- 1Postgraduate Program in Health Sciences, Federal University of Rio Grande do Norte, Natal, Brazil
- 2Division of Cardiology, Department of Medicine, UC San Diego, San Diego, CA, United States
- 3Department of Immunology, Institute of Biomedical Sciences, University of São Paulo, São Paulo, Brazil
- 4Department of Clinical Medicine, Center for Health Sciences, Federal University of Rio Grande do Norte, Natal, Brazil
- 5Department of Clinical and Toxicological Analyses, School of Pharmaceutical Sciences, University of Sao Paulo, São Paulo, Brazil
- 6Department of Cardiology, Boston Children’s Hospital/Harvard Medical School, Boston, MA, United States
- 7Division of Molecular Medicine, Department of Medicine, University of São Paulo School of Medicine, São Paulo, Brazil
- 8Laboratory of Medical Investigation, University of São Paulo School of Medicine, São Paulo, Brazil
- 9Interunit Postgraduate Program on Bioinformatics, Institute of Mathematics and Statistics, University of Sao Paulo, Sao Paulo, Brazil
- 10Department of Nutrition, Center for Health Sciences, Federal University of Rio Grande do Norte, Natal, Brazil
- 11Division of Orthopedic Surgery, Schroeder Arthritis Institute and Data Science Discovery Centre for Chronic Diseases, Krembil Research Institute, University Health Network, Toronto, ON, Canada
- 12Departments of Medical Biophysics and Computer Science, and Faculty of Dentistry, University of Toronto, Toronto, ON, Canada
- 13Slovak Academy of Sciences, Institute of Neuroimmunology, Bratislava, Slovakia
- 14Department of Clinical and Toxicology Analysis, Federal University of Rio Grande do Norte, Natal, Brazil
- 15Translational Medicine, The Hospital for Sick Children (SickKids), Toronto, ON, Canada
Background: Acute ST-elevation myocardial infarction (STEMI) can lead to adverse cardiac remodeling, resulting in left ventricular systolic dysfunction (LVSd) and heart failure. Epigenetic regulators, such as microRNAs, may be involved in the physiopathology of LVSd.
Objective: This study explored microRNAs in peripheral blood mononuclear cells (PBMC) of post-myocardial infarction patients with LVSd.
Methods: Post-STEMI patients were grouped as having (LVSd, n = 9) or not LVSd (non-LVSd, n = 16). The expression of 61 microRNAs was analyzed in PBMC by RT-qPCR and the differentially expressed microRNAs were identified. Principal Component Analysis stratified the microRNAs based on the development of dysfunction. Predictive variables of LVSd were investigated through logistic regression analysis. A system biology approach was used to explore the regulatory molecular network of the disease and an enrichment analysis was performed.
Results: The let-7b-5p (AUC: 0.807; 95% CI: 0.63–0.98; p = 0.013), miR-125a-3p (AUC: 0.800; 95% CI: 0.61–0.99; p = 0.036) and miR-326 (AUC: 0.783; 95% CI: 0.54–1.00; p = 0.028) were upregulated in LVSd (p < 0.05) and discriminated LVSd from non-LVSd. Multivariate logistic regression analysis showed let-7b-5p (OR: 16.00; 95% CI: 1.54–166.05; p = 0.020) and miR-326 (OR: 28.00; 95% CI: 2.42–323.70; p = 0.008) as predictors of LVSd. The enrichment analysis revealed association of the targets of these three microRNAs with immunological response, cell-cell adhesion, and cardiac changes.
Conclusion: LVSd alters the expression of let-7b-5p, miR-326, and miR-125a-3p in PBMC from post-STEMI, indicating their potential involvement in the cardiac dysfunction physiopathology and highlighting these miRNAs as possible LVSd biomarkers.
Introduction
Acute myocardial infarction (AMI) is a severe cardiovascular disease leading to progressive cardiac remodeling (1). Cardiac remodeling is a compensatory, adaptive, and functional process in response to a given aggression. When sustained, it can progress to adverse remodeling, with structural changes in cardiomyocytes, endothelium, smooth muscle cells, interstitial cells, and extracellular matrix (2). Potential factors involved in cardiac remodeling are energetic metabolism disturbance, oxidative stress, inflammation, neurohormonal activation, contractility reduction of cardiomyocytes, calcium transport imbalance, cell death, and fibrosis (3).
The adverse remodeling majority occurs during the first 3–6 months following ST-segment elevation myocardial infarction (STEMI) (4) and reaches approximately 40% of patients with STEMI (5). This pathological remodeling plays an essential role in the development of left ventricular systolic dysfunction (LVSd) and subsequent heart failure (HF) (2), which is a source of concern given that 70% of patients who develop HF after AMI die within 7.6 years (6). Therefore, the prediction of LVSd in post-myocardial infarction (post-MI) patients is essential to provide treatment that attenuates or even reverses the process and improves poor outcomes (7).
The detection of morphological changes in the clinical diagnosis of ventricular dysfunction is still based on cardiac imaging tests such as echocardiography and nuclear magnetic resonance (3). At the same time, molecular biomarkers are limited to proteins that indicate cardiac hemodynamic stress (e.g., N-terminal pro-B-type natriuretic peptide) or cardiomyocyte damage (e.g., cardiac troponin) (8). Despite study advances, there is still a need to understand the molecular mechanisms of left ventricular remodeling and to find early biomarkers for accurate LVSd detection and therapeutic targets to prevent the development of HF.
Among the molecular network involved in HF, microRNAs (miRNAs) are implicated in various pathological processes in the post-MI heart, suggesting that their up- or downregulation modulates metabolic pathways related to cardiac remodeling and HF (9). miRNAs are small non-coding RNAs that regulate gene expression by post-transcriptionally binding to the 3′-untranslated region of target mRNAs and inducing gene silencing by translational inhibition or mRNA degradation (10, 11). Several studies support the potential of miRNAs as physiological biomarkers, therapeutic mediators, and even as a predictor of therapeutic efficacy or poor prognosis of various immune-mediated diseases (12), such as cancer (13), “Alzheimer's disease” (14), and HF (15).
Of note, the immune system plays an essential role in cardiac disease (16) and miRNAs of peripheral blood mononuclear cells (PBMC) have been shown to be dysregulated in cardiovascular diseases (17). Thus, these molecules may be used as biomarkers to identify patients with atherosclerotic coronary artery disease at risk of acute coronary syndromes (18) or even to differentiate HF patients from healthy subjects (19). In this study, the expression of miRNAs was assessed in PBMC of post-MI patients with LVSd, and a system biology approach was used to explore the regulatory molecular network of the disease, focusing on pathways involved with cardiac remodeling, cell-cell communications, and immunological changes.
Materials and methods
Study subjects
Patients who experienced STEMI at least two months before enrollment and were treated in Onofre Lopes University Hospital of the UFRN, Brazil, between July 2018 and December 2019 were eligible for this cross-sectional study. The patients were recruited during their routine appointment at the Cardiology Outpatient Unit. The exclusion criteria were other causes of LVSd or HF, such as congenital cardiomyopathies, dilated cardiomyopathy, atrial fibrillation, Chagas disease, and non-treated hypertension. Patients who were referred for cardiac transplants were also excluded. Demographic, clinical, and echocardiographic characteristics were obtained from electronic medical records and personal interviews. The sex of participants was defined based on self-report and was used as a classification of male or female based on biological distinction. The clinical outcome of the post-MI patients was determined by left ventricular ejection fraction (LVEF) measured at ± three months from the date of venous blood collection. Patients were grouped as having LVSd (LVEF ≤ 40%) or not (non-LVSd, LVEF > 40%) (20).
Study approval
The Ethics Committees of the Federal University of Rio Grande do Norte (UFRN), Brazil (CAAE #65856317.1.0000.5292) approved the study protocol. The study was conducted according to good clinical practices and the Helsinki guidelines (as revised in 2013). All subjects signed an approved written informed consent before enrollment. All experiments were performed following relevant guidelines and regulations.
Biochemical and hematological measurements
Hemoglobin concentration, hematocrit, leukocytes, and platelet numbers were measured in whole blood samples using an automated XT-2000i (Sysmex Corporation, Kobe, Japan). Fasting serum glucose, total cholesterol, high-density lipoprotein cholesterol, low-density lipoprotein cholesterol, triglycerides, sodium, potassium, urea and creatinine concentrations, alanine aminotransferase, and aspartate aminotransferase enzymatic activity were measured using Wiener kits and a CMD-800 automatic biochemistry analyzer (Wiener Laboratories, Rosario, Argentina).
miRNA expression
PBMC were isolated from 4 ml of whole blood in EDTA using ammonium chloride as lysis solution and were stored in Trizol (Invitrogen, Waltham, MA, USA) at −80°C until analysis. Total RNA was extracted using Trizol according to the “manufacturer's protocol”. RNA concentration and purity were measured using a Nanodrop spectrophotometer (NanoDrop ND-1000, Montchanin, DE, USA). RNA integrity was determined by agarose gel electrophoresis (2% agarose/MOPS). The gel was stained with GelRed (Uniscience, São Paulo, SP, Brazil), revealing the presence of two sharp bands at approximately 5 and 1.8 Kb, corresponding to 28S and 18S ribosomal RNA, respectively.
The reverse transcription of miRNA samples was performed using TaqMan™ Advanced miRNA cDNA Synthesis Kit (Applied Biosystems, Waltham, MA, USA). For the analysis, 61 miRNAs previously described in the literature were selected for their relationship with cardiovascular diseases (see Supplementary Table S1). The miRNAs' expression was measured by Real-Time quantitative PCR (RT-qPCR) using a custom TaqMan™ Advanced miRNA Human A and B 96-well Plates, TaqMan™ Fast Advanced Master Mix Kit (Applied Biosystems, Waltham, MA, USA) and were performed in QuantStudio™ 5 Real-Time PCR System, 96-well. No miRNA showed cycle threshold (Ct) values higher than 35 in the analysis. Relative miRNA levels were measured using the comparative Ct method (2−ΔΔCt for calculate the fold-change value; and 2−ΔCt for other statistical analysis) (21) normalized by miR-16-5p as a reference, a miRNA abundantly expressed in all tissues and commonly used as a control (22, 23). The miRNAs differentially expressed in the LVSd, as compared with the non-LVSd, were selected considering a fold-change of |1.5| and p-value < 0.05 (see Supplementary Table S1).
Principal component analysis
Principal Component Analysis (PCA) was used to stratify the miRNAs based on the post-MI patients who developed LVSd or not. Before the analysis, the miRNAs expression data were log2-transformed. PCA was performed using the R package factoextra and the prcomp function, in which data was centered and scaled. The number of principal components was chosen according to Kaiser Criterion. References for all statistical tools used in this study are listed in Supplementary Table Si.
Prediction of miRNAs targets
The target genes of differentially expressed miRNAs were identified using the microRNA Data Integration Portal (mirDIP ver. 5.2; https://ophid.utoronto.ca/mirDIP). All annotated interaction data are presented in Supplementary Material. In addition, the miRTarBase 9.0 (https://mirtarbase.cuhk.edu.cn/) and DIANA TOOLS-Tarbase ver.8 (https://dianalab.e-ce.uth.gr/) were used to select the experimentally validated miRNA-mRNA interactions. Only interactions validated by robust evidence methods (reporter assay, western blot, and qPCR) were selected. References for all bioinformatics tools used in this study are listed in Supplementary Table Si.
Enrichment analysis and data visualization
The experimentally validated miRNA target genes were used for enrichment analysis. Biological processes (GO) were analyzed using EnrichR (http://amp.pharm.mssm.edu/Enrichr/), and the enriched terms were filtered according to adjusted p-value < 0.05 and Z-score (correction to the test) in a combined score provided by EnrichR database. The biological process terms presented in the bubble heatmap were selected based on the criterion of overrepresentation (log2 combined score >2) in at least two miRNAs. Concomitantly, we searched for the enriched pathways using the Kyoto Encyclopedia of Genes and Genomes (KEGG) using Database for Annotation, Visualization and Integrated Discovery (DAVID ver.2021; https://david.ncifcrf.gov/). We selected the pathways with a p-value threshold < 0.05 after correction for False Discovery Rate (FDR). Only experimentally validated miRNA-mRNA interactions were used for this analysis.
We plotted the biological processes associated with the three differentially expressed miRNAs (let-7b-5p, miR-326, miR-125a-3p) in a bubble-based heat map with hierarchical clustering using the web tool Morpheus (https://software.broadinstitute.org/morpheus/) with Euclidian distance metric. We created a mirrored bar plot using the GraphPad Prism ver.9.2 (GraphPad Software, Inc., San Diego, CA, USA) to represent the enriched pathways from the KEGG.
Molecular network of miRNA-mRNA interactions
The miRNA-gene network was prepared using mirDIP ver. 5.2 (https://ophid.utoronto.ca/mirDIP), considering all sources and top 1% gene targets. Only shared targets are highlighted with names, but all gene targets are annotated with Gene Ontology biological process, as per color legend (Figure 3A). Also, shared mRNAs target genes among three miRNAs were displayed using the Circos software ver. 0.63–10 (http://circos.ca/) (see Supplementary Figure S1).
Taking advantage of curated tissue annotation for both miRNA and gene targets, Figure 3B highlights tissues associated with the three miRNAs. To further characterize biological relationships among the three miRNAs, we have generated a physical protein interaction network among miRNA targets using Integrated Interactions Database (IID ver. 2021-05; http://ophid.utoronto.ca/iid). All direct human interactions among the target genes are shown, highlighting those relevant to heart and cardiovascular disease (edge color as per legend). We differentiate whether only one of the interactors has the annotation (partially transparent edge with color as per legend) or both interacting partner share the annotation (solid edge with color as per legend), and whether the annotation is valid for both heart and cardiovascular system disease (strong solid edge with color as per legend). In addition, node color corresponds to Gene Ontology biological process. All annotated interaction data are presented in Supplementary Material.
All networks were obtained using NAViGaTOR ver 3.0.16. Network images were exported in SVG format into Adobe Illustrator ver. 27 to prepare final figures with legends.
Statistical analysis
The distribution of the continuous variables was analyzed using the Shapiro-Wilk test. Variables with normal distribution are shown as mean and standard deviation and were compared by t-test for independent variables. Those with skewed distributions are shown as median and interquartile range and compared using Mann-Whitney U-test. Spearman's correlation tests assessed the correlation analysis between differentially expressed miRNAs. Categorical variables were compared using Fisher's exact test and are shown as relative and absolute frequency.
Assessing the discriminatory power of the selected miRNA as an LVSd predictor, a receiver operating curve (ROC) was constructed, and the area under the curve (AUC) was calculated with a 95% confidence interval (CI). The cut-off point for miRNA was identified based on an excellent combination of sensitivity and specificity (AUC ≥ 0.7).
Univariate logistic regression analysis was performed to evaluate the variables that predict post-MI LVSd. Multivariate logistic regression analysis was performed using a forward stepwise method only with statistically significant miRNAs in the univariate analysis and adjusted by sex, body mass index, smoking, alcohol intake, diabetes, and time from AMI to enrollment as independent variables.
Statistical analysis was performed using SPSS Statistics V23.0 software (IBM, IL, USA), GraphPad PRISM, version 5.0 (GraphPad Software, Inc., San Diego, CA, USA), or R program (v4.1) using packages ggplot2 (v3.4.0) and the p-value < 0.05 was considered statistically significant.
Results
Patient characteristics
The description of clinical characteristics of post-MI patients is shown in Table 1. Most of the patients enrolled in the study were men, and 36% developed LVSd. They presented LVEF < 40%. The groups did not differ in terms of age, BMI, chronic diseases, and habits such as physical activity, alcohol intake, and smoking, as well as concerning biochemical data (Table 2). This similarity between the participants was expected because both groups are composed of people who have already suffered STEMI and were undergoing medical follow-up.
Regarding the medication, diuretic and vasodilator drugs were more frequent in patients with LVSd (p < 0.05), possibly because they are medications commonly used in treating this condition. The time from AMI to enrollment was longer for LVSd patients (p < 0.05), which highlights the fact that the development of dysfunction may be time-related. Therefore, maintenance of cardiac function monitoring after AMI may be necessary for detecting LVSd in these patients.
miRNAs differently expressed in post-MI patients with LVSd
The relative miRNA levels of the 61 miRNAs are shown in Supplementary Table S1. Among the miRNAs analyzed, let-7b-5p (p = 0.013), miR-326 (p = 0.028), and miR-125a-3p (p = 0.036) were upregulated in post-MI patients with LVSd (Figure 1A). Figure 1B shows AUC and optimal cut-off values (AUC ≥ 0.7) for three miRNAs: let-7b-5p (AUC: 0.807; 95% CI: 0.63–0.98; p = 0.013), miR-326 (AUC: 0.783; 95% CI: 0.54–1.00; p = 0.028), and miR-125a-3p (AUC: 0.800; 95% CI: 0.61–0.99; p = 0.036). No significant correlations among miRNAs were detected (Supplementary Table S2).
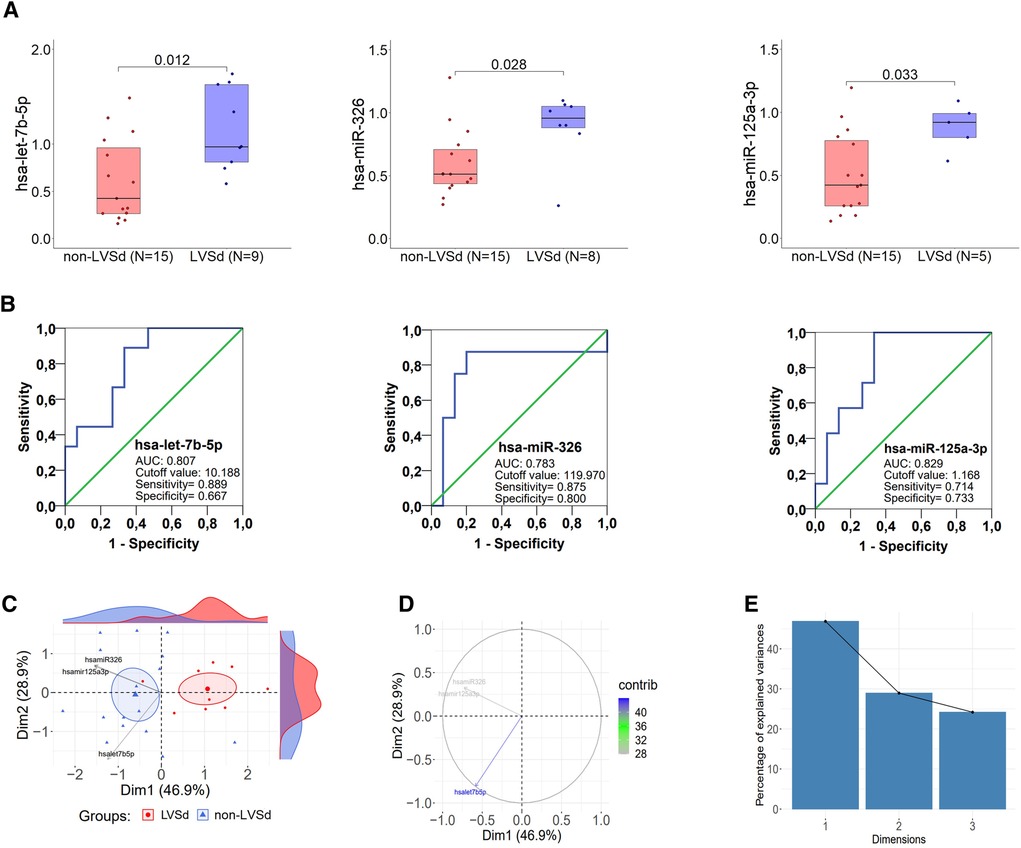
Figure 1. miRNAs stratify post-MI patients based on left ventricular ejection fraction. (A) Differentially expressed microRNAs in PBMCs between post-MI patients with left ventricular systolic dysfunction (LVSd) and non-LVSd. Data are shown as the median of relative expression (2−ΔCT×104) and were compared using U-Mann Whitney; *p-value significant (<0.05). (B) Evaluation of the clinical value of microRNA in diagnosing left ventricular systolic dysfunction; Receiver operating characteristic (ROC) curve based on let-7b-5p, miR-326, and miR-125a-3p expression. (C) Principal component analysis (PCA) with spectral decomposition based on 3 different miRNAs expression shows the stratification of non-LVSd and LVSd patients. miRNAs with positive correlation point to the same side of the plot, contrasting with negatively correlated miRNAs, which point to opposite sides. Only the miRNAs highly contributing to the stratification of non-LVSd from LVSd patients are shown (let-7b-5p, miR-326, and miR-125a-3p). Small blue and red circles are concentration ellipses around the mean points of each group. The histograms aside the PCA, represent the density of the individual distribution. (D) Graphs of the three miRNAs obtained by PCA indicate miRNAs highly associated with post-MI patients with LVSd. (E) Scree plot showing eigenvalues, demonstrating the percentage of variances explained by each principal component.
miRNA predictors of LVSd in post-MI patients
The univariate binary logistic regression analysis with miRNA categorized according to the cut-off value showed that the high expression of let-7b-5p (OR: 16.00; 95% CI: 1.54–166.05; p = 0.020) and miR-326 (OR: 28.00; 95% CI: 2.42–323.70; p = 0.008) were able to predict LVSd in post-MI patients, but not the miR-125a-3p (OR: 10.00; 95% CI: 0.91–110.28; p = 0.060) (Table 3). The other variables tested were also not significant in this analysis.
The multivariate models were built up with the let-7b-5p and miR-326 individually, and adjusted by sex, body mass index, smoking, alcohol intake, diabetes, and time from AMI to enrollment (Table 4). For both models, only let-7b-5p and miR-326 remained significant by the forward stepwise method, demonstrating their robust predictive capacity independently of the characteristics of the participants.
miRNAs expression stratifies post-MI patients according to LVSd
Next, we performed principal component analysis (PCA) to examine the association between the expression of miRNAs and individuals (observations) while stratifying groups based on the level of the miRNA. This analysis indicated that miRNAs expression levels could be used to stratify post-MI patients according to LVSd (Figures 1C–E). The miRNAs miR-326, miR-125a-3p, and let-7b-5p seemed to play a significant role in stratifying post-MI patients by LVSd. This analysis indicated that these three miRNAs could be biomarkers for post-MI outcomes.
miRNAs-targets are involved with immune response and cardiovascular pathogenesis in post-MI patients with LVSd
Based on strong validated evidence of the two miRNA-target tools, we found 91 target genes of let-7b-5p, miR-326, and miR-125a-3p (Supplementary Table S3). Results were obtained by enrichment analysis based on the Gene ontology (GO) and Kyoto Encyclopedia of Genes and Genomes (KEGG) databases (Supplementary Tables S4, S5). Among the GO biological processes (Figure 2A), terms related to adverse cardiac remodeling were evidenced, such as positive regulation of cardiac epithelial to mesenchymal transition, regulation of cardiac hypertrophy, regulation of adherent's junction organization, cardiac endothelial cell differentiation, regulation of angiogenesis, cytokine-mediated signaling pathway, positive regulation of T cell proliferation, positive regulation of T cell activation, regulation of mast cell chemotaxis.
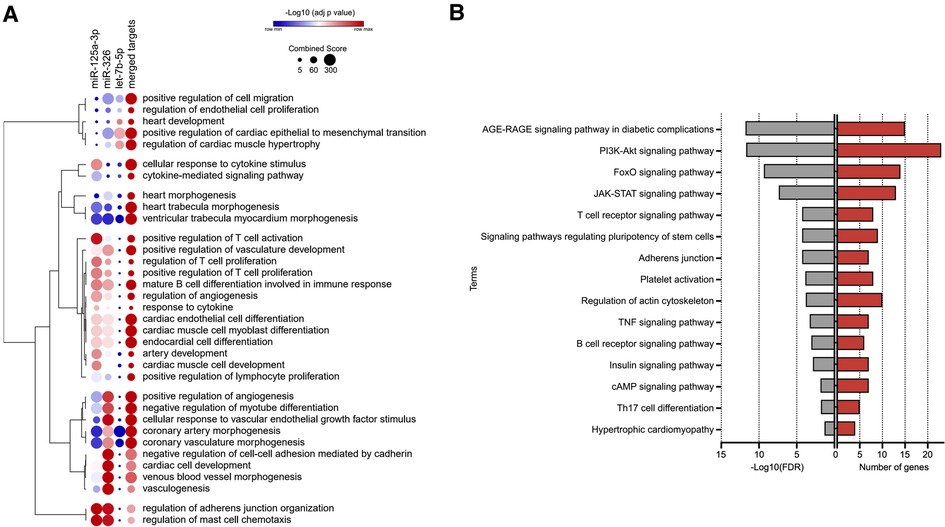
Figure 2. The gene ontology (GO) and Kyoto encyclopedia of genes and genomes (KEGG) pathway enrichment analyses for the validated targets of microRNAs predictors of left ventricular dysfunction in post-myocardial infarction patients; (A) terms of GO biological processes related to adverse cardiac remodeling; (B) terms of KEGG pathways related to adverse cardiac remodeling.
The KEGG pathway enrichment analysis (Figure 2B) revealed enriched signaling pathways, such as those that regulate the expression of growth factors, cytokines, cell proliferation, and apoptosis, including the AGE-RAGE signaling pathway in diabetic complications, PI3K-Akt signaling pathway, FoxO signaling pathway, and JAK-STAT signaling pathway. In addition, pathways related to immune response, cardiac hypertrophy, and adherents' junctions also appeared statistically enriched.
Finally, to better understand the involvement of these three miRNAs with cardiovascular pathogenesis, we performed an interaction analysis with the target genes related to cardiac processes. This analysis revealed an interconnected network of target genes modulated by let-7b-5p, miR-326, and miR-125a-3p (Figures 3A–C). Figure 3A displays the overlapping target genes of the three miRNAs, indicating a complex combination of target multiplicity and miRNA cooperativeness on the LVSd. Meanwhile, Figure 3B shows the relation between miR-326 and the left ventricle and the association of miR-125a-3p and let-7b-5p with the heart and left ventricle. Figure 3C displays the interactions of these miRNA targets represented by their physical protein-protein interaction (PPI) and gene ontology (GO) relationships.
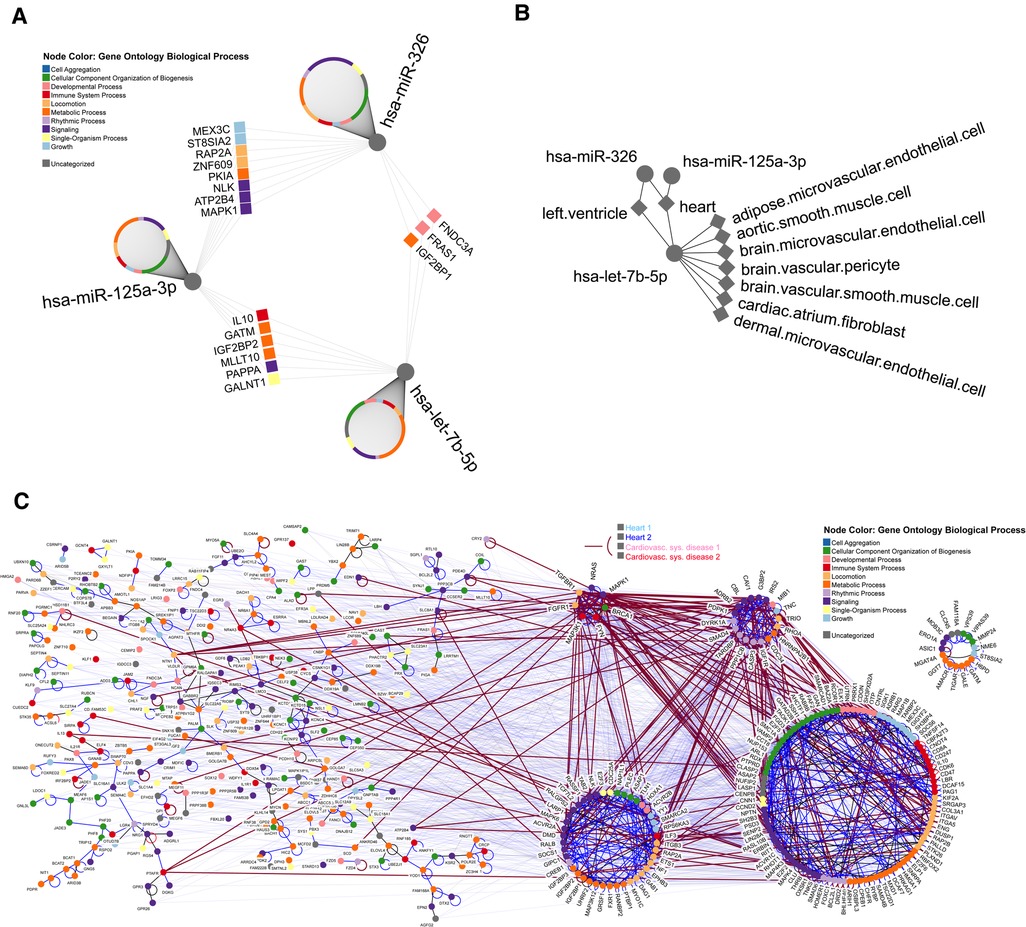
Figure 3. Molecular network of miRNA-mRNA interactions. The miRNA-gene network was prepared using the top 1% of gene targets. (A) Gene Ontology (GO) biological process across all gene targets, as per color legend. Only shared targets are highlighted with names. (B) Tissues associated with the three miRNAs. (C) Physical protein interaction network among miRNA targets. All direct human interactions among the target genes are shown, highlighting those relevant to heart and cardiovascular disease. With edge thickness, transparency, and color we differentiate whether only one of the interactors has the annotation (color as per legend and partially transparent) or both interacting partners share the annotation (color as per legend and strong edge) and whether the annotation is valid for both heart and cardiovascular system disease. In addition, node color corresponds to the GO biological process.
Discussion
The present study investigated the expression of miRNAs from PBMCs and found that let-7b-5p, miR-326, and miR-125a-3p were upregulated in LVSd patients, highlighting let-7b-5p and miR-326 as independent predictors of cardiac dysfunction in post-MI patients. Thus, this work expands the molecular mechanisms involved in post-MI cardiac remodeling, since the role of miRNAs in this condition remains poorly explored. Particularly, LVSd is a consequence of adverse cardiac remodeling, which affects approximately 40% of patients with STEMI and is associated with increased morbidity and mortality. Myocardial infarction impacts the immune system and causes changes in the blood transcriptome landscape, which relate to the development of LVSd in post-MI (24, 25). Thus, a better understanding of the involvement of essential modulators of gene expression, such as let-7b-5p, miR-326, and miR-125a-3p is fundamental to identifying new therapeutic targets and disease biomarkers.
Several transcriptomic changes have been investigated in post-MI patients, showing the immune system's role during cardiac remodeling (25, 26). In this context, our results suggest a dysregulated expression pattern of the miRNAs let-7b-5p, miR-326, and miR-125a-3p, with involvement in immunological pathways (i.e., regulation of mast cell chemotaxis, positive regulation of T cell proliferation), cell-cell communication (i.e., regulation of adherens junction organization, cytokine-mediated signaling pathway), and cardiac changes (i. e., regulation of cardiac hypertrophy, regulation of angiogenesis). The let-7b-5p is a well-studied miRNA that plays an essential role in targeting important genes or pathways involved in the development of cardiovascular diseases (27–29). This miRNA was found in pericardial fluid exosomes and was able to restore the angiogenic ability of endothelial cells, as well as improve post-ischemic blood flow and angiogenesis in mice (30). Interestingly, Kuosmanen et al. (31) reported that let-7b-5p is one of the five most abundant miRNAs in the pericardial fluid of HF patients and Marques et al. (32) described the expression of cardio-miRNAs in the transcardiac gradient of advanced HF patients, showing that the failing heart releases let-7b. These findings are in agreement with our study, suggesting that cardiac injury alters the expression of let-7b-5p and is related to HF's pathophysiology.
Another dysregulated miRNA identified was miR-326, which has an essential modulatory function in the immune system (33), and a relationship with various autoimmune diseases (34, 35). In the cardiovascular context, Danaii et al. (36) showed that the levels of miR-326 from PBMC were increased in slow coronary flow patients, and Dolati et al. (37) report its expression was upregulated in elderly patients with ischemic stroke associated with disease severity and IL-17 production. To the best of our knowledge, our study is the first to demonstrate the differential expression of miR-326 in PBMCs of post-MI patients. In addition to the importance of miR-326 in immune regulation, this study showed that this miRNA appears to regulate the angiogenesis process, targeting essential genes, such as FGF1 and VEGF. Similar findings were reported by Li et al. (38), who described that miR-326 improved the cardiac function of ischemic hearts through enhanced angiogenesis.
It has been shown that miR-125a-3p reduced vascular smooth muscle cell growth and migration, inhibiting the occurrence of vascular stenosis (39) and that miR-125a-3p promotes cellular apoptosis in a model of atherosclerosis pathogeny treated with ox-LDL (40). The expression of miR-125a-3p was investigated in failing heart, but no statistical difference was found when compared with healthy controls (41). However, intravenous injection of miR-125a-3p adenovirus improved cardiac function and fibrosis while reducing inflammatory responses in mice with diabetic cardiomyopathy (42). The high expression of miR-125a-3p in post-MI patients seems to be involved in the cellular response to cytokine stimulus, regulation of mast cell chemotaxis, and other biological processes involving activation, proliferation, and differentiation of immune cells, which can explain the cardiac remodeling and worse prognosis in these patients.
Noteworthy, these miRNAs may regulate signaling pathways involved in cardiac dysfunction. The AGE-RAGE signaling pathway is a key mechanism responsible for increased matrix contraction and myofibroblast differentiation, with stiffening of the left ventricle and poor heart function in diabetics (43). The PI3K-Akt signaling pathway activation has been shown to promote cardioprotection after AMI, and reduced PI3K-Akt expression led to myocardial fibrosis and reduced left ventricular function (44). The pivotal role of the FoxO signaling pathway in the autophagic activity induced by the pathological process has been related to the development of HF (45). In contrast, the protective role of FoxO transcription factors was shown in response to acute ischemia/reperfusion injury by limiting ROS production and cell death in the heart (46). JAK/STAT pathway is another important pathway enriched in our analysis, which has been related to the apoptotic response after MI and implicated in promoting myocardial angiogenesis, improving cardiac function (47).
Despite the interesting findings, this study has some limitations. The sample size was limited, requiring a larger sample to validate the findings of this study. Other limitation is that the ventricular dysfunction was analyzed in post-MI patients regardless of HF symptoms. Therefore, it is important to explore the role of let-7b-5p, miR-326, and miR-125a-3p in HF patients and disease progression. Also, as only PBMC miRNAs were analyzed, inferences about their direct role in the cardiac structure are limited. Further studies with analysis of miRNAs in cardiac tissue may provide a more comprehensive understanding of their role in cardiac remodeling.
In conclusion, this study demonstrates that let-7b-5p, miR-326, and miR-125a-3p are upregulated in PBMCs from patients with post-MI LVSd. These miRNAs appear to regulate pathways involved in cardiovascular pathogenesis, suggesting the involvement of the immune system in the pathophysiology of cardiac dysfunction through the differentiated expression of miRNAs. Furthermore, this study demonstrates that the expression of let-7b and miR-326 can predict LVSd and support future research that validates their potential application as LVSd biomarkers in post-MI patients.
Data availability statement
The original contributions presented in the study are included in the article/Supplementary Material, further inquiries can be directed to the corresponding author.
Ethics statement
The studies involving human participants were reviewed and approved by The Ethics Committees of the Federal University of Rio Grande do Norte (UFRN), Brazil. The patients/participants provided their written informed consent to participate in this study.
Author contributions
VNS and MSC: designed the research; RCSDK: contributed to patient recruitment and acquiring clinical data; MSC: recruited patients, obtained data, and obtained blood samples. MHH and RDCH: provided reagents; MSC and RHB: performed laboratory analysis; PPF, IJ, and RCSDK: performed the enrichment analysis; RCSDK, ADL, and OCM: performed the statistical analysis; IJ: performed network analyses; KBSS: designed the graphic abstract; KBSS, RVZD, and BZR: provided scientific insights; RCSDK: wrote the manuscript; MHH, RDCH, VNS, ADL, OCM, and BZR: performed a critical revision of the manuscript. All authors contributed to the article and approved the submitted version.
Funding
This study was financed in part by the Coordination for the Improvement of Higher Education Personnel - Brazil (CAPES) - Finance Code 001. This work was supported by the São Paulo Research Foundation (FAPESP grant 2020/09146-1 to PPF; 2018/18886-9, 2020/01688-0, and 2020/07069-0 to OCM). IJ was supported in part by funding from Natural Sciences Research Council (NSERC #203475), Canada Foundation for Innovation (CFI #225404, #30865), Ontario Research Fund (RDI #34876), IBM, and Ian Lawson van Toch Fund. The funders had no role in the study's design; the collection, analyses, or interpretation of data; the writing of the manuscript; or the decision to publish the results.
Acknowledgments
We acknowledge the National Council for Scientific and Technological Development (CNPq) Brazil (grant: 309482/2022-4 to OCM). AL, VS, MH, and RH are recipients of fellowships from the National Council for Scientific and Technological Development (CNPq)-Brazil, and RD is a recipient of a fellowship from “Coordenação de Aperfeiçoamento de Pessoal de Nível Superior—Brasil” (CAPES)—Finance Code 001. When performing this work, MS was a fellowship recipient from CAPES—Finance Code 001.
Conflict of interest
The authors declare that the research was conducted in the absence of any commercial or financial relationships that could be construed as a potential conflict of interest.
Publisher's note
All claims expressed in this article are solely those of the authors and do not necessarily represent those of their affiliated organizations, or those of the publisher, the editors and the reviewers. Any product that may be evaluated in this article, or claim that may be made by its manufacturer, is not guaranteed or endorsed by the publisher.
Supplementary material
The Supplementary Material for this article can be found online at: https://www.frontiersin.org/articles/10.3389/fcvm.2023.1151855/full#supplementary-material.
References
1. Liu X, Gao J, Xia Q, Lu T, Wang F. Increased mortality and aggravation of heart failure in liver X receptor-α knockout mice after myocardial infarction. Heart Vessels. (2016) 31(8):1370–9. doi: 10.1007/s00380-015-0781-y
2. Heusch G, Libby P, Gersh B, Yellon D, Böhm M, Lopaschuk G, et al. Cardiovascular remodelling in coronary artery disease and heart failure. Lancet. (2014) 383(9932):1933–43. doi: 10.1016/S0140-6736(14)60107-0
3. Azevedo PS, Polegato BF, Minicucci MF, Paiva SAR, Zornoff LAM. Cardiac remodeling: concepts, clinical impact, pathophysiological mechanisms and pharmacologic treatment. Arq Bras Cardiol. (2016) 106(1):62–9. doi: 10.5935/abc.20160005
4. Del Buono MG, Garmendia CM, Seropian IM, Gonzalez G, Berrocal DH, Biondi-Zoccai G, et al. Heart failure after ST-elevation myocardial infarction: beyond left ventricular adverse remodeling. Curr Probl Cardiol. (2022) 101215. doi: 10.1016/j.cpcardiol.2022.101215
5. Minicucci MF, Azevedo PS, Polegato BF, Paiva SAR, Zornoff LAM. Heart failure after myocardial infarction: clinical implications and treatment. Clin Cardiol. (2011) 34(7):410–4. doi: 10.1002/clc.20922
6. Gerber Y, Weston SA, Enriquez-Sarano M, Berardi C, Chamberlain AM, Manemann SM, et al. Mortality associated with heart failure after myocardial infarction: a contemporary community perspective. Circ Hear Fail. (2016) 9(1):e002460. doi: 10.1161/CIRCHEARTFAILURE.115.002460.
7. Zornoff LAM, Paiva SAR, Duarte DR, Spadaro J. Ventricular remodeling after myocardial infarction: concepts and clinical implications. Arq Bras Cardiol. (2009) 92(2):150–64. doi: 10.1590/s0066-782x2009000200013
8. Shah R, Ziegler O, Yeri A, Liu X, Murthy V, Rabideau D, et al. MicroRNAs associated with reverse left ventricular remodeling in humans identify pathways of heart failure progression. Circ Hear Fail. (2018) 11(2):e004278. doi: 10.1161/CIRCHEARTFAILURE.117.004278
9. Zhang X, Schulze PC. MicroRNAs in heart failure: non-coding regulators of metabolic function. Biochim Biophys Acta. (2016) 1862(12):2276–87. doi: 10.1016/j.bbadis.2016.08.009
10. Huntzinger E, Izaurralde E. Gene silencing by microRNAs: contributions of translational repression and mRNA decay. Nat Rev Genet. (2011) 12:99–110. doi: 10.1038/nrg2936
11. Hammond SM An overview of microRNAs. Adv Drug Deliv Rev. (2015) 87:3–14. doi: 10.1016/j.addr.2015.05.001
12. Pozniak T, Shcharbin D, Bryszewska M. Circulating microRNAs in medicine. Int J Mol Sci. (2022) 23(7):3996. doi: 10.3390/ijms23073996
13. Dioguardi M, Spirito F, Sovereto D, Alovisi M, Aiuto R, Garcovich D, et al. The prognostic role of miR-31 in head and neck squamous cell carcinoma: systematic review and meta-analysis with trial sequential analysis. Int J Environ Res Public Health. (2022) 19(9):5334. doi: 10.3390/ijerph19095334.
14. Yuen SC, Liang X, Zhu H, Jia Y, Leung Sw. Prediction of differentially expressed microRNAs in blood as potential biomarkers for Alzheimer's Disease by meta-analysis and adaptive boosting ensemble learning. Alzheimer's Res Ther. (2021) 13(1):126. doi: 10.1186/s13195-021-00862-z.
15. Gholaminejad A, Zare N, Dana N, Shafie D, Mani A, Javanmard SH. A meta-analysis of microRNA expression profiling studies in heart failure. Heart Fail Rev. (2021) 26(4):997–1021. doi: 10.1007/s10741-020-10071-9
16. He B, Quan L-P, Cai C-Y, Yu D-Y, Yan W, Wei Q-J, et al. Dysregulation and imbalance of innate and adaptive immunity are involved in the cardiomyopathy progression. Front Cardiovasc Med. (2022) 9. doi: 10.3389/fcvm.2022.973279
17. Gupta MK, Halley C, Duan ZH, Lappe J, Viterna J, Jana S, et al. MiRNA-548c: a specific signature in circulating PBMCs from dilated cardiomyopathy patients. J Mol Cell Cardiol. (2013) 62:131–41. doi: 10.1016/j.yjmcc.2013.05.011
18. Hoekstra M, van der Lans CAC, Halvorsen B, Gullestad L, Kuiper J, Aukrust P, et al. The peripheral blood mononuclear cell microRNA signature of coronary artery disease. Biochem Biophys Res Commun. (2010) 394(3):792–7. doi: 10.1016/j.bbrc.2010.03.075
19. Voellenkle C, Van Rooij J, Cappuzzello C, Greco S, Arcelli D, Di Vito L, et al. MicroRNA signatures in peripheral blood mononuclear cells of chronic heart failure patients. Physiol Genomics. (2010) 42(3):420–6. doi: 10.1152/physiolgenomics.00211.2009
20. Yancy CW, Jessup M, Bozkurt B, Butler J, Casey DE, Drazner MH, et al. 2013 ACCF/AHA guideline for the management of heart failure: a report of the American college of cardiology foundation/American heart association task force on practice guidelines. J Am Coll Cardiol. (2013) 62(16):e147–239. doi: 10.1016/j.jacc.2013.05.019
21. Livak KJ, Schmittgen TD. Analysis of relative gene expression data using real-time quantitative PCR and the 2−ΔΔCT method. Methods. (2001) 25(4):402–8. doi: 10.1006/meth.2001.1262
22. Meyer SU, Pfaffl MW, Ulbrich SE. Normalization strategies for microRNA profiling experiments: a ‘normal’ way to a hidden layer of complexity? Biotechnol Lett. (2010) 32(12):1777–88.doi: 10.1007/s10529-010-0380-z
23. Liang Y, Ridzon D, Wong L, Chen C. Characterization of microRNA expression profiles in normal human tissues. BMC Genomics. (2007) 8(1):166. doi: 10.1007/s10529-010-0380-z
24. Smih F, Desmoulin F, Berry M, Turkieh A, Harmancey R, Iacovoni J, et al. Blood signature of pre-heart failure: a microarrays study. PLoS One. (2011) 6(6):e20414. doi: 10.1371/journal.pone.0020414
25. Sweet ME, Cocciolo A, Slavov D, Jones KL, Sweet JR, Graw SL, et al. Transcriptome analysis of human heart failure reveals dysregulated cell adhesion in dilated cardiomyopathy and activated immune pathways in ischemic heart failure. BMC Genomics. (2018) 19(1):1–14. doi: 10.1186/s12864-018-5213-9
26. Kologrivova I, Shtatolkina M, Suslova T, Ryabov V. Cells of the immune system in cardiac remodeling: main players in resolution of inflammation and repair after myocardial infarction. Front Immunol. (2021) 12(April). doi: 10.3389/fimmu.2021.664457
27. Zhang R, Su H, Ma X, Xu X, Liang L, Ma G, et al. MiRNA let-7b promotes the development of hypoxic pulmonary hypertension by targeting ACE2. Am J Physiol Lung Cell Mol Physiol. (2019) 316:547–57. doi: 10.1152/ajplung.00387.2018
28. Zhu L, Li Q, Qi D, Niu F, Li Q, Yang H, et al. Atherosclerosis-associated endothelial cell apoptosis by miRNA let7-b-mediated downregulation of HAS-2. J Cell Biochem. (2020) 121(8–9):3961–72. doi: 10.1002/jcb.29537
29. Zhang Y, Tang S, Yang W. Du F. Let-7b-5p suppresses the proliferation and migration of pulmonary artery smooth muscle cells via down-regulating IGF1. Clinics. (2022) 77:100051. doi: 10.1016/j.clinsp.2022.100051
30. Beltrami C, Besnier M, Shantikumar S, Shearn AIU, Rajakaruna C, Laftah A, et al. Human pericardial fluid contains exosomes enriched with cardiovascular-expressed MicroRNAs and promotes therapeutic angiogenesis. Mol Ther. (2017) 25(3):679–93. doi: 10.1016/j.ymthe.2016.12.022
31. Kuosmanen SM, Hartikainen J, Hippeläinen M, Kokki H, Levonen AL, Tavi P. MicroRNA profiling of pericardial fluid samples from patients with heart failure. PLoS One. (2015) 10(3):e0119646. doi: 10.1371/journal.pone.0119646
32. Marques FZ, Vizi D, Khammy O, Mariani JA, Kaye DM. The transcardiac gradient of cardio-microRNAs in the failing heart. Eur J Heart Fail. (2016) 18(8):1000–8. doi: 10.1002/ejhf.517
33. Vega-Cárdenas M, Uresti-Rivera EE, Cortés-García JD, Briones-Espinoza M, Ruíz-Rodríguez VM, Reynaga-Hernández E, et al. Increased levels of adipose tissue-resident Th17 cells in obesity associated with miR-326. Immunol Lett. (2019) 211:60–7. doi: 10.1016/j.imlet.2019.05.010
34. Azhir Z, Dehghanian F, Hojati Z. Increased expression of microRNAs, miR-20a and miR-326 in PBMCs of patients with type 1 diabetes. Mol Biol Rep. (2018) 45(6):1973–80. doi: 10.1007/s11033-018-4352-z
35. Liu Y, Cui X, Wang S, Liu J, Zhao N, Huang M, et al. Elevated MicroRNA-326 levels regulate the IL-23/IL-23R/Th17 cell axis in Hashimoto's Thyroiditis by targeting a disintegrin and metalloprotease 17. Thyroid. (2020) 30(9):1327–37. doi: 10.1089/thy.2019.0552
36. Danaii S, Shiri S, Dolati S, Ahmadi M, Ghahremani-Nasab L, Amiri A, et al. The association between inflammatory cytokines and miRNAs with slow coronary flow phenomenon. Iran J Allergy Asthma Immunol. (2020) 19(1):56–64. doi: 10.18502/ijaai.v19i1.2418
37. Dolati S, Ahmadi M, Khalili M, Taheraghdam AA, Siahmansouri H, Babaloo Z, et al. Peripheral Th17/treg imbalance in elderly patients with ischemic stroke. Neurol Sci. (2018) 39(4):647–54. doi: 10.1007/s10072-018-3250-4
38. Li X, Xue X, Sun Y, Chen L, Zhao T, Yang W, et al. MicroRNA-326-5p enhances therapeutic potential of endothelial progenitor cells for myocardial infarction. Stem Cell Res Ther. (2019) 10(1):323. doi: 10.1186/s13287-019-1413-8.
39. Hu W, Chang G, Zhang M, Li Y, Yin L, Huang Y, et al. MicroRNA-125a-3p affects smooth muscle cell function in vascular stenosis. J Mol Cell Cardiol. (2019) 136(September):85–94. doi: 10.1016/j.yjmcc.2019.08.014
40. Xia F, Zeng Q. Mir-125a-3p aggravates ox-LDL-induced HUVEC injury through BAMBI. J Biochem Mol Toxicol. (2022) 36(11):e23198. doi: 10.1002/jbt.23198
41. Li S, Hu D, Hu S, Sun Y, Zhang Y, Li H, et al. Association of rs2070600 in advanced glycosylation end-product specific receptor with prognosis of heart failure. ESC Hear Fail. (2020) 7(6):3561–72. doi: 10.1002/ehf2.12769
42. Liu Y, Zhu Y, Liu S, Liu J, Li X. NORAD Lentivirus shRNA mitigates fibrosis and inflammatory responses in diabetic cardiomyopathy via the ceRNA network of NORAD/miR-125a-3p/Fyn. Inflamm Res. (2021) 70(10–12):1113–27. doi: 10.1007/s00011-021-01500-y
43. Burr SD, Stewart JA. Extracellular matrix components isolated from diabetic mice alter cardiac fibroblast function through the AGE/RAGE signaling cascade. Life Sci. (2020) 250:117569. doi: 10.1016/j.lfs.2020.117569.32201277
44. Walkowski B, Kleibert M, Majka M, Wojciechowska M. Insight into the role of the PI3K/Akt pathway in ischemic injury and post-infarct left ventricular remodeling in normal and diabetic heart. Cells. (2022) 11(9):1553. doi: 10.3390/cells11091553
45. Ferdous A, Battiprolu PK, Ni YG, Rothermel BA, Hill JA, Fox O. Autophagy, and cardiac remodeling. J Cardiovasc Transl Res. (2010) 3(4):355–64. doi: 10.1007/s12265-010-9200-z
46. Sengupta A, Molkentin JD, Paik JH, DePinho RA, Yutzey KE. Foxo transcription factors promote cardiomyocyte survival upon induction of oxidative stress. J Biol Chem. (2011) 286(9):7468–78. doi: 10.1074/jbc.M110.179242
Keywords: miRNA, PBMC, myocardial infarction, left ventricular systolic dysfunction, heart failure, enrichment analysis
Citation: Dantas-Komatsu RCS, Cruz MS, Freire PP, Diniz RVZ, Bortolin RH, Cabral-Marques O, Souza Kamilla Batista da Silva, Hirata MH, Hirata RDC, Reis BZ, Jurisica I, Silbiger VN and Luchessi AD (2023) The let-7b-5p, miR-326, and miR-125a-3p are associated with left ventricular systolic dysfunction in post-myocardial infarction. Front. Cardiovasc. Med. 10:1151855. doi: 10.3389/fcvm.2023.1151855
Received: 26 January 2023; Accepted: 19 April 2023;
Published: 12 May 2023.
Edited by:
Jun Yu, Temple University, United StatesReviewed by:
Ciprian Rezus, Grigore T. Popa University of Medicine and Pharmacy, RomaniaRongxue Wu, The University of Chicago, United States
© 2023 Dantas-Komatsu, Cruz, Freire, Diniz, Bortolin, Cabral-Marques, Souza, Hirata, Hirata, Reis, Jurisica, Silbiger and Luchessi. This is an open-access article distributed under the terms of the Creative Commons Attribution License (CC BY). The use, distribution or reproduction in other forums is permitted, provided the original author(s) and the copyright owner(s) are credited and that the original publication in this journal is cited, in accordance with accepted academic practice. No use, distribution or reproduction is permitted which does not comply with these terms.
*Correspondence: Vivian Nogueira Silbiger dml2aWFuLnNpbGJpZ2VyQHVmcm4uYnI=