- 1Department of Pediatrics II (Neonatology), Medical University of Innsbruck, Innsbruck, Austria
- 2Department of Pediatrics I, Medical University of Innsbruck, Innsbruck, Austria
- 3Department of Pediatrics III (Cardiology), Medical University of Innsbruck, Innsbruck, Austria
- 4Department of Neurology, Medical University of Innsbruck, Innsbruck, Austria
- 5Department of Pediatrics, Bruneck Hospital, Bruneck, Italy
- 6Central Institute of Clinical Chemistry and Laboratory Medicine Medical University of Innsbruck, Innsbruck, Austria
Background: Homocysteine (Hcy) has been associated with an adverse cardiovascular risk profile in adolescents. Assessment of the association between plasma Hcy levels and clinical/laboratory factors might improve our understanding of the pathogenesis of cardiovascular disease.
Methods: Hcy was measured in 1,900 14- to 19-year-old participants of prospective population-based EVA-TYROL Study (44.3% males, mean age 16.4 years) between 2015 and 2018. Factors associated with Hcy were assessed by physical examination, standardized interviews, and fasting blood analysis.
Results: Mean plasma Hcy was 11.3 ± 4.5 µmol/L. Distribution of Hcy was characterized by extreme right skew. Males exhibited higher Hcy and sex differences increased with increasing age. Univariate associations with Hcy emerged for age, sex, body mass index, high-density lipoprotein cholesterol, and for factors pertaining to blood pressure, glucose metabolism, renal function, and diet quality, whereas the most important multivariate predictors of Hcy were sex and creatinine.
Discussion: Clinical and laboratory factors associated with Hcy in adolescents were manifold, with sex and high creatinine identified as strongest independent determinants. These results may aid when interpreting future studies investigating the vascular risk of homocysteine.
1. Introduction
Adolescence constitutes a critical developmental period during which adverse cardiovascular risk profiles are commonly acquired and consequently a window of opportunity for primary prevention of cardiovascular disease (CVD) (1). Adolescents are already vulnerable to and develop subclinical atherosclerosis upon exposure to traditional cardiovascular risk factors, including arterial hypertension, dyslipidemia, diabetes, and smoking (2). This relationship extends to the sulfur-containing amino acid homocysteine (Hcy), which possesses biological functions in methionine metabolism and associates with subclinical atherosclerosis in children with homocysteinuria (3) as well as community-dwelling, generally healthy youngsters (4–7).
Established causes of hyperhomocysteinemia (HHcy) in children and adolescents include genetic mutations interfering with methionine metabolism, deficiencies of folic acid, vitamin B-6, or vitamin B-12, and end-stage renal disease. Although the causal relation of Hcy to cardiovascular diseases remains uncertain (8, 9), HHcy has further been reported to be embedded within an adverse CVD risk profile in adolescents (10–13). The study objective was to investigate clinical and laboratory factors associated with plasma Hcy levels in a large cohort of community-dwelling adolescents as it may aid in understanding the pathogenesis of CVD and in assessing the vascular risk of homocysteine at this age.
2. Materials and methods
2.1. Study participants and design
The Early Vascular Aging (EVA) Tyrol Study is a prospective cohort study investigating CVD risk profiles in adolescents, conducted between May 2015 and July 2018 in the federal state of Tyrol, Austria, and the city of Bruneck, South Tyrol, Italy. Generally, all Tyrolean high and vocational schools were informed about the EVA-Tyrol study by the local education authority. Schools and local training companies were then able to apply or were additionally actively contacted and invited to participate in the study by the study team. The aim was to include whole school classes/all apprentices at a company rather than individual volunteers to guarantee an unbiased sample. Inclusion criteria were pupils in 10th grade (mean age 15–16 years) at baseline and 12th grade at follow-up/control group (mean ages 17–18 years). Schools and training companies were randomly assigned to either the intervention or the control group. Exclusion criteria were adolescents attending the military service and persons with impaired power of judgement. Prior disease was no reason for exclusion. Although the study administered a health intervention to a subgroup of participants, none of the participants included in the current analysis received any intervention; hence, the current analysis was observational. The study protocol has been published elsewhere (14). The EVA-Tyrol study was registered at ClinicalTrials.gov (ClinicalTrials.gov Identifier: NCT03929692), was approved by the local ethics committee of the Medical University of Innsbruck (approval number AN 2015–0005 345/4.13), and all the participants and their legal representatives gave informed consent.
2.2. Laboratory analysis
Blood samples were drawn after an overnight fast, immediately cooled, and laboratory parameters measured within 24 h after venipuncture. Heparin plasma tubes were used for laboratory measurement of total plasma Hcy and were immediately delivered to the testing facility (iso-certified central institute for medical and chemical laboratory-diagnostics – ZIMCL, Tirol Kliniken, Innsbruck, Austria). Homocysteine was analyzed by The ARCHITECT Homocysteine assay (Abbott Laboratories, Abbott Park, IL), which is a one-step immunoassay for the quantitative determination of total L-homocysteine in human plasma using chemiluminescence microparticle immunoassay technology. Dithiothreitol reduces bound or dimerised homocysteine (oxidized form) to free homocysteine. Free homocysteine is further converted to S-adenosyl homocysteine. The S-adenosyl homocysteine then contests with acridinium-labeled S-adenosyl cysteine for particle-bound monoclonal antibody. Chemiluminescence is resulting from a reaction mixture and measured as relative light units which is an indirect parameter for the amount of homocysteine in the sample (15). Total cholesterol, low-density cholesterol (LDL-C), high-density cholesterol (HDL-C), and creatinine were assessed by standard enzymatic colorimetric assays (Cobas 8,000, Roche Diagnostics, Rotkreuz, Switzerland). Glomerular filtration rate (GFR) was calculated using the Schwartz equation [GFR in ml/min per 1.73 m2 = (height in cm/creatinine in mg/dl) × 0.413] (16). Serum glucose and insulin were analyzed by a hexokinase method (Cobas 8,000, Roche Diagnostics, Rotkreuz, Switzerland). Measurements of glycated hemoglobin (HbA1c) were performed by high-pressure liquid chromatography (HA 8,180 T, Menarini Diagnostics, Florence, Italy). The Homeostatic Model Assessment for Insulin Resistance (HOMA) index was calculated as HOMA index = (fasting insulin [mIU/L] × fasting glucose [mmol/L]/22.5). Detailed descriptions of laboratory analysis methods have been published (14).
2.3. Genetic testing
Participants with very high homocysteine levels (>97th percentile of the empirical distribution corresponding to >20 µmol/L) were invited for further in-house check-up and genetic testing for the MTHFR polymorphism. Genomic DNA was extracted from EDTA blood samples. The MTHFR-polymorphism (NM_005957.4) c.665C > T (p.Ala222Val; rs1801133) was detected by PCR amplification and Sanger sequencing from genomic DNA using standard PCR protocol (GoTaq G2 DNA Polymerase, Promega) and sequence specific primer for exon 5 (fwd: 5′-TCCCTGTGGTCTCTTCATCC-3′; rev: 5′-CTGGGAAGAACTCAGCGAAC-3′). Sequence analysis was performed using the SeqPilot® software (JSI medical systems GmbH, Ettenheim, Germany).
2.4. Anthropometry
Weight and height were measured using medical precision scales and a Harpenden stadiometer (Holtain, Crymych, United Kingdom). Body mass index (BMI) was calculated as the ratio of weight in kg divided by the square of height in meters. Systolic and diastolic blood pressures were measured on both arms after a 5-minute seated rest (automated oscillometric device OMRON M4-I, Omron Healthcare Co., Lake Forest, IL) and the mean of three measurements was used. BMI and blood pressure were assessed using sex- and age-specific grow reference data (17, 18). We opted to use BMI as well as systolic and diastolic blood pressures instead of their z-scores because in our sample high correlations between raw variables and their age- and sex-dependent z-scores were observed.
2.5. Assessment of lifestyle risk factors
Lifestyle risk factors were assessed by in-person physician interviews and by lifestyle questionnaires. The adolescents were asked how many minutes of moderate or vigorous activity they do per day according to the American Heart Association definitions of ideal cardiovascular health. Smoking was also categorized according to the American Heart Association recommendations. Current smoking status was defined as non-smoker when never tried smoking or never smoked a whole cigarette and as smoker when tried prior 30 days or smoked regularly. Healthy diet was assessed using the Dietary Approach to Stop Hypertension (DASH) score (19). Moreover, dietary habits were evaluated by the Adolescent Food Habits Checklist (AFHC), which focuses on behavioral aspects of diet in adolescents (20).
2.6. Statistical analysis
Baseline characteristics of the study population are given as count (percentage) or mean ± standard deviation. The distribution of Hcy was non-normal by Shapiro–Wilk test and upon histogram inspection, with the latter indicating marked right-skew. Generalized additive models for location, scale, and shape (GAMLSS) were used to fit the age- and sex-conditional distribution of Hcy using the Box-Cox t distribution and fitting penalized varying coefficient terms for age individually by sex (21). Spearman correlation was used to investigate univariable associations of Hcy with demographic, anthropometric, lifestyle, hemodynamic, and complete blood count parameters. Multivariable independent associations of variables significantly univariably associated with Hcy were assessed using L1-regularized linear regression (the LASSO), using the regularization hyperparameter lambda that minimized 10-fold cross-validated mean squared error (22). For this analysis, Hcy was transformed towards normality by an inverse normal transform. As LASSO is focused on predictive performance, we complemented LASSO results with results from a conventional linear regression model including the predictors selected by LASSO to obtain confidence intervals and p-values. Analysis was conducted using R 4.2.1 (R Foundation for Statistical Computing, Vienna, Austria) and SPSS 27 (IBM, Chicago, USA). p-values are two-sided and an alpha level of 0.05 is used.
3. Results
The EVA-Tyrol study included 2,102 participants in its observational arm, 2,046 of which were at most 19 years old, and 1,900 had plasma Hcy measurements available and formed the study population. Participants were on average 16.4 ± 1.10 (mean ± standard deviation) years old and 44.3% were male. Characteristics of the study population are shown in Table 1.
Plasma Hcy showed a distinct distribution characterized by extreme right-skew (Figure 1). Average Hcy was 11.3 ± 4.48 µmol/L, and males featured higher Hcy than females overall and among subjects with lower Hcy ≤ 97th percentile (corresponding to 20 µmol/L) as well as among subjects with Hcy > 97th percentile. The sex difference was accentuated at the high range of Hcy, and increased with age (Figure 2). Although males showed a larger increase during the course of adolescence, in both males and females the increase slowed around age 16 (Figure 2). Reference percentiles for Hcy are shown in Table 2.
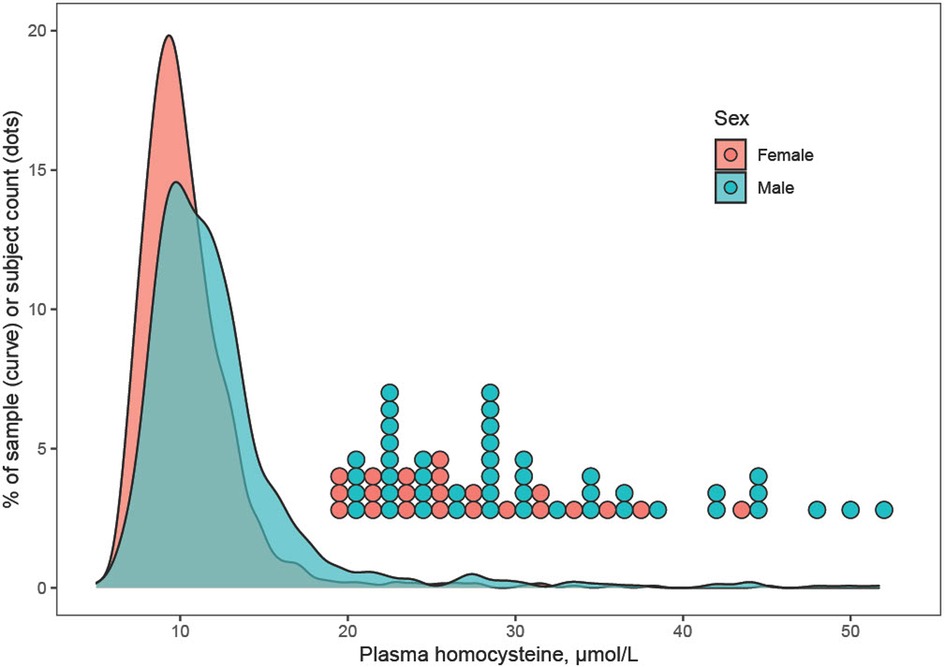
Figure 1. Distribution of plasma homocysteine by sex. The distribution of homocysteine (Hcy) showed extreme right-skew, which may indicate a mixture distribution including carriers of methylenetetrahydrofolate reductase or other mutations that associate with elevated Hcy. Males tended to feature higher Hcy than females, and this was true for subjects with Hcy ≤ 97th percentile as well as for subjects with very high Hcy > 97th percentile (corresponding to >20 µmol/L). For the latter, individual data points are shown as a dotplot histogram.
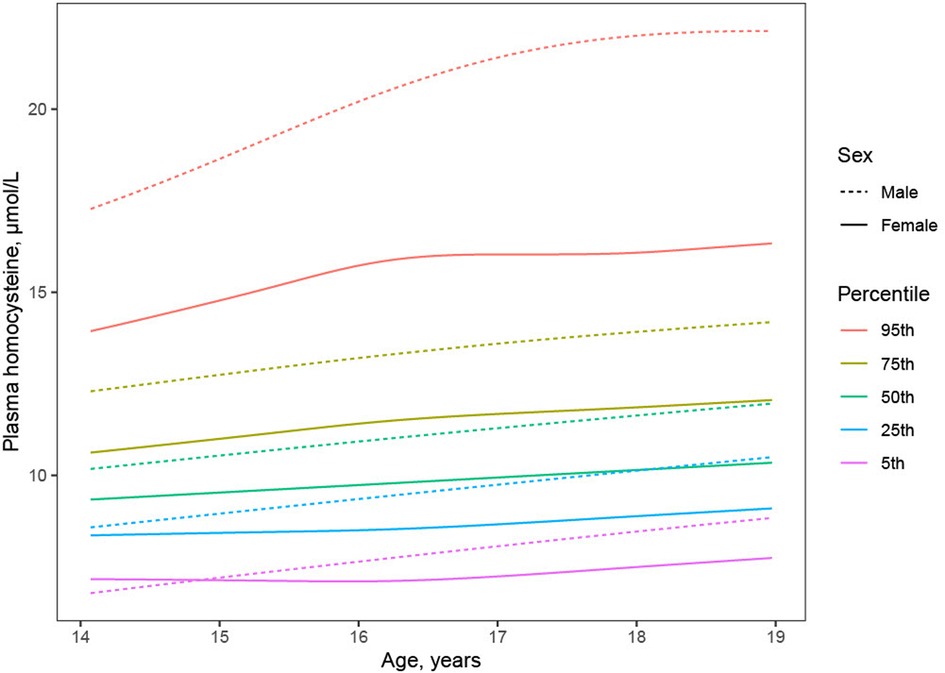
Figure 2. Trajectory of plasma homocysteine during adolescence by sex. Although males (dashed lines) compared to females (solid lines) showed higher average Hcy (black lines) throughout adolescence, investigation of Hcy percentiles conditional on age (colored lines) revealed higher male Hcy at the high end of the distribution at all ages, faster increase of Hcy in males than in females, and plateauing of Hcy in females but not in males around age 16.
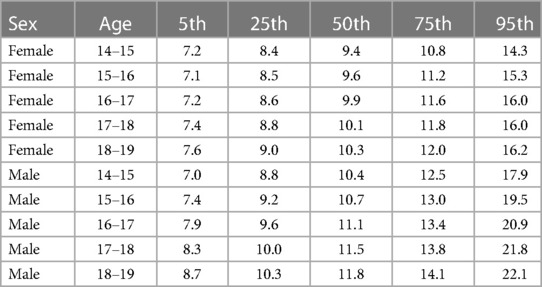
Table 2. Percentiles of total plasma homocysteine levels in µmol/L according to sex and age in years.
Factors associated with Hcy were numerous among the candidate clinical and laboratory parameters examined (Table 3). Direct associations with Hcy emerged for age, creatinine, BMI, systolic and diastolic BP, whereas inverse associations were detected for GFR and HDL-C as well as laboratory measures of glucose metabolism (HbA1c, insulin, and HOMA-IR). Better diet quality as assessed by the DASH and AFHC scores was likewise inversely associated with Hcy, and Hcy was lower with higher fruit intake but higher with higher meat and sweet beverage intake (Table 3).
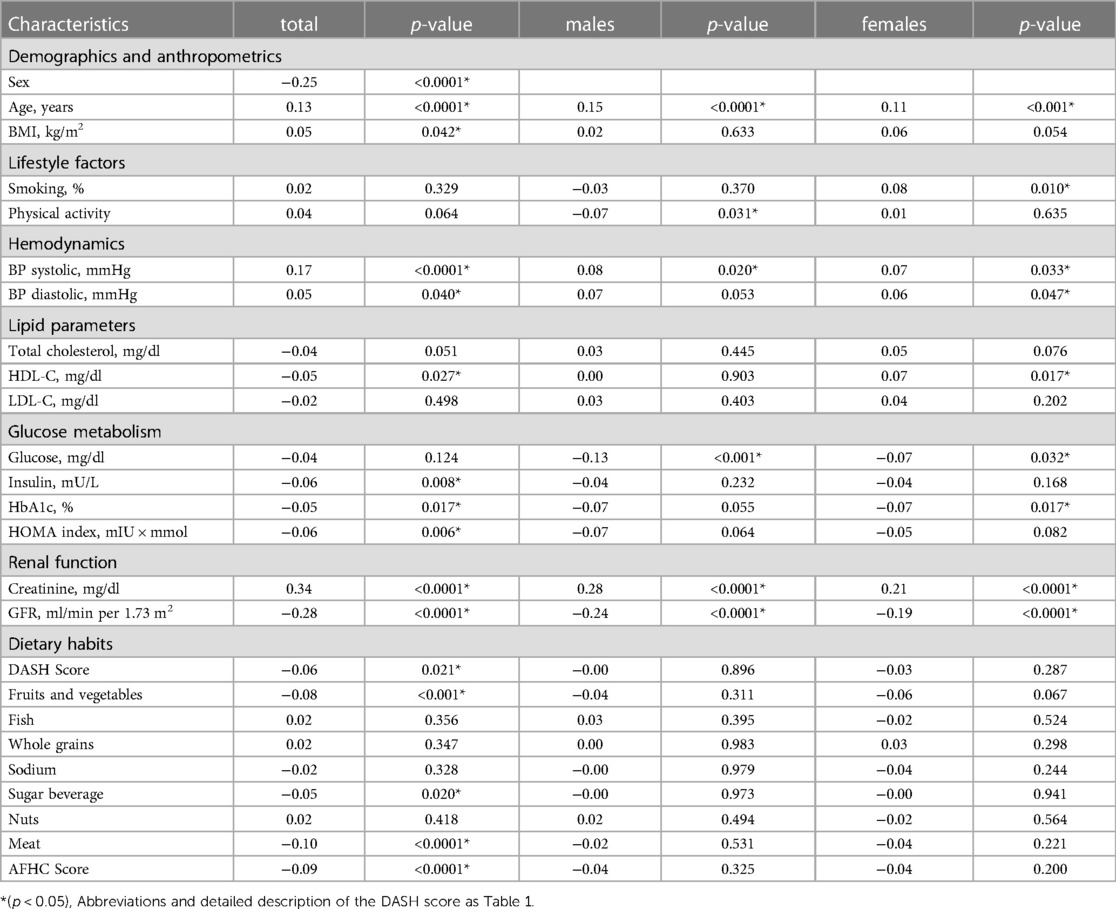
Table 3. Univariate spearman correlation analysis of homocysteine with clinical/laboratory characteristics.
Joint independent associated factors with Hcy were determined using L1-regularized linear regression after a normalizing transformation of the Hcy distribution. This analysis indicated that the strongest multivariable correlates of Hcy were sex and creatinine. Further variables selected by the LASSO were AFHC score, diastolic and systolic BP, HbA1c, HOMA index, age and sweet beverages, but these associations were not statistically significant (Table 4).
67 participants with Hcy above the 97th percentile were invited for further in-house check-up, 38 participated, with consent to genetic testing available for 13. None of these 13 were negative for the MTHFR polymorphism (c.665C > T), two were heterozygous, and 11 were homozygous.
4. Discussion
This large-scale observational study describes plasma Hcy distributions and trajectories during adolescence and defines its important univariable and multivariable associated factors. Key findings include (i) a distinct Hcy distribution characterized by extreme right-skew, (ii) sex-differential age trajectories of Hcy, and (iii) a paramount dependence of Hcy on sex and creatinine with lesser importance of a host of other anthropometric, lifestyle, dietary, and laboratory parameters.
The distribution of plasma Hcy was similar, and average Hcy moderately higher than previously reported in adolescent cohorts (Figure 1) (11, 23–25) and Hcy increased with age. Although increases of Hcy during adolescence, as during childhood and during adulthood have been reported, the current study describes a greater increase in males and a plateauing of Hcy in particular in subjects with high Hcy around age 16 (Figure 2), refining prior findings of higher Hcy levels in boys than in girls (4, 26, 27).
Possible mechanisms underlying sex differences in Hcy include the enzyme cysthathionine β synthase (CBS), a key enzyme in the transsulfuration pathway that facilitates conversion of Hcy to cysteine. Testosterone-dependent CBS downregulation may be one cause of higher Hcy in males (28, 29). Another cause may lie in creatine metabolism. Guanidinoacetate methyltransferase is an enzyme that metabolizes guanidinoacetate to creatine, resulting in higher Hcy production. Creatinine is further processed by nonenzymatic degration of creatine. Males, due to higher muscle mass, feature higher creatine turnover rates and consequently higher production of Hcy (11, 30). In line, we found a significant association of Hcy with creatinine (Table 3), which was among the strongest univariable and multivariable predictors of Hcy (Table 4).
Impaired renal clearance of Hcy further links creatinine and GFR to HHcy. Increases in Hcy have been demonstrated in children receiving renal replacement therapy and children with impaired renal function after kidney transplantation (31). A recent study investigated the relationship between homocysteine and serum uric acid in a 12- to 19-year-old cohort from the NHANES study in the U.S (32). Likewise, they found a strong correlation between low GFR and high homocysteine levels, however didn't investigate independent effects of GFR on homocysteine in this adolescent cohort. Yet, the Bogalusa Heart Study in community-based 24- to 42-year-old young adults found an independent adverse relationship of GFR with homocysteine (33). It can be cautiously assumed that independent effects of GFR on homocysteine might become apparent with decreasing GFR and with increasing age.
Further univariable associated factors with Hcy (Table 3) included systolic and diastolic BP, whereas inverse associations were detected for laboratory measures of glucose metabolism (HbA1c, insulin, and HOMA-IR) and dietary habits.
The associations of systolic and diastolic blood pressures with Hcy found here are consistent with prior studies in adolescent and adult cohorts (12, 13, 23, 34). Although one prior study reported a U-shaped relation between BP and Hcy, indicating that high as well as low Hcy may affect BP (11), we could not corroborate this finding (not shown). It has been hypothesized that high Hcy causes vascular dysfunction by inducing oxidative stress, followed by endothelial dysfunction and vascular remodeling resulting in arterial hypertension (35). Previous investigations have described a causal relationship of HHcy and subclinical early vessel pathologies in children with comorbidities such as obesity or hypertension (7, 36).
To the best of our knowledge, this study is the first to describe an inverse association between Hcy and HbA1c. So far, only one smaller cohort of 12- to 16-year old adolescents investigated HbA1c in relation to Hcy and found no significant relationship (12). We detected moderate univariate inverse correlations of Hcy with insulin and HOMA index. According to the literature, a relationship between Hcy and hyperinsulinemia is controversially debated (37, 38). Our findings stand in line with previous investigations, which demonstrated that acute hyperinsulinemia leads to a decrease of plasma Hcy levels in non-diabetic patients (39). In contrast, patients with insulin resistance and type 2 diabetes show higher homocysteine levels. It has been suggested that insulin modulates CBS activity, and modulation becomes disturbed in insulin resistance (40). This hypothesis would be consistent with the results of a study in Austrian obese children and adolescents that identified fat mass-associated hyperinsulinism as a contributor to HHcy (41).
So far, few studies have investigated the association between dietary habits and Hcy in adolescents (42, 43). Better diet quality as assessed by the DASH and AFHC scores was likewise inversely associated with Hcy, and Hcy was lower with higher fruit intake but higher with higher meat and sweet beverage intake (Table 3). This is in line with prior reports of amelioration of Hcy levels after modification of diet towards a DASH-style diet (44). The inverse association between Hcy and meat intake that we found is consistent with prior findings in adults and adolescents of a direct association between high intake of animal protein and HHcy. Methionine is the only dietary precursor of Hcy and is mainly found in red meats, poultry, fish, dairy products, and eggs. Hence, high methionine consumption may directly result in high Hcy levels (43, 45). In our study, low fruit and vegetable intake was associated with Hcy. Likewise, a beneficial effect of fruit and vegetable intake on Hcy levels has been described in adult cohorts, possibly explained by a higher intake of micronutrients, in particular folate (46). Overall, associations of dietary components with Hcy were modest. Further detailed analyses of micronutrient intakes and their relation to Hcy in this study cohort are planned.
Strengths of this study include the large, representative, well-characterized study population that was suited to determine plasma Hcy levels and associated factors in healthy community-dwelling adolescents. Limitations include that vitamin status of participants was unknown, although Hcy metabolism is known to depend on vitamins B-6, B-12 and on folate levels. Moreover, complete information on the MTHFR polymorphism, which is a predisposing factor for HHcy, was only available in a very small subset of our sample and is therefore not further discussed (47).
When addressing the cardiovascular risk of homocysteine reduced, free-oxidized and protein-bound forms of Hcy, cysteine and cysteinylglycerine referred to as redox thiol status should be considered in future studies (48). Studies in adults have shown that increased oxidative stress measured by the concentration of oxidized and reduced thiol markers might predict early atherosclerosis (49, 50). Hence, associations of oxidized and reduced forms of Hcy with cardiovascular risk factors should be further taken into account in adolescent cohort studies on the vascular risk of homocysteine.
In summary, this study defines clinical and laboratory factors associated with plasma Hcy levels in adolescents with sex and creatinine showing the strongest independent effects on Hcy. Some previously described risk factors of Hcy in pediatric and adult populations, including high systolic blood pressure and insulin resistance, could not be confirmed as independent associated factors in this cohort of healthy adolescents. Taken together, these results may aid when interpreting future studies on the vascular risk of homocysteine.
Data availability statement
The raw data supporting the conclusions of this article will be made available by the authors, without undue reservation.
Ethics statement
The studies involving human participants were reviewed and approved by the local ethics committee of the Medical University of Innsbruck (approval number AN 2015-0005 345/4.13). Written informed consent to participate in the study was provided by all the participants and their legal representatives.
Author contributions
NG and RP: analyzed data and drafted the manuscript. UK-K and MK: composed and conducted the study design. NG, CH, AS, KS, BB, SK, RG, UK-K and MK: were involved in participant recruitment, data collection and processing. AG: was responsible for laboratory blood analysis. All authors contributed to the article and approved the submitted version.
Funding
The Early Vascular Ageing (EVA) Tyrol Study is supported by the Excellence Initiative Competence Centers for Excellent Technologies (COMET) of the Austrian Research Promotion Agency FFG: “Research Center of Excellence in Vascular Ageing - Tyrol, VASCage” (K-Project No. 843536) funded by Bundesministerium für Verkehr, Innovation und Technologie, Bundesministerium für Wissenschaft, Forschung und Wirtschaft, Wirtschaftsagentur Wien and Standortagentur Tirol. The funders had no role in study design, data collection, analysis, decision to publish, or preparation of the manuscript.
Acknowledgment
We thank all the adolescents for participating in the study. The EVA study group members are attached as supplementary material. Special thanks goes to Martina Huemer for her intellectual contribution to this study.
Conflict of interest
The authors declare that the research was conducted in the absence of any commercial or financial relationships that could be construed as a potential conflict of interest.
Publisher's note
All claims expressed in this article are solely those of the authors and do not necessarily represent those of their affiliated organizations, or those of the publisher, the editors and the reviewers. Any product that may be evaluated in this article, or claim that may be made by its manufacturer, is not guaranteed or endorsed by the publisher.
Supplementary material
The Supplementary Material for this article can be found online at: https://www.frontiersin.org/articles/10.3389/fcvm.2023.1140990/full#supplementary-material
Abbreviations
AFHC, adolescent food habits checklist; BMI, body mass index; BP, blood pressure; CBS, cysthathione β synthase; CVD, cardiovascular disease; DASH, dietary approach to stop hypertension; EVA, early vascular aging; Hcy, homocysteine; HHcy, hyperhomocysteinemia; HDL-C, high-density cholesterol; HOMA, homeostatic model assessment for insulin resistance; LDL-C, low-density cholesterol; MTHFR, methylenetetrahydrofolate reductase.
References
1. Steinberger J, Daniels SR, Hagberg N, Isasi CR, Kelly AS, Lloyd-Jones D, et al. Cardiovascular health promotion in children: challenges and opportunities for 2020 and beyond: a scientific statement from the American heart association. Circulation. (2016) 134(12):e236–55. doi: 10.1161/CIR.0000000000000441
2. Berenson GS, Srinivasan SR, Bao W, Newman WP 3rd, Tracy RE, Wattigney WA. Association between multiple cardiovascular risk factors and atherosclerosis in children and young adults. The bogalusa heart study. N Engl J Med. (1998) 338(23):1650–6. doi: 10.1056/NEJM199806043382302
3. McCully KS. Vascular pathology of homocysteinemia: implications for the pathogenesis of arteriosclerosis. Am J Pathol. (1969) 56(1):111–28.5792556
4. Osganian SK, Stampfer MJ, Spiegelman D, Rimm E, Cutler JA, Feldman HA, et al. Distribution of and factors associated with serum homocysteine levels in children: child and adolescent trial for cardiovascular health. JAMA. (1999) 281(13):1189–96. doi: 10.1001/jama.281.13.1189
5. Welch GN, Loscalzo J. Homocysteine and atherothrombosis. N Engl J Med. (1998) 338(15):1042–50. doi: 10.1056/NEJM199804093381507
6. Knoflach M, Kiechl S, Penz D, Zangerle A, Schmidauer C, Rossmann A, et al. Cardiovascular risk factors and atherosclerosis in young women: atherosclerosis risk factors in female youngsters (arfy study). Stroke. (2009) 40(4):1063–9. doi: 10.1161/STROKEAHA.108.525675
7. Erkocoglu M, Ozon ZA, Gocmen R, Alikasifoglu A, Gonc N, Kandemir N. Carotid intima media thickness in adolescents with increased risk for atherosclerosis. Turk J Pediatr. (2013) 55(5):510–8.24382532
8. Marti-Carvajal AJ, Sola I, Lathyris D, Dayer M. Homocysteine-lowering interventions for preventing cardiovascular events. Cochrane Database Syst Rev. (2017) 8(8):CD006612. doi: 10.1002/14651858.CD006612.pub5
9. Smith AD, Refsum H. Homocysteine - from disease biomarker to disease prevention. J Intern Med. (2021) 290(4):826–54. doi: 10.1111/joim.13279
10. de Oliveira Leite L, Costa Dias Pitangueira J, Ferreira Damascena N, Ribas de Farias Costa P. Homocysteine levels and cardiovascular risk factors in children and adolescents: systematic review and meta-analysis. Nutr Rev. (2021) 79(9):1067–78. doi: 10.1093/nutrit/nuaa116
11. du Plessis JP, Nienaber-Rousseau C, Lammertyn L, Schutte AE, Pieters M, Kruger HS. The relationship of circulating homocysteine with fibrinogen, blood pressure, and other cardiovascular measures in African adolescents. J Pediatr. (2021) 234:158–63 e2. doi: 10.1016/j.jpeds.2021.03.034
12. Gillum RF. Distribution of total serum homocysteine and its association with parental history and cardiovascular risk factors at ages 12–16 years: the third national health and nutrition examination survey. Ann Epidemiol. (2004) 14(3):229–33. doi: 10.1016/S1047-2797(03)00242-4
13. Nygard O, Vollset SE, Refsum H, Stensvold I, Tverdal A, Nordrehaug JE, et al. Total plasma homocysteine and cardiovascular risk profile. The hordaland homocysteine study. JAMA. (1995) 274(19):1526–33. doi: 10.1001/jama.1995.03530190040032
14. Bernar B, Gande N, Stock KA, Staudt A, Pechlaner R, Geiger R, et al. The tyrolean early vascular ageing-study (eva-tyrol): study protocol for a non-randomized controlled trial: effect of a cardiovascular health promotion program in youth, a prospective cohort study. BMC Cardiovasc Disord. (2020) 20(1):59. doi: 10.1186/s12872-020-01357-9
15. Abbott, Abbott Architect System - Homocysteine (2008). Available at: http://www.ilexmedical.com/files/PDF/Homocysteine_ARC.pdf
16. Schwartz GJ, Munoz A, Schneider MF, Mak RH, Kaskel F, Warady BA, et al. New equations to estimate Gfr in children with Ckd. J Am Soc Nephrol. (2009) 20(3):629–37. doi: 10.1681/ASN.2008030287
17. Kromeyer-Hauschild K, Wabitsch M, Kunze D, Geller F, Geiß HC, Hesse V, et al. Perzentile für den Body-mass-Index für das Kindes- und Jugendalter unter Heranziehung verschiedener deutscher Stichproben. Monatsschr Kinderh. (2001) 149(8):807–18. doi: 10.1007/s001120170107
18. Neuhauser HK, Thamm M, Ellert U, Hense HW, Rosario AS. Blood pressure percentiles by age and height from nonoverweight children and adolescents in Germany. Pediatrics. (2011) 127(4):e978–88. doi: 10.1542/peds.2010-1290
19. Lloyd-Jones DM, Hong Y, Labarthe D, Mozaffarian D, Appel LJ, Van Horn L, et al. Defining and setting national goals for cardiovascular health promotion and disease reduction: the American heart association’s strategic impact goal through 2020 and beyond. Circulation. (2010) 121(4):586–613. doi: 10.1161/CIRCULATIONAHA.109.192703
20. Johnson F, Wardle J, Griffith J. The adolescent food habits checklist: reliability and validity of a measure of healthy eating behaviour in adolescents. Eur J Clin Nutr. (2002) 56(7):644–9. doi: 10.1038/sj.ejcn.1601371
21. Rigby RA, Stasinopoulos DM. Generalized additive models for location, scale and shape. Appl Statist. (2005) 54:507–54. doi: 10.1111/j.1467-9876.2005.00510.x
22. Hastie T, Tibshirani R, Friedman J. The elements of statistical learning: Data mining, inference, and prediction, 2nd Edn. New York: Springer Series in Statistics (2009). 68. doi: 10.1007/978-0-387-84858-7
23. Huemer M, Vonblon K, Fodinger M, Krumpholz R, Hubmann M, Ulmer H, et al. Total homocysteine, folate, and cobalamin, and their relation to genetic polymorphisms, lifestyle and body mass index in healthy children and adolescents. Pediatr Res. (2006) 60(6):764–9. doi: 10.1203/01.pdr.0000246099.39469.18
24. Kerr MA, Livingstone B, Bates CJ, Bradbury I, Scott JM, Ward M, et al. Folate, related B vitamins, and homocysteine in childhood and adolescence: potential implications for disease risk in later life. Pediatrics. (2009) 123(2):627–35. doi: 10.1542/peds.2008-1049
25. Gonzalez-Gross M, Benser J, Breidenassel C, Albers U, Huybrechts I, Valtuena J, et al. Gender and age influence blood folate, vitamin B12, vitamin B6, and homocysteine levels in European adolescents: the Helena study. Nutr Res. (2012) 32(11):817–26. doi: 10.1016/j.nutres.2012.09.016
26. Akanji AO, Thalib L, Al-Isa AN. Folate, vitamin B(1)(2) and total homocysteine levels in arab adolescent subjects: reference ranges and potential determinants. Nutr Metab Cardiovasc Dis. (2012) 22(10):900–6. doi: 10.1016/j.numecd.2010.10.020
27. Must A, Jacques PF, Rogers G, Rosenberg IH, Selhub J. Serum total homocysteine concentrations in children and adolescents: results from the third national health and nutrition examination survey (Nhanes Iii). J Nutr. (2003) 133(8):2643–9. doi: 10.1093/jn/133.8.2643
28. Vitvitsky V, Prudova A, Stabler S, Dayal S, Lentz SR, Banerjee R. Testosterone regulation of renal cystathionine beta-synthase: implications for sex-dependent differences in plasma homocysteine levels. Am J Physiol Renal Physiol. (2007) 293(2):F594–F600. doi: 10.1152/ajprenal.00171.2007
29. Prudova A, Albin M, Bauman Z, Lin A, Vitvitsky V, Banerjee R. Testosterone regulation of homocysteine metabolism modulates redox status in human prostate cancer cells. Antioxid Redox Signal. (2007) 9(11):1875–81. doi: 10.1089/ars.2007.1712
30. Rauh M, Verwied S, Knerr I, Dorr HG, Sonnichsen A, Koletzko B. Homocysteine concentrations in a German cohort of 500 individuals: reference ranges and determinants of plasma levels in healthy children and their parents. Amino Acids. (2001) 20(4):409–18. doi: 10.1007/s007260170037
31. Lilien M, Duran M, Van Hoeck K, Poll-The BT, Schroder C. Hyperhomocyst(E)Inaemia in children with chronic renal failure. Nephrol Dial Transplant. (1999) 14(2):366–8. doi: 10.1093/ndt/14.2.366
32. Shi Y, Wu Z, Wu J, Chen Z, Li P. Serum homocysteine level is positively correlated with Serum uric acid level in U.S. Adolescents: a cross sectional study. Front Nutr. (2022) 9:818836. doi: 10.3389/fnut.2022.818836
33. Ruan L, Chen W, Srinivasan SR, Xu J, Toprak A, Berenson GS. Plasma homocysteine is adversely associated with glomerular filtration rate in asymptomatic black and white young adults: the bogalusa heart study. Eur J Epidemiol. (2009) 24(6):315–9. doi: 10.1007/s10654-009-9340-0
34. Lim U, Cassano PA. Homocysteine and blood pressure in the third national health and nutrition examination survey, 1988–1994. Am J Epidemiol. (2002) 156(12):1105–13. doi: 10.1093/aje/kwf157
35. Steed MM, Tyagi SC. Mechanisms of cardiovascular remodeling in hyperhomocysteinemia. Antioxid Redox Signal. (2011) 15(7):1927–43. doi: 10.1089/ars.2010.3721
36. Zhu W, Huang X, Li M, Neubauer H. Elevated plasma homocysteine in obese schoolchildren with early atherosclerosis. Eur J Pediatr. (2006) 165(5):326–31. doi: 10.1007/s00431-005-0033-8
37. Budak N, Yazici C, Ozturk A, Bayram F, Mazicioglu MM, Kurtoglu S. Is plasma homocysteine level associated with metabolic syndrome components in adolescents? Metab Syndr Relat Disord. (2009) 7(4):357–62. doi: 10.1089/met.2008.0037
38. Bjorck J, Hellgren M, Rastam L, Lindblad U. Associations between serum insulin and homocysteine in a Swedish population-a potential link between the metabolic syndrome and hyperhomocysteinemia: the skaraborg project. Metab Clin Exp. (2006) 55(8):1007–13. doi: 10.1016/j.metabol.2006.03.010
39. Bar-On H, Kidron M, Friedlander Y, Ben-Yehuda A, Selhub J, Rosenberg IH, et al. Plasma total homocysteine levels in subjects with hyperinsulinemia. J Intern Med. (2000) 247(2):287–94. doi: 10.1046/j.1365-2796.2000.00592.x
40. Fonseca VA, Mudaliar S, Schmidt B, Fink LM, Kern PA, Henry RR. Plasma homocysteine concentrations are regulated by acute hyperinsulinemia in nondiabetic but not type 2 diabetic subjects. Metab Clin Exp. (1998) 47(6):686–9. doi: 10.1016/S0026-0495(98)90031-2
41. Gallistl S, Sudi K, Mangge H, Erwa W, Borkenstein M. Insulin is an independent correlate of plasma homocysteine levels in obese children and adolescents. Diabetes Care. (2000) 23(9):1348–52. doi: 10.2337/diacare.23.9.1348
42. Iglesia I, Huybrechts I, Gonzalez-Gross M, Mouratidou T, Santabarbara J, Chajes V, et al. Folate and vitamin B12 concentrations are associated with plasma dha and epa fatty acids in European adolescents: the healthy lifestyle in Europe by nutrition in adolescence (Helena) study. Br J Nutr. (2017) 117(1):124–33. doi: 10.1017/S0007114516004414
43. Lutsey PL, Steffen LM, Feldman HA, Hoelscher DH, Webber LS, Luepker RV, et al. Serum homocysteine is related to food intake in adolescents: the child and adolescent trial for cardiovascular health. Am J Clin Nutr. (2006) 83(6):1380–6. doi: 10.1093/ajcn/83.6.1380
44. Appel LJ, Miller ER 3rd, Jee SH, Stolzenberg-Solomon R, Lin PH, Erlinger T, et al. Effect of dietary patterns on serum homocysteine: results of a randomized, controlled feeding study. Circulation. (2000) 102(8):852–7. doi: 10.1161/01.CIR.102.8.852
45. Xiao Y, Zhang Y, Wang M, Li X, Xia M, Ling W. Dietary protein and plasma total homocysteine, cysteine concentrations in coronary angiographic subjects. Nutr J. (2013) 12(1):144. doi: 10.1186/1475-2891-12-144
46. Gao X, Bermudez OI, Tucker KL. Plasma C-reactive protein and homocysteine concentrations are related to frequent fruit and vegetable intake in hispanic and non-hispanic white elders. J Nutr. (2004) 134(4):913–8. doi: 10.1093/jn/134.4.913
47. Cortese C, Motti C. Mthfr gene polymorphism, homocysteine and cardiovascular disease. Public Health Nutr. (2001) 4(2B):493–7. doi: 10.1079/PHN2001159
48. Ueland PM, Mansoor MA, Guttormsen AB, Muller F, Aukrust P, Refsum H, et al. Reduced, oxidized and protein-bound forms of homocysteine and other aminothiols in plasma comprise the redox thiol status–a possible element of the extracellular antioxidant defense system. J Nutr. (1996) 126(4 Suppl):1281S–4S. doi: 10.1093/jn/126.suppl_4.1281S
49. Patel RS, Ghasemzadeh N, Eapen DJ, Sher S, Arshad S, Ko YA, et al. Novel biomarker of oxidative stress is associated with risk of death in patients with coronary artery disease. Circulation. (2016) 133(4):361–9. doi: 10.1161/CIRCULATIONAHA.115.019790
Keywords: cardiovascular risk factors, homocysteine, epidemiology, adolescents, health prevention
Citation: Gande N, Hochmayr C, Staudt A, Bernar B, Stock K, Kiechl SJ, Geiger R, Griesmacher A, Scholl-Bürgi S, Knoflach M, Pechlaner R, Kiechl-Kohlendorfer U and the (Early Vascular Ageing) EVA Study Group (2023) Plasma homocysteine levels and associated factors in community-dwelling adolescents: the EVA-TYROL study. Front. Cardiovasc. Med. 10:1140990. doi: 10.3389/fcvm.2023.1140990
Received: 31 January 2023; Accepted: 1 June 2023;
Published: 23 June 2023.
Edited by:
Dennis W. T. Nilsen, Stavanger University Hospital, NorwayReviewed by:
Nataša Marčun Varda, Maribor University Medical Centre, SloveniaMostafa Waly, Sultan Qaboos University, Oman
Priscila Ribas De Farias Costa, Federal University of Bahia (UFBA), Brazil
Luana Leite, Federal University of Bahia (UFBA), Brazil, in collaboration with reviewer PRFC
© 2023 Gande, Hochmayr, Staudt, Bernar, Stock, Kiechl, Geiger, Griesmacher, Scholl-Bürgi, Knoflach, Pechlaner, Kiechl-Kohlendorfer and the (Early Vascular Ageing) EVA Study Group. This is an open-access article distributed under the terms of the Creative Commons Attribution License (CC BY). The use, distribution or reproduction in other forums is permitted, provided the original author(s) and the copyright owner(s) are credited and that the original publication in this journal is cited, in accordance with accepted academic practice. No use, distribution or reproduction is permitted which does not comply with these terms.
*Correspondence: Raimund Pechlaner cmFpbXVuZC5wZWNobGFuZXJAaS1tZWQuYWMuYXQ=