 64(9):1268–73. doi: 10.1136/ard.2004.031484
131. Mavrogeni S, Bratis K, Koutsogeorgopoulou L, Karabela G, Savropoulos E, Katsifis G, et al. Myocardial perfusion in peripheral raynaud’s phenomenon. Evaluation using stress cardiovascular magnetic resonance. Int J Cardiol. (2017) 228:444–8. doi: 10.1016/j.ijcard.2016.11.242
132. Hunt SA, Abraham WT, Chin MH, Feldman AM, Francis GS, Ganiats TG, et al. ACC/AHA 2005 guideline update for the diagnosis and management of chronic heart failure in the adult: a report of the American College of Cardiology/American Heart Association Task Force on Practice Guidelines (writing committee to update the 2001 guidelines for the evaluation and management of heart failure): developed in collaboration with the American College of Chest Physicians and the International Society for Heart and Lung Transplantation: endorsed by the heart rhythm society. Circulation. (2005) 112(12):e154–235. doi: 10.1161/CIRCULATIONAHA.105.167586
133. Gaine S, Chin K, Coghlan G, Channick R, Di Scala L, Galie N, et al. Selexipag for the treatment of connective tissue disease-associated pulmonary arterial hypertension. Eur Respir J. (2017) 50(2):1602493. doi: 10.1183/13993003.02493-2016
134. Coghlan JG, Galie N, Barbera JA, Frost AE, Ghofrani HA, Hoeper MM, et al. Initial combination therapy with ambrisentan and tadalafil in connective tissue disease-associated pulmonary arterial hypertension (CTD-PAH): subgroup analysis from the AMBITION trial. Ann Rheum Dis. (2017) 76(7):1219–27. doi: 10.1136/annrheumdis-2016-210236
135. Kowal-Bielecka O, Fransen J, Avouac J, Becker M, Kulak A, Allanore Y, et al. Update of EULAR recommendations for the treatment of systemic sclerosis. Ann Rheum Dis. (2017) 76(8):1327–39. doi: 10.1136/annrheumdis-2016-209909
136. Alabed S, Shahin Y, Garg P, Alandejani F, Johns CS, Lewis RA, et al. Cardiac-MRI predicts clinical worsening and mortality in pulmonary arterial hypertension: a systematic review and meta-analysis. JACC Cardiovasc Imaging. (2021) 14(5):931–42. doi: 10.1016/j.jcmg.2020.08.013
137. Lewis RA, Johns CS, Cogliano M, Capener D, Tubman E, Elliot CA, et al. Identification of cardiac magnetic resonance imaging thresholds for risk stratification in pulmonary arterial hypertension. Am J Respir Crit Care Med. (2020) 201(4):458–68. doi: 10.1164/rccm.201909-1771OC
138. Baggen VJ, Leiner T, Post MC, van Dijk AP, Roos-Hesselink JW, Boersma E, et al. Cardiac magnetic resonance findings predicting mortality in patients with pulmonary arterial hypertension: a systematic review and meta-analysis. Eur Radiol. (2016) 26(11):3771–80. doi: 10.1007/s00330-016-4217-6
139. Addetia K, Bhave NM, Tabit CE, Gomberg-Maitland M, Freed BH, Dill KE, et al. Sample size and cost analysis for pulmonary arterial hypertension drug trials using various imaging modalities to assess right ventricular size and function end points. Circ Cardiovasc Imaging. (2014) 7(1):115–24. doi: 10.1161/CIRCIMAGING.113.000932
140. Alabed S, Garg P, Johns CS, Alandejani F, Shahin Y, Dwivedi K, et al. Cardiac magnetic resonance in pulmonary hypertension-an update. Curr Cardiovasc Imaging Rep. (2020) 13(12):30. doi: 10.1007/s12410-020-09550-2
141. Dong Y, Sun J, Yang D, He J, Cheng W, Wan K, et al. Right ventricular septomarginal trabeculation hypertrophy is associated with disease severity in patients with pulmonary arterial hypertension. Int J Cardiovasc Imaging. (2018) 34(9):1439–49. doi: 10.1007/s10554-018-1347-8
142. Wilkins MR, Paul GA, Strange JW, Tunariu N, Gin-Sing W, Banya WA, et al. Sildenafil versus endothelin receptor antagonist for pulmonary hypertension (SERAPH) study. Am J Respir Crit Care Med. (2005) 171(11):1292–7. doi: 10.1164/rccm.200410-1411OC
143. Mercurio V, Mukherjee M, Tedford RJ, Zamanian RT, Khair RM, Sato T, et al. Improvement in right ventricular strain with ambrisentan and tadalafil upfront therapy in Scleroderma-associated pulmonary arterial hypertension. Am J Respir Crit Care Med. (2018) 197(3):388–91. doi: 10.1164/rccm.201704-0789LE
144. Hassoun PM, Zamanian RT, Damico R, Lechtzin N, Khair R, Kolb TM, et al. Ambrisentan and tadalafil up-front combination therapy in Scleroderma-associated pulmonary arterial hypertension. Am J Respir Crit Care Med. (2015) 192(9):1102–10. doi: 10.1164/rccm.201507-1398OC
145. Mangoni AA, Reid DM, Knights KM. Non-steroidal anti-inflammatory drugs and atherothrombotic risk in older patients: where do we stand? Age Ageing. (2010) 39(5):530–3. doi: 10.1093/ageing/afq099
146. Nuki G. Colchicine: its mechanism of action and efficacy in crystal-induced inflammation. Curr Rheumatol Rep. (2008) 10(3):218–27. doi: 10.1007/s11926-008-0036-3
147. Kirwan JR, Bijlsma JW, Boers M, Shea BJ. Effects of glucocorticoids on radiological progression in rheumatoid arthritis. Cochrane Database Syst Rev. (2007) 2007(1):CD006356. doi: 10.1002/14651858.CD006356
148. Gasparyan AY, Ayvazyan L, Cocco G, Kitas GD. Adverse cardiovascular effects of antirheumatic drugs: implications for clinical practice and research. Curr Pharm Des. (2012) 18(11):1543–55. doi: 10.2174/138161212799504759
149. Shalata W, Abu-Salman A, Steckbeck R, Mathew Jacob B, Massalha I, Yakobson A. Cardiac toxicity associated with immune checkpoint inhibitors: a systematic review. Cancers (Basel). (2021) 13(20):5218. doi: 10.3390/cancers13205218
150. Zhang L, Awadalla M, Mahmood SS, Nohria A, Hassan MZO, Thuny F, et al. Cardiovascular magnetic resonance in immune checkpoint inhibitor-associated myocarditis. Eur Heart J. (2020) 41(18):1733–43. doi: 10.1093/eurheartj/ehaa051
151. Yogasundaram H, Putko BN, Tien J, Paterson DI, Cujec B, Ringrose J, et al. Hydroxychloroquine-induced cardiomyopathy: case report, pathophysiology, diagnosis, and treatment. Can J Cardiol. (2014) 30(12):1706–15. doi: 10.1016/j.cjca.2014.08.016
152. Gallo-Bernal S, Patino-Jaramillo N, Calixto CA, Higuera SA, Forero JF, Lara Fernandes J, et al. Nephrogenic systemic fibrosis in patients with chronic kidney disease after the use of gadolinium-based contrast agents: a review for the cardiovascular imager. Diagnostics (Basel). (2022) 12(8):1816. doi: 10.3390/diagnostics12081816.36010167
153. Prince MR, Lee HG, Lee CH, Youn SW, Lee IH, Yoon W, et al. Safety of gadobutrol in over 23,000 patients: the GARDIAN study, a global multicentre, prospective, non-interventional study. Eur Radiol. (2017) 27(1):286–95. doi: 10.1007/s00330-016-4268-8
154. Ghadimi M, Sapra A. Magnetic resonance imaging contraindications. Treasure Island (FL): StatPearls (2022).
155. Mavrogeni SI, Sfikakis PP, Dimitroulas T, Koutsogeorgopoulou L, Katsifis G, Markousis-Mavrogenis G, et al. Can cardiovascular magnetic resonance prompt early cardiovascular/rheumatic treatment in autoimmune rheumatic diseases? Current practice and future perspectives. Rheumatol Int. (2018) 38(6):949–58. doi: 10.1007/s00296-018-4004-6
156. Baessler B, Luecke C, Lurz J, Klingel K, von Roeder M, de Waha S, et al. Cardiac MRI texture analysis of T1 and T2 maps in patients with infarctlike acute myocarditis. Radiology. (2018) 289(2):357–65. doi: 10.1148/radiol.2018180411
157. Lin ACW, Seale H, Hamilton-Craig C, Morris NR, Strugnell W. Quantification of biventricular strain and assessment of ventriculo-ventricular interaction in pulmonary arterial hypertension using exercise cardiac magnetic resonance imaging and myocardial feature tracking. J Magn Reson Imaging. (2019) 49(5):1427–36. doi: 10.1002/jmri.26517
158. Higgins AY, Arbune A, Soufer A, Ragheb E, Kwan JM, Lamy J, et al. Left ventricular myocardial strain and tissue characterization by cardiac magnetic resonance imaging in immune checkpoint inhibitor associated cardiotoxicity. PLoS One. (2021) 16(2):e0246764. doi: 10.1371/journal.pone.0246764
159. Cerne JW, Pathrose A, Gordon DZ, Sarnari R, Veer M, Blaisdell J, et al. Evaluation of pulmonary hypertension using 4D flow MRI. J Magn Reson Imaging. (2022) 56(1):234–45. doi: 10.1002/jmri.27967
160. Rol N, de Raaf MA, Sun XQ, Kuiper VP, da Silva Goncalves Bos D, Happe C, et al. Nintedanib improves cardiac fibrosis but leaves pulmonary vascular remodelling unaltered in experimental pulmonary hypertension. Cardiovasc Res. 2019;115(2):432–9. doi: 10.1093/cvr/cvy186
Keywords: autoimmune, rheumatic, cardiac magnetic resonance, biomarkers, prognostication, inflammation, microvasculopathy, cardiotoxicity
Citation: Gulhane A and Ordovas K (2023) Cardiac magnetic resonance assessment of cardiac involvement in autoimmune diseases. Front. Cardiovasc. Med. 10:1215907. doi: 10.3389/fcvm.2023.1215907
Received: 2 May 2023; Accepted: 11 September 2023;
Published: 22 September 2023.
Edited by:
Katherine C. Wu, Johns Hopkins University, United StatesReviewed by:
Andreas Rolf, Kerckhoff Klinik, GermanyIsabella Leo, University of Magna Graecia, Italy
© 2023 Gulhane and Ordovas. This is an open-access article distributed under the terms of the Creative Commons Attribution License (CC BY). The use, distribution or reproduction in other forums is permitted, provided the original author(s) and the copyright owner(s) are credited and that the original publication in this journal is cited, in accordance with accepted academic practice. No use, distribution or reproduction is permitted which does not comply with these terms.
*Correspondence: Avanti Gulhane YXZhbnRpZ0B1dy5lZHU=