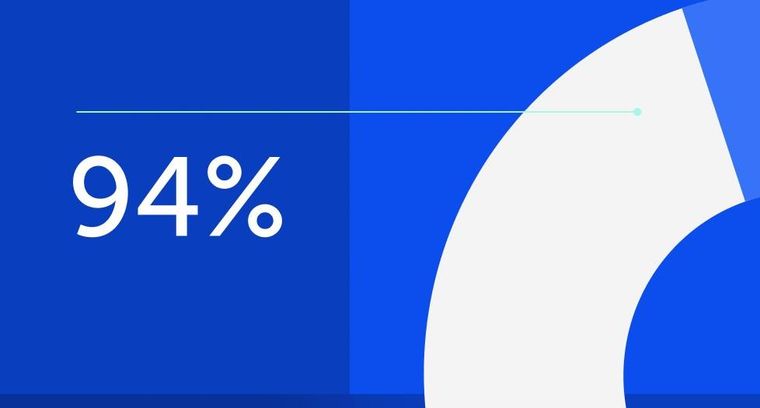
94% of researchers rate our articles as excellent or good
Learn more about the work of our research integrity team to safeguard the quality of each article we publish.
Find out more
REVIEW article
Front. Cardiovasc. Med., 02 March 2023
Sec. Sex and Gender in Cardiovascular Medicine
Volume 10 - 2023 | https://doi.org/10.3389/fcvm.2023.1129348
This article is part of the Research TopicSex Differences in the Inflammatory ResponseView all 4 articles
In the past decade there has been a growing interest in understanding sex and gender differences in myocarditis and dilated cardiomyopathy (DCM), and the purpose of this review is to provide an update on this topic including epidemiology, pathogenesis and clinical presentation, diagnosis and management. Recently, many clinical studies have been conducted examining sex differences in myocarditis. Studies consistently report that myocarditis occurs more often in men than women with a sex ratio ranging from 1:2–4 female to male. Studies reveal that DCM also has a sex ratio of around 1:3 women to men and this is also true for familial/genetic forms of DCM. Animal models have demonstrated that DCM develops after myocarditis in susceptible mouse strains and evidence exists for this progress clinically as well. A consistent finding is that myocarditis occurs primarily in men under 50 years of age, but in women after age 50 or post-menopause. In contrast, DCM typically occurs after age 50, although the age that post-myocarditis DCM occurs has not been investigated. In a small study, more men with myocarditis presented with symptoms of chest pain while women presented with dyspnea. Men with myocarditis have been found to have higher levels of heart failure biomarkers soluble ST2, creatine kinase, myoglobin and T helper 17-associated cytokines while women develop a better regulatory immune response. Studies of the pathogenesis of disease have found that Toll-like receptor (TLR)2 and TLR4 signaling pathways play a central role in increasing inflammation during myocarditis and in promoting remodeling and fibrosis that leads to DCM, and all of these pathways are elevated in males. Management of myocarditis follows heart failure guidelines and there are currently no disease-specific therapies. Research on standard heart failure medications reveal important sex differences. Overall, many advances in our understanding of the effect of biologic sex on myocarditis and DCM have occurred over the past decade, but many gaps in our understanding remain. A better understanding of sex and gender effects are needed to develop disease-targeted and individualized medicine approaches in the future.
Men have an increased incidence of most cardiovascular diseases (CVDs) including atherosclerosis, myocardial infarction, myocarditis, dilated cardiomyopathy (DCM) and heart failure (1, 2). In 2013 we reviewed the topic of sex differences in myocarditis and DCM (3). At that time the National Institutes of Health (NIH) had not updated its guidance for the inclusion of sex as a biological variable (SABV) in study design, data analysis and reporting of findings for NIH supported studies (4–6), and few studies in the literature focused on the topic. In that review, we called for greater translational research efforts in understanding the pathogenesis of sex differences in myocarditis and expressed the need to develop multicenter biobanks that could link specific phenotypes of samples with a special focus on including both sexes (3). Nearly a decade later, there is a growing interest in understanding sex and gender differences in myocarditis and DCM with many publications on the topic. However, many of the same needs still exist, as described in the Executive Summary from the 2022 National Heart, Lung and Blood Institute (NHLBI) of NIH workshop on sex and gender outcomes related to COVID-19 (7). The focus of this review is to provide an update on sex and gender differences in myocarditis and DCM including recent information on the epidemiology, pathogenesis of disease, and clinical presentation, diagnosis and management.
First, sex and gender are not interchangeable terms. Sex refers to biological differences attributed to chromosomes, hormones, reproductive anatomy, gene expression, etc., and typically refers to a binary of male or female but can include intersex (5, 8, 9). Gender, on the other hand, is a social construct that is rooted in biology but affected by environment and experience. Gender is not a binary, but a broad spectrum where individuals may identify as cis or transgender, non-binary, gender-neutral, or in other ways as they define their own gender (8, 10, 11). Biological sex does not change over time, but gender varies in different cultures and with time. This review summarizes data from studies over the past decade which focused on cis-gendered populations, but few if any studies have examined the role of gender on myocarditis or DCM. Although we do not specifically report data on gender differences in this review of myocarditis and DCM because the research has not yet been conducted (i.e., studies designed to understand the effect of gender on disease outcomes), it is important in a discussion of sex differences to understand that there are also environmental and social interactions (gender differences) that affect sex differences outcomes both for animal studies and human data.
Myocarditis is defined by the World Health Organization (WHO) and the International Society and Federation of Cardiology (ISFC) as myocardial inflammation (12) that can cause loss of heart function including sudden cardiac death (13, 14), heart failure, and/or DCM (15, 16). Myocarditis encompasses a number of subtypes including lymphocytic myocarditis, the most common form of myocarditis and the main topic of this review, fulminant myocarditis, giant cell myocarditis, eosinophilic myocarditis and autoimmune myocarditis. These types are not necessarily distinct from each other–lymphocytic myocarditis can be autoimmune and giant cell and eosinophilic myocarditis are often indistinguishable clinically. Regarding some classifications, the presence of necrosis (12, 17–19) and/or viral genome (19) is required for a definitive myocarditis or viral myocarditis diagnosis. Scientific statements and position statements differ with respect to the diagnostic certainty of cardiac magnetic resonance imaging (cMRI) for myocarditis. Histologic confirmation by endomyocardial biopsy (EMB) or surgical tissue analysis is required for a definite diagnosis of myocarditis based on the European Society of Cardiology Working Group on Myocardial and Pericardial Diseases position statement (19, 20). In contrast, cMRI is considered sufficient for a definite diagnosis of myocarditis according to the American Heart Association (AHA) scientific statement and the Brighton Criteria for vaccine-associated myocarditis (21, 22). Endomyocardial biopsies are not common practice in the United States, and the AHA statement recommends viral genome analysis only in cases of diagnostic uncertainty where the biopsy findings will directly influence treatment such as in suspected giant cell myocarditis or sarcoidosis (23). In 2018, native T1 and T2 weighted parametric mapping by cMRI were added to a modified 2009 Lake Louise criteria (LLC) for the diagnosis of myocarditis (24).
Dilated cardiomyopathy is a morphological disease classified by dilation of the ventricle(s) and/or impairment of contractional function (25) in the absence of other cardiac disease, including coronary artery disease (CAD), hypertension, or congenital heart disease (26–28). The WHO definition of DCM adds that these characteristics that disrupt the form and function of the heart may lead to other serious conditions including arrhythmia or heart failure (12, 22). DCM is further classified as either genetic or non-genetic, with environmental factors contributing to the pathogenesis of disease (29–31). The functional impairment that is characteristic of DCM is progressive–as the dilated heart pumps harder to keep up with systolic demand this increases strain which increases dilation, so that the long term consequence is heart failure (16). Much like myocarditis, DCM can have a number of causes that lead to cardiac damage, remodeling, and progression to DCM (32). Myocardial inflammation can initiate remodeling pathways that replace the damaged myocardium with fibrosis that over time leads to dilatation, which has been demonstrated in viral and autoimmune animal models of myocarditis; thus, myocarditis is regarded as a significant cause of DCM (33). If DCM is not found to have a genetic or any other clearly identifiable cause it is termed idiopathic DCM, with this group constituting the largest category of DCM (34). Aside from animal models, several clinical studies have shown that myocarditis can progress to DCM, which is sometimes referred to as inflammatory DCM although the term inflammatory cardiomyopathy is sometimes also used to refer to acute myocarditis (35–38). It is quite possible that all types of DCM have myocardial inflammation that are recruited to the heart if for no other reason than to heal fibrotic scar tissue, which is observed in animal models of myocarditis that progress to DCM (35, 36, 39–41). However, biopsies are rarely performed in patients with DCM to confirm the presence of inflammation. Additionally, there have been studies of idiopathic DCM that find evidence of viruses and cardiac inflammation suggesting the possibility that DCM may have progressed from myocarditis (42).
As heart failure is an important clinical outcome of both myocarditis and DCM, it warrants definition. Heart failure results from the inability of the heart to meet the metabolic needs of the body at normal pressures (43). Heart failure is a spectrum currently classified by clinical and functional measures that can occur suddenly resulting in sudden cardiac death or gradually develop as occurs when myocarditis progresses to DCM, which is often termed chronic heart failure. Myocarditis is an important cause of sudden cardiac death, particularly if highly exertional exercise occurs about a week after symptoms of a viral infection (44, 45). Typically, patients have no signs or symptoms of heart concerns prior to exercise. New York Heart Association (NYHA) class categorizes the severity of heart failure based on clinical cardiovascular fitness. Cardiac systolic function is also stratified into three categories of left ventricular ejection fraction (LVEF) (46): heart failure with reduced ejection fraction (HFrEF) (LVEF <40%), heart failure with middle range ejection fraction (HFmEF), and heart failure with preserved ejection fraction (HFpEF) (LVEF >49%) (46). This developing terminology indicates the limitations in diagnosing heart failure in the cardiovascular field.
Historically the epidemiology of myocarditis has been based mainly on small, single-center studies. The latest Global Burden of Disease (GBD) statistics place the prevalence of myocarditis and cardiomyopathy worldwide at 10.2 to 105.6 per 100,000 (47, 48), with an annual occurrence estimated at around 1.8 million cases (47). One recent study in Sweden reported that the incidence of myocarditis rose from 6.3 to 8.6 per 100,000 from 2000 to 2014 (49). In 2019, GBD statistics reported a mortality rate in patients with myocarditis aged 35–39 of 4.4 per 100,000 in women and 6.1 per 100,000 in men, indicating that more men die of myocarditis than women worldwide (47). Past studies examining sex differences in patients with myocarditis reported only a slightly higher prevalence of myocarditis in males than females (sex ratio female to male of 1:1.5 to 1:1.7) (50–52). In the past decade many clinical studies have been conducted examining sex differences in myocarditis. Studies consistently report that myocarditis occurs more often in men than women with a sex ratio ranging from 1:2–4 female to male (Table 1). Additionally, studies have found that myocarditis occurs more often in men under 50 years of age but in women after age 50 or post-menopause (25, 53–58). Several large studies found that myocarditis was most prevalent in young adult males aged 16–20 (53, 58), but one study of sudden cardiac death corroborated from autopsy in 42 cases reported that myocarditis occurred most often in males aged 36–45 years (Table 1) (56). Thus, overall myocarditis occurs most often in young men under age 50 who have higher biomarker levels (e.g., troponin, sST2) and worse outcomes, including mortality, compared to women (Table 1). Myocarditis occurs most often in women after menopause, and a recent study found that women with autoimmune myocarditis (confirmed presence of heart autoantibodies and anti-nuclear autoantibodies) had worse outcomes compared to men (Table 1) (59).
Clinical and animal studies have demonstrated that myocarditis can progress to DCM (37–40, 60, 61). DCM has an estimated prevalence of 1 in 250 to 500 people (62) and the incidence has increased over the past decade (47). The worldwide prevalence of cardiomyopathy/DCM is higher in men and increases with age (47). Women have been reported to have better long-term survival from DCM following myocarditis, with more men requiring heart transplants (37, 43). We recently reviewed sex differences in DCM and reported an average overall 1:2.5 female to male sex ratio in a meta-analysis of 31 studies from a search of around 1,200 studies in the literature (32). Of the 31 studies reported in the study, most did not analyze data by sex. The overall sex ratio from the meta-analysis was similar to the population-based study of DCM conducted in Olmsted County, Minnesota in 1989, using the Rochester Epidemiology Project, which reported an age-adjusted sex ratio for both incidence and prevalence of 1:3 female to male (63). Similar to myocarditis, recent studies examining sex differences in DCM report that DCM occurs more often in men (60–77%) and that men have lower LVEF, worse outcomes, and higher mortality compared to women (Table 2). However, in contrast to myocarditis, DCM occurs more often after age 60 in both men and women (Table 2). However, the age that DCM occurs post-myocarditis has not been specifically examined.
Until recently there were almost no reports of genetic associations with myocarditis; however, a number of sporadic cases of genetic associations of myocarditis emerged in the literature in recent years (64–72). Recurrent cases of myocarditis and patients presenting with ventricular arrhythmia most strongly suggest genetics could be a factor, especially in children (73–76). This was confirmed in adults in a recent international study of 23 hospitals that compared 36 patients with myocarditis with desmosomal gene variants to those with myocarditis without variants. The study found that patients with variants were at increased risk of recurrent myocarditis and ventricular arrhythmias, and that more women were affected than men (p = 0.01) (75). Another recent study performed next generation DNA sequencing on 36 patients with biopsy-confirmed myocarditis and found that 31% had evidence of genetic variants that have been associated with cardiomyopathy including Titin (TTN) (n = 8), Desmoplakin (DSP) (n = 1), Filamin C (FLNC) (n = 1) and RNA binding protein 20 (RBM20) (n = 1) (77). A large study performed genetic sequencing on patients with myocarditis from three different registries and identified that 19 of 117 patients (16%) had a genetic variant associated with cardiomyopathy or neuromuscular disorders compared to 34 of 468 controls (7%, p = 0.003) (78). Pediatric cases with variants occurred more often in females, but in adults with myocarditis variants were found more often in males (78). TTN mutations were the most commonly found mutations in this study, occurring in 6 out of 8 males. The recent consensus statement for genetic testing in patients with inherited CVD does not recommend genetic testing in patients with myocarditis (79), but evidence may be lacking simply because researchers have not looked for the relationship before now. These recent studies suggest that it may be warranted. Why would myocarditis that is associated with genetic variants display sex differences? One possible explanation is that physical damage to the heart that occurs from genetic causes (i.e., pathogenic variants in titin) could be aggravated by secondary factors such as damage from toxins (i.e., chemotherapy agents like doxorubicin), autoimmunity (i.e., immune complex deposition) and/or infections (i.e., coxsackievirus) leading to inflammation that is sex-specific (32). Thus, the genetic issue may remain functionally and/or symptomatically “hidden” until a second “hit” occurs that may drive inflammation and cardiomyopathy (32).
Dilated cardiomyopathy is inherited in about 30–40% of all cases, which has been reviewed extensively previously (34, 62, 80–82). Most familial DCM has an autosomal dominant inheritance pattern, which is expected to affect men and women equally. However, other inheritance patterns have been identified, including autosomal recessive, X-linked, and mitochondrial, where X-linked and mitochondrial DCM occur more often in women (83). Importantly, large scale genome-wide association studies that examined the risk associated with particular genetic profiles have found significant sex differences, indicating the importance of analyzing genetic data by sex (84). The most common genes associated with cardiomyopathy/DCM are listed in Table 3 in descending order [see also (32)]. However, more than 40 nuclear encoded or mitochondrial genes have been associated with DCM and fall into four major categories: proteins forming the myocyte cytoskeleton, sarcomeric proteins, nuclear envelope proteins, and calcium homeostasis/mitochondrial function regulators (82).
As shown in Table 3, familial DCM typically occurs more often in males, with a reported female to male sex ratio of 1:2–3 (32, 43, 85, 86). Titin truncating variants (TTNtv) show higher penetrance and younger age at presentation in men, who have higher rates of atrial fibrillation and worse cardiac function than women with these variants (82, 87). TTNtv women are at increased risk of developing peripartum cardiomyopathy, suggesting a role for sex hormones in influencing gene expression (88). Women with Lamin A/C (LMNA) DCM were found to have 45% less risk for life-threatening arrhythmia than men (89). Male LMNA mutation carriers present clinical manifestations at a younger age than females (90). Twelve studies that provided data on the sex distribution of LMNA variants in DCM patients and 6 similar studies on Myosin binding protein 3 (MYBPC3) variants found that 98 out of 152 patients with LMNA variants (69%) and 60 out of 76 patients with MYBPC3 variants (79%) were male (82). In contrast, the male proportion was significantly lower in cardiac Phospholamban (PLN) mutation carriers with DCM (46%). PLN was the only mutation examined with a female to male ratio >1 (54% female) (82). In one multicenter study, there was a trend toward a lower risk of major cardiovascular events in women who had Filamin C (FLNC) genetic variants (91). Males with pathogenic variants in the gene for RBM20 were both significantly younger and had lower ejection fraction at diagnosis than females (p < 0.01) (92). Additionally, 35% of affected males (n = 11 of 31) needed a cardiac transplant while none of the affected females (n = 22) were this severe (p < 0.001) (92). Thus, although sex differences are found in familial DCM, many environmental factors contribute to these differences including damage to the heart from infections (e.g., viruses), toxins (e.g., chemotherapy, alcohol), inflammation (e.g., viral or autoimmune myocarditis), sex differences that exist in the basic physiology of the heart, and social factors that contribute to gender differences.
First, it is important to realize that every cell has a “sex” based on their sex chromosomes and is impacted by sex hormones from in utero throughout the lifespan. This results in clear differences in cardiac physiology and gene profiles according to sex (93). Female hearts are on average 25% smaller than male hearts with a smaller ventricular mass and diameter, yet greater cardiomyocyte contractility and ejection fraction (2, 94–97). The number and size (hypertrophy) of cardiac cells differ by sex for all cell types including cardiac myocytes and fibroblasts–which make up the greatest mass of the heart–with 30% of men having greater hypertrophy than women (1, 98). The sex difference in cardiac size is most apparent during adulthood, with myocardial mass better preserved in women as they age (2, 99, 100). Women have smaller coronary vessels than men, (101) premenopausal women have lower blood pressure (102, 103) but a faster resting heart rate than men (104), and women have higher LVEF percentages compared with men (105, 106). Hearts from men and women respond similarly to exercise, which stimulates healthy cardiac enlargement in both sexes, but is more pronounced in females (1, 2).
Many of these sex-specific effects on cardiac function are mediated by sex hormone receptors that signal using both non-genomic (receptors expressed on the surface of cells) and classic genomic mechanisms (107–111). It is important to realize that cells from both men and women express both estrogen receptors (ERs) and androgen receptors (ARs), just in differing ratios. For example, women have higher levels of ERs in/on their arteries than men (108). 17β-Estradiol (E2) signaling through ERs has been shown to prevent cardiac hypertrophy, inhibit reactive oxygen species-induced cardiac damage, prevent apoptosis in cardiac myocytes, and oppose mechanisms that lead to cardiac remodeling and fibrosis (Table 4) (112). The cardioprotective benefit of estrogen in females rapidly declines during menopause (around age 50) when older males have higher levels of circulating estrogen than aging females (113, 114). Little data exists on the effects of cycling hormones or hormonal changes during pregnancy on normal cardiac physiology in women. Hormone receptors also mediate their effects through sex hormone-dependent regulation of miRNAs that are delivered by extracellular vesicles (109, 110, 115). Additionally, chromosomal genotype and epigenetic regulation can also drive sex differences (1). The role of gender on basic cardiac function is understudied with virtually nothing in the literature.
Most of our understanding of the pathogenesis of myocarditis and its progression to DCM comes from animal models. Several animal models of viral myocarditis and autoimmune myocarditis exist [reviewed in 15, 39, 116–118]. The first investigators to study sex differences in myocarditis were Huber and Woodruff, who reported in 1981 that male BALB/c mice had worse myocarditis in response to coxsackievirus B3 (CVB3) infection than females (119). Dr. Huber continued to study sex differences in myocarditis for her entire career, creating much of the field’s foundational understanding of sex differences in myocarditis. Some important findings include that males have more cardiac viral replication than females, which can be increased in females by the addition of testosterone (120). Male BALB/c mice with CVB3 myocarditis have a greater T helper (Th)1 response while females have a greater Th2 response, which can be converted to a Th1 response by the addition of testosterone (121). The predominant immune cell response in the Huber model of CVB3 myocarditis are γδ T cells, which vary by sex with males having more Vγ4 while females have more Vγ1 T cells and B cells (122). Using C57BL/6J chromosome Y consomic mice, Huber et al. showed that the Y chromosome also influences sex differences in myocarditis, although sex hormones mediate the largest effect (123). Genes that are expressed on the X chromosome such as TLR7 and TLR8 have also been found to play roles in regulating the innate immune response to infection contributing to sex differences in certain autoimmune disease models (124, 125). Roberts et al. reported sex differences in Toll-like receptor (TLR)2 and TLR4 signaling in C57BL/6 mice with CVB3 myocarditis, where there was increased expression of TLR2 in females but TLR4 in males (126, 127). And importantly, ERα was found to protect female mice with CVB3 myocarditis by decreasing the Th1 response while increasing regulatory T cells (Treg) while ERβ had the opposite effect (128). However, sex differences in the inflammatory infiltrate differ between models of viral myocarditis. The CVB3 model used by Huber (and many other researchers) causes widespread cardiac apoptosis/necrosis with only a low level of cardiac inflammation (15%) while the majority (70%) of mice die by day 7 with few surviving to develop DCM (117, 118). Using this model, investigators recently showed that an X-linked gene Midline 1/Md1 that regulates TRIM18 expression reduces type I interferon levels in male C57BL/6 mice in response to CVB3 infection altering survival and myocarditis (129, 130). However, the investigators did not examine whether this gene contributed to sex differences in the immune response. Future studies should examine whether X-linked genes such as Md1/TRIM18, TLR7 and TLR8 contribute to sex differences in the immune response to viral infection and myocarditis to better understand the role of the X chromosome in disease pathogenesis.
Around 20 years after Dr. Huber started studying sex differences in CVB3 myocarditis, Dr. Fairweather developed a new model of CVB3 myocarditis based on the idea that myocarditis was an autoimmune disease, which used a mild CVB3 infection as the adjuvant combined with damaged heart protein as the antigen (15, 39, 116). In this model of myocarditis, male BALB/c mice develop worse myocarditis with a dominant immune infiltrate of CD11b/complement receptor 3 (CR3)+ immune cells including neutrophils, macrophages, mast cells and dendritic cells, but there are no sex differences in cardiac viral replication (Figure 1) (40, 131, 132). There is low apoptosis and relatively high inflammation (males average 60% vs. females 25% inflammation) and no deaths with all (100%) male and female BALB/c mice progressing to DCM (41). Males develop worse myocarditis and DCM and sequencing showed that the gene changes that lead to remodeling and fibrosis occur during acute myocarditis, and then time is all that is needed for collagen deposition and fibrosis to occur, with dilatation emerging around 30 days later (41, 133). This model is very similar to clinical lymphocytic myocarditis. Fairweather’s model showed that the dominant innate (first minutes and hours) and adaptive (during acute myocarditis at day 10 after infection) immune response in males is characterized by upregulation of complement and TLR4 on macrophages and mast cells (131, 132, 134, 135). IL-1β and IL-18 (interferon/IFNγ-inducing factor), which are produced from TLR4 signaling, lead to a dominant Th1 and M1 response in male BALB/c mice and cooperate with enzymes released from mast cells (i.e., α1-antichymotrypsin/serpin A 3n) to promote remodeling (3, 41, 133, 136, 137). Surprisingly, TLR4 was found to be present on alternatively activated M2 macrophages that resemble myeloid-derived suppressor cells with a profibrotic M2b phenotype in males, whereas females developed classic M2a macrophages with regulatory receptors like T cell immunoglobulin mucin (Tim)-3 (135). In contrast, a classic IFNγ and Th1 response protects against acute and chronic myocarditis/DCM in BALB/c mice by decreasing viral replication and preventing remodeling and fibrosis that leads to DCM (40, 134, 138). Fairweather showed that testosterone promotes, while 17β-estradiol inhibits myocarditis in BALB/c mice (55).
Figure 1. Sex differences in the pathogenesis of viral myocarditis and DCM. The summary of mechanisms of sex differences are primarily based on the Fairweather model of viral myocarditis (see text for full description). DCM, dilated cardiomyopathy; E2, estrogen/17β-estradiol; ♀, female; IFN, interferon; IL, interleukin; ♂, male; Te, testosterone; Th, T helper cells; TLR, Toll-like receptor.
When C57BL/6 mice were used in this autoimmune viral model of CVB3 myocarditis, they developed a fulminant type of myocarditis (80% inflammation) characterized by a dominant classic Th1-type immune response but without deaths in wild type mice (139, 140). Fairweather found that a Th2-type immune response driven by activation of mast cells and a “mixed” Th1/Th2/M2b immune response in male BALB/c mice is required for the progression of myocarditis to DCM because of the critical role mast cells play in remodeling (Figure 1) (40, 139). In contrast, C57BL/6 mice, and other black background mice–which inherently have very few mast cells–do not progress to DCM after developing myocarditis (40, 141). Importantly, regardless of the organ, remodeling and fibrosis are known to require mast cells and are associated with a dominant Th2-type profibrotic immune response associated with IL-4, IL-33 and TGFβ1 (41, 133, 139). It is likely that IL-1β produced from mast cells and M2b macrophages in males increases IL-6, which in combination with TGFβ1, promotes a Th17 immune response that contributes to fibrosis and DCM (Figure 1) (142).
The effects of estrogen and/or female sex or testosterone and/or male sex on myocarditis and DCM from in vivo and in vitro studies of myocarditis/DCM are summarized in Tables 5, 6. Studies by other investigators using their own viral or autoimmune myocarditis animal models have found similar results to ours (Figure 1 and Tables 5, 6). Cardiac inflammation during myocarditis is strongly influenced by sex hormones on and in immune cells, as well as on and in cardiac tissue cells (3). ERα is primarily found in the uterus, liver, kidney, and heart (128). Sex hormone receptors are also located on and in many cells of the immune system including T cells, B cells, monocytes, macrophages, dendritic cells, and mast cells in humans and rodents (121). Importantly, only monocyte/macrophages and mast cells have both nuclear and membrane ERs and ARs (32, 121). ERα primarily controls E2 modulation of dendritic cell maturation, T cell cytokine production, and immunoglobulin responses (143–145). In contrast, signaling through ERβ up-regulates inducible nitric oxide synthase (iNOS) and nitric oxide generation with ERα suppressing this response (137, 146). Several important studies now show that when ERα and ERβ are co-expressed in the same cell, these receptors may exert opposing effects on gene expression and thus counterbalance each other (147–150).
Table 5. Effect of estrogen and/or female sex on an inflammatory response or myocarditis from animal models or tissue culture.
Table 6. Effect of testosterone and/or male sex on an inflammatory response or myocarditis from animal models or tissue culture.
In general, estrogen has been found to increase immunoglobulin synthesis and inhibit B cell apoptosis resulting in increased antibody and autoantibody levels in females (151, 152), suppress both T and B cell lymphopoiesis (153), enhance dendritic cell differentiation and antigen presentation (154), suppress TNFα and IL-6 levels, (155, 156) increase IL-4 and IFNγ production (143, 157), and promote FoxP3+ T regulatory cell development. (32, 128, 158, 159).
During CVB3 myocarditis in the autoimmune CVB3 model, females generate a robust immune response to infection, but they regulate or inhibit the inflammatory response very well after clearing the viral infection by upregulating almost every regulatory immune feature, including antibodies, CR1, Tim-3, CTLA4, Treg, IL-10, anti-inflammatory and anti-fibrotic M2a-type macrophages, and so forth. In contrast, males develop a robust proinflammatory immune response but tend not to regulate it well, instead promoting mast cell and M2b cell responses that are both proinflammatory and profibrotic (Figure 1).
Patients with myocarditis can be asymptomatic or present with reduced exercise capacity, fatigue, and dyspnea. Around 60% of patients present with antecedent arthralgias, malaise, fever, sweats, or chills consistent with viral infections (e.g., diarrhea and/or vomiting with coxsackievirus, dyspnea with SARS-CoV-2) that they report occurred 1–2 weeks before the onset of symptoms (22, 25, 160). Patients may present with arrhythmias in the form of syncope, palpitations due to heart block, life-threatening bradyarrhythmias, and ventricular tachyarrhythmias or even sudden cardiac death, which is often associated with exertional exercise (22). Chest pain can range from mild to acute pain which is associated with myopericarditis in 35% of cases (18, 25). Severe cases of myocarditis can mimic myocardial infarction (161). Severe forms of myocarditis, such as fulminant myocarditis, can progress rapidly and result in acute myocardial failure and cardiogenic shock.
A recent study examined sex differences in clinical presentation of patients with myocarditis and found 82% (n = 63) were male, the mean age of patients was the same (around age 40), and BMI was similar at around 27 kg/m2 (162). Percent LVEF was similar in men (51 ± 13%) compared to women (57 ± 12%, p = 0.14). However, more men presented with symptoms of chest pain while women presented more often with dyspnea. Classic laboratory tests such as high sensitivity troponin T (hs-TnT), C-reactive protein (CRP), N-terminal-pro hormone-probrain natriuretic peptide (NT-proBNP), leukocytes, and thrombocytes were not found to display sex differences except for myoglobin and creatine kinase, with creatine kinase remaining significantly different after controlling for sex-specific reference ranges (162). Overall, comorbidities such as non-obstructive CAD, arterial hypertension (aHTN), hyperlipidemia, diabetes mellitus, atrial fibrillation, atrial flutter, and heart failure were rare and did not differ by sex (162). This is likely due to myocarditis occurring in young, relatively healthy patients. Larger studies are needed to better understand sex differences in the clinical presentation of myocarditis.
Several comorbidities increase the risk of heart failure including hypertension, diabetes, obesity, smoking history, and hyperlipidemia. Many of these factors increase risk more in women than men (163). Type 2 diabetes is an important risk factor for heart failure for both sexes, but data from the Framingham Heart Study found that diabetic women had a five-fold greater risk of heart failure while diabetic men had a two-fold greater risk (164). Similar findings have been reported for the risk of hypertension and heart failure (163). In another example, obesity is more prevalent in women than men and its association with heart failure risk is greater in women. However, heart failure in women is more closely associated with HFpEF, while risk of heart failure in myocarditis is associated with HFrEF (163, 165). A recent study of patients with HFpEF from three clinical trials (4,458 women and 4,010 men) found that women were older, more often obese and had hypertension while men were more likely to develop atrial fibrillation and CAD (166). Despite these risk factors, women had a lower risk of death compared to men (166). Importantly, all of these risk factors are higher in Black than White women and men (167), while myocarditis in the US occurs predominantly in White individuals. Thus, these factors are less likely to play an important role in the risk of developing myocarditis because it occurs in young individuals, prior to the clinical onset of many of these risk factors. Currently, the greatest known risk factors for myocarditis are young age and male sex, with the most frequent etiologic agents being viral infections worldwide and Trypanosoma cruzi in South America (Chagas disease).
Currently, we are not aware of any studies that examine the effect of gender on myocarditis and DCM outcomes based on their study design. These studies are needed in order to understand the contribution of gender to clinical sex differences outcomes.
Men are at an increased risk of developing heart failure from a number of CVDs including atherosclerosis, myocardial infarct, myocarditis, and DCM (168). Heart failure prevalence increases with advancing age in both genders, but increases dramatically in women >55 years of age in spite of better LV function than men (107, 169, 170). The strongest predictor of mortality in men is NYHA classification (107). Patients with chronic heart failure who have elevated levels of inflammation or inflammatory mediators/biomarkers have a worse prognosis (171). There are a number of traditional biomarkers that are used to diagnose myocarditis such as high sensitivity troponin T, troponin I, NT-proBNP, creatine kinase, and kappa or lambda immunoglobulin free light chains, but these markers are not disease specific (22, 162, 172). Tumor necrosis factor (TNF) and IL-6 are well known serum biomarkers of heart failure, along with IL-1β, but IL-1β is better as a tissue biomarker rather than a serum biomarker, as its sera levels are low. IL-1β contributes to Th17 responses by increasing the level of IL-6 where it combines with IL-23 and TGFβ-1 to drive Th17 immune responses while inhibiting regulatory T cells (Figure 1).
Over the past 10 years, a number of novel serum biomarkers have been reported that may improve the diagnosis of myocarditis and predict progression to cardiomyopathy/DCM and heart failure including sera soluble ST2 (sST2), myoglobin, IL-17-associated miRs, Th17-associated cytokines, and Th17 vs. Treg ratios (55, 142, 173–175). A study of around 300 patients with clinically suspected and/or biopsy confirmed myocarditis revealed that sST2 was elevated only in men under 50 years of age but not in women, and that higher sST2 levels were associated with elevated NYHA class heart failure (55). There was a trend for higher sST2 levels in women over 50 years of age with myocarditis, but the study was underpowered to confirm the relationship in women (55). Note that soluble sST2 that is a biomarker for heart failure is also known as interleukin-1 receptor-like 1 (IL1RL1 GeneID: 9173). This biomarker has been frequently confused, especially in the CVD literature, with the gene with the same abbreviation called suppression of tumorigenesis-2 (ST2 GeneID: 6761), which is found on chromosome 11 and represents a putative locus associated with cancer. Another study found an association between increased serum sST2 levels and fulminant myocarditis, a severe form of myocarditis that requires rapid detection to prevent a fatal outcome (176). However, only four patients were examined and so sex differences were not reported. Recently, sera myoglobin levels were found to be a strong predictor of acute myocarditis based on cardiac MRI and this sera biomarker was also detected in a viral model of myocarditis (174). Myoglobin was found to be significantly increased in men with myocarditis compared to women (p = 0.04) (162). In that study, there were no sex difference in hs-TnT, CRP, or NT-proBNP (162). Th17 cells and their associated cytokines (i.e., IL-17A, IL-6, IL-23) were found to directly correlate with heart failure in a study of patients with clinically suspected myocarditis/acute DCM (142). Importantly, IL-17A levels were found to be increased in males compared to females. Men were also found to have an association between a polymorphism in IL-17, rs763780, and an increased risk of DCM (142). This association of IL-17/Th17-associated cytokines with myocarditis was recently confirmed by separate investigators. They went on to identify a Th17-associated microRNA that was detected in the serum of patients and mice with myocarditis (autoimmune viral model) but not in patients or mouse models of myocardial infarct (175). The group did not report whether sex differences exist in the biomarker, but that the microRNA retained its diagnostic value in models after adjustment for age, sex, ejection fraction, and serum troponin level (175). Future studies should examine whether sex and age differences exist in these biomarkers which may provide insight into their role in the pathogenesis of disease.
Standard treatment strategies for myocarditis and DCM remain as guideline-based heart failure therapies. There is only limited available literature on whether sex differences exist in the use of pharmacologic therapies for myocarditis or DCM. The Intervention in Myocarditis and Acute Cardiomyopathy-2 (IMAC-2) trial, which included 373 patients (38% women), found no significant difference in the use of angiotensin receptor blockers (ARBs), angiotensin converting enzyme (ACE) inhibitors or beta-blockers between men and women (37).
Most trials and meta-analyses suggest that medications used for the treatment of HFrEF reduce event rates in women. However, individual and sex-specific differences in drug absorption, distribution, metabolism, and excretion could affect drug doses needed for optimal efficacy and safety in patients with myocarditis and DCM (112, 177–180). Although HFrEF guidelines have a gender neutral dose recommendation for medications, women typically have a lower body weight, higher proportion of body fat, and lower plasma volume than men (181). This might result in higher maximum plasma concentrations of ARBs, ACE inhibitors, and beta-blockers in women compared to men using a similar dose. A post hoc analysis by Santema et al., reported sex-based differences in clinical outcomes in patients with HFrEF (182). In the Biostat-HF and ASIAN-HF registries, women treated with ACE inhibitors, ARBs, and beta-blockers had approximately 30% lower risk of death or hospitalization for heart failure at 50% of the guideline recommended doses (182). Women had no further benefit at higher doses. These data suggest that a lower target dose based on sex might be more appropriate (182). This is important because women with heart failure experience twice higher rates of adverse events (e.g., ACE inhibitor cough) from medication compared to men (180, 183, 184).
Importantly, fewer women compose clinical heart failure trials that guidelines are based on (185). Although it can be viewed that fewer women are recruited to trials, the sex difference in composition in trials may simply reflect sex differences in disease indicating that trials need to go longer to increase the number of women enrolled in the study. Challenges to this include the increased cost, yet it may be necessary to improve our understanding of sex and gender differences in disease. One important outcome of the underrepresentation of women is that a lack of evidence leads to women being less likely to be prescribed certain evidence-based medications; and when these medications are prescribed for women, dosing tends to be suboptimal (186, 187). It has been reported that male physicians use lower drug dosages and fewer drugs in female patients (188). In contrast, female physicians were found to have superior communication skills leading to better therapy adherence and improved outcomes in their patients as a consequence (189). Additionally, male patients seem to be less therapy adherent than female patients (190). These differences reflect a complex combination of sex and gender effects on the efficacy of available therapies.
While it has been established that biologic sex influences the function of the autonomic nervous system, clinical trials investigating beta-blockers have not sufficiently addressed this fact (191). Most beta-blocker trials were conducted at a time when investigators did not appreciate the effect of sex/sex hormones on pharmacokinetics. No studies to our knowledge have specifically examined the effect of beta-blockers on myocarditis or examined sex differences in their effect.
In a study of sex differences in congestive heart failure outcomes after using bisoprolol or metoprolol, both β1-selective β-adrenergic antagonists, Simon et al. found that women had improved survival for all factors examined but that the survival benefit in women was regardless of beta-blocker therapy (192). In contrast, a study conducting post hoc analysis of data in women with heart failure (n = 898) found that metoprolol improved outcomes in women similar to men (193), which was confirmed later by a meta-analysis of three clinical trials examining the effect of beta-blockers on heart failure (194). There is a need for studies to be conducted with the current understanding of the importance of sex differences to determine whether conclusions from these older studies are correct.
The influence of estrogen on the renin-angiotensin-aldosterone-system (RAAS) has been discussed in numerous publications, but its clinical implications remain unknown (191, 195–198). Over the years a number of clinical trials have shown the beneficial effects of ACE inhibitors in HFrEF patients without considering whether sex differences exist (199–202). However, several studies have examined sex differences. Garg et al. showed a 3% higher survival for men compared to women who received ACE inhibitor therapy in a meta-analysis of approximately 7,000 patients (23% women) enrolled in 32 randomized trials (203). The ATLAS trial compared low and high doses of lisinopril (2.5–5 mg daily vs. 32.5–35 mg daily) in 3,164 patients (648 women) with NYHA class II to IV chronic heart failure and LVEF ≤30% in a double blind randomized controlled trial (204). Patients receiving a high dose of lisinopril had a lower risk of death or hospitalization, which benefited men more than women. Finally, a meta-analysis of the six largest ACE inhibitor trials also found a significant survival benefit for men, but not for women (205). These data indicate that the benefit of ACE inhibitors is greater in men than women.
Patients with systolic heart failure who cannot tolerate ACE inhibitor therapy are sometimes treated with ARBs. In the Effects of High-dose vs. Low-dose Losartan on Clinical Outcomes in Patients with Heart Failure (HEAAL) study, the authors performed a randomized, double-blind trial in 255 sites with 3,846 heart failure patients (30% women) with a NYHA class II-IV, LVEF ≤40% and an ACE inhibitor intolerance (206). Patients were allocated into low vs. high dose groups (50 mg vs. 150 mg) and their all-cause mortality was compared. The higher dose was more beneficial for men, while the outcome for women did not differ between the two dose levels (206). A population study comparing sex differences in therapy response of ARBs vs. ACE inhibitors in 19,698 patients (10,223 women) found that women seem to benefit more from ARBs than men (207). These findings correlate with those of other authors (208, 209).
Neprilysin is a membrane bound endopeptidase that cleaves and degrades vasoactive peptides, including natriuretic peptides, bradykinin, and adrenomedullin. Neprilysin inhibition has been evaluated to counteract neurohormonal overactivation in the treatment of heart failure. However, neprilysin also breaks down angiotensin II, so there is limited efficacy with lone neprilysin inhibition. The combination of ACE inhibitor therapy and neprilysin inhibition was associated with increased angioedema (210). Angiotensin receptor-neprilysin inhibition (ARNI), in the form of sacubitril-valsartan, was compared to enalapril in the Prospective Comparison of (ARNI) with Angiotensin Converting Enzyme Inhibitor (ACEI) to Determine Impact on Global Mortality and Morbidity in Heart Failure (PARADIGM-HF) trial (211). ARNI therapy was associated with a reduction in the risk of heart failure hospitalization and death, and there is now a class I indication for ARNI therapy in the treatment of patients with HFrEF (46). In the PARADIGM-HF trial, only 21.8% of patients enrolled were women. However, ARNI therapy was shown to reduce cardiovascular mortality and heart failure hospitalizations in both men and women (HR 0.80, 90.72–0.90; HR 0.77, 0.62–0.95, respectively; p = 0.63) (212). A previous prospective registry in 10 centers examined sex differences in efficacy, safety, and tolerability of sacubitril-valsartan and found no difference in discontinuation of ARNI therapy, no difference in received dose, and no difference in adverse events between women and men. A greater proportion of women did have an improved functional class, and female sex was considered an independent predictor of functional class improvement (213). In a post hoc analysis of patients with HFrEF enrolled in the Prospective Study of Biomarkers, Symptom Improvement and Ventricular Remodeling During Entresto Therapy for Heart Failure (PROVE-HF) trial, sex differences in biomarkers, health status, and remodeling endpoints were evaluated. This analysis demonstrated a reduction in NT-proBNP and improvement in cardiac remodeling parameters after initiation of ARNI therapy in both men and women. Women experienced improvement in cardiac remodeling parameters earlier, and women also experienced greater improvement in perceived quality of life based on changes in their Kansas City Cardiomyopathy questionnaire. This reiterated the benefit of ARNI therapy for both sexes.
Angiotensin receptor-neprilysin inhibition therapy has a class IIb indication for treatment of heart failure with preserved ejection fraction. This recommendation was based on the Prospective Comparison of ARNI with ARB Global Outcomes in Heart Failure with Preserved Ejection Fraction (PARAGON-HF) trial in which the effects of sacubitril-valsartan were compared with valsartan (214). Of the participants analyzed, 52% were women, which is one of the largest populations of women studied in a HFpEF trial. This trial did not meet its primary endpoint, identified as the composite of first and recurrent heart failure hospitalizations and cardiovascular death. A separate subgroup analysis found a more favorable treatment effect in women compared with men, with sacubitril-valsartan leading to a greater reduction in HF hospitalizations. Further analysis demonstrated that women enrolled in the trial were older, more obese, and had a lower median NT-proBNP and lower estimated glomerular filtration rate compared with men, highlighting the difference in clinical profile between women and men with HFpEF.
Sodium-glucose cotransporter-2 inhibitors (SGLT2i) have been shown to reduce HF hospitalizations and cardiovascular death across all ranges of LVEF, and there is now a class I indication for SGLT2 inhibitors in HFrEF and a class IIa indication for HFpEF (46). SGLT2 inhibitors block glucose absorption at the proximal renal tubule. Additional mechanisms of action making them beneficial in heart failure are speculative, including reduction in fibrosis and potential improvement in myocardial metabolism and endothelial function. Recent trials, including Dapagliflozin and Prevention of Adverse Outcomes in Heart Failure (DAPA-HF) (215) and the Empagliflozin Cardiovascular Outcome Event Trial in Type 2 Diabetes Mellitus (EMPA-REG) (216), demonstrated a disproportionately lower enrollment of women compared with men with 23.4 and 29%, respectively, similar to other HFrEF trials. A prespecified subgroup analysis of DAPA-HF evaluated sex differences in drug efficacy. This study found that dapagliflozin reduced the risk of worsening heart failure and improved symptoms and quality of life similarly between men and women. This prespecified subgroup analysis also highlighted the different clinical profile between men and women with HFrEF, with women being older, less likely to have an ischemic etiology, with worse renal function, and with lower rates of HF hospitalizations and cardiovascular death compared to men. The effects of dapagliflozin on estimated glomerular filtration rate, body weight, and systolic blood pressure appeared to be similar between men and women. Safety data and tolerability for dapagliflozin in women were reassuring, with no increased adverse events specifically in women (217).
As discussed earlier, sex may influence the effect of drugs on the RAAS. Similar to ACE inhibitors and ARBs, there is no major study on aldosterone antagonists examining sex differences. Women have physiologically higher aldosterone levels, whilst aldosterone is known as a stimulator for proinflammatory pathways, cardiac remodeling, and the development of epicardial adipose tissue which is a driver of HFpEF pathogenesis (218–221). This indicates that inhibiting the final common path of RAAS might be more beneficial for women compared to men (218–220, 222). The Treatment Of Preserved Cardiac function in heart failure with an Aldosterone antagonist Trial (TOPCAT) was a randomized, double-blinded trial that compared spironolactone vs. placebo in 3,445 patients with HFpEF. In a post hoc analysis of the study population, Shah et al. found women to benefit from spironolactone across the whole LVEF spectrum compared to men who had a benefit only at lower LVEF (183, 223). All-cause mortality was also reduced by 34% in women, with no advantage for men (223). Another post hoc analysis performed by Merrill et al. confirmed the possibility of sex specific all-cause mortality in patients treated with spironolactone (224). This effect was only shown in all-cause mortality and not cardiovascular mortality alone, which the authors attribute to the lack of statistical power (224).
The Digitalis Investigation Group trial showed that digoxin reduces hospitalization for heart failure but has no effect on mortality (225). Several post hoc subgroup analyses of the Digitalis Intervention Group trial population created controversy regarding digoxin therapy in women. Rathore et al. showed that all-cause mortality in HFrEF patients treated with digitalis was 5.8% higher in women (95% CI 0.5–11.1) (226). In another retrospective study including 1,926 women from the Digitalis Investigation Group trial population, digoxin treatment was found to be a significant independent covariate predicting all-cause mortality (227). Adams et al. performed a continuous multivariable analysis on 4,944 HFrEF patients of the Digitalis Investigation Group trial population, showing a significant linear relationship between serum digoxin concentration and mortality but no differences between the sexes (228).
The risk of sudden cardiac death remans higher in men than women at all age groups (229), and develops in women about 10 years later than in men. Women are underrepresented in randomized implantable cardiac defibrillator (ICD) trials and are 40% less likely to obtain ICD therapy than men (230, 231). A meta-analysis of five ICD clinical trials found no significant difference in overall mortality between women and men, but women experienced significantly fewer appropriate ICD interventions (232). Importantly, women have a higher chance of complications during and after device implantation (233, 234). A recent study of 4,506 heart failure patients (76% male) found that women with ICDs had significantly less first and recurrent life-threatening ventricular arrhythmias than men, especially in patients with non-ischemic cardiomyopathy, suggesting that this therapy may be less effective for women (235).
Cardiac resynchronization therapy-defibrillator (CRT-D) implantation is an established therapy for patients with chronic heart failure and a broad QRS complex. In a meta-analysis of pooled individual patient level data from three CRT-D trials, women with a relatively narrow QRS width between 130 and 149 ms benefited from CRT-D more than men. In this group, women had a 76% reduction in heart failure or death (absolute CRT-D to ICD difference, 23%; HR 0.24, 95% CI 0.11–0.53; p < 0.001) (236, 237). Women who receive CRT therapy show a therapy response of 90% over a wide range of QRS duration (130–175 ms) (238). Women with left bundle branch block and CRT do have significantly higher ventricular tachycardia-free survival than men, as shown in a multicenter retrospective study in 460 patients (105 women) of the Incidence of Arrhythmia in Spanish Population With a Medtronic Implantable Cardiac Defibrillator Implant national registry (UMBRELLA) (239). All-cause mortality did not differ between sexes in this study (239). Because females have such impressive benefits from CRT, improved screening and advocacy for CRT implantation in women should be considered (240).
Continuous flow left ventricular assist devices (LVAD) are utilized as bridge to transplant therapy (BTT) or destination therapy in chronic end-stage heart failure as well as patients with acute heart failure with hemodynamic compromise and cardiogenic shock, including patients with myocarditis. No large-scale clinical trials have investigated the use of these devices specifically in patients with myocarditis. One study of ventricular assist devices (VADs) used in 11 patients (6 women) with acute viral myocarditis showed similar survival among women and men, but more men required reoperation and more women developed right heart failure (241). A review of the use of VADs as a bridge to transplant in 6 patients (2 women) with giant cell myocarditis reported that 3 men and 1 woman were still alive after transplant, whereas 1 woman died of an embolic stroke and 1 man died of a hemorrhagic stroke before transplant (21).
Although mechanical circulatory support has increased for both women and men, particularly in the current era of continuous flow LVADs, information on sex and gender differences remains limited because sex-specific results are infrequently reported. Continuous flow LVADs have replaced the prior generation of pulsatile flow LVADs. Prior studies have also demonstrated that women in the pulsatile flow era had increased risk of mortality, with female sex being an independent predictor for hemorrhagic and ischemic stroke (242). More recent studies did not demonstrate sex differences in neurologic outcomes, and in the current continuous flow era, female sex does not appear to correlate with increased risk of mortality (243).
A previous study that systematically compared outcomes in women and men using LVADs as a bridge to transplant reported no survival difference between the sexes, but fewer women than men underwent heart transplantation (244). In the study, more women (72 of 104, 69%) than men (184 of 362, 51%) had non-ischemic cardiomyopathy. Adverse event rates were similar between women and men except for hemorrhagic stroke, which occurred more frequently in women, and device-related infections, which occurred more frequently in men (244). DeFilippis et al. recently described a United Network for Organ Sharing (UNOS) sample in BTT LVAD recipients, and women were found to have an increased risk of waitlist mortality (245). Maukel et al. found that in patients with LVADs implanted as destination therapy, women had increased rates of device replacement and recovery compared with men, but men and women did not differ in clinical outcomes including death or transplant (246). The HeartMate 3 LVAD is the only currently commercially available durable continuous-flow LVAD after a recall of the HeartWare device. In MOMENTUM 3 (Multicenter Study of MagLev Technology in Patients Undergoing Mechanical Circulatory Support Therapy with HeartMate 3), HeartMate 3 was found to be superior to the prior generation axial continuous-flow LVAD (HeartMate II) in the primary endpoint of survival free of disabling stroke or reoperation to replace or remove the device. There was no sex difference in stroke risk, but it is important to note that the HeartMate 3 cohort only consisted of 31 women (247).
An evaluation of all LVAD-related Emergency Department visits from 2010 to 2018 found significant sex differences. 27% of LVAD-related emergency department visits were female patients while only 21% of all patients with LVAD support in the study were female, indicating that women were seeking care in the Emergency Department more frequently than men. Importantly, women were found to have a lower likelihood of hospital admission compared with men despite similar presentation and were less likely to undergo cardiac catheterization or additional testing, highlighting inequities in their treatment (248).
Cardiac transplantation is reserved for patients who are refractory to optimal medical therapy and mechanical circulatory support. Men are more frequently referred for heart transplantation than women in both the US and Europe. In a recent retrospective analysis of patients referred for advanced heart failure therapies, only 26.6% were women (249). In an assessment by the International Society for Heart and Lung Transplantation, only 23% of patients who underwent heart transplantation between 2005 and 2010 were women (250). A study that examined DCM specifically found an even greater gender imbalance in referral for heart transplantation of 1:6 at the German Heart Center Berlin and 1:5 in the Eurotransplant database (251). The authors concluded that the sex difference imbalance seemed to be due to referral bias, as women in both transplantation cohorts had more severe heart failure but fewer relative contraindications than men at the time of referral. There is no data on whether there are sex differences in referral for transplantation for patients with myocarditis. In October 2018, the UNOS implemented a new donor heart allocation system, and recent analyses have highlighted new trends in the allocation system, such as increased temporary mechanical circulatory support (252). Further studies are needed to determine the impact on sex differences in outcomes with the new allocation system.
There are a number of areas that are important to consider for the future. Disaggregation of sex-based analyses is a critical part of studies that include both sexes (8). Not reporting sex-based analyses can give an incorrect depiction of a disease or treatment as sex-neutral in risk or effect. This is particularly important for studies involving the immune response, where hormone response elements often alter gene responses in opposite directions (i.e., estrogen response element decreases genes while androgen response element increases the same genes) so if the analysis is not conducted according to sex then the two sexes cancel each other out to suggest no relationship. Even among cardiovascular clinical trials that include both sexes, a minority (33%) were found to actually report the data analysis (253). Future work needs to include data, even if non-significant, between the sexes (1). Although many advances have been made in the past decade, many of the same gaps remain. There is a need for a better understanding of the causes of myocarditis in order to identify more specific biomarkers of disease onset and progression. This can be better accomplished using translational models of myocarditis and by obtaining cardiac biopsies. There remains a need to understand the effect of genes that are expressed on the X chromosome in mediating sex differences in the immune response during myocarditis. There is also a need to bank serum and tissue samples of myocarditis and DCM patients in the US, as has been done for years overseas, so that a better understanding of the viruses and other causes of myocarditis can be identified, and disease-specific therapies developed. Myocarditis differs profoundly by sex, and data in animal and human studies need to interpret their findings in relation to sex and gender differences to improve care for myocarditis and DCM patients in the future. In order to gain an understanding of how gender affects myocarditis and DCM, studies need to be designed specifically to examine this question.
Over the last decade, interest in myocarditis and DCM has dramatically increased and many new insights have been gained. Once a limited topic on what has been considered a rare condition, myocarditis has entered the public eye with the outbreak of the worldwide SARS-CoV-2 pandemic. While the past decade of research has brought tremendous insight into the pathogenesis of myocarditis and DCM, many of the same problems identified a decade ago remain. There are no unified global standards for the diagnosis of myocarditis. Most of the clinical insights on myocarditis have come from biopsy samples, which are rarely taken in the US and especially for the most common form of myocarditis, lymphocytic myocarditis, which hinders progress. There are few biobanks of myocarditis samples in the US which stymies evidence-based research advances. Even with the increased understanding of the importance of sex and gender, there is lack of analysis of sex and age differences in most studies, both clinically and in animal models. Furthermore, there is a lack of studies that specifically examine the role of gender on disease pathogenesis or outcomes. Importantly, older studies need to be reassessed considering our current understanding of the effects of sex and age on myocarditis and DCM in all facets of the disease, from epidemiology and pathogenesis, to current treatment guidelines and therapies. Fortunately, highly translational animal models of myocarditis that progress to DCM exist and are increasing our understanding of the role of sex and age in disease. In conclusion, a heightened understanding of sex and gender differences is critical for improving diagnostic strategies and clinical management that will lead to optimal sex- and gender-based care for patients with myocarditis and DCM.
DF and DB: conceptualization and writing—original draft. DF: project administration. DF, DB, NM, BH, ML, LC, and KB: writing—review and editing. All authors have read and agreed to the published version of the manuscript.
This work was supported by the National Institutes of Health (NIH) R01 HL164520, R21 AI145356, R21 AI152318, and R21 AI154927 to DF; American Heart Association 20TPA35490415 to DF; NIH grants TL1 TR002380 to DB and DF; R21 AI163302 to KB; R01 HL135165 to LC; the For Elyse Foundation to DF; and the Mayo Clinic Center for Regenerative Medicine to DF.
DF is on the advisory board of Cytokinetics. BH is inventor on patents that use RNA for diagnosis of myocarditis.
The remaining authors declare that the research was conducted in the absence of any commercial or financial relationships that could be construed as a potential conflict of interest.
All claims expressed in this article are solely those of the authors and do not necessarily represent those of their affiliated organizations, or those of the publisher, the editors and the reviewers. Any product that may be evaluated in this article, or claim that may be made by its manufacturer, is not guaranteed or endorsed by the publisher.
CVD, cardiovascular disease(s); ACE, angiotensin converting enzyme; ACEI, angiotensin converting enzyme inhibitor; AHA, American Heart Association; aHTN, arterial hypertension; AR, androgen receptor(s); ARB, angiotensin receptor blocker(s); ARNI, angiotensin receptor-neprilysin inhibition; BTT, bridge to transplant; CAD, coronary artery disease; cMRI, cardiac magnetic resonance imaging; CR, complement receptor; CRP, C-reactive protein; CRT-D, cardiac resynchronization therapy-defibrillator; CVB3, coxsackievirus B3; DAPA-HF, Dapagliflozin and Prevention of Adverse Outcomes in Heart Failure; DCM, dilated cardiomyopathy; DSP, desmoplakin; E2, 17 β -estradiol; EMB, endomyocardial biopsy; EMPA-REG, Empagliflozin Cardiovascular Outcome Event Trial in Type 2 Diabetes Mellitus; ER, estrogen receptor(s); ESC, European Society of Cardiology; FLNC, filamin C; GBD, Global Burden of Disease; HEAAL, effects of high-dose versus low-dose Losartan on Clinical Outcomes in Patients with Heart Failure study; HFmEF, heart failure with mid-range ejection fraction; HFpEF, heart failure with preserved ejection fraction; HFrEF, heart failure with reduced ejection fraction; hsTpnT, high-sensitivity troponin T; ICD, implantable cardiac defibrillator; IFN, interferon; IL, interleukin; IL1R1, interleukin-1 receptor-like 1; IMAC-2, Intervention in Myocarditis and Acute Cardiomyopathy-2; iNOS, inducible nitric oxide synthase; ISFC, International Society and Federation of Cardiology; LLC, Lake Louise criteria; LMNA/C, lamin A/C; LVAD, left ventricular assist devices; LVEF, left ventricular ejection fraction; MOMENTUM 3, Multicenter Study of MagLev Technology in Patients Undergoing Mechanical Circulatory Support Therapy with HeartMate 3; MYBPC3, myosin binding protein 3; NHLBI, National Heart, Lung and Blood Institute; NIH, National Institutes of Health; NT-proBNP, N-terminal -pro hormone-probrain natriuretic peptide; NYHA, New York Heart Association; PARADIGM-HF, Global Mortality and Morbidity in Heart Failure; PARAGON-HF, Prospective Comparison of ARNI with ARB Global Outcomes in Heart Failure with Preserved Ejection Fraction; PLN, cardiac phospholamban; PROVE-HF, Prospective Study of Biomarkers, Symptom Improvement and Ventricular Remodeling During Entresto Therapy for Heart Failure; RAAS, renin-angiotensin-aldosterone-system; RMB20, RNA binding protein 20; SABV, sex as a biological variable; SCD, sudden cardiac death; SGLT2i, sodium-glucose cotransporter-2 inhibitors; sST2, soluble ST2; Th, T helper; Tim, T cell immunoglobulin mucin; TLR, Toll-like receptor; TNF, tumor necrosis factor; TnT-hs, high sensitivity troponin T; TOPCAT, Treatment of Preserved Cardiac Function Heart Failure With an Aldosterone Antagonist; Treg, regulatory T cells; TTN, titin; TTNtv, titin truncating variants; UMBRELLA, Incidence of Arrhythmia in Spanish Population With a Medtronic Implantable Cardiac Defibrillator Implant; UNOS, United Network for Organ Sharing; WHO, World Health Organization.
1. Walker C, Schroeder M, Aguado B, Anseth K, Leinwand L. Matters of the heart: cellular sex differences. J Mol Cell Cardiol. (2021) 160:42–55. doi: 10.1016/j.yjmcc.2021.04.010
2. St Pierre S, Peirlinck M, Kuhl E. Sex matters: a comprehensive comparison of female and male hearts. Front Physiol. (2022) 13:831179. doi: 10.3389/fphys.2022.831179
3. Fairweather D, Cooper L Jr., Blauwet L. Sex and gender differences in myocarditis and dilated cardiomyopathy. Curr Probl Cardiol. (2013) 38:7–46. doi: 10.1016/j.cpcardiol.2012.07.003
4. Waltz M, Fisher J, Lyerly A, Walker R. Evaluating the National Institutes of Health’s sex as a biological variable policy: conflicting accounts from the front lines of animal research. J Womens Health (Larchmt). (2021) 30:348–54. doi: 10.1089/jwh.2020.8674
5. Wizemann T, Pardue M. Exploring the Biological Contributions to Human Health: Does Sex Matter?. Washington (DC): The National Academies Press (2001).
6. Health NIO. Consideration of Sex as a Biological Variable in NIH-funded Research. Maryland, MD: National Institutes of Health (2015).
7. National Heart Lung, and Blood Institute. Sex/Gender-Specific COVID-19 Outcomes and Management Relevant for Heart, Lung, Blood, and Sleep Disorders: From Bench to Bedside. Maryland, MD: National Institutes of Health (2022).
8. Heidari S, Babor T, De Castro P, Tort S, Curno M. Sex and gender equity in research: rationale for the SAGER guidelines and recommended use. Res Integr Peer Rev. (2016) 1:2. doi: 10.1186/s41073-016-0007-6
9. Canadian Institutes of Health Research. What a Difference Sex and Gender Make: A Gender, Sex and Health Research Casebook. Ottawa, ON: Canadian Institutes of Health Research (2012).
10. Hoffmann D, Tarzian A. The girl who cried pain: a bias against women in the treatment of pain. J Law Med Ethics. (2001) 29:13–27. doi: 10.1111/j.1748-720X.2001.tb00037.x
11. Ventura-Clapier R, Dworatzek E, Seeland U, Kararigas G, Arnal J, Brunelleschi S, et al. Sex in basic research: concepts in the cardiovascular field. Cardiovasc Res. (2017) 113:711–24. doi: 10.1093/cvr/cvx066
12. Richardson P, McKenna W, Bristow M, Maisch B, Mautner B, O’Connell J, et al. Report of the 1995 World Health Organization/International Society and Federation of Cardiology Task Force on the definition and classification of cardiomyopathies. Circulation. (1996) 93:841–2. doi: 10.1161/01.CIR.93.5.841
13. Risgaard B, Winkel B, Jabbari R, Behr E, Ingemann-Hansen O, Thomsen J, et al. Burden of sudden cardiac death in persons aged 1 to 49 years: nationwide study in Denmark. Circ Arrhythm Electrophysiol. (2014) 7:205–11. doi: 10.1161/CIRCEP.113.001421
14. Cho Y, Park T, Yang D, Park H, Chae J, Chae S, et al. Arrhythmogenic right ventricular cardiomyopathy and sudden cardiac death in young Koreans. Circ J. (2003) 67:925–8. doi: 10.1253/circj.67.925
15. Myers J, Fairweather D, Huber S, Cunningham M. Autoimmune myocarditis, valvulitis, and cardiomyopathy. Curr Protoc Immunol. (2013). Chapter 15:Unit 15.14.1-51. doi: 10.1002/0471142735.im1514s101
16. Bracamonte-Baran W, Cihakova D. Cardiac autoimmunity: myocarditis. Adv Exp Med Biol. (2017) 1003:187–221. doi: 10.1007/978-3-319-57613-8_10
18. Fung G, Luo H, Qiu Y, Yang D, McManus B. Myocarditis. Circ Res. (2016) 118:496–514. doi: 10.1161/CIRCRESAHA.115.306573
19. Caforio A, Pankuweit S, Arbustini E, Basso C, Gimeno-Blanes J, Felix S, et al. Current state of knowledge on aetiology, diagnosis, management, and therapy of myocarditis: a position statement of the European Society of Cardiology working group on myocardial and pericardial diseases. Eur Heart J. (2013) 34. 2636–48, 48a–d. doi: 10.1093/eurheartj/eht210
20. Bozkurt B, Colvin M, Cook J, Cooper L, Deswal A, Fonarow G, et al. Current diagnostic and treatment strategies for specific dilated cardiomyopathies: a scientific statement from the American Heart Association. Circulation. (2016) 134:e579–646. doi: 10.1161/CIR.0000000000000455
21. McMurray J, Adamopoulos S, Anker S, Auricchio A, Bohm M, Dickstein K, et al. ESC guidelines for the diagnosis and treatment of acute and chronic heart failure 2012: the task force for the diagnosis and treatment of acute and chronic heart failure 2012 of the European Society of Cardiology. developed in collaboration with the Heart Failure Association (HFA) of the ESC. Eur Heart J. (2012) 33: 1787–847.
22. Tschope C, Ammirati E, Bozkurt B, Caforio A, Cooper L, Felix S, et al. Myocarditis and inflammatory cardiomyopathy: current evidence and future directions. Nat Rev Cardiol. (2021) 18:169–93. doi: 10.1038/s41569-020-00435-x
23. Kociol R, Cooper L, Fang J, Moslehi J, Pang P, Sabe M, et al. Recognition and initial management of fulminant myocarditis: a scientific statement from the American Heart Association. Circulation. (2020) 141:e69–92. doi: 10.1161/CIR.0000000000000745
24. Ferreira V, Schulz-Menger J, Holmvang G, Kramer C, Carbone I, Sechtem U, et al. Cardiovascular magnetic resonance in nonischemic myocardial inflammation: expert recommendations. J Am Coll Cardiol. (2018) 72:3158–76. doi: 10.1016/j.jacc.2018.09.072
25. Rroku A, Kottwitz J, Heidecker B. Update on myocarditis - what we know so far and where we may be heading. Eur Heart J Acute Cardiovasc Care (2020) 22:2048872620910109. doi: 10.1177/2048872620910109
26. Schiebinger L, Leopold S, Miller V. Editorial policies for sex and gender analysis. Lancet. (2016) 388:2841–2. doi: 10.1016/S0140-6736(16)32392-3
27. Arbustini E, Narula N, Dec G, Reddy K, Greenberg B, Kushwaha S, et al. The MOGE(S) classification for a phenotype-genotype nomenclature of cardiomyopathy: endorsed by the World Heart Federation. J Am Coll Cardiol. (2013) 62:2046–72. doi: 10.1016/j.gheart.2013.11.001
28. Braunwald E. Cardiomyopathies: an overview. Circ Res. (2017) 121:711–21. doi: 10.1161/CIRCRESAHA.117.311812
29. Pinto Y, Elliott P, Arbustini E, Adler Y, Anastasakis A, Bohm M, et al. Proposal for a revised definition of dilated cardiomyopathy, hypokinetic non-dilated cardiomyopathy, and its implications for clinical practice: a position statement of the ESC working group on myocardial and pericardial diseases. Eur Heart J. (2016) 37:1850–8. doi: 10.1093/eurheartj/ehv727
30. Brigden W. Uncommon myocardial diseases; the non-coronary cardiomyopathies. Lancet. (1957) 273:1179–84. doi: 10.1016/S0140-6736(57)90159-9
31. Report of the WHO/ISFC task force on the definition and classification of cardiomyopathies. Br Heart J. (1980) 44:672–3. doi: 10.1136/hrt.44.6.672
32. Jain A, Norton N, Bruno K, Cooper L Jr., Atwal P, Fairweather D. Sex differences, genetic and environmental influences on dilated cardiomyopathy. J Clin Med. (2021) 10:2289. doi: 10.3390/jcm10112289
33. Maron B, Towbin J, Thiene G, Antzelevitch C, Corrado D, Arnett D, et al. Contemporary definitions and classification of the cardiomyopathies: an American Heart Association scientific statement from the council on clinical cardiology, heart failure and transplantation committee; quality of care and outcomes research and functional genomics and translational biology interdisciplinary working groups; and council on epidemiology and prevention. Circulation. (2006) 113:1807–16. doi: 10.1161/CIRCULATIONAHA.106.174287
34. Schultheiss H, Fairweather D, Caforio A, Escher F, Hershberger R, Lipshultz S, et al. Dilated cardiomyopathy. Nat Rev Dis Primers. (2019) 5:32. doi: 10.1038/s41572-019-0084-1
35. Wu L, Ong S, Talor M, Barin J, Baldeviano G, Kass D, et al. Cardiac fibroblasts mediate IL-17A-driven inflammatory dilated cardiomyopathy. J Exp Med. (2014) 211:1449–64. doi: 10.1084/jem.20132126
36. Diny N, Baldeviano G, Talor M, Barin J, Ong S, Bedja D, et al. Eosinophil-derived IL-4 drives progression of myocarditis to inflammatory dilated cardiomyopathy. J Exp Med. (2017) 214:943–57. doi: 10.1084/jem.20161702
37. McNamara D, Starling R, Cooper L, Boehmer J, Mather P, Janosko K, et al. Clinical and demographic predictors of outcomes in recent onset dilated cardiomyopathy: results of the IMAC (Intervention in Myocarditis and Acute Cardiomyopathy)-2 study. J Am Coll Cardiol. (2011) 58:1112–8. doi: 10.1016/j.jacc.2011.05.033
38. Kindermann I, Kindermann M, Kandolf R, Klingel K, Bultmann B, Muller T, et al. Predictors of outcome in patients with suspected myocarditis. Circulation. (2008) 118:639–48. doi: 10.1161/CIRCULATIONAHA.108.769489
39. Fairweather D, Kaya Z, Shellam G, Lawson C, Rose N. From infection to autoimmunity. J Autoimmun. (2001) 16:175–86. doi: 10.1006/jaut.2000.0492
40. Fairweather D, Frisancho-Kiss S, Yusung S, Barrett M, Davis S, Gatewood S, et al. Interferon-gamma protects against chronic viral myocarditis by reducing mast cell degranulation, fibrosis, and the profibrotic cytokines transforming growth factor-beta 1, interleukin-1 beta, and interleukin-4 in the heart. Am J Pathol. (2004) 165:1883–94. doi: 10.1016/S0002-9440(10)63241-5
41. Coronado M, Brandt J, Kim E, Bucek A, Bedja D, Abston. Testosterone and interleukin-1beta increase cardiac remodeling during coxsackievirus B3 myocarditis via serpin A 3n. Am J Physiol Heart Circ Physiol. (2012) 302:H1726–36. doi: 10.1152/ajpheart.00783.2011
42. Kuhl U, Pauschinger M, Noutsias M, Seeberg B, Bock T, Lassner D, et al. High prevalence of viral genomes and multiple viral infections in the myocardium of adults with “idiopathic” left ventricular dysfunction. Circulation. (2005) 111:887–93. doi: 10.1161/01.CIR.0000155616.07901.35
43. De Bellis A, De Angelis G, Fabris E, Cannata A, Merlo M, Sinagra G. Gender-related differences in heart failure: beyond the “one-size-fits-all” paradigm. Heart Fail Rev. (2020) 25:245–55. doi: 10.1007/s10741-019-09824-y
44. Maron B, Doerer J, Haas T, Tierney D, Mueller F. Sudden deaths in young competitive athletes: analysis of 1866 deaths in the United States, 1980-2006. Circulation. (2009) 119:1085–92. doi: 10.1161/CIRCULATIONAHA.108.804617
45. Bryde R, Cooper L Jr., Fairweather D, Di Florio D, Martinez M. Exercise after acute myocarditis: when and how to return to sports. Cardiol Clin. (2023) 41:107–15. doi: 10.1016/j.ccl.2022.08.009
46. Heidenreich P, Bozkurt B, Aguilar D, Allen L, Byun J, Colvin M, et al. 2022 AHA/ACC/HFSA guideline for the management of heart failure: a report of the American College of Cardiology/American Heart Association joint committee on clinical practice guidelines. J Am Coll Cardiol. (2022) 79:e263–421.
47. Roth G, Mensah G, Johnson C, Addolorato G, Ammirati E, Baddour L, et al. Global burden of cardiovascular diseases and risk factors, 1990-2019: update from the GBD 2019 study. J Am Coll Cardiol. (2020) 76:2982–3021.
48. Zhang K, Cheng X, Qu N, Song H, Luo Y, Ye T, et al. Global burden of cardiomyopathy and myocarditis in the older adults from 1990 to 2019. Front Public Health. (2022) 10:1018385. doi: 10.3389/fpubh.2022.1018385
49. Fu M, Kontogeorgos S, Thunstrom E, Zverkova Sandstrom T, Kroon C, Bollano E, et al. Trends in myocarditis incidence, complications and mortality in Sweden from 2000 to 2014. Sci Rep. (2022) 12:1810. doi: 10.1038/s41598-022-05951-z
50. Caforio A, Calabrese F, Angelini A, Tona F, Vinci A, Bottaro S, et al. A prospective study of biopsy-proven myocarditis: prognostic relevance of clinical and aetiopathogenetic features at diagnosis. Eur Heart J. (2007) 28:1326–33. doi: 10.1093/eurheartj/ehm076
51. Magnani J, Danik H, Dec G Jr., DiSalvo T. Survival in biopsy-proven myocarditis: a long-term retrospective analysis of the histopathologic, clinical, and hemodynamic predictors. Am Heart J. (2006) 151:463–70. doi: 10.1016/j.ahj.2005.03.037
52. Mason J, O’Connell J, Herskowitz A, Rose N, McManus B, Billingham M, et al. A clinical trial of immunosuppressive therapy for myocarditis. the myocarditis treatment trial investigators. N Engl J Med. (1995) 333:269–75. doi: 10.1056/NEJM199508033330501
53. Kyto V, Sipila J, Rautava P. The effects of gender and age on occurrence of clinically suspected myocarditis in adulthood. Heart. (2013) 99:1681–4. doi: 10.1136/heartjnl-2013-304449
54. Laufer-Perl M, Havakuk O, Shacham Y, Steinvil A, Letourneau-Shesaf S, Chorin E, et al. Sex-based differences in prevalence and clinical presentation among pericarditis and myopericarditis patients. Am J Emerg Med. (2017) 35:201–5. doi: 10.1016/j.ajem.2016.10.039
55. Coronado M, Bruno K, Blauwet L, Tschope C, Cunningham M, Pankuweit S, et al. Elevated sera sST2 is associated with heart failure in men ≤50 years old with myocarditis. J Am Heart Assoc. (2019) 8:e008968. doi: 10.1161/JAHA.118.008968
56. Lynge T, Nielsen T, Gregers Winkel B, Tfelt-Hansen J, Banner J. Sudden cardiac death caused by myocarditis in persons aged 1-49 years: a nationwide study of 14 294 deaths in Denmark. Forensic Sci Res. (2019) 4:247–56. doi: 10.1080/20961790.2019.1595352
57. Wong B, Christiansen J. Clinical characteristics and prognostic factors of myocarditis in New Zealand patients. Heart Lung Circ. (2020) 29:1139–45. doi: 10.1016/j.hlc.2020.01.007
58. Ozieranski K, Tyminska A, Kruk M, Kon B, Skwarek A, Opolski G, et al. Occurrence, trends, management and outcomes of patients hospitalized with clinically suspected myocarditis-ten-year perspectives from the MYO-PL nationwide database. J Clin Med. (2021) 10:4672. doi: 10.3390/jcm10204672
59. Baritussio A, Schiavo A, Basso C, Giordani A, Cheng C, Pontara E, et al. Predictors of relapse, death or heart transplantation in myocarditis before the introduction of immunosuppression: negative prognostic impact of female gender, fulminant onset, lower ejection fraction and serum autoantibodies. Eur J Heart Fail. (2022) 24:1033–44. doi: 10.1002/ejhf.2496
60. Afanasyeva M, Wang Y, Kaya Z, Park S, Zilliox M, Schofield B, et al. Experimental autoimmune myocarditis in A/J mice is an interleukin-4-dependent disease with a Th2 phenotype. Am J Pathol. (2001) 159:193–203. doi: 10.1016/S0002-9440(10)61685-9
61. Baldeviano G, Barin J, Talor M, Srinivasan S, Bedja D, Zheng D, et al. Interleukin-17A is dispensable for myocarditis but essential for the progression to dilated cardiomyopathy. Circ Res. (2010) 106:1646–55. doi: 10.1161/CIRCRESAHA.109.213157
62. Hershberger R, Hedges D, Morales A. Dilated cardiomyopathy: the complexity of a diverse genetic architecture. Nat Rev Cardiol. (2013) 10:531–47. doi: 10.1038/nrcardio.2013.105
63. Codd M, Sugrue D, Gersh B, Melton L III. Epidemiology of idiopathic dilated and hypertrophic cardiomyopathy. A population-based study in Olmsted County, Minnesota, 1975-1984. Circulation. (1989) 80:564–72. doi: 10.1161/01.CIR.80.3.564
64. Lopez-Ayala J, Pastor-Quirante F, Gonzalez-Carrillo J, Lopez-Cuenca D, Sanchez-Munoz J, Oliva-Sandoval M, et al. Genetics of myocarditis in arrhythmogenic right ventricular dysplasia. Heart Rhythm. (2015) 12:766–73. doi: 10.1016/j.hrthm.2015.01.001
65. Belkaya S, Kontorovich A, Byun M, Mulero-Navarro S, Bajolle F, Cobat A, et al. Autosomal recessive cardiomyopathy presenting as acute myocarditis. J Am Coll Cardiol. (2017) 69:1653–65. doi: 10.1016/j.jacc.2017.01.043
66. Martins D, Ovaert C, Khraiche D, Boddaert N, Bonnet D, Raimondi F. Myocardial inflammation detected by cardiac MRI in arrhythmogenic right ventricular cardiomyopathy: a paediatric case series. Int J Cardiol. (2018) 271:81–6. doi: 10.1016/j.ijcard.2018.05.116
67. Reichl K, Kreykes S, Martin C, Shenoy C. Desmoplakin variant-associated arrhythmogenic cardiomyopathy presenting as acute myocarditis. Circ Genom Precis Med. (2018) 11:e002373. doi: 10.1161/CIRCGEN.118.002373
68. Kissopoulou A, Fernlund E, Holmgren C, Isaksson E, Karlsson J, Green H, et al. Monozygotic twins with myocarditis and a novel likely pathogenic desmoplakin gene variant. ESC Heart Fail. (2020) 7:1210–6. doi: 10.1002/ehf2.12658
69. Piriou N, Marteau L, Kyndt F, Serfaty J, Toquet C, Le Gloan L, et al. Familial screening in case of acute myocarditis reveals inherited arrhythmogenic left ventricular cardiomyopathies. ESC Heart Fail. (2020) 7:1520–33. doi: 10.1002/ehf2.12686
70. Poller W, Haas J, Klingel K, Kuhnisch J, Gast M, Kaya Z, et al. Familial recurrent myocarditis triggered by exercise in patients with a truncating variant of the desmoplakin gene. J Am Heart Assoc. (2020) 9:e015289. doi: 10.1161/JAHA.119.015289
71. Alley R, Grizzard J, Rao K, Markley R, Trankle C. Inflammatory episodes of desmoplakin cardiomyopathy masquerading as myocarditis: unique features on cardiac magnetic resonance imaging. JACC Cardiovasc Imaging. (2021) 14:1466–9. doi: 10.1016/j.jcmg.2020.07.028
72. Scheel P III, Murray B, Tichnell C, James C, Tandri H, Calkins H, et al. Arrhythmogenic right ventricular cardiomyopathy presenting as clinical myocarditis in women. Am J Cardiol. (2021) 145:128–34. doi: 10.1016/j.amjcard.2020.12.090
73. Kontorovich A, Tang Y, Patel N, Georgievskaya Z, Shadrina M, Williams N, et al. Burden of cardiomyopathic genetic variation in lethal pediatric myocarditis. Circ Genom Precis Med. (2021) 14:e003426. doi: 10.1161/CIRCGEN.121.003426
74. Seidel F, Holtgrewe M, Al-Wakeel-Marquard N, Opgen-Rhein B, Dartsch J, Herbst C, et al. Pathogenic variants associated with dilated cardiomyopathy predict outcome in pediatric myocarditis. Circ Genom Precis Med. (2021) 14:e003250. doi: 10.1161/CIRCGEN.120.003250
75. Ammirati E, Raimondi F, Piriou N, Sardo Infirri L, Mohiddin S, Mazzanti A, et al. Acute myocarditis associated with desmosomal gene variants. JACC Heart Fail. (2022) 10:714–27. doi: 10.1016/j.jchf.2022.06.013
76. McNally E, Selgrade D. Genetic testing for myocarditis. JACC Heart Fail. (2022) 10:728–30. doi: 10.1016/j.jchf.2022.07.003
77. Artico J, Merlo M, Delcaro G, Cannata A, Gentile P, De Angelis G, et al. Lymphocytic myocarditis: a genetically predisposed disease? J Am Coll Cardiol. (2020) 75:3098–100. doi: 10.1016/j.jacc.2020.04.048
78. Kontorovich A, Patel N, Moscati A, Richter F, Peter I, Purevjav E, et al. Myopathic cardiac genotypes increase risk for myocarditis. JACC Basic Transl Sci. (2021) 6:584–92. doi: 10.1016/j.jacbts.2021.06.001
79. Musunuru K, Hershberger R, Day S, Klinedinst N, Landstrom A, Parikh V, et al. Genetic testing for inherited cardiovascular diseases: a scientific statement from the American Heart Association. Circ Genom Precis Med. (2020) 13:e000067. doi: 10.1161/HCG.0000000000000067
80. McNally E, Golbus J, Puckelwartz M. Genetic mutations and mechanisms in dilated cardiomyopathy. J Clin Invest. (2013) 123:19–26. doi: 10.1172/JCI62862
81. Harakalova M, Kummeling G, Sammani A, Linschoten M, Baas A, van der Smagt J, et al. A systematic analysis of genetic dilated cardiomyopathy reveals numerous ubiquitously expressed and muscle-specific genes. Eur J Heart Fail. (2015) 17:484–93. doi: 10.1002/ejhf.255
82. Kayvanpour E, Sedaghat-Hamedani F, Amr A, Lai A, Haas J, Holzer D, et al. Genotype-phenotype associations in dilated cardiomyopathy: meta-analysis on more than 8000 individuals. Clin Res Cardiol. (2017) 106:127–39. doi: 10.1007/s00392-016-1033-6
83. de Denus S, Mottet F, Korol S, Feroz Zada Y, Provost S, Mongrain I, et al. A genetic association study of heart failure: more evidence for the role of BAG3 in idiopathic dilated cardiomyopathy. ESC Heart Fail. (2020) 7:4384–9. doi: 10.1002/ehf2.12934
84. Liu L, Schaub M, Sirota M, Butte A. Sex differences in disease risk from reported genome-wide association study findings. Hum Genet. (2012) 131:353–64. doi: 10.1007/s00439-011-1081-y
85. Pelliccia F, Limongelli G, Autore C, Gimeno-Blanes J, Basso C, Elliott P. Sex-related differences in cardiomyopathies. Int J Cardiol. (2019) 286:239–43. doi: 10.1016/j.ijcard.2018.10.091
86. Argiro A, Ho C, Day S, van der Velden J, Cerbai E, Saberi S, et al. Sex-related differences in genetic cardiomyopathies. J Am Heart Assoc. (2022) 11:e024947. doi: 10.1161/JAHA.121.024947
87. Akhtar M, Lorenzini M, Cicerchia M, Ochoa J, Hey T, Sabater Molina M, et al. Clinical phenotypes and prognosis of dilated cardiomyopathy caused by truncating variants in the TTN gene. Circ Heart Fail. (2020) 13:e006832. doi: 10.1161/CIRCHEARTFAILURE.119.006832
88. Ware J, Li J, Mazaika E, Yasso C, DeSouza T, Cappola T, et al. Shared genetic predisposition in peripartum and dilated cardiomyopathies. N Engl J Med. (2016) 374:233–41. doi: 10.1056/NEJMoa1505517
89. Wahbi K, Ben Yaou R, Gandjbakhch E, Anselme F, Gossios T, Lakdawala N, et al. Development and validation of a new risk prediction score for life-threatening ventricular tachyarrhythmias in laminopathies. Circulation. (2019) 140:293–302. doi: 10.1161/CIRCULATIONAHA.119.044322
90. Ollila L, Nikus K, Holmstrom M, Jalanko M, Jurkko R, Kaartinen M, et al. Clinical disease presentation and ECG characteristics of LMNA mutation carriers. Open Heart. (2017) 4:e000474. doi: 10.1136/openhrt-2016-000474
91. Ortiz-Genga M, Cuenca S, Dal Ferro M, Zorio E, Salgado-Aranda R, Climent V, et al. Truncating FLNC mutations are associated with higher risk dilated and arrhythmogenic cardiomyopathies. J Am Coll Cardiol. (2016) 68:2440–51. doi: 10.1016/j.jacc.2016.09.927
92. Hey T, Rasmussen T, Madsen T, Aagaard M, Harbo M, Molgaard H, et al. Pathogenic RBM20-variants are associated with a severe disease expression in male patients with dilated cardiomyopathy. Circ Heart Fail. (2019) 12:e005700. doi: 10.1161/CIRCHEARTFAILURE.118.005700
93. Dworatzek E, Baczko I, Kararigas G. Effects of aging on cardiac extracellular matrix in men and women. Proteomics Clin Appl. (2016) 10:84–91. doi: 10.1002/prca.201500031
94. Gray H. Anatomy of the Human Body. 20th ed. Philedelphia, PA: Lea and Febiger (1918). doi: 10.5962/bhl.title.20311
95. Molina D, DiMaio V. Normal organ weights in women: part II-the brain, lungs, liver, spleen, and kidneys. Am J Forensic Med Pathol. (2015) 36:182–7. doi: 10.1097/PAF.0000000000000175
96. Molina D, DiMaio V. Normal organ weights in men: part I-the heart. Am J Forensic Med Pathol. (2012) 33:362–7. doi: 10.1097/PAF.0b013e31823d298b
97. Sheikhazadi A, Sadr S, Ghadyani M, Taheri S, Manouchehri A, Nazparvar B, et al. Study of the normal internal organ weights in Tehran’s population. J Forensic Leg Med. (2010) 17:78–83. doi: 10.1016/j.jflm.2009.07.012
98. de Simone G, Devereux R, Daniels S, Meyer R. Gender differences in left ventricular growth. Hypertension. (1995) 26(6 Pt. 1):979–83. doi: 10.1161/01.HYP.26.6.979
99. Olivetti G, Giordano G, Corradi D, Melissari M, Lagrasta C, Gambert S, et al. Gender differences and aging: effects on the human heart. J Am Coll Cardiol. (1995) 26:1068–79. doi: 10.1016/0735-1097(95)00282-8
100. Dannenberg A, Levy D, Garrison R. Impact of age on echocardiographic left ventricular mass in a healthy population (the Framingham Study). Am J Cardiol. (1989) 64:1066–8. doi: 10.1016/0002-9149(89)90816-3
101. Kim S, Apple S, Mintz G, McMillan T, Canos D, Maehara A, et al. The importance of gender on coronary artery size: in-vivo assessment by intravascular ultrasound. Clin Cardiol. (2004) 27:291–4. doi: 10.1002/clc.4960270511
102. Burt V, Whelton P, Roccella E, Brown C, Cutler J, Higgins M, et al. Prevalence of hypertension in the US adult population. results from the third national health and nutrition examination survey, 1988-1991. Hypertension. (1995) 25:305–13. doi: 10.1161/01.HYP.25.3.305
103. Stamler J, Stamler R, Riedlinger W, Algera G, Roberts R. Hypertension screening of 1 million Americans. community hypertension evaluation clinic (CHEC) program, 1973 through 1975. JAMA. (1976) 235:2299–306. doi: 10.1001/jama.235.21.2299
104. Bazett H. The time relations of the blood-pressure changes after excision of the adrenal glands, with some observations on blood volume changes. J Physiol. (1920) 53:320–39. doi: 10.1113/jphysiol.1920.sp001881
105. Chung A, Das S, Leonard D, Peshock R, Kazi F, Abdullah S, et al. Women have higher left ventricular ejection fractions than men independent of differences in left ventricular volume: the Dallas Heart Study. Circulation. (2006) 113:1597–604. doi: 10.1161/CIRCULATIONAHA.105.574400
106. Merz C, Moriel M, Rozanski A, Klein J, Berman D. Gender-related differences in exercise ventricular function among healthy subjects and patients. Am Heart J. (1996) 131:704–9. doi: 10.1016/S0002-8703(96)90274-4
107. Regitz-Zagrosek V, Seeland U. Sex and gender differences in myocardial hypertrophy and heart failure. Wien Med Wochenschr. (2011) 161:109–16. doi: 10.1007/s10354-011-0892-8
108. Vitale C, Mendelsohn M, Rosano G. Gender differences in the cardiovascular effect of sex hormones. Nat Rev Cardiol. (2009) 6:532–42. doi: 10.1038/nrcardio.2009.105
109. Queiros A, Eschen C, Fliegner D, Kararigas G, Dworatzek E, Westphal C, et al. Sex- and estrogen-dependent regulation of a miRNA network in the healthy and hypertrophied heart. Int J Cardiol. (2013) 169:331–8. doi: 10.1016/j.ijcard.2013.09.002
110. Meyer S, van der Meer P, van Tintelen J, van den Berg M. Sex differences in cardiomyopathies. Eur J Heart Fail. (2014) 16:238–47. doi: 10.1002/ejhf.15
111. Buskiewicz I, Huber S, Fairweather D. Sex hormone receptor expression in the immune system. 1st ed. In: M Gretchen Neigh editor. Sex Differences in Physiology. Amsterdam: Elsevier Inc (2016). p. 45–60. doi: 10.1016/B978-0-12-802388-4.00004-5
112. Regitz-Zagrosek V. Therapeutic implications of the gender-specific aspects of cardiovascular disease. Nat Rev Drug Discov. (2006) 5:425–38. doi: 10.1038/nrd2032
113. Sharma A, Aoun P, Wigham J, Weist S, Veldhuis J. Estradiol, but not testosterone, heightens cortisol-mediated negative feedback on pulsatile ACTH secretion and ACTH approximate entropy in unstressed older men and women. Am J Physiol Regul Integr Comp Physiol. (2014) 306:R627–35. doi: 10.1152/ajpregu.00551.2013
114. Leinwand L. Sex is a potent modifier of the cardiovascular system. J Clin Invest. (2003) 112:302–7. doi: 10.1172/JCI200319429
115. Beetler D, Di Florio D, Bruno K, Ikezu T, March K, Cooper L Jr., et al. Extracellular vesicles as personalized medicine. Mol Aspects Med. (2022) 91:101155. doi: 10.1016/j.mam.2022.101155
116. Cihakova D, Sharma R, Fairweather D, Afanasyeva M, Rose N. Animal models for autoimmune myocarditis and autoimmune thyroiditis. Methods Mol Med. (2004) 102:175–93. doi: 10.1385/1-59259-805-6:175
117. Fairweather D, Stafford K, Sung Y. Update on coxsackievirus B3 myocarditis. Curr Opin Rheumatol. (2012) 24:401–7. doi: 10.1097/BOR.0b013e328353372d
118. Poli V, Bruno K, Fairweather D. Autoimmune myocarditis: animal models. In: A Caforio editor. Myocarditis: Pathogenesis, Diagnosis and Treatment. Cham: Springer International Publishing (2020). p. 111–28. doi: 10.1007/978-3-030-35276-9_6
119. Huber S, Job L, Woodruff J. Sex-related differences in the pattern of coxsackievirus B-3-induced immune spleen cell cytotoxicity against virus-infected myofibers. Infect Immun. (1981) 32:68–73. doi: 10.1128/iai.32.1.68-73.1981
120. Lyden D, Olszewski J, Feran M, Job L, Huber S. Coxsackievirus B-3-induced myocarditis. effect of sex steroids on viremia and infectivity of cardiocytes. Am J Pathol. (1987) 126:432–8.
121. Huber S, Pfaeffle B. Differential Th1 and Th2 cell responses in male and female BALB/c mice infected with coxsackievirus group B type 3. J Virol. (1994) 68:5126–32. doi: 10.1128/jvi.68.8.5126-5132.1994
122. Huber S, Sartini D. T cells expressing the Vgamma1 T-cell receptor enhance virus-neutralizing antibody response during coxsackievirus B3 infection of BALB/c mice: differences in male and female mice. Viral Immunol. (2005) 18:730–9. doi: 10.1089/vim.2005.18.730
123. Case L, Toussaint L, Moussawi M, Roberts B, Saligrama N, Brossay L, et al. Chromosome Y regulates survival following murine coxsackievirus B3 infection. G3 (Bethesda). (2012) 2:115–21. doi: 10.1534/g3.111.001610
124. Umiker B, Andersson S, Fernandez L, Korgaokar P, Larbi A, Pilichowska M, et al. Dosage of X-linked toll-like receptor 8 determines gender differences in the development of systemic lupus erythematosus. Eur J Immunol. (2014) 44:1503–16. doi: 10.1002/eji.201344283
125. Souyris M, Mejia J, Chaumeil J, Guery J. Female predisposition to TLR7-driven autoimmunity: gene dosage and the escape from X chromosome inactivation. Semin Immunopathol. (2019) 41:153–64. doi: 10.1007/s00281-018-0712-y
126. Roberts B, Dragon J, Moussawi M, Huber S. Sex-specific signaling through toll-like receptors 2 and 4 contributes to survival outcome of coxsackievirus B3 infection in C57Bl/6 mice. Biol Sex Differ. (2012) 3:25. doi: 10.1186/2042-6410-3-25
127. Roberts B, Moussawi M, Huber S. Sex differences in TLR2 and TLR4 expression and their effect on coxsackievirus-induced autoimmune myocarditis. Exp Mol Pathol. (2013) 94:58–64. doi: 10.1016/j.yexmp.2012.06.005
128. Huber S. ERbeta and ERalpha differentially regulate NKT and Vgamma4(+) T-cell activation and T-regulatory cell response in coxsackievirus B3 infected mice. J Clin Cell Immunol. (2015) 6:1–9. doi: 10.4172/2155-9899.1000372
129. Fang M, Zhang A, Du Y, Lu W, Wang J, Minze L, et al. TRIM18 is a critical regulator of viral myocarditis and organ inflammation. J Biomed Sci. (2022) 29:55. doi: 10.1186/s12929-022-00840-z
130. Quaderi N, Schweiger S, Gaudenz K, Franco B, Rugarli E, Berger W, et al. Opitz G/BBB syndrome, a defect of midline development, is due to mutations in a new RING finger gene on Xp22. Nat Genet. (1997) 17:285–91. doi: 10.1038/ng1197-285
131. Fairweather D, Frisancho-Kiss S, Njoku D, Nyland J, Kaya Z, Yusung S, et al. Complement receptor 1 and 2 deficiency increases coxsackievirus B3-induced myocarditis, dilated cardiomyopathy, and heart failure by increasing macrophages, IL-1beta, and immune complex deposition in the heart. J Immunol. (2006) 176:3516–24. doi: 10.4049/jimmunol.176.6.3516
132. Frisancho-Kiss S, Davis S, Nyland J, Frisancho J, Cihakova D, Barrett M, et al. Cutting edge: cross-regulation by TLR4 and T cell Ig mucin-3 determines sex differences in inflammatory heart disease. J Immunol. (2007) 178:6710–4. doi: 10.4049/jimmunol.178.11.6710
133. Fairweather D, Frisancho-Kiss S, Gatewood S, Njoku D, Steele R, Barrett M, et al. Mast cells and innate cytokines are associated with susceptibility to autoimmune heart disease following coxsackievirus B3 infection. Autoimmunity. (2004) 37:131–45. doi: 10.1080/0891693042000196200
134. Frisancho-Kiss S, Nyland J, Davis S, Frisancho J, Barrett M, Rose N, et al. Sex differences in coxsackievirus B3-induced myocarditis: IL-12Rbeta1 signaling and IFN-gamma increase inflammation in males independent from STAT4. Brain Res. (2006) 1126:139–47. doi: 10.1016/j.brainres.2006.08.003
135. Frisancho-Kiss S, Coronado M, Frisancho J, Lau V, Rose N, Klein S, et al. Gonadectomy of male BALB/c mice increases Tim-3(+) alternatively activated M2 macrophages, Tim-3(+) T cells, Th2 cells and Treg in the heart during acute coxsackievirus-induced myocarditis. Brain Behav Immun. (2009) 23:649–57. doi: 10.1016/j.bbi.2008.12.002
136. Fairweather D, Yusung S, Frisancho S, Barrett M, Gatewood S, Steele R, et al. IL-12 receptor beta 1 and Toll-like receptor 4 increase IL-1 beta- and IL-18-associated myocarditis and coxsackievirus replication. J Immunol. (2003) 170:4731–7. doi: 10.4049/jimmunol.170.9.4731
137. Di Florio D, Sin J, Coronado M, Atwal P, Fairweather D. Sex differences in inflammation, redox biology, mitochondria and autoimmunity. Redox Biol. (2020) 31:101482. doi: 10.1016/j.redox.2020.101482
138. Fairweather D, Frisancho-Kiss S, Yusung S, Barrett M, Davis S, Steele R, et al. IL-12 protects against coxsackievirus B3-induced myocarditis by increasing IFN-gamma and macrophage and neutrophil populations in the heart. J Immunol. (2005) 174:261–9. doi: 10.4049/jimmunol.174.1.261
139. Abston Coronado M, Bucek A, Bedja D, Shin J, Kim J, et al. Th2 regulation of viral myocarditis in mice: different roles for TLR3 versus TRIF in progression to chronic disease. Clin Dev Immunol. (2012) 2012:129486. doi: 10.1155/2012/129486
140. Abston Coronado M, Bucek A, Onyimba J, Brandt J, Frisancho J, et al. TLR3 deficiency induces chronic inflammatory cardiomyopathy in resistant mice following coxsackievirus B3 infection: role for IL-4. Am J Physiol Regul Integr Comp Physiol. (2013) 304:R267–77. doi: 10.1152/ajpregu.00516.2011
141. Abston Barin J, Cihakova D, Bucek A, Coronado M, Brandt J, et al. IL-33 independently induces eosinophilic pericarditis and cardiac dilation: ST2 improves cardiac function. Circ Heart Fail. (2012) 5:366–75. doi: 10.1161/CIRCHEARTFAILURE.111.963769
142. Myers J, Cooper L, Kem D, Stavrakis S, Kosanke S, Shevach E, et al. Cardiac myosin-Th17 responses promote heart failure in human myocarditis. JCI Insight. (2016) 1:e85851. doi: 10.1172/jci.insight.85851
143. Lambert K, Curran E, Judy B, Milligan G, Lubahn D, Estes D. Estrogen receptor alpha (ERalpha) deficiency in macrophages results in increased stimulation of CD4+ T cells while 17beta-estradiol acts through ERalpha to increase IL-4 and GATA-3 expression in CD4+ T cells independent of antigen presentation. J Immunol. (2005) 175:5716–23. doi: 10.4049/jimmunol.175.9.5716
144. Carreras E, Turner S, Paharkova-Vatchkova V, Mao A, Dascher C, Kovats S. Estradiol acts directly on bone marrow myeloid progenitors to differentially regulate GM-CSF or Flt3 ligand-mediated dendritic cell differentiation. J Immunol. (2008) 180:727–38. doi: 10.4049/jimmunol.180.2.727
145. Douin-Echinard V, Laffont S, Seillet C, Delpy L, Krust A, Chambon P, et al. Estrogen receptor alpha, but not beta, is required for optimal dendritic cell differentiation and CD40-induced cytokine production. J Immunol. (2008) 180:3661–9. doi: 10.4049/jimmunol.180.6.3661
146. Tsutsumi S, Zhang X, Takata K, Takahashi K, Karas R, Kurachi H, et al. Differential regulation of the inducible nitric oxide synthase gene by estrogen receptors 1 and 2. J Endocrinol. (2008) 199:267–73. doi: 10.1677/JOE-07-0292
147. Liu H, Buenafe A, Matejuk A, Ito A, Zamora A, Dwyer J, et al. Estrogen inhibition of EAE involves effects on dendritic cell function. J Neurosci Res. (2002) 70:238–48. doi: 10.1002/jnr.10409
148. Matthews J, Gustafsson J. Estrogen signaling: a subtle balance between ER alpha and ER beta. Mol Interv. (2003) 3:281–92. doi: 10.1124/mi.3.5.281
149. Lindberg M, Moverare S, Skrtic S, Gao H, Dahlman-Wright K, Gustafsson J, et al. Estrogen receptor (ER)-beta reduces ERalpha-regulated gene transcription, supporting a “ying yang” relationship between ERalpha and ERbeta in mice. Mol Endocrinol. (2003) 17:203–8. doi: 10.1210/me.2002-0206
150. Paech K, Webb P, Kuiper G, Nilsson S, Gustafsson J, Kushner P, et al. Differential ligand activation of estrogen receptors ERalpha and ERbeta at AP1 sites. Science. (1997) 277:1508–10. doi: 10.1126/science.277.5331.1508
151. Smithson G, Couse J, Lubahn D, Korach K, Kincade P. The role of estrogen receptors and androgen receptors in sex steroid regulation of B lymphopoiesis. J Immunol. (1998) 161:27–34. doi: 10.4049/jimmunol.161.1.27
152. Grimaldi C, Cleary J, Dagtas A, Moussai D, Diamond B. Estrogen alters thresholds for B cell apoptosis and activation. J Clin Invest. (2002) 109:1625–33. doi: 10.1172/JCI0214873
153. Islander U, Erlandsson M, Hasseus B, Jonsson C, Ohlsson C, Gustafsson J, et al. Influence of oestrogen receptor alpha and beta on the immune system in aged female mice. Immunology. (2003) 110:149–57. doi: 10.1046/j.1365-2567.2003.01704.x
154. Paharkova-Vatchkova V, Maldonado R, Kovats S. Estrogen preferentially promotes the differentiation of CD11c+ CD11b(intermediate) dendritic cells from bone marrow precursors. J Immunol. (2004) 172:1426–36. doi: 10.4049/jimmunol.172.3.1426
155. Ito A, Bebo B Jr., Matejuk A, Zamora A, Silverman M, Fyfe-Johnson A, et al. Estrogen treatment down-regulates TNF-alpha production and reduces the severity of experimental autoimmune encephalomyelitis in cytokine knockout mice. J Immunol. (2001) 167:542–52. doi: 10.4049/jimmunol.167.1.542
156. Stein B, Yang M. Repression of the interleukin-6 promoter by estrogen receptor is mediated by NF-kappa B and C/EBP beta. Mol Cell Biol. (1995) 15:4971–9. doi: 10.1128/MCB.15.9.4971
157. Karpuzoglu-Sahin E, Hissong B, Ansar Ahmed S. Interferon-gamma levels are upregulated by 17-beta-estradiol and diethylstilbestrol. J Reprod Immunol. (2001) 52:113–27. doi: 10.1016/S0165-0378(01)00117-6
158. Polanczyk M, Hopke C, Huan J, Vandenbark A, Offner H. Enhanced FoxP3 expression and treg cell function in pregnant and estrogen-treated mice. J Neuroimmunol. (2005) 170:85–92. doi: 10.1016/j.jneuroim.2005.08.023
159. Tai P, Wang J, Jin H, Song X, Yan J, Kang Y, et al. Induction of regulatory T cells by physiological level estrogen. J Cell Physiol. (2008) 214:456–64. doi: 10.1002/jcp.21221
160. Siripanthong B, Asatryan B, Hanff T, Chatha S, Khanji M, Ricci F, et al. The pathogenesis and long-term consequences of COVID-19 cardiac injury. JACC Basic Transl Sci. (2022) 7:294–308. doi: 10.1016/j.jacbts.2021.10.011
161. Martin T, Tawfik S, Moravec C, Pak T, Kirk J. BAG3 expression and sarcomere localization in the human heart are linked to HSF-1 and are differentially affected by sex and disease. Am J Physiol Heart Circ Physiol. (2021) 320:H2339–50. doi: 10.1152/ajpheart.00419.2020
162. Patriki D, Kottwitz J, Berg J, Landmesser U, Luscher T, Heidecker B. Clinical presentation and laboratory findings in men versus women with myocarditis. J Womens Health (Larchmt). (2020) 29:193–9. doi: 10.1089/jwh.2018.7618
163. Lala A, Tayal U, Hamo C, Youmans Q, Al-Khatib S, Bozkurt B, et al. Sex differences in heart failure. J Card Fail. (2022) 28:477–98. doi: 10.1016/j.cardfail.2021.10.006
164. Regensteiner J, Golden S, Huebschmann A, Barrett-Connor E, Chang A, Chyun D, et al. Sex differences in the cardiovascular consequences of diabetes mellitus: a scientific statement from the American heart association. Circulation. (2015) 132:2424–47. doi: 10.1161/CIR.0000000000000343
165. Cesaroni G, Mureddu G, Agabiti N, Mayer F, Stafoggia M, Forastiere F, et al. Sex differences in factors associated with heart failure and diastolic left ventricular dysfunction: a cross-sectional population-based study. BMC Public Health. (2021) 21:415. doi: 10.1186/s12889-021-10442-3
166. Dewan P, Rorth R, Raparelli V, Campbell R, Shen L, Jhund P, et al. Sex-related differences in heart failure with preserved ejection fraction. Circ Heart Fail. (2019) 12:e006539. doi: 10.1161/CIRCHEARTFAILURE.119.006539
167. Sinha A, Ning H, Carnethon M, Allen N, Wilkins J, Lloyd-Jones D, et al. Race- and sex-specific population attributable fractions of incident heart failure: a population-based cohort study from the lifetime risk pooling project. Circ Heart Fail. (2021) 14:e008113. doi: 10.1161/CIRCHEARTFAILURE.120.008113
168. Cleland J, Swedberg K, Follath F, Komajda M, Cohen-Solal A, Aguilar J, et al. The euroheart failure survey programme– a survey on the quality of care among patients with heart failure in Europe. Part 1: patient characteristics and diagnosis. Eur Heart J. (2003) 24:442–63. doi: 10.1016/S0195-668X(02)00823-0
169. Barsheshet A, Brenyo A, Goldenberg I, Moss A. Sex-related differences in patients’ responses to heart failure therapy. Nat Rev Cardiol. (2012) 9:234–42. doi: 10.1038/nrcardio.2012.10
170. Lenzen M, Rosengren A, Scholte op Reimer WJ, Follath F, Boersma E, Simoons ML, et al. Management of patients with heart failure in clinical practice: differences between men and women. Heart. (2008) 94:e10. doi: 10.1136/hrt.2006.099523
171. Robinson D, Huber S, Moussawi M, Roberts B, Teuscher C, Watkins R, et al. Sex chromosome complement contributes to sex differences in coxsackievirus B3 but not influenza A virus pathogenesis. Biol Sex Differ. (2011) 2:8. doi: 10.1186/2042-6410-2-8
172. Matsumori A, Auda M, Bruno K, Shapiro K, Kato T, Nakamura T, et al. Cardiovascular factors associated with COVID-19 from an international registry of primarily Japanese patients. Diagnostics (Basel). (2022) 12:2350. doi: 10.3390/diagnostics12102350
173. Peng Y, Zhou B, Wang Y, Shi S, Zhang K, Zhang L, et al. Analysis of IL-17 gene polymorphisms in Chinese patients with dilated cardiomyopathy. Hum Immunol. (2013) 74:635–9. doi: 10.1016/j.humimm.2013.01.019
174. Kottwitz J, Bruno K, Berg J, Salomon G, Fairweather D, Elhassan M, et al. Myoglobin for detection of high-risk patients with acute myocarditis. J Cardiovasc Transl Res. (2020) 13:853–63. doi: 10.1007/s12265-020-09957-8
175. Blanco-Dominguez R, Sanchez-Diaz R, de la Fuente H, Jimenez-Borreguero LJ, Matesanz-Marin A, Relano M, et al. A novel circulating MicroRNA for the detection of acute myocarditis.. N Engl J Med. (2021) 384:2014–27.
176. Wang J, He M, Li H, Chen Y, Nie X, Cai Y, et al. Soluble ST2 is a sensitive and specific biomarker for fulminant myocarditis. J Am Heart Assoc. (2022) 11:e024417. doi: 10.1161/JAHA.121.024417
177. Tamargo J, Caballero R, Delpon E. Sex-related differences in the pharmacological treatment of heart failure. Pharmacol Ther. (2022) 229:107891. doi: 10.1016/j.pharmthera.2021.107891
178. Soldin O, Mattison D. Sex differences in pharmacokinetics and pharmacodynamics. Clin Pharmacokinet. (2009) 48:143–57. doi: 10.2165/00003088-200948030-00001
179. Rosano G, Lewis B, Agewall S, Wassmann S, Vitale C, Schmidt H, et al. Gender differences in the effect of cardiovascular drugs: a position document of the working group on pharmacology and drug therapy of the ESC. Eur Heart J. (2015) 36:2677–80. doi: 10.1093/eurheartj/ehv161
180. Jochmann N, Stangl K, Garbe E, Baumann G, Stangl V. Female-specific aspects in the pharmacotherapy of chronic cardiovascular diseases. Eur Heart J. (2005) 26:1585–95. doi: 10.1093/eurheartj/ehi397
181. Writing Committee ACC/AHA Joint Committee Members; 2022 AHA/ACC/HFSA guideline for the management of heart failure. J Card Fail. (2022) 28:e1–167. doi: 10.1016/j.cardfail.2022.03.346
182. Santema B, Ouwerkerk W, Tromp J, Sama I, Ravera A, Regitz-Zagrosek V, et al. Identifying optimal doses of heart failure medications in men compared with women: a prospective, observational, cohort study. Lancet. (2019) 394:1254–63. doi: 10.1016/S0140-6736(19)31792-1
183. Lam C, Arnott C, Beale A, Chandramouli C, Hilfiker-Kleiner D, Kaye D, et al. Sex differences in heart failure. Eur Heart J. (2019) 40:3859c–68c. doi: 10.1093/eurheartj/ehz835
184. Petrie M, Dawson N, Murdoch D, Davie A, McMurray J. Failure of women’s hearts. Circulation. (1999) 99:2334–41. doi: 10.1161/01.CIR.99.17.2334
185. Norberg H. Clinical trial enrolment favours men. Eur Heart J. (2019) 40:1104–5. doi: 10.1093/eurheartj/ehz146
186. Tamargo J, Rosano G, Walther T, Duarte J, Niessner A, Kaski J, et al. Gender differences in the effects of cardiovascular drugs. Eur Heart J Cardiovasc Pharmacother. (2017) 3:163–82. doi: 10.1093/ehjcvp/pvw042
187. O’Meara E, Clayton T, McEntegart M, McMurray J, Pina I, Granger C, et al. Sex differences in clinical characteristics and prognosis in a broad spectrum of patients with heart failure: results of the candesartan in heart failure: assessment of reduction in mortality and morbidity (CHARM) program. Circulation. (2007) 115:3111–20. doi: 10.1161/CIRCULATIONAHA.106.673442
188. Baumhakel M, Muller U, Bohm M. Influence of gender of physicians and patients on guideline-recommended treatment of chronic heart failure in a cross-sectional study. Eur J Heart Fail. (2009) 11:299–303. doi: 10.1093/eurjhf/hfn041
189. Gouni-Berthold I, Berthold H. Role of physician gender in drug therapy. Handb Exp Pharmacol. (2012) 214:183–208. doi: 10.1007/978-3-642-30726-3_10
190. Kayibanda J, Girouard C, Gregoire J, Demers E, Moisan J. Adherence to the evidence-based heart failure drug treatment: are there sex-specific differences among new users? Res Social Adm Pharm. (2018) 14:915–20. doi: 10.1016/j.sapharm.2017.10.010
191. Greiten L, Holditch S, Arunachalam S, Miller V. Should there be sex-specific criteria for the diagnosis and treatment of heart failure? J Cardiovasc Transl Res. (2014) 7:139–55. doi: 10.1007/s12265-013-9514-8
192. Simon T, Mary-Krause M, Funck-Brentano C, Jaillon P. Sex differences in the prognosis of congestive heart failure: results from the cardiac insufficiency bisoprolol study (CIBIS II). Circulation. (2001) 103:375–80. doi: 10.1161/01.CIR.103.3.375
193. Ghali J, Pina I, Gottlieb S, Deedwania P, Wikstrand J, Group M. Metoprolol CR/XL in female patients with heart failure: analysis of the experience in metoprolol extended-release randomized intervention trial in heart failure (MERIT-HF). Circulation. (2002) 105:1585–91. doi: 10.1161/01.CIR.0000012546.20194.33
194. Ghali J, Krause-Steinrauf H, Adams K, Khan S, Rosenberg Y, Yancy C, et al. Gender differences in advanced heart failure: insights from the BEST study. J Am Coll Cardiol. (2003) 42:2128–34. doi: 10.1016/j.jacc.2003.05.012
195. Sandberg K, Ji H. Sex differences in primary hypertension. Biol Sex Differ. (2012) 3:7. doi: 10.1186/2042-6410-3-7
196. Fischer M, Baessler A, Schunkert H. Renin angiotensin system and gender differences in the cardiovascular system. Cardiovasc Res. (2002) 53:672–7. doi: 10.1016/S0008-6363(01)00479-5
197. Kuroski de Bold ML. Estrogen, natriuretic peptides and the renin-angiotensin system. Cardiovasc Res. (1999) 41:524–31. doi: 10.1016/S0008-6363(98)00324-1
198. Karjalainen A, Ruskoaho H, Vuolteenaho O, Heikkinen J, Backstrom A, Savolainen M, et al. Effects of estrogen replacement therapy on natriuretic peptides and blood pressure. Maturitas. (2004) 47:201–8. doi: 10.1016/S0378-5122(03)00279-2
199. SOLVD Investigators, Yusuf S, Pitt B, Davis C, Hood W, Cohn J. Effect of enalapril on survival in patients with reduced left ventricular ejection fraction and congestive heart failure. N Engl J Med. (1991) 325:293–302. doi: 10.1056/NEJM199108013250501
200. CONSENSUS Trial Study Group. Effects of enalapril on mortality in severe congestive heart failure. results of the cooperative north scandinavian enalapril survival study (CONSENSUS). N Engl J Med. (1987) 316:1429–35. doi: 10.1056/NEJM198706043162301
201. Magnani B, Magelli C. Captopril in mild heart failure: preliminary observations of a long-term, double-blind, placebo-controlled multicentre trial. Postgrad Med J. (1986) 62(Suppl. 1):153–8.
202. Bussmann W, Storger H, Hadler D, Reifart N, Fassbinder W, Jungmann E, et al. Long-term treatment of severe chronic heart failure with captopril: a double-blind, randomized, placebo-controlled, long-term study. J Cardiovasc Pharmacol. (1987) 9(Suppl. 2):S50–60. doi: 10.1097/00005344-198700002-00012
203. Garg R, Yusuf S. Overview of randomized trials of angiotensin-converting enzyme inhibitors on mortality and morbidity in patients with heart failure. collaborative group on ACE inhibitor trials. JAMA. (1995) 273:1450–6. doi: 10.1001/jama.273.18.1450
204. Packer M, Poole-Wilson P, Armstrong P, Cleland J, Horowitz J, Massie B, et al. Comparative effects of low and high doses of the angiotensin-converting enzyme inhibitor, lisinopril, on morbidity and mortality in chronic heart failure. ATLAS Study Group. Circulation. (1999) 100:2312–8. doi: 10.1161/01.CIR.100.23.2312
205. Shekelle P, Rich M, Morton S, Atkinson C, Tu W, Maglione M, et al. Efficacy of angiotensin-converting enzyme inhibitors and beta-blockers in the management of left ventricular systolic dysfunction according to race, gender, and diabetic status: a meta-analysis of major clinical trials. J Am Coll Cardiol. (2003) 41:1529–38. doi: 10.1016/S0735-1097(03)00262-6
206. Konstam M, Neaton J, Dickstein K, Drexler H, Komajda M, Martinez F, et al. Effects of high-dose versus low-dose losartan on clinical outcomes in patients with heart failure (HEAAL study): a randomised, double-blind trial. Lancet. (2009) 374:1840–8. doi: 10.1016/S0140-6736(09)61913-9
207. Hudson M, Rahme E, Behlouli H, Sheppard R, Pilote L. Sex differences in the effectiveness of angiotensin receptor blockers and angiotensin converting enzyme inhibitors in patients with congestive heart failure–a population study. Eur J Heart Fail. (2007) 9:602–9. doi: 10.1016/j.ejheart.2007.02.001
208. Aimo A, Vergaro G, Barison A, Maffei S, Borrelli C, Morrone D, et al. Sex-related differences in chronic heart failure. Int J Cardiol. (2018) 255:145–51. doi: 10.1016/j.ijcard.2017.10.068
209. Ghali J, Lindenfeld J. Sex differences in response to chronic heart failure therapies. Expert Rev Cardiovasc Ther. (2008) 6:555–65. doi: 10.1586/14779072.6.4.555
210. Packer M, Califf R, Konstam M, Krum H, McMurray J, Rouleau J, et al. Comparison of omapatrilat and enalapril in patients with chronic heart failure: the omapatrilat versus enalapril randomized trial of utility in reducing events (OVERTURE). Circulation. (2002) 106:920–6. doi: 10.1161/01.CIR.0000029801.86489.50
211. McMurray J, Packer M, Desai A, Gong J, Lefkowitz M, Rizkala A, et al. Angiotensin-neprilysin inhibition versus enalapril in heart failure. N Engl J Med. (2014) 371:993–1004. doi: 10.1056/NEJMoa1409077
212. Danielson C, Lileikyte G, Ouwerkerk W, S P Lam C, Erlinge D, Teng TK. Sex differences in efficacy of pharmacological therapies in heart failure with reduced ejection fraction: a meta-analysis. ESC Heart Fail. (2022) 9:2753–61. doi: 10.1002/ehf2.13974
213. Vicent L, Ayesta A, Esteban-Fernandez A, Gomez-Bueno M, De-Juan J, Diez-Villanueva P, et al. Sex influence on the efficacy and safety of Sacubitril/Valsartan. Cardiology. (2019) 142:73–8. doi: 10.1159/000498984
214. Solomon S, McMurray J, Anand I, Ge J, Lam C, Maggioni A, et al. Angiotensin-neprilysin inhibition in heart failure with preserved ejection fraction. N Engl J Med. (2019) 381:1609–20.
215. McMurray J, Solomon S, Inzucchi S, Kober L, Kosiborod M, Martinez F, et al. Dapagliflozin in patients with heart failure and reduced ejection fraction. N Engl J Med. (2019) 381:1995–2008.
216. Zinman B, Wanner C, Lachin J, Fitchett D, Bluhmki E, Hantel S, et al. Empagliflozin, cardiovascular outcomes, and mortality in type 2 diabetes. N Engl J Med. (2015) 373:2117–28. doi: 10.1056/NEJMoa1504720
217. Butt J, Docherty K, Petrie M, Schou M, Kosiborod M, O’Meara E, et al. Efficacy and safety of dapagliflozin in men and women with heart failure with reduced ejection fraction: a prespecified analysis of the dapagliflozin and prevention of adverse outcomes in heart failure trial. JAMA Cardiol. (2021) 6:678–89. doi: 10.1001/jamacardio.2021.0379
218. Packer M. Epicardial adipose tissue may mediate deleterious effects of obesity and inflammation on the myocardium. J Am Coll Cardiol. (2018) 71:2360–72. doi: 10.1016/j.jacc.2018.03.509
219. Lam C, Carson P, Anand I, Rector T, Kuskowski M, Komajda M, et al. Sex differences in clinical characteristics and outcomes in elderly patients with heart failure and preserved ejection fraction: the irbesartan in heart failure with preserved ejection fraction (I-PRESERVE) trial. Circ Heart Fail. (2012) 5:571–8. doi: 10.1161/CIRCHEARTFAILURE.112.970061
220. Packer M, Lam C, Lund L, Maurer M, Borlaug B. Characterization of the inflammatory-metabolic phenotype of heart failure with a preserved ejection fraction: a hypothesis to explain influence of sex on the evolution and potential treatment of the disease. Eur J Heart Fail. (2020) 22:1551–67. doi: 10.1002/ejhf.1902
221. Ferrario C, Jessup J, Smith R. Hemodynamic and hormonal patterns of untreated essential hypertension in men and women. Ther Adv Cardiovasc Dis. (2013) 7:293–305. doi: 10.1177/1753944713513221
222. Kanashiro-Takeuchi R, Heidecker B, Lamirault G, Dharamsi J, Hare J. Sex-specific impact of aldosterone receptor antagonism on ventricular remodeling and gene expression after myocardial infarction. Clin Transl Sci. (2009) 2:134–42. doi: 10.1111/j.1752-8062.2009.00094.x
223. Shah A, Claggett B, Sweitzer N, Shah S, Anand I, Liu L, et al. Prognostic importance of impaired systolic function in heart failure with preserved ejection fraction and the impact of spironolactone. Circulation. (2015) 132:402–14. doi: 10.1161/CIRCULATIONAHA.115.015884
224. Merrill M, Sweitzer N, Lindenfeld J, Kao D. Sex differences in outcomes and responses to spironolactone in heart failure with preserved ejection fraction: a secondary analysis of TOPCAT trial. JACC Heart Fail. (2019) 7:228–38. doi: 10.1016/j.jchf.2019.01.003
225. Digitalis Investigation Group. The effect of digoxin on mortality and morbidity in patients with heart failure. N Engl J Med. (1997) 336:525–33. doi: 10.1056/NEJM199702203360801
226. Rathore S, Wang Y, Krumholz H. Sex-based differences in the effect of digoxin for the treatment of heart failure. N Engl J Med. (2002) 347:1403–11. doi: 10.1056/NEJMoa021266
227. Ahmed A, Aronow W, Fleg J. Predictors of mortality and hospitalization in women with heart failure in the Digitalis Investigation Group trial. Am J Ther. (2006) 13:325–31. doi: 10.1097/00045391-200607000-00009
228. Adams K Jr., Patterson J, Gattis W, O’Connor C, Lee C, Schwartz T, et al. Relationship of serum digoxin concentration to mortality and morbidity in women in the digitalis investigation group trial: a retrospective analysis. J Am Coll Cardiol. (2005) 46:497–504. doi: 10.1016/j.jacc.2005.02.091
229. Al-Khatib S, Stevenson W, Ackerman M, Bryant W, Callans D, Curtis A, et al. 2017 AHA/ACC/HRS guideline for management of patients with ventricular arrhythmias and the prevention of sudden cardiac death: executive summary: a report of the American College of Cardiology/American Heart Association task force on clinical practice guidelines and the heart rhythm society. Heart Rhythm. (2018) 15:e190–252.
230. Alaeddini J, Wood M, Amin M, Ellenbogen K. Gender disparity in the use of cardiac resynchronization therapy in the United States. Pacing Clin Electrophysiol. (2008) 31:468–72. doi: 10.1111/j.1540-8159.2008.01016.x
231. Curtis L, Al-Khatib S, Shea A, Hammill B, Hernandez A, Schulman K. Sex differences in the use of implantable cardioverter-defibrillators for primary and secondary prevention of sudden cardiac death. JAMA. (2007) 298:1517–24. doi: 10.1001/jama.298.13.1517
232. Santangeli P, Pelargonio G, Dello Russo A, Casella M, Bisceglia C, Bartoletti S, et al. Gender differences in clinical outcome and primary prevention defibrillator benefit in patients with severe left ventricular dysfunction: a systematic review and meta-analysis. Heart Rhythm. (2010) 7:876–82. doi: 10.1016/j.hrthm.2010.03.042
233. Hsich E. Sex differences in advanced heart failure therapies. Circulation. (2019) 139:1080–93. doi: 10.1161/CIRCULATIONAHA.118.037369
234. Russo A, Daugherty S, Masoudi F, Wang Y, Curtis J, Lampert R. Gender and outcomes after primary prevention implantable cardioverter-defibrillator implantation: findings from the national cardiovascular data registry (NCDR). Am Heart J. (2015) 170:330–8. doi: 10.1016/j.ahj.2015.02.025
235. Saxena S, Goldenberg I, McNitt S, Hsich E, Kutyifa V, Bragazzi N, et al. Sex differences in the risk of first and recurrent ventricular tachyarrhythmias among patients receiving an implantable cardioverter-defibrillator for primary prevention. JAMA Netw Open. (2022) 5:e2217153. doi: 10.1001/jamanetworkopen.2022.17153
236. Zusterzeel R, Curtis J, Canos D, Sanders W, Selzman K, Pina I, et al. Sex-specific mortality risk by QRS morphology and duration in patients receiving CRT: results from the NCDR. J Am Coll Cardiol. (2014) 64:887–94. doi: 10.1016/j.jacc.2014.06.1162
237. Zusterzeel R, Selzman K, Sanders W, Canos D, O’Callaghan K, Carpenter J, et al. Cardiac resynchronization therapy in women: US food and drug administration meta-analysis of patient-level data. JAMA Intern Med. (2014) 174:1340–8. doi: 10.1001/jamainternmed.2014.2717
238. Costanzo M. Cardiac resynchronization therapy in women. Heart Fail Clin. (2017) 13:165–78. doi: 10.1016/j.hfc.2016.07.013
239. Quesada A, Arteaga F, Romero-Villafranca R, Perez-Alvarez L, Martinez-Ferrer J, Alzueta-Rodriguez J, et al. Sex-specific ventricular arrhythmias and mortality in cardiac resynchronization therapy recipients. JACC Clin Electrophysiol. (2021) 7:705–15. doi: 10.1016/j.jacep.2020.10.009
240. Schuchert A, Muto C, Maounis T, Frank R, Ella R, Polauck A, et al. Gender-related safety and efficacy of cardiac resynchronization therapy. Clin Cardiol. (2013) 36:683–90. doi: 10.1002/clc.22203
241. Topkara V, Dang N, Barili F, Martens T, George I, Cheema F, et al. Ventricular assist device use for the treatment of acute viral myocarditis. J Thorac Cardiovasc Surg. (2006) 131:1190–1. doi: 10.1016/j.jtcvs.2005.08.073
242. Acharya D, Loyaga-Rendon R, Morgan C, Sands K, Pamboukian S, Rajapreyar I, et al. INTERMACS analysis of stroke during support with continuous-flow left ventricular assist devices: risk factors and outcomes. JACC Heart Fail. (2017) 5:703–11. doi: 10.1016/j.jchf.2017.06.014
243. Joshi A, Lerman J, Sajja A, Dahiya G, Gokhale A, Dey A, et al. Sex-based differences in left ventricular assist device utilization: insights from the nationwide inpatient sample 2004 to 2016. Circ Heart Fail. (2019) 12:e006082. doi: 10.1161/CIRCHEARTFAILURE.119.006082
244. Bogaev R, Pamboukian S, Moore S, Chen L, John R, Boyle A, et al. Comparison of outcomes in women versus men using a continuous-flow left ventricular assist device as a bridge to transplantation. J Heart Lung Transplant. (2011) 30:515–22. doi: 10.1016/j.healun.2010.12.009
245. DeFilippis E, Truby L, Garan A, Givens R, Takeda K, Takayama H, et al. Sex-related differences in use and outcomes of left ventricular assist devices as bridge to transplantation. JACC Heart Fail. (2019) 7:250–7. doi: 10.1016/j.jchf.2019.01.008
246. Maukel L, Weidner G, Beyersmann J, Spaderna H. Sex differences in recovery and device replacement after left ventricular assist device implantation as destination therapy. J Am Heart Assoc. (2022) 11:e023294. doi: 10.1161/JAHA.121.023294
247. Mehra M, Naka Y, Uriel N, Goldstein D, Cleveland J Jr., Colombo P, et al. A fully magnetically levitated circulatory pump for advanced heart failure. N Engl J Med. (2017) 376:440–50. doi: 10.1056/NEJMoa1610426
248. Reza N, Edwards J, Katcoff H, Mondal A, Griffis H, Rossano J, et al. Sex differences in left ventricular assist device-related emergency department encounters in the United States. J Card Fail. (2022) 28:1445–55. doi: 10.1016/j.cardfail.2022.05.005
249. Herr J, Ravichandran A, Sheikh F, Lala A, Chien C, Hsiao S, et al. Practices of referring patients to advanced heart failure centers. J Card Fail. (2021) 27:1251–9. doi: 10.1016/j.cardfail.2021.05.024
250. Stehlik J, Edwards L, Kucheryavaya A, Benden C, Christie J, Dobbels F, et al. The registry of the international society for heart and lung transplantation: twenty-eighth adult heart transplant report–2011. J Heart Lung Transplant. (2011) 30:1078–94. doi: 10.1016/j.healun.2011.08.003
251. Regitz-Zagrosek V, Petrov G, Lehmkuhl E, Smits J, Babitsch B, Brunhuber C, et al. Heart transplantation in women with dilated cardiomyopathy. Transplantation. (2010) 89:236–44. doi: 10.1097/TP.0b013e3181c35255
252. Varshney A, Berg D, Katz J, Baird-Zars V, Bohula E, Carnicelli A, et al. Use of temporary mechanical circulatory support for management of cardiogenic shock before and after the united network for organ sharing donor heart allocation system changes. JAMA Cardiol. (2020) 5:703–8. doi: 10.1001/jamacardio.2020.0692
253. Johnson J, Greaves L, Repta R. Better science with sex and gender: facilitating the use of a sex and gender-based analysis in health research. Int J Equity Health. (2009) 8:14. doi: 10.1186/1475-9276-8-14
254. Shah Z, Mohammed M, Vuddanda V, Ansari M, Masoomi R, Gupta K. National trends, gender, management, and outcomes of patients hospitalized for myocarditis. Am J Cardiol. (2019) 124:131–6. doi: 10.1016/j.amjcard.2019.03.036
255. Hassan K, Kyriakakis C, Doubell A, Van Zyl G, Claassen M, Zaharie D, et al. Prevalence of cardiotropic viruses in adults with clinically suspected myocarditis in South Africa. Open Heart (2022) 9:e001942. doi: 10.1136/openhrt-2021-001942
256. van Rijsingen I, Nannenberg E, Arbustini E, Elliott P, Mogensen J, Hermans-van Ast J, et al. Gender-specific differences in major cardiac events and mortality in lamin A/C mutation carriers. Eur J Heart Fail. (2013) 15:376–84. doi: 10.1093/eurjhf/hfs191
257. Doesch C, Dierks D, Haghi D, Schimpf R, Kuschyk J, Suselbeck T, et al. Right ventricular dysfunction, late gadolinium enhancement, and female gender predict poor outcome in patients with dilated cardiomyopathy. Int J Cardiol. (2014) 177:429–35. doi: 10.1016/j.ijcard.2014.09.004
258. Li X, Cai C, Luo R, Jiang R, Zeng J, Tang Y, et al. The usefulness of age and sex to predict all-cause mortality in patients with dilated cardiomyopathy: a single-center cohort study. Clin Interv Aging. (2015) 10:1479–86. doi: 10.2147/CIA.S88565
259. Halliday B, Gulati A, Ali A, Newsome S, Lota A, Tayal U, et al. Sex- and age-based differences in the natural history and outcome of dilated cardiomyopathy. Eur J Heart Fail. (2018) 20:1392–400. doi: 10.1002/ejhf.1216
260. Thapa R, Kanchan CK, Khatri R, Khatri D, Deo RK, Shah D. An echocardiographic evaluation of dilated cardiomyopathy in a tertiary care hospital. JNMA J Nepal Med Assoc. (2019) 57:33–6. doi: 10.31729/jnma.3992
261. Lennermann D, Backs J, van den Hoogenhof M. New insights in RBM20 cardiomyopathy. Curr Heart Fail Rep. (2020) 17:234–46. doi: 10.1007/s11897-020-00475-x
262. Sack M, Rader D, Cannon R III. Oestrogen and inhibition of oxidation of low-density lipoproteins in postmenopausal women. Lancet. (1994) 343:269–70. doi: 10.1016/S0140-6736(94)91117-7
263. Wang J, Irwin R, Brinton R. Activation of estrogen receptor alpha increases and estrogen receptor beta decreases apolipoprotein E expression in hippocampus in vitro and in vivo. Proc Natl Acad Sci USA. (2006) 103:16983–8. doi: 10.1073/pnas.0608128103
264. Lamkanfi M, Dixit V. Mechanisms and functions of inflammasomes. Cell. (2014) 157:1013–22. doi: 10.1016/j.cell.2014.04.007
265. Trexler C, Odell A, Jeong M, Dowell R, Leinwand L. Transcriptome and functional profile of cardiac myocytes is influenced by biological sex. Circ Cardiovasc Genet. (2017) 10:e001770. doi: 10.1161/CIRCGENETICS.117.001770
266. Huang H, He J, Yuan Y, Aoyagi E, Takenaka H, Itagaki T, et al. Opposing effects of estradiol and progesterone on the oxidative stress-induced production of chemokine and proinflammatory cytokines in murine peritoneal macrophages. J Med Invest. (2008) 55:133–41. doi: 10.2152/jmi.55.133
267. Sobolewska A, Gajewska M, Zarzynska J, Gajkowska B, Motyl T. IGF-I, EGF, and sex steroids regulate autophagy in bovine mammary epithelial cells via the mTOR pathway. Eur J Cell Biol. (2009) 88:117–30. doi: 10.1016/j.ejcb.2008.09.004
268. Coto-Montes A, Tomas-Zapico C, Martinez-Fraga J, Vega-Naredo I, Sierra V, Caballero B, et al. Sexual autophagic differences in the androgen-dependent flank organ of Syrian hamsters. J Androl. (2009) 30:113–21. doi: 10.2164/jandrol.108.005355
269. Yan H, Yang W, Zhou F, Pan Q, Allred K, Allred C, et al. Estrogen protects cardiac function and energy metabolism in dilated cardiomyopathy induced by loss of cardiac IRS1 and IRS2. Circ Heart Fail. (2022) 15:e008758. doi: 10.1161/CIRCHEARTFAILURE.121.008758
270. Wittnich C, Tan L, Wallen J, Belanger M. Sex differences in myocardial metabolism and cardiac function: an emerging concept. Pflugers Arch. (2013) 465:719–29. doi: 10.1007/s00424-013-1232-1
271. Tsuji M, Kawasaki T, Matsuda T, Arai T, Gojo S, Takeuchi J. Sexual dimorphisms of mRNA and miRNA in human/murine heart disease. PLoS One. (2017) 12:e0177988. doi: 10.1371/journal.pone.0177988
272. Mirna M, Schmutzler L, Topf A, Hoppe U, Lichtenauer M. Biological sex and its impact on clinical characteristics in patients presenting with myocarditis. Med Princ Pract. (2022) 31:74–82. doi: 10.1159/000520870
273. Mendelsohn M, Karas R. Molecular and cellular basis of cardiovascular gender differences. Science. (2005) 308:1583–7. doi: 10.1126/science.1112062
274. Isensee J, Ruiz Noppinger P. Sexually dimorphic gene expression in mammalian somatic tissue. Gend Med. (2007) 4(Suppl. B):S75–95. doi: 10.1016/S1550-8579(07)80049-0
275. Legato M. Gender and the heart: sex-specific differences in normal anatomy and physiology. J Gend Specif Med. (2000) 3:15–8.
276. Deslypere J, Vermeulen A. Influence of age on steroid concentrations in skin and striated muscle in women and in cardiac muscle and lung tissue in men. J Clin Endocrinol Metab. (1985) 61:648–53. doi: 10.1210/jcem-61-4-648
277. Melchert R, Kennedy R, Acosta D Jr. Cardiovascular effects of steroidal agents. In: D Acosta Jr. editor. Cardiovascular Toxicology. London: Taylor & Francis (2001). p. 425–75.
278. Scheuer J, Malhotra A, Schaible T, Capasso J. Effects of gonadectomy and hormonal replacement on rat hearts. Circ Res. (1987) 61:12–9. doi: 10.1161/01.RES.61.1.12
279. Mallat Z, Fornes P, Costagliola R, Esposito B, Belmin J, Lecomte D, et al. Age and gender effects on cardiomyocyte apoptosis in the normal human heart. J Gerontol A Biol Sci Med Sci. (2001) 56:M719–23. doi: 10.1093/gerona/56.11.M719
280. Sheppard R, Bedi M, Kubota T, Semigran M, Dec W, Holubkov R, et al. Myocardial expression of fas and recovery of left ventricular function in patients with recent-onset cardiomyopathy. J Am Coll Cardiol. (2005) 46:1036–42. doi: 10.1016/j.jacc.2005.05.067
281. Straub R. The complex role of estrogens in inflammation. Endocr Rev. (2007) 28:521–74. doi: 10.1210/er.2007-0001
282. Rubtsov A, Rubtsova K, Kappler J, Marrack P. Genetic and hormonal factors in female-biased autoimmunity. Autoimmun Rev. (2010) 9:494–8. doi: 10.1016/j.autrev.2010.02.008
283. Lahita R. Sex hormones and immune function. In: M Legato editor. Principles of Gender-Specific Medicine. Burlington, MA: Elsevier (2016). p. 15–26.
284. James A, Choisy S, Hancox J. Recent advances in understanding sex differences in cardiac repolarization. Prog Biophys Mol Biol. (2007) 94:265–319. doi: 10.1016/j.pbiomolbio.2005.05.010
285. McCrohon J, Death A, Nakhla S, Jessup W, Handelsman D, Stanley K, et al. Androgen receptor expression is greater in macrophages from male than from female donors. a sex difference with implications for atherogenesis. Circulation. (2000) 101:224–6. doi: 10.1161/01.CIR.101.3.224
286. Sader M, McGrath K, Hill M, Bradstock K, Jimenez M, Handelsman D, et al. Androgen receptor gene expression in leucocytes is hormonally regulated: implications for gender differences in disease pathogenesis. Clin Endocrinol (Oxf). (2005) 62:56–63. doi: 10.1111/j.1365-2265.2004.02173.x
287. Brechenmacher S, Bruns C, Van den Engel N, Angele P, Loehe F, Jauch K, et al. Influence of surgical trauma on the mRNA expression of sex hormone receptors in PBMCs in male and female patients. Langenbecks Arch Surg. (2008) 393:871–6. doi: 10.1007/s00423-008-0304-8
288. Fairweather D. Sex differences in inflammation during atherosclerosis. Clin Med Insights Cardiol. (2014) 8(Suppl. 3):49–59. doi: 10.4137/CMC.S17068
289. Su N, Yue Y, Xiong S. Monocytic myeloid-derived suppressor cells from females, but not males, alleviate CVB3-induced myocarditis by increasing regulatory and CD4(+)IL-10(+) T cells. Sci Rep. (2016) 6:22658. doi: 10.1038/srep22658
290. Huber S. Coxsackievirus B3-induced myocarditis: infection of females during the estrus phase of the ovarian cycle leads to activation of T regulatory cells. Virology. (2008) 378:292–8. doi: 10.1016/j.virol.2008.05.015
291. Huber S, Kupperman J, Newell M. Estradiol prevents and testosterone promotes Fas-dependent apoptosis in CD4+ Th2 cells by altering Bcl 2 expression. Lupus. (1999) 8:384–7. doi: 10.1177/096120339900800511
292. Rettew J, Huet Y, Marriott I. Estrogens augment cell surface TLR4 expression on murine macrophages and regulate sepsis susceptibility in vivo. Endocrinology. (2009) 150:3877–84. doi: 10.1210/en.2009-0098
293. Deshpande R, Khalili H, Pergolizzi R, Michael S, Chang M. Estradiol down-regulates LPS-induced cytokine production and NFkB activation in murine macrophages. Am J Reprod Immunol. (1997) 38:46–54. doi: 10.1111/j.1600-0897.1997.tb00275.x
294. Evans M, Eckert A, Lai K, Adelman S, Harnish D. Reciprocal antagonism between estrogen receptor and NF-kappaB activity in vivo. Circ Res. (2001) 89:823–30. doi: 10.1161/hh2101.098543
295. Chadwick C, Chippari S, Matelan E, Borges-Marcucci L, Eckert A, Keith J Jr., et al. Identification of pathway-selective estrogen receptor ligands that inhibit NF-kappaB transcriptional activity. Proc Natl Acad Sci USA. (2005) 102:2543–8. doi: 10.1073/pnas.0405841102
296. Demyanets S, Pfaffenberger S, Kaun C, Rega G, Speidl W, Kastl S, et al. The estrogen metabolite 17beta-dihydroequilenin counteracts interleukin-1alpha induced expression of inflammatory mediators in human endothelial cells in vitro via NF-kappaB pathway. Thromb Haemost. (2006) 95:107–16. doi: 10.1160/TH05-05-0333
297. Villablanca A, Jayachandran M, Banka C. Atherosclerosis and sex hormones: current concepts. Clin Sci (Lond). (2010) 119:493–513. doi: 10.1042/CS20100248
298. Rodriguez-Menocal L, Faridi M, Martinez L, Shehadeh L, Duque J, Wei Y, et al. Macrophage-derived IL-18 and increased fibrinogen deposition are age-related inflammatory signatures of vascular remodeling. Am J Physiol Heart Circ Physiol. (2014) 306:H641–53. doi: 10.1152/ajpheart.00641.2013
299. Kalogeropoulos A, Georgiopoulou V, Psaty B, Rodondi N, Smith A, Harrison D, et al. Inflammatory markers and incident heart failure risk in older adults: the health ABC (health, aging, and body composition) study. J Am Coll Cardiol. (2010) 55:2129–37. doi: 10.1016/j.jacc.2009.12.045
300. Medenwald D, Kors J, Loppnow H, Thiery J, Kluttig A, Nuding S, et al. Inflammation and prolonged QT time: results from the cardiovascular disease, living and ageing in halle (CARLA) study. PLoS One. (2014) 9:e95994. doi: 10.1371/journal.pone.0095994
301. Okwan-Duodu D, Umpierrez G, Brawley O, Diaz R. Obesity-driven inflammation and cancer risk: role of myeloid derived suppressor cells and alternately activated macrophages. Am J Cancer Res. (2013) 3:21–33.
302. Li K, Xu W, Guo Q, Jiang Z, Wang P, Yue Y, et al. Differential macrophage polarization in male and female BALB/c mice infected with coxsackievirus B3 defines susceptibility to viral myocarditis. Circ Res. (2009) 105:353–64. doi: 10.1161/CIRCRESAHA.109.195230
303. Lutz M, Milenkovic I, Regelsberger G, Kovacs G. Distinct patterns of sirtuin expression during progression of Alzheimer’s disease. Neuromolecular Med. (2014) 16:405–14. doi: 10.1007/s12017-014-8288-8
304. Cook I. Sexual dimorphism of humoral immunity with human vaccines. Vaccine. (2008) 26:3551–5. doi: 10.1016/j.vaccine.2008.04.054
305. Klein S, Pekosz A. Sex-based biology and the rational design of influenza vaccination strategies. J Infect Dis. (2014) 209(Suppl. 3):S114–9. doi: 10.1093/infdis/jiu066
306. Zhou N, Yue Y, Xiong S. Sex hormone contributes to sexually dimorphic susceptibility in CVB3-Induced viral myocarditis via modulating IFN-gamma(+) NK cell production. Can J Cardiol. (2018) 34:492–501. doi: 10.1016/j.cjca.2018.01.002
307. Haghmorad D, Salehipour Z, Nosratabadi R, Rastin M, Kokhaei P, Mahmoudi M, et al. Medium-dose estrogen ameliorates experimental autoimmune encephalomyelitis in ovariectomized mice. J Immunotoxicol. (2016) 13:885–96. doi: 10.1080/1547691X.2016.1223768
308. Lengi A, Phillips R, Karpuzoglu E, Ahmed S. 17beta-estradiol downregulates interferon regulatory factor-1 in murine splenocytes. J Mol Endocrinol. (2006) 37:421–32. doi: 10.1677/jme.1.02122
309. Nakaya M, Yamasaki M, Miyazaki Y, Tachibana H, Yamada K. Estrogenic compounds suppressed interferon-gamma production in mouse splenocytes through direct cell-cell interaction. In Vitro Cell Dev Biol Anim. (2003) 39:383–7. doi: 10.1290/1543-706X(2003)039<0383:ECSIPI>2.0.CO;2
310. Barcena M, Pozdniakova S, Haritonow N, Breiter P, Kuhl A, Milting H, et al. Dilated cardiomyopathy impairs mitochondrial biogenesis and promotes inflammation in an age- and sex-dependent manner. Aging (Albany NY). (2020) 12:24117–33. doi: 10.18632/aging.202283
311. Hodgin J, Krege J, Reddick R, Korach K, Smithies O, Maeda N. Estrogen receptor alpha is a major mediator of 17beta-estradiol’s atheroprotective effects on lesion size in Apoe-/- mice. J Clin Invest. (2001) 107:333–40. doi: 10.1172/JCI11320
312. Mahmoodzadeh S, Leber J, Zhang X, Jaisser F, Messaoudi S, Morano I, et al. Cardiomyocyte-specific estrogen receptor alpha increases angiogenesis, lymphangiogenesis and reduces fibrosis in the female mouse heart post-myocardial infarction. J Cell Sci Ther. (2014) 5:153. doi: 10.4172/2157-7013.1000153
313. Barcena M, Niehues M, Christiansen C, Estepa M, Haritonow N, Sadighi A, et al. Male macrophages and fibroblasts from C57/BL6J mice are more susceptible to inflammatory stimuli. Front Immunol. (2021) 12:758767. doi: 10.3389/fimmu.2021.758767
314. Li S, Gupte A. The role of estrogen in cardiac metabolism and diastolic function. Methodist Debakey Cardiovasc J. (2017) 13:4–8. doi: 10.14797/mdcj-13-1-4
315. Liu L, Yue Y, Xiong S. NK-derived IFN-gamma/IL-4 triggers the sexually disparate polarization of macrophages in CVB3-induced myocarditis. J Mol Cell Cardiol. (2014) 76:15–25. doi: 10.1016/j.yjmcc.2014.07.021
316. Giron-Gonzalez J, Moral F, Elvira J, Garcia-Gil D, Guerrero F, Gavilan I, et al. Consistent production of a higher TH1:TH2 cytokine ratio by stimulated T cells in men compared with women. Eur J Endocrinol. (2000) 143:31–6. doi: 10.1530/eje.0.1430031
317. Giltay E, Fonk J, von Blomberg B, Drexhage H, Schalkwijk C, Gooren L. In vivo effects of sex steroids on lymphocyte responsiveness and immunoglobulin levels in humans. J Clin Endocrinol Metab. (2000) 85:1648–57. doi: 10.1210/jcem.85.4.6562
318. Loria R. Immune up-regulation and tumor apoptosis by androstene steroids. Steroids. (2002) 67:953–66. doi: 10.1016/S0039-128X(02)00043-0
319. Desai K, Michalowska A, Kondaiah P, Ward J, Shih J, Green J. Gene expression profiling identifies a unique androgen-mediated inflammatory/immune signature and a PTEN (phosphatase and tensin homolog deleted on chromosome 10)-mediated apoptotic response specific to the rat ventral prostate. Mol Endocrinol. (2004) 18:2895–907. doi: 10.1210/me.2004-0033
320. Cavasin M, Tao Z, Yu A, Yang X. Testosterone enhances early cardiac remodeling after myocardial infarction, causing rupture and degrading cardiac function. Am J Physiol Heart Circ Physiol. (2006) 290:H2043–50. doi: 10.1152/ajpheart.01121.2005
321. Cocker M, Abdel-Aty H, Strohm O, Friedrich M. Age and gender effects on the extent of myocardial involvement in acute myocarditis: a cardiovascular magnetic resonance study. Heart. (2009) 95:1925–30. doi: 10.1136/hrt.2008.164061
322. Haddad G, Saunders L, Crosby S, Carles M, del Monte F, King K, et al. Human cardiac-specific cDNA array for idiopathic dilated cardiomyopathy: sex-related differences. Physiol Genomics. (2008) 33:267–77. doi: 10.1152/physiolgenomics.00265.2007
323. Yeung F, Hoberg J, Ramsey C, Keller M, Jones D, Frye R, et al. Modulation of NF-kappaB-dependent transcription and cell survival by the SIRT1 deacetylase. EMBO J. (2004) 23:2369–80. doi: 10.1038/sj.emboj.7600244
Keywords: sex differences, gender differences, genetics, epidemiology, pathogenesis, devices, therapy
Citation: Fairweather D, Beetler DJ, Musigk N, Heidecker B, Lyle MA, Cooper LT Jr and Bruno KA (2023) Sex and gender differences in myocarditis and dilated cardiomyopathy: An update. Front. Cardiovasc. Med. 10:1129348. doi: 10.3389/fcvm.2023.1129348
Received: 21 December 2022; Accepted: 06 February 2023;
Published: 02 March 2023.
Edited by:
Sally A. Huber, University of Vermont, United StatesReviewed by:
Jay Reddy, University of Nebraska-Lincoln, United StatesCopyright © 2023 Fairweather, Beetler, Musigk, Heidecker, Lyle, Cooper and Bruno. This is an open-access article distributed under the terms of the Creative Commons Attribution License (CC BY). The use, distribution or reproduction in other forums is permitted, provided the original author(s) and the copyright owner(s) are credited and that the original publication in this journal is cited, in accordance with accepted academic practice. No use, distribution or reproduction is permitted which does not comply with these terms.
*Correspondence: DeLisa Fairweather, RmFpcndlYXRoZXIuRGVMaXNhQG1heW8uZWR1
†These authors share first authorship
Disclaimer: All claims expressed in this article are solely those of the authors and do not necessarily represent those of their affiliated organizations, or those of the publisher, the editors and the reviewers. Any product that may be evaluated in this article or claim that may be made by its manufacturer is not guaranteed or endorsed by the publisher.
Research integrity at Frontiers
Learn more about the work of our research integrity team to safeguard the quality of each article we publish.