- 1Ningbo Institute of Medical Science, The Affiliated Hospital of Medical School of Ningbo University, Ningbo, Zhejiang, China
- 2Department of Cardiovascular Center, The Affiliated Hospital of Medical School of Ningbo University, Ningbo, Zhejiang, China
The human body seems like a “balance integrator.” On the one hand, the body constantly actively receives various outside stimuli and signals to induce changes. On the other hand, several internal regulations would be initiated to adapt to these changes. In most cases, the body could keep the balance in vitro and in vivo to reach a healthy body. However, in some cases, the body can only get to a pathological balance. Actively exposed to unhealthy lifestyles and passively adapting to individual primary diseases lead to a similarly inner environment for both heart failure and cancer. To cope with these stimuli, the body must activate the system regulation mechanism and face the mutual interference. This review summarized the association between heart failure and cancer from active exposure to passive adaption. Moreover, we hope to inspire researchers to contemplate these two diseases from the angle of overall body consideration.
Introduction
The most hazardous and complicated diseases are heart failure and cancer. So far, clinical and basic researchers have formed theoretical principles and related treatments for each disease. However, according to epidemiology studies, heart failure and cancer coexist in a similar population. Although not very comprehensive, the new emerging Cardiac-Oncology has been set up to mainly focus on the heart toxic during the anti-cancer treatment process. Many reviews have given excellent summaries about their associations from the co-incidence, similar risk factors, and correlated regulation mechanisms, which indicated some underlying clues for both diseases (1, 2). Considering each individual as a whole study subject, heart failure and cancer are different manifestations of physical problems but can originate from similar physical backgrounds.
Heart failure and cancer populations tend to actively expose themselves to similar lifestyles or have to adapt to their pre-existing physical condition (Figure 1). These stimulations may disturb the primary balance of the individual. They will activate several regulation systems to amend those unbalance, even though some are not physical but pathological, such as the neuroendocrine system, immune system, gut microbiome, and intercellular communication via various cytokines and molecules. Furthermore, both could produce mutual interference during their progression and drug treatment. Our review aims to focus on these two diseases and summarize their correlation from active exposure to passive adaptation.
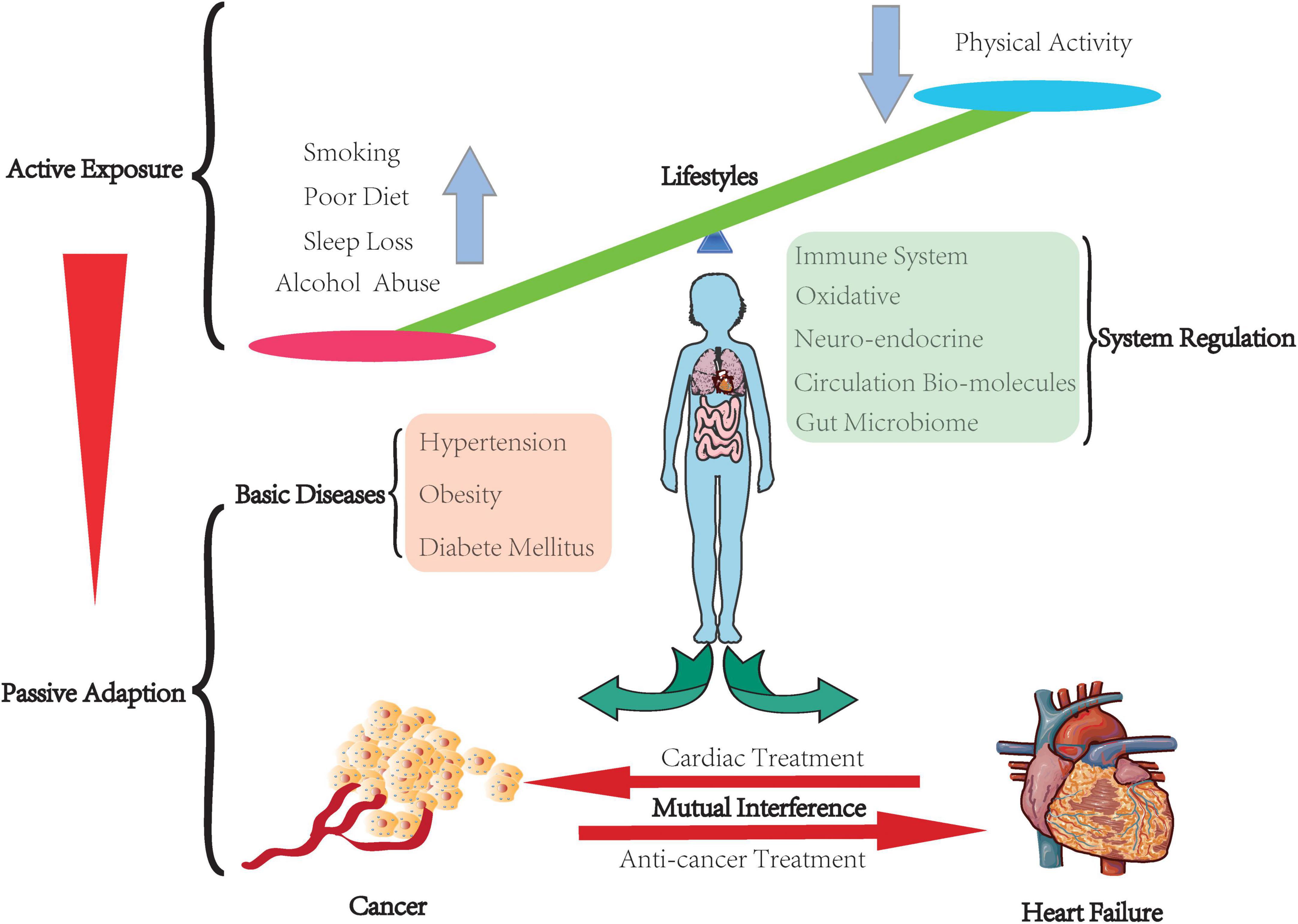
Figure 1. Balance in the human body. The human body keeps the balance through people actively exposing to their lifestyles, which leads to various inter-environment. Some of them have to face their basic diseases. The body has to passively adapt to this inter-environment by arousing the system regulation to correct those temporary unbalance or compensate to a certain pathological state. Furthermore, cancer and heart failure have mutual interference.
Active exposure
An unhealthy lifestyle leads to a vast significant influence on the incidence of cardiovascular (CV) diseases and cancer. Unfortunately, many unhealthy lifestyles exist for humans, such as smoking, alcohol addiction, lack of physical exercise, loss of sleep, and poor diet.
Smoking is an essential factor for heart failure and various cancers. On the one hand, tobacco increases the risk of heart failure (HF) by coronary artery disease-dependent mechanisms (3). Continued smoking deteriorates the prognosis of patients with HF, while suspending smoking could decrease the risk of major adverse cardiac events (3). On the other hand, smoking has essential effects on various kinds of cancers, such as lung cancer (4), breast cancer (5), cervical cancer (6), and liver cancer (7). The mechanisms involve several secondary toxicities of tobacco, including irritants, carcinogens, pro-inflammatory stimuli, and oxidizing agents (8). Among them, nicotine contributes to the pathogenesis of CV diseases and cancer (9, 10). It could directly depress apoptosis and promote angiogenesis (8). Likely, smoking-related cancer is also prevalent in patients with CV diseases (11).
Although low-to-moderate alcohol consumption might be beneficial for cardiac function, a chronically large quantity of ethanol consumption is toxic to the heart and vascular, even progressing to heart failure (12). In addition, pre-existing cardiovascular diseases may be deteriorated by alcohol abuse, such as hypertension and cardiomyopathy. Again, about 4% of cancer in the global world is caused by alcohol consumption (13). In addition, it could increase the risk of digestive system cancer and sex-related cancer, such as breast cancer (13). Harriet Rumgay has reviewed the mechanism by which ethanol could produce metabolite to acetaldehyde, which could cause DNA damage and inhibit DNA synthesis and repair (13).
Physical activity has been widely studied as a protection factor for HF patients. Exercise can provide primary prevention for past onset HF and secondary prevention for present HF and can also be used as a prognostic factor for predicting the future of HF patients (14). A meta-analysis result indicated that high levels of total physical activity, leisure-time activity, vigorous activity, occupational activity, walking and bicycling combined, and cardio-respiratory fitness could reduce the risk of heart failure (15). As a comparison, in cancer, a systematic review compared the highest to lowest physical activity levels and summarized that approximately 10–20% risk reduction could be reached in bladder, breast, colon, endometrial, esophageal adenocarcinoma, renal, and gastric cancer (16). Again, according to 18 systematic reviews and meta-analyses, a great deal of physical activity could reduce 40–50% risk of all-cause and cancer-specific mortality in patients with breast, colorectal, or prostate cancer (16). About 40% reduction in cancer incidence and cancer-related death is benefited from increased physical activity (17).
Sleep loss could also result in harmful outcomes, including heart diseases, certain cancers, and all-cause mortality (18–22). According to the data from the health and retirement study in the United States, insomnia symptoms, both cumulatively and individually, are associated with incident HF (23). Sleep loss is also related to various kinds of cancer, such as neck and head cancer (24), prostate cancer (25), and malignant brain tumor (26). Michael et al. reported that sleep loss might activate spontaneous cellular innate immunity (27). They hypothesize that treatments for short sleep duration have the potential to inhibit inflammation and decrease the risk for inflammatory disorders and some cancers in humans (27).
The habit of diet is also crucial for people. Poor diet is present in different ways and contexts. As we all know, some familiar diet habits could cause acute injury and chronic toxicity to the human body. For example, hot food would break down the esophageal mucosa and cause esophageal cancer (28), while moldy food would produce aflatoxin and be associated with liver cancer (29). Furthermore, some new evidence indicated the interaction between diet habits and the human body. For example, an excessive high-fat diet (HFD) would induce toxicity to the heart in rats by promoting cardiac injury biomarker leakage into plasma and altering heart rate and electrocardiogram pattern, as well as plasma ion levels (30). In addition, HFD could induce apoptosis and inflammation in rat hearts, which was supported by detecting higher expression levels of Bax and caspase-3 and a large amount of cardiac cellular DNA fragmentation (30). Similarly, HFD could induce colorectal tumorigenesis by destroying the gut barrier and leading to dysregulation of microbial and metabolomic (31). Besides, excessive intake of red meat is related to cancer and heart failure. According to an umbrella review, red meat consumption was related to a growing risk of overall cancer mortality, including non-Hodgkin lymphoma (NHL), bladder, breast, colorectal, endometrial, esophageal, gastric, lung, and nasopharyngeal cancer (32). A cohort study that involved 29,682 participants found that excessive intake of processed red meat, unprocessed red meat, and poultry, but not fish, was significantly associated with exposure to cardiovascular diseases and all-cause mortality (33). On the contrary, healthy diet patterns could reduce cancer and heart failure incidence, like adequate blood sugar control decreases the incidence of CV disease and cancer (34). Several famous healthy diet patterns could prevent HF. For example, Dietary Approaches to Stop Hypertension (DASH) advocates high potassium and low sodium, sulforaphane (SFA), and total fat (35), while MedDiet (Mediterranean) stresses more unsaturated fatty acids (UFA) (36), which are rich mainly in antioxidants and anti-inflammatory nutrients, and offers a solid and inverse correlation with cardiovascular diseases (37). MedDiet and DASH diets are particularly rich in plant-based foods but limited in processed foods and red meat (38).
Passive adaption
Basic diseases: Acceptation of imperfections
Various chronic diseases would force the human body to passively adopt these changes and create a unique background for populations with different diseases.
For the terms of heart, hypertension, obesity, and diabetes mellitus (DM) are harmful to vascular and metabolism, which would finally lead to heart failure (39–47). These changes often start with different interrelated processes but end with HF. Obesity and diabetes mellitus always cause damage to the vascular by inducing inflammation and atherosclerosis to increase vessel stiffness and peripheral vascular resistance because of long-term immersion in high blood fatty acid and sugar. Increased vascular resistance leads to hypertension, forms high pressure for cardiac afterload, and forces myocardial hypertrophy. Furthermore, a high concentration of blood fatty acid and sugar would increase the blood volume, which increases cardiac preload. Overall, the heart always tries to adapt to handle increased preload and afterload, but then it would not endure them, followed by decompensation, leading to heart failure. Some treatments can be used to reverse such dysfunction. For example, strict glycemic control and high-quality insulin therapy could reduce severe cardiac dysfunction in patients with diabetes mellitus (48). Besides, it is interesting that there is a paradox between obesity and HF. Obese people are more likely to develop HF, but they have a survival advantage (49). However, the mechanism is not very clear.
In terms of cancer, although the underlying mechanisms are not very clear, a series of reports supported the significant relationship between chronic diseases and cancer. A large prospective cohort study of over 400,000 subjects indicated that chronic diseases (CV, diabetes, chronic kidney disease, pulmonary disease, and gouty arthritis) were independently related to cancer incidence based on a regular risk score (50). Moreover, the accumulative score of chronic diseases has a dose-dependent relationship with cancer incidence and mortality (50). For example, in patients with hypertension, a 10mmHg increment in blood pressure was associated with an increased risk of cancer incidence (HR 1.07, 95% CI 1.04–1.09) in men and cancer-related mortality (HR 1.12, 95% CI 1.08–1.15 and HR 1.06, 95% CI 1.02–1.11, respectively) both in men and women (51). Obesity is associated with a chronic pro-inflammatory state, which could induce DNA damage and cancer incidence (52). Fatty tissue also plays the role of a sizeable endocrine organ, which could produce a great deal of estrogen and promotes hormone-related cancers, such as ovarian and breast cancer (53). Furthermore, adipose tissue could secrete many adipokines related to cellular survival. For example, leptin was one of the well-known adipokines with cell-proliferative effects (54), while another well-known adipokine, adiponectin, was reported to have anti-proliferative effects (54). Besides, insulin and insulin-like growth factors (IGF-1) were increased in obese subjects. High levels of IGF-1 were reported to relate to the development of cancer (55), which was hypothesized and observed to increase cancer incidence by promoting cell proliferation (56–58). Therefore, lowering weight or reducing the weight loss by surgery would reduce cancer risk (59, 60).
Systematic adjustment
Immune system: Chronic inflammation
The immune system is involved in developing cancer and HF (61, 62). Atsushi Anzai has a well-reviewed immune system in CV diseases (63). There are several stages during the HF process. In the early stage after myocardial infarction (MI), different immune cells move to the injury area and try to constrain and restore the primary damage. While in the late stage, a low grade of chronic activation could induce heart modification (64, 65). First, neutrophils rapidly move to the damaged area and activate a pro-inflammatory phase after MI. The infiltrated neutrophil population changed along with the healing process and gradually acquired surface lectin SiglecF (66). Then, macrophages infiltrate and resolute the necrotic tissue and start the process of scar formation in the coming 3–30 days. In HFpEF (heart failure with preserved ejection fraction) heart, cardiac macrophages induce myocardial cell death and interstitial fibrosis. Stefan Frantz et al. have systematically reviewed the function of macrophages in different stages of ischemic heart diseases (67). Interestingly, macrophages show heterogeneity because of their sources. CCR2+ (C-C chemokine receptor +) macrophages come from embryonic, the primary resident population in a healthy heart. However, ischemic cardiac injury induces monocyte-derived CCR2– macrophages to infiltrate the heart (68, 69). Engulfment results in increased fatty acid in macrophages, activate mitochondrial respiration, and initiate anti-inflammatory responses during the wound healing process (68, 70). The following remodeling phase will involve low-grade inflammation and non-infarcted myocardium, which are regulated by cytokines and innate immune receptors. It is a long, complicated process involving not only various kinds of immune cells but also numerous pro-inflammatory cytokines, which are increased and may contribute to the development of HF (71). Activated dendritic cells induce B- and T-cell proliferation by migrating from injury myocardium to pericardial adipose tissue fat-associated lymphoid clusters (FALCs) (66, 68). In response to acute injury of the heart, a group of innate B cells within FALCs expressed a considerable amount of granulocyte-macrophage colony-stimulating factor (GM-CSF), induced interleukin-23 (IL23), and interleukin-17 (IL17) secreted from immune cells (66, 68). However, some regulation mechanisms play protective effects. A subpopulation of macrophages with GATA binding protein 6 (GATA6) expression could inhibit excessive cardiac fibrosis (68), while Group 2 Innate lymphoid cells (ILC2) population stimulated by interleukin-2 (IL2) expanded in pericardial adipose tissue to protect cardiac function (68).
In cancer, the abnormal immune system is also related to cancer progression and metastases (72). Currently, immune therapy focused on the immune checkpoint has obtained spectacular results (73). However, limitations were revealed by the wide use of these drugs. Targeting the tumor immune system may be at the cost of deteriorating the myocardial immune system (74). Meijers et al. reported that new-onset cancer could be predicted by high-sensitivity C-reactive protein and mid-regional pro-adrenomedullin (75).
Inflammation could also play a crossroads between CV diseases and cancer. In Canakinumab Antiinflammatory Thrombosis Outcome Study (CANTOS) trial, the interleukin-1 (IL-1) blocker canakinumab was used to test whether IL-1 inhibition could attenuate coronary events in future (76). Compared with placebo, canakinumab can reduce about 25% of major adverse CV events (HR 0.75, 95% CI 0.66–0.85) (76). More interestingly, treatment with canakinumab could also decrease the incidence of lung cancer and mortality in a significant dose-dependent manner [Incidence: HR 0.33, 95% CI 0.18–0.59, P < 0.0001; Mortality: HR 0.23, 95% CI 0.10–0.54, P = 0.0002] (77).
Oxidative stress
Oxidative stress originates from unbalancing between reactive oxygen species (ROS) generation and antioxidant (78), which was defined as an excessive accumulation of ROS relating to antioxidant defense (78). ROS can be produced through several sources (78). Among them, mitochondria produce ROS by transporting a single electron to molecular oxygen (78). Mitochondrial oxidative phosphorylation provides high energy to support the heart’s function and is involved in cancer progression (79).
In HF, Hill and Singal reported that antioxidant deficits and oxidative stress coexist in patients of HF after MI, which may affect cardiac function (80). Also, ischemia or hypoxia would induce ROS increase and be related to myocyte damage of MI (81). Because of abnormal mitochondrial metabolism in HF, glycolysis increases lactate production in a failing heart (82).
In cancer, altered mitochondrial metabolism promotes the glycolysis to adapt to the rapid proliferate tumor cells. Pyruvate dehydrogenase (PDH) and PDH kinase (PDK) are two key modulators. PDH controls the rate of glucose oxidation, while PDK inhibits PDH (82). Interestingly, PDK upregulation and PDH inhibition are in both HF and tumor cells (83). On the one hand, dichloro (a PDK inhibitor) could enhance PDH activity to lower ischemic injury and improve cardiac function. On the other hand, it could also reduce cancer development (83).
Neuroendocrine: Non-coordinated activation
As we all know, the neuroendocrine system plays the most crucial role in cardiovascular regulation. For the cardiovascular system, there are many neurohormonal pathways, firstly serving as a compensation mechanism, including the renin-angiotensin-aldosterone system (RAAS), sympathetic nervous system (SNS), and natriuretic peptides system (NPS) (Figure 2). They could be promptly activated to react to cardiovascular events when a certain balance was disrupted at a sudden time. Still, gradually chronic neuroendocrine non-coordinated activation contributes to disease progression by promoting cardiac remodeling and deteriorating heart function (84).
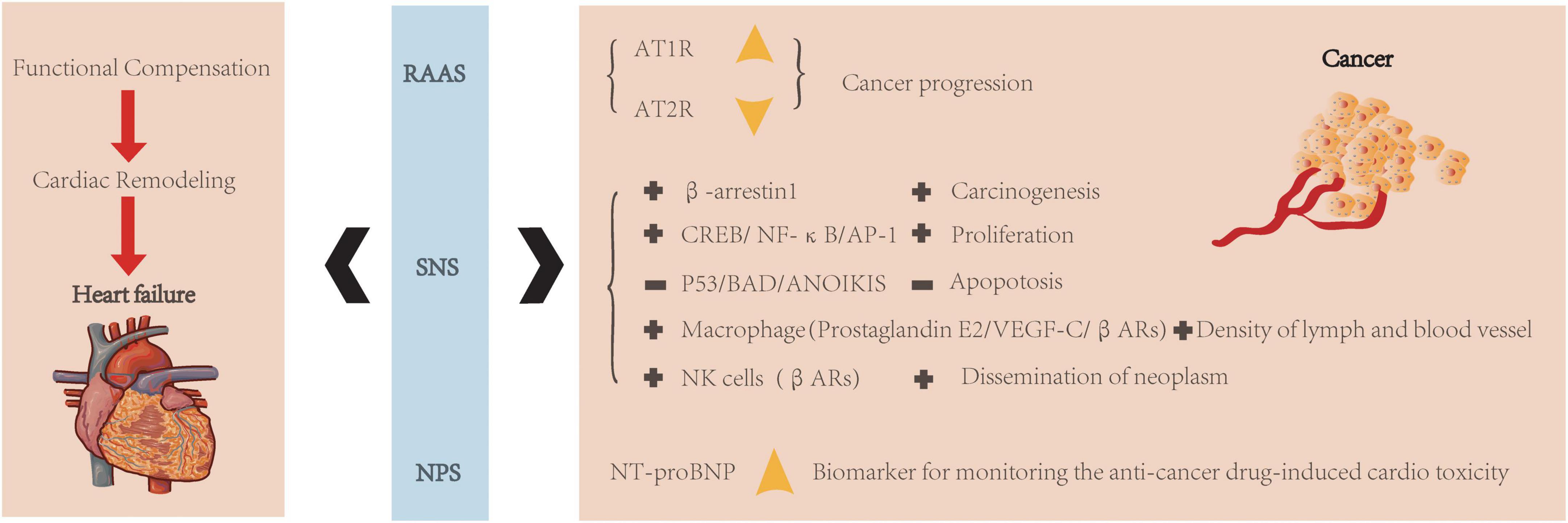
Figure 2. Neuroendocrine regulation of heart failure and cancer. Neuroendocrine system (RAAS, SNS, and NPS) is related to both heart and cancer. They were always aroused to realize functional compensation, but following with cardiac remodeling and then leads to heart failure. In RAAS, increased AT1R and decreased AT2R were associated with cancer progression. In SNS, various kinds of signaling pathways are related to cancer progression (+: increased expression; -: decreased expression). In NPS, NT-proBNP could be a biomarker for monitoring anti-cancer treatment-induced cardiotoxicity.
However, compared to the heart, local RAAS hormones and receptors differ in various cancers (85). For example, angiotensin II receptor type 1 (AT1R) increased expression during cancer progression, while Ang II/At2R signaling exerts the opposite effect (85) (Figure 2). Over-activity of SNS may result in carcinogenesis via the β-arrestin-1 signaling pathway (86). It also could induce cell proliferation through specific molecular pathways, such as cAMP-response element binding protein (CREB), nuclear factor-k-gene binding (NF-kB), and activator protein-1 (AP-1) (87). Besides, it confers resistance to apoptosis through various mechanisms, such as inhibition of p53 (87), proapoptotic protein BAD (bcl2 associated death promoter) (88), and anoikis (89). Because of the broad expression of β1 and β2 ARs (β1 and β2 adrenoceptor agonists) in cancer, β-blockers might be a candidate target for cancer treatment (90, 91). The SNS could also modify the cancer microenvironment (92). For example, in response to the β-adrenoceptor agonist (βAR) stimulation, tumor-associated macrophages release prostaglandin E2 and stimulate vascular endothelial growth factor C (VEGF-C) expression to increase lymph and blood vessel density (93). Moreover, the SNS could suppress natural killer cells by activating βAR activity promoting neoplasms dissemination (94). Besides, the role of natriuretic peptides in carcinogenesis has also been accessed (94). In addition, N-terminal pro-brain natriuretic peptide (NT-proBNP) has been verified to be involved in cancer progression and could play as a biomarker for monitoring anti-cancer drug-induced cardiotoxicity (95).
Circulation molecules: Exosome-mediated indirectly regulation
Microvesicles, especially exosomes, play an essential role in various diseases. They bring nuclear acid and protein molecules to participate in cell communication. Exosomal miRNAs are vital in diagnosis biomarkers for certain diseases because of the tissue specificity. For example, in the heart of a patient with heart failure, secreted Exo-microRNA-21-5p damages the regenerative potential of the heart (96). Exo-miR-92b-5p has also been verified as a biomarker for HF (97). In cancer, a massive amount of miRNAs not only regulate cancer progression (98) but also participate in creating the tumor microenvironment. For example, exo-miR-522 derived from cancer-associated fibroblasts inhibited ferroptosis in the cancer cell and led to chemoresistance in GC (gastric cancer) (99). For example, tumor-derived exo-miR-934 can regulate the communication between colorectal cancer cells and tumor-associated macrophages to stimulate colorectal cancer liver metastasis (100).
Because of tissue-specific differences, many kinds of circulation miRNAs were studied as biomarkers of certain diseases, such as heart failure and cancer. However, circulation exosomal miRNAs might affect the whole body. In this review, we summarized the function and mechanism for several kinds of miRNAs and tried to analyze the entire effect of specific miRNAs on both heart and cancer (Table 1).
Gut microbiome: Bidirectional regulation
The human microbiome is composed of various microorganisms (142, 143). Bacteroidetes, Firmicutes, Proteobacteria, and Actinobacteria are the four main bacterial species (144). The human microbial ecosystem is not only composed of a part of the human but also positively participates in human health and disease by regulating the function of the mucosal barrier, immune state, growth of pathological organisms, and metabolisms (145–149).
Accumulating evidence indicated that changes in the gut microbial community were involved in cardiovascular disease (150). Coriobacteriaceae, Erysipelotrichaceae, Ruminococcaceae (family level), and Blautia (genus level) were decreased in chronic HF (151). Eubacterium rectal and Dorea long catena from the Lachnospiraceae family (152) and Faecalibacterium from the Ruminococcaceae family were decreasing in older patients (152) and patients with HF (153). In addition, the metabolism derived from the gut is closely related to HF. In 2013, trimethylamine-N-oxide (TMAO) was first reported as a predictor of CV events (154). Decreasing butyrate and increasing TMAO have been consistently verified in heart failure (150). TMAO accumulation stimulates platelet aggregation, promotes foam cell formation, induces inflammation, and reduces reverse cholesterol transport (154–157).
In contrast, the microbiome is also related to the carcinogenesis and progression of various cancers by producing toxic metabolites or carcinogens (158). In addition, it can cause inflammation or immune suppression and indirectly lead to carcinogenesis (158). In patients with CRC, the fecal microbiota belongs to Bacteroidetes (mainly Porphyromonas and Prevotella) and Firmicutes (mainly Enterococcus and Streptococcus) (159). Helicobacter Pylori is GC-related bacteria. It can suppress macrophages and T cells via protein VacA (159) and inhibit epithelial cell apoptosis (160). Compared to the sterile stomach in healthy individuals, the gut of GC patients harbors a complex microbial ecosystem, including Proteobacteria, Firmicutes, Actinobacteria, Bacteroides, and Fusobacteriaphyla (161, 162). In liver cancer, a high-fat diet led to the enrichment of Clostridium species and accelerated the progression of liver cancer by producing excess secondary BA deoxycholic acid (163). However, growing evidence showed that bacteria could defend gastroenteric tumors by promoting the host’s anti-tumor immunity (164–167).
Researchers have observed massive data about the gut microbiome changes in certain cancers or heart failure (Figure 3). However, we lack data on cancer combined with heart failure. The gut microbiome could not only indicate the prognosis of cancer and heart failure but also have the potential as probiotics to treat the two diseases. The critical point is that the experimental design cohort should focus on combining both cancers and heart failure so that we can find out the categories and mechanisms of the particular gut microbiome and consciously adjust their component to help deal with both cancers and heart failure.
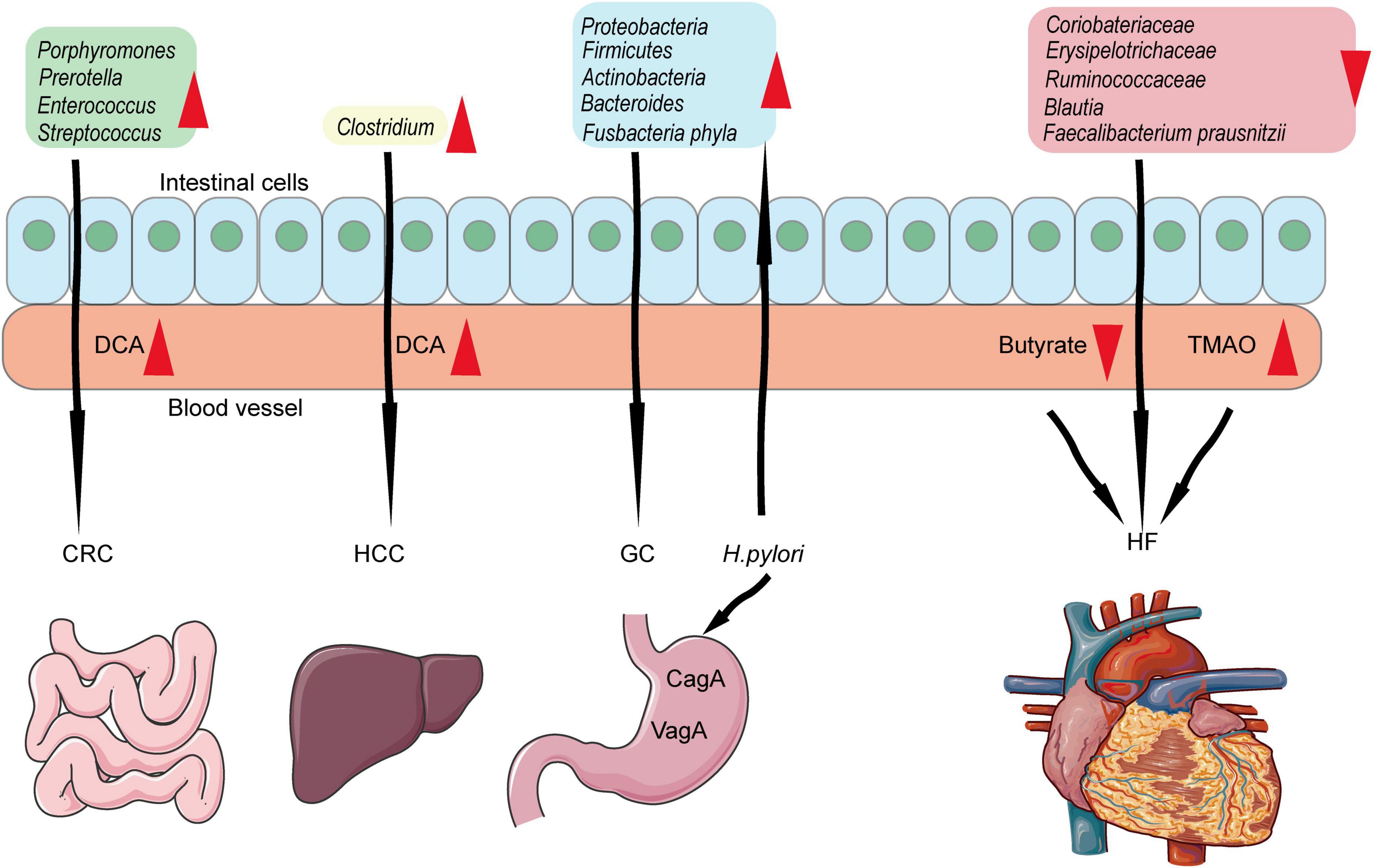
Figure 3. Associations among gut microbiome, cancers, and heart failure. Porphyromonas, Prevotella, Enterococcus, and Streptococcus increased in intestinal and produced DCA which lead to CRC formation and progression. Clostridium is related to HCC. Proteobacteria, Firmicutes, Actinobacteria, Bacteroides, and Fusobacteriaphyla are related to GC. Besides, Helicobacter pylori could induce GC-related gut microbiome which in turn lead to GC. Coriobacteriaceae, Erysipelotrichaceae, Ruminococcaceae, Blautia, and Faecalibacterium are related to heart failure by producing TMAO and decreasing butyrate.
Mutual interference
The causal relationship between cancer and heart failure
Considering the angle of the individual, it is hard to distinguish the causal relationship between cancer and heart failure. Apart from cancer, infection and multi-organ failure are the second ranks of death in cancer patients (168). However, it is still unclear about the cause of death in advanced cancer (169). Anker et al. have proposed a hypothesis: “advanced cancer is also a heart failure syndrome” in their review (170). They hypothesized that cancer is related to severe tissue inflammation, oxidative stress, and local neurohormonal abnormal activation resulting in heart atrophy, increasing ventricular wall stress, and arrhythmias due to electrical instability and death (170). Furthermore, they hypothesized that heart atrophy might be the tip of the iceberg on the progression of loss of skeletal muscle mass (170). In turn, we can also hypothesize that heart failure is the cause of cancer. Heart failure leads to inadequate organ perfusion, inducing tissue ischemia and hypoxia and promoting carcinogenesis and progression. Some researchers have provided data to support this hypothesis. For example, heart failure patients secrete more circulating factors (serpinA3 and A1, fibronectin, ceruloplasmin, and paraoxonase 1) to promote cancer growth (75). For another, a meta-analysis summarized that heart failure increased the risk of cancer incidence and mortality (171).
Toxic to heart during the anti-cancer treatment
In a majority of anti-cancer treatments, no matter whether for common chemotherapeutic drugs or target inhibitors, the effects are not specific to the tumor itself. Their mechanisms are based on cell biology, which processes in every cell, killing cancer and causing organ damage. As we all know, the common side effect is losing hair, vomiting, and losing weight. Besides, the various kinds of toxicity to the heart by anti-cancer treatments are receiving more and more attention in the present study, which leads to the establishment of Cardio-Oncology. In addition, the particular point is that the cardiovascular response to cancer treatment may differ in age and sex (172–174). Large numbers of researchers have reported related heart toxicity mechanisms during anti-cancer treatment. Moreover, many reviews have well summarized this part of the section (175). We tried to list several kinds of drugs and associated mechanisms for heart failure in Table 2.
Although many side effects on the heart have been found during cancer treatment, some measures can be used to reduce these side effects. For example, empagliflozin (EMPA) could prevent doxorubicin-induced cardiotoxicity (176, 177) by inhibiting ferroptosis, fibrosis, apoptosis, and inflammation through nucleotide-binding oligomerization domain, leucine-rich repeat and pyrin domain-containing 3 (NLRP3) and myeloid differentiation factor 88 (MyD88)-related pathways (178). Such combined treatment is deserved to be encouraged to make more attempts.
Cancer risk and prognosis during the cardiac-related treatment
It is challenging to clarify the relationship between CV drugs and cancer risk. According to a large meta-analysis, angiotensin receptor blockers (ARBs), angiotensin-converting enzyme inhibitors (ACEIs), β-blockers, diuretics, and calcium channel blockers (CCBs) caused 5–10% cancer risk or cancer-related death (212). However, in the other two meta-analyses, data showed an uncertain result. In type 2 DM, the overall occurrence of cancer was negatively related to losartan [odds ratio (OR) 0.78, 95% CI 0.63–0.97], but was positively related to candesartan (OR 1.79, 95% CI 1.05–3.06) and telmisartan (OR 1.54, 95% CI 0.97–2.43) (213). Although aspirin was reported to prevent adverse events in CV diseases (214), low-dose aspirin in a low –medium CV risk population was not associated with a lower cancer incidence (215). The mechanisms of aspirin are different in CV diseases and cancer. Antiplatelet effects are the prominent mechanism of aspirin in CV diseases, while cyclooxygenase-dependent and independent mechanisms play the leading roles in cancer (216). Compared with control, low-dose aspirin was related to markedly higher sensitivity for detecting advanced colorectal neoplasms (217). In this review, we listed several main cardiovascular drugs and cancer risk mechanisms in Table 3.
Summary
This review discussed the relationships between heart failure and cancer from active exposure to passive adaption. People actively select various lifestyles, such as smoking habits, anchor, diet, sleep, and physical activity. These would construct a specific internal environment to passively adapt to these stimuli and try to keep the balance of each individual by inducing various regulation systems, like the neuroendocrine system, immune system, gut microbiome, and intercellular molecules communication through microvesicle transportation. Besides, clinical treatments used in heart failure or cancer could also cause mutual interference with each other. The above series of outer exposures and inner system responses will help us better understand why these two complicated diseases always exist in a similar population and how to coordinate various treatments for both diseases. The best aim for curing heart failure or cancer is not only to reduce the side effect to the lowest level but also to reach a win–win situation for both heart failure and cancer.
Author contributions
YD contributed to the concept of review and wrote the manuscript. TW contributed to the concept of review and revised the manuscript. Both authors contributed to the article and approved the submitted version.
Funding
This work was supported by the National Natural Science Foundation of China (grant number: 81602136), the Natural Science Foundation of Ningbo (grant number: 2018A610394), and the Zhejiang Provincial Medical and Health Science Foundation (grant number: 2019KY613).
Conflict of interest
The authors declare that the research was conducted in the absence of any commercial or financial relationships that could be construed as a potential conflict of interest.
Publisher’s note
All claims expressed in this article are solely those of the authors and do not necessarily represent those of their affiliated organizations, or those of the publisher, the editors and the reviewers. Any product that may be evaluated in this article, or claim that may be made by its manufacturer, is not guaranteed or endorsed by the publisher.
References
1. Bertero E, Canepa M, Maack C, Ameri P. Linking heart failure to cancer: background evidence and research perspectives. Circulation. (2018) 138:735–42. doi: 10.1161/CIRCULATIONAHA.118.033603
2. Lam BQ, Srivastava R, Morvant J, Shankar S, Srivastava RK. Association of diabetes mellitus and alcohol abuse with cancer: molecular mechanisms and clinical significance. Cells. (2021) 10:3077. doi: 10.3390/cells10113077
3. Grubb AF, Greene SJ, Fudim M, Dewald T, Mentz RJ. Drugs of abuse and heart failure. J Card Fail. (2021) 27:1260–75.
4. Loeb LA, Ernster VL, Warner KE, Abbotts J, Laszlo J. Smoking and lung cancer: an overview. Cancer Res. (1984) 44:5940–58.
5. Macacu A, Autier P, Boniol M, Boyle P. Active and passive smoking and risk of breast cancer: a meta-analysis. Breast Cancer Res Treat. (2015) 154:213–24. doi: 10.1007/s10549-015-3628-4
6. Su B, Qin W, Xue F, Wei X, Guan Q, Jiang W, et al. The relation of passive smoking with cervical cancer: a systematic review and meta-analysis. Medicine (Baltimore). (2018) 97:e13061. doi: 10.1097/MD.0000000000013061
7. Jain D, Chaudhary P, Varshney N, Bin Razzak KS, Verma D, Khan Zahra TR, et al. Tobacco smoking and liver cancer risk: potential avenues for carcinogenesis. J Oncol. (2021) 2021:5905357. doi: 10.1155/2021/5905357
8. Morris PB, Ference BA, Jahangir E, Feldman DN, Ryan JJ, Bahrami H, et al. Cardiovascular effects of exposure to cigarette smoke and electronic cigarettes: clinical perspectives from the prevention of cardiovascular disease section leadership council and early career councils of the American college of cardiology. J Am Coll Cardiol. (2015) 66:1378–91. doi: 10.1016/j.jacc.2015.07.037
9. Sanner T, Grimsrud TK. Nicotine: carcinogenicity and effects on response to cancer treatment – a review. Front Oncol. (2015) 5:196. doi: 10.3389/fonc.2015.00196
10. Ambrose JA, Barua RS. The pathophysiology of cigarette smoking and cardiovascular disease: an update. J Am Coll Cardiol. (2004) 43:1731–7. doi: 10.1016/j.jacc.2003.12.047
11. van Kruijsdijk RC, van der Graaf Y, Peeters PH, Visseren FL. Cancer risk in patients with manifest vascular disease: effects of smoking, obesity, and metabolic syndrome. Cancer Epidemiol Biomarkers Prev. (2013) 22:1267–77. doi: 10.1158/1055-9965.EPI-13-0090
12. Gardner JD, Mouton AJ. Alcohol effects on cardiac function. Compr Physiol. (2015) 5:791–802. doi: 10.1002/cphy.c140046
13. Rumgay H, Murphy N, Ferrari P, Soerjomataram I. Alcohol and cancer: epidemiology and biological mechanisms. Nutrients. (2021) 13:3173. doi: 10.3390/nu13093173
14. Cattadori G, Segurini C, Picozzi A, Padeletti L, Anza C. Exercise and heart failure: an update. ESC Heart Fail. (2018) 5:222–32. doi: 10.1002/ehf2.12225
15. Aune D, Schlesinger S, Leitzmann MF, Tonstad S, Norat T, Riboli E, et al. Physical activity and the risk of heart failure: a systematic review and dose-response meta-analysis of prospective studies. Eur J Epidemiol. (2021) 36:367–81. doi: 10.1007/s10654-020-00693-6
16. McTiernan A, Friedenreich CM, Katzmarzyk PT, Powell KE, Macko R, Buchner D, et al. Physical activity in cancer prevention and survival: a systematic review. Med Sci Sports Exerc. (2019) 51:1252–61. doi: 10.1249/MSS.0000000000001937
17. Kushi LH, Doyle C, McCullough M, Rock CL, Demark-Wahnefried W, Bandera EV, et al. American cancer society guidelines on nutrition and physical activity for cancer prevention: reducing the risk of cancer with healthy food choices and physical activity. CA Cancer J Clin. (2012) 62:30–67. doi: 10.3322/caac.20140
18. Dew MA, Hoch CC, Buysse DJ, Monk TH, Begley AE, Houck PR, et al. healthy older adults’ sleep predicts all-cause mortality at 4 to 19 years of follow-up. Psychosom Med. (2003) 65:63–73. doi: 10.1097/01.psy.0000039756.23250.7c
19. Irwin MR. Depression and insomnia in cancer: prevalence, risk factors, and effects on cancer outcomes. Curr Psychiatry Rep. (2013) 15:404. doi: 10.1007/s11920-013-0404-1
20. Irwin MR. Why sleep is important for health: a psychoneuroimmunology perspective. Annu Rev Psychol. (2015) 66:143–72. doi: 10.1146/annurev-psych-010213-115205
21. Li Y, Zhang X, Winkelman JW, Redline S, Hu FB, Stampfer M, et al. Association between Insomnia symptoms and mortality: a prospective study of U.S. Men. Circulation. (2014) 129:737–46. doi: 10.1161/CIRCULATIONAHA.113.004500
22. Vgontzas AN, Liao D, Pejovic S, Calhoun S, Karataraki M, Basta M, et al. Insomnia with short sleep duration and mortality: the Penn state cohort. Sleep. (2010) 33:1159–64. doi: 10.1093/sleep/33.9.1159
23. Mahmood A, Ray M, Dobalian A, Ward KD, Ahn S. Insomnia symptoms and incident heart failure: a population-based cohort study. Eur Heart J. (2021) 42:4169–76. doi: 10.1093/eurheartj/ehab500
24. Seifen C, Huppertz T, Matthias C, Gouveris H. Obstructive sleep apnea in patients with head and neck cancer-more than just a comorbidity? Medicina (Kaunas). (2021) 57:1174. doi: 10.3390/medicina57111174
25. Sparasci D, Napoli I, Rossi L, Pereira-Mestre R, Manconi M, Treglia G, et al. Prostate cancer and sleep disorders: a systematic review. Cancers (Basel). (2022) 14:1784. doi: 10.3390/cancers14071784
26. Oreskovic D, Kastelancic A, Raguz M, Dlaka D, Predrijevac N, Matec D, et al. The vicious interplay between disrupted sleep and malignant brain tumors: a narrative review. Croat Med J. (2021) 62:376–86. doi: 10.3325/cmj.2021.62.376
27. Irwin MR, Witarama T, Caudill M, Olmstead R, Breen EC. Sleep loss activates cellular inflammation and signal transducer and activator of transcription (Stat) family proteins in humans. Brain Behav Immun. (2015) 47:86–92. doi: 10.1016/j.bbi.2014.09.017
28. Shen Y, Xie S, Zhao L, Song G, Shao Y, Hao C, et al. Estimating individualized absolute risk for esophageal squamous cell carcinoma: a population-based study in high-risk areas of China. Front Oncol. (2020) 10:598603. doi: 10.3389/fonc.2020.598603
29. Groopman JD, Smith JW, Rivera-Andrade A, Alvarez CS, Kroker-Lobos MF, Egner PA, et al. Aflatoxin and the etiology of liver cancer and its implications for Guatemala. World Mycotoxin J. (2021) 14:305–17. doi: 10.3920/wmj2020.2641
30. Feriani A, Bizzarri M, Tir M, Aldawood N, Alobaid H, Allagui MS, et al. High-fat diet-induced aggravation of cardiovascular impairment in permethrin-treated Wistar rats. Ecotoxicol Environ Saf. (2021) 222:112461. doi: 10.1016/j.ecoenv.2021.112461
31. Yang J, Wei H, Zhou Y, Szeto CH, Li C, Lin Y, et al. High-fat diet promotes colorectal tumorigenesis through modulating gut microbiota and metabolites. Gastroenterology. (2022) 162:135–149.e2. doi: 10.1053/j.gastro.2021.08.041.
32. Huang Y, Cao D, Chen Z, Chen B, Li J, Guo J, et al. Red and processed meat consumption and cancer outcomes: umbrella review. Food Chem. (2021) 356:129697. doi: 10.1016/j.foodchem.2021.129697
33. Zhong VW, Van Horn L, Greenland P, Carnethon MR, Ning H, Wilkins JT, et al. Associations of processed meat, unprocessed red meat, poultry, or fish intake with incident cardiovascular disease and all-cause mortality. JAMA Intern Med. (2020) 180:503–12. doi: 10.1001/jamainternmed.2019.6969
34. Rasmussen-Torvik LJ, Shay CM, Abramson JG, Friedrich CA, Nettleton JA, Prizment AE, et al. Ideal cardiovascular health is inversely associated with incident cancer: the atherosclerosis risk in communities study. Circulation. (2013) 127:1270–5. doi: 10.1161/CIRCULATIONAHA.112.001183
35. Rifai L, Silver MA. A review of the dash diet as an optimal dietary plan for symptomatic heart failure. Prog Cardiovasc Dis. (2016) 58:548–54. doi: 10.1016/j.pcad.2015.11.001
36. Martinez-Gonzalez MA. Benefits of the Mediterranean diet beyond the Mediterranean sea and beyond food patterns. BMC Med. (2016) 14:157. doi: 10.1186/s12916-016-0714-3
37. Mentella MC, Scaldaferri F, Ricci C, Gasbarrini A, Miggiano GAD. Cancer and Mediterranean diet: a review. Nutrients. (2019) 11:2059. doi: 10.3390/nu11092059
38. Billingsley HE, Hummel SL, Carbone S. The role of diet and nutrition in heart failure: a state-of-the-art narrative review. Prog Cardiovasc Dis. (2020) 63:538–51. doi: 10.1016/j.pcad.2020.08.004
39. Slivnick J, Lampert BC. Hypertension and heart failure. Heart Fail Clin. (2019) 15:531–41. doi: 10.1016/j.hfc.2019.06.007
40. Di Palo KE, Barone NJ. Hypertension and heart failure: prevention, targets, and treatment. Heart Fail Clin. (2020) 16:99–106. doi: 10.1016/j.hfc.2019.09.001
41. Harada T, Obokata M. Obesity-related heart failure with preserved ejection fraction: pathophysiology, diagnosis, and potential therapies. Heart Fail Clin. (2020) 16:357–68. doi: 10.1016/j.hfc.2020.02.004
42. Pandey A, Patel KV, Vaduganathan M, Sarma S, Haykowsky MJ, Berry JD, et al. Physical activity, fitness, and obesity in heart failure with preserved ejection fraction. JACC Heart Fail. (2018) 6:975–82. doi: 10.1016/j.jchf.2018.09.006
43. Dunlay SM, Givertz MM, Aguilar D, Allen LA, Chan M, Desai AS, et al. Type 2 diabetes mellitus and heart failure: a scientific statement from the American heart association and the heart failure society of America: this statement does not represent an update of the 2017 Acc/Aha/Hfsa heart failure guideline update. Circulation. (2019) 140:e294–324. doi: 10.1161/CIR.0000000000000691
44. Kenny HC, Abel ED. Heart failure in type 2 diabetes mellitus. Circ Res. (2019) 124:121–41. doi: 10.1161/CIRCRESAHA.118.311371
45. Lehrke M, Marx N. Diabetes mellitus and heart failure. Am J Med. (2017) 130:S40–50. doi: 10.1016/j.amjmed.2017.04.010
46. Lawes CM, Vander Hoorn S, Rodgers A. Global burden of blood-pressure-related disease, 2001. Lancet. (2008) 371:1513–8. doi: 10.1016/S0140-6736(08)60655-8
47. Drazner MH. The progression of hypertensive heart disease. Circulation. (2011) 123:327–34. doi: 10.1161/CIRCULATIONAHA.108.845792
48. Konduracka E, Gackowski A, Rostoff P, Galicka-Latala D, Frasik W, Piwowarska W. Diabetes-Specific cardiomyopathy in type 1 diabetes mellitus: no evidence for its occurrence in the era of intensive insulin therapy. Eur Heart J. (2007) 28:2465–71. doi: 10.1093/eurheartj/ehm361
49. Horwich TB, Fonarow GC, Hamilton MA, MacLellan WR, Woo MA, Tillisch JH. The relationship between obesity and mortality in patients with heart failure. J Am Coll Cardiol. (2001) 38:789–95. doi: 10.1016/s0735-1097(01)01448-6
50. Tu H, Wen CP, Tsai SP, Chow WH, Wen C, Ye Y, et al. Cancer risk associated with chronic diseases and disease markers: prospective cohort study. BMJ. (2018) 360:k134. doi: 10.1136/bmj.k134
51. Stocks T, Van Hemelrijck M, Manjer J, Bjorge T, Ulmer H, Hallmans G, et al. Blood pressure and risk of cancer incidence and mortality in the metabolic syndrome and cancer project. Hypertension. (2012) 59:802–10. doi: 10.1161/HYPERTENSIONAHA.111.189258
52. Gregor MF, Hotamisligil GS. Inflammatory mechanisms in obesity. Annu Rev Immunol. (2011) 29:415–45. doi: 10.1146/annurev-immunol-031210-101322
53. Gerard C, Brown KA. Obesity and breast cancer – role of estrogens and the molecular underpinnings of aromatase regulation in breast adipose tissue. Mol Cell Endocrinol. (2018) 466:15–30. doi: 10.1016/j.mce.2017.09.014
54. Vansaun MN. Molecular pathways: adiponectin and leptin signaling in cancer. Clin Cancer Res. (2013) 19:1926–32. doi: 10.1158/1078-0432.CCR-12-0930
55. Gallagher EJ, LeRoith D. Obesity and diabetes: the increased risk of cancer and cancer-related mortality. Physiol Rev. (2015) 95:727–48. doi: 10.1152/physrev.00030.2014
56. Renehan AG, Frystyk J, Flyvbjerg A. Obesity and cancer risk: the role of the insulin-Igf axis. Trends Endocrinol Metab. (2006) 17:328–36. doi: 10.1016/j.tem.2006.08.006
57. Basen-Engquist K, Chang M. Obesity and cancer risk: recent review and evidence. Curr Oncol Rep. (2011) 13:71–6. doi: 10.1007/s11912-010-0139-7
58. Ballotari P, Vicentini M, Manicardi V, Gallo M, Chiatamone Ranieri S, Greci M, et al. Diabetes and risk of cancer incidence: results from a population-based cohort study in Northern Italy. BMC Cancer. (2017) 17:703. doi: 10.1186/s12885-017-3696-4
59. Keum N, Greenwood DC, Lee DH, Kim R, Aune D, Ju W, et al. Adult weight gain and adiposity-related cancers: a dose-response meta-analysis of prospective observational studies. J Natl Cancer Inst. (2015) 107:djv088. doi: 10.1093/jnci/dju428
60. Tee MC, Cao Y, Warnock GL, Hu FB, Chavarro JE. Effect of bariatric surgery on oncologic outcomes: a systematic review and meta-analysis. Surg Endosc. (2013) 27:4449–56. doi: 10.1007/s00464-013-3127-9
61. Swann JB, Smyth MJ. Immune surveillance of tumors. J Clin Invest. (2007) 117:1137–46. doi: 10.1172/JCI31405
62. Zhang Y, Bauersachs J, Langer HF. Immune mechanisms in heart failure. Eur J Heart Fail. (2017) 19:1379–89. doi: 10.1002/ejhf.942
63. Anzai A, Ko S, Fukuda K. Immune and inflammatory networks in myocardial infarction: current research and its potential implications for the clinic. Int J Mol Sci. (2022) 23:5214. doi: 10.3390/ijms23095214
64. Epelman S, Liu PP, Mann DL. Role of innate and adaptive immune mechanisms in cardiac injury and repair. Nat Rev Immunol. (2015) 15:117–29. doi: 10.1038/nri3800
65. Frantz S, Falcao-Pires I, Balligand JL, Bauersachs J, Brutsaert D, Ciccarelli M, et al. The innate immune system in chronic cardiomyopathy: a European society of cardiology (Esc) scientific statement from the working group on myocardial function of the Esc. Eur J Heart Fail. (2018) 20:445–59. doi: 10.1002/ejhf.1138
66. Horckmans M, Bianchini M, Santovito D, Megens RTA, Springael JY, Negri I, et al. Pericardial adipose tissue regulates granulopoiesis, fibrosis, and cardiac function after myocardial infarction. Circulation. (2018) 137:948–60. doi: 10.1161/CIRCULATIONAHA.117.028833
67. Frantz S, Nahrendorf M. Cardiac macrophages and their role in ischaemic heart disease. Cardiovasc Res. (2014) 102:240–8. doi: 10.1093/cvr/cvu025
68. Steffens S, Nahrendorf M, Madonna R. Immune cells in cardiac homeostasis and disease: emerging insights from novel technologies. Eur Heart J. (2022) 43:1533–41. doi: 10.1093/eurheartj/ehab842
69. King KR, Aguirre AD, Ye YX, Sun Y, Roh JD, Ng RP Jr., et al. Irf3 and type I interferons fuel a fatal response to myocardial infarction. Nat Med. (2017) 23:1481–7. doi: 10.1038/nm.4428
70. Vafadarnejad E, Rizzo G, Krampert L, Arampatzi P, Arias-Loza AP, Nazzal Y, et al. Dynamics of cardiac neutrophil diversity in murine myocardial infarction. Circ Res. (2020) 127:e232–49. doi: 10.1161/CIRCRESAHA.120.317200
71. Anker SD, von Haehling S. Inflammatory mediators in chronic heart failure: an overview. Heart. (2004) 90:464–70. doi: 10.1136/hrt.2002.007005
72. Pandya PH, Murray ME, Pollok KE, Renbarger JL. The immune system in cancer pathogenesis: potential therapeutic approaches. J Immunol Res. (2016) 2016:4273943. doi: 10.1155/2016/4273943
73. Pardoll DM. The blockade of immune checkpoints in cancer immunotherapy. Nat Rev Cancer. (2012) 12:252–64. doi: 10.1038/nrc3239
74. Moslehi JJ, Salem JE, Sosman JA, Lebrun-Vignes B, Johnson DB. Increased reporting of fatal immune checkpoint inhibitor-associated myocarditis. Lancet. (2018) 391:933. doi: 10.1016/S0140-6736(18)30533-6
75. Meijers WC, Maglione M, Bakker SJL, Oberhuber R, Kieneker LM, de Jong S, et al. Heart Failure stimulates tumor growth by circulating factors. Circulation. (2018) 138:678–91. doi: 10.1161/CIRCULATIONAHA.117.030816
76. Ridker PM, MacFadyen JG, Everett BM, Libby P, Thuren T, Glynn RJ. Relationship of C-Reactive protein reduction to cardiovascular event reduction following treatment with canakinumab: a secondary analysis from the cantos randomised controlled trial. Lancet. (2018) 391:319–28. doi: 10.1016/S0140-6736(17)32814-3
77. Ridker PM, MacFadyen JG, Thuren T, Everett BM, Libby P, Glynn RJ. Effect of interleukin-1beta Inhibition with canakinumab on incident lung cancer in patients with atherosclerosis: exploratory results from a randomised, double-blind, placebo-controlled trial. Lancet. (2017) 390:1833–42. doi: 10.1016/S0140-6736(17)32247-X
78. Tsutsui H, Kinugawa S, Matsushima S. Oxidative stress and heart failure. Am J Physiol Heart Circ Physiol. (2011) 301:H2181–90. doi: 10.1152/ajpheart.00554.2011
79. Maiuri MC, Kroemer G. Essential role for oxidative phosphorylation in cancer progression. Cell Metab. (2015) 21:11–2. doi: 10.1016/j.cmet.2014.12.013
80. Hill MF, Singal PK. Right and left myocardial antioxidant responses during heart failure subsequent to myocardial infarction. Circulation. (1997) 96:2414–20. doi: 10.1161/01.cir.96.7.2414
81. Perrelli MG, Pagliaro P, Penna C. Ischemia/reperfusion injury and cardioprotective mechanisms: role of mitochondria and reactive oxygen species. World J Cardiol. (2011) 3:186–200. doi: 10.4330/wjc.v3.i6.186
82. Lopaschuk GD, Ussher JR, Folmes CD, Jaswal JS, Stanley WC. Myocardial fatty acid metabolism in health and disease. Physiol Rev. (2010) 90:207–58. doi: 10.1152/physrev.00015.2009
83. Bonnet S, Archer SL, Allalunis-Turner J, Haromy A, Beaulieu C, Thompson R, et al. A mitochondria-K+ channel axis is suppressed in cancer and its normalization promotes apoptosis and inhibits cancer growth. Cancer Cell. (2007) 11:37–51. doi: 10.1016/j.ccr.2006.10.020
84. De Angelis E, Pecoraro M, Rusciano MR, Ciccarelli M, Popolo A. Cross-talk between neurohormonal pathways and the immune system in heart failure: a review of the literature. Int J Mol Sci. (2019) 20:1698. doi: 10.3390/ijms20071698
85. Ino K, Shibata K, Kajiyama H, Yamamoto E, Nagasaka T, Nawa A, et al. Angiotensin Ii Type 1 receptor expression in ovarian cancer and its correlation with tumour angiogenesis and patient survival. Br J Cancer. (2006) 94:552–60. doi: 10.1038/sj.bjc.6602961
86. Hara MR, Kovacs JJ, Whalen EJ, Rajagopal S, Strachan RT, Grant W, et al. A stress response pathway regulates DNA damage through Beta2-adrenoreceptors and Beta-Arrestin-1. Nature. (2011) 477:349–53. doi: 10.1038/nature10368
87. Zhang D, Ma QY, Hu HT, Zhang M. Beta2-adrenergic antagonists suppress pancreatic cancer cell invasion by inhibiting Creb, Nfkappab and Ap-1. Cancer Biol Ther. (2010) 10:19–29. doi: 10.4161/cbt.10.1.11944
88. Hassan S, Karpova Y, Baiz D, Yancey D, Pullikuth A, Flores A, et al. Behavioral stress accelerates prostate cancer development in mice. J Clin Invest. (2013) 123:874–86. doi: 10.1172/JCI63324
89. Sood AK, Armaiz-Pena GN, Halder J, Nick AM, Stone RL, Hu W, et al. Adrenergic modulation of focal adhesion kinase protects human ovarian cancer cells from anoikis. J Clin Invest. (2010) 120:1515–23. doi: 10.1172/JCI40802
90. Coelho M, Soares-Silva C, Brandao D, Marino F, Cosentino M, Ribeiro L. Beta-adrenergic modulation of cancer cell proliferation: available evidence and clinical perspectives. J Cancer Res Clin Oncol. (2017) 143:275–91. doi: 10.1007/s00432-016-2278-1
91. Barron TI, Connolly RM, Sharp L, Bennett K, Visvanathan K. Beta blockers and breast cancer mortality: a population- based study. J Clin Oncol. (2011) 29:2635–44. doi: 10.1200/JCO.2010.33.5422
92. Cole SW, Nagaraja AS, Lutgendorf SK, Green PA, Sood AK. Sympathetic Nervous system regulation of the tumour microenvironment. Nat Rev Cancer. (2015) 15:563–72. doi: 10.1038/nrc3978
93. Armaiz-Pena GN, Gonzalez-Villasana V, Nagaraja AS, Rodriguez-Aguayo C, Sadaoui NC, Stone RL, et al. Adrenergic regulation of monocyte chemotactic protein 1 leads to enhanced macrophage recruitment and ovarian carcinoma growth. Oncotarget. (2015) 6:4266–73. doi: 10.18632/oncotarget.2887
94. Shakhar G, Ben-Eliyahu S. In vivo beta-adrenergic stimulation suppresses natural killer activity and compromises resistance to tumor metastasis in rats. J Immunol. (1998) 160:3251–8.
95. Pavo N, Raderer M, Hulsmann M, Neuhold S, Adlbrecht C, Strunk G, et al. Cardiovascular biomarkers in patients with cancer and their association with all-cause mortality. Heart. (2015) 101:1874–80. doi: 10.1136/heartjnl-2015-307848
96. Qiao L, Hu S, Liu S, Zhang H, Ma H, Huang K, et al. Microrna-21-5p dysregulation in exosomes derived from heart failure patients impairs regenerative potential. J Clin Invest. (2019) 129:2237–50. doi: 10.1172/JCI123135
97. Wu T, Chen Y, Du Y, Tao J, Li W, Zhou Z, et al. Circulating exosomal Mir-92b-5p is a promising diagnostic biomarker of heart failure with reduced ejection fraction patients hospitalized for acute heart failure. J Thorac Dis. (2018) 10:6211–20. doi: 10.21037/jtd.2018.10.52
98. Li X, Zeng Z, Wang J, Wu Y, Chen W, Zheng L, et al. Microrna-9 and breast cancer. Biomed Pharmacother. (2020) 122:109687. doi: 10.1016/j.biopha.2019.109687
99. Zhang H, Deng T, Liu R, Ning T, Yang H, Liu D, et al. Caf secreted Mir-522 suppresses ferroptosis and promotes acquired chemo-resistance in gastric cancer. Mol Cancer. (2020) 19:43. doi: 10.1186/s12943-020-01168-8
100. Zhao S, Mi Y, Guan B, Zheng B, Wei P, Gu Y, et al. Tumor-derived exosomal Mir-934 induces macrophage M2 polarization to promote liver metastasis of colorectal cancer. J Hematol Oncol. (2020) 13:156. doi: 10.1186/s13045-020-00991-2
101. Wu T, Chen Y, Du Y, Tao J, Zhou Z, Yang Z. Serum exosomal Mir-92b-5p as a potential biomarker for acute heart failure caused by dilated cardiomyopathy. Cell Physiol Biochem. (2018) 46:1939–50. doi: 10.1159/000489383
102. Nakano T, Chen IH, Wang CC, Chen PJ, Tseng HP, Huang KT, et al. Circulating exosomal Mir-92b: its role for cancer immunoediting and clinical value for prediction of posttransplant hepatocellular carcinoma recurrence. Am J Transplant. (2019) 19:3250–62. doi: 10.1111/ajt.15490
103. Li M, Shan W, Hong B, Zou J, Li H, Han D, et al. Circulating Mir-92b and Mir-375 for monitoring the chemoresistance and prognosis of small cell lung cancer. Sci Rep. (2020) 10:12705. doi: 10.1038/s41598-020-69615-6
104. Min L, Chen L, Liu S, Yu Y, Guo Q, Li P, et al. Loss of circulating exosomal Mir-92b is a novel biomarker of colorectal cancer at early stage. Int J Med Sci. (2019) 16:1231–7. doi: 10.7150/ijms.34540
105. Gao F, Kataoka M, Liu N, Liang T, Huang ZP, Gu F, et al. Therapeutic role of Mir-19a/19b in cardiac regeneration and protection from myocardial infarction. Nat Commun. (2019) 10:1802. doi: 10.1038/s41467-019-09530-1
106. Kalampogias A, Oikonomou E, Siasos G, Theofilis P, Dimitropoulos S, Gazouli M, et al. Differential expression of micrornas in acute and chronic heart failure. Curr Med Chem. (2022) 29:5130–8. doi: 10.2174/0929867329666220426095655
107. Su Y, Sun Y, Tang Y, Li H, Wang X, Pan X, et al. Circulating Mir-19b-3p as a novel prognostic biomarker for acute heart failure. J Am Heart Assoc. (2021) 10:e022304. doi: 10.1161/JAHA.121.022304
108. Kral J, Korenkova V, Novosadova V, Langerova L, Schneiderova M, Liska V, et al. Expression profile of Mir-17/92 cluster is predictive of treatment response in rectal cancer. Carcinogenesis. (2018) 39:1359–67. doi: 10.1093/carcin/bgy100
109. Wang J, Peng X, Li R, Liu K, Zhang C, Chen X, et al. Evaluation of serum Mir-17-92 Cluster as noninvasive biomarkers for bladder cancer diagnosis. Front Oncol. (2021) 11:795837. doi: 10.3389/fonc.2021.795837
110. Plum PS, Warnecke-Eberz U, Drebber U, Chon SH, Alakus H, Holscher AH, et al. Upregulation of Mir-17-92 cluster is associated with progression and lymph node metastasis in oesophageal adenocarcinoma. Sci Rep. (2019) 9:12113. doi: 10.1038/s41598-019-48624-0
111. Zhang Y, Yin Y, Li S. Detection of circulating exosomal Mir-17-5p serves as a novel non-invasive diagnostic marker for non-small cell lung cancer patients. Pathol Res Pract. (2019) 215:152466. doi: 10.1016/j.prp.2019.152466
112. Chen J, Zhang K, Zhi Y, Wu Y, Chen B, Bai J, et al. Tumor-derived exosomal Mir-19b-3p facilitates M2 macrophage polarization and exosomal Linc00273 secretion to promote lung adenocarcinoma metastasis via hippo pathway. Clin Transl Med. (2021) 11:e478. doi: 10.1002/ctm2.478
113. Duca RB, Massillo C, Dalton GN, Farre PL, Grana KD, Gardner K, et al. Mir-19b-3p and Mir-101-3p as potential biomarkers for prostate cancer diagnosis and prognosis. Am J Cancer Res. (2021) 11:2802–20.
114. Ding H, Wang Y, Hu L, Xue S, Zhang L, Zhang Y, et al. Combined detection of Mir-21-5p, Mir-30a-3p, Mir-30a-5p, Mir-155-5p, Mir-216a and Mir-217 for screening of early heart failure diseases. Biosci Rep. (2020) 40:BSR20191653. doi: 10.1042/BSR20191653
115. Al-Hayali MA, Sozer V, Durmus S, Erdenen F, Altunoglu E, Gelisgen R, et al. Clinical value of circulating microribonucleic acids Mir-1 and Mir-21 in evaluating the diagnosis of acute heart failure in asymptomatic type 2 diabetic patients. Biomolecules. (2019) 9:193. doi: 10.3390/biom9050193
116. Lam J, Clark EC, Fong EL, Lee EJ, Lu S, Tabata Y, et al. Evaluation of cell-laden polyelectrolyte hydrogels incorporating poly(L-Lysine) for applications in cartilage tissue engineering. Biomaterials. (2016) 83:332–46. doi: 10.1016/j.biomaterials.2016.01.020
117. He Q, Ye A, Ye W, Liao X, Qin G, Xu Y, et al. Cancer-secreted exosomal Mir-21-5p induces angiogenesis and vascular permeability by targeting Krit1. Cell Death Dis. (2021) 12:576. doi: 10.1038/s41419-021-03803-8
118. Soliman SE, Abdelaleem AH, Alhanafy AM, Ibrahem RAL, Elhaded ASA, Assar MFA. Circulating Mir-21-5p and Mir-126-3p: diagnostic, prognostic value, and multivariate analysis in non-small-cell lung cancer. Mol Biol Rep. (2021) 48:2543–52. doi: 10.1007/s11033-021-06302-3
119. Emami SS, Nekouian R, Akbari A, Faraji A, Abbasi V, Agah S. Evaluation of circulating Mir-21 and Mir-222 as diagnostic biomarkers for gastric cancer. J Cancer Res Ther. (2019) 15:115–9. doi: 10.4103/jcrt.JCRT_592_17
120. Hannafon BN, Trigoso YD, Calloway CL, Zhao YD, Lum DH, Welm AL, et al. Plasma exosome microRNAs are indicative of breast cancer. Breast Cancer Res. (2016) 18:90. doi: 10.1186/s13058-016-0753-x
121. ParvizHamidi M, Haddad G, Ostadrahimi S, Ostadrahimi N, Sadeghi S, Fayaz S, et al. Circulating Mir-26a and Mir-21 as biomarkers for glioblastoma multiform. Biotechnol Appl Biochem. (2019) 66:261–5. doi: 10.1002/bab.1707
122. Tusong H, Maolakuerban N, Guan J, Rexiati M, Wang WG, Azhati B, et al. Functional analysis of serum micrornas Mir-21 and Mir-106a in renal cell carcinoma. Cancer Biomark. (2017) 18:79–85. doi: 10.3233/CBM-160676
123. Sierzega M, Kaczor M, Kolodziejczyk P, Kulig J, Sanak M, Richter P. Evaluation of serum microrna biomarkers for gastric cancer based on blood and tissue pools profiling: the importance of Mir-21 and Mir-331. Br J Cancer. (2017) 117:266–73. doi: 10.1038/bjc.2017.190
124. Garcia-Elias A, Tajes M, Yanez-Bisbe L, Enjuanes C, Comin-Colet J, Serra SA, et al. Atrial fibrillation in heart failure is associated with high levels of circulating microrna-199a-5p and 22-5p and a defective regulation of intracellular calcium and cell-to-cell communication. Int J Mol Sci. (2021) 22:10377. doi: 10.3390/ijms221910377
125. Vadla GP, Daghat B, Patterson N, Ahmad V, Perez G, Garcia A, et al. Combining plasma extracellular vesicle Let-7b-5p, Mir-184 and Circulating Mir-22-3p levels for Nsclc diagnosis and drug resistance prediction. Sci Rep. (2022) 12:6693. doi: 10.1038/s41598-022-10598-x
126. Diao ZB, Sun TX, Zong Y, Lin BC, Xia YS. Identification of plasma microrna-22 as a marker for the diagnosis, prognosis, and chemosensitivity prediction of osteosarcoma. J Int Med Res. (2020) 48:300060520967818. doi: 10.1177/0300060520967818
127. van Boven N, Kardys I, van Vark LC, Akkerhuis KM, de Ronde MWJ, Khan MAF, et al. Serially measured circulating micrornas and adverse clinical outcomes in patients with acute heart failure. Eur J Heart Fail. (2018) 20:89–96. doi: 10.1002/ejhf.950
128. Yu Z, Rong Z, Sheng J, Luo Z, Zhang J, Li T, et al. Aberrant non-coding Rna expressed in gastric cancer and its diagnostic value. Front Oncol. (2021) 11:606764. doi: 10.3389/fonc.2021.606764
129. Demolli S, Doebele C, Doddaballapur A, Lang V, Fisslthaler B, Chavakis E, et al. Microrna-30 mediates anti-inflammatory effects of shear stress and Klf2 via repression of angiopoietin 2. J Mol Cell Cardiol. (2015) 88:111–9. doi: 10.1016/j.yjmcc.2015.10.009
130. Duisters RF, Tijsen AJ, Schroen B, Leenders JJ, Lentink V, van der Made I, et al. Mir-133 and Mir-30 regulate connective tissue growth factor: implications for a role of micrornas in myocardial matrix remodeling. Circ Res. (2009) 104:170–8; 6p following 178. doi: 10.1161/CIRCRESAHA.108.182535
131. Veitch S, Njock MS, Chandy M, Siraj MA, Chi L, Mak H, et al. Mir-30 promotes fatty acid beta-oxidation and endothelial cell dysfunction and is a circulating biomarker of coronary microvascular dysfunction in pre-clinical models of diabetes. Cardiovasc Diabetol. (2022) 21:31. doi: 10.1186/s12933-022-01458-z
132. Xu R, Liu S, Chen H, Lao L. Microrna-30a downregulation contributes to chemoresistance of osteosarcoma cells through activating beclin-1-mediated autophagy. Oncol Rep. (2016) 35:1757–63. doi: 10.3892/or.2015.4497
133. Croset M, Pantano F, Kan CWS, Bonnelye E, Descotes F, Alix-Panabieres C, et al. Mirna-30 family members inhibit breast cancer invasion, osteomimicry, and bone destruction by directly targeting multiple bone metastasis-associated genes. Cancer Res. (2018) 78:5259–73. doi: 10.1158/0008-5472.CAN-17-3058
134. Ovchinnikova ES, Schmitter D, Vegter EL, Ter Maaten JM, Valente MA, Liu LC, et al. Signature of circulating micrornas in patients with acute heart failure. Eur J Heart Fail. (2016) 18:414–23. doi: 10.1002/ejhf.332
135. Li M, Zhou Y, Xia T, Zhou X, Huang Z, Zhang H, et al. Circulating micrornas from the Mir-106a-363 cluster on chromosome X as novel diagnostic biomarkers for breast cancer. Breast Cancer Res Treat. (2018) 170:257–70. doi: 10.1007/s10549-018-4757-3
136. Cheng Q, Feng F, Zhu L, Zheng Y, Luo X, Liu C, et al. Circulating Mir-106a is a novel prognostic and lymph node metastasis indicator for cholangiocarcinoma. Sci Rep. (2015) 5:16103. doi: 10.1038/srep16103
137. Cochetti G, Poli G, Guelfi G, Boni A, Egidi MG, Mearini E. Different levels of serum micrornas in prostate cancer and benign prostatic hyperplasia: evaluation of potential diagnostic and prognostic role. Onco Targets Ther. (2016) 9:7545–53. doi: 10.2147/OTT.S119027
138. Hanahan D, Weinberg RA. Hallmarks of cancer: the next generation. Cell. (2011) 144:646–74. doi: 10.1016/j.cell.2011.02.013
139. Shirazi-Tehrani E, Firouzabadi N, Tamaddon G, Bahramali E, Vafadar A. Carvedilol alters circulating Mir-1 and Mir-214 in heart failure. Pharmgenomics Pers Med. (2020) 13:375–83. doi: 10.2147/PGPM.S263740
140. Sygitowicz G, Tomaniak M, Blaszczyk O, Koltowski L, Filipiak KJ, Sitkiewicz D. Circulating microribonucleic acids Mir-1, Mir-21 and Mir-208a in patients with symptomatic heart failure: preliminary results. Arch Cardiovasc Dis. (2015) 108:634–42. doi: 10.1016/j.acvd.2015.07.003
141. Rigaud VO, Ferreira LR, Ayub-Ferreira SM, Avila MS, Brandao SM, Cruz FD, et al. Circulating Mir-1 as a potential biomarker of doxorubicin-induced cardiotoxicity in breast cancer patients. Oncotarget. (2017) 8:6994–7002. doi: 10.18632/oncotarget.14355
142. Costello EK, Stagaman K, Dethlefsen L, Bohannan BJ, Relman DA. The application of ecological theory toward an understanding of the human microbiome. Science. (2012) 336:1255–62. doi: 10.1126/science.1224203
143. Cho I, Blaser MJ. The human microbiome: at the interface of health and disease. Nat Rev Genet. (2012) 13:260–70. doi: 10.1038/nrg3182
144. Weir TL, Manter DK, Sheflin AM, Barnett BA, Heuberger AL, Ryan EP. Stool microbiome and metabolome differences between colorectal cancer patients and healthy adults. PLoS One. (2013) 8:e70803. doi: 10.1371/journal.pone.0070803
145. De Santis S, Cavalcanti E, Mastronardi M, Jirillo E, Chieppa M. Nutritional keys for intestinal barrier modulation. Front Immunol. (2015) 6:612. doi: 10.3389/fimmu.2015.00612
146. Vaishnava S, Behrendt CL, Ismail AS, Eckmann L, Hooper LV. Paneth cells directly sense gut commensals and maintain homeostasis at the intestinal host-microbial interface. Proc Natl Acad Sci USA. (2008) 105:20858–63. doi: 10.1073/pnas.0808723105
147. Peterson LW, Artis D. Intestinal epithelial cells: regulators of barrier function and immune homeostasis. Nat Rev Immunol. (2014) 14:141–53. doi: 10.1038/nri3608
148. Chung WS, Walker AW, Louis P, Parkhill J, Vermeiren J, Bosscher D, et al. Modulation of the human gut microbiota by dietary fibres occurs at the species level. BMC Biol. (2016) 14:3. doi: 10.1186/s12915-015-0224-3
149. Sommer F, Backhed F. The gut microbiota engages different signaling pathways to induce duox2 expression in the ileum and colon epithelium. Mucosal Immunol. (2015) 8:372–9. doi: 10.1038/mi.2014.74
150. Troseid M, Andersen GO, Broch K, Hov JR. The gut microbiome in coronary artery disease and heart failure: current knowledge and future directions. EBioMedicine. (2020) 52:102649. doi: 10.1016/j.ebiom.2020.102649
151. Luedde M, Winkler T, Heinsen FA, Ruhlemann MC, Spehlmann ME, Bajrovic A, et al. Heart failure is associated with depletion of core intestinal microbiota. ESC Heart Fail. (2017) 4:282–90. doi: 10.1002/ehf2.12155
152. Kamo T, Akazawa H, Suda W, Saga-Kamo A, Shimizu Y, Yagi H, et al. Dysbiosis and compositional alterations with aging in the gut microbiota of patients with heart failure. PLoS One. (2017) 12:e0174099. doi: 10.1371/journal.pone.0174099
153. Cui X, Ye L, Li J, Jin L, Wang W, Li S, et al. Metagenomic and metabolomic analyses unveil dysbiosis of gut microbiota in chronic heart failure patients. Sci Rep. (2018) 8:635. doi: 10.1038/s41598-017-18756-2
154. Wang Z, Klipfell E, Bennett BJ, Koeth R, Levison BS, Dugar B, et al. Gut flora metabolism of phosphatidylcholine promotes cardiovascular disease. Nature. (2011) 472:57–63. doi: 10.1038/nature09922
155. Zhang Y, Wang Y, Ke B, Du J. Tmao: how gut microbiota contributes to heart failure. Transl Res. (2021) 228:109–25. doi: 10.1016/j.trsl.2020.08.007
156. Tang WHW, Backhed F, Landmesser U, Hazen SL. Intestinal microbiota in cardiovascular health and disease: JACC state-of-the-art review. J Am Coll Cardiol. (2019) 73:2089–105. doi: 10.1016/j.jacc.2019.03.024
157. Zhu W, Buffa JA, Wang Z, Warrier M, Schugar R, Shih DM, et al. Flavin monooxygenase 3, the host hepatic enzyme in the metaorganismal trimethylamine N-oxide-generating pathway, modulates platelet responsiveness and thrombosis risk. J Thromb Haemost. (2018) 16:1857–72. doi: 10.1111/jth.14234
158. Yu LX, Schwabe RF. The gut microbiome and liver cancer: mechanisms and clinical translation. Nat Rev Gastroenterol Hepatol. (2017) 14:527–39. doi: 10.1038/nrgastro.2017.72
159. Wang T, Cai G, Qiu Y, Fei N, Zhang M, Pang X, et al. Structural segregation of gut microbiota between colorectal cancer patients and healthy volunteers. ISME J. (2012) 6:320–9. doi: 10.1038/ismej.2011.109
160. Ohnishi N, Yuasa H, Tanaka S, Sawa H, Miura M, Matsui A, et al. Transgenic expression of Helicobacter pylori Caga induces gastrointestinal and hematopoietic neoplasms in mouse. Proc Natl Acad Sci USA. (2008) 105:1003–8. doi: 10.1073/pnas.0711183105
161. Bik EM, Eckburg PB, Gill SR, Nelson KE, Purdom EA, Francois F, et al. Molecular analysis of the bacterial microbiota in the human stomach. Proc Natl Acad Sci USA. (2006) 103:732–7. doi: 10.1073/pnas.0506655103
162. Dicksved J, Lindberg M, Rosenquist M, Enroth H, Jansson JK, Engstrand L. Molecular characterization of the stomach microbiota in patients with gastric cancer and in controls. J Med Microbiol. (2009) 58:509–16. doi: 10.1099/jmm.0.007302-0
163. Loo TM, Kamachi F, Watanabe Y, Yoshimoto S, Kanda H, Arai Y, et al. Gut microbiota promotes obesity-associated liver cancer through Pge2-mediated suppression of antitumor immunity. Cancer Discov. (2017) 7:522–38. doi: 10.1158/2159-8290.CD-16-0932
164. Zitvogel L, Daillere R, Roberti MP, Routy B, Kroemer G. Anticancer effects of the microbiome and its products. Nat Rev Microbiol. (2017) 15:465–78. doi: 10.1038/nrmicro.2017.44
165. Sears CL, Garrett WS. Microbes, microbiota, and colon cancer. Cell Host Microbe. (2014) 15:317–28. doi: 10.1016/j.chom.2014.02.007
166. Brennan CA, Garrett WS. Gut microbiota, inflammation, and colorectal cancer. Annu Rev Microbiol. (2016) 70:395–411. doi: 10.1146/annurev-micro-102215-095513
167. Hold GL. Gastrointestinal microbiota and colon cancer. Dig Dis. (2016) 34:244–50. doi: 10.1159/000443358
168. Totzeck M, Mincu RI, Heusch G, Rassaf T. Heart failure from cancer therapy: can we prevent it? ESC Heart Fail. (2019) 6:856–62. doi: 10.1002/ehf2.12493
169. Banke A, Fosbol EL, Moller JE, Gislason GH, Andersen M, Bernsdorf M, et al. Long-term effect of epirubicin on incidence of heart failure in women with breast cancer: insight from a randomized clinical trial. Eur J Heart Fail. (2018) 20:1447–53. doi: 10.1002/ejhf.1168
170. Anker MS, Sanz AP, Zamorano JL, Mehra MR, Butler J, Riess H, et al. Advanced cancer is also a heart failure syndrome: a hypothesis. J Cachexia Sarcopenia Muscle. (2021) 12:533–7. doi: 10.1002/jcsm.12694
171. Zhang H, Gao Y, Wang L, Tian L, An N, Yang X, et al. Does Heart failure increase the risk of incident cancer? A meta-analysis and systematic review. Heart Fail Rev. (2020) 25:949–55. doi: 10.1007/s10741-019-09876-0
172. Madonna R, Moscato S, Polizzi E, Pieragostino D, Cufaro MC, Del Boccio P, et al. Connexin 43 and connexin 26 involvement in the ponatinib-induced cardiomyopathy: sex-related differences in a murine model. Int J Mol Sci. (2021) 22:5815. doi: 10.3390/ijms22115815
173. Madonna R, Pieragostino D, Cufaro MC, Del Boccio P, Pucci A, Mattii L, et al. Sex-related differential susceptibility to ponatinib cardiotoxicity and differential modulation of the notch1 signalling pathway in a murine model. J Cell Mol Med. (2022) 26:1380–91. doi: 10.1111/jcmm.17008
174. Wilcox NS, Rotz SJ, Mullen M, Song EJ, Ky Hamilton B, Moslehi J, et al. Sex-Specific cardiovascular risks of cancer and its therapies. Circ Res. (2022) 130:632–51. doi: 10.1161/CIRCRESAHA.121.319901
175. Suter TM, Ewer MS. Cancer drugs and the heart: importance and management. Eur Heart J. (2013) 34:1102–11. doi: 10.1093/eurheartj/ehs181
176. Sabatino J, De Rosa S, Tamme L, Iaconetti C, Sorrentino S, Polimeni A, et al. Empagliflozin prevents doxorubicin-induced myocardial dysfunction. Cardiovasc Diabetol. (2020) 19:66. doi: 10.1186/s12933-020-01040-5
177. Baris VO, Dincsoy AB, Gedikli E, Zirh S, Muftuoglu S, Erdem A. Empagliflozin significantly prevents the doxorubicin-induced acute cardiotoxicity via non-antioxidant pathways. Cardiovasc Toxicol. (2021) 21:747–58. doi: 10.1007/s12012-021-09665-y
178. Quagliariello V, De Laurentiis M, Rea D, Barbieri A, Monti MG, Carbone A, et al. The Sglt-2 inhibitor empagliflozin improves myocardial strain, reduces cardiac fibrosis and pro-inflammatory cytokines in non-diabetic mice treated with doxorubicin. Cardiovasc Diabetol. (2021) 20:150. doi: 10.1186/s12933-021-01346-y
179. Fornari FA, Randolph JK, Yalowich JC, Ritke MK, Gewirtz DA. Interference by doxorubicin with DNA unwinding in Mcf-7 breast tumor cells. Mol Pharmacol. (1994) 45:649–56.
180. Momparler RL, Karon M, Siegel SE, Avila F. Effect of adriamycin on DNA, Rna, and protein synthesis in cell-free systems and intact cells. Cancer Res. (1976) 36:2891–5.
181. Rawat PS, Jaiswal A, Khurana A, Bhatti JS, Navik U. Doxorubicin-induced cardiotoxicity: an update on the molecular mechanism and novel therapeutic strategies for effective management. Biomed Pharmacother. (2021) 139:111708. doi: 10.1016/j.biopha.2021.111708
182. Renu K, V G A, P B TP, Arunachalam S. Molecular mechanism of doxorubicin-induced cardiomyopathy – an update. Eur J Pharmacol. (2018) 818:241–53. doi: 10.1016/j.ejphar.2017.10.043
183. Nebigil CG, Desaubry L. Updates in anthracycline-mediated cardiotoxicity. Front Pharmacol. (2018) 9:1262. doi: 10.3389/fphar.2018.01262
184. Choksey A, Timm KN. Cancer therapy-induced cardiotoxicity-a metabolic perspective on pathogenesis, diagnosis and therapy. Int J Mol Sci. (2021) 23:441. doi: 10.3390/ijms23010441
185. Songbo M, Lang H, Xinyong C, Bin X, Ping Z, Liang S. Oxidative stress injury in doxorubicin-induced cardiotoxicity. Toxicol Lett. (2019) 307:41–8. doi: 10.1016/j.toxlet.2019.02.013
186. Abdel-aleem S, el-Merzabani MM, Sayed-Ahmed M, Taylor DA, Lowe JE. Acute and chronic effects of adriamycin on fatty acid oxidation in isolated cardiac myocytes. J Mol Cell Cardiol. (1997) 29:789–97. doi: 10.1006/jmcc.1996.0323
187. Yang Y, Zhang H, Li X, Yang T, Jiang Q. Effects of Pparalpha/Pgc-1alpha on the myocardial energy metabolism during heart failure in the doxorubicin induced dilated cardiomyopathy in mice. Int J Clin Exp Med. (2014) 7:2435–42.
188. Fang X, Wang H, Han D, Xie E, Yang X, Wei J, et al. Ferroptosis as a target for protection against cardiomyopathy. Proc Natl Acad Sci USA. (2019) 116:2672–80. doi: 10.1073/pnas.1821022116
189. Shrivastav N, Li D, Essigmann JM. Chemical biology of mutagenesis and DNA repair: cellular responses to DNA alkylation. Carcinogenesis. (2010) 31:59–70. doi: 10.1093/carcin/bgp262
190. Drablos F, Feyzi E, Aas PA, Vaagbo CB, Kavli B, Bratlie MS, et al. Alkylation damage in DNA and Rna–repair mechanisms and medical significance. DNA Repair (Amst). (2004) 3:1389–407. doi: 10.1016/j.dnarep.2004.05.004
191. Iqubal A, Iqubal MK, Sharma S, Ansari MA, Najmi AK, Ali SM, et al. Molecular mechanism involved in cyclophosphamide-induced cardiotoxicity: old drug with a new vision. Life Sci. (2019) 218:112–31. doi: 10.1016/j.lfs.2018.12.018
192. Wei SC, Duffy CR, Allison JP. Fundamental mechanisms of immune checkpoint blockade therapy. Cancer Discov. (2018) 8:1069–86. doi: 10.1158/2159-8290.CD-18-0367
193. Seidel JA, Otsuka A, Kabashima K. Anti-Pd-1 and Anti-Ctla-4 Therapies in cancer: mechanisms of action, efficacy, and limitations. Front Oncol. (2018) 8:86. doi: 10.3389/fonc.2018.00086
194. Mocan-Hognogi DL, Tranca S, Farcas AD, Mocan-Hognogi RF, Parvu AV, Bojan AS. Immune checkpoint inhibitors and the heart. Front Cardiovasc Med. (2021) 8:726426. doi: 10.3389/fcvm.2021.726426
195. Madonna R. Multi-target drugs for blood cancer in the elderly: implications of damage and repair in the cardiovascular toxicity. Front Physiol. (2021) 12:792751. doi: 10.3389/fphys.2021.792751
196. Scalzulli E, Grammatico S, Vozella F, Petrucci MT. Proteasome inhibitors for the treatment of multiple myeloma. Expert Opin Pharmacother. (2018) 19:375–86. doi: 10.1080/14656566.2018.1441287
197. Wu P, Oren O, Gertz MA, Yang EH. Proteasome inhibitor-related cardiotoxicity: mechanisms, diagnosis, and management. Curr Oncol Rep. (2020) 22:66. doi: 10.1007/s11912-020-00931-w
198. Thomas A, Teicher BA, Hassan R. Antibody-drug conjugates for cancer therapy. Lancet Oncol. (2016) 17:e254–62. doi: 10.1016/S1470-2045(16)30030-4
199. Ponde NF, Lambertini M, de Azambuja E. Twenty years of Anti-Her2 therapy-associated cardiotoxicity. ESMO Open. (2016) 1:e000073. doi: 10.1136/esmoopen-2016-000073
200. Slamon D, Eiermann W, Robert N, Pienkowski T, Martin M, Press M, et al. Adjuvant trastuzumab in Her2-positive breast cancer. N Engl J Med. (2011) 365:1273–83. doi: 10.1056/NEJMoa0910383
201. Kontermann RE, Brinkmann U. Bispecific antibodies. Drug Discov Today. (2015) 20:838–47. doi: 10.1016/j.drudis.2015.02.008
202. Le X, Nilsson M, Goldman J, Reck M, Nakagawa K, Kato T, et al. Dual Egfr-Vegf pathway inhibition: a promising strategy for patients with Egfr-Mutant Nsclc. J Thorac Oncol. (2021) 16:205–15. doi: 10.1016/j.jtho.2020.10.006
203. Huang J, Hu W, Hu L, Previs RA, Dalton HJ, Yang XY, et al. Dll4 inhibition plus Aflibercept markedly reduces ovarian tumor growth. Mol Cancer Ther. (2016) 15:1344–52. doi: 10.1158/1535-7163.MCT-15-0144
204. Staflin K, Zuch de Zafra CL, Schutt LK, Clark V, Zhong F, Hristopoulos M, et al. Target Arm affinities determine preclinical efficacy and safety of Anti-Her2/Cd3 bispecific antibody. JCI Insight. (2020) 5:e133757. doi: 10.1172/jci.insight.133757
205. Yuan Q, Liang Q, Sun Z, Yuan X, Hou W, Wang Y, et al. Development of bispecific Anti-C-Met/Pd-1 diabodies for the treatment of solid tumors and the effect of C-Met binding affinity on efficacy. Oncoimmunology. (2021) 10:1914954. doi: 10.1080/2162402X.2021.1914954
206. Wei X, Juan ZX, Min FX, Nan C, Hua ZX, Qing FZ, et al. Recombinant immunotoxin Anti-C-Met/Pe38kdel inhibits proliferation and promotes apoptosis of gastric cancer cells. J Exp Clin Cancer Res. (2011) 30:67. doi: 10.1186/1756-9966-30-67
207. Jeong S, Park E, Kim HD, Sung E, Kim H, Jeon J, et al. Novel Anti-4-1bbxpd-L1 Bispecific antibody augments anti-tumor immunity through tumor-directed T-cell activation and checkpoint blockade. J Immunother Cancer. (2021) 9:e002428. doi: 10.1136/jitc-2021-002428
208. Wei SC, Levine JH, Cogdill AP, Zhao Y, Anang NAS, Andrews MC, et al. Distinct cellular mechanisms underlie Anti-Ctla-4 and Anti-Pd-1 checkpoint blockade. Cell. (2017) 170:1120–33.e17. doi: 10.1016/j.cell.2017.07.024
209. Perrot I, Michaud HA, Giraudon-Paoli M, Augier S, Docquier A, Gros L, et al. Blocking antibodies targeting the Cd39/Cd73 immunosuppressive pathway unleash immune responses in combination cancer therapies. Cell Rep. (2019) 27:2411–25.e9. doi: 10.1016/j.celrep.2019.04.091
210. Darvishi B, Farahmand L, Jalili N, Majidzadeh AK. Blinatumomab provoked fatal heart failure. Int Immunopharmacol. (2016) 41:42–6. doi: 10.1016/j.intimp.2016.10.017
211. Kroschinsky F, Stolzel F, von Bonin S, Beutel G, Kochanek M, Kiehl M, et al. New drugs, new toxicities: severe side effects of modern targeted and immunotherapy of cancer and their management. Crit Care. (2017) 21:89. doi: 10.1186/s13054-017-1678-1
212. Sipahi I, Debanne SM, Rowland DY, Simon DI, Fang JC. Angiotensin-receptor blockade and risk of cancer: meta-analysis of randomised controlled trials. Lancet Oncol. (2010) 11:627–36. doi: 10.1016/S1470-2045(10)70106-6
213. Bangalore S, Kumar S, Kjeldsen SE, Makani H, Grossman E, Wetterslev J, et al. Antihypertensive drugs and risk of cancer: network meta-analyses and trial sequential analyses of 324,168 participants from randomised trials. Lancet Oncol. (2011) 12:65–82. doi: 10.1016/S1470-2045(10)70260-6
214. de Boer RA, Meijers WC, van der Meer P, van Veldhuisen DJ. Cancer and heart disease: associations and relations. Eur J Heart Fail. (2019) 21:1515–25. doi: 10.1002/ejhf.1539
215. Gaziano JM, Brotons C, Coppolecchia R, Cricelli C, Darius H, Gorelick PB, et al. Use of Aspirin to reduce risk of initial vascular events in patients at moderate risk of cardiovascular disease (Arrive): a randomised, double-blind, placebo-controlled trial. Lancet. (2018) 392:1036–46. doi: 10.1016/S0140-6736(18)31924-X
216. Thorat MA, Cuzick J. Role of aspirin in cancer prevention. Curr Oncol Rep. (2013) 15:533–40. doi: 10.1007/s11912-013-0351-3
217. Brenner H, Tao S, Haug U. Low-dose aspirin use and performance of immunochemical Fecal occult blood tests. JAMA. (2010) 304:2513–20. doi: 10.1001/jama.2010.1773
218. Li J, Lam ASM, Yau STY, Yiu KKL, Tsoi KKF. Antihypertensive treatments and risks of lung cancer: a large population-based cohort study in Hong Kong. BMC Cancer. (2021) 21:1202. doi: 10.1186/s12885-021-08971-6
219. Lee SH, Chun KJ, Park J, Kim J, Sung JD, Park RW, et al. Angiotensin Converting enzyme inhibitors and incidence of lung cancer in a population based cohort of common data model in Korea. Sci Rep. (2021) 11:18576. doi: 10.1038/s41598-021-97989-8
220. Engineer DR, Burney BO, Hayes TG, Garcia JM. Exposure to Acei/Arb and Beta-blockers is associated with improved survival and decreased tumor progression and hospitalizations in patients with advanced colon cancer. Transl Oncol. (2013) 6:539–45. doi: 10.1593/tlo.13346
221. Lin SY, Lin CL, Lin CC, Hsu WH, Lin CD, Wang IK, et al. Association between angiotensin-converting enzyme inhibitors and lung cancer-a nationwide, population-based, propensity score-matched cohort study. Cancers (Basel). (2020) 12:747. doi: 10.3390/cancers12030747
222. Htoo PT, Sturmer T, Jonsson-Funk M, Pate V, Simpson RJ Jr., Lund JL. Renin-angiotensin-aldosterone system-based antihypertensive agents and the risk of colorectal cancer among medicare beneficiaries. Epidemiology. (2019) 30:867–75. doi: 10.1097/EDE.0000000000001065
223. Scott OW, Tin Tin S, Elwood JM, Cavadino A, Habel LA, Kuper-Hommel M, et al. Post-diagnostic beta blocker use and breast cancer-specific mortality: a population-based cohort study. Breast Cancer Res Treat. (2022) 193:225–35. doi: 10.1007/s10549-022-06528-0
224. Jansen L, Below J, Chang-Claude J, Brenner H, Hoffmeister M. Beta blocker use and colorectal cancer risk: population-based case-control study. Cancer. (2012) 118:3911–9. doi: 10.1002/cncr.26727
Keywords: heart failure, cancer, active exposure, passive adaption, Cardio-Oncology
Citation: Du Y and Wu T (2022) Heart failure and cancer: From active exposure to passive adaption. Front. Cardiovasc. Med. 9:992011. doi: 10.3389/fcvm.2022.992011
Received: 12 July 2022; Accepted: 20 September 2022;
Published: 11 October 2022.
Edited by:
Jun-ichi Abe, University of Texas MD Anderson Cancer Center, United StatesReviewed by:
Claudio Molinari, University of Eastern Piedmont, ItalyFrancesca Uberti, Università del Piemonte Orientale, Italy
Rosalinda Madonna, University of Pisa, Italy
Copyright © 2022 Du and Wu. This is an open-access article distributed under the terms of the Creative Commons Attribution License (CC BY). The use, distribution or reproduction in other forums is permitted, provided the original author(s) and the copyright owner(s) are credited and that the original publication in this journal is cited, in accordance with accepted academic practice. No use, distribution or reproduction is permitted which does not comply with these terms.
*Correspondence: Tao Wu, wutao@nbu.edu.cn