- 1Department of Internal Medicine, University of Alabama at Birmingham, Birmingham, AL, United States
- 2Division of Cardiothoracic Anesthesia, Department of Anesthesiology and Perioperative Medicine, University of Alabama at Birmingham, Birmingham, AL, United States
- 3Division of Cardiovascular Disease, Department of Medicine, University of Alabama at Birmingham, Birmingham, AL, United States
Due to the proportionally high mortality rates associated with isolated tricuspid valve surgery, the invasive treatment of such pathology, historically, has been left largely unaddressed. Recently, there has been an appreciation for the mortality and morbidity of tricuspid valve disease, giving rise to the movement towards identifying less invasive, transcatheter approaches for treatment. Due to the technical complexity of these procedures along with the uniqueness and variability of tricuspid valve anatomy, a better appreciation of the tricuspid valve anatomy and pathology is required for pre-procedural planning. While two-dimensional echocardiography serves as the initial non-invasive modality for tricuspid valve evaluation, three-dimensional echocardiography provides a complete en face view of the tricuspid valve and surrounding structures, as well contributes further information regarding disease etiology and severity. In this review, we discuss the utility of three-dimensional echocardiography as a supplement to two-dimensional imaging to better assess tricuspid valve disease and anatomy to aide in future innovative therapies.
Introduction
Recently, the morbidity and mortality associated with tricuspid valve (TV) pathology has become more appreciated. Five-year survival is < 30% with moderate to severe tricuspid regurgitation (TR), which is associated with increased mortality independent of factors such as ejection fraction and pulmonary artery systolic pressure (1–3). Additionally, worsening of underlying TR after surgery of left sided valves has been documented (4–7). Due to the significant risk that TV disease poses, focus has shifted towards treatment of the TV disease from both surgical, and more recently, transcatheter approaches (5). Surgical TV repair has been linked to a high mortality rate (8), thus prompting the need for minimally invasive options. As many new transcatheter devices and surgical options are being brought to the market, the need for an enhanced knowledge of the TV anatomy and disease severity becomes important for success of these technically complex procedures. In this review, we discuss the role of 3-dimensional (3D) echocardiography as a supplement to 2-dimensional (2D) echocardiography in assessing TV anatomy and pathology (Figures 1–6).
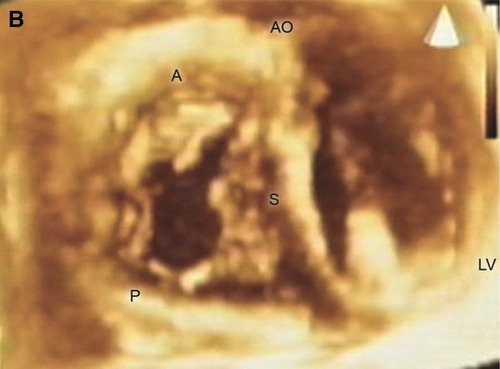
Figure 1. En face view of the TV showing all three leaflets in the open positions. A = anterior leaflet; AO= aorta; LV= left ventricle; P = posterior leaflet; S = septal leaflet. Reproduced with permission from Pothineni K et al (23).
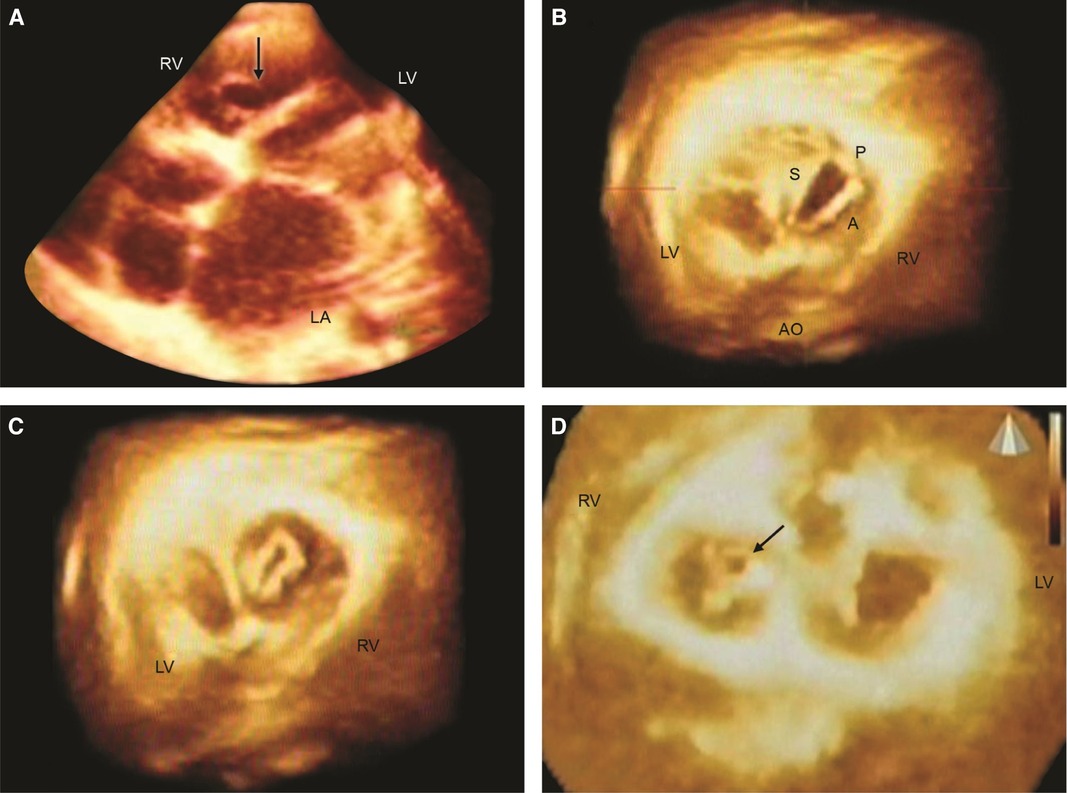
Figure 2. Live/real time three-dimensional transthoracic echocardiography in rheumatic tricuspid valve (TV) stenosis and regurgitation (TR). A: The arrow points to the TV orifice in a patient with TV stenosis. The orifice area measured 2.02 cm2 in diastole. B,C: En face views in another patient with mild TV stenosis but severe TR. The TV orifice area (B) measured 2.4 cm2 in diastole. Systolic frame (C) shows noncoaptation of TV leaflets in the same patient as (B). This measured 0.4 cm2 and resulted in severe TR as assessed by two-dimensional color Doppler. D: En face view from the ventricular aspect in a third patient with rheumatic heart disease showing systolic non coaptation (arrow) of the TV. LA = left atrium; RV = right ventricle. Other abbreviations as in previous figures. Reproduced with permission from Pothineni K et al (23).
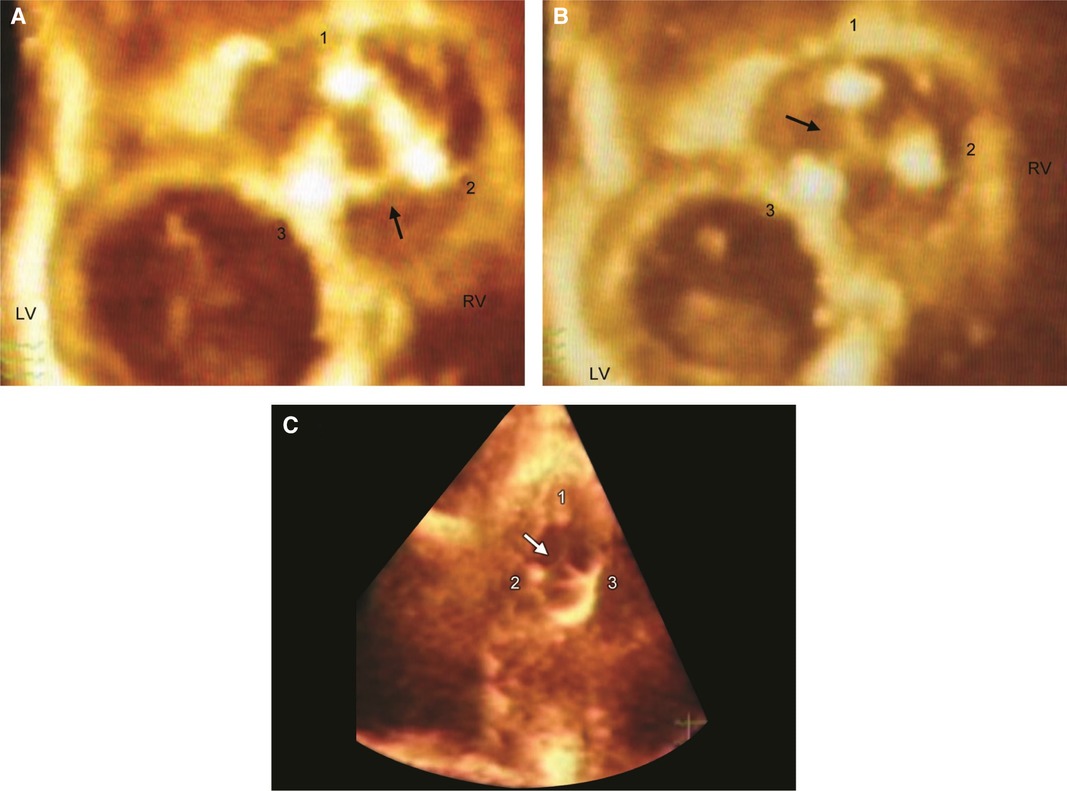
Figure 3. Live/real time three-dimensional transthoracic echocardiography of TV prosthesis. A,B: Normal bioprosthetic TV leaflets (arrow) in open (A) and closed (B) positions. Numbers 1, 2, and 3 represent the three struts of the prosthetic valve. C: Arrow shows systolic noncoaptation of bioprosthetic leaflets in another patient. Abbreviations as in previous figures. Reproduced with permission from Pothineni at al (23).
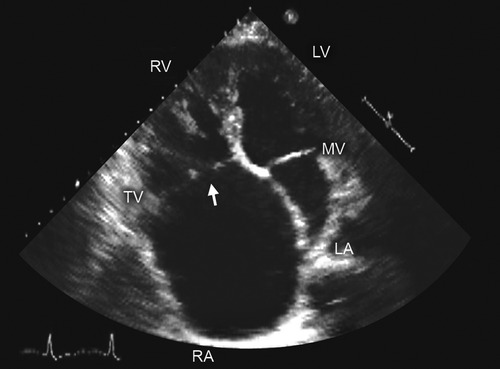
Figure 4. Two-dimensional transthoracic echocardiography. Apical 4 chamber view shows noncoaptation of tricuspid valve (TV) leaflets (arrow) due to a dilated annulus-an important consideration in procedural planning for TV disease. Abbreviations as in previous figures. Reproduced with permission from Murray, et al. (5).
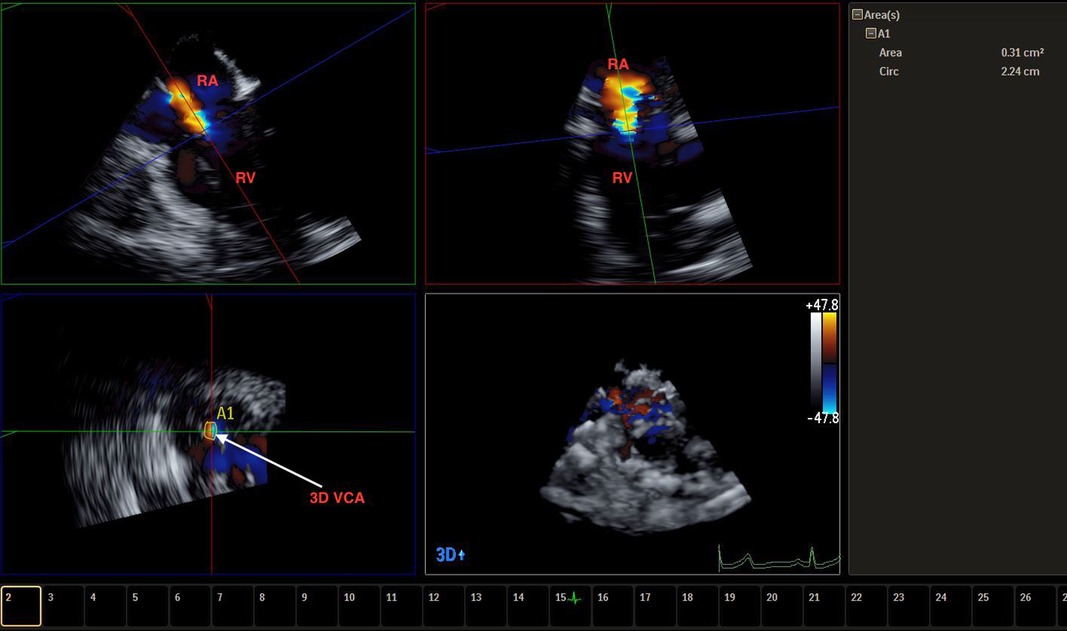
Figure 5. Live/real time transesophageal echocardiographic assessment of TR. The color Doppler data set obtained from a four chamber view was cropped to depict the VC which measured 0.41 cm2 in area (A). Abbreviations as in previous figures.
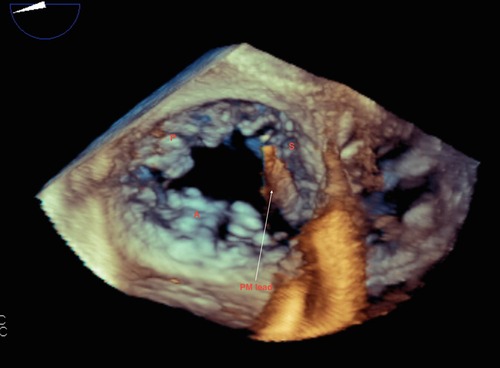
Figure 6. Live/real time transesophageal echocardiography. The arrow points to a pacemaker (PM) lead impinging on the septal leaflet (S) of the tricuspid valve. This caused restricted motion of the septal leaflet resulting in severe TR. Findings were confirmed at surgery. Abbreviations as in previous figures.
Tricuspid valve anatomy
The TV consists of three leaflets: the anterior leaflet (AL), which is largest and quadrangular, the posterior leaflet (PL), which is smallest and triangular, and the septal leaflet (SL), which is semicircular in shape with scalloped indentations (9). Hahn, et al. further assessed tricuspid valve leaflets via 2D and 3D transesophageal echocardiography (TEE) and determined that the TV has three well delineated leaflets in ∼54% of patients, four leaflets in ∼39% of patients (two posterior leaflets are most frequent), and two leaflets in ∼5% of patients (10). There are multiple, small papillary muscles in the TV apparatus and often, there are accessory chordal attachments to the right ventricle (RV) free wall and moderator band, particularly with the SL (9). Unlike the mitral valve, each TV leaflet is attached to one set of papillary muscles, increasing the risk of wide noncoaptation with annular and RV dilation (9). The TV is oval and nonplanar (with the most ovoid shape occurring at end-diastole) (11) and is 20% larger than the MV (9).
In addition to understanding the anatomy and physiology of the TV in diseased conditions, 3D echocardiography has greatly contributed to our knowledge of normal TV annulus (A) geometry (11, 12). TVA measurements obtained via 2D echocardiography have been shown to be consistently underestimated in all views compared to 3D echocardiography (13), owing to the complex geometry of the TVA that 2D imaging is unable to accurately assess. In regards to diseased patients, recent studies using 3D transthoracic echocardiography (TTE) have shown that the TVA evolves to become more planar and circular in patients with secondary TR (STR) (14). The TVA is close in proximity to the right coronary artery, coronary sinus, mitral valve, and aortic valve, all of which are important structures to consider intraprocedurally. Additionally, the TVA is angulated in relation to the superior and inferior vena cava, which can pose technical difficulties during transcatheter procedures (9). The large size of the TV, the significant variability in its shape and dimensions, and the characteristics of the valve itself create difficulty in performing percutaneous repair or replacement (5). In addition to 2D, 3D echocardiography has played a vital role in understanding TV morphology (5, 10, 14–17).
Etiology of TV disease
The etiology of TV disease can be classified into primary, referring to pathologic changes to the TV leaflets (Table 1), or secondary, referring to pathologic changes to the surrounding heart structures (Table 2) (18). Additionally, STR has been further subdivided into atrial, characterized by insufficient leaflet coverage of a dilated TVA, and ventricular, characterized by insufficient leaflet coaptation in the setting of apical displacement with leaflet tethering (19). Finally, cardiac implantable electronic device(CIED) lead-induced TR has been suggested as a third phenotype due to the nuance on whether to classify this as primary or secondary (19).
Procedural considerations for TV disease
Due to the high mortality associated with TV disease and with traditional TV surgery, innovative therapies to repair or replace the TV have been recently brought to market (5). There currently are multiple, albeit some experimental, options to perform transcatheter TV repair(TTVr) (5). Transcatheter approach for tricuspid valve repair has been shown to be beneficial in some patients with STR, but is not appropriate in patients with a TV coaptation gap > 6–8 mm, in patients with primary causes of TV disease, or in patients with tricuspid stenosis (TS), in which a surgical approach has traditionally be recommended (1). Recently, Goldberg et al. have suggested a transcatheter tricuspid valve replacement (TTVR) approach, which may address these deficiencies with TTVr (1). The two categories of TTVR include orthotopic, where the valve is implanted at the TVA, and heterotopic, in which the valve is deployed in one or both of the vena cavae in order to ameliorate the symptoms of right heart failure (1). Potential complications of TTVR include device malfunction, paravalvular leak, valve embolism or thrombus, and acute RV failure due to sudden onset RV pressure increase (1). Additionally, multiplanar reconstruction using 3D echocardiography can help guide deployment of TV edge-to-edge repair (20). These important considerations underscore the importance of accurate assessment of TV and RV anatomy and pathology prior to implementation of these approaches. As guidelines suggest a prompter TV intervention in patients with progressive RV dysfunction (21), accurately assessing the size and function of the RV with 3D imaging has been shown to be of importance (22). Post-procedurally, 3D imaging has been shown to be effective in evaluating prosthetic valves and paravalvular leaks, as it provides an en face view of the anatomy and function of all three TV prosthetic leaflets, which 2D imaging is mostly unable to accomplish (23).
Tricuspid valve echocardiography
Imaging of the TV should be performed by 2DTTE/3DTTE and 2DTEE/3DTEE, if indicated, to evaluate the valve (21, 24, 25), chamber dimensions (26), and RV ejection fraction (22). Due to the uniqueness and variability of the TV (Table 3), 2DTTE is able to visualize all three leaflets simultaneously in only 5%–10% of patients; while simultaneous visualization with 3DTTE is achievable in 85%–90% of patients (27). Additionally, the onset of 3D printing has been conceptualized to better facilitate procedural planning for tricuspid valve procedures (5).
Assessment of the TV involves a comprehensive imaging approach using 2D TTE/TEE, 3D TTE/TEE, color flow Doppler, and in some instances, cardiac magnetic resonance imaging (25). Most of these measures are qualitative or semiquantitative in nature (25). While 2DTTE remains to have a major impact on valvular assessment, the role of 3D assessment has become increasingly recognized (21, 24, 25, 28, 29). In addition to the limitations associated with 2DTTE, 3DTTE allows for evaluation of entire leaflets, including the subvalvular structures, other surrounding structures, and right heart chambers (5, 22). 3D imaging of the TVA has also been compared to cardiac computed tomography (CCT) imaging and shown to underestimate TVA parameters, but these differences were clinically negligible (11, 12), without having to undergo the extensive imaging protocols of CCT as well as reducing the risk of radiation and contrast exposure. Although technical skills are required to obtain a 3D image, many modern 2D transducers are able to acquire 3D images by using 2D planes. En face views of the TV from both the atrial and ventricular short axis can be obtained via 3D imaging, which is usually not possible with 2D imaging (5). En face views allow for better assessment of TV anatomy, anatomic relationships, and leaflet function (5). Additionally, subvalvular structures such as chordae tendinae, papillary muscles, and moderator band can be seen more clearly with 3D TTE (23). 3D TEE and 3D TTE have their distinct advantages. While 3D TEE avoids the poor acoustic windows that TTE may encounter and provides higher resolution, it can be susceptible to dropout artifacts and experience difficulty visualizing anterior structures that are adjacent to the probe such as the TV.
3DTTE offers further information in regard to the severity and etiology of TR. 3DTTE has been shown to identify structural deficits as the cause of TR (30, 31). For example, 3DTTE has been shown to be effective in evaluating the size and function of the right atrium and right ventricle and their association with TR etiology and severity (32, 33). Differentiating the etiology of STR has clinical relevance, particularly as the two have different natural histories, with atrial STR exhibiting a more favorable clinical course and response to TTVR (34, 35) Additionally, en face visualization of TV leaflets provides further clarity into the size, shape, and location of leaflet defects, including causes congenital in nature, identifying vegetations in endocarditis, localizing prolapse of individual TV leaflets, assessing sites of TV chordae rupture, characterizing TV tumors, and evaluating valvular and atrial thrombi (23, 36–39). Studying the degree of TR associated with CIED implantation has revealed mixed results, and this has been attributed to the limited role of 2D TTE in evaluating these patients, where device leads are fully visualized traversing the TVA in only 15% of patients (40, 41). For patients with CIED who have limited 2DTTE assessment, en face visualization with 3D TTE can further characterize the cause and degree of TR. In patients with STR, 3DTTE may assess the size of the non-coaptable TV leaflet area, the extent of tethering, and the degree of displacement of TV leaflets into the RV (5).
3DTTE also offers quantitative assessment of TR, which has been historically difficult to achieve with 2D imaging. One described technique to quantitatively assess TR severity has been to calculate the TV 3D vena contracta (VC) area. Obtaining the velocity time integral of the TR jet via continuous wave Doppler and multiplying it by the VC size will provide the volume of regurgitation (5). A 2D VC width ≥ 0.7 cm has been indicative of severe TR, but this only has moderate sensitivity and moderate specificity, as 2D assessment of VC assumes that the TV orifice is circular or elliptical, which has been shown to be inaccurate (42). Rather, 3D VCA ≥ 0.75 cm2 has been indicative of severe TR with a high sensitivity and high specificity (5, 42). In comparing VC assessment between 2D and 3D imaging, maximal VC diameter is often larger by 3D imaging (25, 43), and 2D imaging of VCA correlates poorly with the corresponding 3D image (42). 3D VCA correlates well with effective regurgitant orifice area (EROA), moderately well with VC diameter, and weakly with jet area/right atrial area ratio (25, 44). The effective orifice area of the TV is often derived from the 2D evaluation of proximal isovelocity surface area (PISA), but this often requires making an often incorrect assumption of the shape of the flow acceleration, which can often underestimate STR severity (45). A corrected 2D PISA method, using a correction factor obtained from referencing 3D volumetric assessment, has been proposed and shown a more accurate assessment of STR severity (45). Recently, there have been suggestions that the TR grading system span beyond that of severe to include “massive” (3D VCA 95–114 mm2) and “torrential” (3D VCA ≥ 115 mm2), citing that many patients have evidence of regurgitant jets that progress multiple grades beyond the current definition of severe, although these numerical cutoffs have not been validated (46, 47). This trend would necessitate a more comprehensive approach to identifying TR severity, in which 3DTTE and 3D TEE could play a vital role.
To capture multiple 3D structures in a dataset, volume angle often must be enlarged, except when assessing the TR VC. This causes a reduction in the frame rate, and therefore temporal resolution. Due to this phenomenon, 3D datasets should be acquired from multiple transthoracic transducer positions that best visualize the TV, with focused 3D examinations being used as a complement to 2D imaging (5, 27). An additional limitation of 3DTTE is it often overestimates leaflet thickness, which is due to blurring or amplification of artifacts (27, 48).
To obtain 3D imaging of the TV, one must begin the exam with the most optimized 2D examination of the TV, often requiring multiple acquisitions from both the left and right parasternal, apical, subcostal transducer approaches. Once visualized, the image is switched to live/real time 3D to ensure the TV is in view. Once confirmed, full-volume mode is applied, which allows focus on the TV without surrounding structures, thus acquiring an image with an increased frame rate resulting in enhanced resolution. When viewing the TV en face, the septal TV leaflet should be located at the 6 ‘clock position, although recent studies have suggested alternative approaches more anatomically or surgically appropriate (27, 49). Once relevant RV structures are inside the cropping plane, gain, compression, and magnification parameters can be adjusted for optimization of the 3D image. Cropping using the multi-slice view allows for more accurate measurements, since each plane is a 2D representation of a 3D image (23). The atrial perspective in a 3D dataset is often used to evaluate primary TR (degenerative, traumatic, etc.), while the ventricular view is often used to evaluate commissures and the regurgitant and/or stenotic orifice in patients with STR (27). In patients with poor transthoracic windows or in patients undergoing percutaneous and surgical procedures of the TV, both 2DTEE and 3DTEE can be performed.
Despite the benefits of 3D imaging, projecting a 3D image onto a 2D screen can limit accurate assessment of spatial relationships and is less accurate in representing specific tissue characteristics such as tissue calcifications and vegetations (27). 3D printing obtained from 3D imaging has been shown to be feasible, accurate, and useful in gathering further understanding of TV anatomy and physiology (50). The onset of 3D printing has allowed proceduralists to view these images as real time 3D objects, which can assist in preprocedural planning (51, 52). This can be particularly helpful in patients with challenging anatomy or for complex, innovative procedures, but is limited by the quality of the 3D image itself.
Conclusion
Morbidity and mortality associated with TV disease is no longer lost on clinicians. Due to the complex anatomy of the TV and nonplanar geometry of the TVA, assessing severity of TV disease can be difficult with 2D imaging alone. 3DTTE/TEE as a supplement to 2D imaging has been shown to provide quantitative data regarding TV pathology, as well as enhance innovative procedural interventions of the TV. This literature review has further gathered information on the technique and benefits associated with 3D echocardiography.
Author contributions
All authors contributed to the conception and design of the work, drafted the manuscript, and revised it critically, approved the final version of the manuscript, and agree to be accountable for all aspects of the works.
Conflict of interest
The authors declare that the research was conducted in the absence of any commercial or financial relationships that could be construed as a potential conflict of interest.
Publisher's note
All claims expressed in this article are solely those of the authors and do not necessarily represent those of their affiliated organizations, or those of the publisher, the editors and the reviewers. Any product that may be evaluated in this article, or claim that may be made by its manufacturer, is not guaranteed or endorsed by the publisher.
References
1. Goldberg YH, Ho E, Chau M, Latib A. Update on transcatheter tricuspid valve replacement therapies. Front Cardiovasc Med. (2021) 8:619558. doi: 10.3389/fcvm.2021.619558
2. Oguz D, Michelena H, Maalouf J, et al. Burden of tricuspid regurgitation in patients diagnosed in the community setting. JACC Cardiovasc Imaging. (2019) 12:433–42. doi: 10.1016/j.jcmg.2018.06.014
3. Nath J, Foster E, Heidenreich PA. Impact of tricuspid regurgitation on long-term survival. J Am Coll Cardiol. (2004) 43:405–9. doi: 10.1016/j.jacc.2003.09.036
4. Shiran A, Sagie A. Tricuspid regurgitation in mitral valve disease incidence, prognostic implications, mechanism, and management. J Am Coll Cardiol. (2009) 53:401–8. doi: 10.1016/j.jacc.2008.09.048
5. Harb S, Nahar T, Nanda N. Assessment of tricuspid valve by two- and three-dimensional echocardiography with special reference to percutaneous repair and prosthetic valve implantation procedures. Echocardiography. (2018) 35:1419–38. doi: 10.1111/echo.14130
6. Dreyfus GD, Corbi PJ, Chan KM, Bahrami T. Secondary tricuspid regurgitation or dilatation: which should be the criteria for surgical repair? Ann Thorac Surg. (2005) 79:127–32. doi: 10.1016/j.athoracsur.2004.06.057
7. Vazquez de Prada JA, Weyman AE, Levine RA. Determinants of functional tricuspid regurgitation in incomplete tricuspid valve closure: doppler color flow study of 109 patients. J Am Coll Cardiol. (1994) 24:446–53. doi: 10.1016/0735-1097(94)90302-6
8. Kilic A, Saha-Chaudhuri P, Rankin JS, Conte JV. Trends and outcomes of tricuspid valve surgery in North America: an analysis of more than 50,000 patients from the society of thoracic surgeons database. Ann Thorac Surg. (2013) 96:1546–52. doi: 10.1016/j.athoracsur.2013.06.031; discussion 1552.24070702
9. Marini C, Latib A, Denti P, et al. Multimodality imaging of the tricuspid valve with implication for percutaneous repair approaches. Heart. (2017) 103:1073–81. doi: 10.1136/heartjnl-2016-310939
10. Hamid N, Kitamura M, Bae R, et al. Proposal for a standard echocardiographic tricuspid valve Nomenclature. JACC Cardiovasc Imaging. (2021) 14:1299–305. doi: 10.1016/j.jcmg.2021.01.012
11. Mihalcea DJ, Guta AC, Radu N, et al. Reference ranges of tricuspid annulus geometry in healthy adults using a dedicated three-dimensional echocardiography software package. Front Cardiovasc Med. (2022) 9:1011931. doi: 10.3389/fcvm.2022.1011931
12. Jenei C, Cavalli G, Besser SA, et al. 3-Dimensional Echocardiographic analysis of the tricuspid Annulus provides new insights into tricuspid valve geometry and dynamics. JACC Cardiovasc Imaging. (2019) 12:401–12. doi: 10.1016/j.jcmg.2017.08.022
13. Veronesi F, Weinert L, Tamborini G, et al. Echocardiographic assessment of the tricuspid Annulus: the effects of the third dimension and measurement methodology. J Am Soc Echocardiogr. (2019) 32:238–47. doi: 10.1016/j.echo.2018.09.008
14. Daimon M, Tran H, Greenberg NL, et al. Three-dimensional geometry of the tricuspid annulus in healthy subjects and in patients with functional tricuspid regurgitation: a real-time, 3-dimensional echocardiographic study. Circulation. (2006) 114:I492–498. doi: 10.1161/circulationaha.105.000257
15. McGhie JS, Frowijn R, Nemes A, et al. Assessment of normal tricuspid valve anatomy in adults by real-time three-dimensional echocardiography. Int J Cardiovasc Imaging. (2007) 23:717–24. doi: 10.1007/s10554-007-9210-3
16. Bergman R, Matyal R, Gerstle J, et al. Tricuspid annular geometry: a three-dimensional transesophageal echocardiographic study. J Cardiothorac Vasc Anesth. (2013) 27:639–46. doi: 10.1053/j.jvca.2012.12.014
17. Medvedofsky D, Patel M, Ferrara P, et al. Comprehensive two-dimensional interrogation of the tricuspid valve using knowledge derived from three-dimensional echocardiography. J Am Soc Echocardiogr. (2016) 29:74–82. doi: 10.1016/j.echo.2015.08.017
18. Milojevic M, Baldus S, Bauersachs J, et al. ESC/EACTS guidelines for the management of valvular heart disease. Eur Heart J. (2022) 43:561–632. doi: 10.1093/eurheartj/ehab395
19. Muraru D, Maisano F, Zamorano JL, et al. Tricuspid regurgitation: recent advances in understanding pathophysiology, severity grading and outcome. Eur Heart J Cardiovasc Imaging. (2022) 23:913–29. doi: 10.1093/ehjci/jeac009
20. Wollborn J, Schuler A, Sheu RD, Shook DC, Nyman CB. Real-Time multiplanar reconstruction imaging using 3-dimensional transesophageal echocardiography in structural heart interventions. J Cardiothorac Vasc Anesth. (2022) 37:570–81. doi: 10.1053/j.jvca.2022.11.011
21. Carabello BA, Erwin JP 3rd, Gentile F, et al. ACC/AHA guideline for the management of patients with valvular heart disease: a report of the American college of cardiology/American heart association joint committee on clinical practice guidelines. J Am Coll Cardiol. (2021) 77:e25–e197. doi: 10.1016/j.jacc.2020.11.018
22. Guida V, Parati G, Muraru D. The added value of 3-dimensional echocardiography to understand the pathophysiology of functional tricuspid regurgitation. JACC Cardiovasc Imaging. (2021) 14:683–9. doi: 10.1016/j.jcmg.2020.04.029
23. Nanda NC, Patel V, Fan P, et al. Live/real time three-dimensional transthoracic echocardiographic assessment of tricuspid valve pathology: incremental value over the two-dimensional technique. Echocardiography. (2007) 24:541–52. doi: 10.1111/j.1540-8175.2007.00451.x
24. Hua L, Handscumacher MD, Chandrasekaran K, et al. Guidelines for the echocardiographic assessment of the right heart in adults: a report from the American society of echocardiography endorsed by the European association of echocardiography, a registered branch of the European society of cardiology, and the Canadian society of echocardiography. J Am Soc Echocardiogr. (2010) 23:685–713. doi: 10.1016/j.echo.2010.05.010; quiz 786–688.20620859
25. Enriquez-Sarano M, Foster E, Grayburn PA, et al. Recommendations for noninvasive evaluation of native valvular regurgitation: a report from the American society of echocardiography developed in collaboration with the society for cardiovascular magnetic resonance. J Am Soc Echocardiogr. (2017) 30:303–71. doi: 10.1016/j.echo.2017.01.007
26. Afilalo J, Armstrong A, Ernande L, et al. Recommendations for cardiac chamber quantification by echocardiography in adults: an update from the American society of echocardiography and the European association of cardiovascular imaging. J Am Soc Echocardiogr. (2015) 28:1–39.e14. doi: 10.1016/j.echo.2014.10.003
27. Faletra FF, Basso C, Badano LP. 3-Dimensional Echocardiography in imaging the tricuspid valve. JACC Cardiovasc Imaging. (2019) 12:500–15. doi: 10.1016/j.jcmg.2018.10.035
28. Dorer DJ, Yosefy C, Fan D, et al. Geometric determinants of functional tricuspid regurgitation: insights from 3-dimensional echocardiography. Circulation. (2006) 114:143–9. doi: 10.1161/circulationaha.106.611889
29. La Canna G, Pepi M, Dulgheru R, et al. Multi-modality imaging assessment of native valvular regurgitation: an EACVI and ESC council of valvular heart disease position paper. Eur Heart J Cardiovasc Imaging. (2022) 23:e171–232. doi: 10.1093/ehjci/jeab253
30. Reddy VK, Nanda S, Bandarupalli N, Pothineni KR, Nanda NC. Traumatic tricuspid papillary muscle and chordae rupture: emerging role of three-dimensional echocardiography. Echocardiography. (2008) 25:653–7. doi: 10.1111/j.1540-8175.2008.00701.x
31. Horton C Jr., Wanat FE, Nekkanti R, Nanda NC. Tricuspid valve fibroelastoma in an elderly patient: transesophageal echocardiographic diagnosis and differentiation from a myxoma. Am J Geriatr Cardiol. (2001) 10:55–8. doi: 10.1111/j.1076-7460.2001.90857.x
32. Ochoa-Jimenez RC, Genovese D, Veronesi F, et al. Right atrial volume is a major determinant of tricuspid annulus area in functional tricuspid regurgitation: a three-dimensional echocardiographic study. Eur Heart J Cardiovasc Imaging. (2021) 22:660–9. doi: 10.1093/ehjci/jeaa286
33. Volpato V, Caravita S, Perger E, et al. Right heart chambers geometry and function in patients with the atrial and the ventricular phenotypes of functional tricuspid regurgitation. Eur Heart J Cardiovasc Imaging. (2022) 23:930–40. doi: 10.1093/ehjci/jeab211
34. Radu N, Cascella A, Tomaselli M, et al. The atrial secondary tricuspid regurgitation is associated to more favorable outcome than the ventricular phenotype. Front Cardiovasc Med. (2022) 9:1022755. doi: 10.3389/fcvm.2022.1022755
35. Kresoja KP, Besler C, Sannino A, et al. Atrial functional tricuspid regurgitation: novel definition and impact on prognosis. Circ Cardiovasc Interv. (2022) 15:e011958. doi: 10.1161/circinterventions.122.011958
36. Nekkanti R, Nanda NC, Ahmed S, Huang WY, Pacifico AD. Transesophageal three-dimensional echocardiographic demonstration of clefts in the anterior tricuspid valve leaflet. Am J Geriatr Cardiol. (2002) 11:329–30. doi: 10.1111/j.1076-7460.2002.00886.x
37. Oz TK, Haj Assad A, Joshi D, et al. The advantages of live/real time three-dimensional transesophageal echocardiography in the assessment of tricuspid valve infective endocarditis. Echocardiography. (2014) 31:1293–309. doi: 10.1111/echo.12785
38. Oates E, Nanda NC, Nanda A, et al. Usefulness of three-dimensional echocardiography in the assessment of valvular involvement in loeffler endocarditis. Echocardiography. (2017) 34:1050–6. doi: 10.1111/echo.13575
39. Sharma GL, Mehta KJ, Öz TK. Transthoracic echocardiographic detection of pulmonary valve involvement in löeffler's endocarditis. Echocardiography. (2014) 31:83–6. doi: 10.1111/echo.12368
40. Addetia K, Harb SC, Hahn RT, Kapadia S, Lang RM. Cardiac implantable electronic device lead-induced tricuspid regurgitation. JACC Cardiovasc Imaging. (2019) 12:622–36. doi: 10.1016/j.jcmg.2018.09.028
41. Al-Mohaissen MA, Chan KL. Prevalence and mechanism of tricuspid regurgitation following implantation of endocardial leads for pacemaker or cardioverter-defibrillator. J Am Soc Echocardiogr. (2012) 25:245–52. doi: 10.1016/j.echo.2011.11.020
42. Patel V, Miller AP, Mehmood F, et al. Quantification of tricuspid regurgitation by live three-dimensional transthoracic echocardiographic measurements of vena contracta area. Echocardiography. (2006) 23:793–800. doi: 10.1111/j.1540-8175.2006.00314.x
43. Sugeng L, Weinert L, Lang RM. Real-time 3-dimensional color Doppler flow of mitral and tricuspid regurgitation: feasibility and initial quantitative comparison with 2-dimensional methods. J Am Soc Echocardiogr. (2007) 20:1050–7. doi: 10.1016/j.echo.2007.01.032
44. Chen TE, Kwon SH, Enriquez-Sarano M, Wong BF, Mankad SV. Three-dimensional color Doppler echocardiographic quantification of tricuspid regurgitation orifice area: comparison with conventional two-dimensional measures. J Am Soc Echocardiogr. (2013) 26:1143–52. doi: 10.1016/j.echo.2013.07.020
45. Gavazzoni M, Heilbron F, Radu N, et al. Impact of correcting the 2D PISA method on the quantification of functional tricuspid regurgitation severity. Eur Heart J Cardiovasc Imaging. (2022) 23:1459–70. doi: 10.1093/ehjci/jeac104
46. Hahn RT, Zamorano JL. The need for a new tricuspid regurgitation grading scheme. Eur Heart J Cardiovasc Imaging. (2017) 18:1342–3. doi: 10.1093/ehjci/jex139
47. Go YY, Dulgheru R, Lancellotti P. The conundrum of tricuspid regurgitation grading. Front Cardiovasc Med. (2018) 5(164). doi: 10.3389/fcvm.2018.00164
48. Leo LA, Moccetti T, Pandian N. Artifacts in three-dimensional transesophageal echocardiography. J Am Soc Echocardiogr. (2014) 27:453–62. doi: 10.1016/j.echo.2014.02.003
49. Latib A, Lurz P, Boekstegers P, et al. Patient selection, echocardiographic screening and treatment strategies for interventional tricuspid repair using the edge-to-edge repair technique. EuroIntervention. (2018) 14:645–53. doi: 10.4244/eij-d-17-01136
50. Dequal D, Frajhof L, Rabischoffsky A, et al. 3D Printing of normal and pathologic tricuspid valves from transthoracic 3D echocardiography data sets. Eur Heart J Cardiovasc Imaging. (2017) 18:802–8. doi: 10.1093/ehjci/jew215
51. Xu B, Elgharably H, Klatte R, et al. Pitfalls and pearls for 3-dimensional printing of the tricuspid valve in the procedural planning of percutaneous transcatheter therapies. JACC Cardiovasc Imaging. (2018) 11:1531–4. doi: 10.1016/j.jcmg.2018.05.003
Keywords: echocardiography, three-dimensional echocardiography, tricuspid valve, two-dimensional echocardiography, transcatheter tricuspid valve repair, transcatheter tricuspid valve replacement, three-dimensional printing
Citation: Jost ZT, Nooli NP, Ali AE, Jaganathan V and Nanda NC (2023) Three-dimensional echocardiography of the tricuspid valve. Front. Cardiovasc. Med. 10:1114715. doi: 10.3389/fcvm.2023.1114715
Received: 2 December 2022; Accepted: 24 February 2023;
Published: 20 March 2023.
Edited by:
Konstantinos Papadopoulos, Interbalkan Medical Center, GreeceReviewed by:
Özge Özden Tok, Memorial Bahçelievler Hospital, TürkiyeConstantinos Papadopoulos, Red Cross Hospital, Greece
Luigi P. Badano, University of Milano Bicocca, Italy
© 2023 Jost, Nooli, Ali, Jaganathan and Nanda. This is an open-access article distributed under the terms of the Creative Commons Attribution License (CC BY). The use, distribution or reproduction in other forums is permitted, provided the original author(s) and the copyright owner(s) are credited and that the original publication in this journal is cited, in accordance with accepted academic practice. No use, distribution or reproduction is permitted which does not comply with these terms.
*Correspondence: Zachary T. Jost emFjaGFyeWpvc3RAdWFibWMuZWR1 Navin C. Nanda bm5hbmRhQHVhYm1jLmVkdQ==
Specialty Section: This article was submitted to Cardiovascular Imaging, a section of the journal Frontiers in Cardiovascular Medicine