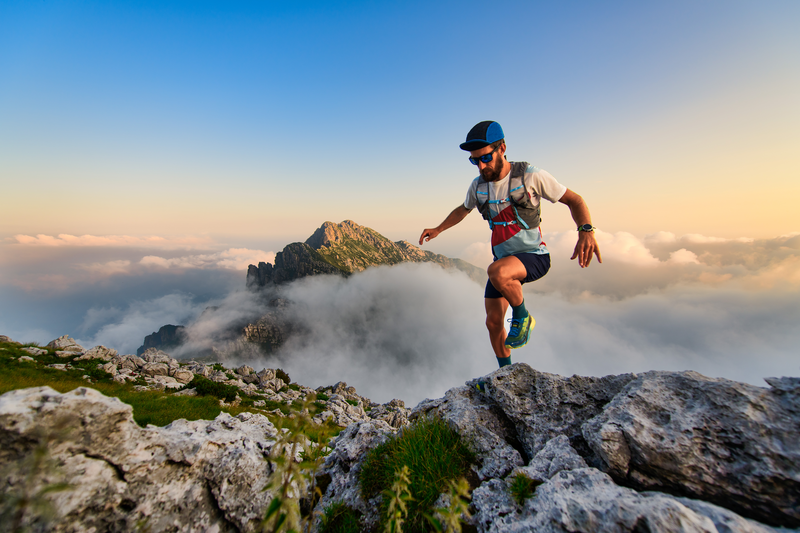
94% of researchers rate our articles as excellent or good
Learn more about the work of our research integrity team to safeguard the quality of each article we publish.
Find out more
EDITORIAL article
Front. Cardiovasc. Med. , 18 April 2023
Sec. Clinical and Translational Cardiovascular Medicine
Volume 10 - 2023 | https://doi.org/10.3389/fcvm.2023.1184030
This article is part of the Research Topic Insights in Cardiovascular Therapeutics: 2022 View all 10 articles
Editorial on the Research Topic
Insights in cardiovascular therapeutics 2022—cardiovascular innate immunity
Thanks to the efforts and support of the authors and editorial team, our Research topic entitled “Insights in Cardiovascular Therapeutics: 2022” in the Frontiers in Cardiovascular Medicine, Cardiovascular Pharmacology and Drug Discovery Section has achieved great success and attracted more than 13,500 views in the past 12 months. Within this topic, we highlight nine original research papers published related to cardiovascular tissue injury and remodeling, cardiovascular innate immunity, trained immunity, and recent advances in cardiovascular therapies. These highlights may serve as the foundation for new developments in cardiovascular pharmacology and drug discovery areas. Looking ahead to 2023, we will continue our work to provide an outstanding platform for cardiologists, translational cardiovascular scientists, and cardiovascular pharmacological scientists to share new findings in clinical cardiology, cardiovascular pharmacology and drug discovery, and translational cardiovascular therapeutics.
Cardiovascular diseases (CVDs) represent a leading cause of death worldwide. However, the specific mechanisms and potential treatment options for CVDs have yet to be fully addressed. Noteworthy, chronic non-resolving low-grade inflammation is known to be a major feature in the pathogenesis of CVDs (1). Increasing evidence indicates that the innate immune system contributes to CVD development (2–4). Recently, it has been discovered that innate immune cells can produce a long-lasting proinflammatory phenotype after certain stimulations by either exogenous pathogen-associated molecular patterns (PAMPs)/damage-associated molecular patterns (DAMPs) or endogenous metabolic stress-derived stimuli (2, 5). This persistent hyper-activation of the innate immune system is referred to trained immunity (also termed as innate immune memory) (4, 6–14).
Trained immunity is a functional status of enhanced innate immune/proinflammatory responses via metabolic reprogramming in generating methyl, acetyl, and other chemical moieties (15), which induces long-term epigenetic reprogramming around the promoters of inflammatory genes (8, 16). These epigenetic changes are associated with immune protection against infections or exacerbated inflammations (11) after re-stimulation (14). In contrast to the adaptive immune system, trained immunity lacks antigen-specific recognition (17–19), but leads to a cross-reaction and protects against different pathogens other than the one to which it was initially exposed (3). Nevertheless, as with adaptive immunity (20), innate immune cells may develop immunological memory after encountering a specific insult to adjust their response to subsequent stimulations (14). Innate immune cells that have been “trained” respond more effectively to the possibility of re-stimulation by the same or different insults. One of the other differences between adaptive immune memory and innate immune memory is that special subsets of adaptive immune cells carry out memory functions (21), whereas innate immune memory is the functional status of all innate immune cells experienced metabolic reprogramming (4). Trained immunity serves as a new mechanism underlying chronic metabolic cardiovascular diseases. In addition, trained immunity can be a qualification criteria for environmental, metabolic, and infectious stimuli to become significant cardiovascular disease risk factors such as hyperlipidemia (22–27), hyperglycemia (28–30), hyperhomocysteinemia (31, 32), cigarette smoke (12, 33, 34), hypertension, infections (11, 35), metabolic syndrome, and obesity (23, 36, 37), which are different from insignificant endogenous metabolites and compounds in the foods in inducing trained immunity and enhancing inflammation (8, 33).
As mentioned above, trained immunity is inseparable from innate immune cells. Classically, phagocytes (macrophages and neutrophils), mast cells, dendritic cells, basophils, eosinophils, natural killer (NK) cells, and innate lymphoid cells are identified as innate immune cells (38). Despite their various types, innate immune cells share a common feature: they are all monocytic and antigen-presenting white blood cells. This type of innate immune cell functionalized the role of cell migration and engulfment in cellular interaction during the immune process or inflammation. With the intensive study of the immune system, scientists discovered that innate immune cells are not limited to white blood cells. However, stressful circumstances could transform somatic cells into innate immune cells. Endothelial cells (ECs), the innermost layer of the vessel wall, play a critical role in maintaining cardiovascular homeostasis in health or contributing to the pathological mechanisms in multiple CVDs (39–41). In 2013 (Journal of Hematology and Oncology (42, 43), we proposed a new concept: that endothelial cells (ECs) actively participate in innate and adaptive immune responses and carry out all the innate immune functions, the same as prototypical innate immune cell macrophages (44, 45). Therefore, we classified ECs as novel immune cells. The same qualities expressed in traditional innate immune cells are also present in ECs, such as danger associated molecular patterns (DAMPs)/pathogen-associated molecular patterns (PAMPs)-sensing; secretions of cytokines, chemokines, and secretomes (35); phagocytic function; antigen presentation; pro/anti-inflammatory; immunosuppression; migration; plasticity; and heterogeneity (43). Forming the trained phenotype requires metabolic reprogramming, including transitioning from oxidative phosphorylation (OXPHOS) to enhanced glycolysis (13). Oxidized low-density lipoprotein (ox-LDL) (46), a well-established risk factor for CVD, plays a key role in the induction of trained immunity. Evidence reports that ox-LDL mediates immunologic memory in ECs by switching OXPHOS to glycolysis, via significantly increases the enrichment of histone 3 lysine 27 trimethylation/histone 3 lysine 27 acetylation (H3K27m3/H3K27ac) and H3K14ac (8) at the enhancers or promoters of proinflammatory cytokines, such as interleukin (IL) 6 and IL8, through mammalian target of rapamycin- hypoxia-inducible factor 1 alpha (mTOR-HIF1α) signaling in ECs (47). In addition to ox-LDL, reactive oxygen species (ROS) (48) are the upstream activator of the leucine-rich repeats (LRR) containing domain, nucleotide oligomerization domain (NOD), and pyrin domain-containing protein 3 (NLRP3) caspase 1 inflammasome, which has a positive correlation with trained immunity activation. Taken together, trained immunity in ECs is functional for inflammation effectiveness and transition to chronic inflammation (4).
In addition, vascular pathologies reshape vascular smooth muscle cells (VSMCs) into six different phenotypes, including contractile, mesenchymal, fibroblast, macrophage (innate immune cell prototype) (49), foam cell-like, osteochondrogenic-like, myofibroblast-like (50), osteogenic, and adipocyte in response to stimulations of DAMPs/PAMPs (51). We also reported that chronic kidney disease -uremic toxins (52) activate the VSMC phenotypic switch (53) and the proinflammatory caspase-1-inflammasome pathway (innate immune sensors) (5) to promote neointima hyperplasia in the carotid artery (54). Others have also reported that ox-LDL induces trained innate immunity in human coronary VSMCs (55). Taken together, we propose a new concept: that VSMCs in pathologies are an innate immune cell type.
Academic research plays a vital role in identifying new therapeutic targets, including understanding target biology and the connections between novel therapeutic targets and disease states. CVDs, as diseases with high mortality and morbidity, have long been the subject of research by scientists or medical experts seeking potential therapies. A comprehensive analysis of trained immunity in relation to CVD might offer novel perspectives on the pathophysiology of the disease and new treatment options. Cui et al. reported that alternate-day fasting (ADF) reduced fasting blood glucose levels and improved endothelium (EC) function in diabetic mice, indicating the therapeutic potential of blocking novel trained immunity-related metabolic pathways, including glycolysis. Ribieras et al. proved that cell adhesion molecule secretion from ECs is critical for inflammation and neovascularization in areas of wound healing and ischemia. Liu et al. demonstrated that interleukin-12 (IL12)p40, the common subunit of IL12 and IL23, was associated with the classic trained immunity stimuli: LPS-induced cardiac injury; Ren et al. showed that the agonistic analog of growth hormone-releasing hormone (GHRH-A) MR409, can effectively attenuate vascular calcification and trained immunity mediator ROS expression and improve EC function and diabetics. Table 1 summarizes nine significant studies on our research topic.
Table 1. Nine highly viewed research papers, published in our special topic entitled “Insights in Cardiovascular Therapeutics: 2022”, are summarized.
KX carried out literature collections and drafted the manuscript. YZ, FS, YS, YL, XJ, and HW provided editing input. XY supervised and edited the manuscript. All authors listed have made a substantial, direct, and intellectual contribution to the work and approved it for publication. All authors contributed to the article and approved the submitted version.
The authors declare that the research was conducted in the absence of any commercial or financial relationships that could be construed as a potential conflict of interest.
All claims expressed in this article are solely those of the authors and do not necessarily represent those of their affiliated organizations, or those of the publisher, the editors and the reviewers. Any product that may be evaluated in this article, or claim that may be made by its manufacturer, is not guaranteed or endorsed by the publisher.
1. Yang XF, Yin Y, Wang H. VASCULAR INFLAMMATION AND ATHEROGENESIS ARE ACTIVATED VIA RECEPTORS FOR PAMPs AND SUPPRESSED BY REGULATORY T CELLS. Drug Discov Today Ther Strateg. (2008) 5:125–42. doi: 10.1016/j.ddstr.2008.11.003
2. Flores-Gomez D, Bekkering S, Netea MG, Riksen NP. Trained immunity in atherosclerotic cardiovascular disease. Arterioscler Thromb Vasc Biol. (2021) 41:62–9. doi: 10.1161/ATVBAHA.120.314216
3. Zieleniewska NA, Kazberuk M, Chlabicz M, Eljaszewicz A, Kaminski K. Trained immunity as a trigger for atherosclerotic cardiovascular disease-A literature review. J Clin Med. (2022) 11(12):3369. doi: 10.3390/jcm11123369
4. Drummer C, Saaoud F, Shao Y, Sun Y, Xu K, Lu Y, et al. Trained immunity and reactivity of macrophages and endothelial cells. Arterioscler Thromb Vasc Biol. (2021) 41:1032–46. doi: 10.1161/ATVBAHA.120.315452
5. Yin Y, Pastrana JL, Li X, Huang X, Mallilankaraman K, Choi ET, et al. Inflammasomes: sensors of metabolic stresses for vascular inflammation. Front Biosci. (2013) 18:638–49. doi: 10.2741/4127
6. Drummer CIV, Saaoud F, Sun Y, Atar D, Xu K, Lu Y, et al. Hyperlipidemia may synergize with hypomethylation in establishing trained immunity and promoting inflammation in NASH and NAFLD. J Immunol Res. (2021) 2021:3928323. doi: 10.1161/ATVBAHA.120.315452
7. Zhong C, Yang X, Feng Y, Yu J. Trained immunity: an underlying driver of inflammatory atherosclerosis. Front Immunol. (2020) 11:284. doi: 10.3389/fimmu.2020.00284
8. Lu Y, Sun Y, Drummer IV C, Nanayakkara GK, Shao Y, Saaoud F, et al. Increased acetylation of H3K14 in the genomic regions that encode trained immunity enzymes in lysophosphatidylcholine-activated human aortic endothelial cells—novel qualification markers for chronic disease risk factors and conditional DAMPs. Redox Biol. (2019) 24:101221. doi: 10.1016/j.redox.2019.101221
9. Fagenson AM, Xu K, Saaoud F, Nanayakkara G, Jhala NC, Liu L, et al. Liver ischemia reperfusion injury, enhanced by trained immunity, is attenuated in caspase 1/caspase 11 double gene knockout mice. Pathogens. (2020) 9(11):879. doi: 10.3390/pathogens9110879
10. Li X, Fang P, Sun Y, Shao Y, Yang WY, Jiang X, et al. Anti-inflammatory cytokines IL-35 and IL-10 block atherogenic lysophosphatidylcholine-induced, mitochondrial ROS-mediated innate immune activation, but spare innate immune memory signature in endothelial cells. Redox Biol. (2020) 28:101373. doi: 10.1016/j.redox.2019.101373
11. Shao Y, Saredy J, Xu K, Sun Y, Saaoud F, Drummer C, et al. Endothelial immunity trained by coronavirus infections, DAMP stimulations and regulated by anti-oxidant NRF2 may contribute to inflammations, myelopoiesis, COVID-19 cytokine storms and thromboembolism. Front Immunol. (2021) 12:653110. doi: 10.3389/fimmu.2021.653110
12. Shao Y, Saaoud F, Cornwell W, Xu K, Kirchhoff A, Lu Y, et al. Cigarette smoke and morphine promote treg plasticity to Th17 via enhancing trained immunity. Cells. (2022) 11(18):2810. doi: 10.3390/cells11182810
13. Saaoud F, Liu L, Xu K, Cueto R, Shao Y, Lu Y, et al. Aorta- and liver-generated TMAO enhances trained immunity for increased inflammation via ER stress/mitochondrial ROS/glycolysis pathways. JCI Insight. (2023) 8(1):e158183. doi: 10.1172/jci.insight.158183
14. Ochando J, Mulder WJM, Madsen JC, Netea MG, Duivenvoorden R. Trained immunity—basic concepts and contributions to immunopathology. Nat Rev Nephrol. (2023) 19:23–37. doi: 10.1038/s41581-022-00633-5
15. Huang Z, Song S, Zhang X, Zeng L, Sun A, Ge J. Metabolic substrates, histone modifications, and heart failure. Biochim Biophys Acta Gene Regul Mech. (2023) 1866:194898. doi: 10.1016/j.bbagrm.2022.194898
16. Millan-Zambrano G, Burton A, Bannister AJ, Schneider R. Histone post-translational modifications—cause and consequence of genome function. Nat Rev Genet. (2022) 23:563–80. doi: 10.1038/s41576-022-00468-7
17. Yang F, Chen IH, Xiong Z, Yan Y, Wang H, Yang XF. Model of stimulation-responsive splicing and strategies in identification of immunogenic isoforms of tumor antigens and autoantigens. Clin Immunol. (2006) 121:121–33. doi: 10.1016/j.clim.2006.06.007
18. Yang F, Yang XF. New concepts in tumor antigens: their significance in future immunotherapies for tumors. Cell Mol Immunol. (2005) 2:331–41.16368059
19. Ng B, Yang F, Huston DP, Yan Y, Yang Y, Xiong Z, et al. Increased noncanonical splicing of autoantigen transcripts provides the structural basis for expression of untolerized epitopes. J Allergy Clin Immunol. (2004) 114:1463–70. doi: 10.1016/j.jaci.2004.09.006
20. Xu K, Yang WY, Nanayakkara GK, Shao Y, Yang F, Hu W, et al. GATA3, HDAC6, and BCL6 regulate FOXP3+ treg plasticity and determine treg conversion into either novel antigen-presenting cell-like treg or Th1-treg. Front Immunol. (2018) 9:45. doi: 10.3389/fimmu.2018.00045
21. Shen H, Wu N, Nanayakkara G, Fu H, Yang Q, Yang WY, et al. Co-signaling receptors regulate T-cell plasticity and immune tolerance. Front Biosci (Landmark Ed). (2019) 24:96–132. doi: 10.2741/4710
22. Yin Y, Li X, Sha X, Xi H, Li YF, Shao Y, et al. Early hyperlipidemia promotes endothelial activation via a caspase-1-sirtuin 1 pathway. Arterioscler Thromb Vasc Biol. (2015) 35:804–16. doi: 10.1161/ATVBAHA.115.305282
23. Virtue A, Johnson C, Lopez-Pastrana J, Shao Y, Fu H, Li X, et al. MicroRNA-155 deficiency leads to decreased atherosclerosis, increased white adipose tissue obesity, and non-alcoholic fatty liver disease: a NOVEL MOUSE MODEL OF OBESITY PARADOX. J Biol Chem. (2017) 292:1267–87. doi: 10.1074/jbc.M116.739839
24. Mai J, Nanayakkara G, Lopez-Pastrana J, Li X, Li YF, Wang X, et al. Interleukin-17A promotes aortic endothelial cell activation via transcriptionally and post-translationally activating p38 MAPK pathway. J Biol Chem. (2016) 291(10):4939–54. doi: 10.1074/jbc.M115.690081
25. Li X, Shao Y, Sha X, Fang P, Kuo YM, Andrews AJ, et al. IL-35 (Interleukin-35) suppresses endothelial cell activation by inhibiting mitochondrial reactive oxygen Species-mediated site-specific acetylation of H3K14 (histone 3 lysine 14). Arterioscler Thromb Vasc Biol. (2018) 38:599–609. doi: 10.1161/ATVBAHA.117.310626
26. Saaoud F, Wang J, Iwanowycz S, Wang Y, Altomare D, Shao Y, et al. Bone marrow deficiency of mRNA decaying protein tristetraprolin increases inflammation and mitochondrial ROS but reduces hepatic lipoprotein production in LDLR knockout mice. Redox Biol. (2020) 37:101609. doi: 10.1016/j.redox.2020.101609
27. Shao Y, Yang WY, Saaoud F, Drummer IV C, Sun Y, Xu K, et al. IL-35 promotes CD4 + Foxp3+ tregs and inhibits atherosclerosis via maintaining CCR5-amplified treg-suppressive mechanisms. JCI Insight. (2021) 6(19):e152511. doi: 10.1172/jci.insight.152511
28. Fang P, Zhang D, Cheng Z, Yan C, Jiang X, Kruger WD, et al. Hyperhomocysteinemia potentiates hyperglycemia-induced inflammatory monocyte differentiation and atherosclerosis. Diabetes. (2014) 63(12):4275–90. doi: 10.2337/db14-0809
29. Yan Y, Xiong Z, Zhang S, Song J, Huang Y, Thornton AM, et al. CD25high T cells with a prolonged survival inhibit development of diabetes. Int J Immunopathol Pharmacol. (2008) 21:767–80. doi: 10.1177/039463200802100401
30. Fang P, Li X, Shan H, Saredy JJ, Cueto R, Xia J, et al. Ly6C(+) inflammatory monocyte differentiation partially mediates hyperhomocysteinemia-induced vascular dysfunction in type 2 diabetic db/db mice. Arterioscler Thromb Vasc Biol. (2019) 39:2097–119. doi: 10.1161/ATVBAHA.119.313138
31. Jamaluddin MD, Chen I, Yang F, Jiang X, Jan M, Liu X, et al. Homocysteine inhibits endothelial cell growth via DNA hypomethylation of the cyclin agene. Blood. (2007) 110:3648–55. doi: 10.1182/blood-2007-06-096701
32. Jamaluddin MS, Yang X, Hyperhomocysteinemia WH. DNA Methylation and vascular disease. Clin Chem Lab Med. (2007) 45:1660–6. doi: 10.1515/CCLM.2007.350
33. Saaoud F, Shao Y, Cornwell W, Wang H, Rogers T, Yang X. Cigarette smoke modulates inflammation and immunity via ROS-regulated trained immunity and trained tolerance mechanisms. Antioxid Redox Signaling. (2022). doi: 10.1089/ars.2022.0087
34. Shao Y, Cornwell W, Xu K, Kirchhoff A, Saasoud F, Lu Y, et al. Chronic exposure to the combination of cigarette smoke and morphine decreases CD4(+) regulatory T cell numbers by reprogramming the treg cell transcriptome. Front Immunol. (2022) 13:887681. doi: 10.3389/fimmu.2022.887681
35. Lu Y, Sun Y, Xu K, Saaoud F, Shao Y, Drummer C, et al. Aorta in pathologies may function as an immune organ by upregulating secretomes for immune and vascular cell activation, differentiation and trans-differentiation-early secretomes may serve as drivers for trained immunity. Front Immunol. (2022) 13:858256. doi: 10.3389/fimmu.2022.858256
36. Johnson C, Drummer C, Virtue A, Gao T, Wu S, Hernandez M, et al. Increased expression of resistin in MicroRNA-155-deficient white adipose tissues may be a possible driver of metabolically healthy obesity transition to classical obesity. Front Physiol. (2018) 9:1297. doi: 10.3389/fphys.2018.01297
37. Johnson C, Drummer IV C, Shan H, Shao Y, Sun Y, Lu Y, et al. A novel subset of CD95(+) pro-inflammatory macrophages overcome miR155 deficiency and may serve as a switch from metabolically healthy obesity to metabolically unhealthy obesity. Front Immunol. (2020) 11:619951. doi: 10.3389/fimmu.2020.619951
38. Holmgren K. Urinary calculi and urinary tract infection. A clinical and microbiological study. Scand J Urol Nephrol Suppl. (1986) 98:1–71.3026032
39. Rubanyi GM. The role of endothelium in cardiovascular homeostasis and diseases. J Cardiovasc Pharmacol. (1993) 22(Suppl 4):S1–14. doi: 10.1097/00005344-199322004-00002
40. Xu K, Saaoud F, Yu S, Ct D, Shao Y, Sun Y, et al. Monocyte adhesion assays for detecting endothelial cell activation in vascular inflammation and atherosclerosis. Methods Mol Biol. (2022) 2419:169–82. doi: 10.1007/978-1-0716-1924-7_10
41. Xu K, Shao Y, Saaoud F, Gillespie A, Drummer C, Liu L, et al. Novel knowledge-based transcriptomic profiling of lipid lysophosphatidylinositol-induced endothelial cell activation. Front Cardiovasc Med. (2021) 8:773473. doi: 10.3389/fcvm.2021.773473
42. Mai J, Virtue A, Shen J, Wang H, Yang XF. An evolving new paradigm: endothelial cells–conditional innate immune cells. J Hematol Oncol. (2013) 6:61. doi: 10.1186/1756-8722-6-61
43. Shao Y, Saredy J, Yang WY, Sun Y, Lu Y, Saaoud F, et al. Vascular endothelial cells and innate immunity. Arterioscler Thromb Vasc Biol. (2020) 40:e138–52. doi: 10.1161/ATVBAHA.120.314330
44. Lai B, Wang J, Fagenson A, Sun Y, Saredy J, Lu Y, et al. Twenty novel disease group-specific and 12 new shared macrophage pathways in eight groups of 34 diseases including 24 inflammatory organ diseases and 10 types of tumors. Front Immunol. (2019) 10:2612. doi: 10.3389/fimmu.2019.02612
45. Drummer C, Saaoud F, Jhala NC, Cueto R, Sun Y, Xu K, et al. Caspase-11 promotes high-fat diet-induced NAFLD by increasing glycolysis, OXPHOS, and pyroptosis in macrophages. Front Immunol. (2023) 14:1113883. doi: 10.3389/fimmu.2023.1113883
46. Sun Y, Lu Y, Saredy J, Wang X, Drummer IV C, Shao Y, et al. ROS Systems are a new integrated network for sensing homeostasis and alarming stresses in organelle metabolic processes. Redox Biol. (2020) 37:101696. doi: 10.1016/j.redox.2020.101696
47. Sohrabi Y, Lagache SMM, Voges VC, Semo D, Sonntag G, Hanemann I, et al. OxLDL-mediated immunologic memory in endothelial cells. J Mol Cell Cardiol. (2020) 146:121–32. doi: 10.1016/j.yjmcc.2020.07.006
48. Li X, Fang P, Li Y, Kuo YM, Andrews AJ, Nanayakkara G, et al. Mitochondrial reactive oxygen Species mediate lysophosphatidylcholine-induced endothelial cell activation. Arterioscler Thromb Vasc Biol. (2016) 36:1090–100. doi: 10.1161/ATVBAHA.115.306964
49. Bennett MR, Sinha S, Owens GK. Vascular smooth muscle cells in atherosclerosis. Circ Res. (2016) 118:692–702. doi: 10.1161/CIRCRESAHA.115.306361
50. Grootaert MOJ, Bennett MR. Vascular smooth muscle cells in atherosclerosis: time for a re-assessment. Cardiovasc Res. (2021) 117:2326–39. doi: 10.1093/cvr/cvab046
51. Yap C, Mieremet A, de Vries CJM, Micha D, de Waard V. Six shades of vascular smooth muscle cells illuminated by KLF4 (kruppel-like factor 4). Arterioscler Thromb Vasc Biol. (2021) 41:2693–707. doi: 10.1161/ATVBAHA.121.316600
52. Sun Y, Johnson C, Zhou J, Wang L, Li YF, Lu Y, et al. Uremic toxins are conditional danger- or homeostasis-associated molecular patterns. Front Biosci (Landmark Ed). (2018) 23:348–87. doi: 10.2741/4595
53. Monroy MA, Fang J, Li S, Ferrer L, Birkenbach MP, Lee IJ, et al. Chronic kidney disease alters vascular smooth muscle cell phenotype. Front Biosci (Landmark Ed). (2015) 20:784–95. doi: 10.2741/4337
54. Ferrer LM, Monroy AM, Lopez-Pastrana J, Nanayakkara G, Cueto R, Li YF, et al. Caspase-1 plays a critical role in accelerating chronic kidney disease-promoted neointimal hyperplasia in the carotid artery. J Cardiovasc Transl Res. (2016) 9(2):135–44. doi: 10.1007/s12265-016-9683-3
Keywords: cardiovascular disease, Innate immunity, trained immunity, endothelial cell, innate immune cell
Citation: Xu K, Zhang Y, Saaoud F, Shao Y, Lu Y, Jiang X, Wang H and Yang X (2023) Editorial: Insights in cardiovascular therapeutics 2022—cardiovascular innate immunity. Front. Cardiovasc. Med. 10:1184030. doi: 10.3389/fcvm.2023.1184030
Received: 10 March 2023; Accepted: 6 April 2023;
Published: 18 April 2023.
Edited by:
Hong S. Lu, University of Kentucky, United States© 2023 Xu, Zhang, Saaoud, Shao, Lu, Jiang, Wang and Yang. This is an open-access article distributed under the terms of the Creative Commons Attribution License (CC BY). The use, distribution or reproduction in other forums is permitted, provided the original author(s) and the copyright owner(s) are credited and that the original publication in this journal is cited, in accordance with accepted academic practice. No use, distribution or reproduction is permitted which does not comply with these terms.
*Correspondence: Xiaofeng Yang eGlhby1mZW5nLnlhbmdAdGVtcGxlLmVkdQ==
Disclaimer: All claims expressed in this article are solely those of the authors and do not necessarily represent those of their affiliated organizations, or those of the publisher, the editors and the reviewers. Any product that may be evaluated in this article or claim that may be made by its manufacturer is not guaranteed or endorsed by the publisher.
Research integrity at Frontiers
Learn more about the work of our research integrity team to safeguard the quality of each article we publish.