- 1Department of Medicine, Jackson Memorial Hospital, University of Miami, Miami, FL, United States
- 2Division of Cardiovascular Medicine, University of Miami Health System, Miami, FL, United States
Heart failure is a clinical syndrome caused by structural cardiac abnormalities that lead to increased intracardiac pressures and decreased cardiac output. Following cardiovascular insult or direct myocardial injury, neurohormonal activation triggers hemodynamic changes and cardiac remodeling to preserve cardiac output. While initially adaptive, cardiac remodeling eventually causes pathologic changes in cardiac structure that often compromise cardiac function. Reverse remodeling is the regression of abnormal cardiac chamber geometry and function after myocardial injury. In recent years, several classes of therapeutics have been associated with greater likelihood of reverse remodeling. Heart failure recovery and heart failure remission, terms encompassing the clinical correlates of reverse remodeling, have been associated with improved survival in patients with heart failure with reduced ejection. As such, identifying predictors of heart failure recovery can have important implications for guiding clinical practice and therapeutic innovation. This review addresses the role of biomarkers and imaging monitoring in predicting structural, functional, and clinical recovery in patients with acute and chronic heart failure.
Introduction
Heart failure (HF) is a dynamic condition with varied clinical presentations and sequelae depending on factors such as etiology, concomitant conduction disease, pharmacologic management, and associated comorbidities (1, 2). For example, patients with heart failure with reduced ejection fraction (HFrEF) may progress to end-stage HF requiring advanced HF therapies or they may recover and be reclassified as patients with heart failure with recovered ejection fraction (HfrecEF) (3). Individuals with HF due to ischemic cardiomyopathy tend to have a worse prognosis due to fundamental differences in functional myocardial muscle mass from prior infarction compared with those with non-ischemic cardiomyopathy (4). In patients with HF and concomitant conduction disease, cardiac resynchronization therapy (CRT) significantly increases survival (5). Similarly, individual drugs of certain classes improve morbidity and mortality in HF patients, while others within the class do not (6). Given the heterogeneity in disease presentation and progression, identifying predictors of HF recovery has important implications for guiding clinical practice.
HF recovery can be defined in many ways. An essential process in countering HF progression is reverse remodeling or regression of the molecular, cellular, and tissue adaptions underlying cardiac remodeling. Cardiac remodeling describes the synchronized genomic, molecular, cellular, and interstitial changes that manifest as changes in size, geometry, and function of the heart following insult or injury. Reverse remodeling describes normative changes in size, geometry, and function of a previously “failing” heart. It is understood to occur following the removal of the triggering injury and the institution of therapeutic interventions which are thought to promote salutary molecular, cellular and interstitial changes. On the macroscopic level, reverse remodeling can restore normal cardiac chamber geometry and function after myocardial injury, a process often described as recovery or remission (4). However, precise criteria for reverse remodeling, recovery, and remission are yet to be completely defined or standardized by either parameters or thresholds. Some studies define reverse remodeling as a reduction in left ventricular (LV) volume or diameter, also described as structural recovery. Other studies use an LV ejection fraction (LVEF) increase by a certain percentage or above a certain threshold, which is described as functional recovery (7). Mortality reduction and improved function are well-recognized as critical clinical outcomes in HF. Reverse remodeling/structural recovery is associated not only with improved myocardial functionality, but it is also associated with improved survival (8, 9). As such, either structural, functional, or clinical recovery will be considered HF recovery in this review.
Many studies have sought to determine prognostic indicators of reverse remodeling and clinical outcomes. Baseline clinical parameters, biomarkers, and imaging findings, such as non-ischemic cause, female sex, and baseline LVEF, show strong predictive value (10–16). While helpful, baseline considerations reflect a single timepoint and, therefore, cannot be used in real-time to track or predict disease progression to modify or personalize treatment plans. This review focuses on the role of serial biomarker measurements (Table 1) and imaging as predictors of structural, functional, and clinical HF recovery over time.
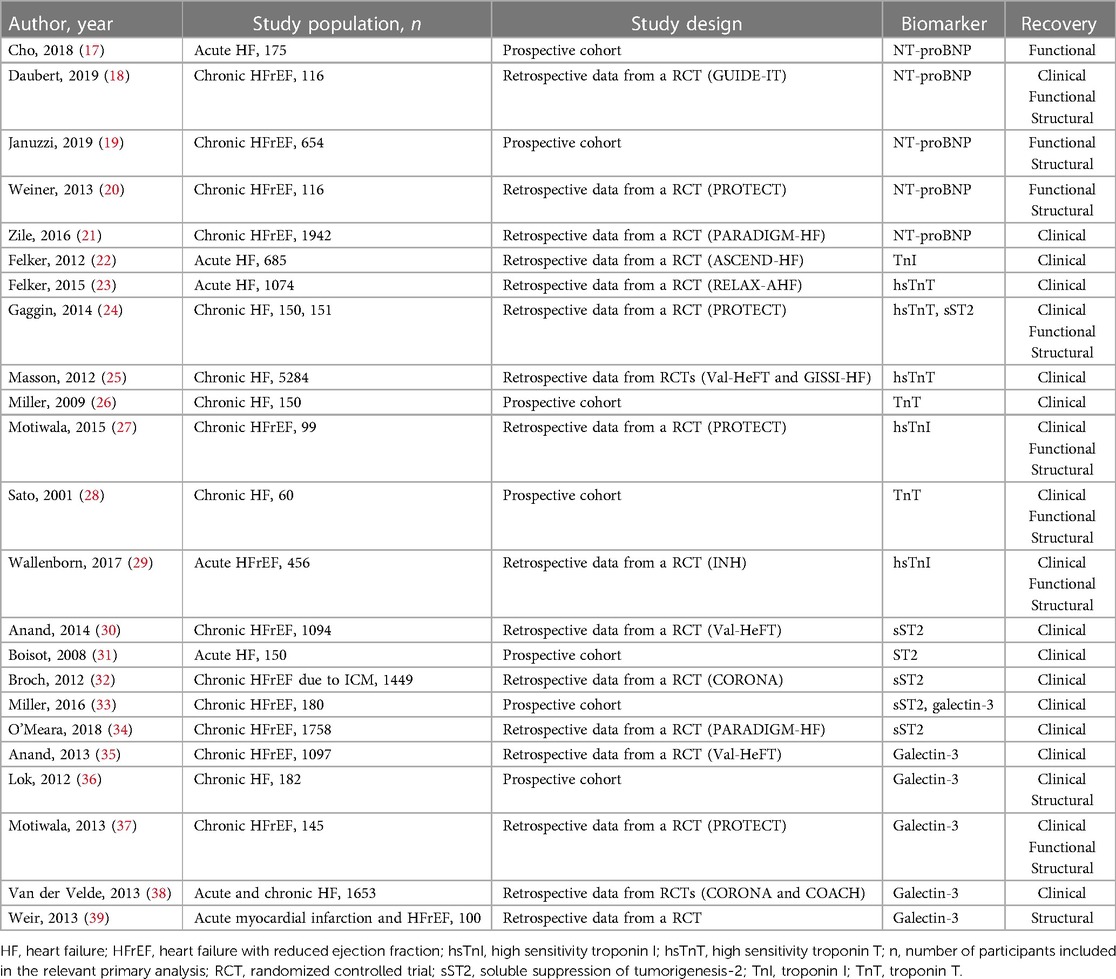
Table 1. Studies evaluating biomarker monitoring to predict structural, functional, and/or clinical recovery in HF patients.
Biomarker predictors
NT-proBNP
Natriuretic peptides, namely B-type natriuretic peptide (BNP) and N-terminal pro-B-type natriuretic peptide (NT-proBNP), are well-established HF biomarkers and indicate myocyte stretch and wall stress. Pro B-type natriuretic peptide is the precursor protein that is cleaved into equimolar concentrations of biologically active BNP and inert NT-proBNP. NT-proBNP circulates in higher plasma concentrations compared to BNP since the former primarily relies on passive renal excretion, whereas the latter is additionally cleared by peripheral receptors and enzymatic breakdown (18, 20). The conversion ratio of NT-proBNP to BNP depends on factors such as atrial fibrillation, age, and renal function but on average is around 6.25:1 (19). Studies evaluating the use of natriuretic peptides for serial monitoring of HF recovery have primarily utilized NT-proBNP and it is unknown whether the results can be extrapolated to BNP. Reduction in NT-proBNP has been associated with greater reverse remodeling and improved clinical outcomes in patients with HFrEF.
Reduction in NT-proBNP over time is a predictor of improved LV volumes and function. The PROTECT study aimed to determine whether NT-proBNP guided HF management to a NT-proBNP goal of <1,000 pg/ml was associated with reverse remodeling as evaluated by echocardiography. 151 participants were randomized to NT-proBNP guided vs. standard of care (SOC) HF management. Both groups saw improved echocardiographic parameters of cardiac structure and function with pharmacologic treatment. However, the effect sizes on all measures of LV volumes and function, some measures of diastolic function and estimated filling pressures, and all measures of right ventricular function were larger in the NT-proBNP guided therapy group compared to the SOC group (17). By the same token, the GUIDE-IT Echo substudy evaluated the effect of achievement of an NT-proBNP goal of <1,000 pg/ml on cardiac structure and function in 116 patients. Patients who achieved the NT-proBNP goal had significantly reduced LV volumes, increased left ventricular ejection fraction (LVEF), and improved global longitudinal strain (GLS) compared with patients who did not reach the NT-proBNP goal. The greater the absolute decrease in NT-proBNP level, the greater the impact on left ventricular volumes (21). Published in the same year as the GUIDE-IT Echo substudy, the PROVE-HF study showed that reduction in NT-proBNP after sacubitril-valsartan use was weakly correlated with improved cardiac structure and function at 6- and 12-months follow-up. There were 654 study participants who completed the study. Interestingly, most of the NT-proBNP reduction was observed 2 weeks after medication initiation when most patients received the lowest dose. Significant improvement in left-sided cardiac volumes and LVEF on echocardiography was observed as early as 6 months and the effect continued until 12 months (28). While the previously mentioned studies followed patients at clinic visits over a period of months, one study looked at NT-proBNP change during one hospitalization and found that NT-proBNP change >1633.5 pg/ml from admission to discharge was a predictor of LV functional recovery (27).
The structural changes associated with NT-proBNP reduction appear to translate into improved clinical outcomes. In the GUIDE-IT Echo cohort, patients who achieved the NT-proBNP goal also had a significant reduction in a composite endpoint of death and HF hospitalization after 12 months. However, it is important to note that the patients who failed to achieve the NT-proBNP goal were significantly more likely to have ischemic heart disease and other comorbidities that may have confounded the results (21). Patients from the PARADIGM-HF trial who had a reduction in NT-proBNP also had lower rates of cardiovascular mortality and HF hospitalization and this relationship was independent of the treatment group (29). These studies, taken together, demonstrate that serial NT-proBNP measurements can help predict structural, functional, and clinical recovery in HF patients.
Troponin
Cardiac troponins (cTn) are well-established markers of myocyte injury and are commonly used to diagnose ischemic cardiovascular events. In patients with chronic HF, persistently elevated troponin concentrations are associated with adverse remodeling and increased mortality (22, 24). While the increase in cTn over time is consistently associated with worse clinical outcomes in HF patients, cTn change appears to be an inconsistent predictor of reverse remodeling.
Studies evaluating cTn change based on the categorization of serial measurements show that HF patients with decreased or persistently low cTn levels at follow-up have significantly improved structural and functional recovery compared to those with increased or persistently high cTn levels. An early study performed in 60 patients with idiopathic dilated cardiomyopathy categorized patients based on their troponin T (TnT) pattern (TnT <0.02 ng/ml throughout the study, TnT ≥0.02 ng/ml initially and then <0.02 ng/ml during follow-up, TnT ≥0.02 ng/ml initially and during follow-up). Patients with TnT <0.02 ng/ml during follow-up had significantly decreased left ventricular diastolic dimension (LVDd) and increased LVEF compared to those with TnT at or above the threshold. However, there was no significant correlation between changes in TnT and changes in LVDd or LVEF (24). Post hoc analysis from the Interdisciplinary Network Heart Failure (INH) study examined hsTnI change as a prognostic marker during the transition from hospitalization for acute decompensated heart failure (ADHF) to chronic heart failure. 456 of the original 875 patients were included in the study. Baseline hsTnI levels measured before discharge and follow-up hsTnI levels measured after 6 months were categorized into tertiles. Patients had hsTnI decrease by at least one tertile (37%), increase by at least one tertile (11%), or remain in the same tertile (52%). At the 6-month follow-up, patients in all tertiles had significant improvement in LVEF. However, LV end-diastolic diameter and systolic tricuspid valve gradient only improved in patients who had hsTnI decrease to or remain in the lowest tertile, suggesting a possible interplay between the degree of myocyte injury and the capacity for cardiac reverse remodeling (23).
Studies evaluating cTn change in HF patients based on time spent in response relative to thresholds show a weak or no effect on cardiac reverse remodeling. Post hoc analyses from the PROTECT trial have examined the predictive value of serially measured high-sensitivity troponins for LV reverse remodeling in patients with HFrEF (22, 26). Participants were scheduled for clinic visits every 3 months up to 12 months, with blood samples collected and frozen at each visit for future biomarker measurement. In one analysis using high-sensitivity troponin I (hsTnI) concentration, 99 of the original 151 patients were included. The median hsTnI concentration for all subjects at baseline (10.9 pg/ml) was used as a threshold to assess the relative percentage of time spent at or below the threshold. While there was no significant correlation with the absolute or relative change in LV volumes, time spent with hsTnI ≤10.9 pg/ml was weakly correlated with the absolute and relative change in LVEF (22). On the other hand, another analysis using a previously defined hsTnT threshold (14 pg/ml) in 150 of the original 151 patients found no significant relationship between time spent at or below threshold and LVEF, LV end-systolic volume index, or LV end-diastolic volume index (26). The difference in results between the two analyses may be explained by the different biomarker thresholds used or by the lack of adjustment for significant differences between groups in the former analysis, as patients with higher troponin levels were older and had worse HF symptoms, worse renal function, and higher baseline NT-proBNP concentrations (22, 26).
Change in cTn is a predictor of clinical outcomes in patients with acute and chronic HF. In three of the previously described studies, patients with persistently high TnT concentrations had increased mortality rates (24); patients with greater time in hsTnI response had decreased incidence of cardiovascular events (22); and patients with high follow-up hsTnI levels had increased cardiovascular re-hospitalization (23). Serial TnI levels were measured on admission and again 48–72 h after in 685 of the original 7,141 ADHF participants in the ASCEND-HF trial. Relative TnI increase ≥20% was a significant predictor of 30-day mortality. Additionally, 48–72 h TnI level was a better predictor of 30-day mortality than baseline TnI level (25). The RELAX-AHF trial was also conducted in patients hospitalized with ADHF. HsTnT levels were measured in the 1,074 participants on admission and days 2, 5, and 14. Higher peak hsTnT and greater peak change were significantly associated with increased 180-day cardiovascular mortality (31). In the INH cohort, patients in whom hsTnI increased or remained in the highest tertile (13%) at 6 months post-discharge had the highest one-year cardiovascular hospitalization rate. In contrast, patients in whom hsTnI decreased or remained in the lowest tertile (60%) had better clinical outcomes (23). A prospective cohort study including 150 HF patients routinely measured TnT levels every 3 months over 2 years. Ambulatory patients with more frequent or persistent troponin elevation had a higher risk of death and cardiac transplantation (32). Lastly, 5,284 chronic HF patients from the Val-HeHF and GISS-HF trials had hsTnT levels measured at randomization and after 4 or 3 months, respectively. Patients whose hsTnT increased above the upper limit of normal (13.5 ng/l) had an increased risk of mortality compared to those whose hsTnT remained below the cutoff. Furthermore, follow-up hsTnT was a slightly better predictor than baseline hsTnT (30).
Soluble St2
Soluble suppression of tumorigenesis-2 (sST2) is a less broadly utilized HF biomarker that reflects myocyte fibrosis and inflammation. However, SST2 change appears to be a reliable predictor of clinical outcomes in HF patients.
Increase in sST2 is a predictor of increased morbidity and mortality. A prospective study of 150 patients hospitalized for ADHF had blood samples collected daily up to 6 times between admission and discharge. Increased ST2 during hospitalization was associated with increased 90-day mortality (33). In a pre-specified substudy of the CORONA trial, which investigated the effect of rosuvastatin on HFrEF patients with ischemic cardiomyopathy, 1,449 of the original 5,011 participants were included who had sST2 measured at baseline and after 3 months. SST2 increase ≥15.5% over 3 months was weakly but significantly associated with increased cardiovascular death, nonfatal myocardial infarction, or stroke (34). The prognostic value of sST2 change was also examined in the Val-HeFT trial, which evaluated the addition of valsartan to standard HF therapy. 1,094 of the original 5,010 HFrEF patients had sST2 measured at baseline, 4 months, and 12 months. An increase in sST2 at 12 months was significantly associated with worse clinical outcomes (40). In the PROTECT cohort, an increase from sST2 ≤ 35 ng/ml to >35 ng/ml was associated with a significantly shorter time to first cardiovascular event, such as worsening HF, HF hospitalization, and cardiac death (26). Lastly, a study of 180 ambulatory HFrEF patients found that while change in sST2 level over time was not predictive, persistently elevated sST2 levels over time were prognostic in identifying increased risk of death or cardiac transplantation (36).
Decrease in sST2 has been associated with both improved and no impact on survival. In the previously mentioned cohort study of 150 ADHF patients, decreased ST2 predicted survival. Patients with ST2 decrease ≥15.5% during hospitalization had a 7% mortality rate, whereas those who did not achieve this threshold had a 33% mortality rate (33). Another study from the PARADIGM-HF trial evaluated 1,758 of the original 8,399 HFrEF patients. Serial sST2 measurements were collected at baseline, 1 month, and 8 months. Reductions in sST2 concentrations at 1 month were associated with reductions in cardiovascular death and HF hospitalization. Furthermore, changes in sST2 were linearly correlated with clinical outcomes, suggesting against the use of specific thresholds to evaluate this relationship (39). On the other hand, sST2 decrease was not associated with improved risk from the Val-HeFT trial. The degree of sST2 change also did not add further prognostic value beyond that of the follow-up value (40). This may be due to the fact that while sacubitril/valsartan was associated with sST2 reduction and improved clinical outcomes in PARADIGM-HF, valsartan in Val-HeFT was associated with attenuation of the rate of rise in sST2 compared with the placebo group over 12 months. This difference may be another example of the potentiating effects of neprilysin inhibition with angiotensin receptor blockade that are still to be fully recognized.
Studies examining the impact of sST2 change on structural and functional HF recovery are sparse. A post hoc analysis of the PROTECT cohort showed that greater time with sST2 ≤ 35 ng/ml predicted a decrease in LV end-diastolic index (26).
Galectin-3
Galectin-3 is another marker of myocyte fibrosis and inflammation. It is unique from the previously described biomarkers in that baseline values have exhibited greater predictive value in heart failure with preserved ejection fraction (HFpEF) as opposed to HFrEF (37). High dose spironolactone (50 mg daily over 25 mg daily) has been shown to reduce galectin-3 concentrations (38). In contrast, valsartan did not lower galectin-3 levels (35). From the PROTECT study, no medication classes (beta blockers, angiotensin converting enzyme inhibitors, angiotensin receptor blockers, mineralocorticoid receptor antagonists, loop diuretics, or thiazide diuretics) significantly affected galectin-3 levels over time (41). Change in galectin-3 appears to be a poor predictor of structural recovery but a good predictor of clinical outcomes.
Change in galectin-3 does not appear to reflect change in LV volumes but may be associated with change in LVEF. Analysis of data from the DEAL-HF trial including 182 patients found no correlation between change in galectin-3 and change in LV volume at 3 or 12 months (42). Data from a randomized controlled trial of 100 patients designed to investigate the effect of eplerenone on reverse remodeling in HFrEF patients after acute myocardial infarction was also used to examine the relationship between galectin-3 and reverse remodeling over 6 months. Structural and functional cardiac parameters were assessed using cardiac magnetic resonance imaging. In line with findings from the DEAL-HF trial, there was no correlation between change in galectin-3 and change in any LV volume measurement (43). Not only was data from the PROTECT trial used to evaluate NT-proBNP, cTn, and sST2 monitoring, but it was also used to evaluate the utility of galectin-3 monitoring. Outcomes were assessed using the median baseline galectin-3 value (20 ng/ml) as a threshold. Neither baseline nor subsequent galectin-3 was associated with LV volume changes. However, a higher percentage of time with low galectin-3 (≤20 ng/ml) was associated with LVEF increase over time (41).
Galectin-3 increase appears to be a predictor of increased morbidity and mortality, but the converse may not be true. From the PROTECT trial, galectin-3 increase ≥15% was significantly associated with increased risk of cardiovascular events at 3, 6, and 9 months. Patients with persistently high galectin-3 levels had the most cardiovascular events, followed by low-to-high, high-to-low, and persistently low galectin-3 levels (41). Another study using data from two large trials (CORONA and COACH) had similar findings. A threshold advised by the US Food and Drug Administration (17.8 ng/ml) was used to divide patients into a low (<17.8 ng/ml) or high (>17.8 ng/ml) galectin-3 category. Patients with low-to-high galectin-3 had significantly increased all-cause mortality and HF hospitalization rates compared to patients with persistently low galectin-3. Conversely, patients with high-to-low galectin-3 had considerably decreased all-cause mortality and HF hospitalization rates compared to patients with persistently high galectin-3. Data analysis was also performed to corroborate these findings using percent change in galectin-3 levels. Galectin-3 increase ≥15% adjusted for baseline galectin-3 among other covariates was significantly associated with increased mortality risk and HF hospitalization compared with patients who stayed within 15% of their baseline galectin-3 level. Although galectin-3 decrease ≥15% was not associated with significant reduction in clinical outcomes (44). In addition to sST2 analysis, data from the Val-HeFT trial was used to evaluate galectin-3 use. Each 1 ng/ml increase in galectin-3 was associated with a 2.9% mortality rate increase at 4 months and 5.2% mortality rate increase at 12 months (35). On the other hand, change in galectin-3 levels was not a predictor of clinical outcomes in two previously mentioned studies (36, 42).
Imaging predictors
Echocardiography
Echocardiography is the first-line imaging modality for assessing cardiac chamber geometry and function. Myocardial deformation imaging is a newer echocardiographic technique using tissue Doppler-based or 2-dimensional speckle tracking-based methods to assess myocardial contractile function (45). Studies evaluating the use of serial echocardiography measurements to predict HF recovery are limited. A substudy of the EchoCRT trial included 614 of the original 809 HFrEF patients with narrow QRS width and ventricular dyssynchrony. Dyssynchrony was determined by echocardiographic findings of tissue Doppler-based longitudinal velocity delay ≥80 ms or speckle tracking-based radial strain delay ≥130 ms. Persistent or worsening dyssynchrony at 6-month follow-up was significantly associated with increased death and HF hospitalization, irrespective of CRT (46). A case report described the temporal pattern of strain parameters and LVEF over 5 time points in patients with HFrEF due to stress-induced cardiomyopathy. At HF presentation, the patient had a global longitudinal strain (GLS) of −6.2% and LVEF of 39%. Two days later, GLS improved to −10.7% with a relatively unchanged LVEF of 40%. Both GLS and LVEF eventually normalized at 3-month and 18-month follow-up. Early improvement in GLS preceded LV functional recovery, suggesting a potential role for GLS monitoring in patients with stress-induced cardiomyopathy to predict LV functional recovery (47).
Although not the focus of this review, GLS monitoring can detect and guide the management of chemotherapy-induced cardiotoxicity. Systematic reviews of echocardiography performed before, during, and after chemotherapy treatment support early reduction in GLS as a predictor of cardiotoxicity, defined as a decrease in LVEF or the development of HF (48, 49). A cohort study of 116 females with HER-2 positive breast cancer had patients undergo an echocardiogram at baseline and every 3 months to determine GLS and LVEF during chemotherapy treatment. A relative decrease in GLS by >15% of baseline was considered subclinical cardiac dysfunction, whereas LVEF reduction to <50% was considered overt HF. The use of GLS identified 27 patients with subclinical cardiac dysfunction when LVEF was still normal. These patients were started on concomitant beta-blockers and ACE-inhibitors, which allowed for the completion of chemotherapy without progression toward overt HF in 23 patients (50). By the same token, a randomized controlled trial of 307 patients treated with anthracycline-based chemotherapy compared GLS-guided vs. LVEF-guided initiation of cardioprotective treatment on LVEF at 12-month follow-up. Although there was no difference in LVEF between groups, the GLS-guided treatment arm had a significantly lower reduction in LVEF when only patients who received cardioprotective therapy were compared (51). Taken together, these findings suggest that GLS monitoring can be used to guide initiation of cardioprotective treatment during chemotherapy to prevent reduction in LVEF or discontinuation of cancer treatment. Studies evaluating the use of GLS monitoring to predict HF recovery are lacking.
Cardiac magnetic resonance imaging
Compared to echocardiography, cardiac magnetic resonance (CMR) imaging has less technician-dependent variation and is not limited by poor acoustic windows, making it a useful alternative or adjunct imaging modality. Specific sequences such as late gadolinium enhancement and T1-weighted are used to assess the presence and degree of myocardial fibrosis (52). Studies of serial CMR measurements in predicting HF recovery are also lacking. The absence of late gadolinium enhancement (LGE) at baseline is a strong independent predictor of reverse remodeling and improved clinical outcomes (53–57). All patients with LGE at baseline had LGE at follow-up and no patients without LGE developed LGE at follow-up, suggesting against a role for serial evaluation of LGE to predict recovery (54). However, more studies are needed to draw any conclusions regarding the role of CMR monitoring in HF recovery.
Conclusions
Reverse remodeling, recovery and remission are overlapping terms, sometimes used synonymously, that describe the regression of maladaptive cardiac structure and function alterations that occur in the setting of heart failure. NT-proBNP, cTn, sST2, and galectin-3 are cardiac biomarkers that may be useful in real-time to help presage the structural and functional changes associated with clinical recovery in heart failure patients and thus may help guide clinical management (Table 2, Central Illustration). Serial monitoring of NT-proBNP, cTn, sST2, and galectin-3 levels can help predict clinical outcomes in HF patients. Over time decreases in NT-proBNP (18, 21) and cTn (23, 24) are predictors of improved clinical recovery, while increases in cTn (22, 24, 26–29), sST2 (25, 30–32), and galectin-3 (38–40) over time are predictors of worse clinical recovery. Moreover, reduction in NT-proBNP is a strong predictor of structural and functional recovery (17–20). Whereas change in cTn (22–25) and galectin-3 (36–38) appear to be unclear and poor predictors of reverse remodeling, respectively. The role of sST2 monitoring in predicting reverse remodeling is promising but yet to be determined (25). Most published studies in imaging such as echocardiography and CMR have focused on the value of baseline measurements as predictive factors (53–60). The potential of serial imaging in predicting HF recovery is a subject for further inquiry. Given the strong association between reverse remodeling and improved clinical outcomes and survival, validated predictors of recovery could have great utility as clinically relevant intermediate surrogates. Accurate predictors of recovery could be beneficial in estimating prognosis, evaluating therapeutic efficacy, and optimizing care for individual patients.
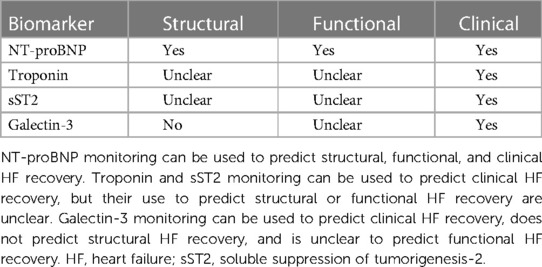
Table 2. Summary of the utility of biomarker monitoring to predict structural, functional, and clinical recovery in HF patients.
Author contributions
CY made substantial contributions to the design of the work, interpretation of data for the work, drafting the work, and revising it critically. LG made substantial contributions to the conception of the work and revising it critically for important intellectual content. All authors approve of the content for publication and agree to be accountable for all aspects of the work. All authors contributed to the article and approved the submitted version.
Funding
Funding to be received for open access publication fees from the University of Miami’s cardiovascular medicine department.
Conflict of interest
The authors declare that the research was conducted in the absence of any commercial or financial relationships that could be construed as a potential conflict of interest.
Publisher's note
All claims expressed in this article are solely those of the authors and do not necessarily represent those of their affiliated organizations, or those of the publisher, the editors and the reviewers. Any product that may be evaluated in this article, or claim that may be made by its manufacturer, is not guaranteed or endorsed by the publisher.
References
1. Lupón J, Gaggin HK, de Antonio M, Domingo M, Galán A, Zamora E, et al. Biomarker-assist score for reverse remodeling prediction in heart failure: the ST2-R2 score. Int J Cardiol. (2015) 184:337–43. doi: 10.1016/j.ijcard.2015.02.019
2. Wilcox JE, Fonarow GC, Yancy CW, Albert NM, Curtis AB, Heywood JT, et al. Factors associated with improvement in ejection fraction in clinical practice among patients with heart failure: findings from IMPROVE HF. Am Heart J. (2012) 163(1):49–56.e2. doi: 10.1016/j.ahj.2011.10.001
3. Wilcox JE, Fang JC, Margulies KB, Mann DL. Heart failure with recovered left ventricular ejection fraction: JACC scientific expert panel. J Am Coll Cardiol. (2020) 76(6):719–34. doi: 10.1016/j.jacc.2020.05.075
4. Kim GH, Uriel N, Burkhoff D. Reverse remodelling and myocardial recovery in heart failure. Nat Rev Cardiol. (2018) 15(2):83–96. doi: 10.1038/nrcardio.2017.139
5. Cleland JG, Daubert JC, Erdmann E, Freemantle N, Gras D, Kappenberger L, et al. The effect of cardiac resynchronization on morbidity and mortality in heart failure. N Engl J Med. (2005) 352(15):1539–49. doi: 10.1056/NEJMoa050496
6. Bao J, Kan R, Chen J, Xuan H, Wang C, Li D, et al. Combination pharmacotherapies for cardiac reverse remodeling in heart failure patients with reduced ejection fraction: a systematic review and network meta-analysis of randomized clinical trials. Pharmacol Res. (2021) 169:105573. doi: 10.1016/j.phrs.2021.105573
7. Aimo A, Fabiani I, Vergaro G, Arzilli C, Chubuchny V, Pasanisi EM, et al. Prognostic value of reverse remodelling criteria in heart failure with reduced or mid-range ejection fraction. ESC Heart Fail. (2021) 8(4):3014–25. doi: 10.1002/ehf2.13396
8. Wang K, Youngson E, Bakal JA, Thomas J, McAlister FA, Oudit GY. Cardiac reverse remodelling and health status in patients with chronic heart failure. ESC Heart Fail. (2021) 8(4):3106–18. doi: 10.1002/ehf2.13417
9. Kramer DG, Trikalinos TA, Kent DM, Antonopoulos GV, Konstam MA, Udelson JE. Quantitative evaluation of drug or device effects on ventricular remodeling as predictors of therapeutic effects on mortality in patients with heart failure and reduced ejection fraction: a meta-analytic approach. J Am Coll Cardiol. (2010) 56(5):392–406. doi: 10.1016/j.jacc.2010.05.011
10. Aimo A, Gaggin HK, Barison A, Emdin M, Januzzi JL Jr. Imaging, biomarker, and clinical predictors of cardiac remodeling in heart failure with reduced ejection fraction. JACC Heart Fail. (2019) 7(9):782–94. doi: 10.1016/j.jchf.2019.06.004
11. Aimo A, Vergaro G, González A, Barison A, Lupón J, Delgado V, et al. Cardiac remodelling - part 2: clinical, imaging and laboratory findings. A review from the study group on biomarkers of the heart failure association of the European society of cardiology. Eur J Heart Fail. (2022) 24(6):944–58. doi: 10.1002/ejhf.2522
12. Motiwala SR, Gaggin HK. Biomarkers to predict reverse remodeling and myocardial recovery in heart failure. Curr Heart Fail Rep. (2016) 13(5):207–18. doi: 10.1007/s11897-016-0303-y
13. Arad M, Nussbaum T, Blechman I, Feinberg MS, Koren-Morag N, Peled Y, et al. Prevalence and clinical predictors of reverse remodeling in patients with dilated cardiomyopathy. Isr Med Assoc J. (2014) 16(7):405–11. PMID: 25167684
14. Oka T, Inoue K, Tanaka K, Toyoshima Y, Isshiki T, Kimura T, et al. Duration of reverse remodeling response to cardiac resynchronization therapy: rates, predictors, and clinical outcomes. Int J Cardiol. (2017) 243:340–6. doi: 10.1016/j.ijcard.2017.05.058
15. Nita N, Scharnbeck D, Schneider LM, Seeger J, Wöhrle J, Rottbauer W, et al. Predictors of left ventricular reverse remodeling after percutaneous therapy for mitral regurgitation with the MitraClip system. Catheter Cardiovasc Interv. (2020) 96(3):687–97. doi: 10.1002/ccd.28779
16. Kobayashi M, Huttin O, Schikowski J, Bozec E, Zohra L, Frimat L, et al. Cardiovascular comorbidities are the main predictors of cardiac reverse remodeling following kidney transplantation. Cardiology. (2020) 145(2):71–6. doi: 10.1159/000504381
17. Weiner RB, Baggish AL, Chen-Tournoux A, Marshall JE, Gaggin HK, Bhardwaj A, et al. Improvement in structural and functional echocardiographic parameters during chronic heart failure therapy guided by natriuretic peptides: mechanistic insights from the ProBNP outpatient tailored chronic heart failure (PROTECT) study. Eur J Heart Fail. (2013) 15(3):342–51. doi: 10.1093/eurjhf/hfs180
18. van Kimmenade RR, Januzzi JL Jr, Bakker JA, Houben AJ, Rennenberg R, Kroon AA, et al. Renal clearance of B-type natriuretic peptide and amino terminal pro-B-type natriuretic peptide a mechanistic study in hypertensive subjects. J Am Coll Cardiol. (2009) 53(10):884–90. doi: 10.1016/j.jacc.2008.11.032
19. Rørth R, Jhund PS, Yilmaz MB, Kristensen SL, Welsh P, Desai AS, et al. Comparison of BNP and NT-proBNP in patients with heart failure and reduced ejection fraction. Circ Heart Fail. (2020) 13(2):e006541. doi: 10.1161/CIRCHEARTFAILURE.119.006541
20. Charles CJ, Espiner EA, Nicholls MG, Richards AM, Yandle TG, Protter A, et al. Clearance receptors and endopeptidase 24.11: equal role in natriuretic peptide metabolism in conscious sheep. Am J Physiol. (1996) 271(2 Pt 2):R373–80. doi: 10.1152/ajpregu.1996.271.2.R373
21. Daubert MA, Adams K, Yow E, Barnhart HX, Douglas PS, Rimmer S, et al. NT-proBNP Goal achievement is associated with significant reverse remodeling and improved clinical outcomes in HFrEF. JACC Heart Fail. (2019) 7(2):158–68. doi: 10.1016/j.jchf.2018.10.014
22. Motiwala SR, Gaggin HK, Gandhi PU, Belcher A, Weiner RB, Baggish AL, et al. Concentrations of highly sensitive cardiac troponin-I predict poor cardiovascular outcomes and adverse remodeling in chronic heart failure. J Cardiovasc Transl Res. (2015) 8(3):164–72. doi: 10.1007/s12265-015-9618-4
23. Wallenborn J, Marx A, Störk S, Güder G, Brenner S, Ertl G, et al. Prognostic significance of serial high-sensitivity troponin I measurements following acute cardiac decompensation-correlation with longer-term clinical outcomes and reverse remodelling. Int J Cardiol. (2017) 232:199–207. doi: 10.1016/j.ijcard.2017.01.021
24. Sato Y, Yamada T, Taniguchi R, Nagai K, Makiyama T, Okada H, et al. Persistently increased serum concentrations of cardiac troponin t in patients with idiopathic dilated cardiomyopathy are predictive of adverse outcomes. Circulation. (2001) 103(3):369–74. doi: 10.1161/01.CIR.103.3.369
25. Felker GM, Hasselblad V, Tang WHW, Hernandez AF, Armstrong PW, Fonarow GC, et al. Troponin I in acute decompensated heart failure: insights from the ASCEND-HF study. Eur J Heart Fail. (2012) 14(11):1257–64. doi: 10.1093/eurjhf/hfs110
26. Gaggin HK, Szymonifka J, Bhardwaj A, Belcher A, De Berardinis B, Motiwala S, et al. Head-to-head comparison of serial soluble ST2, growth differentiation factor-15, and highly-sensitive troponin T measurements in patients with chronic heart failure. JACC Heart Fail. (2014) 2(1):65–72. doi: 10.1016/j.jchf.2013.10.005
27. Cho JY, Kim KH, Song JE, Kim JE, Park H, Yoon HJ, et al. Predictors of left ventricular functional recovery and their impact on clinical outcomes in patients with newly diagnosed dilated cardiomyopathy and heart failure. Heart Lung Circ. (2018) 27(1):41–9. doi: 10.1016/j.hlc.2017.02.013
28. Januzzi JL J, Prescott MF, Butler J, Felker GM, Maisel AS, McCague K, et al. Association of change in N-terminal pro-B-type natriuretic peptide following initiation of sacubitril-valsartan treatment with cardiac structure and function in patients with heart failure with reduced ejection fraction. JAMA. (2019) 322(11):1085–95. doi: 10.1001/jama.2019.12821
29. Zile MR, Claggett BL, Prescott MF, McMurray JJ, Packer M, Rouleau JL, et al. Prognostic implications of changes in N-terminal pro-B-type natriuretic peptide in patients with heart failure. J Am Coll Cardiol. (2016) 68(22):2425–36. doi: 10.1016/j.jacc.2016.09.931
30. Masson S, Anand I, Favero C, Barlera S, Vago T, Bertocchi F, et al. Serial measurement of cardiac troponin T using a highly sensitive assay in patients with chronic heart failure: data from 2 large randomized clinical trials. Circulation. (2012) 125(2):280–8. doi: 10.1161/CIRCULATIONAHA.111.044149
31. Felker GM, Mentz RJ, Teerlink JR, Voors AA, Pang PS, Ponikowski P, et al. Serial high sensitivity cardiac troponin T measurement in acute heart failure: insights from the RELAX-AHF study. Eur J Heart Fail. (2015) 17(12):1262–70. doi: 10.1002/ejhf.341
32. Miller WL, Hartman KA, Burritt MF, Grill DE, Jaffe AS. Profiles of serial changes in cardiac troponin T concentrations and outcome in ambulatory patients with chronic heart failure. J Am Coll Cardiol. (2009) 54(18):1715–21. doi: 10.1016/j.jacc.2009.07.025
33. Boisot S, Beede J, Isakson S, Chiu A, Clopton P, Januzzi J, et al. Serial sampling of ST2 predicts 90-day mortality following destabilized heart failure. J Card Fail. (2008) 14(9):732–8. doi: 10.1016/j.cardfail.2008.06.415
34. Broch K, Ueland T, Nymo SH, Kjekshus J, Hulthe J, Muntendam P, et al. Soluble ST2 is associated with adverse outcome in patients with heart failure of ischaemic aetiology. Eur J Heart Fail. (2012) 14(3):268–77. doi: 10.1093/eurjhf/hfs006
35. Anand IS, Rector TS, Kuskowski M, Adourian A, Muntendam P, Cohn JN. Baseline and serial measurements of galectin-3 in patients with heart failure: relationship to prognosis and effect of treatment with valsartan in the Val-HeFT. Eur J Heart Fail. (2013) 15(5):511–8. doi: 10.1093/eurjhf/hfs205
36. Miller WL, Saenger AK, Grill DE, Slusser JP, Bayes-Genis A, Jaffe AS. Prognostic value of serial measurements of soluble suppression of tumorigenicity 2 and galectin-3 in ambulatory patients with chronic heart failure. J Card Fail. (2016) 22(4):249–55. doi: 10.1016/j.cardfail.2015.07.017
37. de Boer RA, Lok DJ, Jaarsma T, van der Meer P, Voors AA, Hillege HL, et al. Predictive value of plasma galectin-3 levels in heart failure with reduced and preserved ejection fraction. Ann Med. (2011) 43(1):60–8. doi: 10.3109/07853890.2010.538080
38. Tran SK, Ngo TH, Lai TT, Truong GK, Tran KDD, Vo PM, et al. Effectiveness of spironolactone in terms of galectin-3 levels in patients with heart failure with a reduced ejection fraction in the vietnamese population. Healthcare (Basel). (2023) 11(2):1–12. doi: 10.3390/healthcare11020253
39. O’Meara E, Prescott MF, Claggett B, Rouleau JL, Chiang LM, Solomon SD, et al. Independent prognostic value of Serum soluble ST2 measurements in patients with heart failure and a reduced ejection fraction in the PARADIGM-HF trial (prospective comparison of ARNI with ACEI to determine impact on global mortality and morbidity in heart failure). Circ Heart Fail. (2018) 11(5):e004446. doi: 10.1161/CIRCHEARTFAILURE.117.004446
40. Anand IS, Rector TS, Kuskowski M, Snider J, Cohn JN. Prognostic value of soluble ST2 in the valsartan heart failure trial. Circ Heart Fail. (2014) 7(3):418–26. doi: 10.1161/CIRCHEARTFAILURE.113.001036
41. Motiwala SR, Szymonifka J, Belcher A, Weiner RB, Baggish AL, Sluss P, et al. Serial measurement of galectin-3 in patients with chronic heart failure: results from the ProBNP outpatient tailored chronic heart failure therapy (PROTECT) study. Eur J Heart Fail. (2013) 15(10):1157–63. doi: 10.1093/eurjhf/hft075
42. Lok DJ, Lok SI, Bruggink-André de la Porte PW, Badings E, Lipsic E, van Wijngaarden J, et al. Galectin-3 is an independent marker for ventricular remodeling and mortality in patients with chronic heart failure. Clin Res Cardiol. (2013) 102(2):103–10. doi: 10.1007/s00392-012-0500-y
43. Weir RA, Petrie CJ, Murphy CA, Clements S, Steedman T, Miller AM, et al. Galectin-3 and cardiac function in survivors of acute myocardial infarction. Circ Heart Fail. (2013) 6(3):492–8. doi: 10.1161/CIRCHEARTFAILURE.112.000146
44. van der Velde AR, Gullestad L, Ueland T, Aukrust P, Guo Y, Adourian A, et al. Prognostic value of changes in galectin-3 levels over time in patients with heart failure: data from CORONA and COACH. Circ Heart Fail. (2013) 6(2):219–26. doi: 10.1161/CIRCHEARTFAILURE.112.000129
45. Shah AM, Solomon SD. Myocardial deformation imaging. Circulation. (2012) 125(2):e244–e8. doi: 10.1161/CIRCULATIONAHA.111.086348
46. Gorcsan J 3rd, Sogaard P, Bax JJ, Singh JP, Abraham WT, Borer JS, et al. Association of persistent or worsened echocardiographic dyssynchrony with unfavourable clinical outcomes in heart failure patients with narrow QRS width: a subgroup analysis of the EchoCRT trial. Eur Heart J. (2016) 37(1):49–59. doi: 10.1093/eurheartj/ehv418
47. Tibrewala A, Freed BH, Akhter N. Importance of temporal changes in myocardial strain in Takotsubo cardiomyopathy. BMJ Case Rep. (2017) 2017:1–4. doi: 10.1136/bcr-2017-220719
48. Oikonomou EK, Kokkinidis DG, Kampaktsis PN, Amir EA, Marwick TH, Gupta D, et al. Assessment of prognostic value of left ventricular global longitudinal strain for early prediction of chemotherapy-induced cardiotoxicity: a systematic review and meta-analysis. JAMA Cardiol. (2019) 4(10):1007–18. doi: 10.1001/jamacardio.2019.2952
49. Thavendiranathan P, Poulin F, Lim KD, Plana JC, Woo A, Marwick TH. Use of myocardial strain imaging by echocardiography for the early detection of cardiotoxicity in patients during and after cancer chemotherapy: a systematic review. J Am Coll Cardiol. (2014) 63(25 Pt A):2751–68. doi: 10.1016/j.jacc.2014.01.073
50. Santoro C, Esposito R, Lembo M, Sorrentino R, De Santo I, Luciano F, et al. Strain-oriented strategy for guiding cardioprotection initiation of breast cancer patients experiencing cardiac dysfunction. Eur Heart J Cardiovasc Imaging. (2019) 20(12):1345–52. doi: 10.1093/ehjci/jez194
51. Thavendiranathan P, Negishi T, Somerset E, Negishi K, Penicka M, Lemieux J, et al. Strain-guided management of potentially cardiotoxic cancer therapy. J Am Coll Cardiol. (2021) 77(4):392–401. doi: 10.1016/j.jacc.2020.11.020
52. Karamitsos TD, Arvanitaki A, Karvounis H, Neubauer S, Ferreira VM. Myocardial tissue characterization and fibrosis by imaging. JACC Cardiovasc Imaging. (2020) 13(5):1221–34. doi: 10.1016/j.jcmg.2019.06.030
53. Barison A, Aimo A, Ortalda A, Todiere G, Grigoratos C, Passino C, et al. Late gadolinium enhancement as a predictor of functional recovery, need for defibrillator implantation and prognosis in non-ischemic dilated cardiomyopathy. Int J Cardiol. (2018) 250:195–200. doi: 10.1016/j.ijcard.2017.10.043
54. Masci PG, Schuurman R, Andrea B, Ripoli A, Coceani M, Chiappino S, et al. Myocardial fibrosis as a key determinant of left ventricular remodeling in idiopathic dilated cardiomyopathy: a contrast-enhanced cardiovascular magnetic study. Circ Cardiovasc Imaging. (2013) 6(5):790–9. doi: 10.1161/CIRCIMAGING.113.000438
55. Becker MAJ, Cornel JH, van de Ven PM, van Rossum AC, Allaart CP, Germans T. The prognostic value of late gadolinium-enhanced cardiac magnetic resonance imaging in nonischemic dilated cardiomyopathy: a review and meta-analysis. JACC Cardiovasc Imaging. (2018) 11(9):1274–84. doi: 10.1016/j.jcmg.2018.03.006
56. Kida K, Yoneyama K, Kobayashi Y, Takano M, Akashi YJ, Miyake F. Late gadolinium enhancement on cardiac magnetic resonance images predicts reverse remodeling in patients with nonischemic cardiomyopathy treated with carvedilol. Int J Cardiol. (2013) 168(2):1588–9. doi: 10.1016/j.ijcard.2013.01.043
57. Ikeda Y, Inomata T, Fujita T, Iida Y, Nabeta T, Ishii S, et al. Cardiac fibrosis detected by magnetic resonance imaging on predicting time course diversity of left ventricular reverse remodeling in patients with idiopathic dilated cardiomyopathy. Heart Vessels. (2016) 31(11):1817–25. doi: 10.1007/s00380-016-0805-2
Keywords: heart failure recovery, reverse remodeling, predictors, biomarker monitoring, imaging monitoring, heart failure
Citation: Yan CL and Grazette L (2023) A review of biomarker and imaging monitoring to predict heart failure recovery. Front. Cardiovasc. Med. 10:1150336. doi: 10.3389/fcvm.2023.1150336
Received: 24 January 2023; Accepted: 24 March 2023;
Published: 6 April 2023.
Edited by:
Massimo Mapelli, Monzino Cardiology Center (IRCCS), ItalyReviewed by:
Kevin Shah, The University of Utah, United StatesIvica Bosnjak, Osijek Clinical Hospital Center, Croatia
© 2023 Yan and Grazette. This is an open-access article distributed under the terms of the Creative Commons Attribution License (CC BY). The use, distribution or reproduction in other forums is permitted, provided the original author(s) and the copyright owner(s) are credited and that the original publication in this journal is cited, in accordance with accepted academic practice. No use, distribution or reproduction is permitted which does not comply with these terms.
*Correspondence: Crystal Lihong Yan crystaly@med.miami.edu
†ORCID Crystal Lihong Yan orcid.org/0000-0003-1724-4231
Specialty Section: This article was submitted to Heart Failure and Transplantation, a section of the journal Frontiers in Cardiovascular Medicine