- 1Department of Pulmonary and Critical Care Medicine, Center of Respiratory Medicine, China-Japan Friendship Hospital, Beijing, China
- 2National Center for Respiratory Medicine, Beijing, China
- 3Institute of Respiratory Medicine, Chinese Academy of Medical Sciences, Beijing, China
- 4National Clinical Research Center for Respiratory Diseases, Beijing, China
- 5Graduate School of Peking Union Medical College, Chinese Academy of Medical Sciences, and Peking Union Medical College, Beijing, China
- 6Department of Pulmonary and Critical Care Medicine, Quanzhou First Hospital Affiliated to Fujian Medical University, Quanzhou, China
- 7State Key Laboratory of Medical Molecular Biology, Department of Physiology, Institute of Basic Medical Sciences, Chinese Academy of Medical Sciences, Peking Union Medical College, Beijing, China
Background: Oxygen pathway limitation exists in chronic thromboembolic pulmonary hypertension (CTEPH). Pulmonary endarterectomy (PEA) and balloon pulmonary angioplasty (BPA) are two effective interventions for CTEPH, but their effects and comparison of these two interventions on the oxygen pathway are not well demonstrated.
Methods: CTEPH patients with available pulmonary function test, hemodynamics, and blood gas analysis before and after the interventions were included for comparison of oxygen pathway in terms of lung ventilation, lung gas exchange, oxygen delivery, and oxygen extraction between these two interventions.
Results: The change in the percentage of the predicted forced expiratory volume in the 1 s (−3.4 ± 12.7 vs. 3.8 ± 8.7%, P = 0.006) and forced vital capacity (−5.5 ± 13.0 vs. 4.2 ± 9.9%, P = 0.001) among the PEA group (n = 24) and BPA group (n = 46) were significantly different. Patients in the PEA group had a significant increase in their arterial oxygen saturation (from 92.5 ± 3.6 to 94.6 ± 2.4%, P = 0.022), while those in the BPA group had no change, which could be explained by a significant improvement in ventilation/perfusion (−0.48 ± 0.53 vs. −0.17 ± 0.41, P = 0.016). Compared with patients post-BPA, patients post-PEA were characterized by higher oxygen delivery (756.3 ± 229.1 vs. 628.8 ± 188.5 ml/min, P = 0.016) and higher oxygen extraction (203.3 ± 64.8 vs. 151.2 ± 31.9 ml/min, P = 0.001).
Conclusion: Partial amelioration of the oxygen pathway limitations could be achieved in CTEPH patients treated with PEA and BPA. CTEPH patients post-PEA had better performance in lung gas exchange, oxygen delivery, and extraction, while those post-BPA had better lung ventilation. Cardiopulmonary rehabilitation may assist in improving the impairment of the oxygen pathway.
Introduction
Chronic thromboembolic pulmonary hypertension (CTEPH) is considered a severe complication of acute pulmonary embolism with an occurrence of ∼3% (1). Pulmonary endarterectomy (PEA) and balloon pulmonary angioplasty (BPA) are the most effective for CTEPH and have been suggested in the current treatment guidelines (2, 3). Generally, PEA is the recommended treatment for CTEPH patients with operable vascular lesions, while BPA is an alternative option for those not suitable for PEA. The estimated 3-year survival is nearly 90% in CTEPH patients after PEA, compared with 70% of survival in patients without operation (4). Similarly, in a recent meta-analysis study, CTEPH patients were found to have a 97% 3-year survival rate after BPA (5). These encouraging results suggest that PEA and BPA are clearly effective as remedies for the vascular lesions and their fatal consequences in CTEPH.
The oxygen pathway includes multiple complex steps including uptake in the lungs, transportation from alveoli to blood, carrying capacity of the blood, delivery from center to the periphery, extraction of peripheral tissue, and cellular use (6). Impairments in the oxygen pathway can occur in various diseases including heart failure, leukemia, tumors, connective tissue diseases, and respiratory diseases, leading to hypoxia and death (7–12). In patients with CTEPH, occlusion of the pulmonary artery can reduce lung perfusion, causing mismatched ventilation/perfusion and an abnormality in lung function, particularly a decreased lung diffusion capacity (13, 14). Moreover, a recent seminal study found that in addition to the pulmonary gas exchange, multiple steps of the oxygen pathway were defective in patients with CTEPH, including oxygen extraction by peripheral tissues, and pulmonary vascular interventions could partly correct the impaired oxygen pathway (15), in which only 10 patients had available data on the oxygen pathway after intervention. Despite the improved outcomes of CTEPH patients treated with PEA and BPA, the effects of these two interventions on the oxygen pathway are not well demonstrated and have not yet been compared. To better understand the pathophysiological mechanism and to compare the effects of PEA and BPA on the oxygen pathway, we retrospectively collected data, including pulmonary function test, hemodynamics, and blood gas analysis, from CTEPH patients before and after the interventions (PEA or BPA), and different responses of the oxygen pathway in patients treated with PEA versus BPA were revealed.
Materials and methods
Patients’ characteristics
Patients with CTEPH who underwent PEA or BPA from May 2018 to August 2021 in our center, were included in this study. Patients in the BPA group should receive all necessary rounds of BPA. Patients who received both PEA and BPA, or those without pulmonary function test and hemodynamics before and after intervention were not included in this study. Data on the pulmonary function test (MasterScreen SES, Vyaire Medical GmbH, China), right heart catheterization (hemodynamics and blood gas analysis), echocardiogram (Vivid E95, GE Healthcare, USA) before and after PEA or the last session of BPA, were retrospectively collected (Figure 1). A multidisciplinary team comprising pulmonary physicians, BPA interventionalists, cardiac surgeons, ICU doctors, and radiologists made the choice of treatments for patients with CTEPH. A refined multi-session BPA was conducted for non-surgical patients according to the previous studies (16). The target of BPA was to achieve an ultimate mean pulmonary artery pressure (mPAP) of less than 25 mmHg and a decrease of 5–10 mmHg in mPAP in each session in our center. Generally, the follow-up pulmonary function test, right heart catheterization, and echocardiogram were carried out simultaneously 6–12 months after the interventions. This study conformed to the principles outlined in the Declaration of Helsinki and was approved by the institutional board and the ethics committee of the China-Japan Friendship Hospital (2021-136-K94).
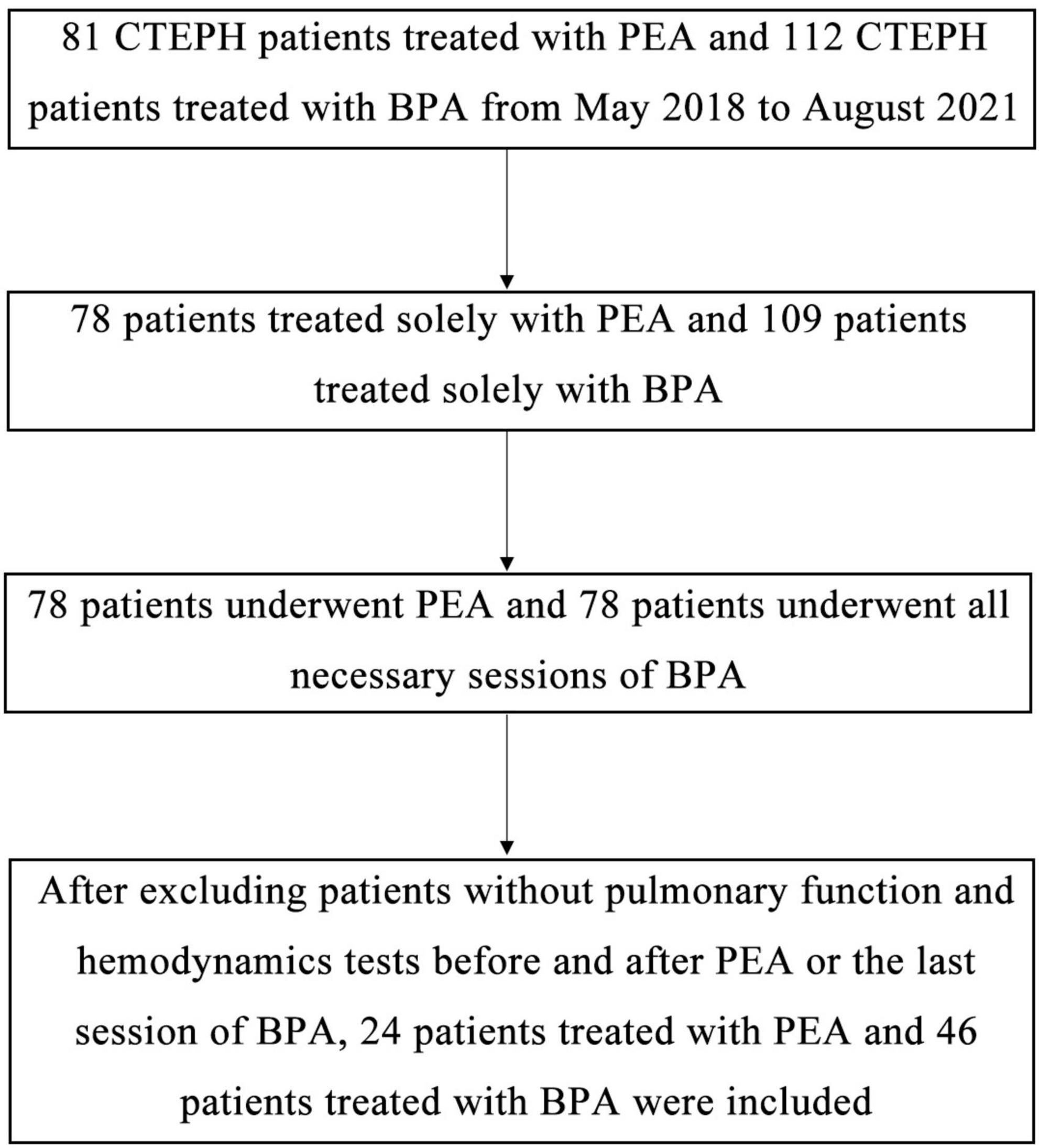
Figure 1. Schematic diagram of the screening process to include or exclude cases. After searching the electronic medical record using ICD-9-CM3, we found 89 patients receiving PEA and 166 patients receiving BPA from May 2018 to August 2021 in our center. After excluding patients without CTEPH, patients receiving both PEA and BPA, patients who have not received all sessions of BPA, and patients without available pulmonary function test before and after intervention, we finally enrolled 24 patients treated with PEA and 46 patients treated with BPA.
Oxygen pathway analysis
In this study, the oxygen pathway was comprised of lung ventilation, lung gas exchange, oxygen delivery, and oxygen extraction. We used the percentage of the predicted forced expiratory volume in the 1 s (FEV1%), forced vital capacity (FVC%), maximum mid-expiratory flow (MMEF%), maximum voluntary ventilation (MVV%), and alveolar ventilation (VA%) to represent lung ventilation. The pulmonary function was tested using MasterScreen (SES, Vyaire Medical GmbH, China) following the American Thoracic Society (ATS) and European Respiratory Society standards (17). Lung gas exchange was assessed as lung diffusion capacity, alveolar partial pressure of oxygen (PalvO2), arterial partial pressure of oxygen (PaO2), arterial saturation of oxygen (SaO2), and alveolar ventilation-to-cardiac output ratio (VA/CO). Lung diffusion capacity was represented by the diffusion capacity of the lungs for carbon monoxide (DLCO) and oxygen (DLO2) (≈1.23 × DLCO) (18). PalvO2 was calculated using the following equation: PalvO2 (mmHg) = 150–1.25 × PaCO2 (mmHg), where PaCO2 represents arterial partial pressure of carbon dioxide (19). Oxygen delivery (DO2) is the total arterial volume of oxygen delivered to peripheral tissues per minute, which was derived from cardiac output (CO, being measured using indirect Fick’s method) and arterial oxygen content (CaO2) using the following equation: DO2 (ml/min) = CO (L/min) × CaO2 (ml/dl) × 10 (19). The oxygen content of blood was calculated using the following equation: O2 content (ml/dl) = 1.36 × hemoglobin (g/dl) × SO2 + 0.003 × PO2 (mmHg), where SO2 represents saturation of oxygen and PO2 represents partial pressure of oxygen (19). Total oxygen extraction (EO2) by the peripheral tissues was defined as the volume gradient of oxygen between arterial blood and mixed venous blood.
Statistical analysis
Data were analyzed using IBM SPSS STATISTICS 19. Continuous variables were presented as mean ± standard deviation or mean (range), and categorical variables were presented as numbers and percentages. Comparisons of BPA and PEA were using unpaired two-sample t-test for continuous variables and χ2 or Fisher’s test for categorical variables, where a correction for logic regression analysis was underwent for the analysis of change in the oxygen pathway indices and four parameters [including the interventional method (PEA or BPA), age (0–49, 50–60, and > 60 year), gender (male or female), targeted medications for pulmonary hypertension (yes or no)] were taken into consideration. Comparisons before and after operation were made using paired t-test. Pearson correlation coefficient was used to evaluate the correlation between two particular parameters. P values < 0.05 were considered statistically significant.
Results
Baseline characteristics and oxygen pathway
We enrolled 24 CTEPH patients who received PEA and 46 CTEPH patients who received all sessions of BPA (Figure 1). There were no significant differences between patients who underwent PEA versus BPA in most baseline parameters except in age (Table 1). Patients in the BPA group were significantly older than those in the PEA group (60.3 ± 9.0 vs. 54.0 ± 12.1 year, P = 0.024).
Impairments were found at each step of the oxygen pathway in patients with CTEPH (Table 2), with no significant differences between the PEA and BPA groups. The MVV% and MMEF% of the CTEPH patients were below normal levels. Lung ventilation-to-lung perfusion was mismatched. A decreased DLO2 was speculated to exist in patients with CTEPH, based on less than 80% of DLCO% (Table 1). Blood gas analysis before PEA and BPA showed comparably decreased PaO2 (66.8 ± 14.1 vs. 61.3 ± 7.9 mmHg, P = 0.088) and SaO2 (92.5 ± 3.6 vs. 91.8 ± 3.0%, P = 0.443). Similar results were found in oxygen saturation in the pulmonary artery (SmvO2) (65.3 ± 8.3 vs. 65.5 ± 8.2%, P = 0.901). Comparably decreased CaO2 (18.8 ± 2.3 vs. 17.6 ± 2.5 ml/dl, P = 0.053) was found in both groups, where the normal value is 20 ml/dl (20). Before the intervention, patients in the PEA group had similar oxygen extraction in peripheral tissue as those in the BPA group (177.5 ± 40.4 vs. 156.3 ± 45.3 ml/min, P = 0.061).
Follow-up
As is shown in Table 3, there were no significant differences in the time since the last intervention among the two groups [330 days (range: 108–1064) in the PEA group and 318 days (range: 41–967) in the BPA group, P = 0.836]. Patients in the BPA group attempted an average of 4.0 ± 1.5 sessions of BPA (range: 2–8). Fourteen (30.4%) patients in the BPA group and 2 (8.3%) patients in the PEA group received targeted medications for pulmonary hypertension after the procedure, which was significantly different between the groups (P = 0.037).
After the intervention, patients with CTEPH had a significant improvement in cardiac function, illustrated by the improvements in 6-min working distance (6MWD), N-terminal pro-B-type natriuretic peptide (NT-proBNP), world health organization functional class (WHO-FC), and smaller right ventricle (Supplementary Figures 1A–C, 2A–H and Supplementary Table 1). Patients in the PEA group had significantly more improvement in their WHO-FC than those in the BPA group (−1.6 ± 0.9 vs. −0.9 ± 0.7, P = 0.001; Table 3). Significantly more reduction in the estimated pulmonary artery systolic pressure (EPASP) (−36.1 ± 26.3 vs. −18.9 ± 22.2 mmHg, P = 0.047) were found in PEA group than BPA group. Interestingly, compared with an increase of tricuspid annular plane systolic excursion (TAPSE) (from 17.4 ± 2.8 to 18.9 ± 2.8 mm, P = 0.003) and tricuspid systolic velocity (S’) (from 10.6 ± 2.1 to 11.4 ± 2.5 cm/s, P = 0.046) in the BPA group, those in the PEA group decreased (from 16.3 ± 3.3 to 12.8 ± 2.8 mm, P = 0.002 for TAPSE; from 10.0 ± 2.7 to 8.0 ± 1.6 cm/s for S’, P = 0.034, respectively), which may be associated with the change in the overall motion of the heart post-PEA and other surgery related injury (21, 22).
Change in the oxygen pathway
Patients with CTEPH treated with BPA have improved lung ventilation, including FEV1%, FVC%, and MVV%, while those treated with PEA have a decreasing trend (Figures 2A–E and Supplementary Table 1). The change in FEV1% (−3.4 ± 12.7 vs. 3.8 ± 8.7%, P = 0.006), FVC% (−5.5 ± 13.0 vs. 4.2 ± 9.9%, P = 0.001), and MVV% (−2.3 ± 9.7% vs. 2.5 ± 6.5%, P = 0.023) were significantly different between the PEA and BPA groups (Table 4 and Figure 3). Both MMEF% and VA% in patients treated with PEA or BPA showed no significant change.
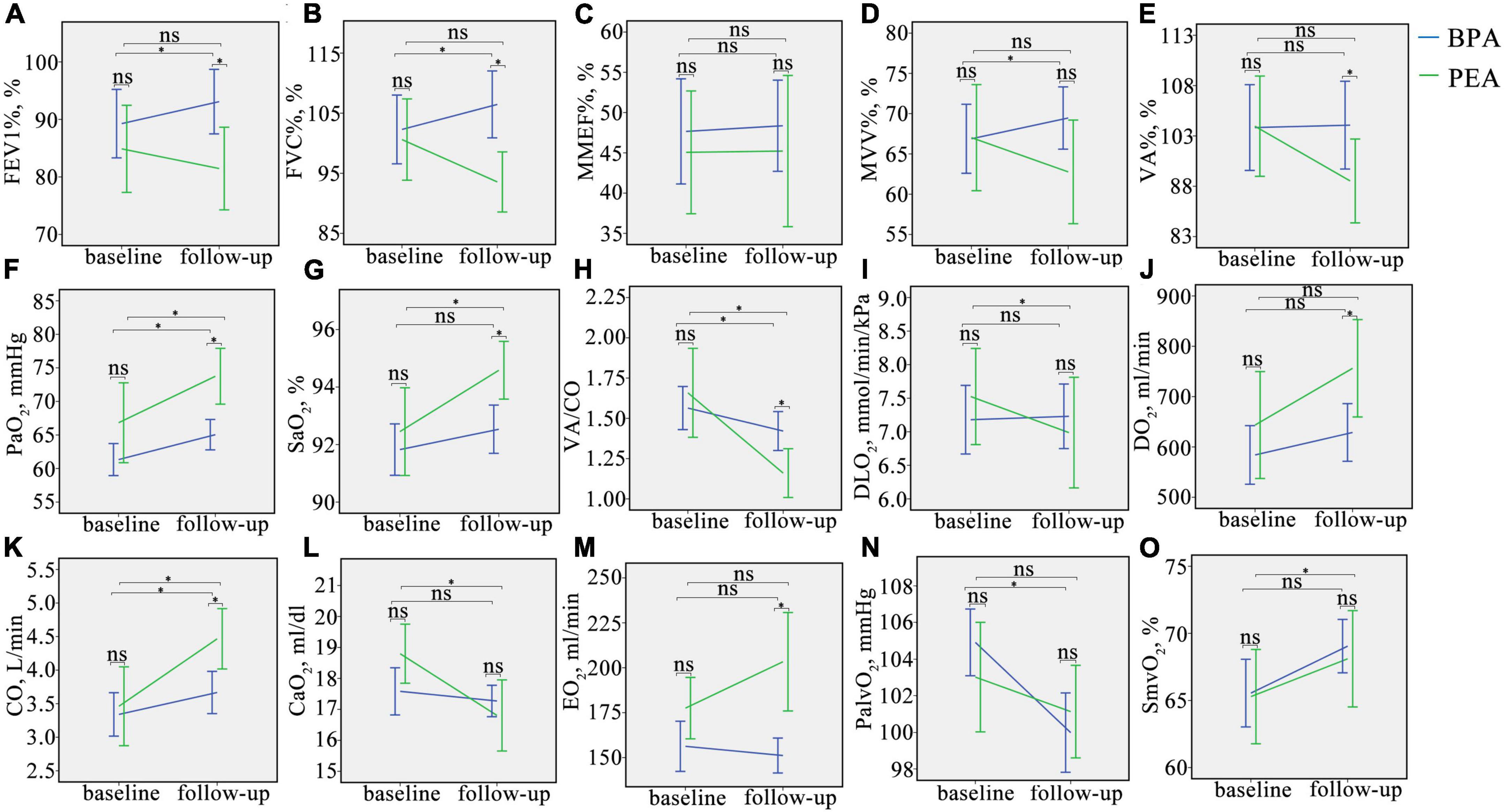
Figure 2. Changes in oxygen pathway parameters in CTEPH patients before and after intervention. (A–O), Change in FEV1%, FVC%, MMEF%, MVV%, VA%, PaO2, SaO2, VA/CO, DLO2, DO2, CO, CaO2, EO2, PalvO2, and SmvO2 in CTEPH patients before and after the intervention. ns, no significant difference, *P < 0.05. FEV1%, the percentage of predicted forced expiratory volume in the 1 s; FVC%, the percentage of predicted forced vital capacity; MMEF%, the percentage of predicted maximum mid-expiratory flow; MVV%, the percentage of predicted maximum voluntary ventilation; VA%, the percentage of predicted alveolar ventilation; PaO2, partial pressure of oxygen in the radial artery; SaO2, saturation of oxygen in the radial artery; VA/CO, alveolar ventilation-to-cardiac output ratio; DLO2, diffusion capacity of the lungs for oxygen; DO2, oxygen delivery; CO, cardiac output; CaO2, arterial oxygen content; PalvO2, alveolar partial pressure of oxygen; EO2, oxygen extraction; SmvO2, mixed venous oxygen saturation.
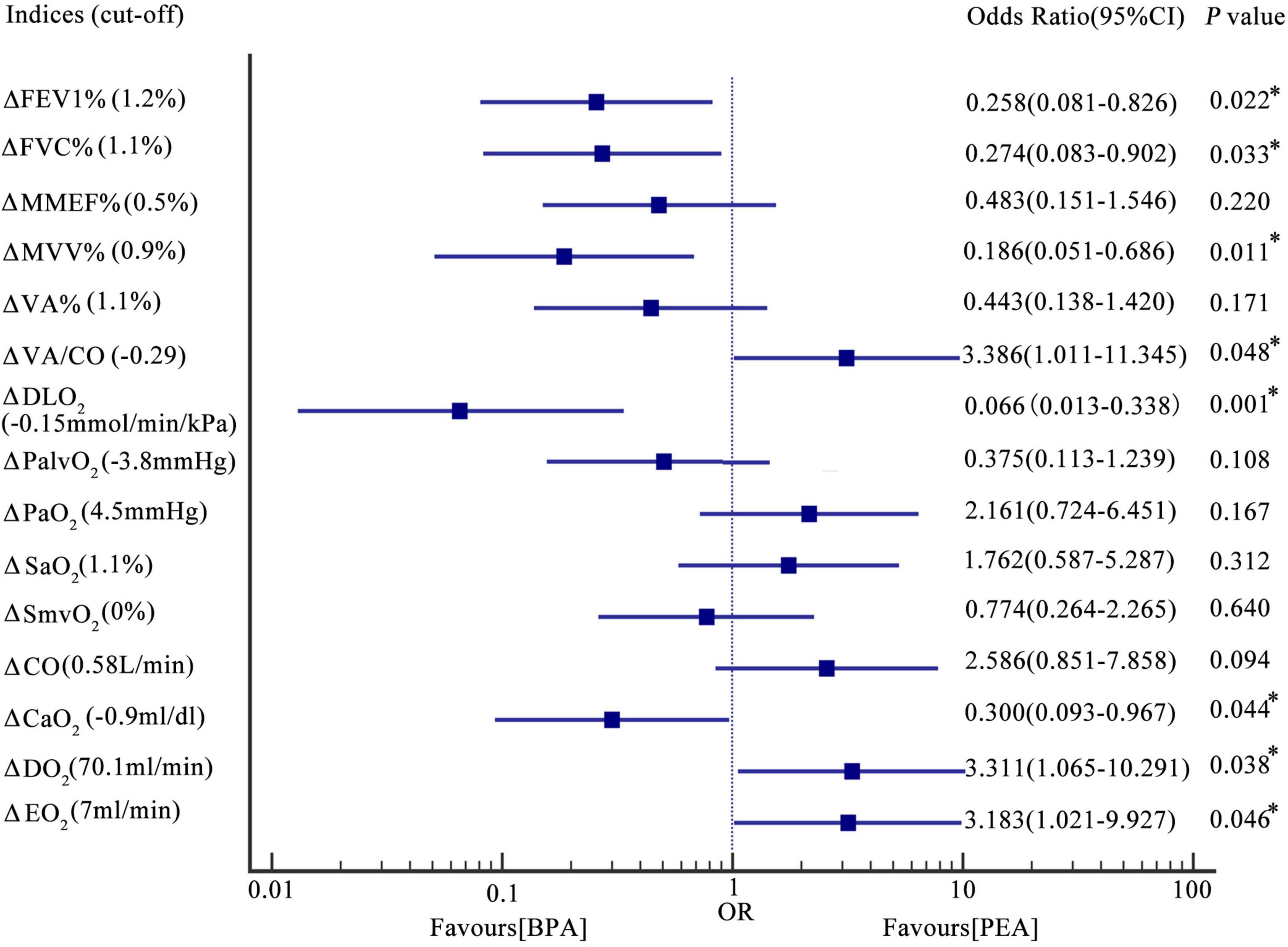
Figure 3. Forest plot-the association between the change in oxygen pathway indices and the interventional method. Results were based on logic regression analysis (see also the statistical analysis section). The incidences of change in FEV1% > 1.2%, FVC% > 1.1%, MVV% > 1.1%, VA/CO < –0.29, DLO2 > –0.15 mmol/min/kPa, CaO2 > –0.9 ml/dl, DO2 > 70.1 ml/min, and EO2 > 7 ml/min in patients post-PEA were 0.258, 0.274, 0.186, 3.386, 0.066, 0.300, 3.311, 3.183 times as those in patients post-BPA. Incidences of change in MMEF% > 0.5%, VA% > 1.1%, PalvO2 < –3.8 mmHg, PaO2 > 4.5 mmHg, SaO2 > 1.1%, SmvO2 > 0%, and CO > 0.58 L/min were comparable between patients post-PEA and BPA. *P < 0.05. Δ, change in the indices. PEA, pulmonary endarterectomy; BPA, balloon pulmonary angioplasty; FEV1%, the percentage of the forced expiratory volume in the 1 s; FVC%, the percentage of the forced vital capacity; MMEF%, the percentage of the predicted maximum mid-expiratory flow; MVV%, the percentage of the predicted maximum voluntary ventilation; VA%, the percentage of the predicted alveolar ventilation; VA/CO, alveolar ventilation-to-cardiac output ratio; DLO2, diffusion capacity of the lungs for oxygen; PaO2, partial pressure of oxygen in the radial artery; PalvO2, alveolar partial pressure of oxygen; SaO2, saturation of oxygen in the radial artery; SmvO2, mixed venous oxygen saturation; CO, cardiac output; CaO2, arterial oxygen content; DO2, oxygen delivery; EO2, oxygen extraction.
In this study, we used PaO2 and SaO2 to assess the efficacy of pulmonary gas exchange. Both lung diffusion capacity and ventilation/perfusion can have an impact on lung gas exchange. Patients post-PEA had a significant higher PaO2 than those post-BPA (73.8 ± 9.8 vs. 65.0 ± 7.5 mmHg, P = 0.022) (Figure 2F and Supplementary Table 1). Meanwhile, patients in the PEA group had a significant increase in SaO2 (from 92.5 ± 3.6 to 94.6 ± 2.4%, P = 0.022) (Figure 2G and Supplementary Table 1), while those in the BPA group had no change in SaO2, which could be explained by a better improvement of VA/CO after PEA (−0.48 ± 0.53 vs. −0.17 ± 0.41, P = 0.016) (Table 4 and Figures 2H, 3). Interestingly, patients in the PEA group had a significant decrease in DLO2 (from 7.53 ± 1 to 6.99 ± 1.95 mmol/min/kPa, P = 0.004), while those in the BPA group had no change (Figure 2I; Supplementary Table 1 and Table 4).
The oxygen delivery (DO2) in patients after PEA was significantly higher than that in patients treated with BPA (756.3 ± 229.1 vs. 628.8 ± 188.5 ml/min, P = 0.016) (Figure 2J and Supplementary Table 1), while a comparable improvement in cardiac output was found among the PEA and BPA groups (1.00 ± 1.61 vs. 0.36 ± 1.05 L/min, P = 0.051) (Table 4 and Figures 2K, 3). Compared with patients post-BPA, those post-PEA had a significant decrease in the arterial oxygen content (−2.0 ± 3.5 vs. −0.3 ± 2.2 ml/dl, P = 0.039) (Table 4 and Figures 2L, 3).
Although the oxygen extraction of peripheral tissues (EO2) at rest in CTEPH patients had no statistically significant change after PEA and BPA (from 177.5 ± 40.4 to 203.3 ± 64.8 ml/min for PEA, P = 0.076 and from 156.3 ± 45.3 to 151.2 ± 31.9 ml/min for BPA, P = 0.243, respectively) (Figure 2M and Supplementary Table 1), patients treated with PEA had a significant increase in change of oxygen extraction than those treated with BPA (25.8 ± 68.1 vs. −7.8 ± 42.7 ml/min, P = 0.016) (Table 4 and Figure 3). In addition, there were no significant difference in the PalvO2 (P = 0.507, Figure 2N) and the SmvO2 (P = 0.611, Figure 2O) after the interventions between the PEA and BPA groups.
Discussion
We retrospectively collected data on CTEPH patients receiving PEA or BPA in our center to assess and compare each step of the oxygen pathway before and after intervention and between these two interventions. Such comparisons had not been performed in previous studies. We found that patients with CTEPH had multiple limitations in the oxygen pathway (Table 2), and partial amelioration could be achieved after PEA or BPA. Interestingly, the oxygen pathway of CTEPH patients responded differently to PEA and BPA. Patients post-PEA showed better lung gas exchange and oxygen delivery, while patients post-BPA had better lung ventilation. Although no significant change in the extraction of oxygen was found in patients after the intervention, patients receiving PEA showed more improvement than those receiving BPA. The effects of PEA and BPA on the oxygen pathway of CTEPH patients are shown in Figure 4.
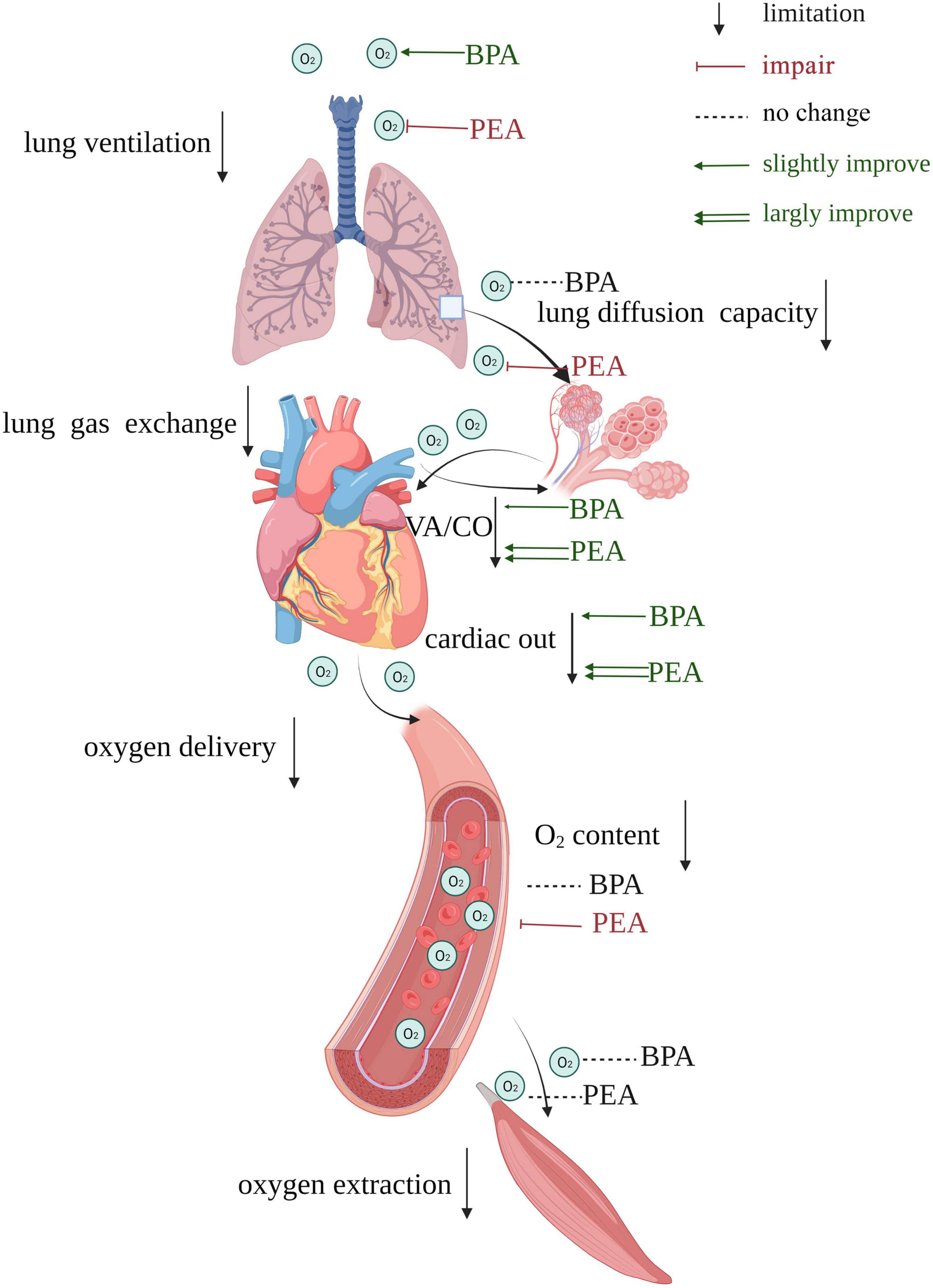
Figure 4. Summary of the effects of PEA versus BPA on the oxygen pathway of patients with CTEPH. Impairments exit in both lung ventilation, lung gas exchange, oxygen delivery, and oxygen extraction. BPA could improve lung ventilation, while PEA causes damage to it. VA/CO gets more improvement in patients receiving PEA than those receiving BPA. PEA impairs lung diffusion capacity, while BPA does not affect it. The cardiac output of patients receiving PEA gets more improvement than those receiving BPA, and PEA could reduce the oxygen content. There is no significant change in oxygen extraction after PEA or BPA. VA/CO, alveolar ventilation-to-cardiac output ratio.
The oxygen uptake involves lung ventilation and lung gas exchange. In this study, we have found that the dysfunction of the small airway in lung ventilation in a single breath (decreased maximum mid-expiratory flow) did not cause insufficient lung ventilation (alveolar ventilation was about 94% of predicted) in patients with CTEPH (Table 2). Maximum mid-expiratory flow (MMEF) is defined as the maximum expiratory flow between 25 and 75% of the FVC and is used to assess small airway obstruction (23). Small airway function is rarely investigated in patients with CTEPH and the MMEF of patients in this study was less than 50% of the predicted value. One previous study has found a potential diagnostic and prognostic value of MMEF in patients with respiratory symptoms without chronic obstructive pulmonary disease (24). In our study, the MMEF was strongly correlated with MVV (r = 0.831, P < 0.001) in patients with CTEPH. The CTEPH patients of this study showed a slight decrease in maximum voluntary ventilation (∼66% of predicted, Table 2), which reflects a decrease in the respiratory ventilation reserve in CTEPH. It is possible that a decrease in MMEF might contribute to the decrease of MVV. For spirometry, FVC and FEV1 are the two most important parameters (25). The FVC in CTEPH patients was normal in this study (Table 1), consistent with previous studies (26, 27). Compared with a decreased FEV1 in previous studies on CTEPH patients, in our study, the FEV1 was normal. The possible decrease in FEV1 in CTEPH may be associated with airway diseases (25). DLCO is used to assess the diffusion capacity of the lung. In our study, the DLCO in patients with CTEPH was nearly 70% of the predicted value, consistent with the results of previous studies (13, 14). Previous pathological studies have reported frequent presentation of the thickened alveolar wall and deficient angiogenesis in the lungs of CTEPH patients (28), suggesting that the abnormalities of both the alveolar membrane and pulmonary capillary might contribute to the decrease of DLCO in CTEPH. Howden et al. have reported a decrease in oxygen delivery in CTEPH patients at peak exercise (15), and the mean oxygen delivery at rest was < 645 ml/min in CTEPH patients in our study, which we think was below the normal level, and the reasons for which were as follows: (i) The oxygen delivery is determined by cardiac output and arterial oxygen content, which could be affected by any pathophysiology with an impact on these indices (19); (ii) The arterial oxygen content is a parameter derived from SaO2 and PaO2 (19); and (iii) The cardiac output index (< 1.9 L/min/m2), SaO2 (< 93%), and PaO2 (< 67 mmHg) in CTEPH patients were all below normal levels in this study. The oxygen extraction of patients with CTEPH in our study was less than 180 ml/min (reference value for adults: 250 ml/min) (29), indicating a secondary impairment of oxygen use in the periphery in patients with CTEPH. The mitochondrial oxidative phosphorylation capacity is equal to 1.8 times the uptake of oxygen (15). The mitochondrial oxidative phosphorylation capacity was not calculated in this study, because the cardiopulmonary excise test was not routinely tested in our center. Nevertheless, Gimenez et al. demonstrated that the uptake of oxygen was strong corrected with MVV (r = 0.765, P < 0.001) (30), and the MVV of CTEPH patients was below the normal level in our study, so it was reasonable to speculate that the mitochondrial oxidative phosphorylation capacity might be decreased.
Chronic thromboembolic pulmonary hypertension patients treated with PEA had a worsened lung diffusion capacity, while patients with CTEPH treated with BPA had an improved lung ventilation. Previous studies have revealed that the lung diffusion capacity in CTEPH patients decreased in the short term (3 weeks) after PEA (14), and could persist for more than 1 year (31), which were consistent with our study (Table 2). Compared with the deterioration of the lung diffusion capacity in CTEPH patients treated with PEA, the impaired lung diffusion capacity was not changed (P = 0.679, Supplementary Table 1) in CTEPH patients treated with BPA in both our study and previous studies (26, 32). The reason for the different effects of the two interventions on lung diffusion capacity was likely due to the high reperfusion pulmonary edema (RPE) caused by a rapid decrease of pulmonary hypertension after PEA. The occurrence of RPE was more than 50% in recent PEA studies (33, 34), compared with less than 10% in BPA (32, 35). Another reason for the decrease of lung diffusion capacity after PEA might be mechanical ventilation complications, leading to alveolar damage, possibly attributable to an increase in surfactant protein type B (34). Of note, we used uncorrected DLCO in this study, which could be another reason for the decline in DLCO for patients post-PEA (36). Interestingly, Akizuki et al. found that the lung diffusion capacity in different lung fields responded differently to BPA (26). In their study, the diffusion capacity in the upper-middle lung field in CTEPH patients was improved after BPA, while that in the upper lung field was decreased. Although the lung diffusion capacity of patients with CTEPH was not improved by PEA or BPA in our study, a mild improvement at peak exercise 6 months after intervention [PEA (n = 8) and BPA (n = 2)] was found in a small prospective study (15). After the intervention, the change in FVC in patients receiving BPA was significantly different from that in patients receiving PEA. The negative trend in FVC in patients post-PEA could be due to the major cardiothoracic surgery they underwent. An increase of FVC in patients receiving BPA was significantly negatively correlated with the change in PVR (r = −0.44, P < 0.01) in a report from Takei et al. (37). This indicated that some unknown factors, in addition to reperfusion of the pulmonary artery, might be involved in the improvement of pulmonary function in CTEPH patients after BPA. Moreover, the change in MVV% was significantly different between patients receiving BPA and PEA (−2.3 ± 9.7% vs. 2.5 ± 6.5%, P = 0.023), and was significantly correlated with the change in FVC (r = 0.803, P < 0.001).
Despite better lung ventilation in patients receiving BPA than those receiving PEA, there was no significant difference in the change in VA (P = 0.126). It seemed that PEA patients had a reduction in anatomical dead space due to the thoracic surgery, which requires further research. On the other hand, patients treated with PEA in this study tended to show more improvement in lung gas exchange, which could be explained by a better VA/CO (1.16 vs. 1.42, P = 0.010), attributable to a higher cardiac output (4.47 ± 1.06 L/min vs. 3.67 ± 1.04 L/min, P = 0.004) after PEA. Moreover, Pearson’s correlation analysis revealed that the change in VA/CO was correlated with the change in PaO2 (r = −0.377, P = 0.003) and SaO2 (r = −0.410, P = 0.001). Those evidences indicated that PEA may be superior to BPA in term of vascular recanalization, which contributed to more reduction in physiological dead space.
In our study, the oxygen delivery in patients treated with PEA was significantly higher than that in patients treated with BPA (P = 0.016), attributable to a better cardiac output after the intervention. Based on the evidence that the cardiac output was significantly improved, even superior to BPA, in inoperable CTPEH patients who received riociguat (38), combination therapy of BPA and riociguat could be prescribed to better improve the oxygen delivery in inoperable CTEPH patients. Interestingly, despite an improvement of SaO2, the oxygen content decreased in patients post-PEA (P = 0.011), which can be explained by the decrease of hemoglobin after PEA (from 14.8 ± 1.9 to 12.9 ± 2.0 g/dl, P = 0.001, Supplementary Table 1). This phenomenon was also found in the paper from Howden et al. (15) possibly attributable to a decrease of erythropoietin resulting from an improvement of hypoxia (39, 40), or loss of hemoglobin caused by the intervention (41).
According to Howden et al., the oxygen extraction by peripheral tissues at peak exercise was normal in patients with CTEPH (15). In spite of this, skeletal muscle dysfunction existed reportedly in CTEPH patients (42), which indicates the decrease in other nutrients supply due to the reduced cardiac output, may result in the muscle dysfunction. In our study, the oxygen extraction at rest showed no change after intervention. However, there was a significant difference in the change in oxygen extraction among patients treated with PEA versus BPA (Table 4 and Figure 3), where patients treated with PEA had a better performance in oxygen extraction than those receiving BPA. The oxygen extraction is comprised of oxygen transporting from capillary to cells and oxygen utilization by the mitochondria (19). Therefore, the higher oxygen extraction in PEA patients could be partly due to change in oxygen transporting from capillary to cells, resulting from significant improvement in oxygen delivery. Interestingly, a normal extraction of peripheral tissues was found at rest and peak exercise, and the muscle diffusion capacity of oxygen was below the normal level in their study, which suggested a potential benefit of cardiopulmonary rehabilitation in CTEPH patients.
Cardiopulmonary rehabilitation could improve the cardiac output of patients with CTEPH, which in turn could improve the mismatched lung ventilation/perfusion and oxygen delivery (43). In addition, based on the evidence that patients with heart failure could improve their oxygen extraction after exercise training (44, 45), the oxygen extraction in CTEPH patients is likely to benefit from cardiopulmonary rehabilitation. Although no previous study has been designed to investigate the effect of cardiopulmonary rehabilitation on lung diffusion capacity, the lung diffusion capacity in CTEPH patients don’t seem to benefit from cardiopulmonary rehabilitation based on the evidence that no superior results have been found in other respiratory diseases (sarcoidosis, pulmonary fibrosis and cystic fibrosis) in previous research (46–48). Riociguat and treprostinil have been approved for patients with CTEPH and could increase the cardiac output (49, 50). Other PH-targeted medications, such as phosphodiesterase-5 inhibitors and macitentan, have the same effect (51, 52). Of note, riociguat could increase the area of lung gas exchange through its pro-angiogenic function (53). However, in our study, there were no significant differences in change in lung gas exchange (0.00 ± 0.69 vs. 0.07 ± 0.89 mmol/min/kPa, P = 0.786 for change in DLO2; 0.6 ± 1.8 vs. 0.6 ± 4.2%, P = 0.995 for change in SaO2, respectively), oxygen delivery (0.74 ± 0.7 vs. 0.21 ± 1.13 L/min, P = 0.129 for change in CO; 91.7 ± 128.4 vs. 28.9 ± 210.3 ml/min, P = 0.341 for change in DO2, respectively), and oxygen extraction (0.2 ± 50.4 vs. −11.0 ± 39.8 ml/min, P = 0.451) between patients treated with and without targeted medications after BPA (Supplementary Table 2). On the contrary, patients treated with targeted medications after BPA had lower improvements in FEV1% (−0.1 ± 7.3 vs. 5.5 ± 8.8%, P = 0.041), MMEF% (−5.5 ± 7.2 vs. 3.4 ± 13.1%, P = 0.022), and MVV% (−0.5 ± 5.3 vs. 3.8 ± 6.7%, P = 0.043) than those without receiving targeted medications. This phenomenon could be explained by the fact that patients with higher pulmonary hypertension were more likely to receive targeted medications according to the contemporary guideline (54). And patients post-BPA, who were treated with targeted medications after the last session of intervention, had higher mean pulmonary artery pressure (29.9 ± 7.6 vs. 25.1 ± 5.9 mmHg, P = 0.030) than those without receiving targeted medications in our study. Many CTEPH patients may have mixed vascular lesions with both proximal and distal lesions. In term of reducing lung ventilation impairment post-PEA, combination therapy of PEA and BPA could be an option for those patients according to our study. Another option could be minimally invasive PEA surgery, which is performed through mini-anterior thoracotomy instead of sternotomy (55). Some CTEPH patients still showed persistent pulmonary hypertension even after several BPA sessions (56), therefore it is essential to identify the predictors of hemodynamic response. Zhihong Liu and her colleagues found a baseline DLCO% < 70% and change in DLCO% > 6% could be an unfavorable predictor for BPA (32). According to the research from Tsuji A. et al., preoperative FEV1 was a predictor of residual pulmonary hypertension after BPA in CTEPH patients (56). Moreover, postoperative PaO2 was a prognostic predictor of patients post-PEA (57). And mixed venous oxygen saturation was reportedly associated with prognosis of patients post-PEA and improved renal function of patients after BPA (58, 59). Therefore, the oxygen pathway parameters could be used to guide decision-making in the management of CTEPH patients.
This study has several limitations, mainly owing to the retrospective design. The main limitation is that the pulmonary function was tested at the upright position, while the hemodynamics was evaluated at the supine position, which could influence the result of VA/CO (60). Secondly, after all exclusions only 24 patients post-PEA and 46 patients post-BPA remained, which is suggesting potential health worker survivor bias. Thirdly, patients not suitable for PEA may be technically more difficult, and may have a poor risk-benefit because of comorbidities, in spite of a comparable pre-interventional WHO-FC and post-interventional mPAP (24.3 ± 8.5 vs. 26.5 ± 6.7 mmHg, P = 0.238, Supplementary Table 2) among the PEA and BPA groups.
In conclusion, in this single-center retrospective study, we evaluated and compared each step of the oxygen pathway in CTEPH patients treated with PEA and BPA. Partial improvements in oxygen limitation could be achieved after PEA and BPA, with key differences in the responses to these treatments. Additional research is warranted to investigate the effect of targeted medications for pulmonary hypertension and the role of the oxygen pathway in the management of CTEPH. Given the cardiopulmonary and peripheral defects in the oxygen pathway, cardiopulmonary rehabilitation can be prescribed to patients treated after PEA and BPA.
Data availability statement
The original contributions presented in this study are included in the article/Supplementary material, further inquiries can be directed to the corresponding author.
Ethics statement
The studies involving human participants were reviewed and approved by the Institutional Board and the Ethics Committee of the China-Japan Friendship Hospital (2021-136-K94). The patients/participants provided their written informed consent to participate in this study.
Author contributions
ZZ had full access to all the data and takes responsibility for its integrity and the data analysis. ZF contributed to the study conception and design, data collection and analysis, and drafting of the manuscript. XT, WX, QG, and JW were involved in the data collection. PY was involved in revising the manuscript. All authors have read and approved the final manuscript.
Funding
This study was supported by the Chinese Academy of Medical Sciences Innovation Fund for Medical Sciences (CIFMS) (2021-I2M-1-049) and funded by the National Natural Science Foundation of China (No. 81970058).
Conflict of interest
The authors declare that the research was conducted in the absence of any commercial or financial relationships that could be construed as a potential conflict of interest.
Publisher’s note
All claims expressed in this article are solely those of the authors and do not necessarily represent those of their affiliated organizations, or those of the publisher, the editors and the reviewers. Any product that may be evaluated in this article, or claim that may be made by its manufacturer, is not guaranteed or endorsed by the publisher.
Supplementary material
The Supplementary Material for this article can be found online at: https://www.frontiersin.org/articles/10.3389/fcvm.2022.990207/full#supplementary-material
References
1. Pang W, Zhang Z, Wang Z, Zhen K, Zhang Y, Gao Q, et al. Higher incidence of chronic thromboembolic pulmonary hypertension after acute pulmonary embolism in Asians than in Europeans: a meta-analysis. Front Med. (2021) 8:721294. doi: 10.3389/fmed.2021.721294
2. Delcroix M, Torbicki A, Gopalan D, Sitbon O, Klok FA, Lang I, et al. ERS statement on chronic thromboembolic pulmonary hypertension. Eur Respir J. (2021) 57:2002828. doi: 10.1183/13993003.02828-2020
3. de Perrot M, Gopalan D, Jenkins D, Lang IM, Fadel E, Delcroix M, et al. Evaluation and management of patients with chronic thromboembolic pulmonary hypertension – consensus statement from the ISHLT. J Heart Lung Transpl. (2021) 40:1301–26. doi: 10.1016/j.healun.2021.07.020
4. Delcroix M, Lang I, Pepke-Zaba J, Jansa P, D’Armini AM, Snijder R, et al. Long-term outcome of patients with chronic thromboembolic pulmonary hypertension: results from an international prospective registry. Circulation. (2016) 133:859–71. doi: 10.1161/CIRCULATIONAHA.115.016522
5. Zhang L, Bai Y, Yan P, He T, Liu B, Wu S, et al. Balloon pulmonary angioplasty vs. pulmonary endarterectomy in patients with chronic thromboembolic pulmonary hypertension: a systematic review and meta-analysis. Heart Fail Rev. (2021) 26:897–917. doi: 10.1007/s10741-020-10070-w
6. Treacher DF, Leach RM. Oxygen transport-1. Basic principles. BMJ. (1998) 317:1302–6. doi: 10.1136/bmj.317.7168.1302
7. Carter JL, Hege K, Kalpage HA, Edwards H, Hüttemann M, Taub JW, et al. Targeting mitochondrial respiration for the treatment of acute myeloid leukemia. Biochem Pharmacol. (2020) 182:114253. doi: 10.1016/j.bcp.2020.114253
8. Houstis NE, Eisman AS, Pappagianopoulos PP, Wooster L, Bailey CS, Wagner PD, et al. Exercise intolerance in heart failure with preserved ejection fraction: diagnosing and ranking its causes using personalized O pathway analysis. Circulation. (2018) 137:148–61. doi: 10.1161/CIRCULATIONAHA.117.029058
9. Pandkar MR, Dhamdhere SG, Shukla S. Oxygen gradient and tumor heterogeneity: the chronicle of a toxic relationship. Biochim Biophys Acta Rev Cancer. (2021) 1876:188553. doi: 10.1016/j.bbcan.2021.188553
10. DeCato TW, Haverkamp H, Hegewald MJ. Cardiopulmonary exercise testing (CPET). Am J Respir Crit Care Med. (2020) 201:1–2. doi: 10.1164/rccm.2011P1
11. van den Akker LE, Heine M, van der Veldt N, Dekker J, de Groot V, Beckerman H. Feasibility and safety of cardiopulmonary exercise testing in multiple sclerosis: a systematic review. Arch Phys Med Rehabil. (2015) 96:2055–66. doi: 10.1016/j.apmr.2015.04.021
12. Venkatesan P. GOLD report: 2022 update. Lancet Respir Med. (2022) 10:e20. doi: 10.1016/S2213-2600(21)00561-0
13. Blanquez-Nadal M, Piliero N, Guillien A, Doutreleau S, Salvat M, Thony F, et al. Exercise hyperventilation and pulmonary gas exchange in chronic thromboembolic pulmonary hypertension: effects of balloon pulmonary angioplasty. J Heart Lung Transpl. (2021) 41:70–79. doi: 10.1016/j.resp.2022.103857
14. Bernstein RJ, Ford RL, Clausen JL, Moser KM. Membrane diffusion and capillary blood volume in chronic thromboembolic pulmonary hypertension. Chest. (1996) 110:1430–6. doi: 10.1378/chest.110.6.1430
15. Howden EJ, Ruiz-Carmona S, Claeys M, De Bosscher R, Willems R, Meyns B, et al. Oxygen pathway limitations in patients with chronic thromboembolic pulmonary hypertension. Circulation. (2021) 143:2061–73. doi: 10.1161/CIRCULATIONAHA.120.052899
16. Mizoguchi H, Ogawa A, Munemasa M, Mikouchi H, Ito H, Matsubara H. Refined balloon pulmonary angioplasty for inoperable patients with chronic thromboembolic pulmonary hypertension. Circ Cardiovasc Interv. (2012) 5:748–55. doi: 10.1161/CIRCINTERVENTIONS.112.971077
17. Miller MR, Hankinson J, Brusasco V, Burgos F, Casaburi R, Coates A, et al. Standardisation of spirometry. Eur Respir J. (2005) 26:319–38.
18. Munkholm M, Marott JL, Bjerre-Kristensen L, Madsen F, Pedersen OF, Lange P, et al. Reference equations for pulmonary diffusing capacity of carbon monoxide and nitric oxide in adult caucasians. Eur Respir J. (2018) 52:1500677. doi: 10.1183/13993003.00677-2015
19. Toffaletti JG, Rackley CR. Monitoring oxygen status. Adv Clin Chem. (2016) 77:103–24. doi: 10.1016/bs.acc.2016.06.003
20. Khurana I. Medical Physiology for Undergraduate Students – E-Book. Chennai: Elsevier India (2011).
21. Surie S, Bouma BJ, Bruin-Bon RA, Hardziyenka M, Kloek JJ, Van der Plas MN, et al. Time course of restoration of systolic and diastolic right ventricular function after pulmonary endarterectomy for chronic thromboembolic pulmonary hypertension. Am Heart J. (2011) 161:1046–52. doi: 10.1016/j.ahj.2011.03.001
22. Giusca S, Dambrauskaite V, Scheurwegs C, D’Hooge J, Claus P, Herbots L, et al. Deformation imaging describes right ventricular function better than longitudinal displacement of the tricuspid ring. Heart. (2010) 96:281–8. doi: 10.1136/hrt.2009.171728
23. Knox-Brown B, Mulhern O, Amaral AFS. Spirometry parameters used to define small airways obstruction in population-based studies: systematic review protocol. BMJ Open. (2021) 11:e052931. doi: 10.1136/bmjopen-2021-052931
24. Güder G, Brenner S, Störk S, Held M, Broekhuizen BD, Lammers JW, et al. Diagnostic and prognostic utility of mid-expiratory flow rate in older community-dwelling persons with respiratory symptoms, but without chronic obstructive pulmonary disease. BMC Pulm Med. (2015) 15:83. doi: 10.1186/s12890-015-0081-4
25. Culver BH, Graham BL, Coates AL, Wanger J, Berry CE, Clarke PK, et al. Recommendations for a standardized pulmonary function report. An official American thoracic society technical statement. Am J Respir Crit Care Med. (2017) 196:1463–72. doi: 10.1164/rccm.201710-1981ST
26. Akizuki M, Serizawa N, Ueno A, Adachi T, Hagiwara N. Effect of balloon pulmonary angioplasty on respiratory function in patients with chronic thromboembolic pulmonary hypertension. Chest. (2017) 151:643–9.
27. Steenhuis LH, Groen HJ, Koëter GH, van der Mark TW. Diffusion capacity and haemodynamics in primary and chronic thromboembolic pulmonary hypertension. Eur Respir J. (2000) 16:276–81.
28. Lang IM, Dorfmüller P, Vonk Noordegraaf A. The pathobiology of chronic thromboembolic pulmonary hypertension. Ann Am Thorac Soc. (2016) 13(Suppl. 3):S215–21. doi: 10.1513/AnnalsATS.201509-620AS
29. Singh H, Singh I. Fundamentals of Medical Physiology-Ebook. Philadelphia: Elsevier India (2018).
30. Gimenez M, Salinas W, Servera E, Kuntz C. VO2 max during progressive and constant bicycle exercise in sedentary men and women. Eur J Appl Physiol Occup Physiol. (1981) 46:237–48. doi: 10.1007/BF00423400
31. Corsico AG, D’Armini AM, Conio V, Sciortino A, Pin M, Grazioli V, et al. Persistent exercise limitation after successful pulmonary endoarterectomy: frequency and determinants. Respir Res. (2019) 20:34.
32. Li X, Zhang Y, Luo Q, Zhao Q, Zeng Q, Yang T, et al. Diffusing capacity for carbon monoxide predicts response to balloon pulmonary angioplasty in patients with inoperable chronic thromboembolic pulmonary hypertension. Front Cardiovasc Med. (2021) 8:762267. doi: 10.3389/fcvm.2021.762267
33. Ikubo Y, Sanada TJ, Tanabe N, Naito A, Shoji H, Nagata J, et al. The extent of enlarged bronchial arteries is not correlated with the development of reperfusion pulmonary EDEMA after pulmonary endarterectomy in patients with chronic thromboembolic pulmonary hypertension. Pulm Circ. (2020) 10:2045894020968677.
34. Stéphan F, Mazeraud A, Laverdure F, Camous J, Fadel E. Evaluation of reperfusion pulmonary edema by extravascular lung water measurements after pulmonary endarterectomy. Crit Care Med. (2017) 45:e409–17.
35. Brenot P, Jaïs X, Taniguchi Y, Garcia Alonso C, Gerardin B, Mussot S, et al. French experience of balloon pulmonary angioplasty for chronic thromboembolic pulmonary hypertension. Eur Respir J. (2019) 53:1802095.
36. Marrades RM, Diaz O, Roca J, Campistol JM, Torregrosa JV, Barberà JA, et al. Adjustment of DLCO for hemoglobin concentration. Am J Respir Crit Care Med. (1997) 155:236–41. doi: 10.1164/ajrccm.155.1.9001318
37. Takei M, Kataoka M, Kawakami T, Kuwahira I, Fukuda K. Respiratory function and oxygenation after balloon pulmonary angioplasty. Int J Cardiol. (2016) 212:190–1. doi: 10.1016/j.ijcard.2016.03.061
38. Kawakami T, Matsubara H, Shinke T, Abe K, Kohsaka S, Hosokawa K, et al. Balloon pulmonary angioplasty versus riociguat in inoperable chronic thromboembolic pulmonary hypertension (MR BPA): an open-label, randomised controlled trial. Lancet Respir Med. (2022) doi: 10.1016/S2213-2600(22)00171-0
39. Goldberg MA, Dunning SP, Bunn HF. Regulation of the erythropoietin gene: evidence that the oxygen sensor is a heme protein. Science. (1988) 242:1412–5.
40. Karamanian VA, Harhay M, Grant GR, Palevsky HI, Grizzle WE, Zamanian RT, et al. Erythropoietin upregulation in pulmonary arterial hypertension. Pulm Circ. (2014) 4:269–79. doi: 10.1086/675990
41. McRae K, Shargall Y, Ma M, Thenganatt J, Slinger P, Granton JT, et al. Feasibility of blood conservation strategies in pulmonary endarterectomy for chronic thromboembolic pulmonary hypertension. Interact Cardiovasc Thorac Surg. (2011) 13:35–8. doi: 10.1510/icvts.2010.252767
42. Tobita K, Goda A, Nishida Y, Takeuchi K, Kikuchi H, Inami T, et al. Factors contributing to exercise capacity in chronic thromboembolic pulmonary hypertension with near-normal hemodynamics. J Heart Lung Transpl. (2021) 40:677–86. doi: 10.1016/j.healun.2021.03.003
43. Ehlken N, Lichtblau M, Klose H, Weidenhammer J, Fischer C, Nechwatal R, et al. Exercise training improves peak oxygen consumption and haemodynamics in patients with severe pulmonary arterial hypertension and inoperable chronic thrombo-embolic pulmonary hypertension: a prospective, randomized, controlled trial. Eur Heart J. (2016) 37:35–44. doi: 10.1093/eurheartj/ehv337
44. Duscha BD, Schulze PC, Robbins JL, Forman DE. Implications of chronic heart failure on peripheral vasculature and skeletal muscle before and after exercise training. Heart Fail Rev. (2008) 13:21–37. doi: 10.1007/s10741-007-9056-8
45. Hirai DM, Musch TI, Poole DC. Exercise training in chronic heart failure: improving skeletal muscle O2 transport and utilization. Am J Physiol Heart Circ Physiol. (2015) 309:H1419–39. doi: 10.1152/ajpheart.00469.2015
46. Karadallı MN, Boşnak-Güçlü M, Camcıoğlu B, Kokturk N, Türktaş H. Effects of inspiratory muscle training in subjects with sarcoidosis: a randomized controlled clinical trial. Respir Care. (2016) 61:483–94. doi: 10.4187/respcare.04312
47. Li X, Yu R, Wang P, Wang A, Huang H. Effects of exercise training on cardiopulmonary function and quality of life in elderly patients with pulmonary fibrosis: a meta-analysis. Int J Environ Res Public Health. (2021) 18:7643.
48. Radtke T, Böni L, Bohnacker P, Maggi-Beba M, Fischer P, Kriemler S, et al. Acute effects of combined exercise and oscillatory positive expiratory pressure therapy on sputum properties and lung diffusing capacity in cystic fibrosis: a randomized, controlled, crossover trial. BMC Pulm Med. (2018) 18:99.
49. Sadushi-Kolici R, Jansa P, Kopec G, Torbicki A, Skoro-Sajer N, Campean IA, et al. Subcutaneous treprostinil for the treatment of severe non-operable chronic thromboembolic pulmonary hypertension (CTREPH): a double-blind, phase 3, randomised controlled trial. Lancet Respir Med. (2019) 7:239–48. doi: 10.1016/S2213-2600(18)30367-9
50. Barnikel M, Kneidinger N, Arnold P, Waelde A, Behr J, Milger K. Riociguat in patients with CTEPH and advanced age and/or comorbidities. J Clin Med. (2022) 11:1084. doi: 10.3390/jcm11041084
51. Ghofrani HA, Simonneau G, D’Armini AM, Fedullo P, Howard LS, Jaïs X, et al. Macitentan for the treatment of inoperable chronic thromboembolic pulmonary hypertension (MERIT-1): results from the multicentre, phase 2, randomised, double-blind, placebo-controlled study. Lancet Respir Med. (2017) 5:785–94. doi: 10.1183/1393003.congress-2017.OA1984
52. Barnes H, Brown Z, Burns A, Williams T. Phosphodiesterase 5 inhibitors for pulmonary hypertension. Cochrane Database Syst Rev. (2019) 1:Cd012621.
53. Quarck R, Wynants M, Verbeken E, Meyns B, Delcroix M. Contribution of inflammation and impaired angiogenesis to the pathobiology of chronic thromboembolic pulmonary hypertension. Eur Respir J. (2015) 46:431–43. doi: 10.1183/09031936.00009914
54. Galiè N, Humbert M, Vachiery JL, Gibbs S, Lang I, Torbicki A, et al. 2015 ESC/ERS guidelines for the diagnosis and treatment of pulmonary hypertension: the joint task force for the diagnosis and treatment of pulmonary hypertension of the European Society of cardiology (ESC) and the European respiratory society (ERS): endorsed by: association for European paediatric and congenital cardiology (AEPC), international society for heart and lung transplantation (ISHLT). Eur Heart J. (2016) 37:67–119. doi: 10.1093/eurheartj/ehv317
55. Higgins JR, Kim NH, Kerr K, Fernandez T, Poch D, Madani MA. Comparison of short term outcomes of minimally invasive versus sternotomy pulmonary thromboendarterectomy. J Heart Lung Transpl. (2018) 37:S25–6. doi: 10.1016/j.healun.2018.01.041
56. Tsuji A, Ogo T, Ueda J, Fukui S, Morita Y, Fukuda T, et al. Predictors of residual pulmonary hypertension after balloon pulmonary angioplasty in patients with chronic thromboembolic pulmonary hypertension. Int J Cardiol. (2017) 226:118–20. doi: 10.1016/j.ijcard.2016.09.132
57. Ghio S, Klersy C, Corsico A, Gamba SL, Monterosso C, Masiglat J, et al. Risk stratification in patients with residual pulmonary hypertension after pulmonary endarterectomy. Int J Cardiol. (2021) 334:116–22. doi: 10.1016/j.ijcard.2021.04.003
58. Isobe S, Itabashi Y, Kawakami T, Kataoka M, Kohsaka S, Tsugu T, et al. Increasing mixed venous oxygen saturation is a predictor of improved renal function after balloon pulmonary angioplasty in patients with chronic thromboembolic pulmonary hypertension. Heart Vessels. (2019) 34:688–97. doi: 10.1007/s00380-018-1284-4
59. Quadery SR, Swift AJ, Billings CG, Thompson AAR, Elliot CA, Hurdman J, et al. The impact of patient choice on survival in chronic thromboembolic pulmonary hypertension. Eur Respir J. (2018) 52:1800589. doi: 10.1183/13993003.00589-2018
Keywords: balloon pulmonary angioplasty (BPA), pulmonary endarterectomy (PEA), chronic thromboembolic pulmonary disease, oxygen pathway, effect
Citation: Fu Z, Tao X, Xie W, Yang P, Gao Q, Wang J and Zhai Z (2022) Different response of the oxygen pathway in patients with chronic thromboembolic pulmonary hypertension treated with pulmonary endarterectomy versus balloon pulmonary angioplasty. Front. Cardiovasc. Med. 9:990207. doi: 10.3389/fcvm.2022.990207
Received: 09 July 2022; Accepted: 31 August 2022;
Published: 27 September 2022.
Edited by:
Marcin Kurzyna, Medical Center of Postgraduate Medication, PolandReviewed by:
Ghaleb Khirfan, Spectrum Health, United StatesNoriaki Emoto, Kobe Pharmaceutical University, Japan
Copyright © 2022 Fu, Tao, Xie, Yang, Gao, Wang and Zhai. This is an open-access article distributed under the terms of the Creative Commons Attribution License (CC BY). The use, distribution or reproduction in other forums is permitted, provided the original author(s) and the copyright owner(s) are credited and that the original publication in this journal is cited, in accordance with accepted academic practice. No use, distribution or reproduction is permitted which does not comply with these terms.
*Correspondence: Zhenguo Zhai, zhaizhenguo2011@126.com