- 1University College London Medical School, University College London, London, United Kingdom
- 2Centre for Adolescent Rheumatology Versus Arthritis, University College London, London, United Kingdom
- 3Centre for Rheumatology Research, Division of Medicine, University College London, London, United Kingdom
- 4Eastman Dental Hospital, University College London Hospital, London, United Kingdom
- 5Department of Paediatric Endocrinology, University College London Hospital, London, United Kingdom
- 6Institute of Child Health, University College London, London, United Kingdom
Worrying trends of increased cardiovascular disease (CVD) risk in children, adolescents and young people in the Modern Era have channelled research and public health strategies to tackle this growing epidemic. However, there are still controversies related to the dynamic of the impact of sex, age and puberty on this risk and on cardiovascular health outcomes later in life. In this comprehensive review of current literature, we examine the relationship between puberty, sex determinants and various traditional CVD-risk factors, as well as subclinical atherosclerosis in young people in general population. In addition, we evaluate the role of chronic inflammation, sex hormone therapy and health-risk behaviours on augmenting traditional CVD-risk factors and health outcomes, ultimately aiming to determine whether tailored management strategies for this age group are justified.
Introduction
Cardiovascular disease (CVD) is the most common cause of death worldwide (1). Atherosclerosis is one of the earliest signs of CVD. It is characterised by progressive accumulation of cholesterol-laden macrophages in the subendothelial layers of larger arteries which progress to more complex fibrous plaques. Acute plaque rupture or erosion can result in the formation of a thrombus, culminating in the clinical manifestation of myocardial infarction or ischaemic stroke (2). Although more prevalent with increased age, atherosclerotic vascular changes have been documented in young people in multiple post-mortem studies at the end of the past century (3–6). Most notably, asymptomatic microscopic lesions have been described in the coronary arteries of infants in the first 5 years of life, which are thought to precede the more invasive fatty streak lesions associated with atherosclerosis (4, 5). Future studies, built on these findings, established associations between the extent of arterial fatty streaks and fibrotic lesions in young people and traditional CVD-risk factors, such as increasing age and body mass index (BMI), hypertension (HP), dyslipidaemia, aberrant glucose tolerance and smoking, as well as chronic inflammation (3, 7, 8). The presence of CVD-risk factors between the ages of 18–30 can strongly predict the development of subclinical atherosclerosis in later adulthood (9). Interestingly, the sexual dimorphism characterising the CVD prevalence in adulthood is also observed in the prevalence of subclinical atherosclerotic lesions in young people, which were detected in 2% vs. 0% of young men vs. women aged 15–19 years, respectively, and 20% vs. 8% in men vs. women aged 30–34 years, respectively (10). One of the main drivers of this sex difference in prevalence is the higher incidence of traditional CVD risk factors in young males compared to females (6). This is reflected in the sex-disaggregated rate of progression of carotid intima-media thickness (CIMT), which is used as a validated marker of subclinical atherosclerosis, where CIMT progression begins in boys around age 6, and in girls around age 9, and increases gradually with age (11).
Adolescence is a key period of profound physiological changes with significant impact for preservation or deterioration of cardiovascular health, often associated with abnormal cardiovascular health metrics, or significant behavioural changes which impact on diet and other health-related outcomes (12). In addition, sex hormones and genes present on sex chromosomes differentially influence the regulation of the immune system (13), which is also reflected in the sex-bias observed in the predisposition to various autoimmune disorders as well as differential risk for chronic inflammation, which subsequently increases the risk for CVD.
In this review, we aim to assess the impact of sex determinants and puberty in driving metabolic abnormalities leading to atherosclerosis progression in young people and CVD-risk later in life, as well as the impact of chronic inflammation and health-risk on augmenting this risk. Identifying CVD-risk factors earlier in life and tailoring management strategies for prevention of CVD is likely to have significant societal implications. We summarised in Table 1 and Figure 1, the main factors contributing to CVD-risk in young people as well as the main effects of puberty and sex determinants which will be discussed in detail in this review.
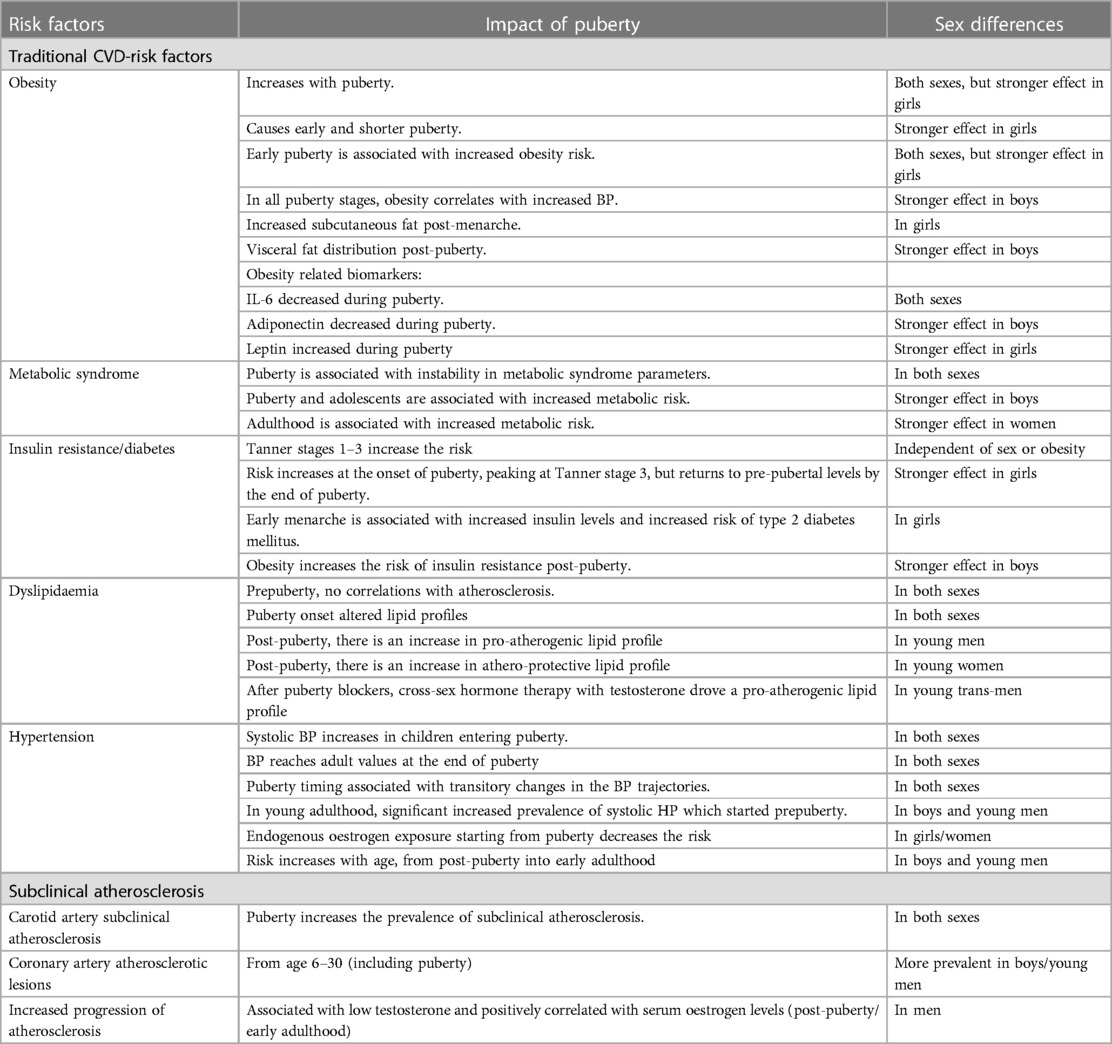
Table 1. Main effects of puberty and sex determinants on traditional CVD-risk factors and subclinical atherosclerosis in young populations.
Sex-biased trends in cardiovascular risk factors in young people in general population
Various traditional CVD-risk factors have been studied in young people and have been included in clinical scores/tools aiming at assessing an individual's risk and tailor public health interventions to minimise the risk for CVD over time. We will focus on the main traditional CVD-risk factors from the perspective of the impact of sex-determinants and puberty on their prevalence, features and trends over time.
Obesity
Between 1975 and 2016, the global prevalence of obesity has nearly tripled (14). As of 2021, WHO estimates an annual mortality of 2.8 million people across all age groups as a direct result of the ongoing obesity epidemic. Targeting excessive weight during childhood and adolescence has been identified as the key intervention in reducing obesity-associated CVD- risk later in life. A higher BMI percentile and central adiposity correlate to a higher risk of obesity and metabolic syndrome in adolescence and adulthood (15, 16). The association between obesity in youth and later life was found to increase with age and is stronger in females (17).
The mechanism by which obesity causes increased CVD- risk is multifactorial. Obesity is associated with chronic mild inflammation, largely mediated by the secretion of inflammatory adipokines by excessive adipose tissue. Higher adiposity throughout puberty was associated with a more atherogenic metabolic profile and greater aortic stiffness (independent of metabolic factors), while reverting to a healthy weight during adolescence prevents this association and results in no difference in arterial stiffness compared to a normal weight control cohort (18). Not all obese individuals are at increased CVD-risk. A high body fat percentage in both adults and children (without additional features of metabolic syndrome—i.e., high blood glucose, insulin resistance, dyslipidaemia) is considered a metabolically healthy obese (MHO) state, and is not associated with increased CVD-risk (19, 20). However, children with prepubertal obesity are at a greater risk of metabolic syndrome, and the pronounced changes to their metabolic parameters during puberty often cause the shift to a metabolically unhealthy status (21, 22).
We summarise below the main effects of obesity on CVD-risk in young populations by sex, including its timing and its bidirectional relationship with puberty.
Obesity in childhood increases the risk of obesity in adulthood and CVD-risk factors in a sex biased way (15). A higher BMI percentile during childhood and adolescence correlates with a high risk of being overweight or obese in adulthood, and the association becomes stronger as age increases. The link observed is stronger in females than in males (17). During all stages of puberty, there is a positive link between trunk fat and systolic and diastolic blood pressure (BP). This association is seen in males only (23).
Obesity is associated with a pro-inflammatory state from an early age. Obesity associated with chronic mild inflammation led to elevated inflammatory markers, including C-reactive protein (CRP), Tumour Necrosis Factor-α (TNF-α), interleukin (IL)-6, IL-18, haptoglobin, macrophage inhibitory factor (MIF) and plasminogen activator inhibitor-1 (24). White adipose tissue has been noted to play an endocrine role, secreting leptin and adiponectin (25, 26). Obesity (particularly visceral fat) in childhood and adolescence increased the risk of metabolic syndrome in adulthood and adolescence, independent of baseline insulin level (16), suggesting that lifestyle factors may contribute more to the development of metabolic syndrome in young people than hereditary factors (27).
Obesity affects the timing of puberty. Obesity causes early and shorter puberty in girls (28–30), which can be explained by the “weight hypothesis”, which suggests that adipokines increase androgen conversion to oestrogen, therefore influencing the timing of puberty. Earlier puberty in obese children is determined by an interplay between molecular factors such as leptin, insulin and oestrogen. There is evidence that serum leptin levels are inversely correlated with age at menarche in girls (31, 32), while there was a close positive correlation between body fat and serum leptin levels in girls throughout puberty (31–35). Children with obesity have lower sex hormone-binding globulin than normal weight children (36), while obese adolescent boys have lower serum testosterone and higher oestradiol than normal weight adolescent males (36, 37). Overweight girls have reduced sleep-associated luteinising hormone (LH) synthesis, the first hormonal change observed at the onset of puberty (38).Puberty timing affects the obesity risk.. Early puberty in girls may be related to obesity due to the effect of oestradiol in increasing the body fat, while early menarche was associated with increased CVD-risk and mortality (39, 40). Early menarche was also related to reduced sex hormone binding globulin and higher oestrogen levels throughout adolescence and into adulthood (41–43), as well as increased risk of metabolic syndrome in adulthood, independent of BMI (44–50). Early puberty is associated with higher accumulation of subcutaneous fat on lower trunk in both males and females (49). The large AVENA study (50) assessed more than 500 children and adolescents and found that the waist circumference and BMI increased in girls as puberty continued, while in boys there were no correlations with the pubertal stage. An increase in total body fat was observed in both females and males throughout puberty, and BMI increased through the 8–18 years interval of observation (51). This was largely driven by the increase in the lean component of BMI, particularly in boys (52). Pubertal stage also affects the adipokine profile (53), and inflammation caused by obesity was somewhat affected by pubertal changes in sex hormones (54).
Obesity related biomarkers are also influenced by puberty and sex hormones. Although no significant difference between prepubertal and pubertal levels of leptin were found independent of body fat content (35), circulating IL-6 levels correlated positively with testosterone and oestradiol levels, and leptin: receptor ratio correlated positively with BMI in both sexes. In addition, IL-6 decreased throughout puberty in both boys and girls but was only found to be correlated to oestradiol and not testosterone. Adiponectin decreases in males from mid-puberty to levels below the ones found in females (53) and levels of circulating androgens were found to decrease plasma adiponectin and may be the cause for increased risk of insulin resistance and atherosclerosis in men (55). Higher serum leptin levels were observed in girls than males pre-, during and post-puberty, even after correcting for increased female adiposity. It is proposed that this may be due to oestradiol stimulating leptin synthesis and testosterone suppressing it (56). At the onset of puberty, leptin increases in girls and decreases in boys, potentially reflecting the increase in fat mass in girls (34), in addition to the potential role of testosterone in suppressing the leptin production (57). By the age of 15, there is an increase in IL-6, IL-8, IL-10 serum concentrations in obese and overweight girls compared to normal weight females even after adjustment for pubertal status, but no significant difference was observed in boys (58).
However, the relationship between early and shorter puberty on CVD-risk later in life is less certain. Data from the Avon Longitudinal Study of Parents and Children (ALSPAC) which recruited children born between April 1, 1991, and December 31, 1992 who were followed-up for 25 years, concluded that earlier puberty was unlikely to have a major impact on pre-clinical CVD-risk in early adulthood, appreciated using a variety of CVD validated outcome measures (59).
There are significant sex differences in adipose tissue distribution around puberty. Over the course of puberty, the prevalence of obesity or excess weight status (measured by BMI) doubles in girls (60). Post menarche, girls have greater subcutaneous fat deposits than pre-menarcheal girls, particularly in the gluteo-femoral region, therefore the sexual dimorphism in fat distribution begins in or is triggered by early puberty (61, 62). Higher oestrogen correlated with gynoid fat distribution in pubertal females (63). Age at menarche negatively correlated to hip and thigh circumference and negatively correlated to waist circumference (61), while early menarche associated with increased adiposity in childhood and increased risk for metabolic syndrome in adulthood (64). In boys, higher serum testosterone was associated with increase in subcutaneous abdominal fat deposition during puberty (63).
Sexual dimorphism in storage of adipose tissue (fat distribution) may underlie the increased CVD-risk in men. Visceral fat and fat deposition around abdomen are favoured in men (central android fat distribution), while subcutaneous fatty deposition in women is largely observed around hips, thighs, and buttocks (gynoid/ gluteal-femoral fat distribution) until menopause, after which there is a shift towards increased deposition of visceral fat (65, 66). Lipoprotein lipase is one of the key enzymes that facilitates the accumulation of adipose tissue. Testosterone inhibits lipoprotein lipase activity in subcutaneous fat in men (67). Lipoprotein activity in women is greater in gluteal subcutaneous fat than in abdominal visceral fat, and higher in abdominal fat in men (68). Distribution of receptors that modulate lipolysis in subcutaneous and intraabdominal fat depots account in part for the sexual dimorphism in body fat deposition. Oestradiol upregulates expression of anti-lipolytic α2-adrenergic receptors in subcutaneous adipocytes but not in abdominal depots (69). The ratio of α2 receptors to lipolytic β1–2 adrenergic receptors is higher in subcutaneous adipose tissue in young women than in men, promoting lower rates of lipolysis in these depots and a reduced lipolytic response to adrenaline and noradrenaline (70).
Increased visceral fat is associated with decreased insulin resistance, increased risk of glucose intolerance and metabolic syndrome (71, 72). Visceral fats have a higher lipolytic rate (65). Higher rate of lipolysis of visceral fat, as observed in males, releases more free fatty acids into the circulation, which in turn causes increased gluconeogenesis and hyperinsulinaemia (73). Subcutaneous fat on the other hand is associated with a lower CVD-risk and do not have the same diabetes-associated risk (74).
Despite good evidence that puberty and sex determinants influence obesity in young people, there is evidence of obesity risks that precede puberty. Children with one obese parent are more than twice as likely to be obese (75), and increased maternal pre-pregnancy weight and gestational weight gain increases the child's adverse CVD-risk. Children of mothers with higher gestation weight gain had higher BMI, waist circumference, fat mass, leptin, CRP and IL6 levels, as well as high density lipoprotein (HDL)-cholesterol and Apolipoprotein (Apo) A1) (76).
In conclusion, obesity in childhood is associated with increased traditional CVD-risk factors overall, as well as increased risk of obesity in adulthood. Obesity triggers early and shorter puberty, while early puberty also predisposes to obesity. There is also evidence of sex dimorphism in fat distribution and obesity-related biomarkers underpinning sex-differencs in CVD-risk from adolescence and young adulthood.
Metabolic syndrome
Metabolic syndrome is defined as a combination of risk factors for CVD, such as diabetes, HP, dyslipidaemia and obesity. The diagnosis of metabolic syndrome in children and adolescents is slightly controversial as only 50% of adolescents diagnosed with metabolic syndrome maintained a stable diagnosis over 3 years (77) and only 30% of obese children and adolescents diagnosed with metabolic syndrome at baseline were found to still maintain the required characteristics at a 60-day follow up (78). This instability may be a result of the dynamic changes observed throughout puberty. Metabolic syndrome in adolescence is higher in males than females by ∼10% (79), but this trend reverses with age as it becomes more prevalent in adult women (80). There is no correlation between any circulating metabolites and measures of atherosclerosis in children, but an inverse correlation between HDL-cholesterol levels and measures of atherosclerosis independent of BMI and BP are present in adulthood (81). However, CVD-risk is nine times higher in children with metabolic syndrome (82). Children with one parent with metabolic syndrome were also at greater risk of obesity and insulin resistance (75). In conclusion, puberty may promote metabolic instability, with a reversal of the sex-bias from male to female from adolescence into adulthood.
Insulin resistance is measured as impaired fasting glucose or impaired glucose tolerance. Hyperglycaemia measured indirectly through levels of glycohemoglobin in post-mortem studies was found to be associated with increases fatty streaks and raised coronary atherosclerotic lesions in young people who died in road traffic accidents from the age of 25, and aortic lesions from the age of 30 (83). In adolescence, obese males have a higher risk for insulin resistance and impaired fasting glucose than obese females (84). Insulin resistance increases at the onset of puberty, peaking at Tanner stage 3, but returns to baseline pre-pubertal levels by the end of puberty (85) and girls are more insulin resistant than boys at all pubertal stages. Serum insulin is highest at Tanner stage 2 in both sexes, independent of body mass and retains a consistent level throughout puberty (86). Between Tanner stages 1–3, there is an increase in fasting glucose, insulin and acute insulin response and an associated reduction in insulin sensitivity independent of sex or obesity status. The reduction in insulin sensitivity was not associated with androgen, oestradiol, visceral fat, or IGF-1 (87) and high levels of insulin in childhood were associated with early menarche (64).
Earlier menarche associated with increased risk of type 2 diabetes mellitus (T2DM) in adulthood independent of BMI at 18 years of age (45). This implies a potential role for sex hormones in the pathophysiology of T2DM (46). Hyperandrogenic profiles in women (e.g., polycystic ovary syndrome) are associated with insulin resistance and glucose intolerance (47, 48), while high oestradiol, high testosterone and low sex hormone binding globulin associated with increased risk of T2DM in women, independent of BMI (46, 88, 89).
In conclusion, puberty onset and early timing of puberty, as well as hyperandrogenic profiles in women increase the insulin resistance and diabetes risk, while early phases of puberty are associated with increased risk in both sexes, independent of obesity.
Dyslipidaemia
Prevalence of dyslipidaemia in children and adolescents across the world is increasing, being four-times more common in children and adolescents with obesity (90). The relationship between lipid abnormalities and atherosclerosis or vascular dysfunction in young people is currently debated (81) as no correlation between lipids and CIMT or pulse wave velocity has been found in children aged 11–12, independent of their BMI or BP. However, associations between these vascular markers and HDL-cholesterol and glucose levels were found in adulthood, suggesting that perhaps these associations arise during/post- puberty. Changes in lipid profiles that occur in puberty however are relevant, as they predispose to increased CVD-risk in adulthood (91). Overall, higher concentrations of triglycerides, low density lipoproteins (LDL) and very low-density lipoproteins (VLDL) cholesterol and ApoB were found in men, with corresponding higher concentrations of HDL-cholesterol, ApoA1, polyunsaturated fatty acids (PUFA) and docosahexaenoic acid (DHA) in women (92, 93). Interestingly, cross-sex hormone therapy in young trans-sexual individuals undergoing gender-reassigning treatment partially reversed the sex bias observed post-puberty in healthy controls (93).
Sex differences in dyslipidaemia
Although no sex difference has been observed in the mean total cholesterol concentration in the Framingham Offspring Study (92), there were sex differences in HDL, LDL and VLDL particles size, which are potentially influenced by sex hormones. Oestrogens have a role in promoting an atheroprotective lipid profile. High serum oestrogen promoted VLDL and LDL clearance as well as increased synthesis of HDL in mouse models through mediation of the hepatic oestrogen receptor α (Erα), the actions of which have been proposed to be modulated by an interaction with Liver X receptor α (LXRα) (94). Contrastingly, cellular study analysing HDL efflux in macrophages in trans-gender women undergoing hormone therapy found reduction in HDL efflux, with a specific decrease in ATP-binding cassette transporter A1 mediated HDL efflux (95).
No sex difference between total HDL concentrations have been reported in the large Framingham Offspring Study either (92). However, young men had a greater concentration of small and intermediate HDL particle subclasses, while women had almost double the concentration of large HDL particles (92). Although women have larger HDL particles than men, this difference is less prominent with increasing age (92). There is some evidence for a stronger inverse correlation between CVD-risk and HDL-cholesterol levels in women than men (96), and this could be attributable to the size of HDL particles (92). This trend seems to be related to the presence of female sex hormones as increased oestradiol serum concentrations correlated with increased HDL-cholesterol in young trans women too (93). ApoA1 is a metabolite for HDL cholesterol which is also affected by sex bias. Women have higher HDL-associated ApoA1 than men while ApoA1 levels were positively corelated with the length of oestradiol therapy and oestradiol serum concentrations in young trans women on cross-sex hormone therapy. However, there was no correlation with testosterone levels in young trans men (93).
Men have a greater total LDL-cholesterol serum concentration than women (92) and smaller LDL particles than women (92, 97–99). This is likely due to a greater concentration of small and intermediate LDL particle subclasses in men, and a greater concentration of large LDL subclasses in women (92). It has been suggested that the sex difference in total LDL particle concentration may be responsible for the sex bias observed in CVD-risk, as the sex difference in LDL concentrations also decreases with age, similar to the trend in CVD risk. There is an increase in small LDL in men before 50 that might underlie the increased CVD risk in middle aged men compared to younger men (92).
Men also have a higher VLDL-cholesterol serum concentration (92), and in particular this affects the larger and intermediate VLDL subclasses as men overall have larger mean VLDL particle sizes (92).
Puberty affects the lipid profiles
Although, no significant difference in lipid profiles were detected between prepubertal girls and boys (93), the onset of puberty altered lipid profiles (100), although these changes normalised post-puberty, raising awareness that changes observed during this puberty should be considered in clinical guidelines for CVD-risk-assessment in adolescents and young people (101).
In conclusion, post-puberty, there is evidence for a trend towards a pro-atherogenic lipid profile in young men or trans-men undergoing cross-sex hormone gender re-assignment therapy with testosterone, while young women have higher concentrations of athero-protective HDL-associated ApoA1.
Hypertension (HP)
It is widely recognised that arterial BP increases with age (102), although has been also gradually increasing in prevalence in younger populations in the recent years Primary HP has been found to be more prevalent in children over age of 6, with associated increased BMI and positive family history (103). The most common causes for secondary HP in children and adolescents are congenital malformations, renal diseases, medications (such as corticosteroids, albuterol and pseudoephedrine), and endocrine causes, while in adolescents, additional factors are related to substance abuse and teen pregnancy (104).
Impact of puberty on HP
Despite less research available overall in younger populations compared to adults, there is evidence that the hormonal and metabolic changes associated with puberty impact BP. The overall prevalence of confirmed HP in children is 2%–5%, with many more being diagnosed with pre-hypertensive states (15.3%) (105). While initial research identified low birth weight as a risk factor for HP in childhood (106, 107), more recent studies found evidence that infants born with appropriate for gestational age or excessive birth weight are both at higher risk for primary HP compared to children with small weight for gestational age (odds ratio = 1.31 and 1.19 respectively) (107). Systolic BP increases in children entering puberty, reaching adult values at the end of puberty, process considered to be significantly influenced by the increased production of sex hormones, growth hormone, insulin-like growth factor-1 and insulin during puberty (108).
Puberty timing also influences the risk of HP. In a large prospective cohort study, which evaluated more than 4,000 children over time, males had significantly increased prevalence of systolic HP compared with females in early adulthood, but this was accrued before puberty, while puberty timing was associated only with small transient differences in systolic BP trajectories post-puberty in both sexes, suggesting that interventions targeting puberty timing are unlikely to influence systolic BP in early adulthood (109–114).
Sex differences in HP are underpinned by various mechanisms
The inhibition of the renin-angiotensin-aldosterone system (RAAS) is a recognised therapeutic option for HP; however, there is evidence that suggests responses to treatment is different in men and in women (115). Endogenous oestrogen is associated with a lower BP in women (116), while there is no consensus from literature data on the effect of exogenous oestrogen, which has been found to decrease (117–119), increase (120–122) or not affect BP measurements (123, 124). These differences may have arisen due to the administration of different oestrogenic compounds at different doses through different routes of administration, and different methods used to measure BP (125). The protective effect of female sex hormones could be explained by the role of oestrogen in promoting vasodilation and exerting cardioprotective effects on the RAAS system. In animal models, oestrogen has been found to upregulate synthesis of angiotensinogen and downregulate synthesis of renin and angiotensin enzyme (ACE) (124, 126, 127), leading protective effects. In rats, oestrogen has been found to reduce calcium efflux in vascular, renal and cardiac cells (128) as well as reduce BP by inhibiting synthesis of angiotensin II (AT II), endothelin 1 (ET1) and catecholamines that all have vasoconstrictive effects (124, 129, 130). One of the mechanisms of oestrogen's effect on BP is mediated through its modulation of expression of ET1, which is a potent vasoconstrictor and its receptors (131). The administration of exogenous 17β-oestradiol to ovariectomised rats decreased the circulating ET1 levels (132, 133) and reduced the expression and activity of endothelin-converting enzyme (133, 134). In vitro studies also find that 17β-oestradiol inhibits ET1 synthesis by increasing nitric oxide synthase (135) +/- decreasing AT II synthesis (136, 137).
Testosterone is pro-hypertensive, through potential modulation of the plasma ET1 levels, resulting in ET1 levels that are 40% higher in men and 90% higher in trans men treated with testosterone therapy than in premenopausal women (138, 139). Testosterone also promotes vasoconstriction and renal sodium reabsorption through the stimulation of AT1 receptor (AT1R) (140–142). In animal studies, it has been noted that males have a higher vascular AT1R:AT2R ratio than females (127, 143, 144).
Sex differences in Hp prevalence in adolescents and young adults
Sex differences in HP in adulthood are well-recognised, with males being more at risk; however, sex differences are particularly pronounced in early adulthood, with one study reporting both sex and ethnic differences in HP among 18- to 29-year-old adults (1.5% in White women vs. 5% White men and 4% and 10% for Black women and men, respectively) (145).
In a large American study of a large representative sample of adolescents followed up to age 24–34, young women were far less likely to be hypertensive compared to men (12% vs. 27%), and there were also sex differences in HP awareness among young people (32% and 25% of hypertensive women and men, respectively were aware of their status) (146).
In conclusion, the prevalence of HP is increased overall from the onset of puberty in both sexes, reaching adult values at the end of puberty, and is significantly influenced by molecular mechanisms subjected to sex hormones and metabolic factors regulation driving the overall increased risk in young males.
Subclinical atherosclerosis in young people in general population
As a consequence of various CVD-risk factors encountered in young population, there is also evidence of presence of subclinical atherosclerosis, usually evaluated on vascular scans or post-mortem studies. Subclinical atherosclerosis is one of the best predictors of CVD later in life. Coronary artery atherosclerotic lesions measured post-mortem in individuals aged 6–30 were more prevalent in men than women (6) All the traditional CVD-risk factors influence the prevalence of subclinical atherosclerosis. Puberty was associated with increased subclinical atherosclerosis in children with a benign obesity phenotype (147). In young people, HP contributes to atherosclerosis by accelerating development of raised lesions rather than fatty streaks. A post-mortem study in people aged 15–34 found that HP, causing increased intimal thickness of small renal arteries, was associated with more extensive raised atherosclerotic lesions in the abdominal aorta and in the right coronary artery (148). A post-mortem histological study of coronary arteries from American young people aged 15–34 found a higher prevalence of advanced atherosclerotic lesions in young men compared to young women (10). Positive correlation between serum oestrogen and progression of carotid atherosclerosis was found in men with intact oestradiol synthesis independent of BMI and other risk factors (149), while low testosterone in men associated with increased progression of atherosclerosis (measured by CIMT) (149).
In conclusion, there is a significant male sex bias in prevalence of subclinical atherosclerosis from early childhood into adulthood.
Effect of chronic inflammation on the cardiovascular risk of young people
Chronic inflammation is one of the known drivers of CVD-risk in patients of all ages. In addition to inflammation, some of the treatments that are used in young people with chronic inflammatory conditions (in particular, corticosteroids or small molecule immunosuppressants, such as Janus Kinase inhibitors and biologic treatments, such as IL6 blockers, etc) have significant metabolic effects (150), which indirectly impact the CVD-risk of a certain individual. Assessment of disease activity in young people with chronic inflammation usually pertain to composite scores aiming to combine objective and subjective assessment of disease manifestations, as well as laboratory parameters which are relevant for systemic inflammation or immune system abnormalities contributing to chronic inflammation (151–153). Despite improvement in quantifying inflammation and improved guidelines to ensure tighter control of disease activity in young people with chronic inflammatory and autoimmune conditions (154–156), there is still evidence of increased CVD-risk in children and young people which is not adequately captured by the CVD-risk assessment tools routinely used for primary care prevention strategies in general population(157, 158).
Long-term inflammation as well as glucocorticoid treatment used in the treatment of many childhood onset diseases, such as juvenile idiopathic arthritis (JIA), juvenile systemic lupus erythematosus (JSLE), juvenile dermatomyositis (JDM), inflammatory bowel disease (IBD), etc, influence the body composition by increasing body fat mass and reducing skeletal muscle mass (159). Obesity is more common in patients with JIA than in the healthy population (160, 161) and is associated with insulin resistance, HP, higher serum triglycerides and early atherosclerosis (162). Obesity and excess weight in JIA population is caused, in part, by glucocorticoid treatment, and functional limitation that causes a less active lifestyle (163) Children with systemic JIA and enthesitis-related arthritis (ERA) had the highest rates of overweight and obesity compared to other JIA subtypes (163). Several studies explored the association between obesity and disease activity or number of active and reported both no association (164, 165), and a positive correlation between obesity and disease activity (particularly on lower limb joints), number of affected joints and higher levels of CRP and ESR compared to the healthy population (166). Children with JIA and a BMI lower than 23 kg/m2 had lower serum leptin than healthy subjects, while children who were overweight or obese had higher prevalence of insulin resistance, lower insulin sensitivity and higher insulin secretion than age matched overweight or obese healthy children. In lean children with systemic JIA, insulin sensitivity was not different to lean age-matched controls (167).
In JDM, a condition associated with muscle and skin inflammation, a unique adipokine profile was found, characterised by higher serum adiponectin and resistin, and lower leptin levels in young women (168). A study of 17 patients with severe JDM, found a very high prevalence of obesity and excess weight, as well as insulin resistance and hypertriglyceridaemia (169). The increased CVD-risk observed in JDM is very likely multifactorial, largely accounted for by chronic inflammation, steroid treatment and poor functional levels leading to increased weight in some patients (170).
No significant difference in the prevalence of obesity in children and adolescents with JSLE compared to healthy controls has been reported (171), although there was a positive correlation between obesity (BMI) in JSLE patients and higher serum levels of TNF-α than in healthy controls. TNF-α is a pro-inflammatory adipokine, associated with a decreased activity of lipoprotein lipase in adipose tissue. It also has a role in the early inflammatory response that contributes to atherosclerosis. Furthermore, it is associated with hyperglycaemia, insulin resistance and dyslipidaemia, mediated by inhibition of insulin receptor autophosphorylation and signal transduction (172, 173). In a small study, it has been found that the prevalence of metabolic syndrome in patients with JSLE was higher than in the healthy population (174).
Children and adolescents with T1DM also had more severe periodontal inflammation (175), which was also associated with increased BMI in young people (176). Periodontal disease is also associated with atherosclerosis risk, potentially from early in life (177). In addition, chronic conditions, such as type II DM (T2DM), RA and SLE which are recognised risk factors for CVD are also associated with periodontal disease, although the majority of studies are derived from adult populations, characterised by a higher prevalence of gum disease (178–180).
Higher prevalence of dyslipidaemia was also observed in young people with T1DM characterised by increased triglycerides and LDL-cholesterol levels, with female adolescents having lower levels of HDL-cholesterol than healthy controls (181). Children with T1DM have higher triglyceride levels, independent of pubertal stage (unlike in healthy controls where TC decreased throughout puberty) which also correlated strongly with ApoB levels (182).
Impact of sex determinants on chronic inflammation and other cardiovascular risk factors relevant to young people's health
There is a recognised female sex bias in autoimmune rheumatic diseases associated with chronic inflammation, although this is less pronounced in diseases with paediatric onset (183). This sex-bias is due to a combination of genetic and epigenetic mechanisms (184), as well as due to impact of sex determinants at the time of puberty on the disease mechanisms and risk overall (185, 186). Many of the autoimmune conditions in young people are rare diseases overall. Therefore, it is not always easy to tease apart sex differences in CVD-risk due to chronic inflammation as many cohort studies are underpowered to detect this. Although, this sex bias is less evident in T1DM (187) and has inverse trends in IBD, where there is evidence of females have a lower risk of Crohn's disease compared with males until puberty, at which point there is a reversal, with females developing higher risk over time (188), the long-term impact of increased CVD-risk in patients with chronic inflammation is less defined by sex differences, suggesting that chronic inflammation is the main driver of this risk (189). However, there is evidence that younger age is an independent factor from increased CVD-risk in patients with RA, which is one of the most prevalent and better studied inflammatory rheumatic conditions (190), suggesting that chronic inflammation rather than increase in prevalence of traditional CVD-risk factor are likely to impact cardiovascular health overall.
No post-pubertal sex differences in serum lipid levels were found between adult men and women with JSLE (93), although in female adolescents with JSLE, dyslipidaemia was more than two times more prevalent than in healthy controls, and these differences were characterised by a lower serum HDL and a higher homocysteine in JSLE cohort (191). Dyslipidaemia in JSLE was also associated with decreased smaller HDL particle subsets than in healthy controls. Active disease accentuated this difference and was further associated with higher VLDL particles when compared to JSLE patients with lower disease activity, and it was related to B- and T-cell lipid rafts, inflammation, and disturbed liver function (192). JSLE patients with active disease also had increase in ApoB:ApoA1 ratio which is a pro-atherosclerotic biomarker (192). In addition, JSLE patients with a high ApoB:ApoA1 ratio (baseline levels correlated positively to SLE disease activity index) had increased cardiometabolic risk conferred by greater number of CD8+ T-lymphocytes and CD8+ T-lymphocyte transcriptomic profile which expressed a higher number of genes associated with interferon signalling and other processes that contribute to atherosclerosis (193).
In conclusion, while chronic inflammatory conditions in adolescents and young people are associated with an increased CVD-risk overall, this is likely driven by both traditional and non-traditional CVD-risk factors, and it is less influenced by puberty or other sex-determinants as the effect of underlying chronic inflammatory disease and treatment seem to override their impact.
Other cardiovascular risk factors relevant for young people
Role of (cross-) sex hormones, hormonal contraception, and hormone replacement therapy (HRT) on cardiovascular risk
The role of sex hormones in the development of atherosclerosis has been a point of contention. It has been suggested that sex-hormones have differential effects in either sex, although there are less published data in young people (194).
Low endogenous testosterone has been linked to increased CVD and mortality in men, suggesting an atheroprotective role in men, albeit through an unclear mechanism (195). It has also been argued however, that causation is uncertain, and low testosterone may be reflective of reduced general health status in men and thus indicative of other factors that may be directly linked to CVD-risk rather than the causative factor itself (196).
There is evidence of increased CVD-risk with oestrogen-combined oral contraceptives in women of reproductive age, in particularly the risk of venous thrombosis (2–7-fold), as well as arterial thrombosis and HP (197, 198). As a consequence, despite benefits in preventing unwanted pregnancies, as well as addressing other medical conditions common in adolescent and young females, such as menstrual irregularities, heavy menstrual bleeding, menstrual discomfort, or required to treat endometriosis, polycystic ovary syndrome, acne and ovarian cysts, combined oral contraceptives are not recommended (WHO-MEC 3) or even contraindicated (WHO-MEC4) in women with cardiac disease, increased thrombotic risk, either venous or arterial, ischaemic heart disease or HP (199).
Emergency contraception (Levonogestrel) has a small effect on blood clotting parameters and increases fibrinogen at 24 and 48 h post-dose, while being associated with a reduction in anti-thrombin III lasting from 2 to 12 h post treatment (200). However, despite these changes in laboratory parameters, there was no evidence of an increased risk of thrombosis in users of emergency contraception (201).
Large-scale studies thus far have been unable to establish a benefit of HRT for cardioprotective purposes. There is however some evidence for a benefit if treatment started immediately post-menopause, leading to the “timing hypothesis”, which suggests that the type of oestrogen used and the time between the start of menopause and initiation of HRT can lead to differential cardiovascular outcomes (202). However, no data are available in younger populations as this as sex hormone therapy is very rarely warranted. The impact of cross-sex hormone therapy in young trans-sex populations is insufficiently studied (203) as there are unmet needs to involve gender-diverse people in research in medicine overall (204).
In conclusion, while associated with a small CVD-risk overall, sex hormone therapies containing oestrogen have to be used cautiously in young female populations at risk, especially as alternatives are available (progesterone only contraceptive preparations or intrauterine devices and barrier protection).
Sex differences in health-risk behaviours contributing to cardiovascular risk in young people
According to the WHO, 10% of mortality due to CVD can be attributed to cigarette smoking (205) Smoking was associated with more extensive fatty streaks and raised atherosclerotic lesions in the abdominal aorta of young people in post-mortem study (206). Inhalation of cigarette smoke, both passively and actively, increases CVD-risk, as 10% of smoking-related mortality can be attributed to passive cigarette smoking (207). Smoking increases the risk of myocardial infarction and stroke (208) and exposure to cigarette smoke affects the regulation of mechanisms responsible for the formation of intravascular thrombi, inducing a hypercoagulable state and contributing to increased risked of acute thrombotic events (208). There is a higher mortality rate in female smokers than male smokers, with female smokers being 25% more likely to develop coronary heart disease (209).
A study conducted on healthy young men and women found that CVD-risk factors occur earlier in young female smokers than male cigarette smokers (210). In women only, exposure to cigarette smoke increased monocyte and lymphocyte counts, whereas in men, neutrophils and eosinophils were significantly increased. Global DNA methylation was reduced more in women than men who smoked, while smoking increased the number of platelets in women, and decreased the number of platelets in men. Smoking also seemed to affect endothelial function in women more than in men by causing a significant increase in asymmetric dimethyl arginine (ADMA), an endogenous inhibitor of nitric oxide synthesis, and L-arginine, a precursor of nitric oxide synthesis in women only. These are both surrogate measures of endothelial dysfunction. The trans-sulphuration pathway involves interconversion of cysteine and homocysteine. The forward reaction in this pathway produces homocysteine, a recognised marker of CVD-risk (211). In the non-smoking control group, homocysteine was found to be lower in females than in males, while exposure to cigarette smoke increased homocysteine levels in women only. Smoking also eradicated the sexual dimorphism in TNF-α release from human monocyte-derived macrophages (hMDMs) as even if in non-smokers, there is a higher basal TNF-α release from hMDMs in men than in women, the smoking increased TNF-α release in women only. Early onset of puberty associated with increased risk of smoking throughout adolescence (212).
Cigarette smoking intensity (acute smoking measured as number of cigarettes smoked per day) has been associated with increased serum concentration of biomarkers of CVD, particularly, markers of systemic inflammation, with hsCRP, being the most sensitive (213).
A large meta-analysis found a dose-dependent relationship between the number of cigarettes smoked per day, with pooled relative risk for coronary heart disease in men of 1.48 for smoking one cigarette per day and 2.04 for 20 cigarettes, while in women, the risk was 1.57 and 2.84, respectively (214).
Long-term exposure (chronic cigarette smoking), commonly assessed using smoking duration (years), or cumulative exposure (pack-years) was also associated with measures of either inflammation or subclinical atherosclerosis (215), which is relevant to adolescents, especially as cigarette smoking initiation rates during early adolescence (11–15 years) showed a marked increase after 1990 especially in West Europe, while smoking initiation during late adolescence (16–20 years) declined, with the exception of South Europe (216).
Recent research has been directed towards understanding the cardiovascular health effects of electronic vaping cigarettes (EVC) and heat-not-burn cigarettes, which are popular alternatives to traditional combustion cigarettes. An analysis of 7 systematic reviews found that acute EVC use was associated with several toxic effects, including detrimental impact on BP management, tachycardia, worsened arterial stiffness, as well as increased prevalence of atrial fibrillation and myocardial infarction, even if the causal link is still debated (217).
Adolescence is also associated with increased use of illicit substances (218), with potential devastating impact on cardiovascular health (219). Many studies investigated sex and gender differences as well as contextual factors and relationships associated with substance use and academic and health-related outcomes (220–224), highlighting the complexity of this phenomenon which cannot be simply disaggregated by sex. Significant atherosclerosis has been particularly linked to cocaine use compared to opioid use and other poor-health related factors (225). Illicit substance use is one of the main drivers of CVD in young people overall (226).
Is there any need for tailored CVD-risk strategies in young people?
There is ample evidence in the literature that puberty and adolescence are very dynamic periods in the life of young people associated with various metabolic changes and CVD-risk trends, some of which are reversable. Correct phenotyping of an individual, as well as assessment of risk over time are important strategies for managing the potential health consequences of having increased CVD-risk or subclinical atherosclerosis later in life. We would argue that certain groups of young people, especially in the context of genetic predisposition, concomitant chronic inflammatory conditions or at the time of intense vulnerability driven by physiological, socio-economic or psychological factors would benefit from tailored management strategies, irrespective of being aimed at addressing chronic inflammation, improving physical exercise or diet, or tackling health-risk associated behaviours. In addition, early identification of increased CVD-risk through improved detection strategies as well as dynamic assessment of this risk over time are likely to lead to improved outcomes. Although, there is evidence of a male bias in increased CVD-risk in young people, especially post-puberty, future research is needed to establish whether this can be addressed by sex-biased therapeutic and management strategies from early life.
Conclusions
There is an unmet need for better CVD-risk assessment and management strategies in young people overall, as although the traditional risk factors are clearly linked to subclinical atherosclerosis, there are other contributing determinants, related to pubertal changes, chronic inflammation and treatment addressing inflammation, as well as health-risk behaviours that are particularly relevant in this population. There are well documented sex differences in CVD-risk and subclinical atherosclerosis which maintain the male-biased predominance observed in the older populations; however, in the context of chronic inflammatory conditions, the upregulation of the pro-inflammatory pathways or the use of various treatments associated with metabolic effects with role in CVD-risk modulation seem to override the sex and puberty driven differences observed in the general population. Further research is needed to capture the long-term outcomes of young people with chronic inflammatory diseases and contrast them with the impact of traditional CVD-risk factors in the general population, disaggregated by sex, to give us the possibility to properly investigate whether sex and gender-tailored CVD-risk management strategies are warranted.
Author contributions
Conceptualization, CC and AA; methodology, CC and AA; investigation, AA and CC; data curation, AA and CC; writing—original draft preparation, AA and CC; writing—review and editing, all authors.; visualization, JP and CC; supervision, CC; project administration, CC and AA; funding acquisition, GR, EJ and CC. All authors contributed to the article and approved the submitted version.
Funding
This work was performed within the Centre for Adolescent Rheumatology Versus Arthritis at UCL, UCLH and GOSH supported by grants from Versus Arthritis (21593, 22856 and 20164) and NIHR UCLH Biomedical Research Centre grant BRC773/III/CC/101350. The views expressed are those of the authors and not necessarily those of the NHS, the NIHR or the Department of Health.
Conflict of interest
The authors declare that the research was conducted in the absence of any commercial or financial relationships that could be construed as a potential conflict of interest.
Publisher's note
All claims expressed in this article are solely those of the authors and do not necessarily represent those of their affiliated organizations, or those of the publisher, the editors and the reviewers. Any product that may be evaluated in this article, or claim that may be made by its manufacturer, is not guaranteed or endorsed by the publisher.
References
1. WHO. Fact Sheet: Noncommunicable diseases. (cited 2023 Jan 23). Available at: https://www.who.int/en/news-room/fact-sheets/detail/noncommunicable-diseases (2015).
3. Berenson GS, Srinivasan SR, Bao W, Newman WP, Tracy RE, Wattigney WA. Association between multiple cardiovascular risk factors and atherosclerosis in children and young adults. The Bogalusa Heart Study. N Engl J Med. (1998) 338(23):1650–6. doi: 10.1056/NEJM199806043382302
4. Stary HC, Chandler AB, Glagov S, Guyton JR, Insull W, Rosenfeld ME, et al. A definition of initial, fatty streak, and intermediate lesions of atherosclerosis. A report from the committee on vascular lesions of the council on arteriosclerosis, American heart association. Circulation. (1994) 89(5):2462–78. doi: 10.1161/01.CIR.89.5.2462
5. Stary HC. Macrophages, macrophage foam cells, and eccentric intimal thickening in the coronary arteries of young children. Atherosclerosis. (1987) 64(2–3):91–108. doi: 10.1016/0021-9150(87)90234-6
6. Berenson GS, Wattigney WA, Tracy RE, Newman WP, Srinivasan SR, Webber LS, et al. Atherosclerosis of the aorta and coronary arteries and cardiovascular risk factors in persons aged 6 to 30 years and studied at necropsy (the bogalusa heart study). Am J Cardiol. (1992) 70(9):851–8. doi: 10.1016/0002-9149(92)90726-F
7. McGill J, McMahan CA, Herderick EE, Malcom GT, Tracy RE, Jack P. Origin of atherosclerosis in childhood and adolescence. Am J Clin Nutr. (2000) 72(5 Suppl):1307S–15S. doi: 10.1093/ajcn/72.5.1307s
8. Del Rincón I, Polak JF, O’Leary DH, Battafarano DF, Erikson JM, Restrepo JF, et al. Systemic inflammation and cardiovascular risk factors predict rapid progression of atherosclerosis in rheumatoid arthritis. Ann Rheum Dis. (2015) 74(6):1118–23. doi: 10.1136/annrheumdis-2013-205058
9. Gidding SS, Rana JS, Prendergast C, McGill H, Carr JJ, Liu K, et al. Pathobiological determinants of atherosclerosis in youth (PDAY) risk score in young adults predicts coronary artery and abdominal aorta calcium in middle age. Circulation. (2016) 133(2):139–46. doi: 10.1161/CIRCULATIONAHA.115.018042
10. McGill HC, McMahan CA, Zieske AW, Tracy RE, Malcom GT, Herderick EE, et al. Association of coronary heart disease risk factors with microscopic qualities of coronary atherosclerosis in youth. Circulation. (2000) 102(4):374–9. doi: 10.1161/01.CIR.102.4.374
11. Juonala M, Magnussen CG, Venn A, Dwyer T, Burns TL, Davis PH, et al. Influence of age on associations between childhood risk factors and carotid intima-media thickness in adulthood: the cardiovascular risk in young Finns study, the childhood determinants of adult health study, the bogalusa heart study, and the muscatine study for the international childhood cardiovascular cohort (i3C) consortium. Circulation. (2010) 122(24):2514–20. doi: 10.1161/CIRCULATIONAHA.110.966465
12. Henriksson P, Henriksson H, Labayen I, Huybrechts I, Gracia-Marco L, Ortega FB, et al. Correlates of ideal cardiovascular health in European adolescents: the HELENA study. Nutr Metab Cardiovasc Dis. (2018) 28(2):187–94. doi: 10.1016/j.numecd.2017.10.018
13. Klein SL, Flanagan KL. Sex differences in immune responses. Nat Rev Immunol. (2016) 16(10):626–38. doi: 10.1038/nri.2016.90
15. Han JC, Lawlor DA, Kimm SY. Childhood obesity. Lancet. (2010) 375(9727):1737–48. doi: 10.1016/S0140-6736(10)60171-7
16. Srinivasan SR, Myers L, Berenson GS. Predictability of childhood adiposity and insulin for developing insulin resistance syndrome (syndrome X) in young adulthood: the Bogalusa Heart Study. Diabetes. (2002) 51(1):204–9. doi: 10.2337/diabetes.51.1.204
17. Guo SS, Wu W, Chumlea WC, Roche AF. Predicting overweight and obesity in adulthood from body mass index values in childhood and adolescence. Am J Clin Nutr. (2002) 76(3):653–8. doi: 10.1093/ajcn/76.3.653
18. Dangardt F, Charakida M, Georgiopoulos G, Chiesa ST, Rapala A, Wade KH, et al. Association between fat mass through adolescence and arterial stiffness: a population-based study from the avon longitudinal study of parents and children. Lancet Child Adolesc Health. (2019) 3(7):474–81. doi: 10.1016/S2352-4642(19)30105-1
19. Prince RL, Kuk JL, Ambler KA, Dhaliwal J, Ball GDC. Predictors of metabolically healthy obesity in children. Diabetes Care. (2014) 37(5):1462–8. doi: 10.2337/dc13-1697
20. Reinehr T, Wolters B, Knop C, Lass N, Holl RW. Strong effect of pubertal Status on metabolic health in obese children: a longitudinal study. J Clin Endocrinol Metab. (2015) 100(1):301–8. doi: 10.1210/jc.2014-2674
21. Tobisch B, Blatniczky L, Barkai L. Cardiometabolic risk factors and insulin resistance in obese children and adolescents: relation to puberty. Pediatr Obes. (2015) 10(1):37–44. doi: 10.1111/j.2047-6310.2013.00202.x
22. Pilia S, Casini MR, Foschini ML, Minerba L, Musiu MC, Marras V, et al. The effect of puberty on insulin resistance in obese children. J Endocrinol Invest. (2009) 32(5):401–5. doi: 10.1007/BF03346475
23. He Q, Horlick M, Fedun B, Wang J, Pierson RN, Heshka S, et al. Trunk fat and blood pressure in children through puberty. Circulation. (2002) 105(9):1093–8. doi: 10.1161/hc0902.104706
24. Hauner H. Secretory factors from human adipose tissue and their functional role. Proc Nutr Soc. (2005) 64(2):163–9. doi: 10.1079/PNS2005428
25. Trayhurn P, Wood IS. Adipokines: inflammation and the pleiotropic role of white adipose tissue (2004).
26. Trayhurn P, Beattie JH. Physiological role of adipose tissue: white adipose tissue as an endocrine and secretory organ. Proc Nutr Soc. (2001) 60:329–39. doi: 10.1079/PNS200194
27. Despres JP. Abdominal obesity as important component of insulin-resistance syndrome. Nutrition. (1993) 9(5):452–9.8286886
28. Aksglaede L, Juul A, Olsen LW, Sørensen TIA. Age at puberty and the emerging obesity epidemic. PLoS One. (2009) 4(12):e8450. doi: 10.1371/journal.pone.0008450
29. De Leonibus C, Marcovecchio ML, Chiavaroli V, De Giorgis T, Chiarelli F, Mohn A. Timing of puberty and physical growth in obese children: a longitudinal study in boys and girls. Pediatr Obes. (2014) 9(4):292–9. doi: 10.1111/j.2047-6310.2013.00176.x
30. Rosenfield RL, Lipton RB, Drum ML. Thelarche, pubarche, and menarche attainment in children with normal and elevated body mass index. Pediatrics. (2009) 123(1):84–8. doi: 10.1542/peds.2008-0146
31. Bandini LG, Must A, Naumova EN, Anderson SE, Caprio S, Spadano-Gasbarro JL, et al. Change in leptin, body composition and other hormones around menarche—a visual representation. Acta Paediatr. (2008) 97(10):1454–9. doi: 10.1111/j.1651-2227.2008.00948.x
32. Matkovic V, Ilich JZ, Skugor M, Badenhop NE, Goel P, Clairmont A, et al. Leptin is inversely related to age at menarche in human females. J Clin Endocrinol Metab. (1997) 82(10):3239–45.9329346
33. Ellis KJ, Nicolson M. Leptin levels and body fatness in children: effects of gender, ethnicity, and sexual development. Pediatr Res. (1997) 42(4):484–8. doi: 10.1203/00006450-199710000-00010
34. Ahmed ML, Ong KKL, Morrell DJ, Cox L, Drayer N, Perry L, et al. Longitudinal study of leptin concentrations during puberty: sex differences and relationship to changes in body composition. J Clin Endocrinol Metab. (1999) 84(3):899–905.10084568
35. Arslanian S, Suprasongsin C, Kalhan SC, Drash AL, Brna R, Janosky JE. Plasma leptin in children: relationship to puberty, gender, body composition, insulin sensitivity, and energy expenditure. Metab Clin Exp. (1998) 47(3):309–12. doi: 10.1016/S0026-0495(98)90262-1
36. Nokoff N, Thurston J, Hilkin A, Pyle L, Zeitler PS, Nadeau KJ, et al. Sex differences in effects of obesity on reproductive hormones and glucose metabolism in early puberty. J Clin Endocrinol Metab. (2019) 104:4390–7. doi: 10.1210/jc.2018-02747
37. Moriarty-Kelsey M, Harwood JEF, Travers SH, Zeitler PS, Nadeau KJ. Testosterone, obesity and insulin resistance in young males: evidence for an association between gonadal dysfunction and insulin resistance during puberty. J Pediatr Endocrinol Metab. (2010) 23(12):1281–7. doi: 10.1515/jpem.2010.202
38. Rosenfield RL, Bordini B. Evidence that obesity and androgens have independent and opposing effects on gonadotropin production from puberty to maturity. Brain Res. (2010) 1364:186–97. doi: 10.1016/j.brainres.2010.08.088
39. Lakshman R, Forouhi NG, Sharp SJ, Luben R, Bingham SA, Khaw KT, et al. Early age at menarche associated with cardiovascular disease and mortality. J Clin Endocrinol Metab. (2009) 94(12):4953–60. doi: 10.1210/jc.2009-1789
40. Jacobsen BK, Oda K, Knutsen SF, Fraser GE. Age at menarche, total mortality and mortality from ischaemic heart disease and stroke: the adventist health study, 1976-88. Int J Epidemiol. (2009) 38(1):245–52. doi: 10.1093/ije/dyn251
41. Vihko R, Apter D. Endocrine characteristics of adolescent menstrual cycles: impact of early menarche. J Steroid Biochem. (1984) 20(1):231–6. doi: 10.1016/0022-4731(84)90209-7
42. Apter D, Reinilä M, Vihko R. Some endocrine characteristics of early menarche, a risk factor for breast cancer, are preserved into adulthood. Int J Cancer. (1989) 44(5):783–7. doi: 10.1002/ijc.2910440506
43. Apter D, Bolton NJ, Hammond GL, Vihko R. Serum sex hormone-binding globulin during puberty in girls and in different types of adolescent menstrual cycles. Acta Endocrinol (Copenh). (1984) 107(3):413–9.6391059
44. Kim Y, Je Y. Early menarche and risk of metabolic syndrome: a systematic review and meta-analysis. J Womens Health. (2019) 28(1):77–86. doi: 10.1089/jwh.2018.6998
45. He C, Zhang C, Hunter DJ, Hankinson SE, Buck Louis GM, Hediger ML, et al. Age at menarche and risk of type 2 diabetes: results from 2 large prospective cohort studies. Am J Epidemiol. (2009) 171(3):334–44. doi: 10.1093/aje/kwp372
46. Ding EL, Song Y, Malik VS, Liu S. Sex differences of endogenous sex hormones and risk of type 2 diabetes: a systematic review and meta-analysis. JAMA. (2006) 295(11):1288–99. doi: 10.1001/jama.295.11.1288
47. Toprak S, Yönem A, Çakir B, Güler S, Azal Ö, Özata M, et al. Insulin resistance in nonobese patients with polycystic ovary syndrome. Horm Res. (2001) 55(2):65–70.11509861
48. Oh JY, Barrett-Connor E, Wedick NM, Wingard DL. Endogenous sex hormones and the development of type 2 diabetes in older men and women: the rancho bernardo study. Diabetes Care. (2002) 25(1):55–60. doi: 10.2337/diacare.25.1.55
49. Kozieł S, Malina RM. Variation in relative fat distribution associated with maturational timing: the wrocław growth study. Ann Hum Biol. (2009) 32(6):691–701. http://dx.doi.org/101080/03014460500268531
50. Ruiz JR, Ortega FB, Tresaco B, Wärnberg J, Mesa JL, González-Gross M, et al. Serum lipids, body mass index and waist circumference during pubertal development in spanish adolescents: the AVENA study. Horm Metab Res. (2006) 38(12):832–7. doi: 10.1055/s-2006-956503
51. Asghari G, Mirmiran P, Rezaeemanesh A, Mahdavi M, Azizi F, Hadaegh F. Changes in ideal cardiovascular health among Iranian adolescents: 2007-2008 to 2015-2017. BMC Pediatr. (2022) 22(1):450. doi: 10.1186/s12887-022-03504-x
52. Siervogel RM, Maynard LM, Wisemandle WA, Roche AF, Guo SS, Chumlea WC, et al. Annual changes in total body fat and fat-free mass in children from 8 to 18 years in relation to changes in body mass Index: the fels longitudinal study. Ann N Y Acad Sci. (2000) 904(1):420–3. doi: 10.1111/j.1749-6632.2000.tb06494.x
53. Martos-Moreno GÁ, Barrios V, Argente J. Normative data for adiponectin, resistin, interleukin 6, and leptin/receptor ratio in a healthy spanish pediatric population: relationship with sex steroids. Eur J Endocrinol. (2006) 155(3):429–34. doi: 10.1530/eje.1.02227
54. Tam CS, Garnett SP, Cowell CT, Heilbronn LK, Lee JW, Wong M, et al. IL-6, IL-8 and IL-10 levels in healthy weight and overweight children. Horm Res Paediatr. (2010) 73(2):128–34. doi: 10.1159/000277632
55. Nishizawa H, Shimomura L, Kishida K, Maeda N, Kuriyama H, Nagaretani H, et al. Androgens decrease plasma adiponectin, an insulin-sensitizing adipocyte-derived protein. Diabetes. (2002) 51(9):2734–41. doi: 10.2337/diabetes.51.9.2734
56. Demerath EW, Towne B, Wisemandle W, Blangero J, Chumlea WC, Siervogel RM. Serum leptin concentration, body composition, and gonadal hormones during puberty. Int J Obes Relat Metab Disord. (1999) 23(7):678–85. doi: 10.1038/sj.ijo.0800902
57. Jockenhövel F, Blum WF, Vogel E, Englaro P, Müller-Wieland D, Reinwein D, et al. Testosterone substitution normalizes elevated serum leptin levels in hypogonadal men. J Clin Endocrinol Metab. (1997) 82(8):2510–3. doi: 10.1210/jcem.82.8.4174
58. Saputri NKD, Sidiartha IGLS, Utama IMGDL Correlation between interleukin-6 serum and hepcidin serum in obese children. Am J Pediatr. (2020) 6(1):27–30. doi: 10.11648/j.ajp.20200601.14
59. Maher GM, Ryan L, McCarthy FP, Hughes A, Park C, Fraser A, et al. Puberty timing and markers of cardiovascular structure and function at 25 years: a prospective cohort study. BMC Med. (2021) 19(1):78. doi: 10.1186/s12916-021-01949-y
60. Kimm SYS, Barton BA, Obarzanek E, McMahon RP, Kronsberg SS, Waclawiw MA, et al. Obesity development during adolescence in a biracial cohort: the NHLBI growth and health study. Pediatrics. (2002 Nov 1) 110(5):e54–e54. doi: 10.1542/peds.110.5.e54
61. Lassek WD, Gaulin SJC. Brief communication: menarche is related to fat distribution. Am J Phys Anthropol. (2007) 133(4):1147–51. doi: 10.1002/ajpa.20644
62. Bhadra M, Mukhopadhyay A, Chakraborty R, Bose K, Koziel S, Ulijaszek S. Relative fat distribution in relation to menarcheal status among bengalee hindu girls of West Bengal, India. J Nat Sci Biol Med. (2013) 4(2):369–73. doi: 10.4103/0976-9668.116997
63. Roemmich JN, Rogol AD. Hormonal changes during puberty and their relationship to fat distribution. J Hum Biol. (1999) 11:209–24. doi: 10.1002/(SICI)1520-6300(1999)11:2%3C209::AID-AJHB9%3E3.0.CO;2-G
64. Frontini MG, Srinivasan SR, Berenson GS. Longitudinal changes in risk variables underlying metabolic syndrome X from childhood to young adulthood in female subjects with a history of early menarche: the bogalusa heart study. Int J Obes Relat Metab Disord. (2003) 27(11):1398–404. doi: 10.1038/sj.ijo.0802422
65. Palmer BF, Clegg DJ. The sexual dimorphism of obesity. Mol Cell Endocrinol. (2015) 402:113–9. doi: 10.1016/j.mce.2014.11.029
66. Lovejoy JC, Champagne CM, De Jonge L, Xie H, Smith SR. Increased visceral fat and decreased energy expenditure during the menopausal transition. Int J Obes. (2008) 32:949–58. doi: 10.1038/ijo.2008.25
67. Ramirez ME, McMurry MP, Wiebke GA, Felten KJ, Ren K, Meikle AW, et al. Evidence for sex steroid inhibition of lipoprotein lipase in men: comparison of abdominal and femoral adipose tissue. Metab Clin Exp. (1997) 46(2):179–85. doi: 10.1016/S0026-0495(97)90299-7
68. Arner P, Lithell H, Wahrenberg H, Bronnegard M. Expression of lipoprotein lipase in different human subcutaneous adipose tissue regions. J Lipid Res. (1991) 32(3):423–9. doi: 10.1016/S0022-2275(20)42065-6
69. Pedersen SB, Kristensen K, Hermann PA, Katzenellenbogen JA, Richelsen B. Estrogen controls lipolysis by up-regulating α2A-adrenergic receptors directly in human adipose tissue through the estrogen receptor α. Implications for the female fat distribution. J Clin Endocrinol Metab. (2004) 89(4):1869–78. doi: 10.1210/jc.2003-031327
70. Gavin KM, Cooper EE, Raymer DK, Hickner RC. Estradiol effects on subcutaneous adipose tissue lipolysis in premenopausal women are adipose tissue depot specific and treatment dependent. Am J Physiol Endocrinol Metab. (2013) 304(11):1167–74. doi: 10.1152/ajpendo.00023.2013
71. Caprio S, Perry R, Kursawe R. Adolescent obesity and insulin resistance: roles of ectopic fat accumulation and adipose inflammation. Gastroenterology. (2017) 152(7):P1638–46. doi: 10.1053/j.gastro.2016.12.051
72. Raji A, Seely EW, Arky RA, Simonson DC. Body fat distribution and insulin resistance in healthy Asian Indians and caucasians. J Clin Endocrinol Metab. (2001) 86(11):5366–71. doi: 10.1210/jcem.86.11.7992
73. Shulman GI. Ectopic fat in insulin resistance, dyslipidemia, and cardiometabolic disease. N Engl J Med. (2014) 12(18):1131–72. doi: 10.1056/NEJMra1011035
74. Van Pelt RE, Evans EM, Schechtman KB, Ehsani AA, Kohrt WM. Contributions of total and regional fat mass to risk for cardiovascular disease in older women. Am J Physiol - Endocrinol Metab. (2002) 282(5):45–5. doi: 10.1152/ajpendo004672001
75. Meigs JB, Tracy RP. Invited commentary: insulin resistance syndrome? Syndrome X? Multiple metabolic syndrome? A syndrome at all? Factor analysis reveals patterns in the fabric of correlated metabolic risk factors. Am J Epidemiol. (2000) 152(10):908–12. doi: 10.1093/aje/152.10.908
76. Fraser A, Tilling K, Macdonald-Wallis C, Sattar N, Brion MJ, Benfield L, et al. Epidemiology and Prevention Association of Maternal Weight Gain in Pregnancy With Offspring Obesity and Metabolic and Vascular Traits in Childhood (2010).
77. Goodman E, Daniels SR, Meigs JB, Dolan LM. Instability in the diagnosis of metabolic syndrome in adolescents. Circulation. (2007) 115(17):2316–22. doi: 10.1161/CIRCULATIONAHA.106.669994
78. Gustafson JK, Yanoff LB, Easter BD, Brady SM, Keil MF, Roberts MD, et al. The stability of metabolic syndrome in children and adolescents. J Clin Endocrinol Metab. (2009) 94(12):4828–34. doi: 10.1210/jc.2008-2665
79. Miller JM, Kaylor MB, Johannsson M, Bay C, Churilla JR. Prevalence of metabolic syndrome and individual criterion in US adolescents: 2001–2010 national health and nutrition examination survey. Metab Syndr Relat Disord. (2014) 12(10):527–32. https://home.liebertpub.com/met. doi: 10.1089/met.2014.0055
80. Rochlani Y, Pothineni NV, Mehta JL. Metabolic syndrome: does it differ between women and men? Cardiovasc Drugs Ther. (2015) 29(4):329–38. doi: 10.1007/s10557-015-6593-6
81. Juonala M, Ellul S, Lawlor DA, Santos Ferreira DL, Carlin JB, Cheung M, et al. A cross-cohort study examining the associations of metabolomic profile and subclinical atherosclerosis in children and their parents: the child health CheckPoint study and avon longitudinal study of parents and children. J Am Heart Assoc. (2019) 8(14):e011852–e011852. doi: 10.1161/JAHA.118.011852
82. Cook S, Weitzman M, Auinger P, Nguyen M, Dietz WH. Prevalence of a metabolic syndrome phenotype in adolescents: findings from the third national health and nutrition examination survey, 1988-1994. Arch Pediatr Adolesc Med. (2003) 157(8):821–7. doi: 10.1001/archpedi.157.8.821
83. McGill HC, McMahan CA, Malcom GT, Oalmann MC, Strong JP. Relation of glycohemoglobin and adiposity to atherosclerosis in youth. Pathobiological determinants of atherosclerosis in youth (PDAY) research group. Arterioscler Thromb Vasc Biol. (1995) 15(4):431–40. doi: 10.1161/01.ATV.15.4.431
84. Aldhoon-Hainerová I, Zamrazilová H, Dušátková L, Sedláčková B, Hlavatý P, Hill M, et al. Glucose homeostasis and insulin resistance: prevalence, gender differences and predictors in adolescents. Diabetol Metab Syndr. (2014) 6(1):100. doi: 10.1186/1758-5996-6-100
85. Moran A, Jacobs DR, Steinberger J, Hong CP, Prineas R, Luepker R, et al. Insulin resistance during puberty: results from clamp studies in 357 children. Diabetes. (1999) 48(10):2039–44. doi: 10.2337/diabetes.48.10.2039
86. Potau N, Ibañez L, Riqué S, Carrascosa A. Pubertal changes in insulin secretion and peripheral insulin sensitivity. Horm Res. (1997) 48(5):219–26. doi: 10.1159/000185519
87. Goran MI, Gower BA. Longitudinal study on pubertal insulin resistance. Diabetes. (2001) 50(11):2444–50. doi: 10.2337/diabetes.50.11.2444
88. Tsai EC, Matsumoto AM, Fujimoto WY, Boyko EJ. Association of bioavailable, free, and total testosterone with insulin resistance: influence of sex hormone-binding globulin and body fat. Diabetes Care. (2004) 27(4):861–8. doi: 10.2337/diacare.27.4.861
89. Ding EL, Song Y, Manson JE, Rifai N, Buring JE, Liu S. Plasma sex steroid hormones and risk of developing type 2 diabetes in women: a prospective study. Diabetologia. (2007) 50(10):2076–84. doi: 10.1007/s00125-007-0785-y
90. Burlutskaya AV, Tril VE, Polischuk LV, Pokrovskii VM. Dyslipidemia in pediatrician’s practice. Rev Cardiovasc Med. (2021) 22(3):817–34. doi: 10.31083/j.rcm2203088
91. Zimmet P, Alberti G, Kaufman F, Tajima N, Silink M, Arslanian S, et al. The metabolic syndrome in children and adolescents. Lancet. (2007) 369(9579):2059–61. doi: 10.1016/S0140-6736(07)60958-1
92. Freedman DS, Otvos JD, Jeyarajah EJ, Shalaurova I, Cupples A, Parise H, et al. Sex and age differences in lipoprotein subclasses measured by nuclear magnetic resonance spectroscopy: the framingham study. Clin Chem. (2004) 50(7):1189–200. doi: 10.1373/clinchem.2004.032763
93. Robinson GA, Peng J, Peckham H, Pineda-Torra I, Jury EC. Sex hormones drive changes in lipoprotein metabolism (2021).
94. Della Torre S, Mitro N, Fontana R, Gomaraschi M, Favari E, Recordati C, et al. An essential role for liver ERα in coupling hepatic metabolism to the reproductive cycle. Cell Rep. (2016) 15(2):360–71. doi: 10.1016/j.celrep.2016.03.019
95. van Velzen DM, Adorni MP, Zimetti F, Strazzella A, Simsek S, Sirtori CR, et al. The effect of transgender hormonal treatment on high density lipoprotein cholesterol efflux capacity. Atherosclerosis. (2021) 323:44–53. doi: 10.1016/j.atherosclerosis.2021.03.008
96. Roeters Van Lennep JE, Westerveld HT, Erkelens DW, Van Der Wall EE. Risk factors for coronary heart disease: implications of gender. Cardiovasc Res. (2002) 53(3):538–49. doi: 10.1016/S0008-6363(01)00388-1
97. Tan CE, Forster L, Caslake MJ, Bedford D, Watson TDG, McConnell M, et al. Relations between plasma lipids and postheparin plasma lipases and VLDL and LDL subfraction patterns in normolipemic men and women. Arterioscler Thromb Vasc Biol. (1995) 15(11):1839–48. doi: 10.1161/01.ATV.15.11.1839
98. Campos H, Blijlevens E, McNamara JR, Ordovas JM, Posner BM, Wilson PWF, et al. LDL Particle size distribution. Results from the framingham offspring study. Arterioscler Thromb. (1992) 12(12):1410–9. doi: 10.1161/01.ATV.12.12.1410
99. McNamara JR, Campos H, Ordovas JM, Peterson J, Wilson PW, Schaefer EJ. Effect of gender, age, and lipid status on low density lipoprotein subfraction distribution. Results from the framingham offspring study. Arteriosclerosis. (1987) 7(5):483–90. doi: 10.1161/01.ATV.7.5.483
100. Freedman DS, Bowman BA, Srinivasan SR, Berenson GS, Otvos JD. Distribution and correlates of high-density lipoprotein subclasses among children and adolescents. Metab Clin Exp. (2001) 50(3):370–6. doi: 10.1053/meta.2001.21027
101. Eissa MA, Mihalopoulos NL, Holubkov R, Dai S, Labarthe DR. Changes in fasting lipids during puberty. J Pediatr. (2016) 170:199–205. doi: 10.1016/j.jpeds.2015.11.018
102. Hilliard LM, Sampson AK, Brown RD, Denton KM. The “his and hers” of the renin-angiotensin system. Curr Hypertens Rep. (2013) 15(1):71–9. doi: 10.1007/s11906-012-0319-y
103. Flynn J, Zhang Y, Solar-Yohay S, Shi V. Clinical and demographic characteristics of children with hypertension. Hypertension. (2012) 60(4):1047–54. doi: 10.1161/HYPERTENSIONAHA.112.197525
104. Ashraf M, Irshad M, Parry NA. Pediatric hypertension: an updated review. Clin Hypertens. (2020) 26(1):22. doi: 10.1186/s40885-020-00156-w
105. Bell CS, Samuel JP, Samuels JA. Prevalence of hypertension in children. Hypertension. (2019) 73(1):148–52. doi: 10.1161/HYPERTENSIONAHA.118.11673
106. Edvardsson VO, Steinthorsdottir SD, Eliasdottir SB, Indridason OS, Palsson R. Birth weight and childhood blood pressure. Curr Hypertens Rep. (2012) 14(6):596–602. doi: 10.1007/s11906-012-0311-6
107. Weres A, Baran J, Czenczek-Lewandowska E, Leszczak J, Mazur A. Impact of birth weight and length on primary hypertension in children. Int J Environ Res Public Health. (2019) 16(23):4649. doi: 10.3390/ijerph16234649
108. Wojcik M, Starzyk JB, Drozdz M, Drozdz D. Effects of puberty on blood pressure trajectories—underlying processes. Curr Hypertens Rep. (2023) 25(7):117–25. doi: 10.1007/s11906-023-01241-9
109. O’Neill KN, Bell JA, Davey Smith G, Tilling K, Kearney PM, O’Keeffe LM. Puberty timing and sex-specific trajectories of systolic blood pressure: a prospective cohort study. Hypertension. (2022) 79(8):1755–64. doi: 10.1161/HYPERTENSIONAHA.121.18531
110. Colafella KMM, Denton KM. Sex-specific differences in hypertension and associated cardiovascular disease. Nat Rev Nephrol. (2018) 14(3):185–201. doi: 10.1038/nrneph.2017.189
111. Pilote L, Dasgupta K, Guru V, Humphries KH, McGrath J, Norris C, et al. A comprehensive view of sex-specific issues related to cardiovascular disease. CMAJ. (2007) 176(6):S1–44. doi: 10.1503/cmaj.051455
112. Kent AL, Kecskes Z, Shadbolt B, Falk MC. Normative blood pressure data in the early neonatal period. Pediatr Nephrol. (2007) 22(9):1335–41. doi: 10.1007/s00467-007-0480-8
113. Flynn JT, Daniels SR, Hayman LL, Maahs DM, McCrindle BW, Mitsnefes M, et al. Update: ambulatory blood pressure monitoring in children and adolescents: a scientific statement from the American heart association. Hypertension. (2014) 63(5):1116–35. doi: 10.1161/HYP.0000000000000007
114. Shen W, Zhang T, Li S, Zhang H, Xi B, Shen H, et al. Race and sex differences of long-term blood pressure profiles from childhood and adult hypertension: the bogalusa heart study. Hypertension. (2017) 70(1):66–74. doi: 10.1161/HYPERTENSIONAHA.117.09537
115. Cadeddu C, Franconi F, Cassisa L, Campesi I, Pepe A, Cugusi L, et al. Arterial hypertension in the female world: pathophysiology and therapy. J Cardiovasc Med. (2016) 17(4):229–36. doi: 10.2459/JCM.0000000000000315
116. Dubey RK, Oparil S, Imthurn B, Jackson EK. Sex hormones and hypertension. Cardiovasc Res. (2002) 53(3):688–708. doi: 10.1016/S0008-6363(01)00527-2
117. Butkevich A, Abraham C, Phillips RA. Hormone replacement therapy and 24-hour blood pressure profile of postmenopausal women. Am J Hypertens. (2000) 13(9):1039–41. doi: 10.1016/S0895-7061(00)00284-3
118. Cacciatore B, Paakkari I, Hasselblatt R, Nieminen MS, Toivonen J, Tikkanen MI, et al. Randomized comparison between orally and transdermally administered hormone replacement therapy regimens of long-term effects on 24-hour ambulatory blood pressure in postmenopausal women. Am J Obstet Gynecol. (2001) 184(5):904–9. doi: 10.1067/mob.2001.111246
119. Szekacs B, Vajo Z, Acs N, Hada P, Csuzi L, Bezeredi J, et al. Hormone replacement therapy reduces mean 24-hour blood pressure and its variability in postmenopausal women with treated hypertension. Menopause. (2000) 7(1):31–5. doi: 10.1097/00042192-200007010-00006
120. Crane MG, Harris JJ, Winsor W. Hypertension, oral contraceptive agents, and conjugated estrogens. Ann Intern Med. (1971) 74(1):13–21. doi: 10.7326/0003-4819-74-1-13
121. Notelovitz M. Effect of Natural Oestrogens on Blood Pressure and Weight in Postmenopausal Women.
122. Utian WH. Effect of postmenopausal estrogen therapy on diastolic blood pressure and bodyweight. Maturitas. (1978) 1(1):3–8. doi: 10.1016/0378-5122(78)90003-8
123. Karalis I, Beevers DG, Beevers M, Lip GYH. Hormone replacement therapy and arterial blood pressure in postmenopausal women with hypertension. Blood Press. (2005) 14(1):38–44. doi: 10.1080/08037050510008788
124. Schunkert H, Jan Danser AH, Hense HW, Derkx FHM, Kürzinger S, Riegger GAJ. Effects of estrogen replacement therapy on the renin-angiotensin system in postmenopausal women. Circulation. (1997) 95(1):39–45. doi: 10.1161/01.CIR.95.1.39
125. Ashraf MS, Vongpatanasin W. Estrogen and hypertension. Curr Hypertens Rep. (2006) 8(5):368–76. doi: 10.1007/s11906-006-0080-1
126. Klett C, Ganten D, Hellmann W, Kaling M, Ryffel GU, Weimar-Ehl T, et al. Regulation of hepatic angiotensinogen synthesis and secretion by steroid hormones. Endocrinology. (1992) 130(6):3660–8. doi: 10.1210/endo.130.6.1597163
127. Brosnihan KB, Hodgin JB, Smithies O, Maeda N, Gallagher P. Tissue-specific regulation of ACE/ACE2 and AT1/AT2 receptor gene expression by oestrogen in apolipoprotein E/oestrogen receptor-α knock-out mice. Exp Physiol. (2008) 93(5):658–64. doi: 10.1113/expphysiol.2007.041806
128. Mendelsohn ME, Karas RH. The protective effects of estrogen on the cardiovascular system. NEMJ. (1999) 340(23):1801–11. doi: 10.1056/NEJM199906103402306
129. Pasqualini C, Leviel V, Guibert B, Faucon-Biguet N, Kerdelhué B. Inhibitory actions of acute estradiol treatment on the activity and quantity of tyrosine hydroxylase in the median eminence of ovariectomized rats. J Neuroendocrinol. (1991) 3(5):575–80. doi: 10.1111/j.1365-2826.1991.tb00319.x
130. Ylikorkala O, Orpana A, Puolakka J, Pyörälä T, Viinikka L. Postmenopausal hormonal replacement decreases plasma levels of endothelin-1. J Clin Endocrinol Metab. (1995) 80(11):3384–7.7593457
131. Yanagisawa M, Kurihara H, Kimura S, Tomobe Y, Kobayashi M, Mitsui Y, et al. A novel potent vasoconstrictor peptide produced by vascular endothelial cells. Nature. (1988) 332(6163):411–5. doi: 10.1038/332411a0
132. Akishita M, Ouchi Y, Miyoshi H, Orimo A, Kozaki K, Eto M, et al. Estrogen inhibits endothelin-1 production and c-fos gene expression in rat aorta. Atherosclerosis. (1996) 125(1):27–38. doi: 10.1016/0021-9150(96)05836-4
133. Tan Z, Wang TH, Yang D, Fu XD, Pan JY. Mechanisms of 17β-estradiol on the production of ET-1 in ovariectomized rats. Life Sci. (2003) 73(21):2665–74. doi: 10.1016/S0024-3205(03)00605-2
134. Rodrigo MC, Martin DS, Eyster KM. Vascular ECE-1 mRNA expression decreases in response to estrogens. Life Sci. (2003) 73(23):2973–83. doi: 10.1016/j.lfs.2003.05.001
135. Akishita M, Kozaki K, Eto M, Yoshizumi M, Ishikawa M, Toba K, et al. Estrogen attenuates endothelin-1 production by bovine endothelial cells via estrogen receptor. Biochem Biophys Res Commun. (1998) 251(1):17–21. doi: 10.1006/bbrc.1998.9409
136. Hong HJ, Liu JC, Chan P, Juan SH, Loh SH, Lin JG, et al. 17beta-estradiol Downregulates angiotensin-II-induced endothelin-1 gene expression in rat aortic smooth muscle cells. J Biomed Sci. (2004) 11(1):27–36.14730207
137. Chao HH, Chen JJ, Chen CH, Lin H, Cheng CF, Lian WS, et al. Inhibition of angiotensin II induced endothelin-1 gene expression by 17-β-oestradiol in rat cardiac fibroblasts. Heart. (2005) 91(5):664–9. doi: 10.1136/hrt.2003.031898
138. Polderman KH, Stehouwer CDA, Van Kamp GJ, Dekker GA, Verheugt FWA, Gooren LJG. Influence of sex hormones on plasma endothelin levels. Ann Intern Med. (1993) 118(6):429–32. doi: 10.7326/0003-4819-118-6-199303150-00006
139. Van Kesteren PJM, Kooistra T, Lansink M, Van Kamp GJ, Asscheman H, Gooren LJG, et al. The effects of sex steroids on plasma levels of marker proteins of endothelial cell functioning. Thromb Haemost. (1998) 79(5):1029–33. doi: 10.1055/s-0037-1615115
140. Sampson AK, Jennings GLR, Chin-Dusting JPF. Y are males so difficult to understand?: a case where “x” does not mark the spot. Hypertension. (2012) 59(3):525–31. doi: 10.1161/HYPERTENSIONAHA.111.187880
141. Sullivan JC. Sex and the renin-angiotensin system: inequality between the sexes in response to RAS stimulation and inhibition. Am J Physiol Regul Integr Comp Physiol. (2008) 294(4):1220–6. doi: 10.1152/ajpregu008642007
142. Ely D, Liu B. Testosterone increases: sodium reabsorption, blood pressure, and renal pathology in female spontaneously hypertensive rats on a high sodium diet. Adv Pharmacol Sci. (2011) 2011:817835. doi: 10.1155/2011/817835
143. Sampson AK, Moritz KM, Denton KM. Postnatal ontogeny of angiotensin receptors and ACE2 in male and female rats. Gend Med. (2012) 9(1):21–32. doi: 10.1016/j.genm.2011.12.003
144. Silva-Antonialli MM, Tostes RCA, Fernandes L, Fior-Chadi DR, Akamine EH, Carvalho MHC, et al. A lower ratio of AT1/AT2 receptors of angiotensin II is found in female than in male spontaneously hypertensive rats. Cardiovasc Res. (2004) 62(3):587–93. doi: 10.1016/j.cardiores.2004.01.020
145. Cutler JA, Sorlie PD, Wolz M, Thom T, Fields LE, Roccella EJ. Trends in hypertension prevalence, awareness, treatment, and control rates in United States adults between 1988 and 1994 and 1999-2004. Hypertension. (2008) 52(5):818–27. doi: 10.1161/HYPERTENSIONAHA.108.113357
146. Everett B, Zajacova A. Gender differences in hypertension and hypertension awareness among young adults. Biodemography Soc Biol. (2015) 61(1):1–17. doi: 10.1080/19485565.2014.929488
147. Gilardini L, Pasqualinotto L, Di Matteo S, Caffetto K, Croci M, Girola A, et al. Factors associated with early atherosclerosis and arterial calcifications in young subjects with a benign phenotype of obesity. Obesity. (2011) 19(8):1684–9. doi: 10.1038/oby.2010.342
148. McGill HC, Alex McMahan C, Tracy RE, Oalmann MC, Frederick Cornhill J, Herderick EE, et al. Relation of a postmortem renal Index of hypertension to atherosclerosis and coronary artery size in young men and women. Arterioscler Thromb Vasc Biol. (1998) 18(7):1108–18. doi: 10.1161/01.ATV.18.7.1108
149. Muller M, Van Den Beld AW, Bots ML, Grobbee DE, Lamberts SWJ, Van Der Schouw YT. Endogenous sex hormones and progression of carotid atherosclerosis in elderly men. Circulation. (2004) 109(17):2074–9. doi: 10.1161/01.CIR.0000125854.51637.06
150. Robinson G, Pineda-Torra I, Ciurtin C, Jury EC. Lipid metabolism in autoimmune rheumatic disease: implications for modern and conventional therapies. J Clin Invest. (2022) 132(2):e148552. doi: 10.1172/JCI148552
151. Jones A, Cotton A, Guinto J, Wilton J, Ciurtin C. Outcome measures of disease activity for rare autoimmune rheumatic diseases. Br J Hosp Med. (2018) 79(7):396–401. doi: 10.12968/hmed.2018.79.7.396
152. Brown GA, Cotton A, Guinto J, Sharma A, Ciurtin C. Outcome measures of disease activity in inflammatory arthritis. Br J Hosp Med. (2017) 78(8):432–7. doi: 10.12968/hmed.2017.78.8.432
153. Van Andel EM, Koopmann BDM, Crouwel F, Noomen CG, de Boer NKH, van Asseldonk DP, et al. Systematic review of development and content validity of patient-reported outcome measures in inflammatory bowel disease: do we measure what we measure? J Crohn’s Colitis. (2020) 14(9):1299–315. doi: 10.1093/ecco-jcc/jjaa057
154. Smith EMD, Aggarwal A, Ainsworth J, Al-Abadi E, Avcin T, Bortey L, et al. Towards development of treat to target (T2T) in childhood-onset systemic lupus erythematosus: pReS-endorsed overarching principles and points-to-consider from an international task force. Ann Rheum Dis. (2023) 82(6):788–98. doi: 10.1136/ard-2022-223328
155. Collison J. Treat-to-target possible for JIA. Nat Rev Rheumatol. (2018) 14(12):684. doi: 10.1038/s41584-018-0119-0
156. Nimri R, Lebenthal Y, Shalitin S, Benzaquen H, Demol S, Phillip M. Metabolic control by insulin detemir in basal-bolus therapy: treat-to-target study in children and adolescents with type 1 diabetes. Pediatr Diabetes. (2013) 14(3):196–202. doi: 10.1111/pedi.12012
157. Ciurtin C, Robinson GA, Pineda-Torra I, Jury EC. Challenges in implementing cardiovascular risk scores for assessment of young people with childhood-onset autoimmune rheumatic conditions. Front Med (Lausanne). (2022) 9:814905. doi: 10.3389/fmed.2022.814905
158. Ciurtin C, Robinson GA, Pineda-Torra I, Jury EC. Comorbidity in young patients with juvenile systemic lupus erythematosus: how can we improve management? Clin Rheumatol. (2022) 41(4):961–4. doi: 10.1007/s10067-022-06093-3
159. Zonana-Nacach A, Barr SG, Magder LS, Petri M. Damage in systemic lupus erythematosus and its association with corticosteroids. Arthritis Rheumatol. (2000) 43(8):1801–8. doi: 10.1002/1529-0131(200008)43:8%3C1801::AID-ANR16%3E3.0.CO;2-O
160. Głowińska-Olszewska B, Bossowski A, Dobreńko E, Hryniewicz A, Konstantynowicz J, Milewski R, et al. Subclinical cardiovascular system changes in obese patients with juvenile idiopathic arthritis. Mediators Inflamm. (2013) 2013:436702. doi: 10.1155/2013/436702
161. Amine B, Ibn Yacoub Y, Rostom S, Hajjaj-Hassouni N. Prevalence of overweight among Moroccan children and adolescents with juvenile idiopathic arthritis. Joint Bone Spine. (2011) 78(6):584–6. doi: 10.1016/j.jbspin.2011.02.001
162. Głowińska-Olszewska B, Dobreńko E, Hryniewicz A, Tołwińska J, Żelazowska-Rutkowska B, Wojciuk M, et al. Influence of obesity on the early atherosclerosis in children with juvenile idiopathic arthritis. Reumatologia/Rheumatology. (2011) 49(4):231–8.
163. Schenck S, Niewerth M, Sengler C, Trauzeddel R, Thon A, Minden K, et al. Prevalence of overweight in children and adolescents with juvenile idiopathic arthritis. Scand J Rheumatol. (2015) 44(4):288–95. doi: 10.3109/03009742.2014.999351
164. Pelajo CF, Lopez-Benitez JM, Miller LC. Obesity and disease activity in juvenile idiopathic arthritis. Pediatr Rheumatol Online J. (2012) 10:3. doi: 10.1186/1546-0096-10-3
165. Souza L, Machado SH, Bredemeier M, Brenol JCT, Xavier RM. Effect of inflammatory activity and glucocorticoid [corrected] use on nutritional variables in patients with juvenile idiopathic arthritis. J Rheumatol. (2006) 33(3):601–8.16511929
166. Giani T, De Masi S, Maccora I, Tirelli F, Simonini G, Falconi M, et al. The influence of overweight and obesity on treatment response in juvenile idiopathic arthritis. Front Pharmacol. (2019) 10:637. doi: 10.3389/fphar.2019.00637
167. Suppasit P, Vilaiyuk S, Poomthavorn P, Pongratanakul S, Khlairit P, Mahachoklertwattana P. Glucose metabolism in systemic juvenile idiopathic arthritis. Pediatric Rheumatology. (2022) 20(1):1–8. doi: 10.1186/s12969-022-00714-6
168. Silva MG, Borba EF, de Mello SBV, Shinjo SK. Serum adipocytokine profile and metabolic syndrome in young adult female dermatomyositis patients. Clinics. (2016) 71(12):709–14. doi: 10.6061/clinics/2016(12)06
169. Coyle K, Rother KI, Weise M, Ahmed A, Miller FW, Rider LG. Metabolic abnormalities and cardiovascular risk factors in children with myositis. J Pediatr. (2009) 155(6):882–7. doi: 10.1016/j.jpeds.2009.06.009
170. Held M, Sestan M, Jelusic M. Obesity as a comorbidity in children and adolescents with autoimmune rheumatic diseases. Rheumatol Int. (2022) 1:1–11. doi: 10.1007/s00296-022-05238-6
171. Sinicato NA, Postal M, Peres FA, Peliçari KDO, Marini R, Dos Santos ADO, et al. Obesity and cytokines in childhood-onset systemic lupus erythematosus. J Immunol Res. (2014) 2014:162047. doi: 10.1155/2014/162047
172. Mackay AG, Oliver JD, Perenna Rogers M. Regulation of lipoprotein lipase activity and mRNA content in rat epididymal adipose tissue in vitro by recombinant tumour necrosis factor. Biochem J. (1990) 269:123–6. doi: 10.1042/bj2690123
173. Pittas AG, Joseph NA, Greenberg AS. Adipocytokines and insulin resistance. J Clin Endocrinol Metab. (2004) 89(2):447–52. doi: 10.1210/jc.2003-031005
174. Sinicato NA, Postal M, de Oliveira Peliçari K, Rittner L, Marini R, Appenzeller S. Prevalence and features of metabolic syndrome in childhood-onset systemic lupus erythematosus. Clin Rheumatol. (2017) 36(7):1527–35. doi: 10.1007/s10067-017-3602-0
175. Lalla E, Cheng B, Lal S, Tucker S, Greenberg E, Goland R, et al. Periodontal changes in children and adolescents with diabetes: a case-control study. Diabetes Care. (2006) 29(2):295–9. doi: 10.2337/diacare.29.02.06.dc05-1355
176. Vaziri F, Bahrololoomi Z, Savabieh Z, Sezavar K. The relationship between children’s body mass index and periodontal status. J Indian Soc Periodontol. (2022) 26(1):64–8. doi: 10.4103/jisp.jisp_899_20.35136319
177. Karami S, Ghobadi N, Pakravan A, Dabirian M, Sobouti F. Periodontal diseases and possible future cardiovascular events, are they related? An overview. J Pediatr Rev. (2017) 6(1):44–8. doi: 10.5812/jpr.11144
178. D’Aiuto F, Gkranias N, Bhowruth D, Khan T, Orlandi M, Suvan J, et al. Systemic effects of periodontitis treatment in patients with type 2 diabetes: a 12 month, single-centre, investigator-masked, randomised trial. Lancet Diabetes Endocrinol. (2018) 6(12):954–65. doi: 10.1016/S2213-8587(18)30038-X
179. Hussain SB, Leira Y, Zehra SA, Botelho J, Machado V, Ciurtin C, et al. Periodontitis and systemic lupus erythematosus: a systematic review and meta-analysis. J Periodontal Res. (2022) 57(1):1–10. doi: 10.1111/jre.12936
180. Hussain SB, Botelho J, Machado V, Zehra SA, Mendes JJ, Ciurtin C, et al. Is there a bidirectional association between rheumatoid arthritis and periodontitis? A systematic review and meta-analysis. Semin Arthritis Rheum. (2020) 50. doi: 10.1016/j.semarthrit.2020.01.009
181. Homma TK, Endo CM, Saruhashi T, Mori API, de Noronha RM, Monte O, et al. Dyslipidemia in young patients with type 1 diabetes mellitus. Arch Endocrinol Metab. (2015) 59(3):215–9. doi: 10.1590/2359-3997000000040
182. Polak M, Souchon PF, Benali K, Tubiana-Rufi N, Czernichow P. Type 1 diabetic children have abnormal lipid profiles during pubertal years. Pediatr Diabetes. (2000) 1(2):74–81. doi: 10.1034/j.1399-5448.2000.010204.x
183. Cattalini M, Soliani M, Caparello MC, Cimaz R. Sex differences in pediatric rheumatology. Clin Rev Allergy Immunol. (2019) 56(3):293–307. doi: 10.1007/s12016-017-8642-3
184. Lambert NC. Nonendocrine mechanisms of sex bias in rheumatic diseases. Nat Rev Rheumatol. (2019) 15(11):673–86. doi: 10.1038/s41584-019-0307-6
185. de Gruijter NM, Naja M, Peckham H, Radziszewska A, Kinsella M, Glenister J, et al. A systematic review exploring the bidirectional relationship between puberty and autoimmune rheumatic diseases. Pediatr Rheumatol Online J. (2021) 19(1):47. doi: 10.1186/s12969-021-00528-y
186. Webb K, Peckham H, Radziszewska A, Menon M, Oliveri P, Simpson F, et al. Sex and pubertal differences in the type 1 interferon pathway associate with both X chromosome number and serum sex hormone concentration. Front Immunol. (2019) 9:3167. doi: 10.3389/fimmu.2018.03167
188. Rustgi SD, Kayal M, Shah SC. Sex-based differences in inflammatory bowel diseases: a review. Therap Adv Gastroenterol. (2020) 13:1756284820915043. doi: 10.1177/1756284820915043
189. Wolfe F, Freundlich B, Straus WL. Increase in cardiovascular and cerebrovascular disease prevalence in rheumatoid arthritis. J Rheumatol. (2003) 30(1):36–40.12508387
190. Fransen J, Kazemi-Bajestani SMR, Bredie SJH, Popa CD. Rheumatoid arthritis disadvantages younger patients for cardiovascular diseases: a meta-analysis. PLoS One. (2016) 11(6):e0157360. doi: 10.1371/journal.pone.0157360
191. Ortiz TT, Terreri MT, Caetano M, Souza FS, D’Almeida V, Sarni ROS, et al. Dyslipidemia in pediatric systemic lupus erythematosus: the relationship with disease activity and plasma homocysteine and cysteine concentrations. Ann Nutr Metab. (2013) 63(1–2):77–82. doi: 10.1159/000351076
192. Robinson GA, Peng J, Pineda-Torra I, Ciurtin C, Jury EC. Metabolomics defines complex patterns of dyslipidaemia in juvenile-sle patients associated with inflammation and potential cardiovascular disease risk. Metabolites. (2022) 12(1):3. doi: 10.3390/metabo12010003
193. Robinson GA, Waddington KE, Coelewij L, Peng J, Naja M, Wincup C, et al. Increased apolipoprotein-B:a1 ratio predicts cardiometabolic risk in patients with juvenile onset SLE. EBioMedicine. (2021) 65:103243. doi: 10.1016/j.ebiom.2021.103243
194. Rosano GMC. Androgens and coronary artery disease. A sex-specific effect of sex hormones? Eur Heart J. (2000) 21(11):868–71. doi: 10.1053/euhj.1999.2050
195. Kelly DM, Jones TH. Testosterone: a vascular hormone in health and disease. Article J Endocrinol. (2013) 217(3):R47–71.23549841
196. Araujo AB, Dixon JM, Suarez EA, Murad MH, Guey LT, Wittert GA. Clinical review: endogenous testosterone and mortality in men: a systematic review and meta-analysis. J Clin Endocrinol Metab. (2011) 96(10):3007–19. doi: 10.1210/jc.2011-1137
197. Lidegaard Ø, Løkkegaard E, Svendsen AL, Agger C. Hormonal contraception and risk of venous thromboembolism: national follow-up study. Br Med J. (2009) 339:7720. doi: 10.1136/bmj.b2890
198. Lidegaard Ø, Løkkegaard E, Jensen A, Skovlund CW, Keiding N. Thrombotic stroke and myocardial infarction with hormonal contraception. Obstet Gynecol Surv. (2012) 366:2257–66. doi: 10.1056/NEJMoa1111840
199. Roos-Hesselink JW, Cornette J, Sliwa K, Pieper PG, Veldtman GR, Johnson MR. Contraception and cardiovascular disease. Eur Heart J. (2015) 36.
200. Van Rooijen M, Bremme K, Rosing J, Silveira A, Thomassen S. 69 Rapid activation of haemostasis after hormonal emergency contraception. Thromb Res. (2007) 119. doi: 10.1016/S0049-3848(07)70114-7
201. Shen J, Che Y, Showell E, Chen K, Cheng L. Interventions for emergency contraception. Cochrane Database Syst Rev. (2019) 2019. doi: 10.1002/14651858.CD001324.pub
202. Dubey RK, Imthurn B, Barton M, Jackson EK. Vascular consequences of menopause and hormone therapy: importance of timing of treatment and type of estrogen. Cardiovasc Res. (2005) 66(2):295–306. doi: 10.1016/j.cardiores.2004.12.012
203. Peckham H, Webb K, Rosser EC, Butler G, Ciurtin C. Gender-Diverse inclusion in immunological research: benefits to science and health. Front Med (Lausanne). (2022) 9:909789. doi: 10.3389/fmed.2022.909789
204. Henrickson M, Giwa S, Hafford-Letchfield T, Cocker C, Mulé NJ, Schaub J, et al. Research ethics with gender and sexually diverse persons. Int J Environ Res Public Health. (2022) 17(18):6615. doi: 10.3390/ijerph17186615
206. McGill HC, Alex McMahan C, Malcom GT, Oalmann MC, Strong JP. Effects of Serum lipoproteins and smoking on atherosclerosis in young men and women. Arterioscler Thromb Vasc Biol. (1997) 17(1):95–106. doi: 10.1161/01.ATV.17.1.95
207. Gallucci G, Tartarone A, Lerose R, Lalinga AV, Capobianco AM. Cardiovascular risk of smoking and benefits of smoking cessation. J Thorac Dis. (2020) 12(7):3866. doi: 10.21037/jtd.2020.02.47
208. Barua RS, Ambrose JA. Mechanisms of coronary thrombosis in cigarette smoke exposure. Arterioscler Thromb Vasc Biol. (2013) 33(7):1460–7. doi: 10.1161/ATVBAHA.112.300154
209. Huxley RR, Woodward M. Cigarette smoking as a risk factor for coronary heart disease in women compared with men: a systematic review and meta-analysis of prospective cohort studies. Lancet. (2011) 378(9799):1297–305. doi: 10.1016/S0140-6736(11)60781-2
210. Campesi I, Carru C, Zinellu A, Occhioni S, Sanna M, Palermo M, et al. Regular cigarette smoking influences the transsulfuration pathway, endothelial function, and inflammation biomarkers in a sex-gender specific manner in healthy young humans. Am J Transl Res. (2013) 5(5):497.23977409
211. Wierzbicki AS. Homocysteine and cardiovascular disease: a review of the evidence. Diab Vasc Dis Res. (2007) 4(2):143–9. doi: 10.3132/dvdr.2007.033
212. Van Jaarsveld CHM, Fidler JA, Simon AE, Wardle J. Persistent impact of pubertal timing on trends in smoking, food choice, activity, and stress in adolescence. Psychosom Med. (2007) 69(8):798–806. doi: 10.1097/PSY.0b013e3181576106
213. Al Rifai M, DeFillippis AP, McEvoy JW, Hall ME, Acien AN, Jones MR, et al. The relationship between smoking intensity and subclinical cardiovascular injury: the multi-ethnic study of atherosclerosis (MESA). Atherosclerosis. (2017) 258:119–30. doi: 10.1016/j.atherosclerosis.2017.01.021
214. Hackshaw A, Morris JK, Boniface S, Tang JL, Milenkovi D. Low cigarette consumption and risk of coronary heart disease and stroke: meta-analysis of 141 cohort studies in 55 study reports. Br Med J. (2018) 360:j5855. doi: 10.1136/bmj.j5855
215. McEvoy JW, Nasir K, Defilippis AP, Lima JAC, Bluemke DA, Hundley WG, et al. Relationship of cigarette smoking with inflammation and subclinical vascular disease: the multi-ethnic study of atherosclerosis. Arterioscler Thromb Vasc Biol. (2015) 35:1002–10. doi: 10.1161/ATVBAHA.114.304960
216. Marcon A, Pesce G, Calciano L, Bellisario V, Dharmage SC, Garcia-Aymerich J, et al. Trends in smoking initiation in Europe over 40 years: a retrospective cohort study. PLoS One. (2018) 13(8). doi: 10.1371/journal.pone.0201881
217. Peruzzi M, Biondi-Zoccai G, Carnevale R, Cavarretta E, Frati G, Versaci F. Vaping cardiovascular health risks: an updated Umbrella review. Curr Emerg Hosp Med Rep. (2020) 8(3):103–9. doi: 10.1007/s40138-020-00219-0
218. Wong WC, Ford KA, Pagels NE, McCutcheon JE, Marinelli M. Adolescents are more vulnerable to cocaine addiction: behavioral and electrophysiological evidence. J Neurosci. (2013) 33(11):4913–22. doi: 10.1523/JNEUROSCI.1371-12.2013
219. Morentin B, Ballesteros J, Callado LF, Meana JJ. Recent cocaine use is a significant risk factor for sudden cardiovascular death in 15-49-year-old subjects: a forensic case-control study. Addiction. (2014) 109(12). doi: 10.1111/add.12691
221. Velázquez JAV, Gamiño MNB, Ito DAF, Bautista CF, de López MLG, Buenabad NGA, et al. Contextual factors associated with marijuana use in school population. Salud Mental. (2017) 40(3).
222. Lac A, Unger JB, Basáñez T, Ritt-Olson A, Soto DW, Baezconde-Garbanati L. Marijuana use among latino adolescents: gender differences in protective familial factors. Subst Use Misuse. (2011) 46(5):644–55. doi: 10.3109/10826084.2010.528121
223. Rusby JC, Light JM, Crowley R, Westling E. Influence of parent-youth relationship, parental monitoring, and parent substance use on adolescent substance use onset. J Fam Psychol. (2018) 32(3):310–20. doi: 10.1037/fam0000350
224. Henry KL, Smith EA, Caldwell LL. Deterioration of academic achievement and marijuana use onset among rural adolescents. Health Educ Res. (2007) 22(3):372–84. doi: 10.1093/her/cyl083
225. Darke S, Kaye S, Duflou J. Comparative cardiac pathology among deaths due to cocaine toxicity, opioid toxicity and non-drug-related causes. Addiction. (2006) 101(12):1771–7. doi: 10.1111/j.1360-0443.2006.01601.x
Keywords: cardiovascular risk factors, children and young poeple, chronic inflammation, puberty, obesity, sex determinants
Citation: Allalou A, Peng J, Robinson GA, Marruganti C, D’Aiuto F, Butler G, Jury EC and Ciurtin C (2023) Impact of puberty, sex determinants and chronic inflammation on cardiovascular risk in young people. Front. Cardiovasc. Med. 10:1191119. doi: 10.3389/fcvm.2023.1191119
Received: 21 March 2023; Accepted: 14 June 2023;
Published: 27 June 2023.
Edited by:
Zhen-Yu Zhang, KU Leuven, BelgiumReviewed by:
Kate Denton, Monash University, AustraliaVannina Gonzalez Marrachelli, University of Valencia, Spain
© 2023 Allalou, Peng, Robinson, Marruganti, D'Aiuto, Butler, Jury and Ciurtin. This is an open-access article distributed under the terms of the Creative Commons Attribution License (CC BY). The use, distribution or reproduction in other forums is permitted, provided the original author(s) and the copyright owner(s) are credited and that the original publication in this journal is cited, in accordance with accepted academic practice. No use, distribution or reproduction is permitted which does not comply with these terms.
*Correspondence: Coziana Ciurtin Yy5jaXVydGluQHVjbC5hYy51aw==