- 1Cardiovascular Research Center, IRCCS San Raffaele Scientific Institute, Milano, Italy
- 2Università Vita Salute San Raffaele, Milano, Italy
- 3Cardiac Intensive Care Unit, IRCCS San Raffaele Scientific Institute, Milano, Italy
- 4Department of Anesthesia and Intensive Care, IRCCS San Raffaele Scientific Institute, Milano, Italy
Introduction: MicroRNAs (miRs) emerged as promising diagnostic and therapeutic biomarkers in cardiovascular diseases. The potential clinical utility of platelet miRs in the setting of left ventricular assist device (LVAD) support is unexplored.
Methods: We prospectively measured the expression levels of 12 platelet miRs involved in platelet activation, coagulation, and cardiovascular diseases in LVAD patients by quantitative real-time polymerase chain reaction. Data were longitudinally measured before LVAD implant and after 1, 6, and 12 months of LVAD support, and compared with those measured in healthy volunteers (controls). In silico analysis was also performed to identify pathways targeted by differentially expressed miRs.
Results: Data from 15 consecutive patients and 5 controls were analyzed. Pre-implant expression levels of platelet miR-126, miR-374b, miR-223, and miR-320a were significantly different in patients vs. controls. The expression levels of platelet miR-25, miR-144, miR-320, and miR-451a changed significantly over the course of LVAD support; in silico analysis revealed that these miRs are implicated in both cardiac- and coagulation-associated pathways. Furthermore, the patients who suffered from bleeding (n = 5, 33%) had significantly higher pre-implant expression levels of platelet miR-151a and miR-454 with respect to the patients who did not. The same miRs were also differentially expressed in bleeders following LVAD implantation early before the clinical manifestation of the events.
Discussion: This study provides a proof-of-concept evidence of significant modulation of platelet miRs expression driven by LVADs. The possible existence of a platelet miRs signature predictive of the development of bleeding events warrants further validation studies.
1. Introduction
Left ventricular assist device (LVAD) therapy improves the functional status and quality of life of patients with advanced heart failure (HF), and long-term survival with modern LVADs continues to increase (1–3). However, hemocompatibility-related adverse events (HRAEs), i.e., bleeding and thromboembolic complications, are frequent in LVAD patients (2, 3).
HRAEs are the result of a progressive change in the coagulation profile of the patients toward a pro-thrombotic/pro-hemorrhagic state driven by different synergic contributory mechanisms (4–14). Nevertheless, the lack of association between HRAEs and standard coagulation parameters highlights a critical gap in their diagnostic and therapeutic pathway. In this scenario, standardized and reliable criteria to stratify LVAD patients according to thrombotic or hemorrhagic risk are missing, and to define a patient-tailored strategy to prevent HRAEs remains a challenge. In particular, tools and biomarkers that are able to predict the derangement of platelet function toward a pro-thrombotic or a pro-hemorrhagic profile are not available.
This clinical need could be potentially met by circulating microRNAs (miRs), tiny non-coding RNAs with regulatory function on multiple target genes, which recently emerged as disease-associated biomarkers in cardiovascular diseases, including HF (15–17).
To date, the investigation on miRs in patients with LVADs is in its infancy, and studies on plasma or cardiac tissue provided heterogeneous data on their predictive potential and relationship with myocardial or vascular remodeling (18–22).
Previous studies highlighted that platelets possess a miR repertoire and processing machinery related to hypo/hyper-reactivity (23), and that miR target genes could be de-repressed upon platelet activation, affecting platelet function both at gene and protein levels in individually variable manner (24). To date, the relation between platelet miRs expression in LVAD patients and occurrence of HRAEs is unexplored.
On this background, we analyzed, for the first time, longitudinal changes in the levels of expression of platelet miRs in the setting of LVAD support. The present study provides a proof-of-concept of the variation of platelet miRs in the setting of durable LVAD support.
2. Material and methods
This is a prospective observational study conducted in consecutive patients with advanced HF according to the 2022 American College of Cardiology/American Heart Association/Heart Failure Society of America guideline for the management of heart failure (25) who were listed for LVAD implantation between February 2019 and December 2020 at San Raffaele Scientific Institute (Milano, Italy). The study conforms to the ethical guidelines of the Declaration of Helsinki and was approved by the local IRB (protocol ID: PASVAD, approved: June 2017; ClinicalTrials.gov ID: NCT03255928). All patients signed informed consent to participate in the study.
The expression levels of a panel of 12 platelet miRs (Table 1) were longitudinally measured pre-implant (t0, i.e., between 24 and 48 h before the device implant), and following 1 (t1), 6 (t2), and 12 (t3) months of LVAD support. The miRs expression levels were measured in both platelet-rich plasma (PRP) and platelet-poor plasma (PPP) pellet samples by quantitative real-time polymerase chain reaction (RT-qPCR), as described in the Supplementary Methods, and compared with those measured in n = 5 healthy volunteers matched for age and sex (controls). MiR values measured in PRP were normalized against the platelet count in each blood sample.
The 12 miRs we analyzed were selected according to previous evidence of their involvement in coagulation, platelet activation, and cardiovascular disease (Supplementary Table S1).
The pre-implant demographics and clinical characteristics of the patients, changes in the clinical data over the course of support, and clinical outcomes, including HRAEs (non-surgical bleeding and thromboembolic complications) were also recorded. The INTERMACS definitions for adverse events were applied (26). Data were retrieved at the longest available follow-up.
2.1. Statistical analysis
Categorical data are presented as absolute numbers and percentages and were compared by two-tailed X2 test or Fisher’s exact test. Numerical data are presented as medians and interquartile range (IQR: 25th–75th percentiles). The Shapiro–Wilk normality test was applied to assess the normality of data distribution. Comparison between groups was performed throughout the Student’s t-test or the Mann–Whitney U-test for normally and non-normally distributed data, respectively.
Analysis of longitudinal data (t0 to t3) was performed with the ANOVA test for correlated samples or the Friedman test for normally and non-normally distributed data, respectively using the linear mixed effects model [restricted maximum likelihood estimation (REML) method]: the model accounts for both fixed effects (different time-points) and random effects (different observations within a patient); Geisser–Greenhouse correction was applied. Tukey post-hoc test was applied to evaluate differences between different time-points.
Correlation among clinical and experimental variables was analyzed by Spearman-R test. Simple linear regression or non-linear fit test was applied as opportune. Probability values <0.05 were considered significant. Statistical analysis was performed with GraphPad PRISM v.8.2.0 (GraphPad Software, San Diego, CA, United States).
3. Results
3.1. Patient characteristics, clinical outcomes, and occurrence of HRAEs
A total of 18 patients were enrolled. One patient (5%) died before LVAD implantation and two (11%) during early post-operative hospital stay and were excluded. Data of the remaining 15 patients were analyzed. The pre-implant demographic and clinical characteristics of the patients are presented in Table 2 together with descriptive characteristics of the controls.
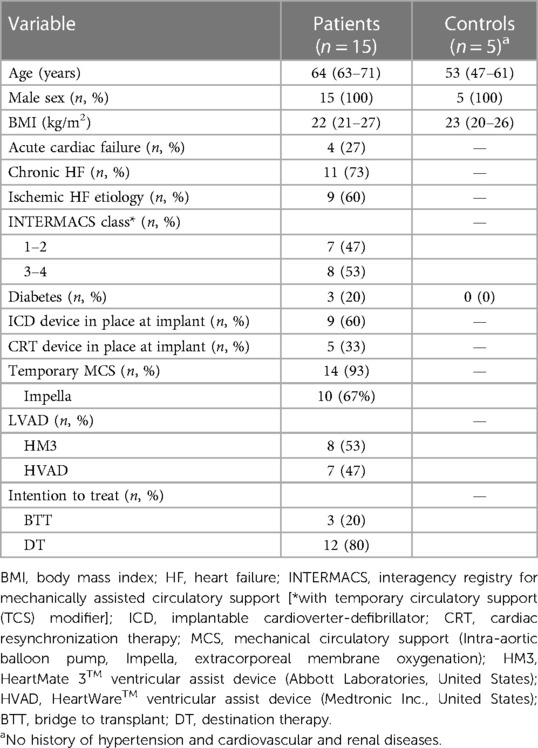
Table 2. Pre-implant demographic and clinical characteristics of the patients and descriptive characteristics of the controls.
The patients were treated according to guideline-directed medical therapy for HF (25). All patients were alive on LVAD support at the longest follow-up. The median time of follow-up at t1, t2, and t3 was 66 (40–90), 210 (184–223), and 480 (416–512) days, respectively. The changes in the clinical data of the patients over the course of LVAD support are reported in Table 3.
We recorded 8 HRAEs: 7 bleeding events in 5 (33%) patients and 1 thromboembolic event (7% of the patients). The details on the type of bleeding and thromboembolic events are reported in Table 3. Two bleeding events (40%) occurred in patients implanted with the HeartWare HVAD (Medtronic Inc., United States) and three (60%) in those implanted with the HeartMate 3 (HM3; Abbott, United States). The patient who developed a thromboembolic event was implanted with the HVAD. At the time of the event, all patients were treated with oral anticoagulant (warfarin) targeted to an international normalized ratio (INR) of 2–2.5; the patients implanted with the HVAD were also on aspirin (300 mg/die). The median duration of LVAD support at the time of a primary HRAE was 175 (144–331) days.
3.2. Pre-implant platelet miRs expression levels
Six out of 12 miRs were differently expressed (DEmiRs; p < 0.05) in patients before LVAD implantation vs. controls: (i) miR-126 and miR-374b in both PRP and PPP, (ii) miR-223 and miR-320a in PRP, and (iii) miR-20b and miR-451 in PPP (Figure 1). Moreover, consistent with the PRP pattern, differences in the expression levels of miR-223 and miR-320a in PPP reached borderline significance (p = 0.053 and p = 0.055, respectively).
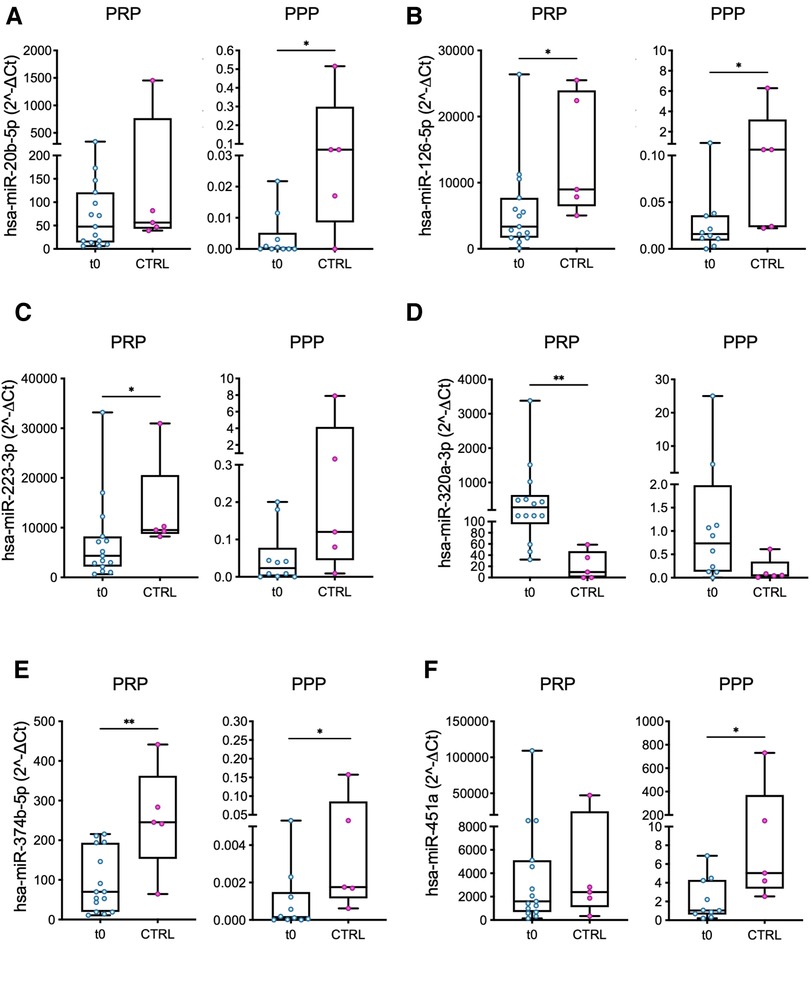
Figure 1. Comparison of miRs expression levels in PRP and PPP between LVAD patients at baseline (t0) and healthy volunteers (controls, CTRL). The miRs with a significant different level of expression between the two groups are shown: (A) miR-20b; (B) miR-126; (C) miR-223; (D) miR-320a; (E) miR-374b; and (F) miR-451a. Values are presented as boxes: dots indicate single values; whisker bars indicate min and max. *p < 0.05; **p < 0.01.
In all but one case (miR-320a), the median values of miRs expression level were lower in patients vs. controls (Figure 1).
The miRs expression levels were higher in PRP with respect to PPP in all the samples (Figure 1), highlighting the actual expression of miRs by platelets and the existence of a minor—though detectable—miR circulating fraction in PPP potentially generated by activated platelets and carried by lipoproteins of bear by microvesicles (27, 28).
In silico analysis revealed that 4 out of 6 DEmiRs, i.e., miR-20b, miR-223, miR-320a, and miR-374b, share a common target gene: the sodium voltage-gated channel alpha subunit 1 (SCN1A). No shared targets were found for miR-126 and miR-451a and the other DEmiRs. (Supplementary File S1).
No significant differences were found between patients and controls for miR-144, miR-151a, miR-19b, miR-25, miR-382, and miR-454 either in PRP or PPP (Supplementary Figure S1).
Subgroup analysis in the patients revealed significant differences in the miRs expression levels according to either patients’ characteristics (ischemic vs. non-ischemic etiology of HF and chronic vs. acute cardiac failure, Figures 2A–C) or treatment received before LVAD implant (Impella device, Figure 2D).
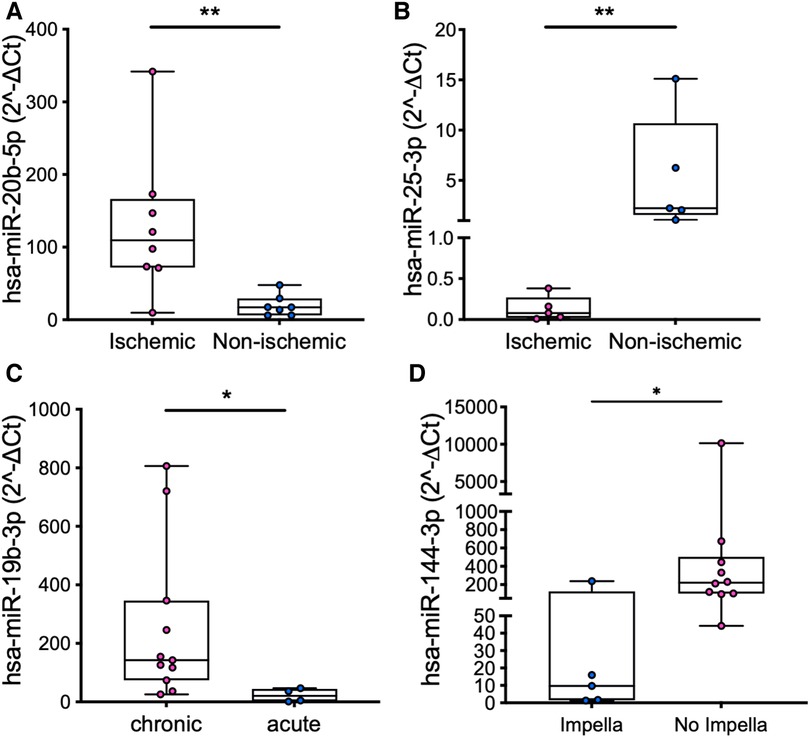
Figure 2. Comparison of the expression levels of miR-20b in PRP (A) and miR-25 in PPP (B) in patients with ischemic vs. non-ischemic etiology of HF, miR-19b in PRP in patients with chronic vs. acute cardiac failure (C), and miR-144 in PRP in patients who were vs. were not treated with an Impella device (D). Values are presented as boxes: dots indicate single values; whisker bars indicate min and max. *p < 0.05; **p < 0.01.
3.3. Changes in platelet miRs expression over the course of LVAD support
The expression levels of four miRs measured in PRP changed significantly from t0 to t3: miR-25, miR-451a, miR-320a, and miR-144, (Figure 3). The differences become significant in the long-term (i.e., at t2 or t3).
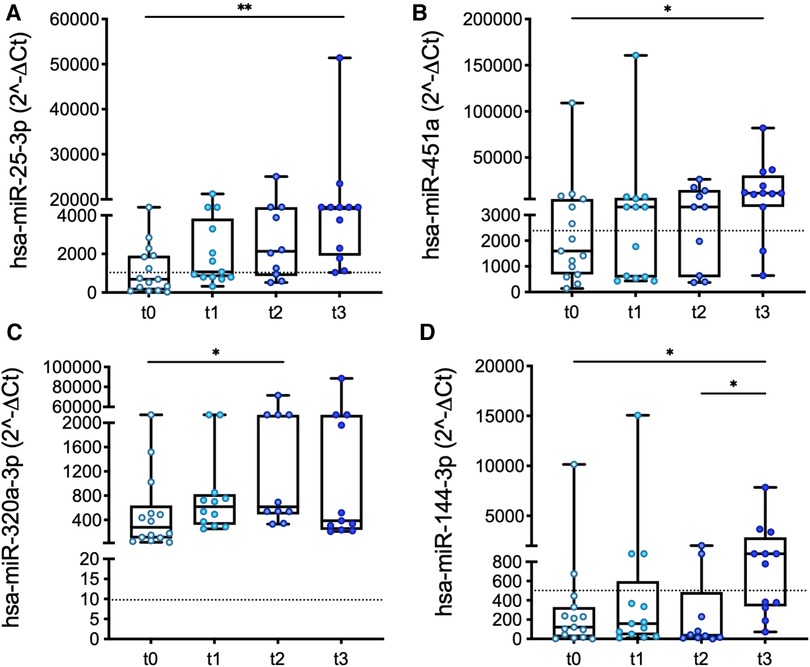
Figure 3. Longitudinal changes in the expression levels of miR-25 (A), miR-451a (B), miR-320a (C), and miR-144 (D) measured in PRP over the course of LVAD support. Values are presented as boxes: dots indicate single values; whisker bars indicate min and max. Dashed lines indicate the median value of expression in controls. *p < 0.05.
Interestingly, significant differences (miR-320a; Figure 1) and/or similarities (miR-25, miR-451a, and miR-144; Figure 1 and Supplementary Figure S1) determined at baseline in patients vs. controls were confirmed at the longest follow-up, except for platelet miR-25, whose expression levels in patients vs. controls were comparable at t0 but then progressively increased in the patients (Figure 3A).
The expression level of miR-126 in PRP—but not of other miRs—showed a good correlation at t3 with D-Dimer and AT-III levels (Figures 4A,B) as well as with metrics of left ventricular function (left ventricular ejection fraction, LVEF; Figure 4C).
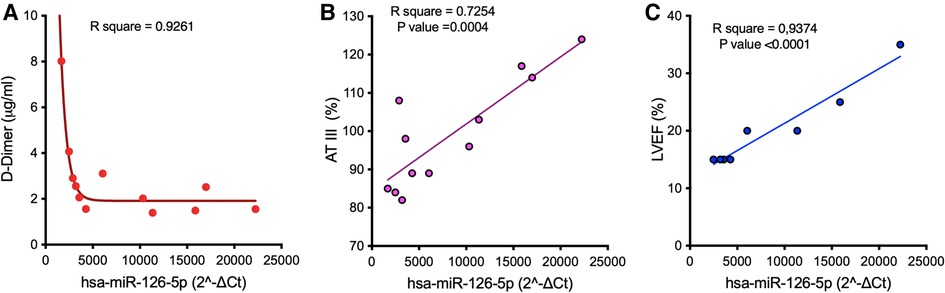
Figure 4. Correlation between the median expression of miR-126 in PRP in samples harvested at t3 from LVAD patients and D-Dimer (A) and AT-III (B) levels, and metrics of left ventricular function (C). AT-III, antithrombin III; LVEF, left ventricular ejection fraction.
No further correlations were found between platelet miRs and other clinical parameters over the course of LVAD support.
In silico analysis failed to show genes shared by the four DEmiRs whereas, after excluding miR-451a, the other three miRs have 13 common target genes, mainly involved in metabolic and oxygen-related cell responses (Supplementary File S1, Figure S2). Of the targeted signaling pathways shared by the four DEmiRs, 36 were of potential relevance in cardiac pathogenesis (Supplementary File S2).
No significant longitudinal changes were found in PPP for all the analyzed miRs.
3.4. Platelet miRs expression and HRAEs
The low number of thromboembolic events (n = 1) hampered a formal correlation analysis of platelet miRs expression and this category of HRAEs. Conversely, the expression levels of platelet miRs were compared in patients who did suffer from bleeding events (n = 5) vs. those who did not develop any HRAE (n = 9).
Pre-implant expression levels of miR-151a and mir-454 in PRP were higher in patients who suffered from a bleeding event (Figure 5; miR-151a: p = 0.04; miR-454: p = 0.03).
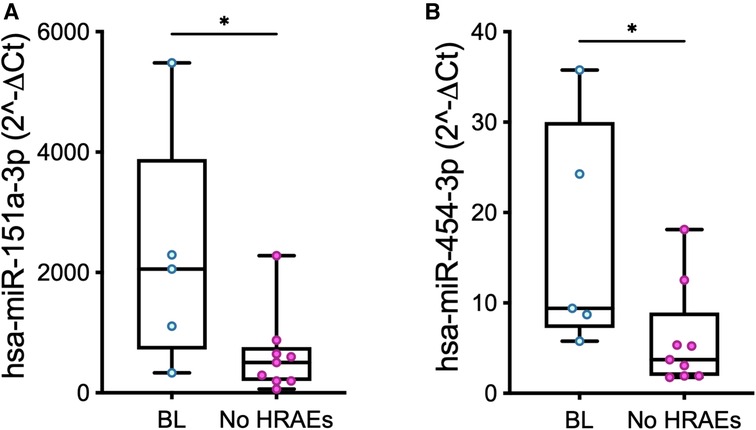
Figure 5. Baseline (t0) expression levels of miR-151a (A) and miR-454 (B) measured in PRP in patients who developed a bleeding event over the course of LVAD support (BL, n = 5) vs. those who did not suffer from HRAEs (no HRAEs, n = 10). Values are presented as boxes: dots indicate single values; whisker bars indicate min and max. *p < 0.05.
In silico analysis showed different pathways involved in cardiac physiopathology targeted by miR-151a (n = 79) or miR-454 (n = 108), including one associated with the complement cascade; some of them (n = 58) appeared shared by both miRs (Supplementary File S3).
An altered expression of the same miRs was also recorded in bleeders early before the occurrence of a bleeding event (Figure 6). Specifically, the median values of miR-151a were 7.8-fold lower at t1 (Figure 6A; p = 0.03) and 5.46-fold higher at t2 (Figure 6B) in bleeders vs. patients who did not suffer from HRAEs; the median values of miR-454 were 1.94-fold and >800-fold lower at t1 (Figure 6C) and t2 (Figure 6D), respectively, in bleeders vs. HRAEs-free patients.
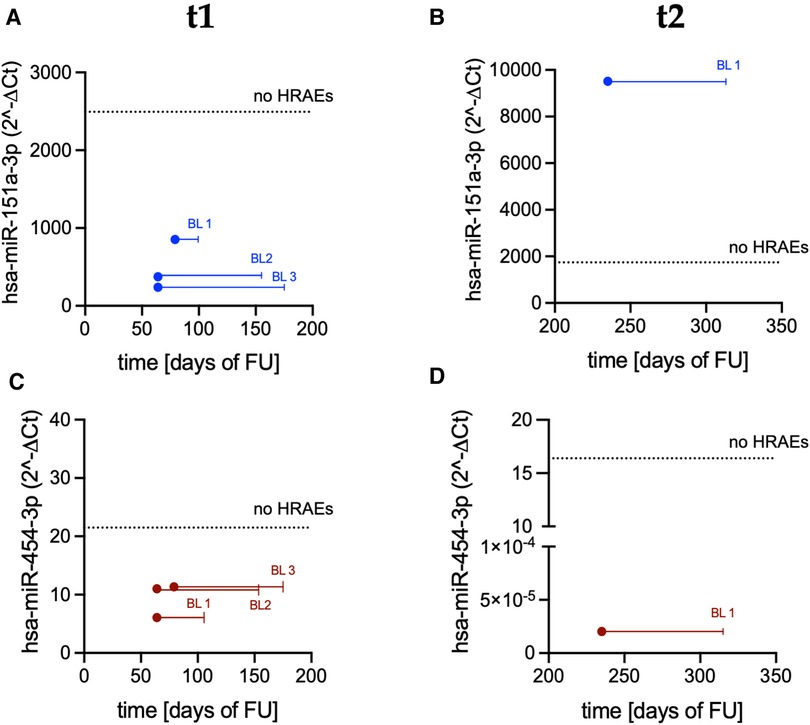
Figure 6. Expression levels of miR-151a and miR-454 measured in PRP before the occurrence of a bleeding event: (A) miR-151a at t1; (B) miR-151a at t2; (C) miR-454 at t1; and (D) miR-454 at t2. Dots indicate miRs expression level in bleeders (BL; t1: n = 3; t2: n = 1). Solid lines indicate temporal distance from the miR measurements to the occurrence of the bleeding event [t1: measurements performed 91 days (median value) before the occurrence of the events; t2: measurements performed 80 days before the occurrence of the event]. Dashed lines indicate the median value of miRs expression in patients who did not suffer from HRAEs (no HRAEs; t1: n = 8; t2: n = 7).
The miRs expression levels at t3 were not analyzed as no data anticipating the events were available (i.e., the events occurred before the scheduled time-point for blood sampling and miR analysis).
4. Discussion
In this proof-of-concept study, we evaluated, for the first time, the expression levels of platelet miRs in the setting of durable LVAD support. Previous works analyzed circulating/tissue miRs in LVAD patients (18–22), but whether changes in platelet miR patterns occur after LVAD implantation remained unexplored.
Our preliminary results suggest a modulatory effect of LVADs upon the platelet miRs processing machinery: this is consistent with the longitudinal changes in the expression levels of four platelet miRs found in PRP over the course of LVAD support (Figure 3).
4.1. Pre-implant DEmiRs: the role of HF and temporary mechanical circulatory support
Baseline miRs differences in patients vs. controls (Figure 1) are likely due to the pathophysiology of advanced HF and targeted medical therapy and are in agreement with previous studies on platelet miRs expression in HF (29–35). In silico analysis highlights a common target gene shared by the four DEmiRs, i.e., the SCN1A, which is known to encode for a tetrodotoxin-sensitive cardiac voltage-gated sodium channel (36). Whether SCN1A is a possible pathogenic player in advanced HF and might have any functional role during LVAD support deserves further dedicated investigation.
Our data also confirm good specificity of miRs to differentiate HF patients according to clinical characteristics (Figures 2A–C) (37–39) and provide preliminary evidence of a distinct platelet miR pattern correlated with pre-implant temporary mechanical circulatory support devices (Figure 2D). These observations, together with their correlation with the clinical course of the patients, deserve further investigation since they may have strong implications for clinical practice, namely, to guide patient-tailored management strategies. Indeed, we can argue that hemodynamic phenomena characteristics of the Impella device (e.g., cardiac unloading, shear stress, blood flow patterns) may have a contributory role in modulating platelet miRs expression, in addition to HF itself.
4.2. Changes in platelet miRs expression driven by LVAD support: recovery of HF symptoms, platelet activation, and structural changes of the supported heart
Following LVAD implantation, interestingly, we observed that pre-implant differences in patients vs. controls did not normalize, i.e., values in patients did not recover to the median control values over the course of LVAD support (Figure 3), suggesting that LVADs prompt a distinctive, LVAD-specific, and miR-related platelet “phenotype.”
These data indicate that after LVAD implantation, the reverse of miRs expression do not match the reverse of signs and symptoms of HF (Table 3) and suggest that platelet miRs may respond to a complex pattern of stimuli beyond HF symptoms. These might include systemic alterations common to LVAD patients (changes in macro- and micro-circulation or coagulation patterns) as well as the effect of medical therapy and mechanical stimuli (shear forces) provided by the device. Interestingly, no differences were observed in the expression levels of platelet miRs between patients implanted with the HVAD or the HM3 (data not shown).
According to previous works on platelet miRs expression, we speculate that our data mirror platelet function abnormalities largely described in LVAD patients [chronic activation/hyper-reactive status, and degranulation and impaired adhesion and aggregation capability (4, 8, 9, 40–43)]. In detail, the progressive increase in the expression levels of platelet miR-25 and miR-451a from t0 to t3 (Figures 3A,B) might indicate an increase in platelet activation over the course of LVAD support (44). Conversely, the changes in the expression of miR-320a and miR-144, two recognized regulators of platelet reactivity, activation, signaling, and aggregation, as well as degranulation (45), did not delineate a pattern over time (Figures 3C,D), which might reflect partial and/or transient activation of platelets and deserve further investigation. We also hypothesize that lack of a clear pattern of expression over time might reflect a continuous “adaptation” of platelet miRs expression to the continuously evolving clinical features of the patients: as discussed earlier, platelet miRs appear to respond indeed to a complex and heterogenous pattern of stimuli. Studies evaluating further changes in platelet miRs expression over longer periods of LVAD support might contribute to clarify these phenomena.
The four DEmiRs shared molecular pathways including that of peroxisome proliferator activated receptor (PPAR), a regulator of platelet activation (46) and TNF-α and mammalian target of rapamycin (mTOR), which have been found to be involved in bleeding (47–50).
Our hypothesis of a dynamic re-programming of platelet miRs toward a low pro-thrombotic (rather pro-hemorrhagic) state over the course of support is corroborated by the correlation between platelet miR-126 and D-Dimer and AT-III plasma levels: indeed, the observed negative (D-Dimer, Figure 4A) and positive (AT-III, Figure 4B) relations with miR-126 might be compatible with lack of pro-thrombotic activation and retention of mature miR-126 into platelets (51). In this regard, it is worth noting that only one patient in our cohort developed a thromboembolic complication, while five (33%) suffered from bleeding. Nevertheless, whether the increase of miR content in platelets (retention) effectively reflects low level of activation or preludes the release of pro-thrombotic miRs needs to be clarified in dedicated mechanistic studies.
According to described mechanisms of genomic, molecular, cellular, and structural changes of the LVAD-unloaded left ventricle (52), we also examined possible correlations between the changes in platelet miRs expression and changes in cardiac function driven by LVAD support. In silico analysis showed different signaling pathways shared by the four DEmiRs having potential relevance in cardiac pathogenesis (Supplementary File S3). Furthermore, the positive correlation found at t3 between platelet miR-126 and LVEF (Figure 4C) confirms the prognostic role of this miR in cardiovascular disease (53), and further supports its association with cardiac function.
4.3. Changes in platelet miRs expression and adverse events
Our study also suggests that abnormal expression levels of platelet miRs might represent novel biomarkers predictive of bleeding events in the LVAD population.
To date, prognostic biomarkers of bleeding relevant to platelet function abnormalities in LVAD patients are scarce. Previous studies demonstrated that acquired platelet dysfunction, secretion defects, and impaired aggregation capability contribute to the development of bleeding in LVAD patients (9, 54–57). Recently, reduced pre-implant expression of platelet P-selectin and GPIIb/IIIa has been proposed to predict bleeding while on LVAD (58).
The pre-implant expression levels of platelet miRs-151a and miR-454 allowed to stratify the subgroup of patients who suffered from bleeding with respect to patients who did not develop HRAEs (Figure 5). Indeed, we reasoned that thromboembolic and bleeding events might be associated with different miR patterns: accordingly, to avoid bias and enhance selective focus on bleeding events, data of the patient who suffered from stroke were excluded.
Our data suggest the existence of a platelet miR-related fingerprint of bleeders, i.e., the retention of miR-151a—classified as thrombomiR—and miR-454—known to be involved in the regulation of the complement cascade (59, 60). On the other hand, the potential targets of these miRs (inside vs. outside platelets) were not identified. In other words, we did not investigate whether a change in the levels of expression of these miRs effectively influences platelet function or activation or whether platelets are only acting as carriers for the miR. Accordingly, we are not proposing altered levels of expression of miRs by platelets to be at the origin of the bleeding event, rather suggesting that the platelet miRs represent novel markers of a pathogenic state (increased risk of bleeding) in some patients. Future mechanistic studies on the correlation between the expression levels of platelet miRs and traditional markers of platelet function/activation (e.g., P-selectin; CD40l and/or PF4), and on the mutual contribution of altered miR patterns and other recognized risk factors to the development of HRAEs are needed to elucidate these phenomena and provide further insights into pathophysiology of LVAD-related complications. As far as potential age-related inferences on altered expression levels of platelet miRs in bleeders is concerned, of note, age at implant was not statistically different in the two groups (bleeders vs. non-HRAEs patients).
On the other hand, no significant differences were found in the expression levels of platelet miR-151-a and miR-454 between bleeders and controls (p = 0.56 and p = 0.75, respectively): these data highlight the need for further analyses aimed at validating a possible contributory role of platelet miRs profile to predispose LVAD patients to HRAEs.
Differences in the expression levels of miR-151a and miR-451 were also observed in bleeders early before the clinical manifestation of the event (Figure 6), further corroborating their prognostic power and the clinical utility of monitoring platelet miRs in LVAD patients. Conversely, coagulation parameters were comparable in bleeders vs. HRAEs-free patients (data not shown) and did not warn for an increased risk of bleeding.
Downregulation of platelet miR-151a in bleeders at t1 possibly indicates abundance of hypo-reactive platelets with inhibited aggregation capability (61, 62). However, the patient who suffered from bleeding at t2 showed an upregulation of mir-151a levels anticipating the event (Figure 6B). This apparent discrepancy might be explained by the different types of bleeding (Table 3) or a modulatory effect of systemic infection, as driveline infection was concomitant in 3 out of the 7 bleeding events (43%). Moreover, we cannot exclude a contribution by other factors (e.g., adverse events not related to hemocompatibility or peculiar conditions and management strategies at the time of the event)—alone or in concert—to different miR-151a expression. In this regard, we emphasize that durable LVAD support is (intrinsically) a multisystemic and dynamic scenario, where features that might amplify the risk of HRAEs and impact platelet miRs expression might be present at baseline or emerge later after LVAD implantation.
5. Study limitations
This is a single-center observational study performed in a small cohort of patients. Potential gender-related differences in platelet miRs expressions were not evaluated. Future studies on a larger, multi-center cohort are therefore warranted to increase the power and significance of our results. In particular, studies on a larger number of patients are required to validate differences in miR patterns associated with different devices. Furthermore, the cut-off miRs expression levels that are predictive of HRAEs were not identified. Moreover, the functional role and clinical implications of some DEmiRs in the specific scenario of LVAD support were not fully elucidated, nor the associated downstream signaling pathways validated. Also, we did not investigate possible association and/or cross-talks between altered miRs expression and different pro-thrombotic/pro-hemorrhagic risk factors in the development of HRAEs, including acquired von Willebrand syndrome and platelet activation levels, nor inferences of changes in anticoagulation/antiplatelet therapy over the course of support.
6. Conclusions
We provide a proof-of-concept evidence of significant modulation of platelet miRs expression driven by durable LVADs and suggest a potential of platelet miRs signature to identify patients at high risk for HRAEs, specifically bleeding. Indeed, we propose two miRs candidates, miR-151a and miR-454, deserving further evaluation for future clinical use. Thus, our study might have important translational relevance related to novel strategies for the prevention of HRAEs targeted to platelet miRs expression, which might overcome evident limitations of available coagulation markers, prediction algorithms, and point-of-care coagulation tests. Future studies to validate our preliminary findings are warranted.
Data availability statement
The raw data supporting the conclusions of this article will be made available by the corresponding authors upon reasonable request.
Ethics statement
The studies involving human participants were reviewed and approved by IRB of the IRCCS San Raffaele Scientific Institute. The patients/participants provided their written informed consent to participate in this study.
Author contributions
Conceptualization: ML, CF, and FC; methodology: ML, CF, and FC; validation: ML, CF, and FC; formal analysis: ML, MB, LB, MP, CF, and FC; investigation: ML, MB, LB, MP, CF, and FC; resources: AS, GL, AZ, CF, and FC; data curation: ML, MB, LB, MP, CF, and FC; writing—original draft preparation: ML, CF, and FC; writing—review and editing: ML, LB, MP, AS, GL, CF, and FC; supervision: AS, AZ, CF, and FC; funding acquisition: GL, AZ, and CF. All authors contributed to the article and approved the submitted version.
Conflict of interest
The authors declare that the research was conducted in the absence of any commercial or financial relationships that could be construed as a potential conflict of interest.
Publisher's note
All claims expressed in this article are solely those of the authors and do not necessarily represent those of their affiliated organizations, or those of the publisher, the editors and the reviewers. Any product that may be evaluated in this article, or claim that may be made by its manufacturer, is not guaranteed or endorsed by the publisher.
Supplementary material
The Supplementary Material for this article can be found online at: https://www.frontiersin.org/articles/10.3389/fcvm.2023.1178556/full#supplementary-material
References
1. Molina EJ, Shah P, Kiernan MS, Cornwell WK 3rd, Copeland H, Takeda K, et al. The Society of Thoracic Surgeons Intermacs 2020 annual report. Ann Thorac Surg. (2021) 111(3):778–92. doi: 10.1016/j.athoracsur.2020.12.038
2. Zimpfer D, Fiane AE, Larbalestier R, Tsui S, Jansz P, Simon A, et al. Long-term survival of patients with advanced heart failure receiving an left ventricular assist device intended as a bridge to transplantation: the registry to evaluate the HeartWare left ventricular assist system. Circ Heart Fail. (2020) 13(3):e006252. doi: 10.1161/CIRCHEARTFAILURE.119.006252
3. Mehra MR, Goldstein DJ, Cleveland JC, Cowger JA, Hall S, Salerno CT, et al. Five-year outcomes in patients with fully magnetically levitated vs. axial-flow left ventricular assist devices in the momentum 3 randomized trial. JAMA. (2022) 328(12):1233–42. doi: 10.1001/jama.2022.16197
4. de Biasi AR, Manning KB, Salemi A. Science for surgeons: understanding pump thrombogenesis in continuous-flow left ventricular assist devices. J Thorac Cardiovasc Surg. (2059) 149(3):667–73. doi: 10.1016/j.jtcvs.2014.11.041
5. Hennessy-Strahs S, Kang J, Krause E, Dowling RD, Rame JE, Bartoli CR. Patient-specific severity of von Willebrand factor degradation identifies patients with a left ventricular assist device at high risk for bleeding. J Thorac Cardiovasc Surg. (2022) S0022-5223(22):00360-9. doi: 10.1016/j.jtcvs.2022.03.018
6. Patel SR, Madan S, Saeed O, Algodi M, Luke A, Gibber M, et al. Association of nasal mucosal vascular alterations, gastrointestinal arteriovenous malformations, and bleeding in patients with continuous-flow left ventricular assist devices. JACC Heart Fail. (2016) 4(12):962–70. doi: 10.1016/j.jchf.2016.08.005
7. Houston BA, Schneider AL, Vaishnav J, Cromwell DM, Miller PE, Faridi KF, et al. Angiotensin II antagonism is associated with reduced risk for gastrointestinal bleeding caused by arteriovenous malformations in patients with left ventricular assist devices. J Heart Lung Transplant. (2017) 36(4):380–5. doi: 10.1016/j.healun.2016.12.016
8. Muslem R, Caliskan K, Leebeek FWG. Acquired coagulopathy in patients with left ventricular assist devices. J Thromb Haemost. (2018) 16(3):429–40. doi: 10.1111/jth.13933
9. Roka-Moiia Y, Miller-Gutierrez S, Palomares DE, Italiano JE, Sheriff J, Bluestein D, et al. Platelet dysfunction during mechanical circulatory support: elevated shear stress promotes downregulation of αIIbβ3 and GPIb via microparticle shedding decreasing platelet aggregability. Arterioscler Thromb Vasc Biol. (2021) 41(4):1319–36. doi: 10.1161/ATVBAHA.120.315583
10. Selmi M, Chiu WC, Chivukula VK, Melisurgo G, Beckman JA, Mahr C, et al. Blood damage in left ventricular assist devices: pump thrombosis or system thrombosis? Int J Artif Organs. (2019) 42(3):113–24. doi: 10.1177/0391398818806162
11. Consolo F, Raimondi Lucchetti M, Tramontin C, Lapenna E, Pappalardo F. Do we need aspirin in HeartMate 3 patients? Eur J Heart Fail. (2019) 21(6):815–7. doi: 10.1002/ejhf.1468
12. Consolo F, Pozzi L, Pieri M, Della Valle P, Redaelli A, D'Angelo A, et al. Influence of different antithrombotic regimens on platelet-mediated thrombin generation in patients with left ventricular assist devices. ASAIO J. (2020) 66(4):415–22. doi: 10.1097/MAT.0000000000001064
13. Consolo F, Marasi A, Della Valle P, Bonora M, Pieri M, Scandroglio AM, et al. Bleeding in patients with continuous-flow left ventricular assist devices: acquired von Willebrand disease or antithrombotics? Eur J Cardiothorac Surg (2022) 62(1):ezab474. doi: 10.1093/ejcts/ezab474
14. Consolo F, Pappalardo F. New antithrombotic strategies to improve outcomes with the HeartMate 3. ASAIO J. (2023) 69(1):e3–6. doi: 10.1097/MAT.0000000000001794
15. Pedersen OB, Grove EL, Kristensen SD, Nissen PH, Hvas AM. MicroRNA as biomarkers for platelet function and maturity in patients with cardiovascular disease. Thromb Haemost. (2022) 122(2):181–95. doi: 10.1055/s-0041-1730375
16. Landry P, Plante I, Ouellet DL, Perron MP, Rousseau G, Provost P. Existence of a microRNA pathway in anucleate platelets. Nat Struct Mol Biol. (2009) 16(9):961–6. doi: 10.1038/nsmb.1651
17. Vegter EL, van der Meer P, de Windt LJ, Pinto YM, Voors AA. MicroRNAs in heart failure: from biomarker to target for therapy. Eur J Heart Fail. (2016) 18(5):457–68. doi: 10.1002/ejhf.495
18. Wang T, O'Brien EC, Rogers JG, Jacoby DL, Chen ME, Testani JM, et al. Plasma levels of MicroRNA-155 are upregulated with long-term left ventricular assist device support. ASAIO J. (2017) 63(5):536–41. doi: 10.1097/MAT.0000000000000564
19. Dlouha D, Ivak P, Netuka I, Novakova S, Konarik M, Tucanova Z, et al. The effect of long-term left ventricular assist device support on flow-sensitive plasma microRNA levels. Int J Cardiol. (2021) 339:138–43. doi: 10.1016/j.ijcard.2021.06.050
20. Lok SI, de Jonge N, van Kuik J, van Geffen AJ, Huibers MM, van der Weide P, et al. MicroRNA expression in myocardial tissue and plasma of patients with end-stage heart failure during LVAD support: comparison of continuous and pulsatile devices. PLoS One. (2015) 10(10):e0136404. doi: 10.1371/journal.pone.0136404
21. Barsanti C, Trivella MG, D'Aurizio R, El Baroudi M, Baumgart M, Groth M, et al. Differential regulation of microRNAs in end-stage failing hearts is associated with left ventricular assist device unloading. Biomed Res Int. (2015) 2015:592512. doi: 10.1155/2015/592512
22. Muthiah K, Humphreys DT, Robson D, Dhital K, Spratt P, Jansz P, et al. Longitudinal structural, functional, and cellular myocardial alterations with chronic centrifugal continuous-flow left ventricular assist device support. J Heart Lung Transplant. (2017) 36(7):722–31. doi: 10.1016/j.healun.2016.05.017
23. Nagalla S, Shaw C, Kong X, Kondkar AA, Edelstein LC, Ma L, et al. Platelet microRNA-mRNA coexpression profiles correlate with platelet reactivity. Blood. (2011) 117(19):5189–97. doi: 10.1182/blood-2010-09-299719
24. Edelstein LC, Bray PF. MicroRNAs in platelet production and activation. Blood. (2011) 117(20):5289–96. doi: 10.1182/blood-2011-01-292011
25. Heidenreich PA, Bozkurt B, Aguilar D, Allen LA, Byun JJ, Colvin MM, et al. 2022 AHA/ACC/HFSA guideline for the management of heart failure: a report of the American College of Cardiology/American Heart Association Joint Committee on Clinical Practice Guidelines. Circulation. (2022) 145(18):e895–1032. doi: 10.1161/CIR.0000000000001063
26. STS INTERMACS database. Available at: https://www.uab.edu/medicine/intermacs/intermacs-documents (Accessed February 14, 2023).
27. Willeit P, Zampetaki A, Dudek K, Kaudewitz D, King A, Kirkby NS, et al. Circulating microRNAs as novel biomarkers for platelet activation. Circ Res. (2013) 112(4):595–600. doi: 10.1161/CIRCRESAHA.111.300539
28. Krammer TL, Mayr M, Hackl M. MicroRNAs as promising biomarkers of platelet activity in antiplatelet therapy monitoring. Int J Mol Sci. (2020) 21(10):3477. doi: 10.3390/ijms21103477
29. Zhang M, Jiang Y, Guo X, Zhang B, Wu J, Sun J, et al. Long non-coding RNA cardiac hypertrophy-associated regulator governs cardiac hypertrophy via regulating miR-20b and the downstream PTEN/AKT pathway. J Cell Mol Med. (2019) 23(11):7685–98. doi: 10.1111/jcmm.14641
30. Fukushima Y, Nakanishi M, Nonogi H, Goto Y, Iwai N. Assessment of plasma miRNAs in congestive heart failure. Circ J. (2011) 75(2):336–40. doi: 10.1253/circj.CJ-10-0457
31. Schneider SIDR, Silvello D, Martinelli NC, Garbin A, Biolo A, Clausell N, et al. Plasma levels of microRNA-21, -126 and -423-5p alter during clinical improvement and are associated with the prognosis of acute heart failure. Mol Med Rep. (2018) 17(3):4736–46. doi: 10.3892/mmr.2018.8428
32. Marfella R, Di Filippo C, Potenza N, Sardu C, Rizzo MR, Siniscalchi M, et al. Circulating microRNA changes in heart failure patients treated with cardiac resynchronization therapy: responders vs. non-responders. Eur J Heart Fail. (2013) 15(11):1277–88. doi: 10.1093/eurjhf/hft088
33. Wong L, Saw E, Lilyanna S, Martinez EC, Lam C, Richards AM. Circulating miR-503 and miR-374b-5p are associated with heart failure. Circulation. (2015) 132(3):A15090. doi: 10.1161/circ.132.suppl_3.15090
34. Zeng Z, Wang K, Li Y, Xia N, Nie S, Lv B, et al. Down-regulation of microRNA-451a facilitates the activation and proliferation of CD4+ T cells by targeting Myc in patients with dilated cardiomyopathy. J Biol Chem. (2017) 292(14):6004–13. doi: 10.1074/jbc.M116.765107
35. Goren Y, Kushnir M, Zafrir B, Tabak S, Lewis BS, Amir O. Serum levels of microRNAs in patients with heart failure. Eur J Heart Fail. (2012) 14(2):147–54. doi: 10.1093/eurjhf/hfr155
36. Maier SK, Westenbroek RE, Yamanushi TT, Dobrzynski H, Boyett MR, Catterall WA, et al. An unexpected requirement for brain-type sodium channels for control of heart rate in the mouse sinoatrial node. Proc Natl Acad Sci U S A. (2003) 100(6):3507–12. doi: 10.1073/pnas.2627986100
37. Keda S, Kong SW, Lu J, Bisping E, Zhang H, Allen PD, et al. Altered microRNA expression in human heart disease. Physiol Genomics. (2007) 31(3):367–73. doi: 10.1152/physiolgenomics.00144.2007
38. Gurha P. MicroRNAs in cardiovascular disease. Curr Opin Cardiol. (2016) 31(3):249–54. doi: 10.1097/HCO.0000000000000280
39. Su Y, Sun Y, Tang Y, Li H, Wang X, Pan X, et al. Circulating miR-19b-3p as a novel prognostic biomarker for acute heart failure. J Am Heart Assoc. (2021) 10(20):e022304. doi: 10.1161/JAHA.121.022304
40. Consolo F, Sferrazza G, Motolone G, Contri R, Valerio L, Lembo R, et al. Platelet activation is a preoperative risk factor for the development of thromboembolic complications in patients with continuous-flow left ventricular assist device. Eur J Heart Fail. (2018) 20(4):792–800. doi: 10.1002/ejhf.1113
41. Consolo F, Sferrazza G, Motolone G, Pieri M, De Bonis M, Zangrillo A, et al. Shear-mediated platelet activation enhances thrombotic complications in patients with LVADs and is reversed after heart transplantation. ASAIO J. (2019) 65(4):e33–5. doi: 10.1097/MAT.0000000000000842
42. Roka-Moiia Y, Walk R, Palomares DE, Ammann KR, Dimasi A, Italiano JE, et al. Platelet activation via shear stress exposure induces a differing pattern of biomarkers of activation versus biochemical agonists. Thromb Haemost. (2020) 120(5):776–92. doi: 10.1055/s-0040-1709524
43. Klaeske K, Dieterlen MT, Eifert S, Scholz U, Garbade J, Jawad K, et al. Device-induced platelet dysfunction in patients after left ventricular assist device implantation. J Thromb Haemost. (2021) 19(5):1331–41. doi: 10.1111/jth.15279
44. Maués JHDS, Aquino Moreira-Nunes CF, Rodriguez Burbano RM. MicroRNAs as a potential quality measurement tool of platelet concentrate stored in blood banks—a review. Cells. (2019) 8(10):1256. doi: 10.3390/cells8101256
45. Wicik Z, Czajka P, Eyileten C, Fitas A, Wolska M, Jakubik D, et al. The role of miRNAs in regulation of platelet activity and related diseases—a bioinformatic analysis. Platelets. (2022) 33(7):1052–64. doi: 10.1080/09537104.2022.2042233
46. Finck BN, Chinetti G, Staels B. PPARs/RXRs in cardiovascular physiology and disease. PPAR Res. (2008) 2008:173780. doi: 10.1155/2008/173780
47. Taylor H, Yerlioglu D, Phen C, Ballauff A, Nedelkopoulou N, Spier I. mTOR inhibitors reduce enteropathy, intestinal bleeding and colectomy rate in patients with juvenile polyposis of infancy with PTEN-BMPR1A deletion. Hum Mol Genet. (2021) 30(14):1273–82. doi: 10.1093/hmg/ddab094
48. Lomas-Neira J, Perl M, Venet F, Chung CS, Ayala A. The role and source of tumor necrosis factor-α in hemorrhage-induced priming for septic lung injury. Shock. (2012) 37(6):611–20. doi: 10.1097/SHK.0b013e318254fa6a
49. Qi J, Lv X, Chen J, Wang H, Chu T, Tang Y, et al. TNF-α increases the risk of bleeding in patients after CAR T-cell therapy: a bleeding model based on a real-world study of Chinese CAR T working party. Hematol Oncol. (2022) 40(1):63–71. doi: 10.1002/hon.2931
50. Spinelli SL, O'Brien JJ, Bancos S, Lehmann GM, Springer DL, Blumberg N, et al. The PPAR-platelet connection: modulators of inflammation and potential cardiovascular effects. PPAR Res. (2008) 2008:328172. doi: 10.1155/2008/328172
51. Zapilko V, Fish RJ, Garcia A, Reny JL, Dunoyer-Geindre S, Lecompte T, et al. MicroRNA-126 is a regulator of platelet-supported thrombin generation. Platelets. (2020) 31(6):746–55. doi: 10.1080/09537104.2020.1775804
52. Tseliou E, Lavine KJ, Wever-Pinzon O, Topkara VK, Meyns B, Adachi I, et al. Biology of myocardial recovery in advanced heart failure with long-term mechanical support. J Heart Lung Transplant. (2022) 41(10):1309–23. doi: 10.1016/j.healun.2022.07.007
53. Khanaghaei M, Tourkianvalashani F, Hekmatimoghaddam S, Ghasemi N, Rahaie M, Khorramshahi V, et al. Circulating miR-126 and miR-499 reflect progression of cardiovascular disease; correlations with uric acid and ejection fraction. Heart Int. (2016) 11(1):e1–9. doi: 10.5301/heartint.5000226
54. Steinlechner B, Dworschak M, Birkenberg B, Duris M, Zeidler P, Fischer H, et al. Platelet dysfunction in outpatients with left ventricular assist devices. Ann Thorac Surg. (2009) 87(1):131–7. doi: 10.1016/j.athoracsur.2008.10.027
55. Baghai M, Heilmann C, Beyersdorf F, Nakamura L, Geisen U, Olschewski M, et al. Platelet dysfunction and acquired von Willebrand syndrome in patients with left ventricular assist devices. Eur J Cardiothorac Surg. (2015) 48(3):421–7. doi: 10.1093/ejcts/ezu510
56. Gurvits GE, Fradkov E. Bleeding with the artificial heart: gastrointestinal hemorrhage in CF-LVAD patients. World J Gastroenterol. (2017) 23(22):3945–53. doi: 10.3748/wjg.v23.i22.3945
57. Geisen U, Brehm K, Trummer G, Berchtold-Herz M, Heilmann C, Beyersdorf F, et al. Platelet secretion defects and acquired von Willebrand syndrome in patients with ventricular assist devices. J Am Heart Assoc. (2018) 7(2):e006519. doi: 10.1161/JAHA.117.006519
58. Klaeske K, Meyer AL, Saeed D, Eifert S, Jawad K, Sieg F, et al. Decreased platelet specific receptor expression of P-selectin and GPIIb/IIIa predict future non-surgical bleeding in patients after left ventricular assist device implantation. Int J Mol Sci. (2022) 23(18):10252. doi: 10.3390/ijms231810252
59. Jankowska KI, Sauna ZE, Atreya CD. Role of microRNAs in hemophilia and thrombosis in humans. Int J Mol Sci. (2020) 21(10):3598. doi: 10.3390/ijms21103598
60. Nourse J, Braun J, Lackner K, Hüttelmaier S, Danckwardt S. Large-scale identification of functional microRNA targeting reveals cooperative regulation of the hemostatic system. J Thromb Haemost. (2018) 16(11):2233–45. doi: 10.1111/jth.14290
61. Krammer TL, Zeibig S, Schrottmaier WC, Pirabe A, Goebel S, Diendorfer AB, et al. Comprehensive characterization of platelet-enriched microRNAs as biomarkers of platelet activation. Cells. (2022) 11(8):1254. doi: 10.3390/cells11081254
Keywords: platelets, microRNA, left ventricular assist device, hemocompatibility, heart failure
Citation: Lombardi M, Bonora M, Baldetti L, Pieri M, Scandroglio AM, Landoni G, Zangrillo A, Foglieni C and Consolo F (2023) Left ventricular assist devices promote changes in the expression levels of platelet microRNAs. Front. Cardiovasc. Med. 10:1178556. doi: 10.3389/fcvm.2023.1178556
Received: 3 March 2023; Accepted: 16 May 2023;
Published: 15 June 2023.
Edited by:
Tobias Eckle, University of Colorado Anschutz Medical Campus, United States© 2023 Lombardi, Bonora, Baldetti, Pieri, Scandroglio, Landoni, Zangrillo, Foglieni and Consolo. This is an open-access article distributed under the terms of the Creative Commons Attribution License (CC BY). The use, distribution or reproduction in other forums is permitted, provided the original author(s) and the copyright owner(s) are credited and that the original publication in this journal is cited, in accordance with accepted academic practice. No use, distribution or reproduction is permitted which does not comply with these terms.
*Correspondence: Chiara Foglieni Zm9nbGllbmkuY2hpYXJhQGhzci5pdA== Filippo Consolo Y29uc29sby5maWxpcHBvQHVuaXNyLml0
†These authors have contributed equally to this work