- Department of Anesthesiology, West China Hospital, Sichuan University, Chengdu, China
One treatment for acute type A aortic dissection is to replace the ascending aorta and aortic arch with a graft during circulatory arrest of the lower body, but this is associated with high mortality and morbidity. Maintaining the balance between oxygen supply and demand during circulatory arrest is the key to reducing morbidity and is the primary challenge during body perfusion. The aim of this review is to summarize current knowledge of body perfusion techniques and to predict future development of this field. We present three perfusion techniques based on deep hypothermic circulatory arrest (DHCA): DHCA alone, DHCA with selective cerebral perfusion, and DHCA with total body perfusion. DHCA was first developed to provide a clear surgical field, but it may contribute to stroke in 4%–15% of patients. Antegrade or retrograde cerebral perfusion can provide blood flow for the brain during circulatory arrest, and it is associated with much lower stroke incidence of 3%–9%. Antegrade cerebral perfusion may be better than retrograde perfusion during longer arrest. In theory, blood flow can be provided to all vital organs through total body perfusion, which can be implemented via either arterial or venous systems, or by combining retrograde inferior vena caval perfusion with antegrade cerebral perfusion. However, whether total body perfusion is better than other techniques require further investigation in large, multicenter studies. Current techniques for perfusion during circulatory arrest remain imperfect, and a technique that effectively perfuses the upper and lower body effectively during circulatory arrest is missing. Total body perfusion should be systematically compared against selective cerebral perfusion for improving outcomes after circulatory arrest.
1. Introduction
Most cases of acute type A aortic dissection (ATAAD) require surgical management. In the DeBakey classification, type I dissections originate in the ascending aorta and extend to at least the aortic arch, type II dissections involve the ascending aorta only (1). Hemiarch replacement surgery was performed when the intimal tear is localized along the ascending aorta or the lesser curvature of the transverse arch. In patients with an intimal tear localized along the greater curvature close to the supra-aortic vessels, total arch replacement surgery (TARS) was performed (2). Since its description in 1957, TARS has been performed, sometimes in combination with frozen elephant trunk (FET) implantation (3). Despite ongoing technical evolution (4), TARS remains a complex, challenging procedure. During FET implantation, a covered stent sutured to the distal end of a conventional tube graft is delivered antegrade to the distal aorta, where it is subsequently replaced. Using an FET means that only one anastomosis is required, greatly simplifying surgery in the next phase (5). The FET provides expansive radial force on the distal aorta and reduces the need for additional operations to manage false lumen, which may improve long-term survival (6). Circulatory arrest is required during TARS to maintain a clear surgical field that is important for proper antegrade placement of the FET and distal aortic anastomosis with the graft, reducing the risk of tearing when the distal aorta is cross-clamped.
The circulatory arrest technique block perfusion of the lower body and thereby cause ischemic injury, contributing to post-TARS mortality rates as high as 28% (7) as well as to rates of complications ranging from 0.8%–9% for stroke (8, 9), 17%–29% for neurological disorder (10), 6%–7% for respiratory failure (11, 12) and 19%–45% for acute kidney injury (13–15).
To attenuate ischemia during TARS, three techniques based on deep hypothermic circulatory arrest (DHCA) have been implemented. The first is DHCA alone, in which no perfusion is performed during arrest. The second is the combination of DHCA with antegrade cerebral perfusion (ACP) or retrograde cerebral perfusion (RCP), which may provide blood flow to the brain, but which still leaves the lower body at risk of ischemia. The third is the combination of DHCA with total body perfusion, which can be achieved through the arterial or venous systems, or through our recently proposed combination of ACP with retrograde inferior vena caval perfusion (RIVP) (16, 17).
Here we review these three approaches in order to guide future improvements in TARS.
2. Discussion
2.1. DHCA
DHCA involves complete circulatory arrest during anastomosis of the descending aorta and the graft. First used in the late 1950s (18), DHCA involves reducing systemic temperature to 18–20 °C. The primary goal during DHCA is to avoid cerebral ischemic injury because of the high oxygen requirements of the nervous system. Metabolism decreases by 5%–7% for each decrease of 1 °C in body temperature (19). As a result, cerebral oxygen consumption at 18 °C is only 12%–25% of the consumption at 37 °C. Hypothermia also protects the brain by promoting neuronal survival (20) and by inhibiting apoptosis (21), free radical production (22) and release of inflammatory cytokines (23) from astrocytes. In these ways, hypothermia decreases the release of excitatory neurotransmitters and protects vascular endothelial cells from ischemic injury (24).
By attenuating systemic hypoxic injury, DHCA prolongs the safe window for circulatory arrest from 5 min at 37 °C to 25 min at 18 °C, making TARS feasible (25). If DHCA alone lasts longer than 25 min, risk of neurological dysfunction increases significantly (26).
Unfortunately, neurological injuries are still prominent even during this safe window. Animal studies show that DHCA leads to elevation of S-100 protein, a marker of neurological injury (27), as well as neuronal apoptosis (28). When DHCA lasts up to 30 min, rate of stroke is 4%; 30–44 min, 7.5%; 45–59 min, more than 10%; 60–120 min or longer, as high as 14.6% (29). These rates may be even higher among patients older than 60 years (30).
DHCA leads to a lack of substrates for energy metabolism. It has been linked to substantial risk of postoperative morbidity and mortality, which can be attributed to dysregulation of cerebral blood flow (31) and coagulation, systemic inflammatory responses, requirement for massive blood transfusion (32), and requirement for long-term cardiopulmonary bypass during cooling and rewarming (33).
2.2. Selective cerebral perfusion
To attenuate cerebral ischemic injury, some cardiac centers proposed “selective cerebral perfusion” in the early 1980s, including antegrade cerebral perfusion (ACP) and retrograde cerebral perfusion (RCP).
2.2.1. RCP
RCP is perfusion of oxygenated blood into the brain via the superior vena cava. It was first reported in the early 1980s (34), and it became widely used in TARS during the 1990s. RCP sends blood in a non-physiological manner, leading to concerns about whether it provides adequate blood supply to the brain. Animal studies have reported cerebral blood flow of only 0.02 ± 0.02 ml/min/100 g (35) or 0.5 ± 0.5 ml/min/100 g (36) under RCP, much lower than the 16 ± 7.7 ml/min/100 g provided by arterial perfusion. Increasing RCP pressure increases blood flow but also risk of cerebral edema. An RCP pressure of 25 mmHg may provide optimal balance of cerebral blood flow and cerebrospinal fluid pressure during deep hypothermia (20 °C) (37).
Studies suggest that RCP can protect the brain from ischemic injury better than DHCA (Table 1). One study of 207 patients undergoing aortic arch surgery with RCP showed rates of 30-day mortality of 10% and permanent neurological deficit of 6% (41). Another study of RCP reported a transient neurological deficit rate of 19%, permanent neurological deficit rate of 9%, and in-hospital mortality of 10% when core temperature was 14–20 °C during circulatory arrest (26). The superiority of RCP has also been supported in much larger studies. A retrospective analysis of 1,193 patients indicated slightly lower stroke rate with RCP than DHCA (2.8% vs. 4.2%) (39). A meta-analysis involving 26,968 patients found that relative to DHCA, RCP was associated with significantly lower operative mortality (OR 0.57, 95% CI 0.45–0.71) and postoperative stroke (OR 0.66, 95% CI 0.54–0.82) than DHCA, regardless of whether the arrest temperature was below or above 20 °C (40).
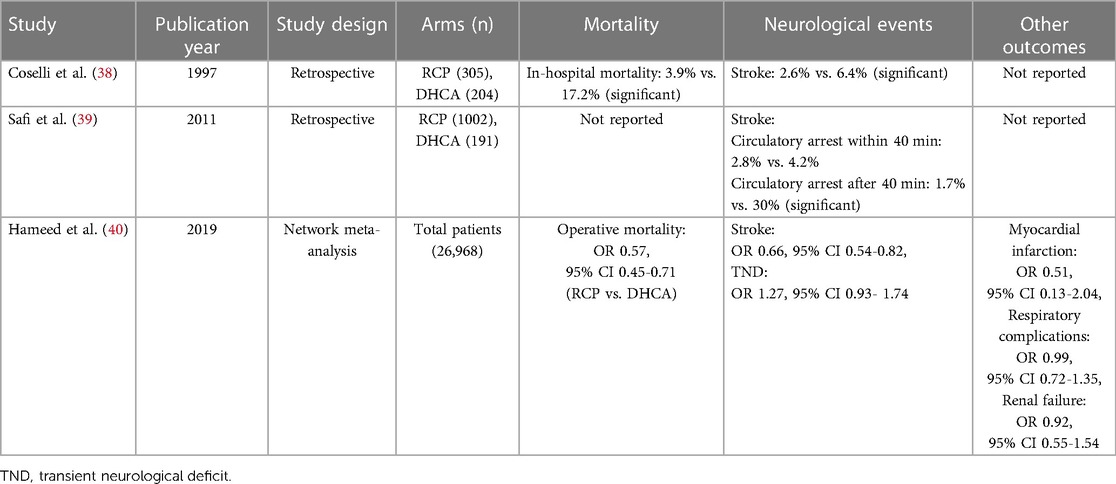
Table 1. Clinical studies comparing deep hypothermic circulatory arrest (DHCA) with retrograde cerebral perfusion (RCP).
However, RCP is not superior to DHCA in protecting vital organs other than the brain, reflected in the similar rates of the following complications for the two techniques: myocardial infarction (OR 0.51, 95% CI 0.13–2.04), respiratory complications (OR 0.99, 95% CI 0.72–1.35), and renal failure (OR 0.92, 95% CI 0.55–1.54) (40). Neither RCP nor DHCA perfuses the lower body may result in the similar rates of visceral complications.
2.2.2. ACP
Uni- or bilateral ACP can be performed during circulatory arrest by inserting one or two catheters, respectively, into one or two of the following arteries: innominate, right subclavian, axillary, left/right common carotid, or radial. Another common approach is to use three catheters to cannulate three neck vessels from the inside. Several studies have shown ACP to be superior to DHCA in terms of postoperative mortality (OR 0.63, 95% CI 0.51–0.76) and cerebral infarction (OR 0.62, 95% CI, 0.51–0.75), but similar to DHCA in terms of transient neurological deficit (OR 1.03, 95% CI 0.78- 1.35), myocardial infarction (OR 1.38, 95% CI 0.56–3.39), respiratory complications (OR 0.89, 95% CI 0.67–1.18) and renal failure (OR 0.87, 95% CI 0.56–1.35) (40).
Whether uni- or bilateral ACP is more appropriate for a given patient should be decided carefully. In unilateral ACP, oxygenated blood reaches the contralateral cerebral hemisphere via the Willis circle. Bilateral ACP is recommended for patients showing insufficient blood flow in the contralateral hemisphere based on cerebral oxygen saturation, electroencephalography, transcranial Doppler and contralateral tympanic temperature (42). Nevertheless, some studies have suggested no significant differences between uni- or bilateral ACP in rates of mortality (7.6% vs. 9.8%, P = 0.19), transient neurological deficit (6.5% vs. 9.3%, P = 0.14) or permanent neurological deficit (5.8% vs. 6.9%, P = 0.53) among patients undergoing TARS (43, 44).
Patients undergoing unilateral ACP do appear to be at higher risk of mortality than those undergoing bilateral ACP during longer circulatory arrest (45). In one study, bilateral ACP was associated with significantly better overall survival than unilateral ACP among patients receiving perfusion longer than 50 min (46).
2.2.3. ACP vs. RCP
Each of the two techniques offers advantages and disadvantages. ACP provides physiological blood flow to the brain, but it cannot remove debris or air embolisms from the perfused arteries, which may increase risk of stroke. RCP cannot perfuse the brain effectively because of venous shunts (33), so there is risk of brain edema, yet it does remove debris and air from arteries, it prevents micro-aggregation of blood cells, and it delays acidosis in the ischemic brain by removing metabolites. Therefore, RCP may be superior to ACP for patients with severe carotid stenosis, carotid dissection, or diffuse atheroma in the arch.
One animal study found similar intracranial pressure and S-100 levels in the ACP and RCP groups (29). However, the ACP showed higher blood glucose and lower glycerol levels, as well as a smaller proportion of apoptotic neurons in cerebral cortex. Numerous clinical studies have reported similar perioperative mortality rates for RCP and ACP (Table 2) (45–52). For example, one study of 8,169 patients with acute aortic dissection or ruptured aneurysm who underwent total arch replacement found no significant differences between ACP and RCP in 30-day mortality (3.2% vs. 4.0%, P = 0.247), hospital mortality (6.0% vs. 7.1%, P = 0.290), incidence of stroke (6.7% vs. 8.6%, P = 0.083), transient neurologic disorder (4.1% vs. 4.4%, P = 0.756), dialysis (3.9% vs. 3.8%, P = 0.828), or pneumonia (8% vs. 7.2%, P = 0.477) (48). While two studies suggested that RCP is associated with significantly higher rate of transient neurological deficit (OR 2.11, 95% CI 1.11–4.02) (48, 49), several studies (47, 50–52) and a meta-analysis (40) associated the two techniques with similar rates of permanent or transient neurological deficits.
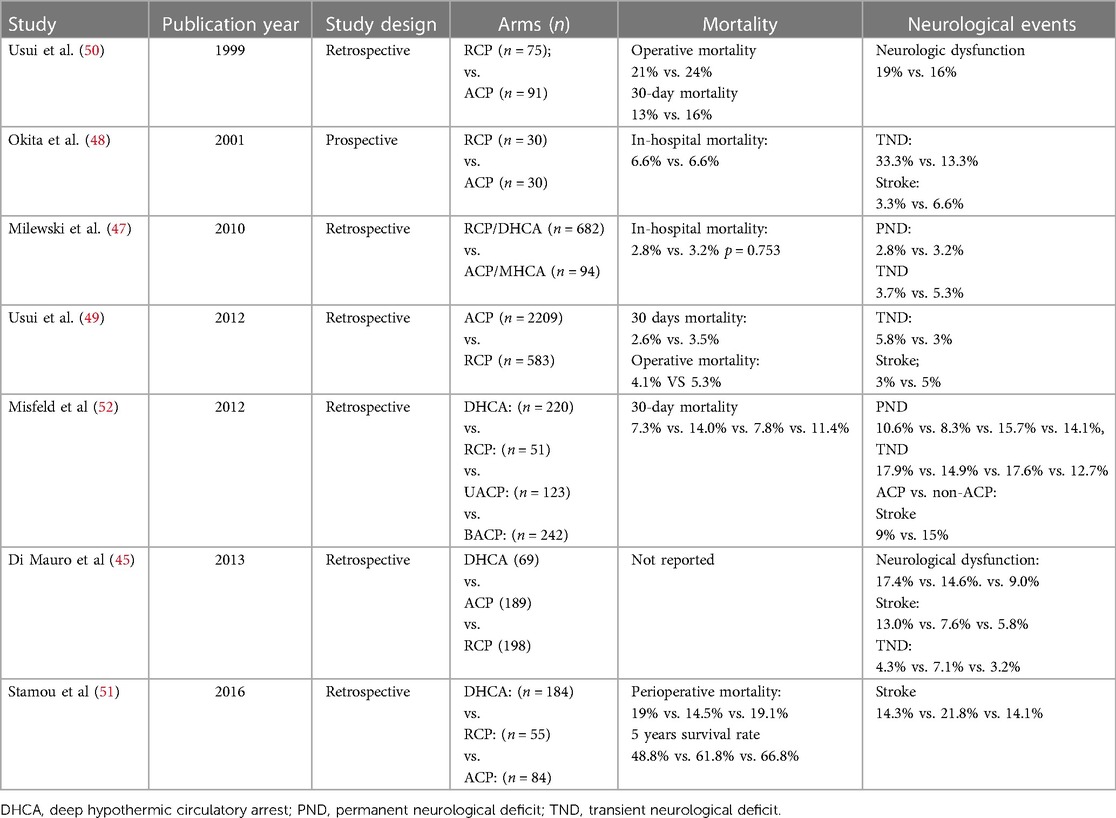
Table 2. Clinical studies comparing retrograde cerebral perfusion (RCP) with antegrade cerebral perfusion (ACP).
When combined with deep hypothermia, ACP may be safe for up to 80 min, whereas little evidence supports the safety of RCP beyond 50 min (53). Future work should explore whether these variable results reflect different durations of circulatory arrest.
2.2.4. DHCA vs. moderate hypothermic circulatory arrest (MHCA)
Whether DHCA, defined as 14.1–20 °C (54), is superior to moderate hypothermia (MHCA), defined as 20.1–28 °C, remains controversial. Since ACP can fulfill the brain's requirements for 1 L/min/m2 of blood flow at 21–25 °C or 1–1.6 L/min/m2 at 28 °C (55), it does not require low circulatory temperatures. This may lead to better outcomes because lower temperature inhibits oxygen release from hemoglobin and lowers the activity of metabolic enzymes in surrounding tissue, which can delay recovery of neurological function. On the other hand, mild hypothermia (28.1–34 °C) may increase risk of severe ischemic injury (56, 57), although ACP under such conditions does improve cerebral blood flow for a limited time.
Numerous clinical studies have compared DHCA with MHCA (Table 3), and many have concluded that the two approaches are associated with similar mortality (51, 58–70). On the other hand, at least one study associated DHCA with significantly higher mortality, both in-hospital (8% vs. 1%) and at 30 days (9% vs. 2%) (67).
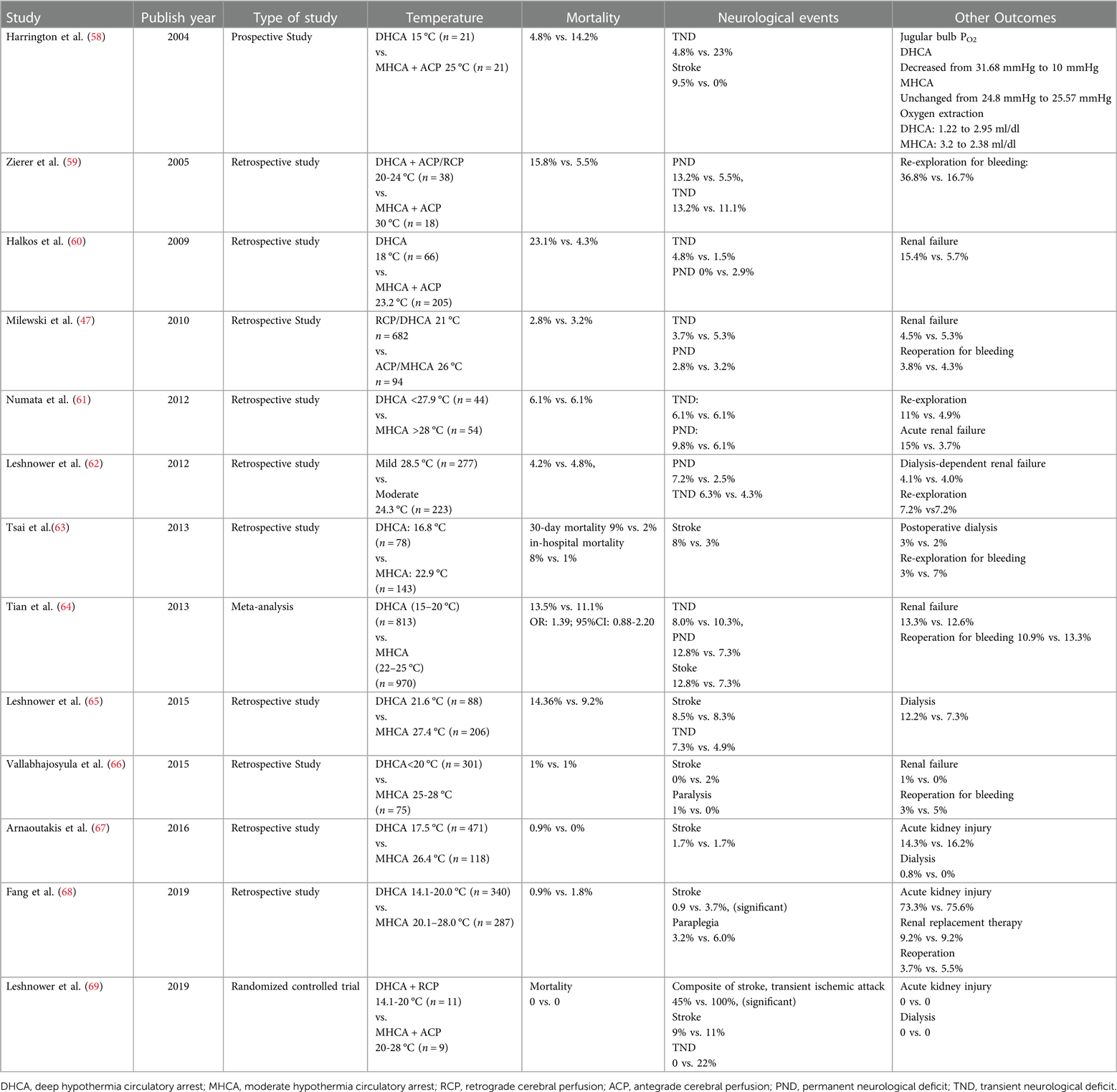
Table 3. Clinical studies comparing deep or medium hypothermic circulatory arrest in combination with selective cerebral perfusion.
While several studies have reported that DHCA and MHCA are similar in rates of transient or permanent neurological deficits (47, 60, 61), meta-analyses suggest that the rate of permanent deficit may be higher for DHCA (64, 70). One of those meta-analyses also found the rate of stroke to be higher for DHCA (12.8% vs. 7.3%, P = 0.004) (64).
DHCA may be associated with higher rates of renal failure (60, 61), although several studies have reported similar rates of acute kidney injury and postoperative dialysis between DHCA and MHCA (51, 62, 63, 65–69). One meta-analysis concluded that DHCA was associated with greater risk of postoperative dialysis (70).
Given that MHCA allows lower temporary neurologic deficit, shorter CPB (70) and mechanical ventilation (61, 70), and intensive care unit stay, the available evidence supports the use of higher core temperatures with ACP in TARS.
2.3. Summary of selective cerebral perfusion techniques
TARS has progressed from involving DHCA to a combination of milder hypothermic arrest with selective cerebral perfusion via ACP or RCP. This has reduced rates of mortality and neurological complications, yet rates of organ dysfunction in the lower body remain high as a result of inadequate perfusion. For example, rates of spinal ischemia or hemiplegic paraplegia range from 4% to 25%, while rates of dialysis range from 6% to 18% (17). These adverse events likely become more frequent with longer circulatory arrest.
3. Total body perfusion
Three methods have been reported to improve lower-body perfusion while maintaining cerebral perfusion during circulatory arrest in TARS.
3.1. Perfusion via the abdominal aorta or femoral artery
In this approach, the proximal end of the descending aorta is often occluded with a balloon during circulatory arrest, and the lower body can be perfused via the femoral artery (71) or through a hollow balloon catheter (72). One study reported good protection of kidney and liver through lower-body balloon perfusion during distal anastomosis in aortic arch repair; a flow rate of 32.9 ± 7.5 ml/kg/min provided blood pressure of 44.1 ± 12.5 mmHg in the lower extremities (72). A study of thoracic or thoracoabdominal aortic repair with balloon deployment reported rates of overall 30-day mortality of 4.75%, renal failure of 4.75%, heart failure of 9.5% and pulmonary complications of 29%, with no incidence of spinal cord neurological deficit or stroke (73). Multivariate analysis in a third study linked aortic balloon occlusion to lower risk of renal and hepatic injury, faster postoperative recovery of consciousness, and reduced need for perioperative red blood cell transfusion (74).
This method is safe for patients with undissected aneurysms, but it may increase risk in those with ATAAD. First, balloon occlusion may cause intimal injury of the descending aorta, which may be aggravated by longer occlusion (75) and atherosclerosis. Second, poor sealing of the balloon may obscure the surgical field and thus increase surgical risk. Third, perfusion through the femoral artery may exacerbate dissection if the inlet has been closed with a rigid elephant trunk but the outlet under the trunk is open.
3.2. Total body retrograde perfusion
Total body retrograde perfusion (TBRP), which involves perfusing the entire body in a retrograde manner, can be achieved, in theory, via both the superior and inferior venae cavae, because the cerebral and visceral organs lack a venous valve. However, we are aware of only two animal studies (76, 77) and one case report (78) that have reported such perfusion. A case series of two patients from our medical center described initiation on TBRP but conversion to RCP after only a few minutes because of inadequate blood flow (79).
A recent variation of TBRP, which could be called “brain-first TBRP”, is a modified form of RCP (80). It provides blood flow first to the brain, then to the lower body via communicating branches, such as the azygos vein, between the superior and inferior venae cavae. In this approach, both superior and inferior venae cavae are tethered with bands around the cannula, and the distal ends of the drainage tubes in both venae cavae are clamped. In the report of this approach, retrograde blood flow was observed in kidney and liver, inferior vena caval pressure was maintained 11 ± 3 mmHg and central venous pressure was maintained at 21 ± 2 mmHg, without fluid retention.
TBRP remains, for the moment, entirely experimental, but it may be worth examining in future studies.
3.3. Combination of retrograde inferior vena caval perfusion (RIVP) with ACP
We speculated that RIVP could provide blood flow to the low body analogously to retrograde cerebral perfusion, leading us to propose the combination of RIVP with ACP during circulatory arrest. To test this idea, we used two pumps to drive blood to the brain and viscerae separately at different perfusion pressures (Figure 1) (16). ACP was maintained at a pressure of 40-60 mmHg and flow rate of 5–12 ml/kg/min. RIVP was maintained at a venous pressure below 25 mmHg, with a blood flow of 9.12 ± 3.01 ml/kg/min. Reversed blood flow in the liver and kidney during RIVP was observed by transesophageal echocardiogram. Blood gas analysis of distal aortic drainage showed the oxygen partial pressure to be 50-80 mmHg (unpublished data), much lower than the 200-300 mmHg in RIVP blood. This indicates that oxygen in RIVP blood was absorbed by the lower body.
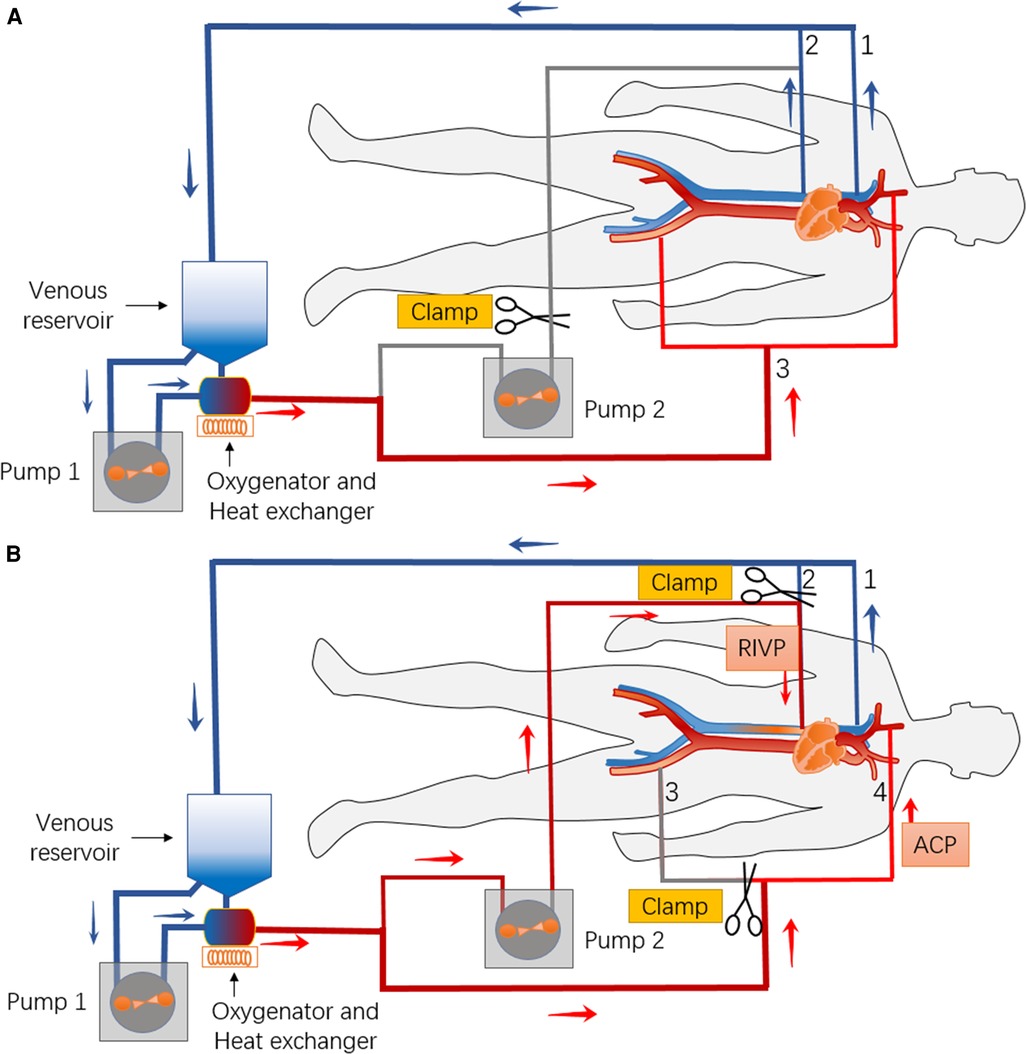
Figure 1. Schematic of the bypass circuit and its connection with the body for combination of retrograde inferior vena caval perfusion (RIVP) with antegrade cerebral perfusion (ACP). (A) Before and after combination of RIVP and ACP, venous blood from the superior vena cava (1) and inferior vena cava (2) is returned to the reservoir and driven by pump 1 to the oxygenator, then back to the body (femoral artery, aorta, innominate artery.) (3). (B) During RIVP and ACP, venous blood from the superior vena cava (1) is returned to the reservoir and driven by pump 1 to the oxygenator. Part of the oxygenated blood is routed for ACP (4), while the other part is driven by pump 2 back to the lower body via the inferior vena cava (2).
A pilot study at our medical center involving 76 TARS patients compared outcomes between those who underwent ACP alone or in combination with RIVP (81). Both groups showed similar rates of the primary aggregate outcome (42% vs. 61%, OR 0.60, 95% CI 0.21–1.62, P = 0.31), which included paraplegia, postoperative dialysis-dependent renal failure, severe liver dysfunction, gastrointestinal complications, and all-cause mortality. However, ACP + RIVP was associated with lower incidence of transient neurological deficits (26% vs. 58%, OR 0.26, 95% CI 0.10–0.67, P = 0.006), shorter intubation duration (25 h vs. 47 h, P = 0.022) and smaller consumption of blood products. Similar results were obtained from interim analysis of more than 200 patients in an ongoing randomized controlled trial at five heart centers in China (unpublished data).
Before RIVP can be widely implemented in the clinic, several questions should be addressed. The association between pressure and blood flow during RIVP under different temperatures should be investigated. The optimal temperature for circulatory arrest should be determined, and whether RIVP can provide adequate oxygen to vital organs should be verified, in particular to the gastrointestinal tract, spinal cord, liver, and kidneys.
4. Conclusion
TARS was first carried out with DHCA, which led to high mortality and morbidity. The use of selective cerebral perfusion and higher body temperatures significantly reduced these adverse outcomes, but transient or permanent neurological defects as well as dysfunction of vital organs in the low body remain frequent. Maintaining total body perfusion during circulatory arrest, such as by combining RIVP and ACP, may be even better than selective cerebral perfusion, but this approach should be tested rigorously and systematically in diverse patient populations.
Author contributions
Conception and design: JL, LD. Analysis and interpretation of the data: YC, JX, ZT. Funding acquisition: JL, LD. Writing—original draft: YC, XL. Writing—review & editing: JL, LD. All authors contributed to the article and approved the submitted version.
Funding
This work was supported by the 1.3.5 Project for Disciplines of Excellence from West China Hospital, Sichuan University under Grant 2017–120 and the Science Foundation of Sichuan Province 2022NSFSC1411.
Acknowledgments
We would like to thank the staff of West China hospital who devoted their time to this 1.3.5 project, WZ for figure design and Creaducate Consulting GmbH (Munich, Germany) for linguistic assistance.
Conflict of interest
The authors declare that the research was conducted in the absence of any commercial or financial relationships that could be construed as a potential conflict of interest.
Publisher's note
All claims expressed in this article are solely those of the authors and do not necessarily represent those of their affiliated organizations, or those of the publisher, the editors and the reviewers. Any product that may be evaluated in this article, or claim that may be made by its manufacturer, is not guaranteed or endorsed by the publisher.
References
1. Braverman AC. Acute aortic dissection: clinician update. Circulation. (2010) 122(2):184–8. doi: 10.1161/CIRCULATIONAHA.110.958975
2. Poon SS, Theologou T, Harrington D, Kuduvalli M, Oo A, Field M. Hemiarch versus total aortic arch replacement in acute type A dissection: a systematic review and meta-analysis. Ann Cardiothorac Surg. (2016) 5(3):156–73. doi: 10.21037/acs.2016.05.06
3. Leone A, Beckmann E, Martens A, Di Marco L, Pantaleo A, Reggiani LB, et al. Total aortic arch replacement with frozen elephant trunk technique: results from two European institutes. J Thorac Cardiovasc Surg. (2020) 159(4):1201–11. doi: 10.1016/j.jtcvs.2019.03.121
4. LeMaire SA, Weldon SA, Coselli JS. Total aortic arch replacement: current approach using the trifurcated graft technique. Ann Cardiothorac Surg. (2013) 2(3):347–52. doi: 10.3978/j.issn.2225-319X.2013.05.02
5. Shrestha M, Martens A, Krüger H, Maeding I, Ius F, Fleissner F, et al. Total aortic arch replacement with the elephant trunk technique: single-centre 30-year results. Eur J Cardiothorac Surg. (2014) 45(2):289–95; discussion 95–6. doi: 10.1093/ejcts/ezt359
6. Chivasso P, Mastrogiovanni G, Miele M, Bruno VD, Rosciano A, Montella AP, et al. Frozen elephant trunk technique in acute type A aortic dissection: is it for all? Medicina (Kaunas, Lithuania). (2021) 57(9):894. doi: 10.3390/medicina57090894
7. Qian H, Hu J, Du L, Xue Y, Meng W, Zhang EY. Modified hypothermic circulatory arrest for emergent repair of acute aortic dissection type a: a single-center experience. J Cardiothorac Surg. (2013) 8:125. doi: 10.1186/1749-8090-8-125
8. Okita Y, Miyata H, Motomura N, Takamoto S. A study of brain protection during total arch replacement comparing antegrade cerebral perfusion versus hypothermic circulatory arrest, with or without retrograde cerebral perfusion: analysis based on the Japan adult cardiovascular surgery database. J Thorac Cardiovasc Surg. (2015) 149(2 Suppl):S65–73. doi: 10.1016/j.jtcvs.2014.08.070
9. McKhann GM, Goldsborough MA, Borowicz LM Jr., Mellits ED, Brookmeyer R, Quaskey SA, et al. Predictors of stroke risk in coronary artery bypass patients. Ann Thorac Surg. (1997) 63(2):516–21. doi: 10.1016/S0003-4975(97)83384-X
10. Gaul C, Dietrich W, Friedrich I, Sirch J, Erbguth FJ. Neurological symptoms in type A aortic dissections. Stroke. (2007) 38(2):292–7. doi: 10.1161/01.STR.0000254594.33408.b1
11. Yokota K, Fujii T, Kimura K, Toriumi T, Sari A. Life-threatening hypoxemic respiratory failure after repair of acute type a aortic dissection: successful treatment with venoarterial extracorporeal life support using a prosthetic graft attached to the right axillary artery. Anesth Analg. (2001) 92(4):872–6. doi: 10.1097/00000539-200104000-00014
12. Morimoto N, Morimoto K, Morimoto Y, Takahashi H, Asano M, Matsumori M, et al. Sivelestat attenuates postoperative pulmonary dysfunction after total arch replacement under deep hypothermia. Eur J Cardiothorac Surg. (2008) 34(4):798–804. doi: 10.1016/j.ejcts.2008.07.010
13. Roh GU, Lee JW, Nam SB, Lee J, Choi JR, Shim YH. Incidence and risk factors of acute kidney injury after thoracic aortic surgery for acute dissection. Ann Thorac Surg. (2012) 94(3):766–71. doi: 10.1016/j.athoracsur.2012.04.057
14. Qiu Z, Chen L, Cao H, Zhang G, Xu F, Chen Q. Analysis of risk factors for acute kidney injury after ascending aortic replacement combined with open placement of triple-branched stent graft in type A aortic dissection: a new technique versus the traditional method in a single Chinese center. Med Sci Monit. (2015) 21:674–80. doi: 10.12659/MSM.892492
15. Pacini D, Pantaleo A, Di Marco L, Leone A, Barberio G, Parolari A, et al. Risk factors for acute kidney injury after surgery of the thoracic aorta using antegrade selective cerebral perfusion and moderate hypothermia. J Thorac Cardiovasc Surg. (2015) 150(1):127–33.e1. doi: 10.1016/j.jtcvs.2015.04.008
16. Lin J, Xiong J, Luo M, Tan Z, Wu Z, Guo Y, et al. Combining cerebral perfusion with retrograde Inferior vena caval perfusion for aortic arch surgery. Ann Thorac Surg. (2019) 107(1):e67–e9. doi: 10.1016/j.athoracsur.2018.08.013
17. Lin J, Tan Z, Yao H, Hu X, Zhang D, Zhao Y, et al. Retrograde Inferior vena caval perfusion for total aortic arch replacement surgery (RIVP-TARS): study protocol for a multicenter, randomized controlled trial. Trials. (2019) 20(1):232. doi: 10.1186/s13063-019-3319-2
18. Drew CE, Anderson IM. Profound hypothermia in cardiac surgery: report of three cases. Lancet (London, England). (1959) 1(7076):748–50. doi: 10.1016/S0140-6736(59)91825-2
19. Erecinska M, Thoresen M, Silver IA. Effects of hypothermia on energy metabolism in mammalian central nervous system. J Cereb Blood Flow Metab. (2003) 23(5):513–30. doi: 10.1097/01.WCB.0000066287.21705.21
20. Kokaia Z, Bengzon J, Metsis M, Kokaia M, Persson H, Lindvall O. Coexpression of neurotrophins and their receptors in neurons of the central nervous system. Proc Natl Acad Sci U S A. (1993) 90(14):6711–5. doi: 10.1073/pnas.90.14.6711
21. Ji X, Luo Y, Ling F, Stetler RA, Lan J, Cao G, et al. Mild hypothermia diminishes oxidative DNA damage and pro-death signaling events after cerebral ischemia: a mechanism for neuroprotection. Front Biosci. (2007) 12:1737–47. doi: 10.2741/2185
22. Gehrmann J, Bonnekoh P, Miyazawa T, Hossmann KA, Kreutzberg GW. Immunocytochemical study of an early microglial activation in ischemia. J Cereb Blood Flow Metab. (1992) 12(2):257–69. doi: 10.1038/jcbfm.1992.36
23. Rundgren M, Karlsson T, Nielsen N, Cronberg T, Johnsson P, Friberg H. Neuron specific enolase and S-100B as predictors of outcome after cardiac arrest and induced hypothermia. Resuscitation. (2009) 80(7):784–9. doi: 10.1016/j.resuscitation.2009.03.025
24. González-Ibarra FP, Varon J, López-Meza EG. Therapeutic hypothermia: critical review of the molecular mechanisms of action. Front Neurol. (2011) 2:4. doi: 10.3389/fneur.2011.00004
25. McCullough JN, Zhang N, Reich DL, Juvonen TS, Klein JJ, Spielvogel D, et al. Cerebral metabolic suppression during hypothermic circulatory arrest in humans. Ann Thorac Surg. (1999) 67(6):1895–9.; discussion 919–21. doi: 10.1016/S0003-4975(99)00441-5
26. Gatti G, Benussi B, Currò P, Forti G, Rauber E, Minati A, et al. The risk of neurological dysfunctions after deep hypothermic circulatory arrest with retrograde cerebral perfusion. J Stroke Cerebrovasc Dis. (2017) 26(12):3009–19. doi: 10.1016/j.jstrokecerebrovasdis.2017.07.034
27. Liang MY, Tang ZX, Chen GX, Rong J, Yao JP, Chen Z, et al. Is selective antegrade cerebral perfusion superior to retrograde cerebral perfusion for brain protection during deep hypothermic circulatory arrest? Metabolic evidence from microdialysis. Crit Care Med. (2014) 42(5):e319–28. doi: 10.1097/CCM.0000000000000220
28. Kajimoto M, Ledee DR, Olson AK, Isern NG, Robillard-Frayne I, Des Rosiers C, et al. Selective cerebral perfusion prevents abnormalities in glutamate cycling and neuronal apoptosis in a model of infant deep hypothermic circulatory arrest and reperfusion. J Cereb Blood Flow Metab. (2016) 36(11):1992–2004. doi: 10.1177/0271678X16666846
29. Gega A, Rizzo JA, Johnson MH, Tranquilli M, Farkas EA, Elefteriades JA. Straight deep hypothermic arrest: experience in 394 patients supports its effectiveness as a sole means of brain preservation. Ann Thorac Surg. (2007) 84(3):759–66; discussion 66–7. doi: 10.1016/j.athoracsur.2007.04.107
30. Ergin MA, Galla JD, Lansman s L, Quintana C, Bodian C, Griepp RB. Hypothermic circulatory arrest in operations on the thoracic aorta. Determinants of operative mortality and neurologic outcome. J Thorac Cardiovasc Surg. (1994) 107(3):788–97; discussion 97–9. doi: 10.1016/S0022-5223(94)70334-5
31. Ehrlich MP, McCullough JN, Zhang N, Weisz DJ, Juvonen T, Bodian CA, et al. Effect of hypothermia on cerebral blood flow and metabolism in the pig. Ann Thorac Surg. (2002) 73(1):191–7. doi: 10.1016/S0003-4975(01)03273-8
32. Perlman R, Callum J, Laflamme C, Tien H, Nascimento B, Beckett A, et al. A recommended early goal-directed management guideline for the prevention of hypothermia-related transfusion, morbidity, and mortality in severely injured trauma patients. Critical Care (London, England). (2016) 20(1):107. doi: 10.1186/s13054-016-1271-z
33. Martini WZ. The effects of hypothermia on fibrinogen metabolism and coagulation function in swine. Metab: Clin and Exp. (2007) 56(2):214–21. doi: 10.1016/j.metabol.2006.09.015
34. Mills NL, Ochsner JL. Massive air embolism during cardiopulmonary bypass. Causes, prevention, and management. J Thorac Cardiovasc Surg. (1980) 80(5):708–17. doi: 10.1016/S0022-5223(19)37716-5
35. Ehrlich MP, Hagl C, McCullough JN, Zhang N, Shiang H, Bodian C, et al. Retrograde cerebral perfusion provides negligible flow through brain capillaries in the pig. J Thorac Cardiovasc Surg. (2001) 122(2):331–8. doi: 10.1067/mtc.2001.115244
36. Boeckxstaens CJ, Flameng WJ. Retrograde cerebral perfusion does not perfuse the brain in nonhuman primates. Ann Thorac Surg. (1995) 60(2):319–27; discussion 27–8. doi: 10.1016/0003-4975(95)00409-E
37. Usui A, Oohara K, Liu TL, Murase M, Tanaka M, Takeuchi E, et al. Determination of optimum retrograde cerebral perfusion conditions. J Thorac Cardiovasc Surg. (1994) 107(1):300–8. doi: 10.1016/S0022-5223(94)70485-6
38. Coselli JS. Retrograde cerebral perfusion is an effective means of neural support during deep hypothermic circulatory arrest. Ann Thorac Surg. (1997) 64(3):908–12. doi: 10.1016/S0003-4975(97)00746-7
39. Safi HJ, Miller CC 3rd, Lee TY, Estrera AL. Repair of ascending and transverse aortic arch. J Thorac Cardiovasc Surg. (2011) 142(3):630–3. doi: 10.1016/j.jtcvs.2010.11.015
40. Hameed I, Rahouma M, Khan FM, Wingo M, Demetres M, Tam DY, et al. Cerebral protection strategies in aortic arch surgery: a network meta-analysis. J Thorac Cardiovasc Surg. (2019) S0022-5223(19)30483-0. doi: 10.1016/j.jtcvs.2019.02.045
41. Perreas K, Samanidis G, Dimitriou S, Kalogris P, Balanika M, Antzaka C, et al. Outcomes after ascending aorta and proximal aortic arch repair using deep hypothermic circulatory arrest with retrograde cerebral perfusion: analysis of 207 patients. Interact Cardiovasc Thorac Surg. (2012) 15(3):456–61. doi: 10.1093/icvts/ivs252
42. Tian DH, Wilson-Smith A, Koo SK, Forrest P, Kiat H, Yan TD. Unilateral versus bilateral antegrade cerebral perfusion: a meta-analysis of comparative studies. Heart Lung Circ. (2019) 28(6):844–9. doi: 10.1016/j.hlc.2019.01.010
43. Urbanski PP, Lenos A, Blume JC, Ziegler V, Griewing B, Schmitt R, et al. Does anatomical completeness of the circle of willis correlate with sufficient cross-perfusion during unilateral cerebral perfusion? Eur J Cardiothorac Surg. (2008) 33(3):402–8. doi: 10.1016/j.ejcts.2007.12.021
44. Angeloni E, Melina G, Refice SK, Roscitano A, Capuano F, Comito C, et al. Unilateral versus bilateral antegrade cerebral protection during aortic surgery: an updated meta-analysis. Ann Thorac Surg. (2015) 99(6):2024–31. doi: 10.1016/j.athoracsur.2015.01.070
45. Di Mauro M, Iacò AL, Di Lorenzo C, Gagliardi M, Varone E, Al Amri H, et al. Cold reperfusion before rewarming reduces neurological events after deep hypothermic circulatory arrest. Eur J Cardiothorac Surg. (2013) 43(1):168–73. doi: 10.1093/ejcts/ezs281
46. Angleitner P, Stelzmueller ME, Mahr S, Kaider A, Laufer G, Ehrlich M. Bilateral or unilateral antegrade cerebral perfusion during surgery for acute type A dissection. J Thorac Cardiovasc Surg. (2020) 159(6):2159–67.e2. doi: 10.1016/j.jtcvs.2019.06.057
47. Milewski RK, Pacini D, Moser GW, Moeller P, Cowie D, Szeto WY, et al. Retrograde and antegrade cerebral perfusion: results in short elective arch reconstructive times. Ann Thorac Surg. (2010) 89(5):1448–57. doi: 10.1016/j.athoracsur.2010.01.056
48. Okita Y, Minatoya K, Tagusari O, Ando M, Nagatsuka K, Kitamura S. Prospective comparative study of brain protection in total aortic arch replacement: deep hypothermic circulatory arrest with retrograde cerebral perfusion or selective antegrade cerebral perfusion. Ann Thorac Surg. (2001) 72(1):72–9. doi: 10.1016/S0003-4975(01)02671-6
49. Usui A, Miyata H, Ueda Y, Motomura N, Takamoto S. Risk-adjusted and case-matched comparative study between antegrade and retrograde cerebral perfusion during aortic arch surgery: based on the Japan adult cardiovascular surgery database: the Japan cardiovascular surgery database organization. Gen Thorac Cardiovasc Surg. (2012) 60(3):132–9. doi: 10.1007/s11748-011-0857-2
50. Usui A, Yasuura K, Watanabe T, Maseki T. Comparative clinical study between retrograde cerebral perfusion and selective cerebral perfusion in surgery for acute type A aortic dissection. Eur J Cardiothorac Surg. (1999) 15(5):571–8. doi: 10.1016/S1010-7940(99)00096-2
51. Stamou SC, Rausch LA, Kouchoukos NT, Lobdell KW, Khabbaz K, Murphy E, et al. Comparison between antegrade and retrograde cerebral perfusion or profound hypothermia as brain protection strategies during repair of type A aortic dissection. Ann Cardiothorac Surg. (2016) 5(4):328–35. doi: 10.21037/acs.2016.04.02
52. Misfeld M, Leontyev S, Borger MA, Gindensperger O, Lehmann S, Legare JF, et al. What is the best strategy for brain protection in patients undergoing aortic arch surgery? A single center experience of 636 patients. Ann Thorac Surg. (2012) 93(5):1502–8. doi: 10.1016/j.athoracsur.2012.01.106
53. Apostolakis E, Shuhaiber JH. Antegrade or retrograde cerebral perfusion as an adjunct during hypothermic circulatory arrest for aortic arch surgery. Expert Rev Cardiovasc Ther. (2007) 5(6):1147–61. doi: 10.1586/14779072.5.6.1147
54. Yan TD, Bannon PG, Bavaria J, Coselli JS, Elefteriades JA, Griepp RB, et al. Consensus on hypothermia in aortic arch surgery. Ann Cardiothorac Surg. (2013) 2(2):163–8. doi: 10.3978/j.issn.2225-319X.2013.03.03
55. Rebeyka IM, Coles JG, Wilson GJ, Watanabe T, Taylor MJ, Adler SF, et al. The effect of low-flow cardiopulmonary bypass on cerebral function: an experimental and clinical study. Ann Thorac Surg. (1987) 43(4):391–6. doi: 10.1016/S0003-4975(10)62812-3
56. Khaladj N, Peterss S, Oetjen P, von Wasielewski R, Hauschild G, Karck M, et al. Hypothermic circulatory arrest with moderate, deep or profound hypothermic selective antegrade cerebral perfusion: which temperature provides best brain protection? Eur J Cardiothorac Surg. (2006) 30(3):492–8. doi: 10.1016/j.ejcts.2006.05.031
57. Strauch JT, Haldenwang PL, Müllem K, Schmalz M, Liakopoulos O, Christ H, et al. Temperature dependence of cerebral blood flow for isolated regions of the brain during selective cerebral perfusion in pigs. Ann Thorac Surg. (2009) 88(5):1506–13. doi: 10.1016/j.athoracsur.2009.07.013
58. Harrington DK, Walker AS, Kaukuntla H, Bracewell RM, Clutton-Brock TH, Faroqui M, et al. Selective antegrade cerebral perfusion attenuates brain metabolic deficit in aortic arch surgery: a prospective randomized trial. Circulation. (2004) 110(11 Suppl 1):Ii231–6. doi: 10.1161/01.CIR.0000138945.78346.9c
59. Zierer A, Aybek T, Risteski P, Dogan S, Wimmer-Greinecker G, Moritz A. Moderate hypothermia (30 degrees C) for surgery of acute type A aortic dissection. Thorac Cardiovasc Surg. (2005) 53(2):74–9. doi: 10.1055/s-2004-830458
60. Halkos ME, Kerendi F, Myung R, Kilgo P, Puskas JD, Chen EP. Selective antegrade cerebral perfusion via right axillary artery cannulation reduces morbidity and mortality after proximal aortic surgery. J Thorac Cardiovasc Surg. (2009) 138(5):1081–9. doi: 10.1016/j.jtcvs.2009.07.045
61. Numata S, Tsutsumi Y, Monta O, Yamazaki S, Seo H, Sugita R, et al. Aortic arch repair with antegrade selective cerebral perfusion using mild to moderate hypothermia of more than 28 °C. Ann Thorac Surg. (2012) 94(1):90–5; discussion 5–6. doi: 10.1016/j.athoracsur.2012.03.055
62. Leshnower BG, Myung RJ, Thourani VH, Halkos ME, Kilgo PD, Puskas JD, et al. Hemiarch replacement at 28 °C: an analysis of mild and moderate hypothermia in 500 patients. Ann Thorac Surg. (2012) 93(6):1910–5; discussion 5–6. doi: 10.1016/j.athoracsur.2012.02.069
63. Tsai JY, Pan W, Lemaire SA, Pisklak P, Lee VV, Bracey AW, et al. Moderate hypothermia during aortic arch surgery is associated with reduced risk of early mortality. J Thorac Cardiovasc Surg. (2013) 146(3):662–7. doi: 10.1016/j.jtcvs.2013.03.004
64. Tian DH, Wan B, Bannon PG, Misfeld M, LeMaire SA, Kazui T, et al. A meta-analysis of deep hypothermic circulatory arrest versus moderate hypothermic circulatory arrest with selective antegrade cerebral perfusion. Ann Cardiothorac Surg. (2013) 2(2):148–58. doi: 10.3978/j.issn.2225-319X.2013.03.13
65. Leshnower BG, Thourani VH, Halkos ME, Sarin EL, Keeling WB, Lamias MJ, et al. Moderate versus deep hypothermia with unilateral selective antegrade cerebral perfusion for acute type A dissection. Ann Thorac Surg. (2015) 100(5):1563–8; discussion 8–9. doi: 10.1016/j.athoracsur.2015.05.032
66. Vallabhajosyula P, Jassar AS, Menon RS, Komlo C, Gutsche J, Desai ND, et al. Moderate versus deep hypothermic circulatory arrest for elective aortic transverse hemiarch reconstruction. Ann Thorac Surg. (2015) 99(5):1511–7. doi: 10.1016/j.athoracsur.2014.12.067
67. Arnaoutakis GJ, Vallabhajosyula P, Bavaria JE, Sultan I, Siki M, Naidu S, et al. The impact of deep versus moderate hypothermia on postoperative kidney function after elective aortic hemiarch repair. Ann Thorac Surg. (2016) 102(4):1313–21. doi: 10.1016/j.athoracsur.2016.04.007
68. Fang Z, Wang G, Liu Q, Zhou H, Zhou S, Lei G, et al. Moderate and deep hypothermic circulatory arrest has a comparable effect on acute kidney injury after total arch replacement with frozen elephant trunk procedure in type A aortic dissection. Interact Cardiovasc Thorac Surg. (2019) 29(1):130–6. doi: 10.1093/icvts/ivz092
69. Leshnower BG, Rangaraju S, Allen JW, Stringer AY, Gleason TG, Chen EP. Deep hypothermia with retrograde cerebral perfusion versus moderate hypothermia with antegrade cerebral perfusion for arch surgery. Ann Thorac Surg. (2019) 107(4):1104–10. doi: 10.1016/j.athoracsur.2018.10.008
70. Tian DH, Weller J, Hasmat S, Preventza O, Forrest P, Kiat H, et al. Temperature selection in antegrade cerebral perfusion for aortic arch surgery: a meta-analysis. Ann Thorac Surg. (2019) 108(1):283–91. doi: 10.1016/j.athoracsur.2018.12.029
71. Abe T, Usui A. The cannulation strategy in surgery for acute type A dissection. Gen Thorac Cardiovasc Surg. (2017) 65(1):1–9. doi: 10.1007/s11748-016-0711-7
72. Takagi H, Mori Y, Iwata H, Umeda Y, Matsuno Y, Hirose H. Aortic balloon occlusion catheter with perfusion lumen for protection of lower body during distal anastomosis in aortic arch repair. J Thorac Cardiovasc Surg. (2002) 123(5):1006–8. doi: 10.1067/mtc.2002.122208
73. Watson DR, Flesher T. Combined distal aortic perfusion and balloon occlusion to facilitate the repair of complex thoracic and thoracoabdominal aortic aneurysms. Eur J Cardiothorac Surg. (2010) 37(6):1474–6. doi: 10.1016/j.ejcts.2010.01.011
74. Li Y, Guo H, Wang L, Liang S, Sun X. Application of aortic balloon occlusion in total aortic arch replacement with frozen elephant trunk on clinical endpoints for aortic dissection patients. Ann Thorac Cardiovasc Surg. (2020) 26(6):332–41. doi: 10.5761/atcs.oa.19-00288
75. Margovsky AI, Lord RS, Chambers AJ. The effect of arterial clamp duration on endothelial injury: an experimental study. Aust N Z J Surg. (1997) 67(7):448–51. doi: 10.1111/j.1445-2197.1997.tb02012.x
76. Rao PV, Stahl RF, Soller BR, Shortt KG, Hsi C, Cotter KJ, et al. Retrograde abdominal visceral perfusion: is it beneficial? Ann Thorac Surg. (1995) 60(6):1704–8. doi: 10.1016/0003-4975(95)00735-0
77. Oohara K, Usui A, Tanaka M, Abe T, Murase M. Determination of organ blood flows during retrograde inferior vena caval perfusion. Ann Thorac Surg. (1994) 58(1):139–45. doi: 10.1016/0003-4975(94)91088-X
78. Yasuura K, Ogawa Y, Okamoto H, Asakura T, Hoshino M, Sawazaki M, et al. Clinical application of total body retrograde perfusion to operation for aortic dissection. Ann Thorac Surg. (1992) 53(4):655–8. doi: 10.1016/0003-4975(92)90328-2
79. Xiong J, Tan Z, Liu X, Yu X, Lin J, Du L. Total body retrograde perfusion during hypothermic circulatory arrest is unsafe. Perfusion. (2020) 35(7):707–9. doi: 10.1177/0267659120906959
80. Yu X, Lin J, Xiong J, Liu X, Tang Z, Du L. Perfusion of brain and viscera using modified retrograde cerebral perfusion for aortic arch surgery. Perfusion. (2022):2676591221092154. doi: 10.1177/02676591221092154
Keywords: type a aortic dissection (TAAD), aortic arch surgery, cardiopulmonary bypass (CBP), deep hypothermic circulatory arrest, perfusion technique
Citation: Cui Y, Liu X, Xiong J, Tan Z, Du L and Lin J (2023) Cardiopulmonary bypass for total aortic arch replacement surgery: A review of three techniques. Front. Cardiovasc. Med. 10:1109401. doi: 10.3389/fcvm.2023.1109401
Received: 27 November 2022; Accepted: 9 March 2023;
Published: 30 March 2023.
Edited by:
Morgan Salmon, University of Michigan, United StatesReviewed by:
Nicolas Henry Pope, Medical University of South Carolina, United StatesTim Kaufeld, Hannover Medical School, Germany
© 2023 Cui, Liu, Xiong, Tan, Du and Lin. This is an open-access article distributed under the terms of the Creative Commons Attribution License (CC BY). The use, distribution or reproduction in other forums is permitted, provided the original author(s) and the copyright owner(s) are credited and that the original publication in this journal is cited, in accordance with accepted academic practice. No use, distribution or reproduction is permitted which does not comply with these terms.
*Correspondence: Jing Lin linjing@wchscu.cn
Specialty Section: This article was submitted to Aortic Surgery and Endovascular Repair, a section of the journal Frontiers in Cardiovascular Medicine