- 1Department of Cardiology, Brugmann Hospital, Université Libre de Bruxelles, Brussels, Belgium
- 2Laboratoray of Physics and Physiology, Université Libre de Bruxelles, Brussels, Belgium
- 3Heart Rhythm Management Centre, European Reference Networks Guard-Heart, Universitair Ziekenhuis Brussel - Vrije Universiteit Brussel, Brussels, Belgium
- 4Department of Cardiology, Erasme Hospital, Université Libre de Bruxelles, Brussels, Belgium
Background: Heart failure (HF) remains a major cause of mortality, morbidity, and poor quality of life. 44% of HF patients present impaired left ventricular ejection fraction (LVEF). Kinocardiography (KCG) technology combines ballistocardiography (BCG) and seismocardiography (SCG). It estimates myocardial contraction and blood flow through the cardiac chambers and major vessels through a wearable device. Kino-HF sought to evaluate the potential of KCG to distinguish HF patients with impaired LVEF from a control group.
Methods: Successive patients with HF and impaired LVEF (iLVEF group) were matched and compared to patients with normal LVEF ≥ 50% (control). A 60 s KCG acquisition followed cardiac ultrasound. The kinetic energy from KCG signals was computed in different phases of the cardiac cycle () as markers of cardiac mechanical function.
Results: Thirty HF patients (67 [59; 71] years, 87% male) were matched with 30 controls (64.5 [49; 73] years, 87% male). SCG , BCG , BCG were lower in HF than controls (p < 0.05), while SCG was similar. Furthermore, a lower SCG was associated with an increased mortality risk during follow-up.
Conclusions: KINO-HF demonstrates that KCG can distinguish HF patients with impaired systolic function from a control group. These favorable results warrant further research on the diagnostic and prognostic capabilities of KCG in HF with impaired LVEF.
Clinical Trial Registration: NCT03157115.
1. Introduction
Heart failure (HF) is a complex syndrome affecting an estimated 1%–2% of the population in developed countries. In the United States, it represents 6.5 million patients, accounting for ∼380.000 deaths and more than 3.5 million hospitalizations per year (1–3).
A major challenge in HF care is to prevent and shorten hospitalizations since they contribute significantly to the human and economic burden on patients and healthcare systems. The first few weeks after discharge entail an elevated mortality (4–6). Close clinical follow-up by general practitioners and cardiologists is needed but not always feasible given the limited resources in many geographical areas (7).
Home monitoring of patients has been investigated for years to intercept clinical or subclinical, indicators of increasing congestion. It aims to improve symptoms, prevent acute decompensation, and thereby the need for hospitalization for HF by enabling caregivers to modify treatment in a timely manner.
Recent years have witnessed an upsurge in the use of ballistocardiography (BCG) and seismocardiography (SCG), two techniques enabling the assessment of the inotropic state based on the measurement of body movements induced by cardiac contraction and blood flow in the cardiac chambers and major vessels (8, 9).
More recently, these techniques have been used in domains such as atrial fibrillation (10) and hypertension detection (11), heart failure monitoring (12, 13), cardiorespiratory fitness assessment (14, 15), and many others. Recent advances in the field of SCG were published by Taebi et al. (16).
Kinocardiography (KCG) is a subject-specific calibrated combination of linear and rotational SCG and BCG techniques. KCG is based on measures of 6 degrees-of-freedom (DOF) combining three-dimensional (3D) linear and 3D angular motion. These are recorded from sensors attached with electrodes to the presternal cutaneous surface (SCG) and the lumbar area (BCG).
In the present study, we used KCG metrics to measure kinetic energy distribution in different cardiac phases and compared them among subjects with impaired left ventricular ejection fraction (iLVEF) and a control group based on 2D-echocardiography.
2. Methods
2.1. Protocols and participants
All-comers between 20 and 85 years old at the echocardiography lab of the Brugmann University Hospital, Belgium, were invited to participate in the study. Exclusion criteria were pregnancy or any type of arrhythmia at the assessment time. In this feasibility study, patients with active right ventricular pacing were not included. Recordings were done in sinus rhythm only. This case-control study was performed between September 2017 and October 2021. The protocol complied with the Declaration of Helsinki and was approved by the local Ethics Committee (Brugmann University Hospital—CCB: B077201732405). The Belgian Federal Agency authorized the prototype device used in this clinical trial for Medicine and Health Products (FAMHP). Upon reception of the written informed consent, the participants' weight and height were measured. Then, they were equipped with the Kinocardiograph described in section 2.3 and were instructed to lie in a supine position on a bed for 5 min for stabilization. A blood-pressure measure was then performed (with Omron, EVOLV, HEM-7600T-E, Japan) after which a KCG recording was acquired for 60 s. Finally, participants were de-instrumented. A left ventricular ejection fraction (LVEF) below 50% was considered impaired.
2.2. Echocardiography
A basic comprehensive 2-dimensional transthoracic echocardiography was performed by a senior cardiologist who was blinded to the KCG measurements, with a Philips Epiq 7 ultrasound machine using a Philips X5-1 transducer (Eindhoven, The Netherlands).
The left ventricular outflow tract (LVOT) dimensions were obtained in the parasternal long-axis view during systole. The velocity time integral (VTI) at the LVOT was measured in the apical five-chamber view using pulsed-wave Doppler, and stroke volume (SV) was calculated using the following equation: SV = LVOT VTI × Cross Sectional Area of the LVOT (17). The left ventricular (LV) ejection fraction (LVEF) was measured with a modified Simpson's method (18). QRS duration was automatically measured for each of the 12-lead morphological analysis and expressed as ms, using the inbuilt software Extended Measurements Report of a Philips PageWriter TC50 electrocardiograph (Philips Medical Systems, Andover, MA, USA).
2.3. Kinocardiography data acquisition and analysis
The Kinocardiograph is a portable device with two embodiments, one of which (BCG) was placed over the lumbar region close to the subject's center of mass and the other one (SCG) over the manubrium sterni (Figure 1). The device records a two-lead ECG at 200 Hz together with 3D linear (Lin) accelerations and 3-DOF rotational (Rot) angular velocities from the sternum and the lumbar region as described in previous publications (19, 20). Ensemble averaging (EA) of signals was performed on all heartbeats. The time reference for the EA is a fixed interval that considers the cardiac activity preceding atrial depolarisation occurring before the R peak. Indeed, the beginning of the n-th interval started before the P wave, at time , where is the time of the n-th R peak and Δ is 200 ms. The end of the cardiac cycle of interest was assumed to be where represent all the RR intervals of the current record. The mean was taken on all the beats to obtain an average ECG signal. Based on this, an EA was calculated for each channel of the BCG and SCG recordings.
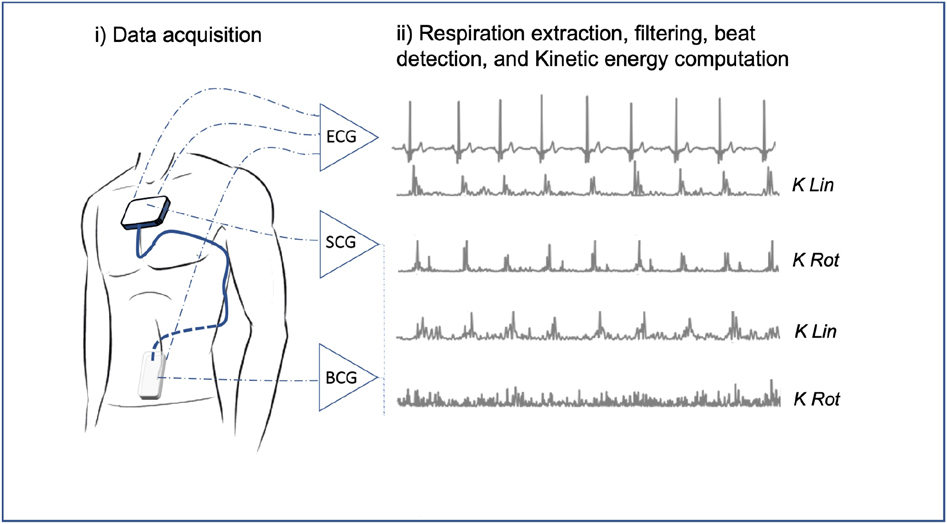
Figure 1. Kinocardiography signals acquisition and kinetic energy computation. From linear accelerations and angular rates raw acquisition on the chest (SCG) and in the lower back (BCG) to the kinetic energy metrics as described in section 2.4. SCG, seismocardiography; BCG, ballistocardiography; K, kinetic energy; Lin, linear; Rot, rotational.
Based on these acquisitions, the time integrals of kinetic energy (BCG , and SCG i , ) were computed in different phases of the cardiac cycle as described in a previous publication (20) and summarized in Figure 2. The three cardiac cycle phases were: from the start of the P wave to the start of the QRS complex (PQ phase, late diastole), from the start of the QRS complex to the end of T wave (QT phase, systole), and from the end of the T wave to the start of the next P wave (TP phase, early diastole). These cardiac phases are illustrated in Figure 2.
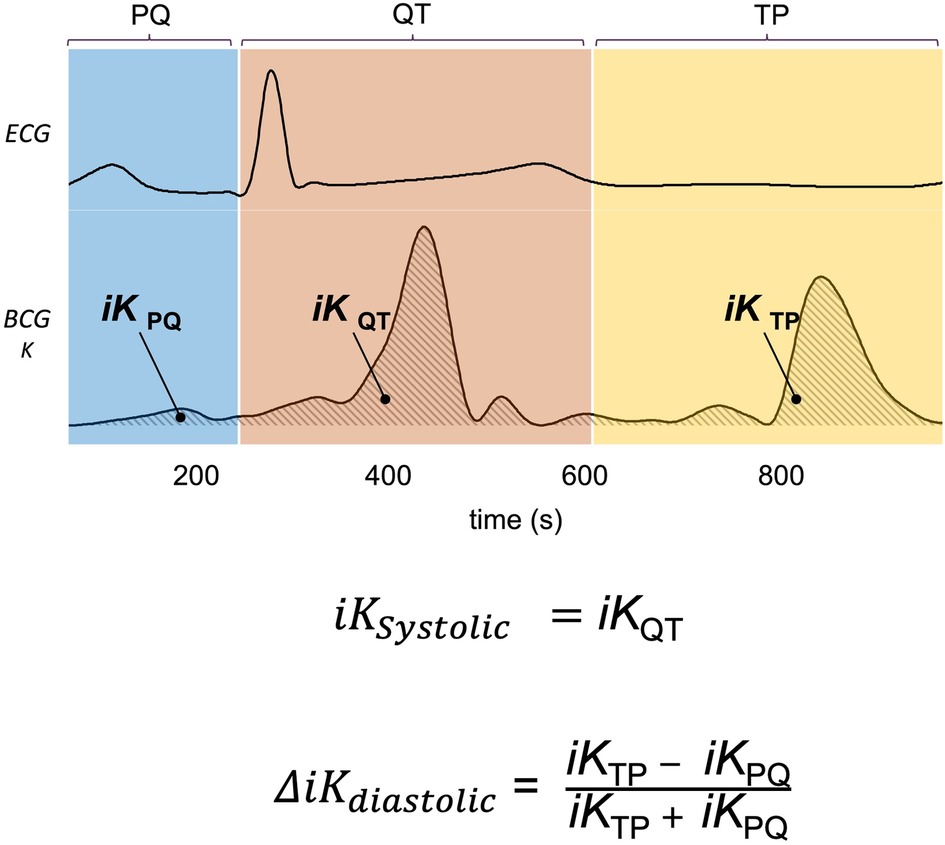
Figure 2. Kinocardiography metrics computation. Illustration of the PQ, QT, and TP phases segmented on the ECG and displayed for BCG kinetic energy (K). The time integrals of K are computed on each of these phases providing , , and respectively. Based on these, and are computed as displayed on the figure and further described in section 2.4.
These metrics have shown to be reproducible (21). Differences in iK metrics have been associated with differences in SV, LVEF, and cardiac output (CO) in healthy subjects during a dobutamine-induced hemodynamic increase (19, 22), with an increase during voluntary apnea (23) and obstructive apnea (24), and with a surge during sympathetic activation (25).
In this work, the following metrics to measure systolic and diastolic impairment were used (Figure 2):
1) The iK during the systolic phase was computed as: (3)
This led to the metrics SCG and BCG for SCG and BCG, respectively. We hypothesize that these metrics reflect the contractility of the left ventricle.
2) The difference of iK between the TP and PQ phases (passive and active filling, respectively) was normalized by iK during the complete diastolic phase (TQ = TP + PQ): (4)
We thus obtained the metrics SCG and BCG for SCG and BCG, respectively. We hypothesized that these metrics reflect the difference of kinetic energy exerted between the passive and active diastolic phases, normalized by the kinetic energy generated throughout the entire ventricular diastole.
2.4. Statistics
All data analyses were performed offline using a proprietary software toolbox under Matlab (Mathworks Inc.®).
Data are presented as median [Q1; Q3]. The characteristics of each group were compared by a two-sample t-test in case of normal distribution or a Wilcoxon signed rank test in case of non-normal distribution. A Lilliefors test was used to test if the difference between sample populations compared was normally distributed. At study closure, an exploratory post-hoc analysis was performed to assess whether KCG metrics have predictability characteristics in the group with iLVEF. A Cox proportional hazards approach was used to assess univariate and multivariate associations with survivability. When several parameters showed significance, they were included in a single model and compared to the initial models with a log-likelihood ratio and a Chi-square distribution with a degree of freedom equal to the number of predictor variables being assessed.
The survival was estimated by the Kaplan–Meier method, with the follow-up period starting at the index echocardiogram until study closure, and group differences assessed with the log-rank test. For each parameter, the cut-off was set as the mean value between the group without and with adverse events. A p-value less than 0.05 was considered significant to compute 95% confidence intervals.
2.5. Estimation of a score and matching
Patients with reduced (rLVEF; LVEF ≤ 40%) or mildly reduced (mrLVEF; LVEF 41%–49%) LVEF were classified based solely on echocardiography parameters according to the 2021 ESC Guidelines (26). rLVEF and mrLVEF were labeled together as iLVEF (iLVEF group). All the other patients with normal LVEF (≥50%) were considered as controls. A matching score was computed to select an equal number of control patients to match the HF group. The matching score was based on age, sex, BMI, and blood pressure. Each patient from the iLVEF group was matched to a patient from the control group with the best matching score possible.
3. Results
This study included 131 patients. 5 patients were excluded for technical issues. In total, 126 patients (63 [49; 71] years old, 54% male) were kept for analyses. 96 patients had a normal LVEF and 30 an impaired LVEF (11 mrLVEF and 19 rLVEF). 30 patients from the group with normal LVEF and the highest matching score based on age, sex, BMI, and blood pressure when compared to the HF group were selected as the matched control group. Clinical characteristics, echocardiographic and KCG parameters, HR, and blood pressure of the iLVEF group, and the matched control group are presented in Table 1.
As presented in Table 1, heart rate, interventricular septal thickness in end diastole (IVSd), left atrial diameter, indexed SV, E/A, and maximum velocity of tricuspid regurgitation were comparable between groups. iLVEF patients had lower systolic and diastolic blood pressure (126 [109; 139] and 70 [64; 81] vs. 136 [117; 146] and 80 [70; 82] mmHg, respectively, p < 0.001). Furthermore, the QRS width was significantly larger in the iLVEF group (104.0 [93.0;127.0] vs. 94.0 [87.5;102.0] ms, p = 0.02) with more complete bundle branch block (BBB) morphology (30% vs. 7%, p = 0.01). The QTc duration was also longer in the iLVEF group (411.7 [381.7; 433.2] vs. 375.3 [346; 397.4] ms, p < 0.01). The left ventricular internal diameter at end diastole (LVIDd) was larger in the iLVEF group (59 [52; 66] vs. 49 [45; 55] mm, p < 0.01), and their diastolic parameter E/e' was higher (11 [8.5;13] vs. 9.3 [6.1;12], p < 0.01). A higher proportion of iLVEF was treated with beta-blockers and ACEi (83% vs. 50%, 77% vs. 50%, p < 0.01 and p < 0.05, respectively). Also, the iLVEF group patients had a higher proportion of history of arrhythmia and coronary artery disease (53% vs. 17% and 53% vs. 20%, respectively, p < 0.01).
Baseline patient characteristics within HF group are presented in Supplementary Material.
3.1. KCG group comparison
The BCG was lower in iLVEF compared to the matched control group (3.6 [1.7; 8.9] µJ.s vs. 5.8 [1.4; 15.0] µJ.s, respectively p < 0.03, Figure 3). The SCG did not differ between iLVEF and the matched control group (190 [65; 880] µJ.s and 570 [80; 1,000] µJ.s, respectively, Figure 3).
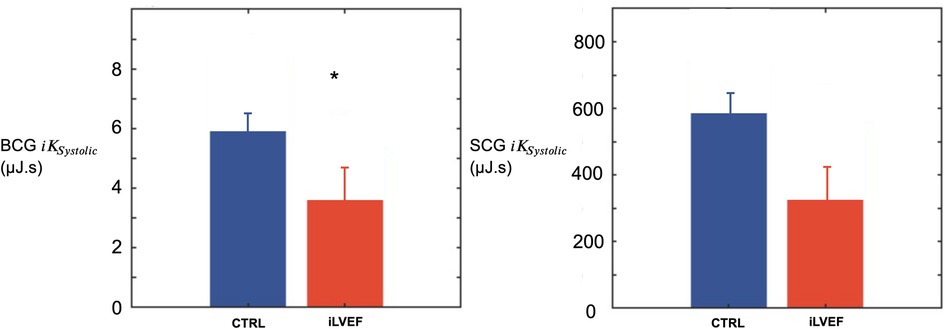
Figure 3. Systolic kinetic energy results. Systolic kinetic energy (mean and standard error of the mean) for BCG and SCG among iLVEF patients and matched controls patients with impaired left ventricular ejection fraction (*: <0.01). CTRL, control; iLVEF, patients with impaired left ventricular ejection fraction.
was found to be lower in the iLVEF group than in the matched control group for both BCG (0.54 [0.35; 0.71] and 0.66 [0.59; 0.81], respectively, p < 0.02, Figure 4) and SCG (0.60 [0.31; 0.79] and 0.73 [0.70; 0.82], respectively, p < 0.01, Figure 4).
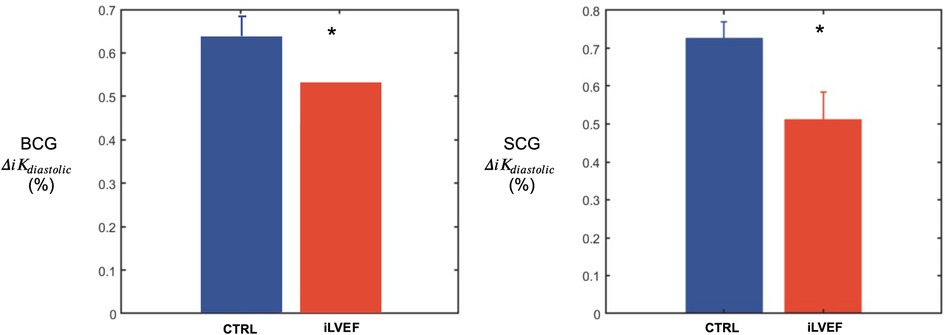
Figure 4. Diastolic kinetic energy results. Diastolic kinetic energy (mean and standard error of the mean) for BCG and SCG among matched controls and iLVEF patients with impaired left ventricular ejection fraction (*: <0.01). CTRL, control; iLVEF, patients with impaired left ventricular ejection fraction.
3.2. Predictors of patients' survival
During a total follow-up of 5 years after first inclusion, 21 participants of the iLVEF group were still alive. Based on multivariate analysis (Table 2), the proportion of patients with iLVEF surviving 4 years was significantly lower in patients with SCG <390 µJ.s compared to SCG >390 µJ.s (log rank p = 0.001) (Figure 5A, Table 2). There was also an observed difference in survival between iLVEF patients with a heart rate >71 bpm and those with a heart rate <71 bpm (log rank p = 0.04, Figure 5B, Table 2). A Cox multivariate model including both SCG and heart rate was generated and showed no significant improvement, as compared to the model including only SCG (p = 0.3). Age, LVEF, chronic kidney disease, and BBB were not significant predictors of survivability in the tested group.
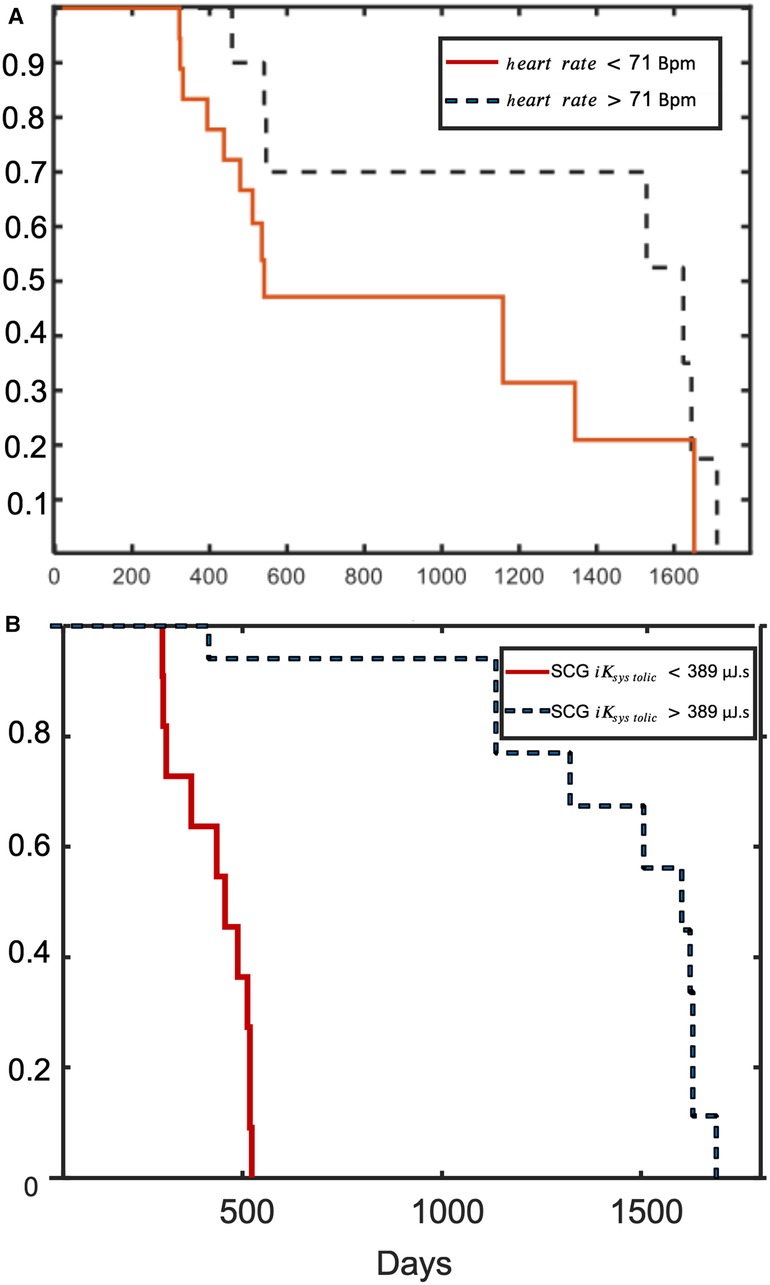
Figure 5. Kaplan–Meier probability of survival. Kaplan–Meier curves of event-free survival according to (A) SCG ; and (B) heart rate.
4. Discussion
In the KINO-HF exploratory study, the comparison of KCG metrics between patients with impaired LVEF and matched control subjects reveals that the iLVEF group is associated with lower values of systolic kinetic energy () and diastolic kinetic energy (). Also, in iLVEF patients, higher values of were associated with a better survival.
4.1. Kinocardiography metrics rationale
Recent studies have shown interest in using intra-ventricular kinetic energy through 4D flow MRI to assess left and right ventricular functions (27, 28). These have shown very promising results allowing a better understanding of the impact of cardiac disease on intra-cardiac hemodynamics but also opening the perspective of new ways to characterize HF.
The aim of KCG is also to compute a kinetic energy but through body surface accelerations and angular rates in a simpler and more indirect way when compared to cardiac MRI derived kinetic energy (MRI KE). In particular, studies have shown that LV systolic MRI KE decreased significantly when comparing controls to patients with myocardial infarction with decreased EF (29, 30). Moreover, others have shown that most flow components of the late (A) diastolic MRI KE increased in HF patients with reduced LVEF in comparison to healthy subjects (31). Inspired by these results, this study introduces two metrics based on KCG: (1) systolic kinetic energy, named , analog to LV systolic MRI KE and (2) diastolic gradient kinetic energy, named , reflecting the E and A MRI KE relative distribution.
4.2. Systolic kinetic energy
BCG was significantly lower in patients from the iLVEF group, reflecting the reduced amount of force developed by the ventricles in these patients. This is in line with the reduction of LV systolic MRI KE as detected by cardiac MRI in patients with impaired LVEF (31), or when comparing controls to patients with myocardial infarction with decreased EF in experimental and clinical settings (29, 30, 32). However, in this study, HF patients and matched control showed comparable resting indexed SV (Table 1). Therefore, the correlation between BCG and SV found in a previous study in healthy participants during a dobutamine-induced increase in contractility (19) is not extensible to pathological cases. The correlation between BCG and SV might mostly be accurate for intra-patient follow-up, as described in two studies: (1) during a cardiac deconditioning measured by cardiac MRI due to long-duration head-down tilt bed rest, where a significant decrease of this metric was shown (33); and (2) in a recent study showing that this metric followed significantly the change in the cardiac hemodynamic load in HF patients (34).
Interestingly, a study showed the high sensitivity of BCG to beta adrenergic stimulation (19), therefore the higher proportion of beta blockers in the HF group compared to the matched control group might have contributed to the decrease in BCG in this study.
The same was not observed for SCG . This might be explained by the discrepancy in the proportion of patients with ventricular dilatation: 41% (iLVEF) vs. 7% (control group) had a LVIDd larger than 60 mm (p < 0.01, Table 1). In our cohort, SCG was found to be much higher in patients with ventricular dilatation than in the other patients with impaired LVEF (380 [80; 980] µJ.s vs. 84 [55; 210] µJ.s, p < 0.0001, Table 3). Since SCG measures a larger cardiac mass displacement in patients with dilated left ventricle, this confounding factor might have increased the SCG values in the iLVEF group leading to values comparable to the control group.
Interestingly, BCG was also found to be higher in patients with ventricular dilatation than in the other iLVEF patients (10 [3.9; 36] µJ.s vs. 2.2 [0.6; 3.9] µJ.s, p < 0.01, Table 3). As such, the ventricular dilation can impede the expected decrease of SCG in the iLVEF group but not the decrease of BCG. The essence of BCG is to measure micromovements of the body in reaction to blood flow through the vasculature, mainly the aorta, making this metric less sensitive to mechanical LV motion and LV dilatation (35).
Furthermore, in this study, a decreased SCG was shown to be a significant predictor of all-cause mortality among patients with impaired LVEF. Similarly, on datasets of nearly the same size, others have shown that some features of the SCG signal can be extracted to predict HF readmission, one of which is the SCG amplitudes (36). However, our result seems to conflict with the elevated values of high in patients with dilated ventricles, who are known to have a worse prognosis if associated with HFrEF. This emphasizes that this result was obtained in a relatively small population with varying LV morphology within the iLVEF group, and larger studies are needed to be able to conclude the predictability of .
4.3. Diastolic kinetic energy
For both BCG and SCG, was found to be significantly decreased in the iLVEF group when compared to the matched control. In contrast, diastolic function parameters such as E/A, or tricuspid regurgitation maximum velocity were equally distributed in both groups. However, as expected, E/e' was higher in the iLVEF group (11 [8.5;13] % vs. 9.3 [6.1;12] %, p < 0.01). sought to reflect energy distribution along the diastole (passive and active filling). MRI studies have shown that HF patients with impaired LV function are characterized by altered diastolic flow routes through the LV and impaired preservation of MRI KE during late diastole (31). In this phase of the heart cycle corresponding to atrial systole, Eriksson et al. further described high MRI KE in HF patients reflecting impaired active relaxation of the myocardium caused by a less compliant myocardium (31). This is in line with our results, where we report a significant increase in the difference of energy developed by the heart during the diastole ().
The differences observed by KCG in the diastolic parameters between iLVEF and control patients might be partly due to reduced LV compliance in patients with impaired LV function. Furthermore, we decomposed the gradient in its early () and late () components normalized by the total diastolic kinetic energy (Table 1). The early diastole energy decreased while the late diastole energy increased in the iLVEF group and thus leading to a lower diastolic gradient (). This aligns with the increase of intracardiac filling pressures during the late diastole in the iLVEF group.
4.4. Limitations
The present work presents some limitations worth noting. The definitions of the systolic and diastolic kinetic energy parameters are inherently an approximation based on ECG detection. Furthermore, we expect a slight delay of the systolic and diastolic events acquired for the BCG energies compared to the ECG segmentation. Moreover, the possibility of using KCG in specific clinical scenarios, such as atrial fibrillation or intracardiac devices, is still to be investigated. Indeed, patients with pacemaker were excluded from this study to avoid the uncertainties of ventricular asynchrony induced by right ventricular stimulation on KCG. However, they should not be excluded in future studies.
The control population was well matched with respect to general characteristics of population such as gender, BMI, and age but presented different characteristics regarding medical treatment and cardiac pathologies such as BBB. However, the control patients were recruited at the cardiology department, and therefore should represent a relevant sample of the population that would need to be discriminated and ruled out of a HF diagnosis. Eligible patients with left BBB recruited in this study were later implanted with a cardiac resynchronization device. The fact that larger LVIDd among iLVEF patients increased contrasts with the results showing that increased predicts poor prognosis. This calls for larger cohorts to be studied to better understand of the effect of HF etiology on the metric.
In this study, both, and have shown group differences when comparing the group with iLVEF to the matched control group. However, the metrics values overlap between both groups, and it is therefore not possible to define a single cut-off value achieving good discrimination between iLVEF and controls. A joint parameter combining BCG and SCG metrics could be developed to achieve this. To a further extent, machine learning algorithms combining more than two metrics could be developed on larger datasets and might provide accurate discrimination between these two groups. Further studies are needed to confirm this potential.
We acknowledge that LVEF is simply one parameter of systolic function among others, and that a normal LVEF does not exclude systolic dysfunction, as in cardiac amyloidosis or when considering the more subtle systolic dysfunction seen in HF patients with preserved ejection fraction.
5. Conclusion
This study demonstrates that patients with iLVEF are associated with lower values of and when compared to a matched control group. In addition, in iLVEF patients, seems to be an independent predictor of survivability. Warranting further studies to evaluate KCG accuracy in different clinical settings and in a blind setup, these scalar metrics might be useful for screening for impaired LVEF.
Data availability statement
The original contributions presented in the study are included in the article/Supplementary Materials, further inquiries can be directed to the corresponding author/s.
Ethics statement
The studies involving human participants were reviewed and approved by local Ethics Committee (Brugmann University Hospital—CCB: B077201732405). The patients/participants provided their written informed consent to participate in this study.
Author contributions
ED, AA, and PB conceived the idea and the design of the study. AH has full access to all the data in the study. AH and JR developed the signal processing algorithms for the ECG and KCG signals. AH performed the statistical analysis. ED and MM enrolled the participants and took outcome measurements. ED drafted the manuscript. MM, AA, AH, JR and PB revised critically the manuscript for important intellectual content. All the authors did proofreading and corrections for this manuscript. All authors contributed to the article and approved the submitted version.
Funding
This study and AH are supported by a grant from the European Space Agency and the Belgian Federal Scientific Policy Office (PRODEX PEA 4000110826) and a grant from the Fonds Erasme. JR is supported by the Fonds de la Recherche Scientifique (Mandat Aspirant F.R.S.—FNRS FC 29801).
Acknowledgments
The authors would like to acknowledge the contribution of Pierre-François Migeotte.
Conflict of interest
AH is consultant for HeartKinetics, a MEDTECH company. The remaining authors declare that the research was conducted in the absence of any commercial or financial relationships that could be construed as a potential conflict of interest.
Publisher's note
All claims expressed in this article are solely those of the authors and do not necessarily represent those of their affiliated organizations, or those of the publisher, the editors and the reviewers. Any product that may be evaluated in this article, or claim that may be made by its manufacturer, is not guaranteed or endorsed by the publisher.
Supplementary material
The Supplementary Material for this article can be found online at: https://www.frontiersin.org/articles/10.3389/fcvm.2023.1096859/full#supplementary-material.
References
1. Groenewegen A, Rutten FH, Mosterd A, Hoes AW. Epidemiology of heart failure. Eur J Heart Fail. (2020) 22:1342–56. doi: 10.1002/ejhf.1858
2. Heidenreich PA, Fonarow GC, Opsha Y, Sandhu AT, Sweitzer NK, Warraich HJ, et al. Economic issues in heart failure in the United States. J Card Fail. (2022) 28:453–66. doi: 10.1016/j.cardfail.2021.12.017
3. Jackson SL, Tong X, King RJ, Loustalot F, Hong Y, Ritchey MD. National burden of heart failure events in the United States, 2006 to 2014. Circ Heart Fail. (2018) 11:e004873. doi: 10.1161/CIRCHEARTFAILURE.117.004873
4. Blumer V, Mentz RJ, Sun JL, Butler J, Metra M, Voors AA, et al. Prognostic role of prior heart failure hospitalization among patients hospitalized for worsening chronic heart failure. Circ Heart Fail. (2021) 14:e007871. doi: 10.1161/CIRCHEARTFAILURE.120.007871
5. Anon. Rehospitalization for heart failure: predict or prevent? Available at: https://pubmed.ncbi.nlm.nih.gov/22825412/ (Accessed April 27, 2022).
6. Solomon SD, Dobson J, Pocock S, Skali H, McMurray JJV, Granger CB, et al. Influence of nonfatal hospitalization for heart failure on subsequent mortality in patients with chronic heart failure. Circulation. (2007) 116:1482–7. doi: 10.1161/CIRCULATIONAHA.107.696906
7. Verdejo HE, Ferreccio C, Castro PF. Heart failure in rural communities. Heart Fail Clin. (2015) 11:515–22. doi: 10.1016/j.hfc.2015.07.011
8. Starr I. The relation of the ballistocardiogram to cardiac function. Am J Cardiol. (1958) 2:737–47. doi: 10.1016/0002-9149(58)90271-6
9. Castiglioni P, Faini A, Parati G, Di Rienzo M. Wearable seismocardiography. Annu Int Conf IEEE Eng Med Biol Soc. (2007) 2007:3954–7. doi: 10.1109/IEMBS.2007.4353199
10. Jaakkola Jussi, Jaakkola Samuli, Lahdenoja Olli, Hurnanen Tero, Koivisto Tero, Pänkäälä M, et al. Mobile phone detection of atrial fibrillation with mechanocardiography. Circulation. (2018) 137:1524–7. doi: 10.1161/CIRCULATIONAHA.117.032804
11. Rajput JS, Sharma M, Kumar TS, Acharya UR. Automated detection of hypertension using continuous wavelet transform and a deep neural network with ballistocardiography signals. Int J Environ Res Public Health. (2022) 19:4014. doi: 10.3390/ijerph19074014
12. Aydemir VB, Fan J, Dowling S, Inan OT, Rehg JM, Klein L. Ballistocardiography for ambulatory detection and prediction of heart failure decompensation. J Card Fail. (2018) 24:S116. doi: 10.1016/j.cardfail.2018.07.425
13. Zhang L, Cai P, Deng Y, Lin J, Wu M, Xiao Z, et al. Using a non-invasive multi-sensor device to evaluate left atrial pressure: an estimated filling pressure derived from ballistocardiography. Ann Transl Med. (2021) 9:1587. doi: 10.21037/atm-21-5161
14. Shandhi MMH, Fan J, Heller AJ, Etemadi M, Inan OT, Klein L. Seismocardiography can assess cardiopulmonary exercise test parameters in patients with heart failure. J Card Fail. (2018) 24:S124–5. doi: 10.1016/j.cardfail.2018.07.446
15. Balali P, Rabineau J, Hossein A, Tordeur C, Debeir O, van de Borne P. Investigating cardiorespiratory interaction using ballistocardiography and seismocardiography—a narrative review. Sensors. (2022) 22:9565. doi: 10.3390/s22239565
16. Taebi A, Solar BE, Bomar AJ, Sandler RH, Mansy HA. Recent advances in seismocardiography. Vibration. (2019) 2:64–86. doi: 10.3390/vibration2010005
17. Huntsman LL, Stewart DK, Barnes SR, Franklin SB, Colocousis JS, Hessel EA. Noninvasive Doppler determination of cardiac output in man. Clinical validation. Circulation. (1983) 67:593–602. doi: 10.1161/01.CIR.67.3.593
18. Gonçalves A, Marcos-Alberca P, Sogaard P, Zamorano JL. Assessment of systolic function. Oxford: Oxford University Press (2011). p. 117–34.
19. Hossein A, Mirica DC, Rabineau J, Rio JID, Morra S, Gorlier D, et al. Accurate detection of dobutamine-induced haemodynamic changes by kino-cardiography: a randomised double-blind placebo-controlled validation study. Sci Rep. (2019) 9:1–11. doi: 10.1038/s41598-019-46823-3
20. Hossein A, Rabineau J, Gorlier D, Pinki F, van de Borne P, Nonclercq A, et al. Effects of acquisition device, sampling rate, and record length on kinocardiography during position-induced haemodynamic changes. Biomed Eng Online. (2021) 20:3. doi: 10.1186/s12938-020-00837-5
21. Hossein A, Rabineau J, Gorlier D, Del Rio JIJ, van de Borne P, Migeotte PF, et al. Kinocardiography derived from ballistocardiography and seismocardiography shows high repeatability in healthy subjects. Sensors. (2021) 21:815. doi: 10.3390/s21030815
22. Morra S, Hossein A, Rabineau J, Gorlier D, Racape J, Migeotte PF, et al. Assessment of left ventricular twist by 3D ballistocardiography and seismocardiography compared with 2D STI echocardiography in a context of enhanced inotropism in healthy subjects. Sci Rep. (2021) 11:683. doi: 10.1038/s41598-020-79933-4
23. Morra S, Hossein A, Gorlier D, Rabineau J, Chaumont M, Migeotte PF, et al. Modification of the mechanical cardiac performance during end-expiratory voluntary apnea recorded with ballistocardiography and seismocardiography. Physiol Meas. (2019) 40:105005. doi: 10.1088/1361-6579/ab4a6a
24. Morra S, Hossein A, Gorlier D, Rabineau J, Chaumont M, Migeotte PF, et al. Ballistocardiography and seismocardiography detection of hemodynamic changes during simulated obstructive apnea. Physiol Meas. (2020) 41:065007. doi: 10.1088/1361-6579/ab924b
25. Morra S, Gauthey A, Hossein A, Rabineau J, Racape J, Gorlier D, et al. Influence of sympathetic activation on myocardial contractility measured with ballistocardiography and seismocardiography during sustained end-expiratory apnea. Am J Physiol Regul Integr Comp Physiol. (2020) 319:R497–506. doi: 10.1152/ajpregu.00142.2020
26. McDonagh TA, Metra M, Adamo M, Gardner RS, Baumbach A, Böhm M, et al. ESC guidelines for the diagnosis and treatment of acute and chronic heart failure: developed by the task force for the diagnosis and treatment of acute and chronic heart failure of the European society of cardiology (ESC) with the special contribution of the heart failure association (HFA) of the ESC. Eur Heart J. (2021) 2021(42):3599–726. doi: 10.1093/eurheartj/ehab368
27. Carlsson M, Heiberg E, Toger J, Arheden H. Quantification of left and right ventricular kinetic energy using four-dimensional intracardiac magnetic resonance imaging flow measurements. Am J Physiol Heart Circ Physiol. (2012) 302:H893–900. doi: 10.1152/ajpheart.00942.2011
28. Anon. Left ventricular blood flow kinetic energy assessment by 4D flow cardiovascular magnetic resonance: a systematic review of the clinical relevance. Available at: https://pubmed.ncbi.nlm.nih.gov/32927744/ (Accessed March 7, 2022).
29. Garg P, Crandon S, Swoboda PP, Fent GJ, Foley JRJ, Chew PG, et al. Left ventricular blood flow kinetic energy after myocardial infarction - insights from 4D flow cardiovascular magnetic resonance. J Cardiovasc Magn Reson. (2018) 20:61. doi: 10.1186/s12968-018-0483-6
30. Garg P, van der Geest RJ, Swoboda PP, Crandon S, Fent GJ, Foley JRJ, et al. Left ventricular thrombus formation in myocardial infarction is associated with altered left ventricular blood flow energetics. Eur Heart J Cardiovasc Imaging. (2019) 20:108–17. doi: 10.1093/ehjci/jey121
31. Eriksson J, Bolger AF, Ebbers T, Carlhäll C-J. Four-dimensional blood flow-specific markers of LV dysfunction in dilated cardiomyopathy. Eur Heart J Cardiovasc Imaging. (2013) 14:417–24. doi: 10.1093/ehjci/jes159
32. Morra S, Pitisci L, Su F, Hossein A, Rabineau J, Racape J, et al. Quantification of cardiac kinetic energy and its changes during transmural myocardial infarction assessed by multi-dimensional seismocardiography. Front Cardiovasc Med. (2021) :8. doi: 10.3389/fcvm.2021.603319
33. Rabineau J, Hossein A, Landreani F, Haut B, Mulder E, Luchitskaya E, et al. Cardiovascular adaptation to simulated microgravity and countermeasure efficacy assessed by ballistocardiography and seismocardiography. Sci Rep. (2020) 10:17694. doi: 10.1038/s41598-020-74150-5
34. Herkert C, Migeotte P-F, Hossein A, Spee RF, Kemps HMC. The kinocardiograph for assessment of changes in haemodynamic load in patients with chronic heart failure with reduced ejection fraction. ESC Heart Fail. (2021) 8:4925–32. doi: 10.1002/ehf2.13522
35. Rabineau J, Nonclercq A, Leiner T, van de Borne P, Migeotte P-F, Haut B. Closed-loop multiscale computational model of human blood circulation. Applications to ballistocardiography. Front Physiol. (2021) :12. doi: 10.3389/fphys.2021.734311
Keywords: heart failure, reduced ejection fraction, e-health, kinocardiography, seismocardiography, ballistocardiography, point-of-care screening, aid-to-diagnosis
Citation: De Keyzer E, Hossein A, Rabineau J, Morissens M, Almorad A and van de Borne P (2023) Non-invasive cardiac kinetic energy distribution: a new marker of heart failure with impaired ejection fraction (KINO-HF). Front. Cardiovasc. Med. 10:1096859. doi: 10.3389/fcvm.2023.1096859
Received: 12 November 2022; Accepted: 10 April 2023;
Published: 2 May 2023.
Edited by:
Matteo Cameli, University of Siena, ItalyReviewed by:
Kouhyar Tavakolian, University of North Dakota, United StatesFarzad Khosrow-khavar, Simon Fraser University, Canada
© 2023 De Keyzer, Hossein, Rabineau, Morissens, Almorad and van de Borne. This is an open-access article distributed under the terms of the Creative Commons Attribution License (CC BY). The use, distribution or reproduction in other forums is permitted, provided the original author(s) and the copyright owner(s) are credited and that the original publication in this journal is cited, in accordance with accepted academic practice. No use, distribution or reproduction is permitted which does not comply with these terms.
*Correspondence: Alexandre Almorad YWxleGFuZHJlLmFsbW9yYWRAdXpicnVzc2VsLmJl
†These authors have contributed equally to this work
Abbreviations ACEi, angiotensin-converting enzyme inhibitor; BBB, bundle branch block; BCG, ballistocardiography; BMI, body mass index; CP, cardiac phase; CO, cardiac output; COPD, chronic obstructive pulmonary disease; HF, heart failure; iLVEF, impaired left ventricular ejection fraction; IVSd, interventricular septal end diastole; K, kinetic energy; LA diam, left atrial diameter; KCG, kinocardiography; LV, left ventricle; LVEF, left ventricular ejection fraction; LVIDd, left ventricular internal diameter end diastole; QTc, QT interval corrected; SCG, seismocardiography; SGLT2i, SGLT2 inhibitor; SV, stroke volume; TR vmax, tricuspid regurgitation maximum velocity; VKA, vitamin K antagonist.