- 1Center for Preclinical Cardiovascular Research, The Texas Heart Institute, Houston, TX, United States
- 2Center for Clinical Research, The Texas Heart Institute, Houston, TX, United States
Heart failure with preserved ejection fraction (HFpEF) is characterized by a complex, heterogeneous spectrum of pathologic features combined with average left ventricular volume and diastolic dysfunction. HFpEF is a significant public health problem associated with high morbidity and mortality rates. Currently, effective treatments for HFpEF represent the greatest unmet need in cardiovascular medicine. A lack of an efficient preclinical model has hampered the development of new devices and medications for HFpEF. Because large animal models have similar physiologic traits as humans and appropriate organ sizes, they are the best option for limiting practical constraints. HFpEF is a highly integrated, multiorgan, systemic disorder requiring a multipronged investigative approach. Here, we review the large animal models of HFpEF reported to date and describe the methods that have been used to create HFpEF, including surgery-induced pressure overloading, medicine-induced pressure overloading, and diet-induced metabolic syndrome. In addition, for the first time to our knowledge, we use two established clinical HFpEF algorithms (HFA-PEFF and H2FPEF scores) to evaluate the currently available large animal models. We also discuss new technologies, such as continuous remote pressure monitors and inflatable aortic cuffs, as well as how the models could be improved. Based on current progress and our own experience, we believe an efficient large animal model of HFpEF should simultaneously encompass multiple pathophysiologic factors, along with multiorgan dysfunction. This could be fully evaluated through available methods (imaging, blood work). Although many models have been studied, only a few studies completely meet clinical score standards. Therefore, it is critical to address the deficiencies of each model and incorporate novel techniques to establish a more reliable model, which will help facilitate the understanding of HFpEF mechanisms and the development of a treatment.
1. Introduction
Heart failure (HF) is a disease in which the heart loses its ability to provide sufficient forward output to meet the perfusion and oxygenation requirements of tissues while maintaining normal filling pressure (1). Heart failure with preserved ejection fraction (HFpEF) is characterized by normal left ventricular ejection fraction (LVEF, mostly defined as LVEF > 50%) and abnormal diastolic function, often with LV concentric remodeling or hypertrophy, but sometimes with normal ventricular geometry and LV volume (2–5). Approximately 50% of HF cases are HFpEF, which is associated with high morbidity and mortality rates (6). Although progress has been made recently using the SGLT-2 inhibitor to treat HFpEF (7, 8), overall, therapy for patients with HFpEF and their prognosis remain a challenge. This is in contrast to the latest breakthrough advances in treating HF with reduced ejection fraction (HFrEF) (9). Importantly, developing new therapies for HFpEF has been slow in part because of the absence of a reliable animal model (10). In this review, we highlight the progress to date in identifying an optimal large animal model for preclinical studies of HFpEF treatments. In addition, we discuss some promising options for the future.
2. Current understanding of the pathophysiology of HFpEF
Accurately understanding the pathophysiology of a disease is essential for developing an animal model. To date, the precise mechanism for the pathophysiology of HFpEF is incompletely understood. Unlike HFrEF, which largely results from ischemic heart disease or structural heart disease and is therefore easily translated into an animal model, HFpEF is a systemic condition with both cardiac and extra-cardiac features (9). Clinical risk factors for HFpEF include aging, obesity, metabolic syndrome, hypertension, sedentary state, coronary disease, and kidney disease, which all cause widespread tissue and cell injury through different mechanisms, such as systemic inflammation, tissue fibrosis, myocardial ischemia, myocyte hypertrophy, and abnormal energetics. These mechanisms further remodel LV structure and decrease LV function and hemodynamic status, finally leading to secondary organ dysfunction presenting with clinical symptoms (9, 11–15) (Figure 1).
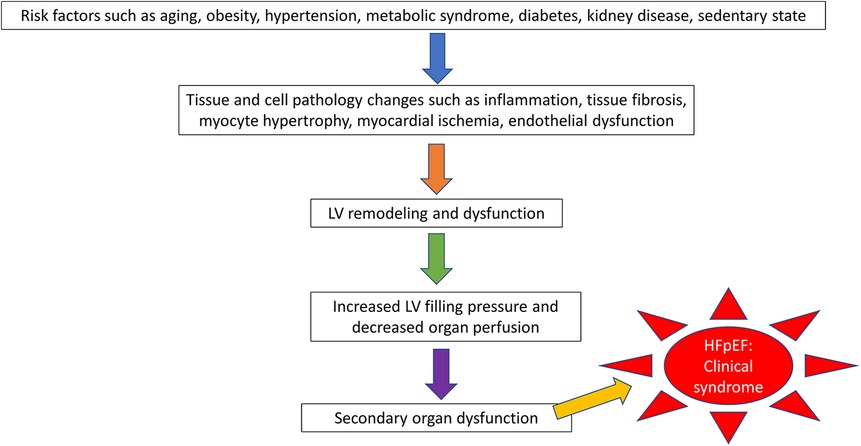
Figure 1. The pathophysiologic progression of heart failure with preserved ejection fraction (hFpEF).
In addition, recent data have shown an important role for the peripheral vascular system in HFpEF. Patients with HFpEF have abnormalities in the systemic vasculature, endothelium, adipocytes, and skeletal muscle (16, 17). Another important concept in the pathophysiology of HFpEF is the abnormal reserve (18). Even when baseline or resting function appears normal, cardiac, vascular, and peripheral reserve for coping with stressors is significantly diminished. Because HFpEF pathophysiology research is evolving quickly, this poses additional challenges to developing animal models that accurately represent human HFpEF.
3. Large animal models of HFpEF
Animal models are widely used to investigate the pathophysiology of HFpEF and to ultimately develop new treatments (19, 20). Because of the heterogeneous nature of HFpEF, designing an animal model in which all facets of HFpEF features are represented is difficult. However, some common features of HFpEF pathophysiology are less variable, such as LV diastolic function change (21), LV hypertrophy (22), and elevated natriuretic peptides (23). Several rodent models of HFpEF with diastolic dysfunction have contributed to our understanding of HFpEF mechanisms (24), but they are limited in that rodent hearts are not of comparable size, structure, or function to the hearts of humans. For device-based therapies in particular, large animal models are the only option for in vivo testing and are considered the last preclinical step before testing in humans. Therefore, large animal models of HFpEF are needed that can simulate HFpEF in humans. Currently, several types of large animal models of HFpEF are available that we will discuss here. To apply objective standards for evaluating the modeling of this complex clinical syndrome in large animals, we used clinical scores, as previously done in mice (24).
4. Clinical scores
Two score systems have recently been used to diagnose HFpEF in patients: HFA-PEFF and H2FPEF (2, 25, 26) (Figure 2). Both HFpEF scores have been validated in various patient cohorts and community studies. Both HFpEF scores have been shown to categorize patients well, especially patients with intermediate and high scores (28–30). Despite some disadvantages of these score systems, such as an overly complicated diagnostic process (31) and misclassification in lower-score patients (32), they have been shown to have prognostic utility, suggesting that they capture key pathophysiologic components that determine outcomes in patients with HFpEF (33, 34).
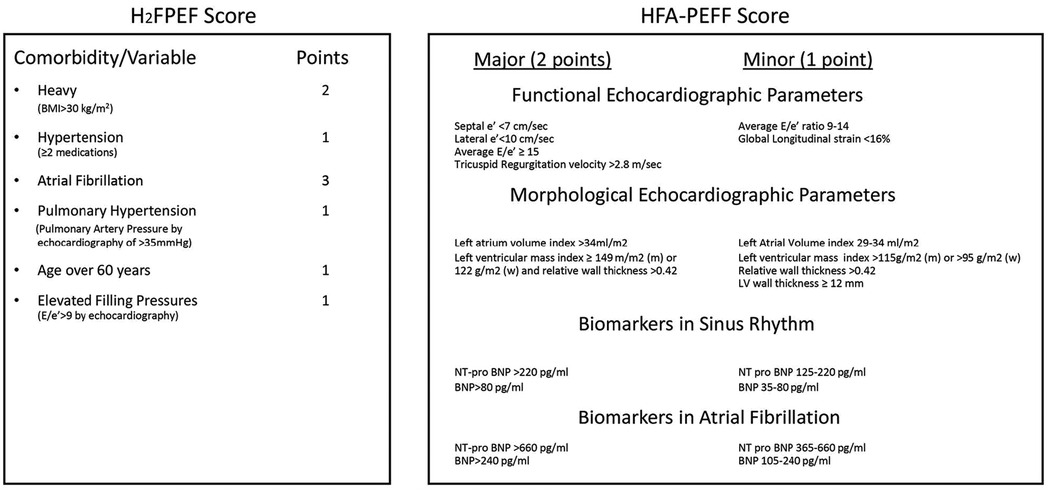
Figure 2. Illustration of the HFA-PEFF and H2FPEF score systems. From (27).
4.1. Clinical diagnostic procedures
As a clinical syndrome, HFpEF is suspected when patients have symptoms or signs of HF with all three of the following features (5, 35) (Figure 3): 1) one or more symptoms of HF, such as dyspnea or fatigue, with or without physical signs of HF; 2) a LVEF ≥50%; and 3) no apparent cause of HF symptoms other than HFpEF. For patients who meet these criteria, it is suggested that the score systems be used for further diagnosis. Details of both score systems are provided in Figure 2. The probability of HFpEF is high when the HFA-PEFF score is 5 or 6, or the H2FPEF score is between 6 and 9. Both scores rely heavily on echocardiography measurements, especially the HFA-PEFF, which provides a direct reflection that cardiac factors, such as LV diastolic dysfunction, dominate the pathologic development of HFpEF. For the H2FPEF, multiple extra-cardiac risk factors are introduced, such as body mass and age.
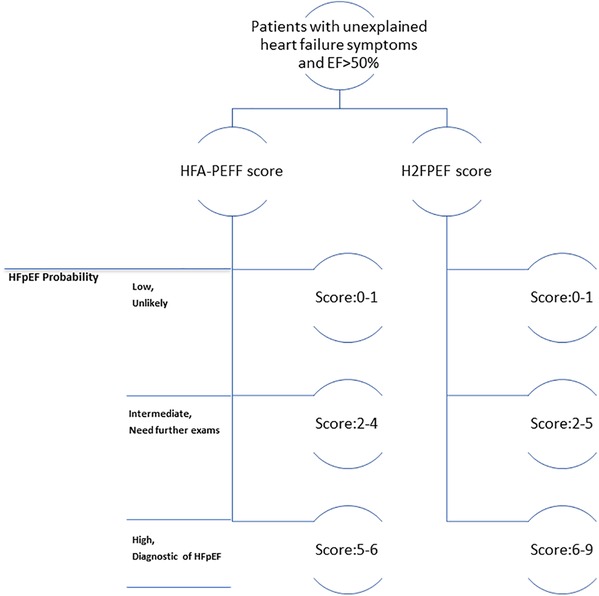
Figure 3. Clinical algorithm flowchart for diagnosing heart failure with preserved ejection fraction (hFpEF).
4.2. Pretest of symptoms and signs of HF
LVEF should be maintained above 50%, which can be measured using transthoracic echocardiography, along with LV dimension and volume. However, because animals cannot verbalize, detecting symptoms in animals is not straightforward. As an alternative, we can use surrogate measurements that provide evidence of HF, such as blood natriuretic peptide level (also included in the HFA-PEFF score) and results of a stress or exercise test. Reduced exercise reserve caused by weakness or fatigue is a typical feature of HFpEF. If a model does not include any of these, it should not be regarded as a complete preclinical model.
4.3. Using the HFA-PEFF score
The HFA-PEFF score includes the following echocardiography measurements for LV function and morphology: LV wall thickness, LV end diastolic diameters, left atrial volume index, LV mass index, mitral inflow (E wave), tissue Doppler septal and lateral wall mitral velocity (e'), peak tricuspid regurgitation velocity (if applicable), and global longitudinal strain. In large animal studies, these parameters can be recorded by using transthoracic echocardiography and should be included for each animal. Therefore, an experienced echocardiographer is required.
4.4. Using the H2FPEF score
Similar to the HFA-PEFF score, the H2FPEF score includes LV diastolic function analysis, as well as the following extracardiac factors.
Obesity. Body weight can be easily measured for a large animal every time the animal is sedated for an echocardiography procedure.
Hypertension. In large animals, monitoring blood pressure without invasive procedures can be difficult. Recently, with the development of telemetry technology, some implantable devices have become available for use in large animals. For example, the implantable easyTel + (EMKA Technologies, Sterling, VA) device (36) can be used to continuously monitor blood pressure in large animals for up to 60 days.
Age. Most large animals are enrolled in studies at a young age. For example, a pig will be considered “aging” when it reaches 13 to 15 years (37). Pigs this age are difficult to obtain from vendors, and keeping large animals until they reach senior age poses a huge financial burden. Thus, age is rarely considered in large animal studies.
The H2FPEF score also includes echocardiography parameters, such as pulmonary artery systolic pressure, Doppler mitral inflow (E wave), and tissue Doppler mitral velocity (e'), which should be acquired by using transthoracic echocardiography. For recording atrial fibrillation, a telemetry device for pigs is recommended that can be implanted into the pig's body and provide continuous electrocardiogram (EKG) monitoring (I-III, avL, avF, avR) (38). For cows and sheep that can be restrained in the stanchion, a regular EKG monitor can be used.
4.5. Gold standard
A major cardiac pathophysiologic change in patients with HFpEF is diastolic dysfunction. Therefore, hemodynamic assessment is the gold standard and may be an advantage for the verification of a model if included in a study. In large animals, hemodynamic measurements, including LV PV-loop, dp/dt, or tau index (39), can be performed by using catheters. In addition, catheters can be used to accurately evaluate pulmonary hypertension. Recently, researchers have used telemetry devices to monitor LV pressure continuously by implanting a pressure sensor into the left ventricle (36). However, per clinical score systems, such hemodynamic assessment is not required in most patients (2). We found that in many studies, hemodynamic measurements are the only method reported for evaluating diastolic function. Credit was still given to studies if they showed a significant diastolic function change.
5. Model validations with clinical scores
5.1. Aortic banding
Aortic banding mimics high blood pressure by constricting the aorta, which in turn induces hypertension and further causes chronic LV pressure overload and hypertrophy. This model is primarily used in pigs. Constrictors can be placed in the aortic root, ascending aorta, or descending aorta. In these studies, LV fibrosis is consistently documented as the major pathologic change. The two types of constrictors are the fixed-size or pressure constrictor (40–49), or the inflatable cuff (50, 51) (Figure 4) that can be used to adjust the size and pressure gradually over time through a subcutaneous tunnel that can be connected externally.
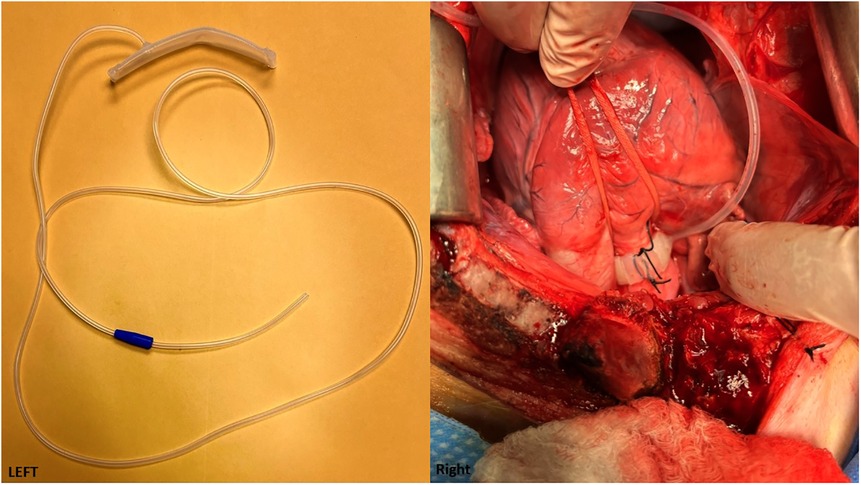
Figure 4. (Left) An adjustable aortic cuff that can be inflated to different pressure levels. (Cuff is from Access Technologies, Skokie, IL, www.norfolkaccess.com.) (Right) Surgical view showing the adjustable aortic cuff attached to the aortic root.
Currently, aortic banding is the most widespread, well-known method for inducing HFpEF. However, in the 12 studies we reviewed, only 4 studies (44–46, 50) were qualified under HFA-PEFF, and none of them were qualified under H2FPEF (Table 1). Among all studies, only the feline model created by Wallner et al. (46) and the pig model by Tan et al. (45) provided thorough echocardiographic evaluation for both diastolic function (including E/e') and morphology change. All other studies focused on the LV morphology changes only, such as wall thickness and left ventricular mass, although a few of them provided hemodynamic analysis. However, not all hemodynamic analyses showed diastolic function change (43). In addition, only a small portion of studies showed brain natriuretic peptide (BNP) results. Therefore, only 25% studies were qualified as an HFpEF model on the basis of the HFA-PEFF score. The H2FPEF score was even worse, with 4 as the highest score. Only one study successfully demonstrated increased weight, in addition to hypertension, in the experimental group (50), and only 2 studies (45, 46) showed both pulmonary hypertension and diastolic function change.
5.2. Aortic stent
An aortic stent has effects similar to aortic banding but is less invasive because it requires a percutaneous procedure instead of open-chest surgery. The stent is ingrown into the aortic wall and does not allow normal aortic growth due to the stent's constant size, which causes partial antegrade obstruction and stenosis in the aorta (Figure 5). To date, this technique has been used in only one study (52) in which a pig showed significantly changed diastolic function and LV morphology, increased BNP, and pulmonary hypertension. The HFA-PEFF and H2FPEF scores were 6 and 3, respectively (Table 2). Pathology showed LV fibrosis and hypertrophy.
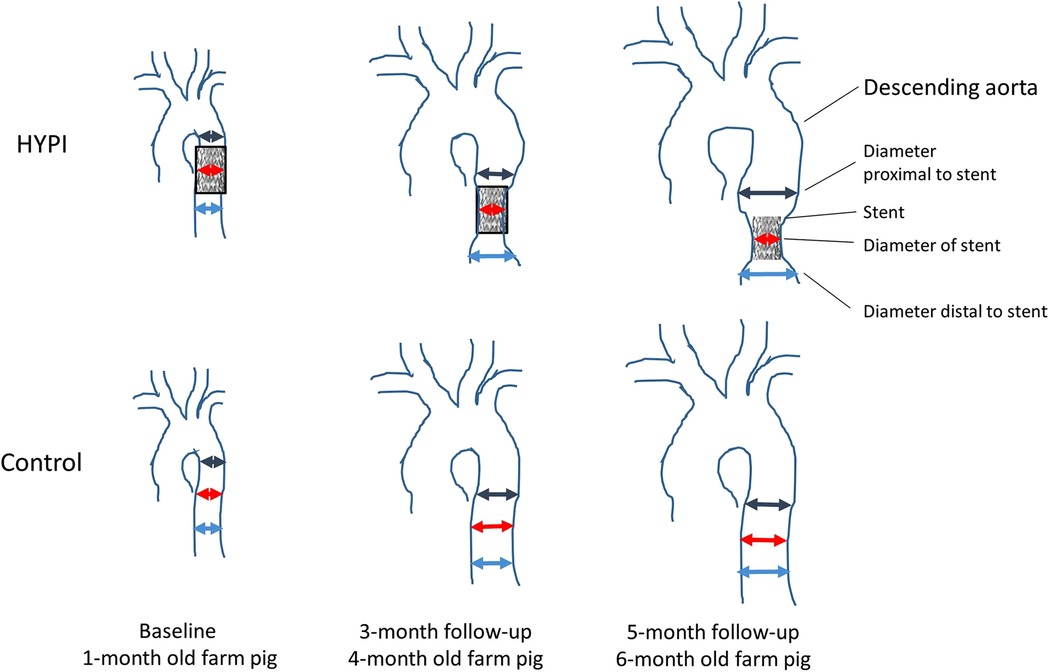
Figure 5. Schematic illustration of the development of artificial aortic isthmus stenosis. HYPI: group of cardiac hypertrophies. From (52).
5.3. Renal wrap
Renal wrap has been used primarily in dogs. Briefly, the procedure includes performing a midline abdominal incision, followed by wrapping a single kidney or both kidneys through silk or sterilized cellophane without constricting the renal vessels. This method produces persistent renal hypertension due to perinephritis. The hypertension causes LV pressure overload and induces LV hypertrophy. Because dogs can reach “old” age more easily than other animal models, these studies may be useful for examining age as a factor. For example, Munagla et al. (53) wrapped both kidneys with silk tissue and compared old dogs with old and young control dogs. They evaluated diastolic function through catheters but did not perform any echocardiographic evaluation. Their results showed impaired LV diastolic function and increased LV mass with persistent hypertension in the experimental group of old dogs. However, they did not include a young experimental group, and the level of myocardial fibrosis was similar between the experimental group consisting of old dogs and the control group consisting of young dogs; therefore, it was challenging to determine the influence of age in this model. Hamdani et al. (54) used the same methods and groups in dogs and obtained similar results, in addition to observing weight gain in the experimental group of old dogs. No studies included any BNP results. Therefore, under either clinical score system, none of these studies qualified as an HFpEF model (Table 3). Although there are reports of other species, such as pig or nonhuman primates, they do not specifically focus on the HFpEF model; therefore, those reports were not included in this review.
5.4. Renal clamp/embolization
For the renal clamping procedure, an adjustable clamp made of pure silver or stainless steel is used, and each clamp is attached to the renal artery at around 1 cm distal from its origin to apply an arbitrary extent of ligation. Many studies have described the use of clamps to induce hypertension in dogs, but none focused on HFpEF. Recently, renal embolization has been used as a new technique to induce hypertension by injecting microspheres through the renal artery to embolize the whole kidney. To date, one study has described renal embolization as one of multiple ways to induce HFpEF. Therefore, this study is discussed in the section “combination models.”
5.5. Medication
Deoxycorticosterone acetate (DOCA) is a mineralocorticoid receptor agonist that acts as a precursor to aldosterone. The medication is administered subcutaneously (100 mg/kg) in pellets and is released over 90 days, with the aim of promoting sodium and water retention. One study (55) demonstrated that treating pigs with DOCA increased mean arterial pressure by approximately 20 mmHg compared with the control. In addition, cardiovascular magnetic resonance imaging analysis showed left atrial enlargement, and E' and E/E' markers were the most different between DOCA and control pigs at rest. Inadequately increased myocardial perfusion reserve during stress may represent a metric for early-stage HFpEF; overall, it had a HFA-PEFF score of 4 and a H2FPEF score of 2 (Table 4).
In most cases, DOCA was combined with other methods to introduce HFpEF, which is discussed in the section “combination models.”
5.6. Diet
Diet can be used as a complementary method for inducing metabolic syndrome in heart failure models. Diets can vary according to the aim of the research, but the most common diet is the Western diet (56, 57), which has a high content of salt and fat. If needed, researchers can supplement with high sugar and calories as a method to induce metabolic disorders that mimic the findings in metabolic syndrome. Diet is most effective in small animals. In large animals, diet is frequently associated with other comorbidities that simulate risk factors related to human HF. However, diet alone is not enough to induce effects on diastolic cardiac function in large animal models.
5.7. Combination models
Because HFpEF in humans is a highly heterogeneous syndrome, more researchers are looking to develop models that introduce multiple risk factors, such as a high-fat diet, hypertension, and diabetes. These models may accurately mimic multi-system dysfunction that closely represents human pathophysiologic change (Table 5).
Sorop et al. (58) introduced a triple-factor model in pigs that included type 2 diabetes induced by streptozotocin, hypercholesterolemia produced by a high-fat diet, and hypertension produced by renal embolization. In addition to targeted risk factors, the experimental pigs presented systemic inflammation and diastolic dysfunction while the EF was maintained in the normal range. However, a high-fat diet did not help the pig to develop obesity; conversely, experimental pigs weighed significantly less than did control pigs, which the authors attributed to growth retardation resulting from renal dysfunction. Therefore, this combination model did not result in a high clinical score.
In another combination model in which multiple methods were used to induce risk factors, renal wrap and DOCA were performed in old dogs (59). At the end of the experiment, these dogs showed significantly elevated blood pressure and deterioration of diastolic function compared with dogs treated only with renal wrap. However, the LV mass between groups was not significantly different. In this study, echocardiography and hemodynamic studies were performed only in the final week. Therefore, the lack of baseline and healthy control data limit the probability that the LV morphology change observed qualified as HFpEF.
The combination of diet and hypertension—the most common multiple comorbidities of HFpEF—has also been applied as a model. In a study of mini-pigs fed a diet high in fat, fructose, and salt combined with DOCA, the pigs developed obesity, hypertension, and diastolic dysfunction with pulmonary hypertension (60). This model demonstrated comprehensive systemic changes. In another model described by Zhang et al. (61), pigs were given a combination of DOCA, angiotensin II (to produce hypertension), and a Western diet. The experimental pigs showed significant changes in diastolic dysfunction, LV hypertrophy, obesity, pulmonary hypertension, and BNP levels. In a study of Ossabaw pigs in which the use of aortic banding was combined with a high-fat diet, similar comprehensive systemic responses were observed (57), including hypertension, cardiac hypertrophy, features of diastolic dysfunction from catheter measurements, peripheral and central vascular dysfunction, and obesity with a systemic inflammatory state. Significant changes were also observed in the experimental group's gene pathways related to cardiac and pulmonary fibrosis, pulmonary hypertension, and atrial fibrillation. Furthermore, the dobutamine-induced functional kinetic reserve was examined on the molecular level. However, under the clinical scoring system, this study did not provide the BNP measurements and lacked direct measurements for pulmonary hypertension and atrial fibrillation, resulting in a score that was not as good as others.
In another study, Schwarzl et al. (62) induced hypertension and hyperlipidemia in landrace pigs by using DOCA and a Western diet containing high amounts of salt, fat, cholesterol, and sugar for 12 weeks. Compared with weight-matched controls, pigs treated with DOCA and a Western diet showed LV concentric hypertrophy and left atrial dilatation; however, no change in BNP was detected, and diastolic function changes from echocardiography were lacking, although the authors provided stress data to show decreased heart functional reserve. Mühlfeld et al. (63) induced HFpEF in pigs by treatment with DOCA combined with a high-salt/high-lipid diet for 3 months and compared them with normal weight-matched pigs. The study was focused on histopathology and DOCA-induced changes in cardiomyocytes (diameter, subcellular composition) within all layers of the LV free wall. DOCA induced changes in the interstitium, which appeared to be more pronounced in the subendocardial ventricular wall layers. However, the study lacked functional analysis, resulting in a very low score.
6. Discussion
Using the two most common clinical score systems, we evaluated large animal models of HFpEF that are currently used in the research field. When we combined all of the single-factor studies or used the most popular single-factor study of aortic banding to compare with the combination models, we found among the large animal studies that HFA-PEFF criteria were easier to meet than those of the H2FPEF score, whether the study was a single-factor or multiple-factor design (Table 6). For the H2FPEF score, the combination models had better scores, but only 2 of them were close to the threshold as high-probability HFpEF. As we mentioned above, the HFA-PEFF score focuses more on cardiac features, whereas the H2FPEF score has more extra-cardiac factors. Therefore, we believe a combination model with multiple risk factors will be more likely to reach a high H2FPEF score and better reflect the heterogeneity of HFpEF. We also noted that the use of an aortic stent, renal wrapping, and DOCA had better H2FPEF or HFA-PEFF scores. Because the number of studies is limited compared with the number of studies of aortic banding and combination models, it is difficult to conclude whether they are better models. From the overall comparison, the combination models still had the better H2PEF scores. New techniques, such as telemetry medicine, will help to facilitate a multiple-factor model more easily than before. Our group is using a triple-factor model in pigs that includes hypertension, diabetes, and hypercholesterolemia. In our model, we integrate many new techniques, such as the use of a continuous LV pressure sensor, EKG sensor, and blood pressure sensor. We hope these sensors can provide more valuable information when we reach the endpoint.
For the models that received a lower score under the H2FPEF, we believe there are several reasons. First, most models failed to produce obesity, even with a high-fat diet, which is an interesting topic. In our own experience, we realize not every animal has the same appetite for a high-fat diet. Animals may lose weight if given a food that they do not want to eat. Also, some other factors introduced into the model, such as renal dysfunction, may further affect the animals' appetite. Second, many models lacked methods for monitoring. For example, atrial fibrillation is an important factor to consider, and most studies made no mention of EKG. Currently, as telemetry becomes more and more popular, we believe the tool can be used to monitor EKG for a long time in animals. For example, in our own studies, we implant an EKG recorder into the pig's body to provide a continuous EKG monitor. We hope that this allows us to capture the EKG changes during the whole period and make the model more reliable.
Echocardiography plays an important role in both of the score systems studied. Therefore, an experienced echocardiographer is essential for the whole study. Unfortunately, many studies did not provide good echocardiographic evaluations, especially for diastolic function. Although hemodynamic measurement is the gold standard for evaluating diastolic function, it is invasive, complicated, expensive, and lacks repeatability and is thus rarely used in clinical evaluations. Therefore, a method that is consistent with clinical application should be applied to validate the model. For example, echocardiography can be used to monitor the animal weekly by noninvasive means and show a clear trend of diastolic function change. In contrast, hemodynamic measurement can be scheduled only 2 or 3 times during the study period because of its invasiveness. Some authors reported that echocardiography was not good because of the animals’ anatomical variation. In our own experience, at least with pigs, good images can be easily obtained through the subcostal view, with clear mitral valve flow and tissue Doppler signals. Therefore, we strongly suggest that diastolic function data be evaluated by using echocardiography and that hemodynamic measurement be reserved for additional verification.
It is important to note that the clinical scores evaluated in this study do not cover all facets of HFpEF. For example, the scores do not account for pathology, change of peripheral vascular function, or stress/exercise tests, which are valuable for understanding the pathological changes in the disease. Just as in the combination model (57) discussed above, a much wider range of systemic analyses was presented that included peripheral vascular changes and gene pathways, but the clinical score was not high. Therefore, when designing studies, the ultimate goal should not be only to improve clinical scores. Monitoring pathologic change and performing novel gene sequence analysis will help improve our understanding of HFpEF. In other words, although some animal models received lower scores in the clinical score systems, this does not mean that they are not a good model. The purpose of using the clinical score systems is to have some objective criteria by which to judge, especially for evaluating a new medication or device, and to help researchers design a comprehensive model that addresses clinical needs (61).
7. Conclusion
HFpEF is a complex disease with multiple contributing comorbidities that make it difficult to develop effective preclinical models. Progress in the field requires agreement on key features of animal models of HFpEF. Two new clinical scores have recently been developed to define these features. With this review, we are the first to our knowledge to propose using these scores to evaluate large animal models of HFpEF and create a checklist for optimizing translational research. Although most animal models do not meet all criteria, some multifactorial models resemble human HFpEF and may be the future of research. Our proposed approach aims to fill major gaps in HFpEF pathophysiology and facilitate the development of new therapeutics.
Author contributions
K.L. and C.O. made substantial contributions to the literature research, conception and design, interpretation of data, and drafting of the manuscript. A.M.R. contributed to the collection and interpretation of data. A.E. and E.P. revised the manuscript critically for important intellectual content. All authors contributed to the article and approved the submitted version.
Acknowledgments
Nicole Stancel, PhD, ELS(D), of Scientific Publications at The Texas Heart Institute, provided editorial support.
Conflict of interest
The authors declare that the research was conducted in the absence of any commercial or financial relationships that could be construed as a potential conflict of interest.
Publisher's note
All claims expressed in this article are solely those of the authors and do not necessarily represent those of their affiliated organizations, or those of the publisher, the editors and the reviewers. Any product that may be evaluated in this article, or claim that may be made by its manufacturer, is not guaranteed or endorsed by the publisher.
References
1. Borlaug BA. The pathophysiology of heart failure with preserved ejection fraction. Nat Rev Cardiol. (2014) 11:507–15. doi: 10.1038/nrcardio.2014.83
2. Borlaug BA. Evaluation and management of heart failure with preserved ejection fraction. Nat Rev Cardiol. (2020) 17:559–73. doi: 10.1038/s41569-020-0363-2
3. Dunlay SM, Roger VL, Redfield MM. Epidemiology of heart failure with preserved ejection fraction. Nat Rev Cardiol. (2017) 14:591–602. doi: 10.1038/nrcardio.2017.65
4. Ma C, Luo H, Fan L, Liu X, Gao C. Heart failure with preserved ejection fraction: an update on pathophysiology, diagnosis, treatment, and prognosis. Braz J Med Biol Res. (2020) 53:e9646. doi: 10.1590/1414-431x20209646
5. Ponikowski P, Voors AA, Anker SD, Bueno H, Cleland JGF, Coats AJS, et al. 2016 ESC guidelines for the diagnosis and treatment of acute and chronic heart failure: the task force for the diagnosis and treatment of acute and chronic heart failure of the European Society of Cardiology (ESC) developed with the special contribution of the Heart Failure Association (HFA) of the ESC. Eur Heart J. (2016) 37:2129–200. doi: 10.1093/eurheartj/ehw128
6. Oktay AA, Rich JD, Shah SJ. The emerging epidemic of heart failure with preserved ejection fraction. Curr Heart Fail Rep. (2013) 10:401–10. doi: 10.1007/s11897-013-0155-7
7. Anker SD, Butler J, Filippatos G, Ferreira JP, Bocchi E, Bohm M, et al. Empagliflozin in heart failure with a preserved ejection fraction. N Engl J Med. (2021) 385:1451–61. doi: 10.1056/NEJMoa2107038
8. Nassif ME, Windsor SL, Borlaug BA, Kitzman DW, Shah SJ, Tang F, et al. The SGLT2 inhibitor dapagliflozin in heart failure with preserved ejection fraction: a multicenter randomized trial. Nat Med. (2021) 27:1954–60. doi: 10.1038/s41591-021-01536-x
9. Pfeffer MA, Shah AM, Borlaug BA. Heart failure with preserved ejection fraction in perspective. Circ Res. (2019) 124:1598–617. doi: 10.1161/CIRCRESAHA.119.313572
10. van Ham WB, Kessler EL, Oerlemans M, Handoko ML, Sluijter JPG, van Veen TAB, et al. Clinical phenotypes of heart failure with preserved ejection fraction to select preclinical animal models. JACC Basic Transl Sci. (2022) 7:844–57. doi: 10.1016/j.jacbts.2021.12.009
11. de Boer RA, Nayor M, deFilippi CR, Enserro D, Bhambhani V, Kizer JR, et al. Association of cardiovascular biomarkers with incident heart failure with preserved and reduced ejection fraction. JAMA Cardiol. (2018) 3:215–24. doi: 10.1001/jamacardio.2017.4987
12. Eaton CB, Pettinger M, Rossouw J, Martin LW, Foraker R, Quddus A, et al. Risk factors for incident hospitalized heart failure with preserved versus reduced ejection fraction in a multiracial cohort of postmenopausal women. Circ Heart Fail. (2016) 9:e002883. doi: 10.1161/CIRCHEARTFAILURE.115.002883
13. Goyal P, Paul T, Almarzooq ZI, Peterson JC, Krishnan U, Swaminathan RV, et al. Sex- and race-related differences in characteristics and outcomes of hospitalizations for heart failure with preserved ejection fraction. J Am Heart Assoc. (2017) 6:e003330. doi: 10.1161/JAHA.116.003330
14. Savji N, Meijers WC, Bartz TM, Bhambhani V, Cushman M, Nayor M, et al. The association of obesity and cardiometabolic traits with incident HFpEF and HFrEF. JACC Heart Fail. (2018) 6:701–9. doi: 10.1016/j.jchf.2018.05.018
15. Tourki B, Halade GV. Heart failure syndrome with preserved ejection fraction is a metabolic cluster of non-resolving inflammation in obesity. Front Cardiovasc Med. (2021) 8:695952. doi: 10.3389/fcvm.2021.695952
16. Molina AJ, Bharadwaj MS, Van Horn C, Nicklas BJ, Lyles MF, Eggebeen J, et al. Skeletal muscle mitochondrial content, oxidative capacity, and Mfn2 expression are reduced in older patients with heart failure and preserved ejection fraction and are related to exercise intolerance. JACC Heart Fail. (2016) 4:636–45. doi: 10.1016/j.jchf.2016.03.011
17. Reddy YNV, Andersen MJ, Obokata M, Koepp KE, Kane GC, Melenovsky V, et al. Arterial stiffening with exercise in patients with heart failure and preserved ejection fraction. J Am Coll Cardiol. (2017) 70:136–48. doi: 10.1016/j.jacc.2017.05.029
18. Houstis NE, Eisman AS, Pappagianopoulos PP, Wooster L, Bailey CS, Wagner PD, et al. Exercise intolerance in heart failure with preserved ejection fraction: diagnosing and ranking its causes using personalized O2 pathway analysis. Circulation. (2018) 137:148–61. doi: 10.1161/CIRCULATIONAHA.117.029058
19. Horgan S, Watson C, Glezeva N, Baugh J. Murine models of diastolic dysfunction and heart failure with preserved ejection fraction. J Card Fail. (2014) 20:984–95. doi: 10.1016/j.cardfail.2014.09.001
20. Vaduganathan M, Patel RB, Michel A, Shah SJ, Senni M, Gheorghiade M, et al. Mode of death in heart failure with preserved ejection fraction. J Am Coll Cardiol. (2017) 69:556–69. doi: 10.1016/j.jacc.2016.10.078
21. De Simone G, Devereux RB, Chinali M, Roman MJ, Barac A, Panza JA, et al. Sex differences in obesity-related changes in left ventricular morphology: the strong heart study. J Hypertens. (2011) 29:1431–8. doi: 10.1097/HJH.0b013e328347a093
22. Tadic M, Cuspidi C, Plein S, Belyavskiy E, Heinzel F, Galderisi M. Sex and heart failure with preserved ejection fraction: from pathophysiology to clinical studies. J Clin Med. (2019) 8:792. doi: 10.3390/jcm8060792
23. Chirinos JA, Orlenko A, Zhao L, Basso MD, Cvijic ME, Li Z, et al. Multiple plasma biomarkers for risk stratification in patients with heart failure and preserved ejection fraction. J Am Coll Cardiol. (2020) 75:1281–95. doi: 10.1016/j.jacc.2019.12.069
24. Withaar C, Lam CSP, Schiattarella GG, de Boer RA, Meems LMG. Heart failure with preserved ejection fraction in humans and mice: embracing clinical complexity in mouse models. Eur Heart J. (2021) 42:4420–30. doi: 10.1093/eurheartj/ehab389
25. Pieske B, Tschope C, de Boer RA, Fraser AG, Anker SD, Donal E, et al. How to diagnose heart failure with preserved ejection fraction: the HFA-PEFF diagnostic algorithm: a consensus recommendation from the Heart Failure Association (HFA) of the European Society of Cardiology (ESC). Eur Heart J. (2019) 40:3297–317. doi: 10.1093/eurheartj/ehz641
26. Reddy YNV, Carter RE, Obokata M, Redfield MM, Borlaug BA. A simple, evidence-based approach to help guide diagnosis of heart failure with preserved ejection fraction. Circulation. (2018) 138:861–70. doi: 10.1161/CIRCULATIONAHA.118.034646
27. Abramov D, Parwani P. Diving into the diagnostic score algorithms of heart failure with preserved ejection fraction. Front Cardiovasc Med. (2021) 8:665424. doi: 10.3389/fcvm.2021.665424
28. Churchill TW, Li SX, Curreri L, Zern EK, Lau ES, Liu EE, et al. Evaluation of 2 existing diagnostic scores for heart failure with preserved ejection fraction against a comprehensively phenotyped cohort. Circulation. (2021) 143:289–91. doi: 10.1161/CIRCULATIONAHA.120.050757
29. Faxen UL, Venkateshvaran A, Shah SJ, Lam CSP, Svedlund S, Saraste A, et al. Generalizability of HFA-PEFF and H2FPEF diagnostic algorithms and associations with heart failure indices and proteomic biomarkers: insights from PROMIS-HFpEF. J Card Fail. (2021) 27:756–65. doi: 10.1016/j.cardfail.2021.02.005
30. Selvaraj S, Myhre PL, Vaduganathan M, Claggett BL, Matsushita K, Kitzman DW, et al. Application of diagnostic algorithms for heart failure with preserved ejection fraction to the community. JACC Heart Fail. (2020) 8:640–53. doi: 10.1016/j.jchf.2020.03.013
31. Stewart Coats AJ. Validating the HFA-PEFF score—or how to define a disease? Eur J Heart Fail. (2020) 22:428–31. doi: 10.1002/ejhf.1770
32. Ho JE, Redfield MM, Lewis GD, Paulus WJ, Lam CSP. Deliberating the diagnostic dilemma of heart failure with preserved ejection fraction. Circulation. (2020) 142:1770–80. doi: 10.1161/CIRCULATIONAHA.119.041818
33. Parcha V, Malla G, Kalra R, Patel N, Sanders-van Wijk S, Pandey A, et al. Diagnostic and prognostic implications of heart failure with preserved ejection fraction scoring systems. ESC Heart Fail. (2021) 8:2089–102. doi: 10.1002/ehf2.13288
34. Sueta D, Yamamoto E, Nishihara T, Tokitsu T, Fujisue K, Oike F, et al. H2FPEF score as a prognostic value in HFpEF patients. Am J Hypertens. (2019) 32:1082–90. doi: 10.1093/ajh/hpz108
35. Yancy CW, Jessup M, Bozkurt B, Butler J, Casey DE Jr., Drazner MH, et al. 2013 ACCF/AHA guideline for the management of heart failure: a report of the American College of Cardiology Foundation/American Heart Association task force on practice guidelines. J Am Coll Cardiol. (2013) 62:e147–239. doi: 10.1016/j.jacc.2013.05.019
36. Geist B, Youngblood BL, Ueyama Y, LaRose A, Hamlin R, Evanchik MJ, et al. Telemetered left-ventricular pressure in yucatan mini-pigs: chronic evaluation in a novel genetic model of non-obstructed hypertrophic cardiomyopathy. J Pharmacol Toxicol Methods. (2019) 99:106595. doi: 10.1016/j.vascn.2019.05.142
37. Hoffe B, Holahan MR. The use of pigs as a translational model for studying neurodegenerative diseases. Front Physiol. (2019) 10:838. doi: 10.3389/fphys.2019.00838
38. Nakamura K, Neidig LE, Yang X, Weber GJ, El-Nachef D, Tsuchida H, et al. Pharmacologic therapy for engraftment arrhythmia induced by transplantation of human cardiomyocytes. Stem Cell Rep. (2021) 16:2473–87. doi: 10.1016/j.stemcr.2021.08.005
39. Zile MR, Baicu CF, Gaasch WH. Diastolic heart failure–abnormalities in active relaxation and passive stiffness of the left ventricle. N Engl J Med. (2004) 350:1953–9. doi: 10.1056/NEJMoa032566
40. Emter CA, Baines CP. Low-intensity aerobic interval training attenuates pathological left ventricular remodeling and mitochondrial dysfunction in aortic-banded miniature swine. Am J Physiol Heart Circ Physiol. (2010) 299:H1348–56. doi: 10.1152/ajpheart.00578.2010
41. Gong G, Liu J, Liang P, Guo T, Hu Q, Ochiai K, et al. Oxidative capacity in failing hearts. Am J Physiol Heart Circ Physiol. (2003) 285:H541–8. doi: 10.1152/ajpheart.01142.2002
42. Hiemstra JA, Gutierrez-Aguilar M, Marshall KD, McCommis KS, Zgoda PJ, Cruz-Rivera N, et al. A new twist on an old idea part 2: cyclosporine preserves normal mitochondrial but not cardiomyocyte function in mini-swine with compensated heart failure. Physiol Rep. (2014) 2:e12050. doi: 10.14814/phy2.12050
43. Ishikawa K, Aguero J, Oh JG, Hammoudi N, Fish LA, Leonardson L, et al. Increased stiffness is the major early abnormality in a pig model of severe aortic stenosis and predisposes to congestive heart failure in the absence of systolic dysfunction. J Am Heart Assoc. (2015) 4:e001925. doi: 10.1161/JAHA.115.001925
44. Marshall KD, Muller BN, Krenz M, Hanft LM, McDonald KS, Dellsperger KC, et al. Heart failure with preserved ejection fraction: chronic low-intensity interval exercise training preserves myocardial O2 balance and diastolic function. J Appl Physiol (1985). (2013) 114:131–47. doi: 10.1152/japplphysiol.01059.2012
45. Tan W, Li X, Zheng S, Li X, Zhang X, Pyle WG, et al. A porcine model of heart failure with preserved ejection fraction induced by chronic pressure overload characterized by cardiac fibrosis and remodeling. Front Cardiovasc Med. (2021) 8:677727. doi: 10.3389/fcvm.2021.677727
46. Wallner M, Eaton DM, Berretta RM, Borghetti G, Wu J, Baker ST, et al. A feline HFpEF model with pulmonary hypertension and compromised pulmonary function. Sci Rep. (2017) 7:16587. doi: 10.1038/s41598-017-15851-2
47. Wang X, Hu Q, Mansoor A, Lee J, Wang Z, Lee T, et al. Bioenergetic and functional consequences of stem cell-based VEGF delivery in pressure-overloaded swine hearts. Am J Physiol Heart Circ Physiol. (2006) 290:H1393–405. doi: 10.1152/ajpheart.00871.2005
48. Xiong Q, Zhang P, Guo J, Swingen C, Jang A, Zhang J. Myocardial ATP hydrolysis rates in vivo: a porcine model of pressure overload-induced hypertrophy. Am J Physiol Heart Circ Physiol. (2015) 309:H450–8. doi: 10.1152/ajpheart.00072.2015
49. Ye Y, Gong G, Ochiai K, Liu J, Zhang J. High-energy phosphate metabolism and creatine kinase in failing hearts: a new porcine model. Circulation. (2001) 103:1570–6. doi: 10.1161/01.CIR.103.11.1570
50. Charles CJ, Lee P, Li RR, Yeung T, Ibraham Mazlan SM, Tay ZW, et al. A porcine model of heart failure with preserved ejection fraction: magnetic resonance imaging and metabolic energetics. ESC Heart Fail. (2020) 7:92–102. doi: 10.1002/ehf2.12536
51. Yarbrough WM, Mukherjee R, Stroud RE, Rivers WT, Oelsen JM, Dixon JA, et al. Progressive induction of left ventricular pressure overload in a large animal model elicits myocardial remodeling and a unique matrix signature. J Thorac Cardiovasc Surg. (2012) 143:215–23. doi: 10.1016/j.jtcvs.2011.09.032
52. Gyongyosi M, Pavo N, Lukovic D, Zlabinger K, Spannbauer A, Traxler D, et al. Porcine model of progressive cardiac hypertrophy and fibrosis with secondary postcapillary pulmonary hypertension. J Transl Med. (2017) 15:202. doi: 10.1186/s12967-017-1299-0
53. Munagala VK, Hart CY, Burnett JC Jr., Meyer DM, Redfield MM. Ventricular structure and function in aged dogs with renal hypertension: a model of experimental diastolic heart failure. Circulation. (2005) 111:1128–35. doi: 10.1161/01.CIR.0000157183.21404.63
54. Hamdani N, Bishu KG, von Frieling-Salewsky M, Redfield MM, Linke WA. Deranged myofilament phosphorylation and function in experimental heart failure with preserved ejection fraction. Cardiovasc Res. (2013) 97:464–71. doi: 10.1093/cvr/cvs353
55. Reiter U, Reiter G, Manninger M, Adelsmayr G, Schipke J, Alogna A, et al. Early-stage heart failure with preserved ejection fraction in the pig: a cardiovascular magnetic resonance study. J Cardiovasc Magn Reson. (2016) 18:63. doi: 10.1186/s12968-016-0283-9
56. Nachar W, Merlet N, Maafi F, Shi Y, Mihalache-Avram T, Mecteau M, et al. Cardiac inflammation and diastolic dysfunction in hypercholesterolemic rabbits. PLoS One. (2019) 14:e0220707. doi: 10.1371/journal.pone.0220707
57. Olver TD, Edwards JC, Jurrissen TJ, Veteto AB, Jones JL, Gao C, et al. Western diet-fed, aortic-banded ossabaw swine: a preclinical model of cardio-metabolic heart failure. JACC Basic Transl Sci. (2019) 4:404–21. doi: 10.1016/j.jacbts.2019.02.004
58. Sorop O, Heinonen I, van Kranenburg M, van de Wouw J, de Beer VJ, Nguyen ITN, et al. Multiple common comorbidities produce left ventricular diastolic dysfunction associated with coronary microvascular dysfunction, oxidative stress, and myocardial stiffening. Cardiovasc Res. (2018) 114:954–64. doi: 10.1093/cvr/cvy038
59. Shapiro BP, Owan TE, Mohammed S, Kruger M, Linke WA, Burnett JC Jr., et al. Mineralocorticoid signaling in transition to heart failure with normal ejection fraction. Hypertension. (2008) 51:289–95. doi: 10.1161/HYPERTENSIONAHA.107.099010
60. Sharp TE 3rd, Scarborough AL, Li Z, Polhemus DJ, Hidalgo HA, Schumacher JD, et al. Novel Gottingen miniswine model of heart failure with preserved ejection fraction integrating multiple comorbidities. JACC Basic Transl Sci. (2021) 6:154–70. doi: 10.1016/j.jacbts.2020.11.012
61. Zhang N, Feng B, Ma X, Sun K, Xu G, Zhou Y. Dapagliflozin improves left ventricular remodeling and aorta sympathetic tone in a pig model of heart failure with preserved ejection fraction. Cardiovasc Diabetol. (2019) 18:107. doi: 10.1186/s12933-019-0914-1
62. Schwarzl M, Hamdani N, Seiler S, Alogna A, Manninger M, Reilly S, et al. A porcine model of hypertensive cardiomyopathy: implications for heart failure with preserved ejection fraction. Am J Physiol Heart Circ Physiol. (2015) 309:H1407–18. doi: 10.1152/ajpheart.00542.2015
Keywords: large animal model, heart failure, HFPEF, HFA-PEFF, H2FPEF
Citation: Li K, Cardoso C, Moctezuma-Ramirez A, Elgalad A and Perin E (2023) Evaluation of large animal models for preclinical studies of heart failure with preserved ejection fraction using clinical score systems. Front. Cardiovasc. Med. 10:1099453. doi: 10.3389/fcvm.2023.1099453
Received: 15 November 2022; Accepted: 2 March 2023;
Published: 23 March 2023.
Edited by:
Sang-Bing Ong, The Chinese University of Hong Kong, ChinaReviewed by:
Thomas E. Sharp III, Louisiana State University, United StatesKiyotaka Fukamachi, Cleveland Clinic, United States
© 2023 Li, Cardoso, Moctezuma-Ramirez, Elgalad and Perin. This is an open-access article distributed under the terms of the Creative Commons Attribution License (CC BY). The use, distribution or reproduction in other forums is permitted, provided the original author(s) and the copyright owner(s) are credited and that the original publication in this journal is cited, in accordance with accepted academic practice. No use, distribution or reproduction is permitted which does not comply with these terms.
*Correspondence: Abdelmotagaly Elgalad aelgalad@texasheart.org
Specialty Section: This article was submitted to Heart Failure and Transplantation, a section of the journal Frontiers in Cardiovascular Medicine