- 1The First Clinical Medical College, Lanzhou University, Lanzhou, China
- 2Department of Heart Center, The First Hospital of Lanzhou University, Lanzhou, China
- 3Key Laboratory for Cardiovascular Diseases of Gansu Province, The First Hospital of Lanzhou University, Lanzhou, China
Purpose of Review: Long-term use of statins had been confirmed to cause an increase in hyperglycemic adverse events (HAEs), whose mechanism has been well understood. Proprotein convertase subtilisin/kexin type 9 (PCSK9) monoclonal antibodies (PCSK9-mAbs), a kind of new lipid-lowering drug, can effectively reduce plasma low-density lipoprotein cholesterol levels in patients with CHD and have been widely used. However, animal experiments, Mendelian randomization studies, clinical researches and Meta-analyses which focused on the relationship between PCSK9-mAbs and HAEs had reached different conclusions, which has attracted great attention from clinicians.
Recent Findings: The newest FOURIER-OLE randomized controlled trial followed PCSK9-mAbs users for over 8 years, whose results suggested that long-term use of PCSK9-mAbs did not increase the incidence of HAEs. Newest Meta-analyses also indicated that there was no relationship between PCSK9-mAbs and NOD. Meanwhile, genetic polymorphisms and variants related to PCSK9 might have effects on HAEs.
Conclusion: According to the results of current studies, there is no significant relationship between PCSK9-mAbs and HAEs. However, longer-term follow-up studies are still needed to confirm it. Although PCSK9 genetic polymorphisms and variants may affect the possible occurrence of HAEs, there is no need to perform relevant genetic testing before applying PCSK9-mAbs.
1. Introduction
Dyslipidemia is an important cause of coronary heart disease (CHD). Therefore, lipid control is extremely important in the long-term management of CHD. Type 2 diabetes mellitus (T2DM), as one of the highest risk factors of CHD, is also a common complication in patients with it.
Statins have a clear and reliable effect on lowering plasma low-density lipoprotein cholesterol (LDL-C) levels, and have been used as the first-choice lipid-lowering drugs in clinical practice for more than 30 years. However, long-term use of statins has been confirmed to cause an increase in hyperglycemic adverse events (HAEs), including impaired fasting plasma glucose (FPG), increased glycated haemoglobin (HbA1c), and even new-onset diabetes (NOD), through various mechanisms such as inhibiting plasma glucose uptake, promoting insulin resistance, and inhibiting insulin secretion (1, 2). In human's circulation, after LDL-C in plasma is bound to LDL receptor (LDLR) on the surface of hepatocytes, it will be endocytosed into hepatocytes and hydrolyzed by lysosomal hydrolases (3). In 2003, Abifadel et al. discovered a human protein convertase playing an important role in lipid metabolism, which was named proprotein convertase subtilisin/kexin type 9 (PCSK9) (4). PCSK9 is mainly produced and secreted by hepatocytes, to increase plasma LDL-C levels by reducing LDLR abundance on the surface of hepatocytes (Figure 1A). PCSK9 monoclonal antibodies (PCSK9-mAbs) are one kind of PCSK9 inhibitors. As a new lipid-lowering drug, it can bind to plasma soluble PCSK9 molecules, preventing them binding to LDLR and reducing degradation of them. In this way, PCSK9-mAbs may increase intracellular transport of LDL-C and reduce concentration in plasma (5). Nowadays, there are kinds of PCSK9 inhibitors, including three PCSK9-mAbs, alirocumab, evolocumab and bococizumab, as well as PCSK9 small interfering RNA (siRNA), inclisiran, and so on. In large clinical trials, PCSK9-mAbs have shown 60% LDL-C reduction and 20%–25% decrease in major cardiovascular events (MACEs) (6–8). Among them, alirocumab and evolocumab had been approved by the US Food and Drug Administration as well as European Medicines Agency in 2015 for treating patients with familial hypercholesterolemia (FH), statin intolerance or contraindication, and atherosclerotic cardiovascular diseases.
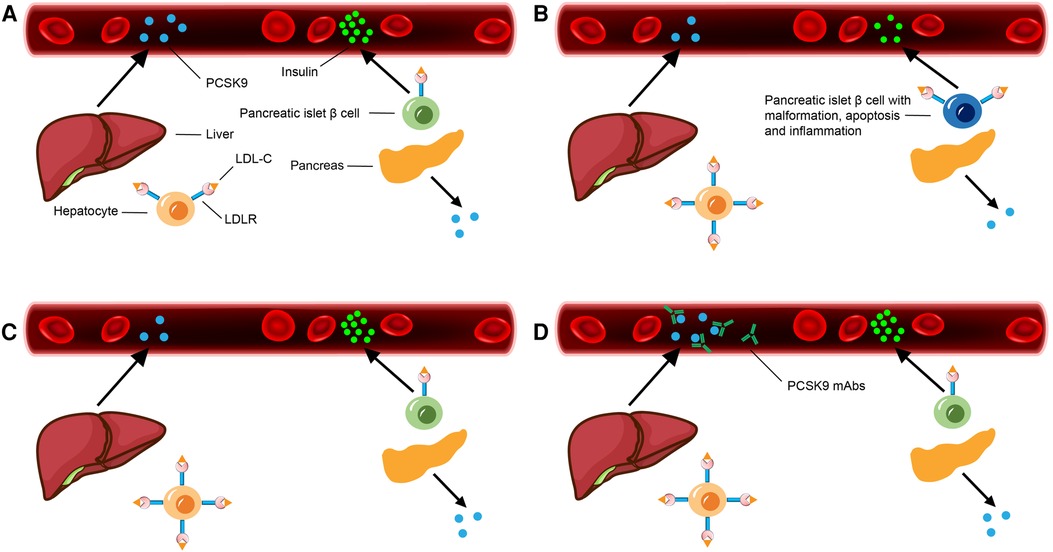
Figure 1. PCSK9 secretion of liver as well as pancreas and insulin secretion of pancreatic islet β cells in different situations. (A) Under normal physiological state. (B) Under physiological state of PCSK9−/− gene individual: PCSK9 secretion of liver and pancreas decreases, LDLR abundance on the surface and LDL-C endocytosis of hepatocytes as well as pancreatic islet β cells increase, with insulin secretion of pancreatic islet β cells decreases significantly. (C) After PCSK9 LSKO: PCSK9 secretion of liver decreases, LDLR abundance on the surface and LDL-C endocytosis of hepatocytes increase. LDLR abundance on the surface, PCSK9 secretion and insulin secretion of pancreatic islet β cells are normal. (D) After applying PCSK9-mAbs: PCSK9 secretion of liver is normal. The secreted PCSK9 is bound with PCSK9-mAbs and degrades in circulation, with LDLR abundance on the surface and LDL-C endocytosis of hepatocytes increase. LDLR abundance on the surface, PCSK9 secretion and insulin secretion of pancreatic islet β cells are normal.
However, animal experiments, Mendelian randomization studies, clinical researches and Meta-analyses which focused on the relationship between PCSK9-mAbs and HAEs had reached different conclusions, which has attracted great attention from clinicians. This article mainly reviews advanced progress of the relationship between PCSK9-mAbs and HAEs.
2. Research status of the relationship between PCSK9 & PCSK9-mAbs and HAEs
2.1. Animal experiment
Previous experiment had shown that, pancreatic islet β cells expressed significant amounts of LDLR (Figure 1A). LDLR could mediate uptake of exogenous lipoproteins through established pancreatic islet β cell lines as well as isolated and cultured islets. Long time exposure to high LDL-C or very low-density lipoprotein cholesterol (VLDL-C) level was lethal for these cells, and this effect was LDLR dependent. However, high density lipoprotein cholesterol (HDL-C) appeared to have a protective effect (9). In an animal experiment, a semi-quantitative immunoblotting (sqIB) analysis of proteins extracted from pancreatic tissue displayed that PCSK9−/− mice expressed twofold more LDLR abundance in this organ than PCSK9+/+ mice. Besides, compared with PCSK9+/+ mice, in pancreas of PCSK9−/− male mice older than 4 months, there was more LDLR and less insulin. In this way, PCSK9−/− male mice were with hypoinsulinemia, hyperglycemia and glucose intolerance. At the same time, their pancreatic islets exhibited signs of malformation, apoptosis and inflammation under microscope (10). These results suggest that, systemic defects in PCSK9 may negatively affect function of pancreatic islet β cells, with harmful effects on glucose homeostasis (Figure 1B).
Another gene knockout (KO) animal experiment focused on glycemic changes in PCSK9 KO mice and albumin AlbCre+/PCSK9LoxP/LoxP (PCSK9 liver selective KO, PCSK9 LSKO) mice. Compared with WT mice, after 20 weeks, PCSK9 KO mice given a standard or high-fat diet both showed significantly impaired glucose clearance, increased insulin levels in pancreatic islet β cells, and decreased insulin as well as C-peptide levels in circulation. As for PCSK9 LSKO mice, PCSK9 levels in circulation were below the limit of detection while maintaining PCSK9 production in other organs, including pancreas (Figure 1C). This situation is very similar to patients treated with PCSK9-mAbs, in which PCSK9 is absent from circulation but can still be produced in extrahepatic organs. Under this condition, their insulin levels in circulation and pancreatic islet β cells, LDLR abundance on the surface of pancreatic islet β cells and LDL-C levels in pancreatic islet β cells were similar to those of WT mice. Thus, glucose tolerance and insulin tolerance also remained normal (11). These results indicate that plasma PCSK9 affects, if any, LDLR abundance on the surface of pancreatic islet β cells slightly, and suggest that anti-PCSK9 therapies targeting circulating PCSK9 may affect LDLR abundance on the surface of pancreatic islet β cells limitedly. These also suggest that circulating and liver-derived PCSK9, the principal targets of PCSK9-mAbs, does not have a significant effect on function and insulin secretion of pancreatic islet β cells.
In this way, the key to determining whether plasma glucose is elevated is whether PCSK9 secretion of pancreatic islet β cells is absent, which directly affects LDLR abundance on the surface of pancreatic islet β cells and cholesterol metabolism balance. The commercial PCSK9-mAbs targeting hepatic PCSK9 deficiency don't affect PCSK9 secretion of pancreatic islet β cells, indicating that application of PCSK9-mAbs is safe.
2.2. Mendelian randomization study
A Meta-analysis showed that, exposure to LDL-C lowering genetic variants in or near NPC1L1 as well as other genes was related to higher risk of T2DM. Meanwhile, it found that, there was a relationship between PCSK9 variants and higher risk of T2DM. This research pointed out that, unlike the relationship between LDL-C lowering alleles and cardiovascular risk, the relationship between these alleles and metabolic risk appeared to be gene-specific, which might suggest there were target-specific adverse consequences of LDL-C lowering agents on the risk of T2DM (12).
Further Mendelian randomization study found that, PCSK9 variants and 3-hydroxy-3-methylglutaryl–coenzyme A reductase (HMGCR) variants had roughly the same effect on the risk of MACEs and T2DM per unit decrease in LDL-C level, whose scores had additive effects. Based on this, they made an assumption that PCSK9-mAbs might increase the risk of NOD like statins too. However, the increased risk of NOD associated with PCSK9 and HMGCR variants appeared to be limited in patients with impaired FPG. Thus, as with statins, any potential increase in the risk of NOD during PCSK9-mAbs therapy might be limited in patients with impaired FPG too. Meanwhile, this study also pointed out that, lifetime exposure to reduced LDL-C levels mediated by genetic variants was related to a much greater reduction in the risk of CHD per unit decrease in LDL-C level than short-term PCSK9-mAbs therapy. In this way, it gave an assumption that, effect of estimated PCSK9 variants on the risk of MACEs (and probably NOD) was likely to be quantitatively much larger than effect of PCSK9-mAbs treatment, measured by unit decrease in LDL-C level, which has been observed in ongoing outcome trials (13).
Another Mendelian randomization study, with a sample size of more than 600,000 patients, conjointly analyzed four independent PCSK9 variants (rs2479409, rs11206510, rs11583680, and rs11591147). This approach assumed additive effects for all single nucleotide polymorphisms, which were well validated in sensitivity analyses. This study concluded that, genetic variants in PCSK9 were related to lower LDL-C levels in plasma and higher risk of T2DM. And these variants were also related to higher FPG, bodyweight, as well as waist-to-hip ratio (14). However, just as another article presented, this study also pointed out that, effect estimates obtained from several Mendelian randomization studies could only represent lifetime exposure to natural genetic variants. Therefore, it might not be directly translated to effect size of any corresponding treatment introduced much later in life since its shorter duration (14, 15).
The newest Mendelian randomization study showed that, for PCSK9-mAbs, LDL-C lowering per standard deviation decrease in PCSK9 expression indicated a modest T2DM risk reduction. However, after adjustment for other lipids and physical activity, the relationship between PCSK9-mAbs application and T2DM was not observed (16). This suggests that the use of PCSK9-mAbs may not be associated with increased risk of HAEs.
2.3. Clinical research and Meta-analysis
As for alirocumab, an early randomized controlled trial, ODYSSEY, followed 3,448 patients without T2DM at baseline for 6 to 18 months. The results showed that, compared with placebo or ezetimibe, effect of alirocumab on transition to NOD couldn't be proved (17). And a prespecified analysis of ODYSSEY randomized controlled trial, including 18,924 patients with CHD, with or without T2DM at baseline, suggested that alirocumab reduced twice MACEs among patients with T2DM as in those without T2DM. At the same time, alirocumab did not increase the risk of NOD (18). Furthermore, a post-hoc subanalysis of ODYSSEY randomized controlled trial conducted in Japan revealed that, the use of alirocumab for 52 weeks resulted in reductions of apolipoprotein B, non-HDL-C, and lipoprotein(a) levels as well as increases of HDL-C and apolipoprotein A-1 levels in patients with or without T2DM at baseline. Importantly, this analysis did not find any apparent association between the use of alirocumab and HAEs (19). However, a further study based on ODYSSEY randomized controlled trial showed that, baseline lipoprotein(a) level might have effects on the relationship between alirocumab and HAEs: at low baseline lipoprotein(a) levels, alirocumab seemed to reduce incidence of T2DM; while at high baseline lipoprotein(a) levels, alirocumab seemed to increase incidence of T2DM (20). When it comes to evolocumab, FOURIER randomized controlled trial followed 27,564 patients for 2.2 years, concluding that it did not increase the risk of NOD in patients without T2DM at baseline, including those with prediabetes (21). The newest FOURIER-OLE randomized controlled trial showed that, compared with the placebo control group, using evolocumab over 8 years significantly reduced incidence of MACEs. At the same time, long-term use of evolocumab did not increase incidence of NOD, suggesting that it was not significantly related with HAEs (22).
When referring to whether the dose of PCSK9-mAbs have effects on HAEs, one clinical research based on ODYSSEY randomized controlled trial found that, compared with those who did not receive alirocumab, there was no significant changes in FPG and HbA1c among patients applied alirocumab at the largest dose (300 mg every 4 weeks) (23). What's more, FOURIER-OLE randomized controlled trial also indicated that, using evolocumab over 8 years at normal dose (140 mg every 2 weeks or 420 mg every 4 weeks) would not increase the incidence of HAEs (22).
Recent comparative drug retrospective research showed that, in a real-world setting, HAEs were reported more common in PCSK9-mAbs treatment compared with ezetimibe, but less common compared with statins. When PCSK9-mAbs were subdivided into alirocumab and evolocumab, this research found that it was evolocumab, but not alirocumab, was associated with HAEs. Notably, HAEs were reported more frequently in patients with T2DM than in patients without T2DM, with most occurring within 6-month treatment and being reversible with drug discontinuation. Meanwhile, this study also emphasized that, PCSK9-mAbs therapy was related to increased report of mild hyperglycemia, but not NOD (24). Furthermore, one clinical study found that, after 1-year treatment, insulin resistance of patients using statins monotherapy was significantly increased, while insulin resistance in patients who applied ezetimibe and statins was no significantly increased, even tended to decrease (25).
Two earlier Meta-analyses both showed that alirocumab and evolocumab had no significant effect on incidence of T2DM and glucose metabolic disorder (26, 27). Another Meta-analysis suggested that, application of PCSK9-mAbs would lead to a small but significant increase in FPG and HbA1c in short term, but this effect was not sufficient to increase incidence of NOD (28). The results of two newest Meta-analyses, including 30 and 32 randomized controlled trials respectively, also indicated that there was no relationship between PCSK9-mAbs and NOD (29, 30). What's more, one Meta-analysis took PCSK9-mAb type into consideration, which found that alirocumab was associated with a significant reduction in the risk of NOD, whereas no significant reduction was observed in evolocumab (31), suggesting that the type of PCSK9-mAbs might have effects on the relationship between PCSK9-mAbs and HAEs. The reason why this happened might be attributed to that the body produced different anti-drug antibodies after applying two different types of PCSK9-mAbs.
According to the current researches, it can conclude that, applying PCSK9-mAbs at permitted dose does not cause significant NOD or T2DM, with the worst effect being a mild increase in FPG.
2.4. Other PCSK9 inhibitors
Inclisiran, a PCSK9 siRNA, is a novel post-transcriptional gene silencing therapy, which has a powerful, dose-dependent, long-lasting effect in lowering LDL-C by inhibiting the synthesis of PCSK9 through RNA interference. Inclisiran is a chemically synthesized siRNA that is covalently linked to a ligand containing three N-acetylgalactosamine residues. These residues lead to hepatocyte specific uptake of siRNA, where inclisiran binds to the RNA-induced silencing complex that breaks PCSK9 mRNA, thereby inhibiting PCSK9 synthesis (32). Therefore, it seems to be an effective drug to treat dyslipidemia and reduce the risk of MACEs (33). Like commercial PCSK9-mAbs, inclisiran is also targeting liver. In consequence, inclisiran may have no effect on HAEs in theory, and this has also been confirmed by clinical researches. ORION randomized clinical trial followed 501 subjects for half a year, whose result showed that there was no clinically meaningful change in HbA1c after using inclisiran (34), indicating that there was no significant relationship between inclisiran and HAEs.
According to evidence level pyramid for different study types (Figure 2), it can summarize the studies mentioned above in Table 1. In conclusion, systematic reviews of randomized controlled trials and randomized controlled trials all support that there is no significant relationship between PCSK9-mAbs and HAEs. Although the results of Mendelian randomization studies are partly contrary to that, the magnifying effect of them should not be ignored.
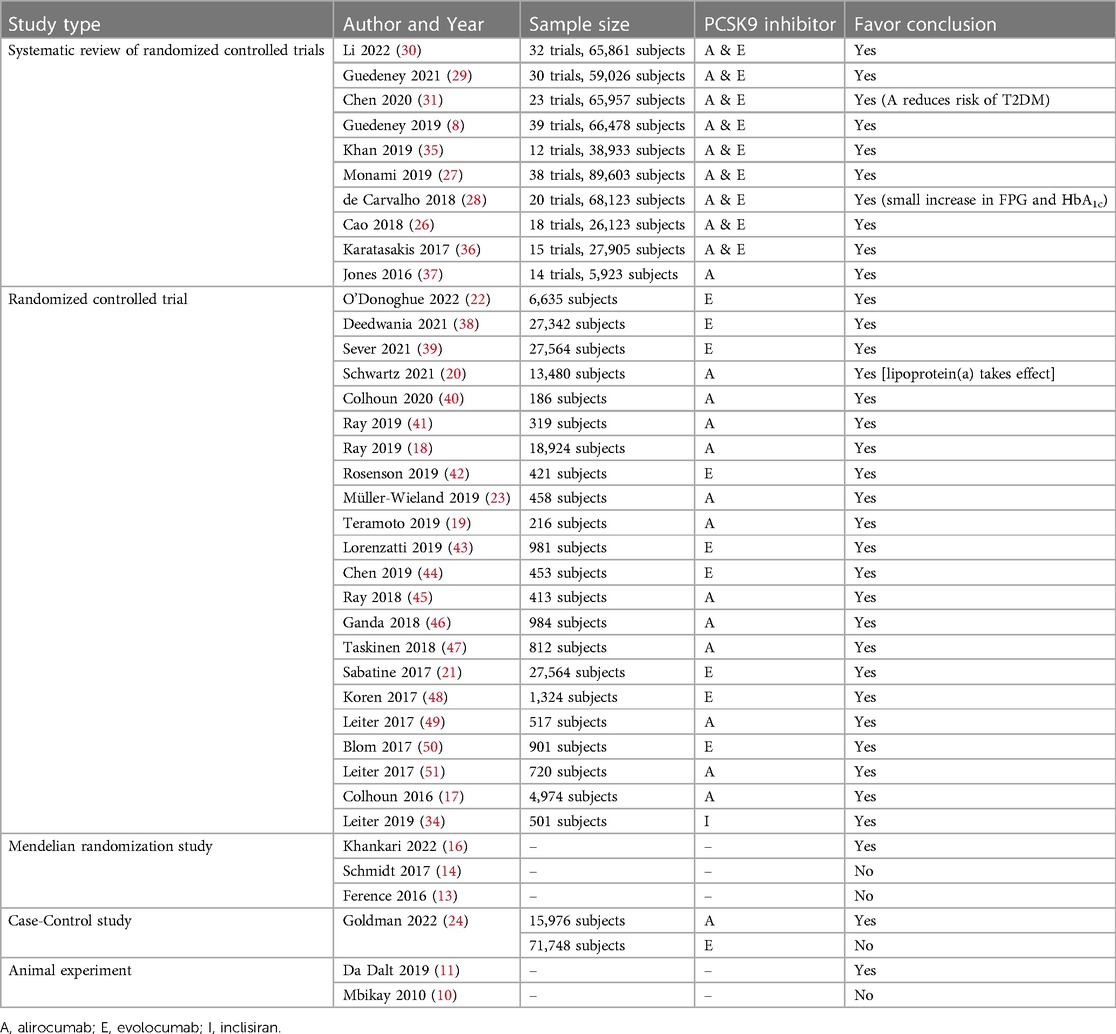
Table 1. Different study types and whether their conclusions favor “no significant relationship between PCSK9 inhibitors and HAEs”.
3. Effects of genetic polymorphisms and variants related to PCSK9 on HAEs
Over secretion of PCSK9 resulting from PCSK9 gain-of-function (PCSK9-GOF) genetic variants may result in relative PCSK9-mAbs deficiency and lead to abnormal responses to PCSK9-mAbs. Conversely, PCSK9 loss-of-function (PCSK9-LOF) genetic variants in patients with FH may lead to unusual responses to PCSK9-mAbs because of the low relative efficacy of PCSK9 in circulation (52). So, it seems that genetic polymorphisms and variants related to PCSK9 may have effects on HAEs.
3.1. PCSK9-LOF genetic variants
InsLEU mutation is one of the PCSK9-LOF mutations. A genetic study in Canadian population with FH showed that, 26% of individuals in this cohort were inherited with PCSK9-InsLEU variant. There was no significant difference in lipid profiles among InsLEU-carriers when compared with non-carriers, while incidence of MACEs was significantly lower. However, higher proportion of InsLEU-carriers were found to be with prediabetes and T2DM than non-carriers. Accordingly, InsLEU-carriers had significantly higher plasma glucose levels and were more often affected with impaired FPG than non-carriers. What's more, they observed a gene dosage effect again, with homozygous or compound InsLEU-carriers having higher FPG than heterozygous InsLEU-carriers and non-carriers. Meanwhile, prevalence of impaired FPG in InsLEU gene polymorphism carriers was almost twice that in non-carriers, and proportion of homozygous or compound InsLEU-carriers with impaired FPG was higher than that of heterozygous InsLEU-carriers and non-carriers, whose trend was remarkable. In this study, after sequencing for PCSK9 exon 1 of 724 French-Canadian FH individuals carrying FH causing LDLR mutations, 188 individuals among them carried additional leucines (L10, L11), and its allelic frequency was 14% (53). Indeed, one previous study had presented that allelic frequency was 19% in a cohort composed of non-FH French-Canadian children (54). According to several studies, allelic frequency in other people ranged from 9.6% to 25% (4, 55–60).
Based on what have presented above, it can conclude that, PCSK9-LOF genetic variants are relatively common among population. In consequence, it is not necessary to test PCSK9 gene in patients before applying PCSK9-mAbs.
3.2. PCSK9-GOF genetic variants
It had proved that there was a link between PCSK9-GOF mutations and high plasma LDL-C levels (61), since they decreased LDLR abundance more efficiently when compared with wild-type PCSK9 (62). Based on this, PCSK9-GOF mutations could lead to FH, whose serum lipoprotein(a) level was elevated (63). A cross-sectional study including 63,320 patients in the Netherlands indicated that, incidence of T2DM in patients with FH was obviously (up to 40%) lower than that in unaffected relatives, with variability by mutation type (9).
In this way, it can reasonably believe that, different from PCSK9-LOF mutations are associated with HAEs, PCSK9-GOF mutations can decrease incidence of HAEs, although the extent of effect depends on mutation type.
3.3. Genes that regulate PCSK9 determine the function of PCSK9
According to the present studies, there are some genes that regulate PCSK9 and determine the function of PCSK9 (64–68).
The first one, cyclase associated protein 1 (CAP1), a new binding partner for PCSK9, has been identified and considered to be required for LDLR degradation by PCSK9. When PCSK9 catalytic domain bound LDLR, cysteine, histidine-rich domain (CHRD) of PCSK9 was found to interact with CAP1, resulted in degradation of the protein complex LDLR/PCSK9/CAP1 in lysosomes through caveolin-dependent mechanism. In this way, there are two different fates for LDLR/PCSK9 complex which depend on binding partners: degradation when dependent on caveolin pathway and recycling when dependent on clathrin pathway. What's more, prevention of PCSK9-mediated LDLR degradation could be found when used hepatocytes cultured with CAP1 siRNA and in heterozygous CAP1 KO mice. Meanwhile, PCSK9-LOF polymorphisms in human showed a defective interaction with CAP1 (64, 65). In this way, if LDLR/PCSK9 complex was degraded through caveolin pathway, the LDL-C endocytosis of hepatocytes would be weakened; on the contrary, if LDLR/PCSK9 complex was recycled through clathrin pathway, the LDL-C endocytosis of hepatocytes would be continued, and CAP1 might take an important role in this process. Based on this, the different fates for LDLR/PCSK9 complex may have effects on glycometabolism.
The other two are sterol regulatory element (SRE) binding proteins (SREBPs) and hepatocyte nuclear factor 1α (HNF1α). In a transgenic experiment, PCSK9 was observed an obvious upregulation in both male and female mice who were transformed with SREBP-1a or SREBP-2 (66, 67). This result suggests that sterol-dependent regulation of PCSK9 is mainly mediated by SREBPs. A further study identified HNF1 binding site as a critical cis-regulatory sequence in PCSK9 promoter, which was highly conserved and located between SRE and Sp1 site. It mutated the core nucleotide sequence of HNF1 site, and the data showed that disruption of HNF1 binding site reduced activity of PCSK9 promoter to only 5% of that in wild type, even lower than SRE mutation. Moreover, it also suggested that liver-enriched transcription factor HNF1α was the principal form of HNF1 factors that bound HNF1 sequence motifs to stimulate PCSK9 transcription (67, 68).
Therefore, it can conclude that, apart from PCSK9 itself, there are several other genes which may have effects on biological characteristics of PCSK9, and these can probably contribute to explaining why some patients developed HAEs after applying PCSK9-mAbs in some clinical researches. However, up to now, there has been no report about effects of these genes on lipid metabolism, suggesting that these gene mutations were often ignored in studies focused on lipid metabolism. In the future, sequence of these genes should be added to related researches.
4. Discussion
So far, animal experiments, Mendelian randomization studies, clinical researches and Meta-analyses which focused on the relationship between PCSK9-mAbs and HAEs have reached different conclusions, whose reasons can be mainly analyzed as follows.
First of all, from the study focusing on PCSK9 variants, it can conclude that insulin secretion of PCSK9−/− mice is significantly lower than that of PCSK9+/+ mice (10), indicating that PCSK9 in systemic circulation has significant effects on function and insulin secretion of pancreatic islet β cells. In Mendelian randomization studies, the content of these studies is still about mutations of PCSK9 gene (13, 14), which is consistent with the nature of animal experiments. The results of further study based on PCSK9 KO mice also confirm it (11).
Second, circulating PCSK9 levels in PCSK9 LSKO mice decrease significantly, while PCSK9 production is normal in other organs, including pancreas (11). This effect is similar to applying PCSK9-mAbs: PCSK9-mAbs are highly targeted and can precisely inhibit PCSK9 production in liver without affecting it in other organs. This has also been confirmed by the results of many clinical researches and Meta-analyses: there is no significant relationship between PCSK9-mAbs and HAEs (17–24, 26–31). Furthermore, applying PCSK9-mAbs and ezetimibe together may help to keep glycometabolism stable, which still needs further clinical researches to confirm.
The key reason for why different kinds of experiments and researches have reached these different results is the highly targeted nature of PCSK9-mAbs, which determines that effect of PCSK9-mAbs on HAEs cannot be deduced simply and directly from conclusions about effect of PCSK9 gene variants on HAEs. At the same time, it should be noted that the reasons for this difference may not be related to duration of medication suggested in the above studies (Figure 1D).
When it comes to polymorphisms of PCSK9 gene, PCSK9-LOF variants can lead to decreased PCSK9 secretion of pancreatic islet β cells, then, impaired FPG, NOD and even T2DM. Meanwhile, PCSK9-GOF variants can lead to FH without any association with HAEs. However, since large scale cohort researches have shown that, genetic variants in PCSK9-LOF and PCSK9-GOF are relatively common (69, 70), it is not necessary to test PCSK9 gene in patients before applying PCSK9-mAbs.
At the same time, there are many other factors in the body which may have effects on biological characteristics of PCSK9, and these can probably contribute to explaining why some patients developed HAEs after applying PCSK9-mAbs in clinical researches.
There are several other published review articles on this topic. We have following advantages and differences compared with the latest review (71). First of all, although the article mentioned role of PCSK9-mAbs in pancreatic islet β cells, it ignored that currently commercial PCSK9-mAbs were targeting liver, whose effect on pancreatic islet β cells was minimal. We have paid attention to this point and believe that it is precisely because of the liver's precise targeting that there have been no adverse events of hyperglycemia. Secondly, we take effects of genetic polymorphisms of PCSK9 on HAEs into consideration, which shows polymorphisms and variants of PCSK9 can also have effects on the development of NOD. What's more, we include the newest Meta-analysis (30), which supports our conclusion “effects of PCSK9-mAbs on HAEs are not clinically significant”.
5. Conclusion
In conclusion, according to the results of current studies, there is no significant relationship between PCSK9-mAbs and HAEs. However, longer-term follow-up studies are still needed to confirm it. Although PCSK9 genetic polymorphisms and variants may affect the possible occurrence of HAEs, there is no need to perform relevant genetic testing before applying PCSK9-mAbs.
Author contributions
RZ: Conceptualization, Methodology, Writing—Original Draft, Visualization. YW: Writing—Review & Editing, Visualization. YP: Methodology, Writing—Review & Editing, Supervision. JZ: Supervision. ZZ: Project Administration, Funding Acquisition. All authors contributed to the article and approved the submitted version.
Funding
This work was supported by National Key Research and Development Program of China (No. 2018YFC1311505); Clinical Cooperative Pilot Project of Traditional Chinese and Western Medicine for Major Difficult Diseases (No. State Office of Traditional Chinese Medicine Medical Administration [2018], No. 3); and Cardiovascular Clinical Research Center of Gansu Province (No.18JR2FA005).
Conflict of interest
The authors declare that the research was conducted in the absence of any commercial or financial relationships that could be construed as a potential conflict of interest.
Publisher's note
All claims expressed in this article are solely those of the authors and do not necessarily represent those of their affiliated organizations, or those of the publisher, the editors and the reviewers. Any product that may be evaluated in this article, or claim that may be made by its manufacturer, is not guaranteed or endorsed by the publisher.
References
1. Wang KL, Liu CJ, Chao TF, Huang CM, Wu CH, Chen SJ, et al. Statins, risk of diabetes, and implications on outcomes in the general population. J Am Coll Cardiol. (2012) 60(14):1231–8. doi: 10.1016/j.jacc.2012.05.019
2. Carmena R, Betteridge DJ. Diabetogenic action of statins: mechanisms. Curr Atheroscler Rep. (2019) 21(6):23. doi: 10.1007/s11883-019-0780-z
3. Goldstein JL, Brown MS. The LDL receptor. Arterioscler Thromb Vasc Biol. (2009) 29(4):431–8. doi: 10.1161/ATVBAHA.108.179564
4. Abifadel M, Varret M, Rabes JP, Allard D, Ouguerram K, Devillers M, et al. Mutations in PCSK9 cause autosomal dominant hypercholesterolemia. Nat Genet. (2003) 34(2):154–6. doi: 10.1038/ng1161
5. Lekuona I. PCSK9 Inhibitors: from innovation to sustainable clinical application. Rev Esp Cardiol (Engl Ed). (2018) 71(12):996–8. doi: 10.1016/j.rec.2018.06.032
6. Sabatine MS, Giugliano RP, Wiviott SD, Raal FJ, Blom DJ, Robinson J, et al. Efficacy and safety of evolocumab in reducing lipids and cardiovascular events. N Engl J Med. (2015) 372(16):1500–9. doi: 10.1056/NEJMoa1500858
7. Sabatine MS, Giugliano RP, Keech AC, Honarpour N, Wiviott SD, Murphy SA, et al. Evolocumab and clinical outcomes in patients with cardiovascular disease. N Engl J Med. (2017) 376(18):1713–22. doi: 10.1056/NEJMoa1615664
8. Guedeney P, Giustino G, Sorrentino S, Claessen BE, Camaj A, Kalkman DN, et al. Efficacy and safety of alirocumab and evolocumab: a systematic review and meta-analysis of randomized controlled trials. Eur Heart J. (2019) 43(7):e17–e25. doi: 10.1093/eurheartj/ehz430
9. Besseling J, Kastelein JJ, Defesche JC, Hutten BA, Hovingh GK. Association between familial hypercholesterolemia and prevalence of type 2 diabetes mellitus. JAMA. (2015) 313(10):1029–36. doi: 10.1001/jama.2015.1206
10. Mbikay M, Sirois F, Mayne J, Wang GS, Chen A, Dewpura T, et al. PCSK9-deficient mice exhibit impaired glucose tolerance and pancreatic islet abnormalities. FEBS Lett. (2010) 584(4):701–6. doi: 10.1016/j.febslet.2009.12.018
11. Da Dalt L, Ruscica M, Bonacina F, Balzarotti G, Dhyani A, Di Cairano E, et al. PCSK9 Deficiency reduces insulin secretion and promotes glucose intolerance: the role of the low-density lipoprotein receptor. Eur Heart J. (2019) 40(4):357–68. doi: 10.1093/eurheartj/ehy357
12. Lotta LA, Sharp SJ, Burgess S, Perry JRB, Stewart ID, Willems SM, et al. Association between low-density lipoprotein cholesterol-lowering genetic variants and risk of type 2 diabetes: a meta-analysis. JAMA. (2016) 316(13):1383–91. doi: 10.1001/jama.2016.14568
13. Ference BA, Robinson JG, Brook RD, Catapano AL, Chapman MJ, Neff DR, et al. Variation in PCSK9 and HMGCR and risk of cardiovascular disease and diabetes. N Engl J Med. (2016) 375(22):2144–53. doi: 10.1056/NEJMoa1604304
14. Schmidt AF, Swerdlow DI, Holmes MV, Patel RS, Fairhurst-Hunter Z, Lyall DM, et al. PCSK9 Genetic variants and risk of type 2 diabetes: a Mendelian randomisation study. Lancet Diabetes Endocrinol. (2017) 5(2):97–105. doi: 10.1016/S2213-8587(16)30396-5
15. Burgess S, Butterworth A, Malarstig A, Thompson SG. Use of Mendelian randomisation to assess potential benefit of clinical intervention. Br Med J. (2012) 345:e7325. doi: 10.1136/bmj.e7325
16. Khankari NK, Keaton JM, Walker VM, Lee KM, Shuey MM, Clarke SL, et al. Using Mendelian randomisation to identify opportunities for type 2 diabetes prevention by repurposing medications used for lipid management. EBioMedicine. (2022) 80:104038. doi: 10.1016/j.ebiom.2022.104038
17. Colhoun HM, Ginsberg HN, Robinson JG, Leiter LA, Müller-Wieland D, Henry RR, et al. No effect of PCSK9 inhibitor alirocumab on the incidence of diabetes in a pooled analysis from 10 ODYSSEY phase 3 studies. Eur Heart J. (2016) 37(39):2981–9. doi: 10.1093/eurheartj/ehw292
18. Ray KK, Colhoun HM, Szarek M, Baccara-Dinet M, Bhatt DL, Bittner VA, et al. Effects of alirocumab on cardiovascular and metabolic outcomes after acute coronary syndrome in patients with or without diabetes: a prespecified analysis of the ODYSSEY OUTCOMES randomised controlled trial. Lancet Diabetes Endocrinol. (2019) 7(8):618–28. doi: 10.1016/s2213-8587(19)30158-5
19. Teramoto T, Usami M, Takagi Y, Baccara-Dinet MT. Efficacy and safety of alirocumab in Japanese patients with diabetes Mellitus: post-hoc subanalysis of ODYSSEY Japan. J Atheroscler Thromb. (2019) 26(3):282–93. doi: 10.5551/jat.45070
20. Schwartz GG, Szarek M, Bittner VA, Bhatt DL, Diaz R, Goodman SG, et al. Relation of lipoprotein(a) levels to incident type 2 diabetes and modification by alirocumab treatment. Diabetes Care. (2021) 44(5):1219–27. doi: 10.2337/dc20-2842
21. Sabatine MS, Leiter LA, Wiviott SD, Giugliano RP, Deedwania P, De Ferrari GM, et al. Cardiovascular safety and efficacy of the PCSK9 inhibitor evolocumab in patients with and without diabetes and the effect of evolocumab on glycaemia and risk of new-onset diabetes: a prespecified analysis of the FOURIER randomised controlled trial. Lancet Diabetes Endocrinol. (2017) 5(12):941–50. doi: 10.1016/s2213-8587(17)30313-3
22. O'Donoghue ML, Giugliano RP, Wiviott SD, Atar D, Keech A, Kuder JF, et al. Long-term evolocumab in patients with established atherosclerotic cardiovascular disease. Circulation. (2022) 146(15):1109–19. doi: 10.1161/circulationaha.122.061620
23. Müller-Wieland D, Rader DJ, Moriarty PM, Bergeron J, Langslet G, Ray KK, et al. Efficacy and safety of alirocumab 300 mg every 4 weeks in individuals with type 2 diabetes on maximally tolerated statin. J Clin Endocrinol Metab. (2019) 104(11):5253–62. doi: 10.1210/jc.2018-02703
24. Goldman A, Raschi E, Cukierman-Yaffe T, Dankner R, Shouval R, Shechter M, et al. Hyperglycaemic disorders associated with PCSK9 inhibitors: a real-world, pharmacovigilance study. Eur J Prev Cardiol. (2022) 29(9):1334–42. doi: 10.1093/eurjpc/zwab209
25. Cho Y, Kim RH, Park H, Wang HJ, Lee H, Kang ES. Effect of ezetimibe on glucose metabolism and inflammatory markers in adipose tissue. Biomedicines. (2020) 8(11):512. doi: 10.3390/biomedicines8110512
26. Cao YX, Liu HH, Dong QT, Li S, Li JJ. Effect of proprotein convertase subtilisin/kexin type 9 (PCSK9) monoclonal antibodies on new-onset diabetes mellitus and glucose metabolism: a systematic review and meta-analysis. Diabetes Obes Metab. (2018) 20(6):1391–8. doi: 10.1111/dom.13235
27. Monami M, Sesti G, Mannucci E. PCSK9 Inhibitor therapy: a systematic review and meta-analysis of metabolic and cardiovascular outcomes in patients with diabetes. Diabetes Obes Metab. (2019) 21(4):903–8. doi: 10.1111/dom.13599
28. de Carvalho LSF, Campos AM, Sposito AC. Proprotein convertase subtilisin/kexin type 9 (PCSK9) inhibitors and incident type 2 diabetes: a systematic review and meta-analysis with over 96,000 patient-years. Diabetes Care. (2018) 41(2):364–7. doi: 10.2337/dc17-1464
29. Guedeney P, Sorrentino S, Giustino G, Chapelle C, Laporte S, Claessen BE, et al. Indirect comparison of the efficacy and safety of alirocumab and evolocumab: a systematic review and network meta-analysis. Eur Heart J Cardiovasc Pharmacother. (2021) 7(3):225–35. doi: 10.1093/ehjcvp/pvaa024
30. Li J, Du H, Wang Y, Aertgeerts B, Guyatt G, Hao Q, et al. Safety of proprotein convertase subtilisin/kexin 9 inhibitors: a systematic review and meta-analysis. Heart. (2022) 108(16):1296–302. doi: 10.1136/heartjnl-2021-320556
31. Chen Q, Wu G, Li C, Qin X, Liu R, Zhang M. Safety of proprotein convertase subtilisin/kexin type 9 monoclonal antibodies in regard to diabetes Mellitus: a systematic review and meta-analysis of randomized controlled trials. Am J Cardiovasc Drugs. (2020) 20(4):343–53. doi: 10.1007/s40256-019-00386-w
32. Wang N, Tall AR. A new approach to PCSK9 therapeutics. Circ Res. (2017) 120(7):1063–5. doi: 10.1161/circresaha.117.310610
33. Santulli G, Jankauskas SS, Gambardella J. Inclisiran: a new milestone on the PCSK9 road to tackle cardiovascular risk. Eur Heart J Cardiovasc Pharmacother. (2021) 7(3):e11–e2. doi: 10.1093/ehjcvp/pvab014
34. Leiter LA, Teoh H, Kallend D, Wright RS, Landmesser U, Wijngaard PLJ, et al. Inclisiran lowers LDL-C and PCSK9 irrespective of diabetes Status: the ORION-1 randomized clinical trial. Diabetes Care. (2019) 42(1):173–6. doi: 10.2337/dc18-1491
35. Khan SU, Rahman H, Okunrintemi V, Riaz H, Khan MS, Sattur S, et al. Association of lowering low-density lipoprotein cholesterol with contemporary lipid-lowering therapies and risk of diabetes Mellitus: a systematic review and meta-analysis. J Am Heart Assoc. (2019) 8(7):e011581. doi: 10.1161/jaha.118.011581
36. Karatasakis A, Danek BA, Karacsonyi J, Rangan BV, Roesle MK, Knickelbine T, et al. Effect of PCSK9 inhibitors on clinical outcomes in patients with hypercholesterolemia: a meta-analysis of 35 randomized controlled trials. J Am Heart Assoc. (2017) 6(12):e006910. doi: 10.1161/jaha.117.006910
37. Jones PH, Bays HE, Chaudhari U, Pordy R, Lorenzato C, Miller K, et al. Safety of alirocumab (A PCSK9 monoclonal antibody) from 14 randomized trials. Am J Cardiol. (2016) 118(12):1805–11. doi: 10.1016/j.amjcard.2016.08.072
38. Deedwania P, Murphy SA, Scheen A, Badariene J, Pineda AL, Honarpour N, et al. Efficacy and safety of PCSK9 inhibition with evolocumab in reducing cardiovascular events in patients with metabolic syndrome receiving statin therapy: secondary analysis from the FOURIER randomized clinical trial. JAMA Cardiol. (2021) 6(2):139–47. doi: 10.1001/jamacardio.2020.3151
39. Sever P, Gouni-Berthold I, Keech A, Giugliano R, Pedersen TR, Im K, et al. LDL-cholesterol lowering with evolocumab, and outcomes according to age and sex in patients in the FOURIER trial. Eur J Prev Cardiol. (2021) 28(8):805–12. doi: 10.1177/2047487320902750
40. Colhoun HM, Leiter LA, Müller-Wieland D, Cariou B, Ray KK, Tinahones FJ, et al. Effect of alirocumab on individuals with type 2 diabetes, high triglycerides, and low high-density lipoprotein cholesterol. Cardiovasc Diabetol. (2020) 19(1):14. doi: 10.1186/s12933-020-0991-1
41. Ray KK, Del Prato S, Müller-Wieland D, Cariou B, Colhoun HM, Tinahones FJ, et al. Alirocumab therapy in individuals with type 2 diabetes mellitus and atherosclerotic cardiovascular disease: analysis of the ODYSSEY DM-DYSLIPIDEMIA and DM-INSULIN studies. Cardiovasc Diabetol. (2019) 18(1):149. doi: 10.1186/s12933-019-0951-9
42. Rosenson RS, Daviglus ML, Handelsman Y, Pozzilli P, Bays H, Monsalvo ML, et al. Efficacy and safety of evolocumab in individuals with type 2 diabetes mellitus: primary results of the randomised controlled BANTING study. Diabetologia. (2019) 62(6):948–58. doi: 10.1007/s00125-019-4856-7
43. Lorenzatti AJ, Eliaschewitz FG, Chen Y, Lu J, Baass A, Monsalvo ML, et al. Randomised study of evolocumab in patients with type 2 diabetes and dyslipidaemia on background statin: primary results of the BERSON clinical trial. Diabetes Obes Metab. (2019) 21(6):1455–63. doi: 10.1111/dom.13680
44. Chen Y, Yuan Z, Lu J, Eliaschewitz FG, Lorenzatti AJ, Monsalvo ML, et al. Randomized study of evolocumab in patients with type 2 diabetes and dyslipidaemia on background statin: pre-specified analysis of the Chinese population from the BERSON clinical trial. Diabetes Obes Metab. (2019) 21(6):1464–73. doi: 10.1111/dom.13700
45. Ray KK, Leiter LA, Müller-Wieland D, Cariou B, Colhoun HM, Henry RR, et al. Alirocumab vs usual lipid-lowering care as add-on to statin therapy in individuals with type 2 diabetes and mixed dyslipidaemia: the ODYSSEY DM-DYSLIPIDEMIA randomized trial. Diabetes Obes Metab. (2018) 20(6):1479–89. doi: 10.1111/dom.13257
46. Ganda OP, Plutzky J, Sanganalmath SK, Bujas-Bobanovic M, Koren A, Mandel J, et al. Efficacy and safety of alirocumab among individuals with diabetes mellitus and atherosclerotic cardiovascular disease in the ODYSSEY phase 3 trials. Diabetes Obes Metab. (2018) 20(10):2389–98. doi: 10.1111/dom.13384
47. Taskinen MR, Del Prato S, Bujas-Bobanovic M, Louie MJ, Letierce A, Thompson D, et al. Efficacy and safety of alirocumab in individuals with type 2 diabetes mellitus with or without mixed dyslipidaemia: analysis of the ODYSSEY LONG TERM trial. Atherosclerosis. (2018) 276:124–30. doi: 10.1016/j.atherosclerosis.2018.07.017
48. Koren MJ, Sabatine MS, Giugliano RP, Langslet G, Wiviott SD, Kassahun H, et al. Long-term low-density lipoprotein cholesterol-lowering efficacy, persistence, and safety of evolocumab in treatment of hypercholesterolemia: results up to 4 years from the open-label OSLER-1 extension study. JAMA Cardiol. (2017) 2(6):598–607. doi: 10.1001/jamacardio.2017.0747
49. Leiter LA, Cariou B, Müller-Wieland D, Colhoun HM, Del Prato S, Tinahones FJ, et al. Efficacy and safety of alirocumab in insulin-treated individuals with type 1 or type 2 diabetes and high cardiovascular risk: the ODYSSEY DM-INSULIN randomized trial. Diabetes Obes Metab. (2017) 19(12):1781–92. doi: 10.1111/dom.13114
50. Blom DJ, Koren MJ, Roth E, Monsalvo ML, Djedjos CS, Nelson P, et al. Evaluation of the efficacy, safety and glycaemic effects of evolocumab (AMG 145) in hypercholesterolaemic patients stratified by glycaemic status and metabolic syndrome. Diabetes Obes Metab. (2017) 19(1):98–107. doi: 10.1111/dom.12788
51. Leiter LA, Zamorano JL, Bujas-Bobanovic M, Louie MJ, Lecorps G, Cannon CP, et al. Lipid-lowering efficacy and safety of alirocumab in patients with or without diabetes: a sub-analysis of ODYSSEY COMBO II. Diabetes Obes Metab. (2017) 19(7):989–96. doi: 10.1111/dom.12909
52. Krittanawong C, Khawaja M, Rosenson RS, Amos CI, Nambi V, Lavie CJ, et al. Association of PCSK9 variants with the risk of atherosclerotic cardiovascular disease and Variable responses to PCSK9 inhibitor therapy. Curr Probl Cardiol. (2022) 47(7):101043. doi: 10.1016/j.cpcardiol.2021.101043
53. Saavedra YGL, Dufour R, Baass A. Familial hypercholesterolemia: pCSK9 InsLEU genetic variant and prediabetes/diabetes risk. J Clin Lipidol. (2015) 9(6):786–93.e1. doi: 10.1016/j.jacl.2015.08.005
54. Baass A, Dubuc G, Tremblay M, Delvin EE, O'Loughlin J, Levy E, et al. Plasma PCSK9 is associated with age, sex, and multiple metabolic markers in a population-based sample of children and adolescents. Clin Chem. (2009) 55(9):1637–45. doi: 10.1373/clinchem.2009.126987
55. Leren TP. Mutations in the PCSK9 gene in Norwegian subjects with autosomal dominant hypercholesterolemia. Clin Genet. (2004) 65(5):419–22. doi: 10.1111/j.0009-9163.2004.0238.x
56. Shioji K, Mannami T, Kokubo Y, Inamoto N, Takagi S, Goto Y, et al. Genetic variants in PCSK9 affect the cholesterol level in Japanese. J Hum Genet. (2004) 49(2):109–14. doi: 10.1007/s10038-003-0114-3
57. Fasano T, Cefalù AB, Di Leo E, Noto D, Pollaccia D, Bocchi L, et al. A novel loss of function mutation of PCSK9 gene in white subjects with low-plasma low-density lipoprotein cholesterol. Arterioscler Thromb Vasc Biol. (2007) 27(3):677–81. doi: 10.1161/01.ATV.0000255311.26383.2f
58. Miyake Y, Kimura R, Kokubo Y, Okayama A, Tomoike H, Yamamura T, et al. Genetic variants in PCSK9 in the Japanese population: rare genetic variants in PCSK9 might collectively contribute to plasma LDL cholesterol levels in the general population. Atherosclerosis. (2008) 196(1):29–36. doi: 10.1016/j.atherosclerosis.2006.12.035
59. Pisciotta L, Sallo R, Rabacchi C, Wunsch A, Calandra S, Bertolini S. Leucine 10 allelic variant in signal peptide of PCSK9 increases the LDL cholesterol-lowering effect of statins in patients with familial hypercholesterolaemia. Nutr Metab Cardiovasc Dis. (2012) 22(10):831–5. doi: 10.1016/j.numecd.2011.04.003
60. Slimani A, Hrira MY, Najah M, Jomaa W, Maatouk F, Hamda KB, et al. PCSK9 Polymorphism in a Tunisian cohort: identification of a new allele, L8, and association of allele L10 with reduced coronary heart disease risk. Mol Cell Probes. (2015) 29(1):1–6. doi: 10.1016/j.mcp.2014.09.001
61. Leigh SE, Leren TP, Humphries SE. Commentary PCSK9 variants: a new database. Atherosclerosis. (2009) 203(1):32–3. doi: 10.1016/j.atherosclerosis.2009.02.006
62. Uribe KB, Chemello K, Larrea-Sebal A, Benito-Vicente A, Galicia-Garcia U, Bourane S, et al. A systematic approach to assess the activity and classification of PCSK9 variants. Int J Mol Sci. (2021) 22(24):13602. doi: 10.3390/ijms222413602
63. Tada H, Kawashiri MA, Yoshida T, Teramoto R, Nohara A, Konno T, et al. Lipoprotein(a) in familial hypercholesterolemia with proprotein convertase subtilisin/kexin type 9 (PCSK9) gain-of-function mutations. Circ J. (2016) 80(2):512–8. doi: 10.1253/circj.CJ-15-0999
64. Jang HD, Lee SE, Yang J, Lee HC, Shin D, Lee H, et al. Cyclase-associated protein 1 is a binding partner of proprotein convertase subtilisin/kexin type-9 and is required for the degradation of low-density lipoprotein receptors by proprotein convertase subtilisin/kexin type-9. Eur Heart J. (2020) 41(2):239–52. doi: 10.1093/eurheartj/ehz566
65. Barale C, Melchionda E, Morotti A, Russo I. PCSK9 biology and its role in atherothrombosis. Int J Mol Sci. (2021) 22(11):5880. doi: 10.3390/ijms22115880
66. Maxwell KN, Soccio RE, Duncan EM, Sehayek E, Breslow JL. Novel putative SREBP and LXR target genes identified by microarray analysis in liver of cholesterol-fed mice. J Lipid Res. (2003) 44(11):2109–19. doi: 10.1194/jlr.M300203-JLR200
67. Hu D, Guo Y, Wu R, Shao T, Long J, Yu B, et al. New insight into metformin-induced cholesterol-lowering effect crosstalk between glucose and cholesterol homeostasis via ChREBP (carbohydrate-responsive element-binding protein)-mediated PCSK9 (proprotein convertase subtilisin/kexin type 9) regulation. Arterioscler Thromb Vasc Biol. (2021) 41(4):e208–e23. doi: 10.1161/atvbaha.120.315708
68. Li H, Dong B, Park SW, Lee HS, Chen W, Liu J. Hepatocyte nuclear factor 1alpha plays a critical role in PCSK9 gene transcription and regulation by the natural hypocholesterolemic compound berberine. J Biol Chem. (2009) 284(42):28885–95. doi: 10.1074/jbc.M109.052407
69. Cohen J, Pertsemlidis A, Kotowski IK, Graham R, Garcia CK, Hobbs HH. Low LDL cholesterol in individuals of African descent resulting from frequent nonsense mutations in PCSK9. Nat Genet. (2005) 37(2):161–5. doi: 10.1038/ng1509
70. Cohen JC, Boerwinkle E, Mosley TH Jr., Hobbs HH. Sequence variations in PCSK9, low LDL, and protection against coronary heart disease. N Engl J Med. (2006) 354(12):1264–72. doi: 10.1056/NEJMoa054013
Keywords: proprotein convertase subtilisin/kexin type 9 monoclonal antibodies, lipid metabolism, hyperglycemic adverse events, coronary heart disease, type 2 diabetes mellitus
Citation: Zhang R, Wang Y, Peng Y, Zhao J and Zhang Z (2023) Advanced progress of the relationship between PCSK9 monoclonal antibodies and hyperglycemic adverse events. Front. Cardiovasc. Med. 10:1117143. doi: 10.3389/fcvm.2023.1117143
Received: 6 December 2022; Accepted: 6 June 2023;
Published: 26 June 2023.
Edited by:
Yunhui Du, Capital Medical University, ChinaReviewed by:
György Paragh, University of Debrecen, HungaryHaili Sun, Capital Medical University, China
© 2023 Zhang, Wang, Peng, Zhao and Zhang. This is an open-access article distributed under the terms of the Creative Commons Attribution License (CC BY). The use, distribution or reproduction in other forums is permitted, provided the original author(s) and the copyright owner(s) are credited and that the original publication in this journal is cited, in accordance with accepted academic practice. No use, distribution or reproduction is permitted which does not comply with these terms.
*Correspondence: Zheng Zhang emhhbmdjY3VAMTYzLmNvbQ==
†These authors have contributed equally to this work
‡ORCID Ruixing Zhang orcid.org/0000-0003-2007-5803