Editorial: Inflammatory factors in coronary heart disease: mechanism, diagnosis, and therapy
- 1Department of Cardiology, Cardiovascular Centre, Beijing Friendship Hospital, Capital Medical University, Beijing, China
- 2Department of Cardiology, Peking University Third Hospital, Beijing, China
- 3Key Laboratory of Cardiovascular Molecular Biology and Regulatory Peptides, Ministry of Health, Beijing, China
- 4Key Laboratory of Molecular Cardiovascular Sciences, Ministry of Education, Beijing, China
- 5Department of Neurology, Peking University International Hospital, Beijing, China
- 6Department of Gastroenterology and Hepatology, The First Medical Center, Chinese People’s Liberation Army General Hospital, Beijing, China
Background: Remote ischemic postconditioning (RIPostC) induced by brief episodes of the limb ischemia is a potential therapeutic strategy for myocardial ischemia/reperfusion injury, achieved by reducing cardiomyocyte death, inflammation and so on. The actual mechanisms underlying cardioprotection conferred by RIPostC remain unclear. Exploring gene expression profiles in myocardium at transcriptional level is helpful to deepen the understanding on the cardioprotective mechanisms of RIPostC. This study aims to investigate the effect of RIPostC on gene expressions in rat myocardium using transcriptome sequencing.
Methods: Rat myocardium samples from the RIPostC group, the control group (myocardial ischemia/reperfusion group) and the sham group were performed transcriptome analysis using RNA sequencing. The levels of cardiac IL-1β, IL-6, IL-10 and TNFα were analyzed by Elisa. The expression levels of candidate genes were verified by qRT-PCR technique. Infarct size was measured by Evans blue and TTC staining. Apoptosis was assessed by TUNEL assays and caspase-3 levels were detected using western blotting.
Results: RIPostC can markedly decrease infarct size and reduce the levels of cardiac IL-1β, IL-6 and increase the level of cardiac IL-10. This transcriptome analysis showed that 2 genes were up-regulated (Prodh1 and ADAMTS15) and 5 genes (Caspase-6, Claudin-5, Sccpdh, Robo4 and AABR07011951.1) were down-regulated in the RIPostC group. Go annotation analysis showed that Go terms mainly included cellular process, metabolic process, cell part, organelle, catalytic activity and binding. The KEGG annotation analysis of DEGs found only one pathway, amino acid metabolism, was up-regulated. The relative mRNA expression levels of ADAMTS15, Caspase-6, Claudin-5 and Prodh1 were verified by qRT-PCR, which were consistent with the RNA-seq results. In addition, the relative expression of ADAMTS15 was negatively correlated with the level of cardiac IL-1β (r = −0.748, P = 0.005) and positively correlated with the level of cardiac IL-10 (r = 0.698, P = 0.012). A negative correlation statistical trend was found between the relative expression of ADAMTS15 and the level of cardiac IL-6 (r = −0.545, P = 0.067).
Conclusions: ADAMTS15 may be a potential inflammation-related gene in regulation of cardioprotection conferred by remote ischemic postconditioning and a possible therapeutic target for myocardial ischemia reperfusion injury in the future.
Introduction
In recent years, early reperfusion therapy has become the most effective treatment for patients with acute myocardial infarction (AMI). However, as a side effect of early reperfusion treatment, ischemia reperfusion (IR) injury almost inevitably causes cardiomyocyte death or no reflows, seriously affecting the prognosis of patients with AMI (1, 2). Therefore, it is necessary to seek effective methods to alleviate IR injury at present. Remote ischemic conditioning (RIC), referring to repeated and transient ischemia/reperfusion episodes of distant organs such as limbs, could effectively reduce myocardial IR injury. In 1993, Przyklenk et al. found that remote ischemic preconditioning (RIPC), performed before prolonged myocardial ischemia, could protect the heart against myocardial IR injury (3). As the time of acute coronary occlusion is unpredictable, the application of RIPC has been greatly limited. Therefore, RIC implemented immediately following myocardial reperfusion, called remote ischemic postconditioning (RIPostC), attracts the attentions of researchers. In 2013, Crimi et al. divided 100 patients with AMI receiving primary percutaneous coronary intervention (PCI) into RIPostC group together with PCI control group, and found that the area under the CK-MB curve decreased significantly in RIPostC group (4). Furthermore, a series of clinical and basic studies confirmed the cardioprotective effect of RIPostC, which made it a feasible strategy in treatment of AMI.
At present, the mechanism of cardioprotection conferred by RIPostC is not clear (5, 6). Previous studies have shown that RIC can induce cardioprotective protein factors, such as hypoxia-inducible factor 1α (HIF-1α), stroma cell-derived factor-1 (SDF-1), nitrite, etc. These factors are usually up-regulated in heart tissue and can activate signal transduction pathways in myocardium, finally lead to significant cardioprotection (7–10). In view of current research highlights, the inflammation hypothesis of cardioprotection becomes an attractive target. RIC has been proved to play a significant anti-inflammatory role in myocardial IR injury and relate to decreased levels of the pro-inflammatory cytokines, such as interleukin-1β (IL-1β) and interleukin-6 (IL-6), after myocardial reperfusion (11). Cai et al. found RIPC can induce the increase of interleukin-10 (IL-10) which was mostly considered as a protective anti-inflammatory cytokine in plasma and heart, and then activate the protective signal pathway in the heart to induce the protection of myocardial IR injury (12–14).
In addition, previous studies on the mechanism of cardioprotection conferred by RIC are mainly focusing on the levels of protein expressions (15–17). The proteomics technology played a prominent role in revealing the mechanism of cardioprotection conferred by RIC. In 2012, Michele hepponstall et al. carried out proteomic analysis on the plasma of 5 healthy volunteers treated with limb RIPC. They found that the expression of 6 proteins changed by using 2-dimensional difference in gel electrophoresis and the expressions of 48 proteins changed by using liquid chromatography-mass spectrometry (LC-MS). The expression of apolipoprotein A-I, fibrin and fibrinogen was changed in the above two technologies (18). Further studies showed that apolipoprotein A-I up-regulated in plasma and heart tissue and played a cardioprotective role in RIPC (19). Transcriptome research is also an important method of functional genome research, which can explore gene expressions and functions more efficiently but is insufficient in RIC research (20, 21). To clarify the effects of RIPostC on gene expressions at transcriptional level in myocardium using transcriptome analysis is of great significance to figure out the mechanism of RIPostC. Therefore, this study aims to investigate the effect of RIPostC on gene expressions in rat myocardium using RNA sequencing.
Materials and methods
Animals
The animal experiment was approved by the Animal Care Committee of Peking University. This study was performed in accordance with the Guide for the Care and Use of Laboratory Animals published by the US National Institutes of Health (NIH Publication No. 85-23, revised 1996). Eighteen male Sprague-Dawley (SD) rats (22) with body weights of 250–300 g were used in this study and were divided into three groups randomly (n = 6):
RIPostC: 30 min of myocardial ischemia followed by 3 h of reperfusion. Four cycles of 5 min ischemia and 5 min reperfusion on lower limbs were applied immediately after 30 min myocardial ischemia.
Con (Control): the same treatment as RIPC, except that no ischemia on lower limbs was applied.
Sham: sham operation was implemented on rats.
Myocardial IR model
SD rats were anesthetized and then subjected to 30 min myocardial ischemia by ligation of left anterior descending coronary artery, followed with 3 h reperfusion by releasing the ligation to simulate myocardial IR.
The implementation of RIC
Tourniquets were used to bind both lower limbs of rats (22), causing bilateral femoral artery occlusion. After the lower limbs ischemia lasting for 5 min, the tourniquets were relaxed and the lower limbs were reperfusion for 5 min, which was repeated for 4 times. The signs of successful femoral artery occlusion were pale skin, decreased body temperature and loss of pulse. Reperfusion is marked by rapid hyperemia and redness of the distal limb, with return of body temperature and presence of pulse.
Assessment of myocardial infarct size and inflammatory level
After myocardial reperfusion in rats, the anterior descending coronary artery was ligated at the original ligation position again. 2 ml of 1% Evans blue solution was injected to dye the non-ischemic myocardium into blue. The heart was frozen and 4–5 slices of frozen heart tissue were quickly cut. The slices were placed into 1% TTC-PBS buffer and incubated in a constant temperature water bath at 37°C for 20 min in dark. Then heart slices were transferred into 10% formalin solution. The infarction area was gray-white, the non-ischemic area was blue, and the ischemic risk area was red and white. Image J software was used for analysis and myocardial infarction area (%) was valued by infarction area/risk area. Rat troponin I (TnI) in plasma and cardiac IL-1β, IL-6, IL-10 and TNFα were analyzed by enzyme linked immunosorbent assay (Elisa) using commercial kits according to the manufacturer's protocol (Abcam, USA).
Terminal deoxynucleotidyl transferase mediated dUTP nick end labelling (TUNEL) assay
For the detection of TUNEL-positive cells, the In Situ Cell Death Detection Kit (Roche, CH) was used according to the manufacturer's instructions.
Western blotting
Western blotting was carried out according to the previous literature description (12). Bands were normalized to eukaryotic translation initiation factor 5 (eIF5) expression.
Transcriptome sequencing
Rat myocardium samples from the RIPostC group (n = 3) and the control group (n = 3) were used for transcriptome analysis by RNA sequencing. Trizol (Invitrogen, USA) was used to extract total RNA from tissue samples. The concentration and purity of RNA were detected using Nanodrop2000. The integrity of RNA was measured by agarose gel electrophoresis, and the value of RNA integrity was acquired using Agilent2100. The cDNA library was established according to the instructions of Illumina TruseqTM RNA sample prep kit. The cDNA library was then sequenced using Illumina novaseq 6000.
Quantitative reverse transcription PCR (qRT-PCR)
Total RNA was extracted from tissues using Trizol (Invitrogen, USA). A total of 2μg RNA were transcribed into cDNA using PrimeScript™ RT Reagent Kit (TAKARA, Japan) according to the manufacturer's instructions. Amplification was performed using SYBR Green Real Time PCR Master Mix (TOYOBO, Japan). The sequences of primers are shown in Table 1. The relative amount of mRNA was calculated by 2−ΔΔCT method. We used α-tubulin as a housekeeping gene, and all reactions were executed in triplicate for the 12 samples (n = 6 for RIPostC group and Con group).
Bioinformatic analysis
Raw reads were processed with fastp software (version 0.19.5). Clean reads were obtained after removal of reads containing poly-N and low-quality reads. Mapped reads were acquired by comparison of clean reads with reference genome Rattus_Norvegicus (Rnor_6.0) using hisat software (version 2.1.0). Then mapped reads were assembled using StringTie (version 2.1.2) software.
The genes/transcripts were compared with NR (version 2020.06), GO (version 2020.0628) and KEGG (version 2020.07) databases, and the annotation of each database was statistically analyzed. TPM (transcripts per million reads) was obtained by RSEM (version 1.3.3) software to detect the expression levels of genes and transcripts. Venn analysis was performed to evaluate the common and unique expressed genes/transcripts. Principal Component Analysis (PCA) was carried out to access the relationship and variation between samples using R (version 3.3.1) software package. DESeq2 (Version 1.24.0) software was used to identify differentially expressed genes (DEGs). An adjusted P-value <0.05 and fold change >2 or fold change <0.5 were considered as thresholds for significantly differential expressions (23). The volcano map and heatmap for DEGs was conducted by R (version 3.3.1). GO and KEGG annotation for DEGs was completed using R (version 3.3.1) software package.
Statistical analysis
The data are expressed in mean ± standard error (SE). R (version 3.3.1) software and Graphpad Prism 8.0 (La Jolla, CA, USA) were used for statistical analysis. Variables were tested for normal distribution using the Kolmogorov–Smirnov test and Q–Q plots. Student's t test was used to compare variables with normal distribution and the Mann–Whitney U test was used to compare variables without a normal distribution. P < 0.05 was considered to be statistically significant. Pearson's correlation was used to investigate the association between the relative expression of ADAMTS15 (2−ΔCT method) and cardiac inflammatory indicators.
Results
RIPostC induce protection against myocardial IR injury in rat model
Rats were subjected to RIPostC immediately after prolonged myocardial ischemia (Figure 1A). We assessed the cardioprotective effect of RIPostC by detecting myocardial infarct area in myocardial IR model using Evans blue-TTC staining method. RIPostC markedly reduced infarct size compared with the Con group (Figures 1B,C). Besides, the level of plasma TnI significantly decreased in the RIPostC group (Figure 1D). In addition, compared to the Con group, the number of TUNEL-positive cells significantly decreased in the RIPostC group, and the level of cleaved-caspase 3 significantly downregulated in the RIPostC group, showing that RIPostC could markedly suppress cardiac apoptosis during myocardial IR (Figure 2 and Supplementary Figure S1). These data illustrated that RIPostC can confer powerful protection against myocardial IR injury.
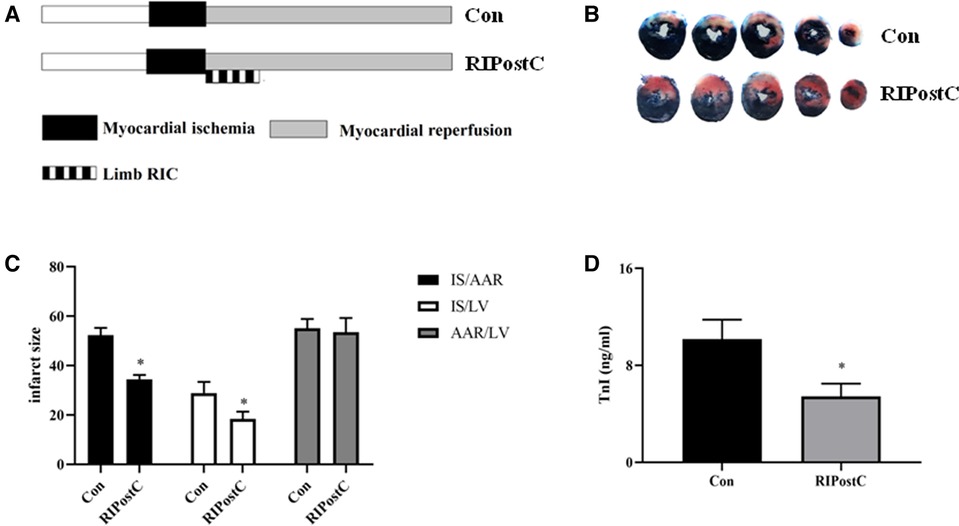
Figure 1. RIPostC significantly reduced cardiac injury in rat myocardial IR model. (A) The protocol of experimental design. (B,C) Evans blue and TTC staining. Representative five heart slices stained by Evans blue and TTC in two groups. IS, infarct size; AAR, the ischemic area at risk; LV, left ventricle. (D) The level of plasma TnI. *Represents P < 0.05, n = 6 for each group.
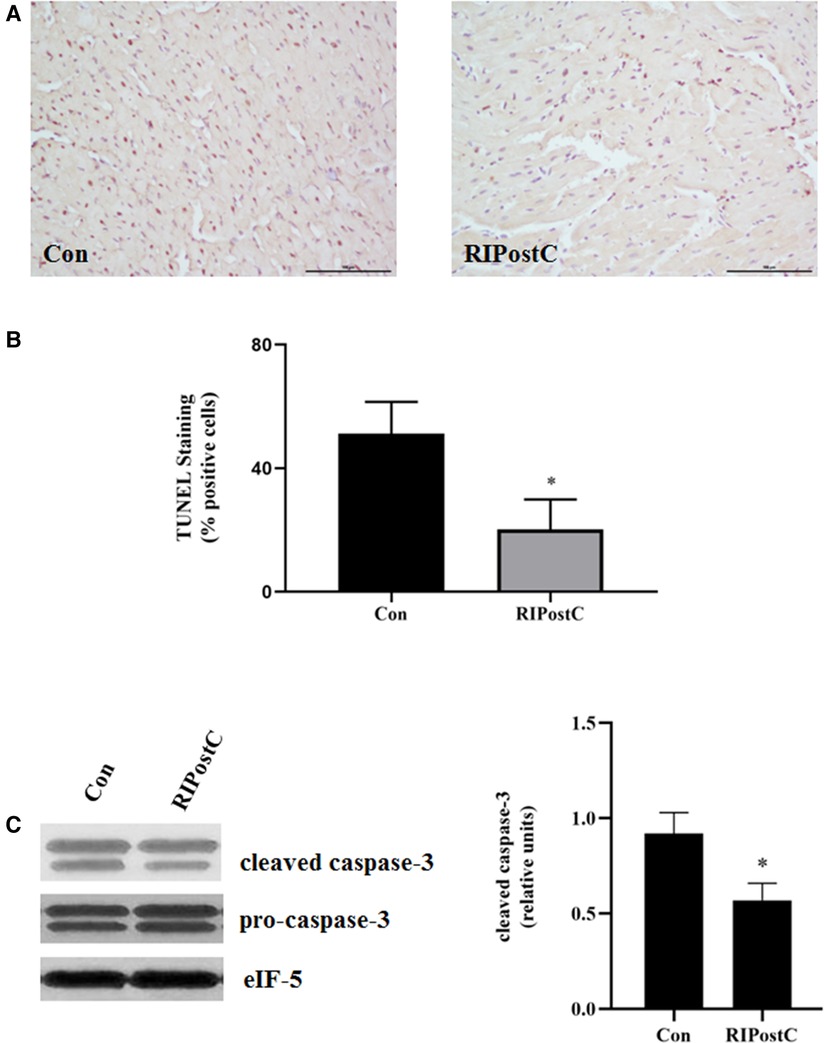
Figure 2. RIPostC significantly reduced the apoptosis of heart. (A) Representative myocardial apoptosis detected by TUNEL assay. (B) The percentage of TUNEL-positive cells in the total cells. Scale bar: 100 µm. *Represents P < 0.05, n = 6 for each group. (C) Detection of caspase-3 levels in heart after RIPostC using western blotting.
RIPostC significantly reduce cardiac inflammation in myocardial IR injury
Compared to the Con group, the levels of cardiac IL-1β and IL-6 significantly decreased in the RIPostC group and the level of cardiac IL-10 significantly elevated (Figure 3 and Supplementary Figure S2). These data showed that RIPostC can play a powerful anti-inflammatory role in myocardial IR injury.
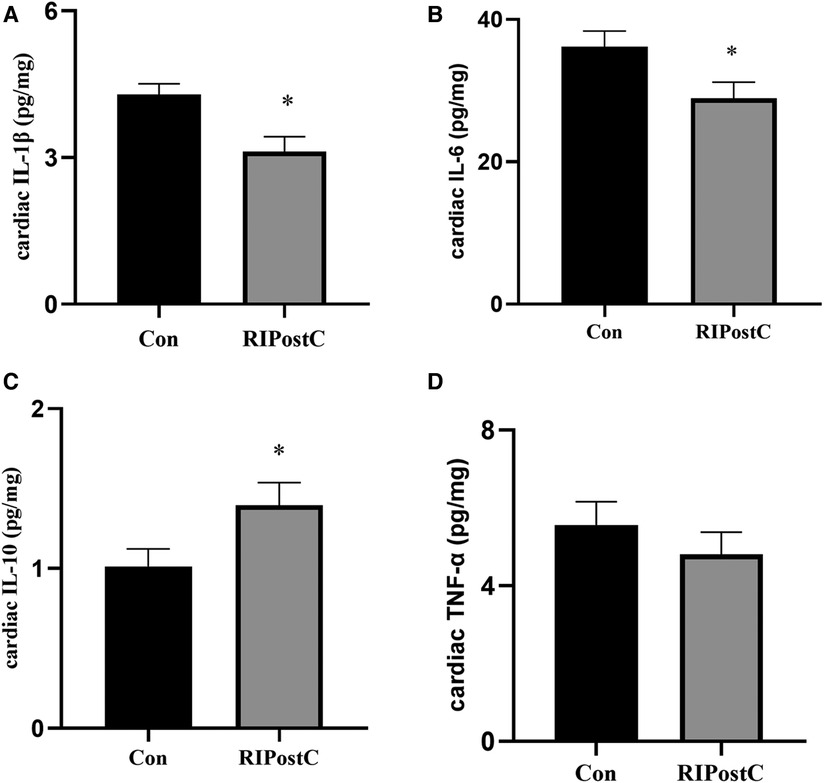
Figure 3. The comparison of the levels of cardiac inflammatory factors between the Con and the RIPostC group. (A–D) The comparison of cardiac IL-1β, IL-6, IL-10 and TNFα levels respectively in the RIPostC and the Con group. *Represents P < 0.05, n = 6 for each group.
Expression differences between the Con and the RIPostC group
The transcriptome analysis of 6 samples was completed, the clean reads of each sample was more than 7.26 GB, and the percentage of Q30 base was >94.38%. The clean reads of each sample were mapped with the specified reference genome, and the alignment rate was >95.29%. 11,493 known genes were expressed in the Con group as well as the RIPostC group, while 287 and 290 genes were only expressed in the Con group or the RIPostC group respectively (Figure 4A). PCA results showed that differences existed in a certain extent on gene expression patterns between the two groups (Figure 4B).
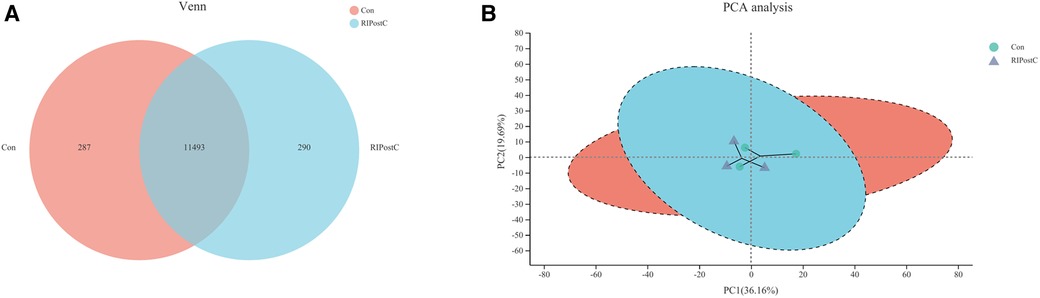
Figure 4. Expression differences between the Con and the RIPostC group. (A) Shared and unique expressed genes displayed by Venn analysis. (B) Differences of expression pattern exhibited by PCA analysis.
DEGs identification and analysis
Volcano map showed that the number of DEGs in the RIPostC group vs. to the Con group was 7. The heatmap displayed the expression levels of DEGs in every sample between two groups. The 2 up-regulated genes are: ADAMTS15 (ADAM metallopeptidase with thrombospondin type 1 motif, 15) and Prodh1 (proline dehydrogenase 1). The 5 down-regulated genes are: Cldn5 (Claudin-5), Sccpdh (saccharopine dehydrogenase), Robo4 (roundabout guidance receptor 4), LOC103689977 (Caspase-6) and AABR07011951.1 (Heat shock cognate 71 kDa protein) (Figure 5). Expression differences between the Con and the Sham group were shown in Supplementary Figure S3 and Table S1.
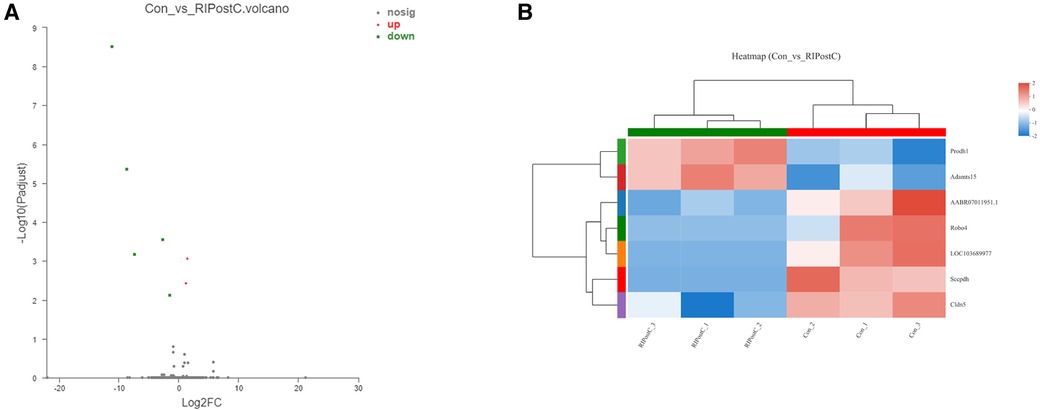
Figure 5. DEGs identification and analysis. (A) Red and green dots represented up and down regulated genes by Volcano map. (B) The expression levels of DEGs and similarity between samples as well as DEGs showed by heatmap.
GO and KEGG annotation analysis of DEGs
To identify the functions of DEGs induced by RIPostC, GO terms were annotated. In biological process, cellular process, metabolic process and response to stimulus correspond to 5, 3 and 2 DEGs respectively. In cellular component, cell part, organelle, and membrane correspond to 5, 3 and 2 DEGs respectively. In molecular function, catalytic activity and binding correspond to 5 different genes respectively. Therefore, this GO annotation analysis showed that GO terms mainly included cellular process, cell part, binding, catalytic activity, organelle and metabolic process (Figure 6A). Meanwhile, the KEGG annotation analysis of DEGs between the two groups found that only one pathway, amino acid metabolism, was up-regulated (Figure 6B).
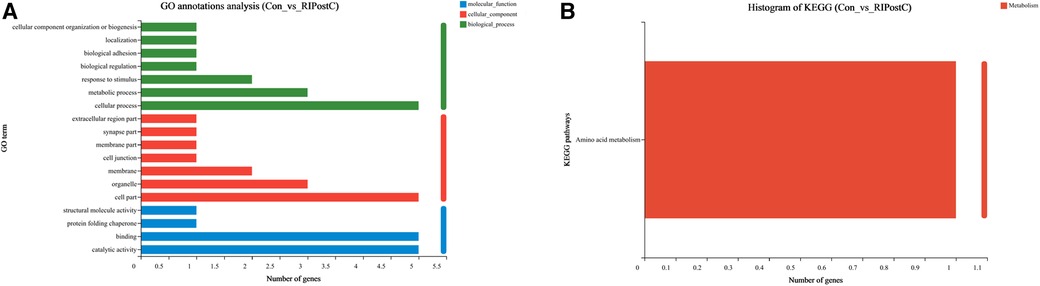
Figure 6. Go and KEGG annotation analysis of DEGs. (A) Annotated GO terms of DEGs. (B) Annotated KEGG pathways of DEGs.
Verification of RNA sequencing data by qRT-PCR
The expression levels of candidate genes were verified by qRT-PCR. As shown in Figure 7, compared to Con group, the relative mRNA expression levels of Caspase-6 and Claudin-5 were decreased, Prodh1 and ADAMTS15 were increased in the RIPostC group, which were consistent with the RNA-seq results.
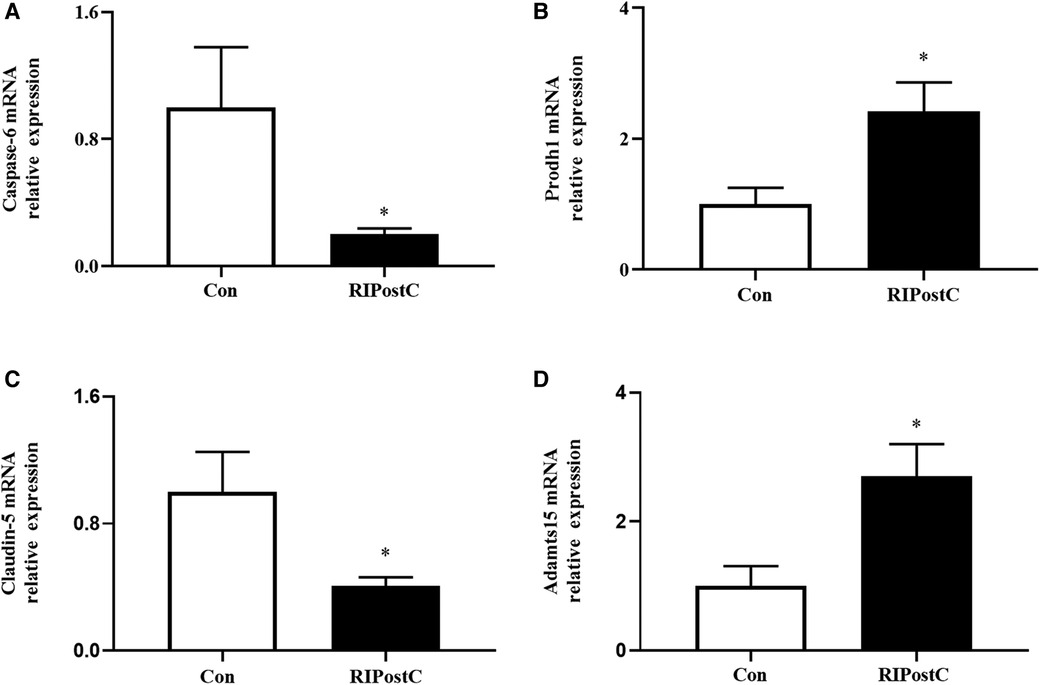
Figure 7. Expression levels of DEGs validated by qRT-PCR. (A–D) The relative mRNA expression comparisons of Caspase-6, Prodh1, Claudin-5 and ADAMTS15 respectively in the RIPostC and the Con group. *Represents P < 0.05, n = 6 for each group.
The correlation between the relative expression of ADAMTS15 and cardiac inflammatory indicators
The scatter diagrams and correlation analysis demonstrated that the relative expression of ADAMTS15 were corelated to the levels of cardiac inflammatory indicators (Figure 8). The relative expression of ADAMTS15 was negatively correlated with the level of cardiac IL-1β (r = −0.748, P = 0.005) and positively correlated with the level of cardiac IL-10 (r = 0.698, P = 0.012). In addition, a negative correlation trend was found between the relative expression of ADAMTS15 and the level of cardiac IL-6 (r = −0.545, P = 0.067). There was no statistical correlation between the relative expression of ADAMTS15 and the level of cardiac TNF-α (r = −0.406, P = 0.190).
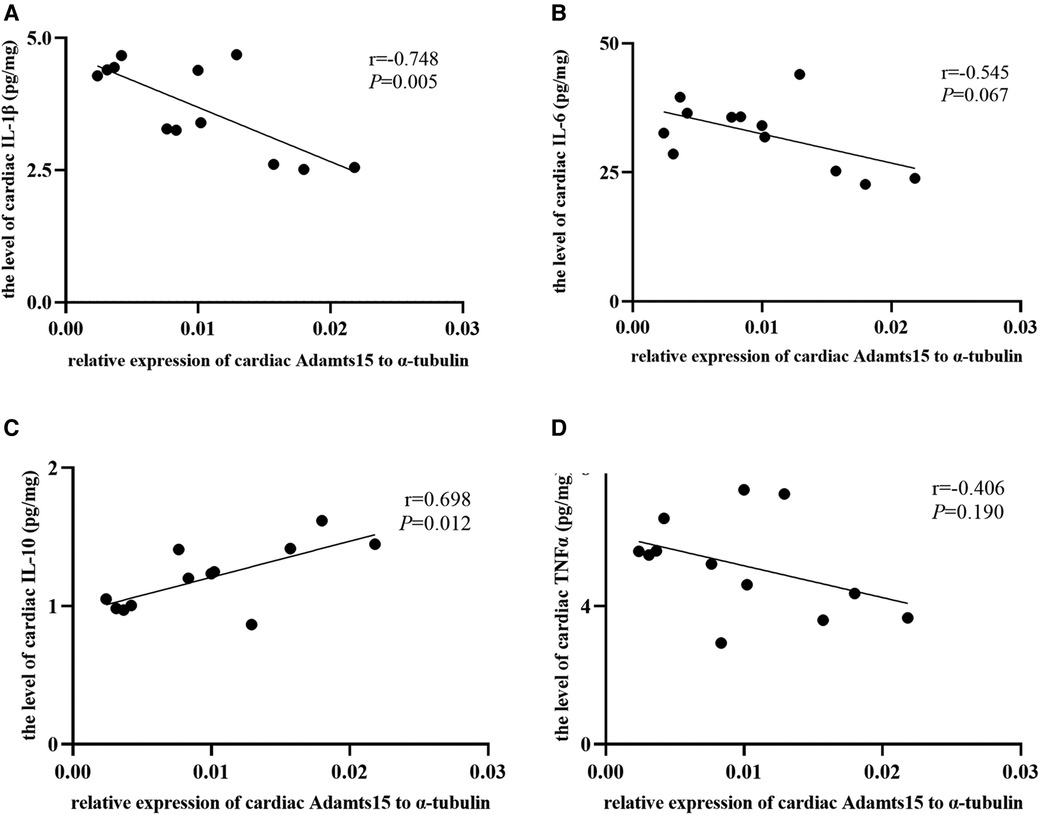
Figure 8. The correlation between the relative expression of ADAMTS15 and the levels of cardiac inflammatory factors. (A–D) The correlation between the relative mRNA expression of ADAMTS15 and the levels of cardiac IL-1β, IL-6, IL-10 and TNFα respectively in the RIPostC and the Con group. *Represents P < 0.05, n = 6 for each group.
Discussion
Inflammation is a key factor of myocardial IR injury. Excessive inflammation mediates a series of interactions between neutrophils and vascular endothelial cells, which plays a fundamental role in the inflammatory process of myocardial IR injury. Inflammatory signal cascades triggered during myocardial IR injury can lead to the release of proinflammatory cytokines, such as IL-1β, IL-6 and IL-18, promote leukocyte adhesion to endothelial cells, and trigger leukocyte infiltration into inflammatory damage. Therefore, anti-inflammatory strategy is a potential effective therapeutic method to alleviate myocardial IR injury (11, 24). In several studies, RIC was associated with the reduction of proinflammatory cytokines, such as IL-1β, IL-6 (11). Besides, RIC was proved to increase the level of protective cytokine IL-10. The up-expression of cardiac IL-10 can alleviate the cytokine response and lead to significant cardioprotection in RIC (12). Consistent with the results in previous studies, we found that RIPostC can reduce the levels of cardiac IL-1β, IL-6 and increase the level of cardiac IL-10, showing powerful anti-inflammatory role of RIPostC.
In this study, we investigated the effect of RIPostC on gene expressions in myocardium by using transcriptome sequencing in rat models. Our results showed that 7 genes were differently expressed between the RIPostC group and the Con group. Go analysis showed that DEGs associated GO terms mainly included cellular process, cell part, binding, catalytic activity, organelle and metabolic process. Further KEGG annotation analysis showed that these DEGs are associated with amino acid metabolism pathway. Compared to the Con group, the relative mRNA expression levels of Caspase-6 and Claudin-5 decreased, Prodh1 and ADAMTS15 increased in the RIPostC group by qRT-PCR, which were consistent with the RNA-seq data. In addition, the relative expression of ADAMTS15 was negatively correlated with the level of cardiac IL-1β and positively correlated with the level of cardiac IL-10. To our knowledge, this is the first study to investigate the transcriptome changes in the myocardium using RNA sequencing technology and demonstrate the overall mechanism changes induced by RIPostC. The potential role of ADAMTS15 in myocardial IR injury and RIPostC was a novel discovery.
ADAMTS are members of proteolytic enzyme family, which are secreted by fibroblasts, smooth muscle cells, macrophages and other cells (25). ADAMTS is a kind of secreted zinc endopeptidase, which contains a signal peptide, a variable length anterior domain, a metalloproteinase domain, an integrin like domain, a central platelet reactive protein type 1 sequence repeat (TSR) motif, a cysteine rich spacer domain and auxiliary domain. The auxiliary domain determines the differences among members of the ADAMTS protein family. ADAMTS are associated with a variety of diseases, including cardiovascular disease (25). Clinical studies have shown that low ADAMTS13 level was associated with an increased risk of AMI (26). ADAMTS5 is considered to have a protective effect on atherosclerosis by regulating the catabolism of vascular proteoglycan and improving the deposition of lipoprotein (27). The expression level of ADAMTS7 was up-regulated in damaged plaques and may promote vascular smooth muscle cells migration and proliferation and aggravate vascular remodeling (28). Therefore, ADAMTS can play key roles in the occurrence and development of coronary heart disease.
Interestingly, ADAMTS is a family of enzymes closely related to acute and chronic inflammation. It is increasingly recognized that inflammation stimulates thrombosis, which in turn promotes inflammation. Up to now, ADAMTS were proved to be key contributors to thrombo-inflammation. For example, literatures have shown that ADAMTS13 gene deficiency mice have proinflammatory and thrombogenic phenotypes. In addition, the administration of recombinant ADAMTS13 can alleviate inflammation in myocardial IR injury. ADAMTS13 can prevent excessive platelet and/or leukocyte recruitment in ischemic myocardium by cleaving von Willebrand factor (vWF). The treatment of wild type mice with recombinant human ADAMTS13 resulted in a smaller infarct area and decreased number of neutrophils infiltrating ischemic myocardium, indicating that ADAMTS13 had an effective anti-inflammatory effect during myocardial IR injury (29, 30).
Similar with other ADAMTS members, ADAMTS15 is also a metalloproteinase with thrombospondin domain. It was reported that ADAMTS15 played a tumor suppressor role in prostate cancer and colorectal cancer (31). However, to our knowledge, the role of ADAMTS15 in myocardial IR injury and RIPostC has not been investigated. In this study, we found that the relative expression of ADAMTS15 was negatively correlated with the level of cardiac IL-1β and positively correlated with the level of cardiac IL-10, indicating that ADAMTS15 may be a potential inflammation-related gene in cardioprotection conferred by RIPostC and a possible effective target for myocardial IR injury. The inflammation-related role and mechanism of ADAMTS15 in RIC need to be further studied in future.
In this study, we found that the expression of Caspase-6 was down-regulated after the implementation of RIPostC. Caspases is a family of enzymes that regulate programmed cell death, inflammation and other biological functions. Caspase-6 is generally identified as an executioner of apoptosis, a kind of non-inflammatory cell death (32, 33). In contrast to apoptosis, other common programmed cell death forms, pyroptosis and necroptosis, for instance, may result in the increase of interleukins release, such as IL-1β and IL-18, to enhance cardiac inflammation in myocardial IR injury, mostly considered to be associated with activation of NLRP3 inflammasome and NF-κβ pathway. Recent studies showed that Caspase-6 may facilitate ZBP1-mediated inflammasome activation and promote the activation of programmed cell death pathways, including pyroptosis and necroptosis (34). Whether Caspase-6, with or not with ADAMTS15, involves in cardioprotection conferred by RIC via regulating inflammation related pyroptosis and necroptosis is an interesting hypothesis and worth exploring in the future.
Claudin-5 is a transmembrane protein, which is widely expressed in epithelium and endothelial cells of brain, heart and so on. Claudin-5 was found to mediate the occurrence and development of cerebral ischemia-reperfusion injury (35, 36). In the ischemic penumbra, the level of Claudin-5 decreased in the early stage of vascular remodeling and increased in the later stage (37). However, the change of Claudin-5 expression over time in myocardial IR was still unknown. The role and mechanism of Claudin-5 in RIPostC need to be further investigated in future. In this study, the expression of Prodh1 is up-regulated after RIPostC. Prodh1 is a kind of enzyme that catalyzes the first step of proline catabolism (38). Wang et al. found that the enhancement of proline metabolism induced by over-expression of prodh decreased the level of reactive oxidative stress and apoptosis, while prodh gene knockout had the opposite effect in rat cardiomyocytes under hypoxia, indicating a cardioprotective role by the enhancement of proline metabolism (39).
Our KEGG annotation analysis showed that amino acid metabolism pathway may involve in regulation of cardioprotection conferred by RIPostC. In 2016, a metabolomic study showed that RIPC was associated with a decrease in ornithine and an increase in kynurenine (KYN) together with glycine concentrations in both rat and human plasma samples (40). Further study showed that inhibition of KYN synthesis may eliminate the cardioprotective effect induced by RIPC (41). At present, there are few studies on the role of amino acid metabolism in cardioprotection induced by RIC, which needs further exploration in our future research work.
Meanwhile, there are some limitations in this study. First, our results of transcriptome sequencing are based on a small sample size, which may not provide enough information to support our hypothesis. Second, the subjects of this study were rats, and species heterogeneity may lead to differences in results (42). Third, RIC is a physiological process involving multiple targets, such as limb, heart and blood. This study only focused on the expressions of DEGs in myocardial tissue and could not fully reflect the mechanism of RIC. Finally, as an association study, this research lacks evidence for causality between the transcriptomics changes and the observed cardioprotection. Thus, further study should be performed to explore how these DEGs involve in cardioprotection induced by RIC, especially ADAMTS15. In addition, the mechanism of the cardioloprotective role of RIPostC should be explored by ex-vivo experiments in the future.
Conclusion
In conclusion, 7 differently expressed genes were found after RIPostC by using transcriptome sequencing in rat models. ADAMTS15 may be a potential inflammation-related gene in regulation of cardioprotection conferred by RIPostC and a possible therapeutic target for myocardial IR injury in future. Caspase-6 mediated cell death may possibly involve in cardioprotection induced by RIPostC. Amino acid metabolism pathway, may involve in regulation of cardioprotection. Further studies are needed to explore the complex mechanisms of cardioprotection induced by RIPostC.
Data availability statement
The data presented in the study were deposited in the NCBI repository which can be found below: https://www.ncbi.nlm.nih.gov/bioproject/PRJNA847371/.
Ethics statement
The animal study was reviewed and approved by The Animal Care Committee of Peking University and the approval number was LA2016063.
Author contributions
BZ and ZL were devoted to the analysis and interpretation of the data. BZ and SZ were devoted to writing for this article. GW and ZL designed this research. All authors contributed to the article and approved the submitted version.
Funding
This study was supported by the National Natural Science Foundation of China (No. 82000328, 81870271).
Conflict of interest
The authors declare that the research was conducted in the absence of any commercial or financial relationships that could be construed as a potential conflict of interest.
Publisher's note
All claims expressed in this article are solely those of the authors and do not necessarily represent those of their affiliated organizations, or those of the publisher, the editors and the reviewers. Any product that may be evaluated in this article, or claim that may be made by its manufacturer, is not guaranteed or endorsed by the publisher.
Supplementary material
The Supplementary Material for this article can be found online at: https://www.frontiersin.org/articles/10.3389/fcvm.2023.1089151/full#supplementary-material.
References
1. Hausenloy DJ, Yellon DM. Ischaemic conditioning and reperfusion injury. Nat Rev Cardiol. (2016) 13:193–209. doi: 10.1038/nrcardio.2016.5
2. Heusch G. Myocardial ischaemia-reperfusion injury and cardioprotection in perspective. Nat Rev Cardiol. (2020) 17:773–89. doi: 10.1038/s41569-020-0403-y
3. Przyklenk K, Bauer B, Ovize M, Kloner RA, Whittaker P. Regional ischemic ‘preconditioning’ protects remote virgin myocardium from subsequent sustained coronary occlusion. Circulation. (1993) 87:893–9. doi: 10.1161/01.cir.87.3.893
4. Crimi G, Pica S, Raineri C, Bramucci E, De Ferrari GM, Klersy C, et al. Remote ischemic post-conditioning of the lower limb during primary percutaneous coronary intervention safely reduces enzymatic infarct size in anterior myocardial infarction: a randomized controlled trial. JACC Cardiovasc Interv. (2013) 6:1055–63. doi: 10.1016/j.jcin.2013.05.011
5. Kleinbongard P, Skyschally A, Heusch G. Cardioprotection by remote ischemic conditioning and its signal transduction. Pflugers Arch. (2017) 469:159–81. doi: 10.1007/s00424-016-1922-6
6. Bell RM, Basalay M, Botker HE, Beikoghli Kalkhoran S, Carr RD, Cunningham J, et al. Remote ischaemic conditioning: defining critical criteria for success-report from the 11th hatter cardiovascular workshop. Basic Res Cardiol. (2022) 117:39. doi: 10.1007/s00395-022-00947-2
7. Cai Z, Luo W, Zhan H, Semenza GL. Hypoxia-inducible factor 1 is required for remote ischemic preconditioning of the heart. Proc Natl Acad Sci U S A. (2013) 110:17462–7. doi: 10.1073/pnas.1317158110
8. Davidson SM, Selvaraj P, He D, Boi-Doku C, Yellon RL, Vicencio JM, et al. Remote ischaemic preconditioning involves signalling through the SDF-1alpha/CXCR4 signalling axis. Basic Res Cardiol. (2013) 108:377. doi: 10.1007/s00395-013-0377-6
9. Rassaf T, Totzeck M, Hendgen-Cotta UB, Shiva S, Heusch G, Kelm M. Circulating nitrite contributes to cardioprotection by remote ischemic preconditioning. Circ Res. (2014) 114:1601–10. doi: 10.1161/CIRCRESAHA.114.303822
10. Heusch G. Molecular basis of cardioprotection: signal transduction in ischemic pre-, post-, and remote conditioning. Circ Res. (2015) 116:674–99. doi: 10.1161/CIRCRESAHA.116.305348
11. Pearce L, Davidson SM, Yellon DM. Does remote ischaemic conditioning reduce inflammation? A focus on innate immunity and cytokine response. Basic Res Cardiol. (2021) 116:12. doi: 10.1007/s00395-021-00852-0
12. Cai ZP, Parajuli N, Zheng X, Becker L. Remote ischemic preconditioning confers late protection against myocardial ischemia-reperfusion injury in mice by upregulating interleukin-10. Basic Res Cardiol. (2012) 107:277. doi: 10.1007/s00395-012-0277-1
13. Lieder HR, Kleinbongard P, Skyschally A, Hagelschuer H, Chilian WM, Heusch G. Vago-splenic axis in signal transduction of remote ischemic preconditioning in pigs and rats. Circ Res. (2018) 123:1152–63. doi: 10.1161/CIRCRESAHA.118.313859
14. Heusch G. The spleen in myocardial infarction. Circ Res. (2019) 124:26–8. doi: 10.1161/CIRCRESAHA.118.314331
15. Donato M, Bin EP VDA, Gelpi RJ. Myocardial remote ischemic preconditioning: from cell biology to clinical application. Mol Cell Biochem. (2021) 476:3857–67. doi: 10.1007/s11010-021-04192-4
16. Heusch G, Botker HE, Przyklenk K, Redington A, Yellon D. Remote ischemic conditioning. J Am Coll Cardiol. (2015) 65:177–95. doi: 10.1016/j.jacc.2014.10.031
17. Gedik N, Kruger M, Thielmann M, Kottenberg E, Skyschally A, Frey UH, et al. Proteomics/phosphoproteomics of left ventricular biopsies from patients with surgical coronary revascularization and pigs with coronary occlusion/reperfusion: remote ischemic preconditioning. Sci Rep. (2017) 7:7629. doi: 10.1038/s41598-017-07883-5
18. Hepponstall M, Ignjatovic V, Binos S, Monagle P, Jones B, Cheung MH, et al. Remote ischemic preconditioning (RIPC) modifies plasma proteome in humans. PLoS One. (2012) 7:e48284. doi: 10.1371/journal.pone.0048284.23139772
19. Hibert P, Prunier-Mirebeau D, Beseme O, Chwastyniak M, Tamareille S, Lamon D, et al. Apolipoprotein a-I is a potential mediator of remote ischemic preconditioning. PLoS One. (2013) 8:e77211. doi: 10.1371/journal.pone.0077211
20. Stark R, Grzelak M, Hadfield J. RNA sequencing: the teenage years. Nat Rev Genet. (2019) 20:631–56. doi: 10.1038/s41576-019-0150-2
21. Baars T, Skyschally A, Klein-Hitpass L, Cario E, Erbel R, Heusch G, et al. microRNA expression and its potential role in cardioprotection by ischemic postconditioning in pigs. Pflugers Arch. (2014) 466:1953–61. doi: 10.1007/s00424-013-1429-3
22. Lieder HR, Irmert A, Kamler M, Heusch G, Kleinbongard P. Sex is no determinant of cardioprotection by ischemic preconditioning in rats, but ischemic/reperfused tissue mass is for remote ischemic preconditioning. Physiol Rep. (2019) 7:e14146. doi: 10.14814/phy2.14146
23. Gupta R, Dewan I, Bharti R, Bhattacharya A. Differential expression analysis for RNA-seq data. ISRN Bioinform. (2012) 2012:817508. doi: 10.5402/2012/817508
24. Marchant DJ, Boyd JH, Lin DC, Granville DJ, Garmaroudi FS, McManus BM. Inflammation in myocardial diseases. Circ Res. (2012) 110:126–44. doi: 10.1161/CIRCRESAHA.111.243170
25. Santamaria S, de Groot R. ADAMTS proteases in cardiovascular physiology and disease. Open Biol. (2020) 10:200333. doi: 10.1098/rsob.200333
26. Eerenberg ES, Teunissen PF, van den Born BJ, Meijers JC, Hollander MR, Jansen M, et al. The role of ADAMTS13 in acute myocardial infarction: cause or consequence? Cardiovasc Res. (2016) 111:194–203. doi: 10.1093/cvr/cvw097
27. Didangelos A, Mayr U, Monaco C, Mayr M. Novel role of ADAMTS-5 protein in proteoglycan turnover and lipoprotein retention in atherosclerosis. J Biol Chem. (2012) 287:19341–5. doi: 10.1074/jbc.C112.350785
28. Kessler T, Zhang L, Liu Z, Yin X, Huang Y, Wang Y, et al. ADAMTS-7 inhibits re-endothelialization of injured arteries and promotes vascular remodeling through cleavage of thrombospondin-1. Circulation. (2015) 131:1191–201. doi: 10.1161/CIRCULATIONAHA.114.014072
29. Gandhi C, Motto DG, Jensen M, Lentz SR, Chauhan AK. ADAMTS13 deficiency exacerbates VWF-dependent acute myocardial ischemia/reperfusion injury in mice. Blood. (2012) 120:5224–30. doi: 10.1182/blood-2012-06-440255
30. De Meyer SF, Savchenko AS, Haas MS, Schatzberg D, Carroll MC, Schiviz A, et al. Protective anti-inflammatory effect of ADAMTS13 on myocardial ischemia/reperfusion injury in mice. Blood. (2012) 120:5217–23. doi: 10.1182/blood-2012-06-439935
31. Binder MJ, McCoombe S, Williams ED, McCulloch DR, Ward AC. ADAMTS-15 has a tumor suppressor role in prostate cancer. Biomolecules. (2020) 10:682. doi: 10.3390/biom10050682
32. Zheng M, Kanneganti TD. The regulation of the ZBP1-NLRP3 inflammasome and its implications in pyroptosis, apoptosis, and necroptosis (PANoptosis). Immunol Rev. (2020) 297:26–38. doi: 10.1111/imr.12909
33. Tubeleviciute-Aydin A, Beautrait A, Lynham J, Sharma G, Gorelik A, Deny LJ, et al. Identification of allosteric inhibitors against active caspase-6. Sci Rep. (2019) 9:5504. doi: 10.1038/s41598-019-41930-7
34. Zheng M, Karki R, Vogel P, Kanneganti TD. Caspase-6 is a key regulator of innate immunity, inflammasome activation, and host defense. Cell. (2020) 181:674–87. doi: 10.1016/j.cell.2020.03.040
35. Kakogiannos N, Ferrari L, Giampietro C, Scalise AA, Maderna C, Rava M, et al. JAM-A acts via C/EBP-alpha to promote claudin-5 expression and enhance endothelial barrier function. Circ Res. (2020) 127:1056–73. doi: 10.1161/CIRCRESAHA.120.316742
36. Hashimoto Y, Campbell M, Tachibana K, Okada Y, Kondoh M. Claudin-5: a pharmacological target to modify the permeability of the blood-brain barrier. Biol Pharm Bull. (2021) 44:1380–90. doi: 10.1248/bpb.b21-00408
37. Sun J, Yu L, Huang S, Lai X, Milner R, Li L. Vascular expression of angiopoietin1, alpha5beta1 integrin and tight junction proteins is tightly regulated during vascular remodeling in the post-ischemic brain. Neuroscience. (2017) 362:248–56. doi: 10.1016/j.neuroscience.2017.08.040
38. Servet C, Ghelis T, Richard L, Zilberstein A, Savoure A. Proline dehydrogenase: a key enzyme in controlling cellular homeostasis. Front Biosci (Landmark Ed). (2012) 17:607–20. doi: 10.2741/3947
39. Wang J, Xue Z, Hua C, Lin J, Shen Z, Song Y, et al. Metabolomic analysis of the ameliorative effect of enhanced proline metabolism on hypoxia-induced injury in cardiomyocytes. Oxid Med Cell Longev. (2020) 2020:8866946. doi: 10.1155/2020/8866946
40. de la Barca JM C, Bakhta O, Kalakech H, Simard G, Tamareille S, Catros V, et al. Metabolic signature of remote ischemic preconditioning involving a cocktail of amino acids and biogenic amines. J Am Heart Assoc. (2016) 5:e003891. doi: 10.1161/JAHA.116.003891
41. Bakhta O, Pascaud A, Dieu X, Beaumont J, Kouassi Nzoughet J, Kamel R, et al. Tryptophane-kynurenine pathway in the remote ischemic conditioning mechanism. Basic Res Cardiol. (2020) 115:13. doi: 10.1007/s00395-019-0770-x
Keywords: acute myocardial infarction, ischemia reperfusion injury, inflammation, remote ischemic postconditioning (RIPostC), ADAMTS15, transcriptome analysis
Citation: Zuo B, Zhu S, Wang G and Li Z (2023) Transcriptome analysis reveals ADAMTS15 is a potential inflammation-related gene in remote ischemic postconditioning. Front. Cardiovasc. Med. 10:1089151. doi: 10.3389/fcvm.2023.1089151
Received: 3 November 2022; Accepted: 17 April 2023;
Published: 10 May 2023.
Edited by:
Tommaso Gori, Johannes Gutenberg University Mainz, GermanyReviewed by:
Gerd Heusch, University of Duisburg-Essen, GermanyBruno Moukette, Indiana University, Purdue University Indianapolis, United States
© 2023 Zuo, Zhu, Wang and Li. This is an open-access article distributed under the terms of the Creative Commons Attribution License (CC BY). The use, distribution or reproduction in other forums is permitted, provided the original author(s) and the copyright owner(s) are credited and that the original publication in this journal is cited, in accordance with accepted academic practice. No use, distribution or reproduction is permitted which does not comply with these terms.
*Correspondence: Zhengpeng Li MTUyMDEzMDQ2MTFAMTYzLmNvbQ== Guisong WangZ3Vpc29uZ3dhbmdAYmptdS5lZHUuY24=