- 1Department of Clinical Internal, Anaesthesiologic and Cardiovascular Sciences, Sapienza University of Rome, Rome, Italy
- 2Mediterranea Cardiocentro-Napoli, Naples, Italy
Editorial on the Research Topic
Gut permeability-related endotoxemia and cardiovascular disease: A new clinical challenge
Introduction
Emerging evidence indicates that gut dysbiosis is a risk factor for cardiovascular disease (CVD) in type 1 diabetes mellitus (1), obesity (2) or hypertension (3). Gut dysbiosis is characterized by an overgrowth of gut bacteria that ultimately leads to enhanced intestinal barrier permeability with bacteria or bacteria products such as lipopolysaccharide (LPS) translocation into the systemic circulation (4). Other triggers of dysbiosis-related endotoxemia include aging, and alcohol abuse (4); also, several conditions such as non-septic pneumonia (5–7), acute phase of myocardial infarction, experimental models of intestinal anoxia (8, 9) and systemic inflammation-associated overproduction of pro-inflammatory cytokines, such as interferon-γ and tumour necrosis factor alpha (7), increase gut permeability and ensuing low-grade endotoxaemia. LPS is the main component of the outer leaflet of gut Gram-negative bacteria and is composed of glycolipid containing carbohydrates and a lipid A portion (4). Low, non-pathologic levels of LPS may be detected in human circulation; LPS values >20 pg/ml but 2-fold lower compared to septic patients, have been recently indicated as reflecting low-grade endotoxemia (10). LPS levels may also change physiologically after food intake as LPS is embedded in freshly synthesized chylomicrons and, thereby, reaches the systemic circulation after crossing the intestinal barrier (11).
The human body encompasses local and systemic mechanisms to counteract LPS translocation or to detoxify it. Local defence mechanisms include the intestinal mucus that separates gut microbiota from the intestinal barrier consisting of epithelial cells, that are held together by adhesion proteins such as tight junction (TJ) proteins, adherence junctions proteins (cadherins and catenins), gap junction (connexin) proteins and desmosomes (desmoglein and desmocollins). Just below the epithelial barrier, the gut vascular barrier is also implicated in modulating bacteria translocation into the portal vein (12). Two sequential major LPS detoxification processes occur at the level of intestinal or liver cells in the case of damage to the intestinal barrier: (1) production and secretion, by intestinal epithelial cells, of the High Density Lipoprotein (HDL) 3 that exerts an anti-inflammatory activity by binding LPS-binding protein (LBP) so failing LPS recognition by its receptor TLR4 at the level of liver macrophages; (2) synthesis of intestinal alkaline phosphatase, which removes the lipid A phosphates of LPS giving formation of monophosphoryl-LPS, that, differently from the entire molecule, acts as TLR4 antagonist and (3) the liver acyloxyacylhydrolase, which deacylated critical fatty acid residues for LPS recognition (4).
There are several methodology to measure gut permeability in human, the simplest ones relate to serum measurement of molecules that provide indirect information such analysis of zonulin or D-lactate. Zonulin is a paracrine protein, with a molecular mass of 47-kDa, released by several cell lines, including intestinal cells, after exposure to gliadin-specific peptides or bacteria (13). Activation of the zonulin pathway induces, by protein kinase C-mediated cytoskeleton reorganisation, the disengagement of the protein ZO-1 from the tight junctional complex followed by an increase in intestinal permeability (14). Studies on type 2 diabetes, obesity (15, 16) or acute or chronic CVD (17) patients revealed an increase in serum levels of zonulin coincidentally with elevated levels of LPS. Analysis of serum D-lactate is another alternative because, if increased, it may reflect an efflux of bacteria or their products into circulation consequent to mucosal injury or experimentally-induced gut injury (18, 19). However, a better approach for analysing gut barrier dysfunction is the direct analysis of urinary excretion of dextrose and mannitol after oral ingestion of the mixture (20); however, such an analysis is cumbersome and requires specific expertise (21). Change of gut barrier functionality with enhanced intestinal permeability is conditio sine qua non to observe increased LPS translocation into the systemic circulation with ensuing endotoxemia (Figure 1). The close relationship between gut dysbiosis-related gut permeability and endotoxemia is supported by an interventional study in animals treated with a large spectrum of antibiotics showing reduced systemic levels of LPS coincidentally with the improvement of gut barrier dysfunctionality (22). Analysis of gut dysfunctionality has been assessed in different clinical settings including patients at risk or with coronary heart disease (CHD), in whom LPS has been shown to localize within the atherosclerotic plaque in close association with activated macrophages and in coronary thrombi suggesting that LPS possess pro-atherosclerotic and prothrombotic properties (4). Accordingly, prospective studies documented that LPS blood levels are predictive of atherosclerotic plaque progression and cardiovascular events such as myocardial infarction and stroke (23). Based on these findings, gut dysbiosis-related intestinal barrier permeability with LPS translocation into systemic circulation may represent a novel mechanism favouring atherosclerotic burden and thrombosis in the arterial circulation (Figure 1).
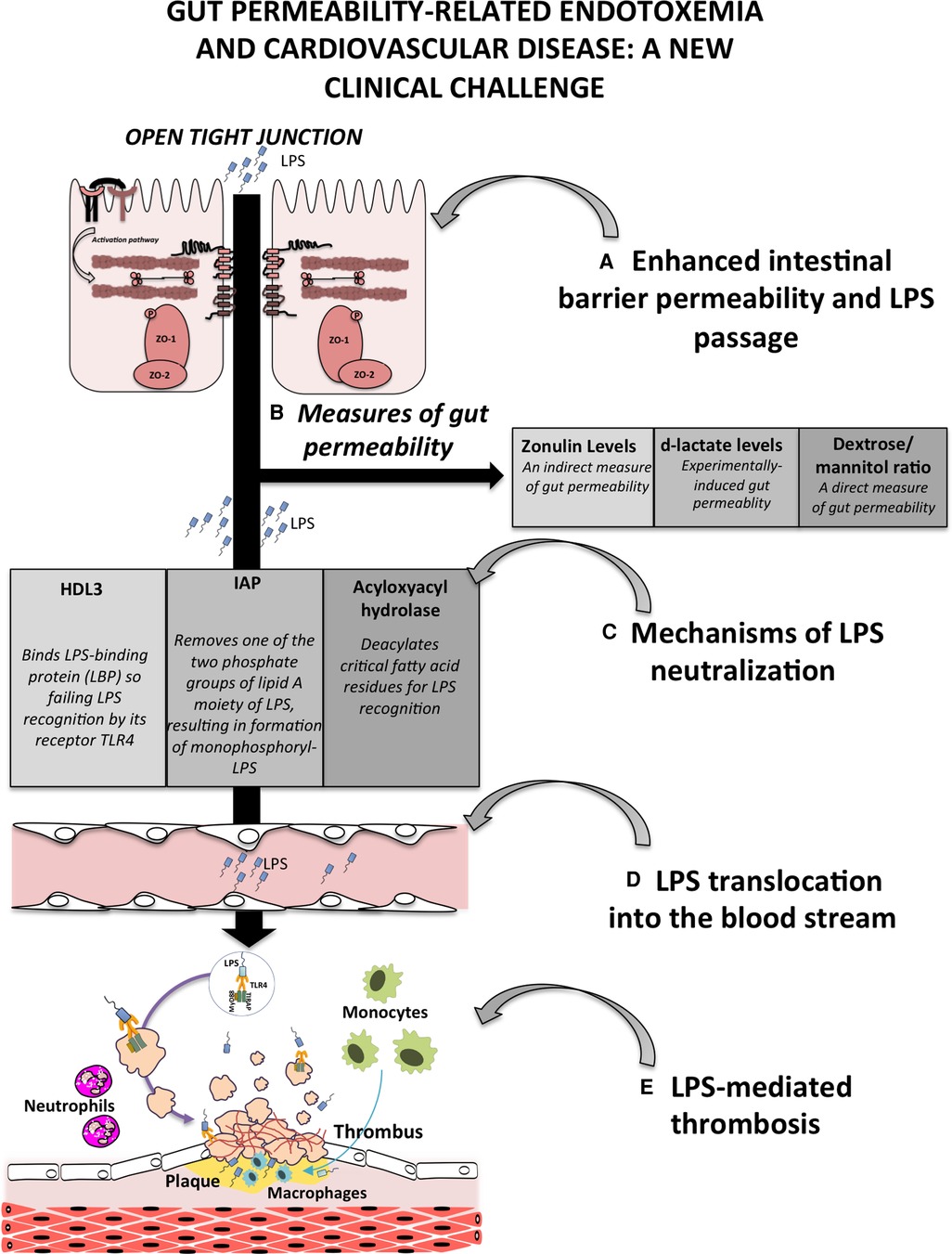
Figure 1. Schematic representation of gut permeability-related endotoxemia and cardiovascular disease. (A) Enhanced intestinal barrier permeability is a consequence of gut dysbiosis that, by the down-regulation of intestinal adhesive proteins, leads to the translocation of bacteria or bacteria products, such as lipopolysaccharide (LPS), into the systemic circulation; (B) gut permeability can be measured by several approaches including serum levels of zonulin, the analysis of d-lactate levels in the blood and the measurement of urinary excretion of dextrose and mannitol; (C) at the level of intestinal or liver cells, several processes occur to detoxify LPS such the production and secretion of the lipoprotein HDL3, the activity of the intestinal alkaline phosphatase (IAP), which dephosphorylates LPS, and the liver acyloxyacylhydrolase, which deacylated critical fatty acid residues for LPS recognition; (D) failure of mechanisms to detoxify LPS favours its translocation into the bloodstream, and finally (E) the atherothrombosis mediated by several cells including platelets, endothelial cells, monocytes, macrophages and neutrophils.
Articles published in this Research Topic reported interesting findings and helped with our further knowledge on this topic. Thus, Long et al. analyzed the current state of gut microbiota scientific research and provided a useful perspective in the field of CHD by bibliometric and visualization software. The authors categorized studies into three key current research hotspots including (1) the mechanism by which gut microbiota metabolites, such as trimethylamine N-oxide (TMAO), short-chain fatty acids (SCFA) or LPS, influence the progression of CHD, (2) microbiota-targeted therapy in CHD management and (3) dietary modification as a possible tool to decelerate the progression of CHD. Moreover, they predict 2 research themes that will play a significant role in the future including the research about novel intervention strategies with natural compounds to modulate gut microbiota and its metabolites in patients with CHD and the study of specific mechanisms of action of gut microbiota affecting CHD.
In the review by Sun et al., the authors discussed the role of various statins affecting the gut microbiota and LDL-C metabolism through the gut-liver axis. After oral administration, statins enter the intestine via the upper gastrointestinal tract and affect the abundance of gut microbiota, which in turn modulates microbiota-derived metabolites such as SCFAs, secondary bile acids (SBAs), TMA, and LPS. These metabolites, transported to the liver through the portal venous system, can regulate cholesterol metabolism and LDL-C levels by several signaling pathways in hepatocytes. Even if preclinical studies have shown the positive effect of statins regulating LDL-C by gut-liver axis, limited studies are reported for humans.
Inflammation promotes every stage of atherosclerosis, from initiation to progression and evolution. Vreeken et al. described the role of endothelial PLXNA4, a protein that results downregulated in inflammatory conditions. The authors demonstrated that inflammatory stimuli reduce endothelial PLXNA4 expression resulting in rearranged cytoskeletal structures and cell-cell junctions. These structural changes increased vascular permeability to inflammatory cells, such as monocytes, into the arterial wall favouring atherosclerosis.
Finally, Zhang et al. aimed to verify the association between the serum levels of alkaline phosphatase (ALP) and stroke in a cohort of adults with hypertension. They found that hypertensive adults with higher serum ALP had a significantly higher risk of first total stroke.
Author contributions
FV: writing—review, and supervision. CN: writing and editing. All authors contributed to the article and approved the submitted version.
Conflict of interest
The authors declare that the research was conducted in the absence of any commercial or financial relationships that could be construed as a potential conflict of interest.
Publisher's note
All claims expressed in this article are solely those of the authors and do not necessarily represent those of their affiliated organizations, or those of the publisher, the editors and the reviewers. Any product that may be evaluated in this article, or claim that may be made by its manufacturer, is not guaranteed or endorsed by the publisher.
References
1. Ghosh SS, Wang J, Yannie PJ, Cooper RC, Sandhu YK, Kakiyama G, et al. Over-expression of intestinal alkaline phosphatase attenuates atherosclerosis. Circ Res. (2021) 128:1646–59. doi: 10.1161/CIRCRESAHA.120.317144
2. Han YH, Onufer EJ, Huang LH, Sprung RW, Davidson WS, Czepielewski RS, et al. Enterically derived high-density lipoprotein restrains liver injury through the portal vein. Science. (2021) 373:1–36. doi: 10.1126/science.abe6729
3. Ascher S, Reinhardt C. The gut microbiota: an emerging risk factor for cardiovascular and cerebrovascular disease. Eur J Immunol. (2018) 48:564–75. doi: 10.1002/eji.201646879
4. Violi F, Cammisotto V, Bartimoccia S, Pignatelli P, Carnevale R, Nocella C. Gut-derived low-grade endotoxaemia, atherothrombosis and cardiovascular disease. Nat Rev Cardiol. (2022) 20:24–37. doi: 10.1038/s41569-022-00737-2
5. Oliva A, Cammisotto V, Cangemi R, Ferro D, Miele MC, De Angelis M, et al. Low-Grade endotoxemia and thrombosis in COVID-19. Clin Transl Gastroenterol. (2021) 12:e00348. doi: 10.14309/ctg.0000000000000348
6. Cangemi R, Pignatelli P, Carnevale R, Bartimoccia S, Nocella C, Falcone M, et al. Low-grade endotoxemia, gut permeability and platelet activation in community-acquired pneumonia. J Infect. (2016) 73:107–14. doi: 10.1016/j.jinf.2016.05.013
7. Hanada S, Pirzadeh M, Carver KY, Deng JC. Respiratory viral infection-induced microbiome alterations and secondary bacterial pneumonia. Front Immunol. (2018) 9:1–15. doi: 10.3389/fimmu.2018.02640
8. Zhou X, Li J, Guo J, Geng B, Ji W, Zhao Q, et al. Gut-dependent microbial translocation induces inflammation and cardiovascular events after ST-elevation myocardial infarction. Microbiome. (2018) 6:66. doi: 10.1186/s40168-018-0441-4
9. Ghosh S, Whitley CS, Haribabu B, Jala VR. Regulation of intestinal barrier function by microbial metabolites. CMGH Cell Mol Gastroenterol Hepatol. (2021) 11:1463–82. doi: 10.1016/j.jcmgh.2021.02.007
10. Cani PD, Amar J, Iglesias MA, Poggi M, Knauf C, Bastelica D, et al. Metabolic endotoxemia initiates obesity and insulin resistance. Diabetes. (2007) 56:1761–72. doi: 10.2337/db06-1491
11. Erridge C, Attina T, Spickett CM, Webb DJ. A high-fat meal induces low-grade endotoxemia: evidence of a novel mechanism of postprandial inflammation. Am J Clin Nutr. (2007) 86:1286–92. doi: 10.1093/ajcn/86.5.1286
12. Spadoni I, Zagato E, Bertocchi A, Paolinelli R, Hot E, Di Sabatino A, et al. A gut-vascular barrier controls the systemic dissemination of bacteria. Science. (2015) 350:830–4. doi: 10.1126/science.aad0135
13. Wood Heickman LK, DeBoer MD, Fasano A. Zonulin as a potential putative biomarker of risk for shared type 1 diabetes and celiac disease autoimmunity. Diabetes Metab Res Rev. (2020) 36:1–27. doi: 10.1002/dmrr.3309
14. Fasano A. Intestinal permeability and its regulation by zonulin: diagnostic and therapeutic implications. Clin Gastroenterol Hepatol. (2012) 10:1096–100. doi: 10.1016/j.cgh.2012.08.012
15. Jayashree B, Bibin YS, Prabhu D, Shanthirani CS, Gokulakrishnan K, Lakshmi BS, et al. Increased circulatory levels of lipopolysaccharide (LPS) and zonulin signify novel biomarkers of proinflammation in patients with type 2 diabetes. Mol Cell Biochem. (2014) 388:203–10. doi: 10.1007/s11010-013-1911-4
16. Aasbrenn M, Lydersen S, Farup PG. Changes in serum zonulin in individuals with morbid obesity after weight-loss interventions: a prospective cohort study. BMC Endocr Disord. (2020) 20:1–20. doi: 10.1186/s12902-020-00594-5
17. Carnevale R, Sciarretta S, Valenti V, di Nonno F, Calvieri C, Nocella C, et al. Low-grade endotoxaemia enhances artery thrombus growth via toll-like receptor 4: implication for myocardial infarction. Eur Heart J. (2020) 41:3156–65. doi: 10.1093/eurheartj/ehz893
18. Smith SM, Eng RHK, Campos JM, Chmel H. D-Lactic acid measurements in the diagnosis of bacterial infections. J Clin Microbiol. (1989) 27:385–8. doi: 10.1128/jcm.27.3.385-388.1989
19. Sun XQ, Fu XB, Zhang R, Lü Y, Deng Q, Jiang XG, et al. Relationship between plasma D(-)-lactate and intestinal damage after severe injuries in rats. World J Gastroenterol. (2001) 7:555–8. doi: 10.3748/wjg.v7.i4.555
20. Fukui H. Gut-liver axis in liver cirrhosis: how to manage leaky gut and endotoxemia. World J Hepatol. (2015) 7:425. doi: 10.4254/wjh.v7.i3.425
21. Sequeira IR, Lentle RG, Kruger MC, Hurst RD. Standardising the lactulose mannitol test of gut permeability to minimise error and promote comparability. PLoS One. (2014) 9:1–11. doi: 10.1371/journal.pone.0099256
22. Cani PD, Bibiloni R, Knauf C, Waget A, Neyrinck AM, Delzenne NM, et al. Changes in gut microbiota control metabolic endotoxemia-induced inflammation in high-fat diet-induced obesity and diabetes in mice. Diabetes. (2008) 57:1470–81. doi: 10.2337/db07-1403
23. Pastori D, Carnevale R, Nocella C, Novo M, Santulli M, Cammisotto V, et al. Gut-derived serum lipopolysaccharide is associated with enhanced risk of Major adverse cardiovascular events in atrial fibrillation: effect of adherence to mediterranean diet. J Am Heart Assoc. (2017) 6:e005784. doi: 10.1161/JAHA.117.005784
Keywords: gut microbiota, gut permeability, thrombosis, cardiovasclar disease, LPS (lipopolysaccharide)
Citation: Violi F and Nocella C (2023) Editorial: Gut permeability-related endotoxemia and cardiovascular disease: A new clinical challenge. Front. Cardiovasc. Med. 10:1118625. doi: 10.3389/fcvm.2023.1118625
Received: 7 December 2022; Accepted: 9 March 2023;
Published: 21 March 2023.
Edited and Reviewed by: Mary G. Sorci-Thomas, Medical College of Wisconsin, United States
© 2023 Violi and Nocella. This is an open-access article distributed under the terms of the Creative Commons Attribution License (CC BY). The use, distribution or reproduction in other forums is permitted, provided the original author(s) and the copyright owner(s) are credited and that the original publication in this journal is cited, in accordance with accepted academic practice. No use, distribution or reproduction is permitted which does not comply with these terms.
*Correspondence: Francesco Violi ZnJhbmNlc2NvLnZpb2xpQHVuaXJvbWExLml0
Specialty Section: This article was submitted to Lipids in Cardiovascular Disease, a section of the journal Frontiers in Cardiovascular Medicine