- 1Department of Neurology, Joint Research Institution of Altitude Health, West China Hospital, Sichuan University, Chengdu, China
- 2Department of Cardiology, West China Hospital, Sichuan University, Chengdu, China
- 3Fujian Provincial Key Laboratory of Neurodegenerative Disease and Aging Research, Institute of Neuroscience, School of Medicine, Xiamen University, Xiamen, China
Brain diseases are a major burden on human health worldwide, and little is known about how most brain diseases develop. It is believed that cardiovascular diseases can affect the function of the brain, and many brain diseases are associated with heart dysfunction, which is called the heart-brain axis. Congenital heart abnormalities with anomalous hemodynamics are common treatable cardiovascular diseases. With the development of cardiovascular surgeries and interventions, the long-term survival of patients with congenital heart abnormalities continues to improve. However, physicians have reported that patients with congenital heart abnormalities have an increased risk of brain diseases in adulthood. To understand the complex association between congenital heart abnormalities and brain diseases, the paper reviews relevant clinical literature. Studies have shown that congenital heart abnormalities are associated with most brain diseases, including stroke, migraine, dementia, infection of the central nervous system, epilepsy, white matter lesions, and affective disorders. However, whether surgeries or other interventions could benefit patients with congenital heart abnormalities and brain diseases remains unclear because of limited evidence.
1. Introduction
Brain diseases are a major health problem worldwide. According to the Global Burden of Disease Study Group, brain disease-related disease burden exceeds 15% of all diseases (1). At the same time, the etiology and risk factors for many brain diseases remain unclear to scientists and clinicians. Brain diseases are like a “black box”. How to subtly open the “black box” is a difficult but important problem for scientists and clinicians.
The heart and the brain are the core organs of the circulatory system and the central nervous system, respectively, which play an important role in the maintenance of normal physiological functions. With the development of clinical understanding, the relationship between the heart and the brain is constantly being revealed (Figure 1A). The brain regulates the function of the heart, and impaired brain function can cause cardiovascular diseases. Studies have found that neurodegenerative diseases and affective disorders can cause the chronic failure of the autonomic nervous system and lead to arrhythmias (2). Seizures, especially generalized tonic‒clonic seizures, could result in the sustained release of neurotransmitters, including epinephrine and norepinephrine, leading to tachycardia, hypertension, and even sudden cardiac death (3). In turn, functional changes in the heart can have important effects on brain function through multiple pathways and can be risk factors or biomarkers for brain disease. Several studies have suggested that nonorganic cardiac rhythm changes can reflect abnormal brain function and be novel biomarkers for various brain diseases, including depression and posttraumatic epilepsy (4, 5). Organic heart disease, such as heart failure and atrial fibrillation, is a risk factor for many neuropsychiatric diseases, including dementia and stroke (6, 7). In addition, researchers have also found that cardiac rhythms and electroencephalogram signals are highly synchronized, further suggesting a functional connection between the heart and the brain (8). Based on the evidence, the concept of the “heart-brain axis” was proposed (9–11), and the “heart-brain axis” has become a new target for brain diseases.
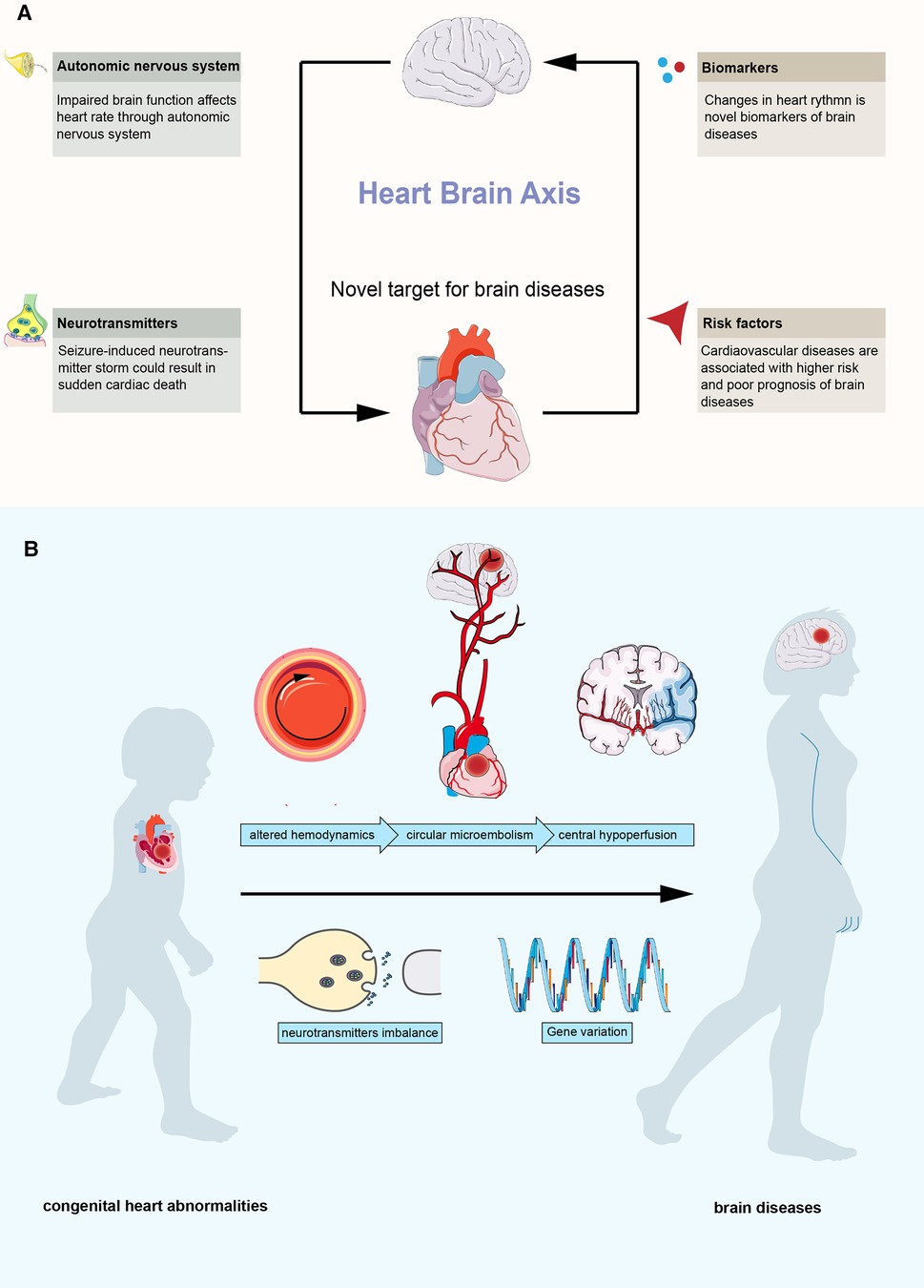
Figure 1. Overview of the heart-brain axis. (A) interactions between heart and brain. (B) possible mechanisms between congenital heart abnormalities and brain diseases.
Congenital heart abnormalities are an important part of cardiovascular disease, and more than 10 million newborns are born with congenital cardiovascular structural abnormalities each year (12). With the heart-brain axis, brain diseases related to congenital cardiovascular structural abnormalities have received increasing attention since the beginning of this century (13–15). In the past, it was believed that the damage to the nervous system in patients with congenital heart abnormalities was mostly attributed to surgical operations, including insufficient blood perfusion of the brain tissue during cardiopulmonary bypass, poor blood oxygen supply, and postoperative microthrombosis. With the continuous advancement of interventional therapy, open heart surgery, and cardiopulmonary bypass technology, the complications of surgery and interventional therapy continue to decrease. The long-term survival of patients with congenital heart abnormalities, especially complex congenital heart abnormalities, continues to improve. However, the situation of congenital heart abnormalities combined with an abnormal nervous system is becoming increasingly prominent, which has caused a great economic and mental burden to families and society (16–18). Current studies have found that most patients with congenital heart disease have neurological dysfunction before surgical treatment. Clinicians have found that congenital heart abnormalities, such as patent foramen ovale (PFO), could be the only abnormal finding in some patients with brain disease without any known risk factors (19–21). These cardiovascular structural abnormalities can cause abnormal intracardiac shunting, and microembolisms tend to occur at abnormal shunts due to altered hemodynamics (22, 23). The brain is the organ most susceptible to circulatory abnormalities. These microembolisms can cause central hypoperfusion, resulting in ischemic and hypoxic changes and related diseases. In addition, abnormal shunting could lead to the production-inactivation imbalance of neurotransmitters (such as serotonin), which contribute to the pathology of brain diseases (24). And several studies reported certain variations could contribute to this association (25, 26) (Figure 1B).
Many clinical studies have suggested that the onset of brain diseases related to congenital heart abnormalities mostly occurs in adulthood, which will have a serious impact on their quality of life and labor capacity (27, 28). Through evidence of the heart-brain axis, an increasing number of patients at high risk may benefit from appropriate closure of treatment (29). This article reviews common congenital heart abnormalities and their relationship with brain diseases and discusses the potential effects of their interventions on the neuropsychiatric system. Through a literature search, this paper found that congenital heart abnormalities were associated with a variety of brain diseases. In addition to stroke and migraine, central infectious diseases, white matter lesions, obstructive sleep apnea, epilepsy, and affective disorders may also be related to congenital heart abnormalities. However, the role of congenital heart abnormalities in the development of brain disease still lacks sufficient evidence. To address the question of how to provide more precise prevention and treatment for patients with congenital heart abnormalities, more high-quality evidence is needed in this field.
2. Congenital heart abnormalities and brain
2.1. Congenital heart abnormalities
Congenital heart abnormalities refer to a class of diseases that are of developmental origin and will not recover within a certain period. According to their severity, congenital heart abnormalities can be divided into simple and complex congenital heart abnormalities. Patients with complex congenital heart abnormalities often require hospitalization in a cardiovascular disease department, and the mortality and disability rates are high, such as transposition of the great arteries and tetralogy of Fallot (18, 30). Although the incidence of complex cardiovascular structural abnormalities is low, almost all complex cardiovascular heart abnormalities are fatal and often accompanied by serious complications, of which brain injury is a common extracardiac complication (31).
Common simple congenital heart abnormalities include PFO, congenital pulmonary arteriovenous fistula (PAVF), atrial septal defect (ASD), ventricular septal defect (VSD), and patent ductus arteriosus (PDA). Simple congenital heart abnormalities are less severe and generally do not affect the normal life of the patient. The incidence of simple congenital heart disease is high. It is estimated that approximately 25%–35% of adults have PFO (1). VSD, ASD, and PDA are the most common congenital heart diseases, accounting for 35.6%, 15.4%, and 10.2%, respectively (32). The incidence of PAVF has not yet been reported, but it is often underestimated because the size of lesions is too small. From the perspective of anatomical shunt, these five congenital cardiovascular structural abnormalities can be divided into right-to-left shunt and left-to-right shunt. Right-to-left shunts, including PFO and PAVF. PFO refers to a foramen ovale that has not closed after age 3 (19). PAVF refers to the congenital pulmonary arteriovenous direct connection without communication through capillaries (33). Left-to-right shunts include ASD, VSD, and PDA (34). ASD refers to the abnormality of the primordial atrial septum during embryonic development, resulting in left and right atrium openings (35). VSD refers to the development of the interventricular septum during the embryonic period, resulting in abnormal communication and left-to-right shunting at the level of the ventricle (36). PDA means that the ductus arteriosus in the fetal period continues to open after birth to form an abnormal shunt (37).
2.2. Crosstalk between congenital heart abnormalities and brain
Clinical evidence has demonstrated that congenital heart abnormalities are associated with pathological changes in certain brain regions. Signoriello and colleagues reported that the white matter lesion of patients with migraine and PFO was mainly in the occipital lobe compared with patients without PFO (38). And an earlier study confirmed this lobe-specific change in patients with cryptogenic stroke and PFO (39). Fontes reported that youth with congenital heart abnormalities had smaller hippocampal volumes compared with healthy controls (40). And similar pathological changes were also observed in children who underwent cardiopulmonary bypass surgery (41). Cordina and colleagues reported reduced brain volume in other brain regions, including the dorsolateral prefrontal cortex and precentral gyrus, the posterior parietal lobe and the middle temporal gyrus in adults with cyanotic congenital heart abnormalities (42). Such evidence suggests a clear relationship between congenital heart disease and pathological changes in brain regions.
Congenital heart abnormalities are associated with pathophysiological consequences, which could explain the fact that patients with congenital heart abnormalities were more likely to develop brain diseases. Hypoxia and oxidative stress are the most common mechanisms in congenital heart abnormalities-related brain diseases. Several studies reported that patients with PFO were at hypoxia state (43, 44), and hypoxia and oxidative stress were related to PFO-related many brain diseases, including stroke, migraine and epilepsy (22, 23, 45). Patients with other congenital heart abnormalities also demonstrated hypoxia and oxidative stress, and these changes could be related to abnormal neurogenesis and cortical growth (46–48). Besides, altered hemodynamics in PFO patients could be related to hypersensitivity of platelets, which could lead to abnormal 5-HT levels and prothrombotic potential (49). These mechanisms partly explain the underlying mechanisms between congenital heart abnormalities and brain diseases. However, there is still a lack of evidence to understand the complex relationship between congenital heart abnormalities and brain diseases, which needs more attention.
3. Right-to-left shunt and brain disease
3.1. PFO
PFO was the first congenital heart abnormality discovered to be related to brain diseases. It is currently believed that its pathogenesis is mainly related to paradoxical embolism caused by right-to-left shunting. Table 1 shows the clinical studies of PFO-related brain diseases, which focus on the composition ratio and odds ratio of PFO in patients with brain diseases. The relationship between PFO and stroke, especially cryptogenic stroke, is relatively clear. According to the data in the table, 47% of cryptogenic stroke patients have PFO, which is much higher than the 25%–35% in the general population. There is a clear association between PFO and migraine with aura. According to the data summarized in the table, 54% of migraine patients with aura have PFO, which is also much higher than the 25%–35% in the general population. For migraine without aura, the case‒control study by Takagi et al. showed that the prevalence of PFO was not significantly higher in patients with migraine without aura than in healthy controls (50). However, in a case‒control study by Tang et al., patients with PFO had a higher risk of migraine without aura than healthy controls (51). According to the data summarized in the table, approximately 28% of patients with migraine without aura have PFO. The relationship between PFO and migraine without aura needs more large sample studies to be explored. Evidence about PFO-related dementia is limited, but existing studies reported that dementia patients, especially Alzheimer's patients, have a higher prevalence of PFO (36.8%) (52). The prevalence of PFO was also higher in patients with obstructive sleep apnea, with data summarized in the table at 46%. However, since most studies are only cross-sectional surveys of a single group, the relationship between the two needs to be confirmed by more controlled studies.
In addition to those listed in the table, Higuchi et al. reported a case of an intramedullary abscess spread through a PFO after dental treatment in 2011. The experience of this 51-year-old man may suggest that PFO also plays a role in central system infectious diseases (71). In addition, Italian researchers found that white matter lesions caused by PFO-related migraine are mostly located in the occipital lobe, which may suggest that white matter lesions caused by PFO have certain spatial characteristics (38). However, the sample size of this study was small (n = 31), and more large-sample studies are needed to confirm this correlation. PFO may also play a role in affective disorders and personality disorders. The Korean research team's study of 19-year-old youth (n = 511) found that patients with congenital heart abnormalities (including PFO, ASD, VSD, PDA) had higher scores in anxiety, depression, somatization symptoms, and personality disorder than the control group (72). However, this result should be treated carefully, as the study only used the score as the outcome rather than a rigorous clinical diagnosis.
3.2. PAVF
PAVF refers to the existence of abnormal blood vessels between the pulmonary artery and the pulmonary vein, which are directly connected to form a pulmonary vascular malformation. It is generally believed that PAVF is a relatively benign structural abnormality. Only large-sized PAVF with a diameter of more than 2 cm or a feeding artery diameter of more than 3 mm may have obvious symptoms and require active intervention (73, 74). However, as an increasing number of clinical cases of small-sized PAVF are reported, the opinion that small-sized PAVF is pathogenic has also been proposed. Table 2 summarizes case reports of brain diseases in which PAVF is a major causative factor. As seen from the table, PAVF with nodules less than 3 cm in diameter also became the only causative factor for brain diseases, including stroke, migraine, and brain abscess. In addition, two retrospective studies reported that in patients with acute ischemic stroke, excluding other risk factors, PAVF-related stroke accounted for 0.02% and 0.5%, respectively (75, 76). Moreover, the age of PAVF-related stroke patients is significantly smaller than that of other stroke patients, suggesting that PAVF-related stroke may be independent of other strokes in clinical characteristics (75). Hence, PAVF could be a novel risk factor for stroke.
In clinical practice, some PFO patients have comorbid PAVF, and PAVF in these patients is often detected due to the discovery of residual shunts after PFO closure. Both PFO and PAVF can cause right-to-left shunting, but the relationship between the two is still unclear. In a large retrospective study, Topiwala et al. found that PFO was a risk factor for PAVF-related stroke, suggesting a synergistic effect between the two (75). However, within a certain range of defects, who is dominant and how to accurately understand the synergy between the two still lacks sufficient evidence. In addition, how to simultaneously diagnose both PFO and PAVF in patients with comorbid PFO and PAVF using one auxiliary test remains a clinical challenge. The diagnosis of PFO relies primarily on contrast ultrasonography through the chest wall or the esophagus. Although ultrasound can also indicate the presence of PAVF (87), the current gold standard for the diagnosis of PAVF is contrast-enhanced chest CT (33).
4. Left-to-right shunt and brain diseases
4.1. ASD
ASD is a representative disease of left-to-right shunt congenital heart abnormalities. Table 3 summarizes current clinical studies of ASD-related brain diseases. ASD is associated with stroke and migraine. Several studies have demonstrated that patients with ASD have a significantly increased risk of stroke and migraine. Based on the data summarized in the table, 2.9% of patients with ASD had stroke [0.13% in the general population (1)], and 7.1% of patients with ASD had migraine [1.46% in the general population (1)]. For stroke, a study from Taiwan, China, showed that ASD patients were less likely to have cardiovascular events after repair, suggesting that ASD repair is beneficial for preventing cardiovascular events (88). For migraine, Magalhães et al. showed that ASD patients with migraine had a greater proportion of migraine with aura (89). However, Kato et al. reported a greater proportion of migraine without aura (90). Further research is needed to determine the relationship between ASD and migraine with and without aura.
For affective disorders, Udholm et al. reported that patients with small, unpatched ASD had higher depression and anxiety scores (P < 0.001), and approximately 17% of ASD patients were diagnosed with at least one psychiatric disorder, the most common being psychosomatic disorders and neurosis (95). This large cohort study suggests that ASD may potentially cause changes in brain function in patients. Future studies are needed to confirm the relationship between ASD and affective disorders. In summary, ASD is expected to become a new target for brain diseases.
4.2. VSD and PDA
The clinical symptoms of VSD and PDA are rather serious, and most patients are repaired after birth. Therefore, there are limited studies on the relationship between VSD and PDA and brain diseases. For VSD, Shuiab reported a 38-year-old patient with ischemic stroke in the occipital lobe who did not find any abnormality other than VSD (96). For migraine, a team from China reviewed the comorbidities of 72 VSD patients and found that 6 (8.3%) suffered from migraine (94). Dhoubhadel et al. reported a case of cryptococcal meningitis caused by VSD in 2021. The 12-year-old girl had normal immunity and no obvious abnormal exposure history. The attending doctor believed that VSD was the only risk factor for cryptococcal meningitis in this case (97). For PDA, Panagopoulos et al. reported a 7-year-old child with recurrent ischemic stroke with comorbid PDA and PFO (98). However, it is unclear which of these two congenital structural heart defects predominated in this patient. In addition, Ke et al. reported a family with a PRRX1 heterozygous mutation that resulted in familial PDA and atrial fibrillation caused by PRRX1 loss of function. The family is at a significantly increased risk of stroke (99). In conclusion, the relationship between VSD and PDA and brain diseases is still unclear, but it should be considered in clinical practice that VSD and PDA may be the etiology or risk factors for some difficult neuropsychiatric cases. Future research is needed to explore the relationship between VSD and PDA and brain disease. Not only unrepaired but also long-term follow-up outcomes of repaired VSD and PDA are also worthy of attention.
5. Complex congenital heart abnormalities
Patients with complex congenital heart abnormalities often have genetic abnormalities, and such genetic diseases are often accompanied by brain damage. For example, children with Down syndrome and DiGeorge syndrome often present with complex congenital heart abnormalities (such as tetralogy of Fallot (TOF/F4), complete transposition of great arteries (CTGA) and single ventricle (SV), and tricuspid atresia (TA)) comorbid brain damage, such as intellectual disability and leukomalacia. These patients are at extremely high risk for seizures (100). In addition, the severe abnormal hemodynamic state associated with complex congenital heart abnormalities can also lead to severe hypoxemia during the growth and development of children. Ischemic and hypoxic damage to the brain occurs at a very early age and mainly manifests as stroke and white matter damage. Due to the high lethality of complex congenital heart abnormalities and the lack of medical records, it is still unclear when patients with complex congenital heart abnormalities begin to develop brain injury. It is unclear whether in utero intervention during pregnancy could alleviate brain injury caused by complex congenital heart abnormalities.
6. Interventions for congenital heart abnormalities and brain diseases
Although there is a certain correlation between congenital heart abnormalities and brain diseases, high-quality randomized controlled trials are still needed to confirm whether the intervention of structural abnormalities helps relieve the disease and prevent recurrence. The only currently recognized effective intervention is PFO closure for secondary prevention of stroke. Closing the PFO after stroke could reduce the risk of recurrent stroke [RR 0.42 (95% CI 0.20, 0.91)] (101). In addition, a meta-analysis pointed out that PFO closure can help migraine relief, especially migraine with aura (102). Some studies suggest that PFO closure can relieve symptoms in OSA patients, but the sample size is small, and its effect is controversial (65, 103). For other congenital cardiovascular structural abnormalities, there is currently a lack of clinical studies to explore whether closure therapy helps relieve the disease and prevent recurrence.
Thanks to current advanced techniques and treatment measures, many patients choose closure therapy before symptoms related to congenital heart abnormalities appear. Whether early-life closure therapy has adverse effects on the neuropsychiatric system is a question that scientists and clinicians need to pay attention to. Table 4 lists closed therapies for simple congenital heart abnormalities and their associated complications. In general, the closed treatment of various congenital heart abnormalities is relatively safe, and the probability of complications is low (30). Arrhythmias such as atrial fibrillation may occur after intracardiac intervention for PFO, ASD, and VSD, but the incidence is low (104). Atrial fibrillation could increase the risk of stroke in patients, but no studies have shown that PFO and VSD closure can increase the risk of postoperative stroke. For ASD, a Danish team reported an increased risk of stroke after ASD closure [HR 5.0 (95% CI 2.3, 11.1)] (92). However, the Taiwanese research team reported that the risk of cardiovascular events was reduced after ASD closure (88), which is contrary to the findings of the Danish team. For PAVF, embolization closure or partial pneumonectomy is generally selected according to the size and location of the PAVF. These interventions are relatively safe, but care should be taken to minimize trauma and reduce the patient's time in bed. Thoracotomy for VSD and PDA is more traumatic, and patients are at increased risk of postoperative neurodevelopmental abnormalities during long-term follow-up, which may be related to intraoperative cardiopulmonary bypass and cerebral hypoperfusion (105).
For the surgical treatment of complex heart abnormalities, due to the complicated operation, the large trauma to the patient, the long cardiopulmonary bypass time, and the need to maintain a long-term deep hypothermia state, the damage to the brain is relatively severe. One-third of children with complex congenital heart abnormalities without preoperative brain damage often develop brain diseases such as white matter lesions, stroke, epilepsy, and cognitive impairment after surgery. Among them, white matter lesions are the most common and more pronounced in children with a single ventricle, aortic malformation, and preoperative arterial hypoxia (113). Cardiopulmonary bypass is a form of circulation that diverts a patient's blood from the heart and lungs to the body. The normal physiological functions of the patient's heart and lungs, such as blood circulation, respiration, and oxygenation are temporarily replaced by extracorporeal circulation machines. During cardiopulmonary bypass, plaque fall, gas emboli, and surgical foreign bodies caused by intubation can become potential cerebrovascular emboli, causing cerebral ischemia-hypoxic injury (114, 115). Deep hypothermic circulatory arrest is commonly used in open heart surgery for complex congenital heart abnormalities. In the state of deep hypothermic circulatory arrest, the energy metabolism of the brain is depleted, causing brain damage through mechanisms such as calcium overload and excitatory amino acid toxicity (116–118). Studies have reported that the incidence of epilepsy after surgery for children with congenital heart abnormalities is 4% to 10%, and the incidence of epilepsy after surgery for complex congenital heart abnormalities is even higher (113). In addition, anesthetic use early in life may also be associated with impairment of brain function, including neuroapoptosis and neurocognitive deficits. However, the causality of this relationship is still debated (119).
In conclusion, surgery and interventional treatment of congenital heart abnormalities could have adverse effects on the brain. For simple congenital heart abnormalities, how to assess the risks brought by surgery and the risks brought by congenital heart abnormalities and properly compare the priorities of the two are the next issues to be solved. For complex heart abnormalities, surgical treatment is unavoidable. How to minimize brain damage by improving surgical procedures, preoperative intervention, and postoperative treatment is also an urgent problem to be solved (120, 121).
7. Discussion
This article summarizes the relationship between brain diseases and common congenital heart abnormalities. Overall, the brain disorders most associated with common congenital heart abnormalities are stroke and migraine, obstructive sleep apnea, dementia, cognitive impairment, white matter lesion, epilepsy, affective disorders, and central system infectious diseases, which may also have a certain relationship with congenital heart abnormalities. Some interventions for congenital heart abnormalities could increase the risk of brain disease. There is insufficient high-quality evidence about whether interventions should be conducted to reduce the risk of brain diseases caused by congenital heart abnormalities.
Apart from the direct relationship of the heart-brain axis, several risk factors could have an influence on both the heart and the brain. Agrimi et.al. reported that mice co-exposed to obesity and psychosocial stress displayed both cardiac and hippocampal dysfunction, which was associated with local brain-derived neurotrophic factor depletion (122). And smoking is a risk factor of both cardiovascular diseases and many brain diseases. Such evidence indicated that certain risk factors could affect the heart-brain axis (123, 124). However, whether such risk factors exist in congenital heart disease-related brain diseases remains unknown. In addition to traditional risk factors, sex or gender is believed to have an impact on the heart-brain axis, especially for the female heart-brain axis (125). For example, females tend to have poorer cognitive outcomes after cardiac operations (126). And for congenital heart diseases, PFO is believed to be a risk factor for stroke in pregnant women (127). However, an earlier study reported poorer neurodevelopmental outcomes in boys born with congenital heart disease requiring early surgical repair (128). How sex or gender influence congenital heart disease-related brain diseases requires more attention.
The heart-brain relationship has been revealed by a growing body of research (129–131). Although the brain, as a relatively independent organ, is isolated from the periphery by the blood‒brain barrier. However, it must take in necessary nutrients through the circulation, and the heart, as the central structure of the circulation, has an important influence on the circulation. Although there are only minor structural changes in congenital heart abnormalities, the impact on circulation is huge. The altered hemodynamics of abnormal shunts have corresponding effects on the brain and may ultimately result in brain disease, which is the heart-brain axis. The heart-brain axis affects the center through peripheral changes, resulting in diseases. The heart-brain axis has the potential to be a bridge to better understand brain function and diseases, enabling scientists and clinicians to unlock the “black box” of brain diseases (11, 132, 133).
The mechanism by which abnormal cardiac structure affects the function of the central nervous system still needs further study. Paradoxical embolism is currently the most mainstream mechanism theory. Abnormal hemodynamics caused by abnormal intracardiac shunts promote embolus formation (22). The emboli enter the cerebral blood vessels through circulation to form embolic ischemia and hypoxia. A hypoperfusion state can induce blood‒brain barrier damage, neuroinflammation, and abnormal neuroelectrophysiological activities, resulting in diseases (134, 135). In addition to the ischemic and hypoxic changes caused by paradoxical embolism, some scholars have suggested that the abnormal production and inactivation of serotonin may also be one of the mechanisms (24). The majority of serotonin inactivation is performed in the lungs. An abnormal intracardiac shunt changes the pulmonary circulation blood volume, which in turn leads to increased or decreased circulating serotonin. As an important neurotransmitter, the imbalance of serotonin will lead to abnormal nerve activity and cause disease (136–139). The mechanism of brain diseases related to congenital heart abnormalities is still inconclusive, and more clinical and basic research is needed to explore the above hypothesis.
Brain disease is a major threat to human health. The heart-brain axis could provide a new driving force for its early prevention and precise treatment, benefiting patients and their families worldwide. The improvement of the heart-brain axis requires the participation of more research teams. Further exploration of the important role of congenital heart abnormalities in the occurrence and development of brain diseases and the development of new therapeutic targets based on this feature can benefit more patients.
Author contributions
LS and LC designed this study. LS and YL did the literature search. YZ, YT, BL and YC helped with data extracted and integration of results. LS and LC wrote the draft of the manuscript. LC supervised the review process. All authors contributed to the article and approved the submitted version.
Funding
This study is supported by grants from the National Natural Science Foundation (Grant No. 82271500).
Acknowledgments
Parts of the figure were drawn using pictures from Servier Medical Art. Servier Medical Art by Servier is licensed under a Creative Commons Attribution 3.0 Unported License (https://creativecommons.org/licenses/by/3.0/).
Conflict of interest
The authors declare that the research was conducted in the absence of any commercial or financial relationships that could be construed as a potential conflict of interest.
Publisher's note
All claims expressed in this article are solely those of the authors and do not necessarily represent those of their affiliated organizations, or those of the publisher, the editors and the reviewers. Any product that may be evaluated in this article, or claim that may be made by its manufacturer, is not guaranteed or endorsed by the publisher.
References
1. Global burden of 369 diseases and injuries in 204 countries and territories, 1990–2019: a systematic analysis for the global burden of disease study 2019. Lancet. (2020) 396(10258):1204–22. doi: 10.1016/s0140-6736(20)30925-9
2. Silvani A, Calandra-Buonaura G, Dampney RA, Cortelli P. Brain-Heart interactions: physiology and clinical implications. Philos Trans A Math Phys Eng Sci. (2016) 374(2067):20150181. doi: 10.1098/rsta.2015.0181
3. Devinsky O, Hesdorffer DC, Thurman DJ, Lhatoo S, Richerson G. Sudden unexpected death in epilepsy: epidemiology, mechanisms, and prevention. Lancet Neurol. (2016) 15(10):1075–88. doi: 10.1016/s1474-4422(16)30158-2
4. Schiweck C, Piette D, Berckmans D, Claes S, Vrieze E. Heart rate and high frequency heart rate variability during stress as biomarker for clinical depression. A systematic review. Psychol Med. (2019) 49(2):200–11. doi: 10.1017/s0033291718001988
5. Bahari F, Ssentongo P, Schiff SJ, Gluckman BJ. A brain-heart biomarker for epileptogenesis. J Neurosci. (2018) 38(39):8473–83. doi: 10.1523/jneurosci.1130-18.2018
6. Doehner W, Ural D, Haeusler KG, Čelutkienė J, Bestetti R, Cavusoglu Y, et al. Heart and brain interaction in patients with heart failure: overview and proposal for a taxonomy. A position paper from the study group on heart and brain interaction of the heart failure association. Eur J Heart Fail. (2018) 20(2):199–215. doi: 10.1002/ejhf.1100
7. Kotalczyk A, Mazurek M, Kalarus Z, Potpara TS, Lip GYH. Stroke prevention strategies in high-risk patients with atrial fibrillation. Nat Rev Cardiol. (2021) 18(4):276–90. doi: 10.1038/s41569-020-00459-3
8. Catrambone V, Barbieri R, Wendt H, Abry P, Valenza G. Functional brain-heart interplay extends to the multifractal domain. Philos Trans A Math Phys Eng Sci. (2021) 379(2212):20200260. doi: 10.1098/rsta.2020.0260
9. Tahsili-Fahadan P, Geocadin RG. Heart-Brain axis: effects of neurologic injury on cardiovascular function. Circ Res. (2017) 120(3):559–72. doi: 10.1161/circresaha.116.308446
10. Thackeray JT. Imaging the molecular footprints of the heart-brain axis in cardiovascular disease. J Nucl Med. (2019) 60(6):728–9. doi: 10.2967/jnumed.118.222315
11. Xu C, Tao X, Ma X, Zhao R, Cao Z. Cognitive dysfunction after heart disease: a manifestation of the heart-brain axis. Oxid Med Cell Longev. (2021) 2021:4899688. doi: 10.1155/2021/4899688
12. Bouma BJ, Mulder BJ. Changing landscape of congenital heart disease. Circ Res. (2017) 120(6):908–22. doi: 10.1161/circresaha.116.309302
13. Aleksonis HA, King TZ. Relationships among structural neuroimaging and neurocognitive outcomes in adolescents and young adults with congenital heart disease: a systematic review. Neuropsychol Rev. (2022. doi: 10.1007/s11065-022-09547-2
14. Jackson JL, Leslie CE, Hondorp SN. Depressive and anxiety symptoms in adult congenital heart disease: prevalence, health impact and treatment. Prog Cardiovasc Dis. (2018) 61(3–4):294–9. doi: 10.1016/j.pcad.2018.07.015
15. Morton PD, Ishibashi N, Jonas RA. Neurodevelopmental abnormalities and congenital heart disease: insights into altered brain maturation. Circ Res. (2017) 120(6):960–77. doi: 10.1161/circresaha.116.309048
16. Jacobsen RM. Outcomes in adult congenital heart disease: neurocognitive issues and transition of care. Pediatr Clin North Am. (2020) 67(5):963–71. doi: 10.1016/j.pcl.2020.06.009
17. van der Bom T, Zomer AC, Zwinderman AH, Meijboom FJ, Bouma BJ, Mulder BJ. The changing epidemiology of congenital heart disease. Nat Rev Cardiol. (2011) 8(1):50–60. doi: 10.1038/nrcardio.2010.166
18. Warnes CA. Adult congenital heart disease: the challenges of a lifetime. Eur Heart J. (2017) 38(26):2041–7. doi: 10.1093/eurheartj/ehw529
19. Homma S, Messé SR, Rundek T, Sun YP, Franke J, Davidson K, et al. Patent foramen Ovale. Nat Rev Dis Primers. (2016) 2:15086. doi: 10.1038/nrdp.2015.86
20. Mojadidi MK, Zaman MO, Elgendy IY, Mahmoud AN, Patel NK, Agarwal N, et al. Cryptogenic stroke and patent foramen ovale. J Am Coll Cardiol. (2018) 71(9):1035–43. doi: 10.1016/j.jacc.2017.12.059
21. Rayhill M, Burch R. Pfo and migraine: is there a role for closure? Curr Neurol Neurosci Rep. (2017) 17(3):20. doi: 10.1007/s11910-017-0730-5
22. Nozari A, Dilekoz E, Sukhotinsky I, Stein T, Eikermann-Haerter K, Liu C, et al. Microemboli may link spreading depression, migraine aura, and patent foramen Ovale. Ann Neurol. (2010) 67(2):221–9. doi: 10.1002/ana.21871
23. Rapp JH, Hollenbeck K, Pan XM. An experimental model of lacunar infarction: embolization of microthrombi. J Vasc Surg. (2008) 48(1):196–200. doi: 10.1016/j.jvs.2008.01.038
24. Borgdorff P, Tangelder GJ. Migraine: possible role of shear-induced platelet aggregation with serotonin release. Headache. (2012) 52(8):1298–318. doi: 10.1111/j.1526-4610.2012.02162.x
25. Moradi Marjaneh M, Kirk EP, Posch MG, Ozcelik C, Berger F, Hetzer R, et al. Investigation of association between pfo complicated by cryptogenic stroke and a common variant of the cardiac transcription factor Gata4. PLoS One. (2011) 6(6):e20711. doi: 10.1371/journal.pone.0020711
26. Paolucci M, Vincenzi C, Romoli M, Amico G, Ceccherini I, Lattanzi S, et al. The genetic landscape of patent foramen Ovale: a systematic review. Genes (Basel). (2021) 12(12):1953. doi: 10.3390/genes12121953
27. Alsheikh-Ali AA, Thaler DE, Kent DM. Patent foramen Ovale in cryptogenic stroke: incidental or pathogenic? Stroke. (2009) 40(7):2349–55. doi: 10.1161/strokeaha.109.547828
28. Bahl R, Kubiak M, Yusoff S, Tung H, Stirrup J, van Wyk A, et al. Proportion of acute ischaemic strokes attributable to a cardiac aetiology in an unselected young patient population: a single centre experience. Clin Med (Lond). (2020) 20(2):174–7. doi: 10.7861/clinmed.2019-0353
29. Khan A, Gurvitz M. Epidemiology of achd: what has changed and what is changing? Prog Cardiovasc Dis. (2018) 61(3–4):275–81. doi: 10.1016/j.pcad.2018.08.004
30. Liu A, Diller GP, Moons P, Daniels CJ, Jenkins KJ, Marelli A. Changing epidemiology of congenital heart disease: effect on outcomes and quality of care in adults. Nat Rev Cardiol. (2022) 20:126–37. doi: 10.1038/s41569-022-00749-y
31. Khoshnood B, Lelong N, Houyel L, Thieulin AC, Jouannic JM, Magnier S, et al. Prevalence, timing of diagnosis and mortality of newborns with congenital heart defects: a population-based study. Heart. (2012) 98(22):1667–73. doi: 10.1136/heartjnl-2012-302543
32. Liu Y, Chen S, Zühlke L, Black GC, Choy MK, Li N, et al. Global birth prevalence of congenital heart defects 1970–2017: updated systematic review and meta-analysis of 260 studies. Int J Epidemiol. (2019) 48(2):455–63. doi: 10.1093/ije/dyz009
33. Contegiacomo A, Del Ciello A, Rella R, Attempati N, Coppolino D, Larici AR, et al. Pulmonary arteriovenous malformations: what the interventional radiologist needs to know. Radiol Med. (2019) 124(10):973–88. doi: 10.1007/s11547-019-01051-7
34. Tobler D, Greutmann M. Simple cardiac shunts in adults: atrial septal defects, ventricular septal defects, patent ductus arteriosus. Heart. (2020) 106(4):307–14. doi: 10.1136/heartjnl-2019-314700
35. Brida M, Chessa M, Celermajer D, Li W, Geva T, Khairy P, et al. Atrial septal defect in adulthood: a new paradigm for congenital heart disease. Eur Heart J. (2022) 43(28):2660–71. doi: 10.1093/eurheartj/ehab646
36. Penny DJ, Vick GW 3rd. Ventricular septal defect. Lancet. (2011) 377(9771):1103–12. doi: 10.1016/s0140-6736(10)61339-6
37. Backes CH, Hill KD, Shelton EL, Slaughter JL, Lewis TR, Weisz DE, et al. Patent ductus arteriosus: a contemporary perspective for the pediatric and adult cardiac care provider. J Am Heart Assoc. (2022) 11(17):e025784. doi: 10.1161/jaha.122.025784
38. Signoriello E, Cirillo M, Puoti G, Signoriello G, Negro A, Koci E, et al. Migraine as possible red flag of pfo presence in suspected demyelinating disease. J Neurol Sci. (2018) 390:222–6. doi: 10.1016/j.jns.2018.04.042
39. Steiner MM, Di Tullio MR, Rundek T, Gan R, Chen X, Liguori C, et al. Patent foramen Ovale size and embolic brain imaging findings among patients with ischemic stroke. Stroke. (1998) 29(5):944–8. doi: 10.1161/01.str.29.5.944
40. Fontes K, Rohlicek CV, Saint-Martin C, Gilbert G, Easson K, Majnemer A, et al. Hippocampal alterations and functional correlates in adolescents and young adults with congenital heart disease. Hum Brain Mapp. (2019) 40(12):3548–60. doi: 10.1002/hbm.24615
41. Naef N, Ciernik A, Latal B, Liamlahi R. Hippocampal volume and cognitive performance in children with congenital heart disease. Pediatr Res. (2023. doi: 10.1038/s41390-022-02457-2
42. Cordina R, Grieve S, Barnett M, Lagopoulos J, Malitz N, Celermajer DS. Brain volumetric, regional cortical thickness and radiographic findings in adults with cyanotic congenital heart disease. Neuroimage Clin. (2014) 4:319–25. doi: 10.1016/j.nicl.2013.12.011
43. Lovering AT, Kelly TS, DiMarco KG, Bradbury KE, Charkoudian N. Implications of a patent foramen Ovale for environmental physiology and pathophysiology: do we know the 'Hole' story? J Physiol. (2022) 600(7):1541–53. doi: 10.1113/jp281108
44. Lovering AT, Stickland MK, Amann M, O'Brien MJ, Hokanson JS, Eldridge MW. Effect of a patent foramen Ovale on pulmonary gas exchange efficiency at rest and during exercise. J Appl Physiol. (2011) 110(5):1354–61. doi: 10.1152/japplphysiol.01246.2010
45. Dong B, Li Y, Ji S, He S, Lai Q, Yang X, et al. Relationship between right-to-left shunt, hypoxia, and epilepsy. Epilepsia Open. (2023. doi: 10.1002/epi4.12710
46. Kelly CJ, Christiaens D, Batalle D, Makropoulos A, Cordero-Grande L, Steinweg JK, et al. Abnormal microstructural development of the cerebral Cortex in neonates with congenital heart disease is associated with impaired cerebral oxygen delivery. J Am Heart Assoc. (2019) 8(5):e009893. doi: 10.1161/jaha.118.009893
47. Sun L, Macgowan CK, Sled JG, Yoo SJ, Manlhiot C, Porayette P, et al. Reduced fetal cerebral oxygen consumption is associated with smaller brain size in fetuses with congenital heart disease. Circulation. (2015) 131(15):1313–23. doi: 10.1161/circulationaha.114.013051
48. Morton PD, Korotcova L, Lewis BK, Bhuvanendran S, Ramachandra SD, Zurakowski D, et al. Abnormal neurogenesis and cortical growth in congenital heart disease. Sci Transl Med. (2017) 9(374):eaah7029. doi: 10.1126/scitranslmed.aah7029
49. Trabattoni D, Brambilla M, Canzano P, Becchetti A, Teruzzi G, Porro B, et al. Migraine in patients undergoing pfo closure: characterization of a platelet-associated pathophysiological mechanism: the learner study. JACC Basic Transl Sci. (2022) 7(6):525–40. doi: 10.1016/j.jacbts.2022.02.002
50. Takagi H, Umemoto T. A meta-analysis of case-control studies of the association of migraine and patent foramen Ovale. J Cardiol. (2016) 67(6):493–503. doi: 10.1016/j.jjcc.2015.09.016
51. Tang Y, Peng A, Peng B, He S, Zhao X, Zhu Y, et al. Association between patent foramen Ovale and migraine without aura: a community-based cross-sectional study in China. BMJ Open. (2022) 12(3):e056937. doi: 10.1136/bmjopen-2021-056937
52. Purandare N, Oude Voshaar RC, McCollum C, Jackson A, Burns A. Paradoxical embolisation and cerebral white matter lesions in dementia. Br J Radiol. (2008) 81(961):30–4. doi: 10.1259/bjr/90498392
53. Ma B, Liu G, Chen X, Zhang J, Liu Y, Shi J. Risk of stroke in patients with patent foramen Ovale: an updated meta-analysis of observational studies. J Stroke Cerebrovasc Dis. (2014) 23(5):1207–15. doi: 10.1016/j.jstrokecerebrovasdis.2013.10.018
54. Giannandrea D, Padiglioni C, Eusebi P, Mengoni A, Romoli M, Galati F, et al. Clinical rope (crope) score predicts patent foramen Ovale detection among stroke patients: a multicenter observational study. Neurol Sci. (2020) 41(11):3227–33. doi: 10.1007/s10072-020-04386-6
55. Ng PY, Ng AK, Subramaniam B, Burns SM, Herisson F, Timm FP, et al. Association of preoperatively diagnosed patent foramen Ovale with perioperative ischemic stroke. Jama. (2018) 319(5):452–62. doi: 10.1001/jama.2017.21899
56. Strambo D, Sirimarco G, Nannoni S, Perlepe K, Ntaios G, Vemmos K, et al. Embolic stroke of undetermined source and patent foramen Ovale: risk of paradoxical embolism score validation and atrial fibrillation prediction. Stroke. (2021) 52(5):1643–52. doi: 10.1161/strokeaha.120.032453
57. Shih EK, Beslow LA, Natarajan SS, Falkensammer CB, Messé SR, Ichord RN. Prevalence of patent foramen Ovale in a cohort of children with cryptogenic ischemic stroke. Neurology. (2021) 97(21):e2096–102. doi: 10.1212/wnl.0000000000012892
58. Ntaios G, Sagris D, Strambo D, Perlepe K, Sirimarco G, Georgiopoulos G, et al. Carotid atherosclerosis and patent foramen Ovale in embolic stroke of undetermined source. J Stroke Cerebrovasc Dis. (2021) 30(1):105409. doi: 10.1016/j.jstrokecerebrovasdis.2020.105409
59. West BH, Noureddin N, Mamzhi Y, Low CG, Coluzzi AC, Shih EJ, et al. Frequency of patent foramen Ovale and migraine in patients with cryptogenic stroke. Stroke. (2018) 49(5):1123–8. doi: 10.1161/strokeaha.117.020160
60. Zhao Q, Liu R, Zhou J, Dong Z, Chen Y. Prevalence and grade of rls in migraine: a prospective study of 251 migraineurs by synchronous test of C-tte and C-tcd. Medicine (Baltimore). (2021) 100(4):e24175. doi: 10.1097/md.0000000000024175
61. Iwasaki A, Suzuki K, Takekawa H, Takashima R, Suzuki A, Suzuki S, et al. Prevalence of right to left shunts in Japanese patients with migraine: a single-center study. Intern Med. (2017) 56(12):1491–5. doi: 10.2169/internalmedicine.56.8099
62. Snijder RJ, Luermans JG, de Heij AH, Thijs V, Schonewille WJ, Van De Bruaene A, et al. Patent foramen Ovale with atrial septal aneurysm is strongly associated with migraine with aura: a large observational study. J Am Heart Assoc. (2016) 5(12):e003771. doi: 10.1161/jaha.116.003771
63. Larrosa D, Ramón C, Alvarez R, Martínez-Camblor P, Cernuda E, Pascual J. No relationship between patent foramen Ovale and migraine frequency. Headache. (2016) 56(9):1466–73. doi: 10.1111/head.12945
64. Purandare N, Welsh S, Hutchinson S, Riding G, Burns A, McCollum C. Cerebral emboli and paradoxical embolisation in dementia: a pilot study. Int J Geriatr Psychiatry. (2005) 20(1):12–6. doi: 10.1002/gps.1202
65. Shaikh ZF, Jaye J, Ward N, Malhotra A, de Villa M, Polkey MI, et al. Patent foramen Ovale in severe obstructive sleep apnea: clinical features and effects of closure. Chest. (2013) 143(1):56–63. doi: 10.1378/chest.12-0334
66. Mojadidi MK, Bokhoor PI, Gevorgyan R, Noureddin N, MacLellan WC, Wen E, et al. Sleep apnea in patients with and without a right-to-left shunt. J Clin Sleep Med. (2015) 11(11):1299–304. doi: 10.5664/jcsm.5190
67. Lau EM, Jaijee SK, Melehan KL, Wong KK, Yee BJ, Grunstein RR, et al. Prevalence of patent foramen Ovale and its impact on oxygen desaturation in obstructive sleep apnea. Int J Cardiol. (2013) 165(1):35–40. doi: 10.1016/j.ijcard.2011.07.050
68. Guchlerner M, Kardos P, Liss-Koch E, Franke J, Wunderlich N, Bertog S, et al. Pfo and right-to-left shunting in patients with obstructive sleep apnea. J Clin Sleep Med. (2012) 8(4):375–80. doi: 10.5664/jcsm.2026
69. Beelke M, Angeli S, Del Sette M, Gandolfo C, Cabano ME, Canovaro P, et al. Prevalence of patent foramen Ovale in subjects with obstructive sleep apnea: a transcranial Doppler ultrasound study. Sleep Med. (2003) 4(3):219–23. doi: 10.1016/s1389-9457(02)00256-3
70. Rimoldi SF, Ott S, Rexhaj E, de Marchi SF, Allemann Y, Gugger M, et al. Patent foramen Ovale closure in obstructive sleep apnea improves blood pressure and cardiovascular function. Hypertension. (2015) 66(5):1050–7. doi: 10.1161/hypertensionaha.115.06303
71. Higuchi K, Ishihara H, Okuda S, Kanda F. A 51-year-old man with intramedullary spinal cord abscess having a patent foramen Ovale. BMJ Case Rep. (2011) 2011. doi: 10.1136/bcr.11.2010.3512
72. Oh CH, Lim HK, Chung J, Yoon SH, Park HC, Park CO. The psychopathological influence of congenital heart disease in Korean male adolescents: an analysis of multiphasic personal inventory test results. Yonsei Med J. (2012) 53(6):1107–12. doi: 10.3349/ymj.2012.53.6.1107
73. Dines DE, Arms RA, Bernatz PE, Gomes MR. Pulmonary arteriovenous fistulas. Mayo Clin Proc. (1974) 49(7):460–5.4834927
74. White RI Jr., Lynch-Nyhan A, Terry P, Buescher PC, Farmlett EJ, Charnas L, et al. Pulmonary arteriovenous malformations: techniques and long-term outcome of embolotherapy. Radiology. (1988) 169(3):663–9. doi: 10.1148/radiology.169.3.3186989
75. Topiwala KK, Patel SD, Pervez M, Shovlin CL, Alberts MJ. Ischemic stroke in patients with pulmonary arteriovenous fistulas. Stroke. (2021) 52(7):e311–5. doi: 10.1161/strokeaha.120.032073
76. Kimura K, Minematsu K, Nakajima M. Isolated pulmonary arteriovenous Fistula without rendu-osler-weber disease as a cause of cryptogenic stroke. J Neurol Neurosurg Psychiatry. (2004) 75(2):311–3. Epub 2004/01/27 14742615
77. Sousa S, Vasco Costa N, Carmona C, Coimbra É, Pita F. Recurrent stroke in a young woman with a single pulmonary arteriovenous Fistula: an unusual association. Case Rep Neurol. (2017) 9(3):293–8. doi: 10.1159/000484682
78. Mehrbod N, Chitsaz A, Saadatnia M, Manouchehri N. Stroke in a patient with pulmonary arteriovenous Fistula: a case report study. Adv Biomed Res. (2013) 2:84. doi: 10.4103/2277-9175.122499
79. Fateh-Moghadam S, Dietz R, Bocksch W. Postoperative pulmonary arteriovenous Fistula resulting in recurrent cryptogenic stroke. Int J Cardiol. (2007) 117(1):e43–5. doi: 10.1016/j.ijcard.2006.11.033
80. Felix S, Jeannin S, Goizet C, Thambo JB, Giraud S, Plauchu H, et al. Stroke following pulmonary arteriovenous Fistula embolization in a patient with hht. Neurology. (2008) 71(24):2012–4. doi: 10.1212/01.wnl.0000336973.27761.45
81. Tomelleri G, Bovi P, Carletti M, Mazzucco S, Bazzoli E, Casilli F, et al. Paradoxical brain embolism in a young man with isolated pulmonary arteriovenous Fistula. Neurol Sci. (2008) 29(3):169–71. doi: 10.1007/s10072-008-0930-5
82. Peters B, Ewert P, Schubert S, Abdul-Khaliq H, Lange PE. Rare case of pulmonary arteriovenous Fistula simulating residual defect after transcatheter closure of patent foramen Ovale for recurrent paradoxical embolism. Catheter Cardiovasc Interv. (2005) 64(3):348–51. doi: 10.1002/ccd.20293
83. Wozniak L, Mielczarek M, Sabiniewicz R. Paradoxical brain embolism in a young man: is it only a patent foramen Ovale? Neurol Neurochir Pol. (2015) 49(1):61–4. doi: 10.1016/j.pjnns.2014.12.003
84. Sen G, Lwin MT, Smith L, Weir N. Young stroke due to pulmonary arteriovenous malformation. BMJ Case Rep. (2021) 14(6):e242581. doi: 10.1136/bcr-2021-242581
85. Kakeshita K, Yoneda T, Koba H, Tanimura K, Ueda T, Kaneda T, et al. Case report: migraine that persisted for over 20 years disappears after treatment for pulmonary arteriovenous Fistula. Clin Case Rep. (2020) 8(10):1872–6. doi: 10.1002/ccr3.3037
86. Shioya H, Kikuchi K, Suda Y, Shindo K, Hashimoto M. Recurrent brain abscess associated with congenital pulmonary arteriovenous Fistula: a case report. No Shinkei Geka. (2004) 32(1):57–63.14978925
87. Velthuis S, Buscarini E, Gossage JR, Snijder RJ, Mager JJ, Post MC. Clinical implications of pulmonary shunting on saline contrast echocardiography. J Am Soc Echocardiogr. (2015) 28(3):255–63. doi: 10.1016/j.echo.2014.12.008
88. Lin YS, Liu PH, Wu LS, Chen YM, Chang CJ, Chu PH. Major adverse cardiovascular events in adult congenital heart disease: a population-based follow-up study from Taiwan. BMC Cardiovasc Disord. (2014) 14:38. doi: 10.1186/1471-2261-14-38
89. Magalhães E, Torreão J, Dias J, Cardoso E, Melo A. Atrial septal defects as a risk factor for migraine: a case-control study. Arq Neuropsiquiatr. (2005) 63(4):1042–6. doi: 10.1590/s0004-282-2005000600024
90. Kato Y, Hayashi T, Kobayashi T, Tanahashi N. Migraine prevalence in patients with atrial septal defect. J Headache Pain. (2013) 14(1):63. doi: 10.1186/1129-2377-14-63
91. Kitamura T, Arakawa S, Murao K, Kitazono T, Ago T. Paradoxical brain embolism in elderly subjects with small atrial septal defects. J Stroke Cerebrovasc Dis. (2018) 27(7):1987–91. doi: 10.1016/j.jstrokecerebrovasdis.2018.02.053
92. Karunanithi Z, Nyboe C, Hjortdal VE. Long-Term risk of atrial fibrillation and stroke in patients with atrial septal defect diagnosed in childhood. Am J Cardiol. (2017) 119(3):461–5. doi: 10.1016/j.amjcard.2016.10.015
93. Nyboe C, Nymann AH, Ovesen AS, Elisabeth Hjortdal V. The burden of migraine in adults with atrial septal defect: a nationwide cohort study. Sci Rep. (2019) 9(1):7410. doi: 10.1038/s41598-019-43895-z
94. Liu Y, Li S, Wang R, Han X, Su M, Cao X, et al. A new perspective of migraine symptoms in patients with congenital heart defect. Headache. (2018) 58(10):1601–11. doi: 10.1111/head.13453
95. Udholm S, Nyboe C, Dantoft TM, Jørgensen T, Rask CU, Hjortdal VE. Small atrial septal defects are associated with psychiatric diagnoses, emotional distress, and lower educational levels. Congenit Heart Dis. (2019) 14(5):803–10. doi: 10.1111/chd.12808
96. Shuiab A. Cerebral infarction and ventricular septal defect. Stroke. (1989) 20(7):957–8. doi: 10.1161/01.str.20.7.957
97. Dhoubhadel BG, Laghu U, Poudel R, Morimoto K, Ariyoshi K. A rare case of cryptococcal meningitis in a child with a congenital heart disease. Case Rep Infect Dis. (2021) 2021:9994804. doi: 10.1155/2021/9994804
98. Panagopoulos D, Loukopoulou S, Karanasios E, Eleftherakis N. Recurrent arterial ischemic strokes in a patient with patent foramen Ovale and ductus arteriosus: presentation of our management and review of the literature. Glob Cardiol Sci Pract. (2019) 2019(2):e201913. doi: 10.21542/gcsp.2019.13
99. Ke ZP, Zhang GF, Guo YH, Sun YM, Wang J, Li N, et al. A novel Prrx1 loss-of-function variation contributing to familial atrial fibrillation and congenital patent ductus arteriosus. Genet Mol Biol. (2022) 45(2):e20210378. doi: 10.1590/1678-4685-gmb-2021-0378
100. Zelano J, Dellborg M, Eriksson P, Mandalenakis Z. Epilepsy in patients with congenital heart disease: a nationwide cohort study. Brain Behav. (2022) 12(8):e2699. doi: 10.1002/brb3.2699
101. Tsivgoulis G, Katsanos AH, Mavridis D, Frogoudaki A, Vrettou AR, Ikonomidis I, et al. Percutaneous patent foramen Ovale closure for secondary stroke prevention: network meta-analysis. Neurology. (2018) 91(1):e8–18. doi: 10.1212/wnl.0000000000005739
102. Zhang QQ, Lu JJ, Yan MY, Hu XW, Qin YR, Wang DP, et al. The efficacy of percutaneous patent foramen Ovale closure on migraine: a meta-analysis of randomized controlled trials and observational studies. Biomed Res Int. (2021) 2021:6643266. doi: 10.1155/2021/6643266
103. Hoole SP, Hernández-Sánchez J, Davies WR, McNab DC, Calvert PA, Rana BS, et al. Effects of patent foramen Ovale closure on obstructive sleep apnea syndrome: pcosa study. Can J Cardiol. (2017) 33(12):1708–15. doi: 10.1016/j.cjca.2017.09.005
104. Krishnamurthy Y, Ben-Ami J, Robbins BT, Sommer RJ. Incidence and time course of atrial fibrillation following patent foramen Ovale closure. Catheter Cardiovasc Interv. (2022) 100(2):219–24. doi: 10.1002/ccd.30247
105. Hövels-Gürich HH, Konrad K, Skorzenski D, Nacken C, Minkenberg R, Messmer BJ, et al. Long-Term neurodevelopmental outcome and exercise capacity after corrective surgery for tetralogy of fallot or ventricular septal defect in infancy. Ann Thorac Surg. (2006) 81(3):958–66. doi: 10.1016/j.athoracsur.2005.09.010
106. Delaney JW, Li JS, Rhodes JF. Major complications associated with transcatheter atrial septal occluder implantation: a review of the medical literature and the manufacturer and user facility device experience (maude) database. Congenit Heart Dis. (2007) 2(4):256–64. doi: 10.1111/j.1747-0803.2007.00107.x
107. Hornung M, Bertog SC, Franke J, Id D, Taaffe M, Wunderlich N, et al. Long-Term results of a randomized trial comparing three different devices for percutaneous closure of a patent foramen Ovale. Eur Heart J. (2013) 34(43):3362–9. doi: 10.1093/eurheartj/eht283
108. Chen JZ, Thijs VN. Atrial fibrillation following patent foramen Ovale closure: systematic review and meta-analysis of observational studies and clinical trials. Stroke. (2021) 52(5):1653–61. doi: 10.1161/strokeaha.120.030293
109. Dumitrescu A, Lane GK, Wilkinson JL, Goh TH, Penny DJ, Davis AM. Transcatheter closure of perimembranous ventricular septal defect. Heart. (2007) 93(7):867.; author reply doi: doi: 10.1136/hrt.2007.119461
110. Jiang D, Han B, Zhao L, Yi Y, Zhang J, Fan Y, et al. Transcatheter device closure of perimembranous and intracristal ventricular septal defects in children: medium- and long-term results. J Am Heart Assoc. (2021) 10(11):e020417. doi: 10.1161/jaha.120.020417
111. Lam JY, Lopushinsky SR, Ma IWY, Dicke F, Brindle ME. Treatment options for pediatric patent ductus arteriosus: systematic review and meta-analysis. Chest. (2015) 148(3):784–93. doi: 10.1378/chest.14-2997
112. Conrad C, Newberry D. Understanding the pathophysiology, implications, and treatment options of patent ductus arteriosus in the neonatal population. Adv Neonatal Care. (2019) 19(3):179–87. doi: 10.1097/anc.0000000000000590
113. Robertson CM, Joffe AR, Sauve RS, Rebeyka IM, Phillipos EZ, Dyck JD, et al. Outcomes from an interprovincial program of newborn open heart surgery. J Pediatr. (2004) 144(1):86–92. doi: 10.1016/j.jpeds.2003.09.048
114. Jufar AH, Lankadeva YR, May CN, Cochrane AD, Marino B, Bellomo R, et al. Renal and cerebral hypoxia and inflammation during cardiopulmonary bypass. Compr Physiol. (2021) 12(1):2799–834. doi: 10.1002/cphy.c210019
115. Reves JG. Toward understanding cerebral blood flow during cardiopulmonary bypass: implications for the central nervous system. Anesthesiology. (2019) 130(4):609–13. doi: 10.1097/aln.0000000000002580
116. Hanley FL, Ito H, Gu M, Hurd R, Riemer RK, Spielman D. Comparison of dynamic brain metabolism during antegrade cerebral perfusion versus deep hypothermic circulatory arrest using proton magnetic resonance spectroscopy. J Thorac Cardiovasc Surg. (2020) 160(4):e225–7. doi: 10.1016/j.jtcvs.2019.10.103
117. Tu LN, Timms AE, Kibiryeva N, Bittel D, Pastuszko A, Nigam V, et al. Transcriptome profiling reveals activation of inflammation and apoptosis in the neonatal Striatum after deep hypothermic circulatory arrest. J Thorac Cardiovasc Surg. (2019) 158(3):882–90.e4. doi: 10.1016/j.jtcvs.2019.02.091
118. Tveita T, Sieck GC. Physiological impact of hypothermia: the good, the bad, and the ugly. Physiology (Bethesda). (2022) 37(2):69–87. doi: 10.1152/physiol.00025.2021
119. Yu D, Li L, Yuan W. Neonatal anesthetic neurotoxicity: insight into the molecular mechanisms of long-term neurocognitive deficits. Biomed Pharmacother. (2017) 87:196–9. doi: 10.1016/j.biopha.2016.12.062
120. Liu M, Li Y, Gao S, Yan S, Zhang Q, Liu G, et al. A novel target to reduce microglial inflammation and neuronal damage after deep hypothermic circulatory arrest. J Thorac Cardiovasc Surg. (2020) 159(6):2431–44.e7. doi: 10.1016/j.jtcvs.2019.06.115
121. Squiers JJ, Arsalan M, Lima B, DiMaio JM. Cerebral protection during deep hypothermic circulatory arrest: can a molecular approach via microrna inhibition improve on a Millennia-old strategy? J Thorac Cardiovasc Surg. (2015) 150(3):684–6. doi: 10.1016/j.jtcvs.2015.05.022
122. Agrimi J, Spalletti C, Baroni C, Keceli G, Zhu G, Caragnano A, et al. Obese mice exposed to psychosocial stress display cardiac and hippocampal dysfunction associated with local brain-derived neurotrophic factor depletion. EBioMedicine. (2019) 47:384–401. doi: 10.1016/j.ebiom.2019.08.042
123. Moshensky A, Brand CS, Alhaddad H, Shin J, Masso-Silva JA, Advani I, et al. Effects of Mango and mint pod-based E-cigarette aerosol inhalation on inflammatory states of the brain, lung, heart, and colon in mice. eLife. (2022) 11:e67621. doi: 10.7554/eLife.67621
124. Tsao CW, Aday AW, Almarzooq ZI, Alonso A, Beaton AZ, Bittencourt MS, et al. Heart disease and stroke statistics-2022 update: a report from the American heart association. Circulation. (2022) 145(8):e153–639. doi: 10.1161/cir.0000000000001052
125. Baroni C, Lionetti V. The impact of sex and gender on heart-brain axis dysfunction: current concepts and novel perspectives. Can J Physiol Pharmacol. (2021) 99(2):151–60. doi: 10.1139/cjpp-2020-0391
126. Hogue CW, Lillie R, Hershey T, Birge S, Nassief AM, Thomas B, et al. Gender influence on cognitive function after cardiac operation. Ann Thorac Surg. (2003) 76(4):1119–25. doi: 10.1016/s0003-4975(03)00817-8
127. Chen L, Deng W, Palacios I, Inglessis-Azuaje I, McMullin D, Zhou D, et al. Patent foramen Ovale (pfo), stroke and pregnancy. J Investig Med. (2016) 64(5):992–1000. doi: 10.1136/jim-2016-000103
128. Majnemer A, Limperopoulos C, Shevell M, Rohlicek C, Rosenblatt B, Tchervenkov C. Gender differences in the developmental outcomes of children with congenital cardiac defects. Cardiol Young. (2012) 22(5):514–9. doi: 10.1017/s1047951111002071
129. Battaglini D, Robba C, Lopes da Silva A, Dos Santos Samary C, Leme Silva P, Dal Pizzol F, et al. Brain-Heart interaction after acute ischemic stroke. Crit Care. (2020) 24(1):163. doi: 10.1186/s13054-020-02885-8
130. Costagliola G, Orsini A, Coll M, Brugada R, Parisi P, Striano P. The brain-heart interaction in epilepsy: implications for diagnosis, therapy, and sudep prevention. Ann Clin Transl Neurol. (2021) 8(7):1557–68. doi: 10.1002/acn3.51382
131. Villringer A, Laufs U. Heart failure, cognition, and brain damage. Eur Heart J. (2021) 42(16):1579–81. doi: 10.1093/eurheartj/ehab061
132. Acharya S, Salgado-Somoza A, Stefanizzi FM, Lumley AI, Zhang L, Glaab E, et al. Non-Coding rnas in the brain-heart axis: the case of Parkinson's disease. Int J Mol Sci. (2020) 21(18):6513. doi: 10.3390/ijms21186513
133. Xu C, Zheng A, He T, Cao Z. Brain-Heart axis and biomarkers of cardiac damage and dysfunction after stroke: a systematic review and meta-analysis. Int J Mol Sci. (2020) 21(7):2347. doi: 10.3390/ijms21072347
134. Yang Z, Huang C, Wen X, Liu W, Huang X, Li Y, et al. Circular rna circ-Foxo3 attenuates blood-brain barrier damage by inducing autophagy during ischemia/reperfusion. Mol Ther. (2022) 30(3):1275–87. doi: 10.1016/j.ymthe.2021.11.004
135. Jurcau A, Simion A. Neuroinflammation in cerebral ischemia and ischemia/reperfusion injuries: from pathophysiology to therapeutic strategies. Int J Mol Sci. (2021) 23(1):14. doi: 10.3390/ijms23010014
136. Goadsby PJ, Holland PR, Martins-Oliveira M, Hoffmann J, Schankin C, Akerman S. Pathophysiology of migraine: a disorder of sensory processing. Physiol Rev. (2017) 97(2):553–622. doi: 10.1152/physrev.00034.2015
137. Villa RF, Ferrari F, Moretti A. Post-Stroke depression: mechanisms and pharmacological treatment. Pharmacol Ther. (2018) 184:131–44. doi: 10.1016/j.pharmthera.2017.11.005
138. Chaumont-Dubel S, Dupuy V, Bockaert J, Bécamel C, Marin P. The 5-ht(6) receptor interactome: new insight in receptor signaling and its impact on brain physiology and pathologies. Neuropharmacology. (2020) 172:107839. doi: 10.1016/j.neuropharm.2019.107839
Keywords: brain disease, congenital heart abnormality, patent foramen ovale, atrial septal defect, pulmonary arteriovenous fistula
Citation: Sha L, Li Y, Zhang Y, Tang Y, Li B, Chen Y and Chen L (2023) Heart-brain axis: Association of congenital heart abnormality and brain diseases. Front. Cardiovasc. Med. 10:1071820. doi: 10.3389/fcvm.2023.1071820
Received: 17 October 2022; Accepted: 13 March 2023;
Published: 29 March 2023.
Edited by:
Arun Bandyopadhyay, Indian Institute of Chemical Biology (CSIR), IndiaReviewed by:
Jahaira Lopez-Pastrana, Jefferson University Hospitals, United StatesVincenzo Lionetti, Sant'Anna School of Advanced Studies, Italy
© 2023 Sha, Li, Zhang, Tang, Li, Chen and Chen. This is an open-access article distributed under the terms of the Creative Commons Attribution License (CC BY). The use, distribution or reproduction in other forums is permitted, provided the original author(s) and the copyright owner(s) are credited and that the original publication in this journal is cited, in accordance with accepted academic practice. No use, distribution or reproduction is permitted which does not comply with these terms.
*Correspondence: Lei Chen leilei_25@126.com
Specialty Section: This article was submitted to General Cardiovascular Medicine, a section of the journal Frontiers in Cardiovascular Medicine