- 1Cardiac Primary Prevention Research Center (CPPRC), Cardiovascular Diseases Research Institute, Tehran University of Medical Sciences, Tehran, Iran
- 2Tehran Heart Center, Cardiovascular Diseases Research Institute, Tehran University of Medical Sciences, Tehran, Iran
- 3Department of Traditional Pharmacy, School of Persian Medicine, Tehran University of Medical Sciences, Tehran, Iran
- 4Evidence-Based Evaluation of Cost-Effectiveness and Clinical Outcomes, The Institute of Pharmaceutical Sciences (TIPS), Tehran University of Medical Sciences, Tehran, Iran
- 5Department of Pharmacotherapy and Outcomes Science, Virginia Commonwealth University, Richmond, VA, United States
- 6Department of Persian Medicine, School of Traditional Medicine, Tehran University of Medical Sciences, Tehran, Iran
Cardiac arrhythmias, characterized by an irregular heartbeat, are associated with high mortality and morbidity. Because of the narrow therapeutic window of antiarrhythmic drugs (AADs), the management of arrhythmia is still challenging. Therefore, searching for new safe, and effective therapeutic options is unavoidable. In this study, the antiarrhythmic effects of medicinal plants and their active constituents were systematically reviewed to introduce some possible candidates for mechanism-based targeting of cardiac arrhythmias. PubMed, Embase, and Cochrane library were searched from inception to June 2021 to find the plant extracts, phytochemicals, and multi-component herbal preparations with antiarrhythmic activities. From 7337 identified results, 57 original studies consisting of 49 preclinical and eight clinical studies were finally included. Three plant extracts, eight multi-component herbal preparations, and 26 phytochemicals were found to have antiarrhythmic effects mostly mediated by affecting K+ channels, followed by modulating Ca2+ channels, upstream target pathways, Nav channels, gap junction channels, and autonomic receptors. The most investigated medicinal plants were Rhodiola crenulata and Vitis vinifera. Resveratrol, Oxymatrine, and Curcumin were the most studied phytochemicals found to have multiple mechanisms of antiarrhythmic action. This review emphasized the importance of research on the cardioprotective effect of medicinal plants and their bioactive compounds to guide the future development of new AADs. The most prevalent limitation of the studies was their unqualified methodology. Thus, future well-designed experimental and clinical studies are necessary to provide more reliable evidence.
Introduction
Cardiac arrhythmias are characterized by disturbances in the heart’s electrical activity and contribute greatly to cardiovascular morbidity and mortality (1). Although antiarrhythmic drugs (AADs) are still one of the most important therapeutic options, several challenges in their administration, including their narrow therapeutic window and adverse drug reactions, remain to be solved and should be considered in future drug discovery (2). Therefore, investigating novel, safe, and effective therapeutic options with antiarrhythmic activity is unavoidable.
Several common AADs drugs, including digoxin, lidocaine, atropine, and amiodarone have originated from medicinal plants (3); thus, researchers have been eager to extend their knowledge about the potential benefits and mechanism of actions of plants and their active ingredients. Over the past two decades, several studies have been designed to show the impact of plants or their ingredients on cardiovascular disease (CVDs); however, the current dearth of information about the clinical aspects of plants renders their application limited (4–6).
This study provides an overview of the preclinical and clinical studies investigating the effects of plant extracts, multi-component herbal preparations, and phytochemicals on cardiac arrhythmias. We also practically categorized these plant-derived medicines based on their mechanism of antiarrhythmic action according to the classification of conventional AADs, dividing them into eight drug classes involving: (0) blockers of the hyperpolarization-activated cyclic nucleotide-gated (HCN) channel-mediated current (If), (I) blockers of the voltage-gated Na+ (Nav) channels, (II) autonomic inhibitors and activators, (III) K+ channel blockers and openers, (IV) Ca2+ handling modulators, (V) mechanosensitive transient receptor potential channels subfamily C (TRPC3/TRPC6) blockers, (VI) gap junction channel modulators, and (VII) upstream target modulators (7). We believe that this categorization can confer practitioners a better understanding of the potential benefits of medicinal plants and persuade researchers to design randomized clinical trials aimed at clarifying the efficacy of plant extracts and phytochemicals. We also highlighted the research gaps in previous studies and discussed future research directions.
Materials and methods
A systematic search was performed using the Medline/PubMed, Embase, and Cochrane Library databases from inception to June 2021. Also, the references of the included studies were reviewed to find potentially relevant research. The keywords used were: (“medicinal plants” OR “herbal medicine” OR “phytochemical” OR “plant extract”) AND (“arrhythmia” OR “antiarrhythmic”). Inclusion criteria were any original English-language preclinical study in which the antiarrhythmic effect and mechanism of antiarrhythmic action of a herbal extract, a plant-derived active compound, or a multi-component herbal preparation were evaluated. We also included all clinical trials that assessed the antiarrhythmic effects of herbal medicine. Reviews, case reports, and original studies with insufficient data were excluded. After removing duplicates, the titles and abstracts were evaluated for eligibility by two independent investigators. Selected studies were further assessed based on their full text. Information on the study design, dose range tested, the model used (in vitro or in vivo study), type of controls (positive or negative), type of extract, active constituent or multi-component herbal preparation, study duration, antiarrhythmic effects, and mechanism of action were extracted from included studies.
The scientific quality of included animal studies was assessed according to the SYRCLE risk of bias (RoB) tool. This tool consists of ten entries with signaling questions to assist the judgment process in assessing selection bias, performance bias, detection bias, attrition bias, reporting bias, and other sources of bias. A “yes” response to each question represents a low risk of bias, which leads the study to high quality. A “No” response to each question shows a high risk of bias and favors the low quality of the study. An unclear risk of bias means that the information is insufficient to make a decision (8). The quality assessment of clinical studies was performed using Jadad scoring system. This scale determines scores from 1 to 7 points and, thereafter, evaluates the clinical studies as follows: high quality (score >4), moderate quality (score of 3 or 4), and low quality (score of <3) (9).
Results
The characteristics and quality of selected studies
Figure 1 shows the process of study selection. Among a total of 7337 primarily retrieved articles, 49 preclinical and eight clinical studies were finally included. Out of the 49 preclinical studies, four (8.1%) corresponded to plant extract, 10 (20.4%) to multi-component herbal preparations, and 35 (71.4%) to phytochemicals. One clinical study focused on phytochemicals, and the other seven were devoted to multi-component herbal preparations. The antiarrhythmic effects were mostly mediated by affecting K+ channels (class III), followed by modulating Ca2+ channels (IV), upstream target pathways (class VII), Nav channels (class I), gap junction channels (class VI), and autonomic receptors (class III). All the studies that investigated the inhibitory effects of herbal medicine on HCN (class 0) and mechanosensitive TRPC (class V) channels were designed in physiological conditions, and we found no study that evaluated such effects in the arrhythmia models. The detailed characteristics of the final selected studies are summarized in Tables 1–4.
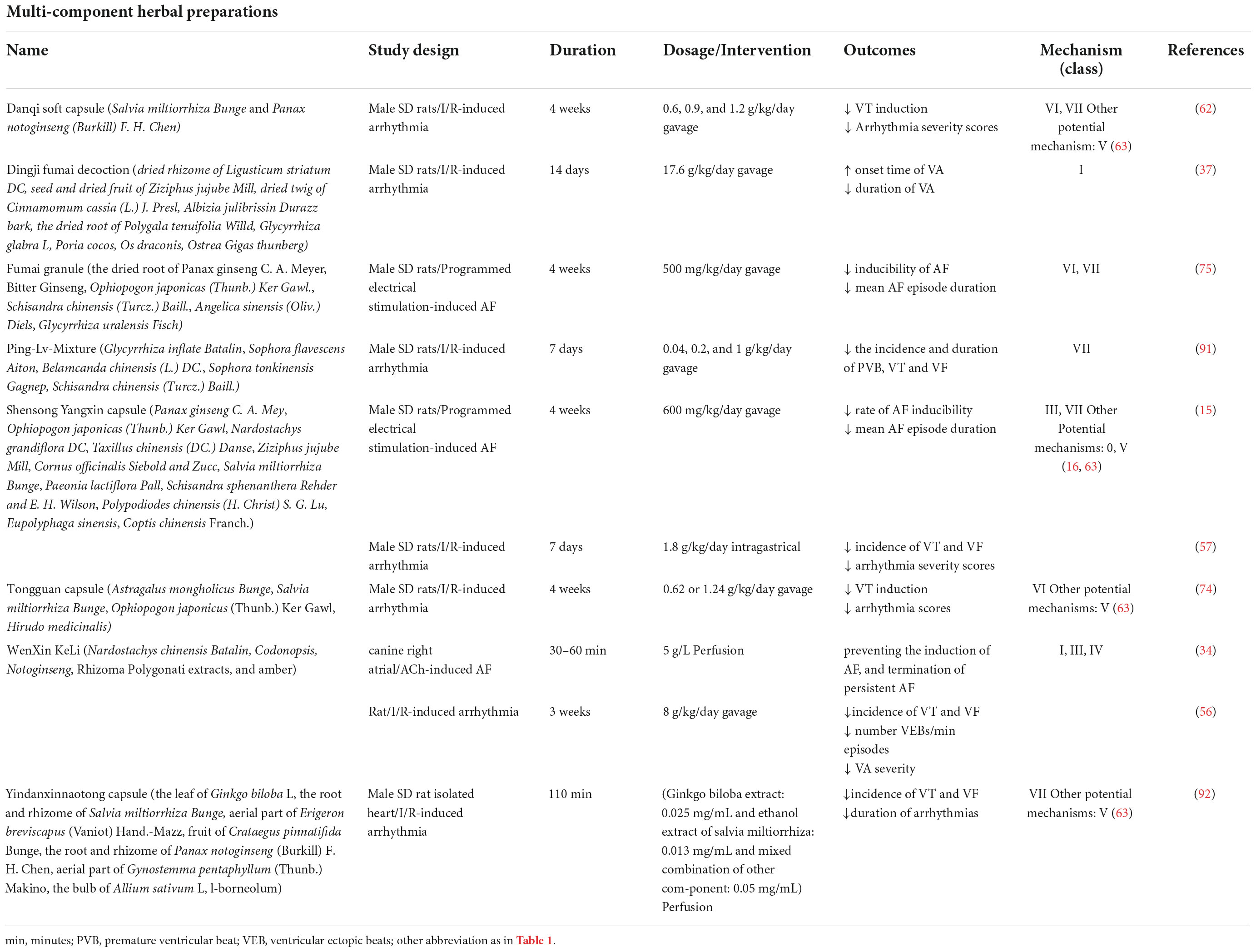
Table 2. Multi-component herbal preparations with antiarrhythmic effects and their mechanism of action.
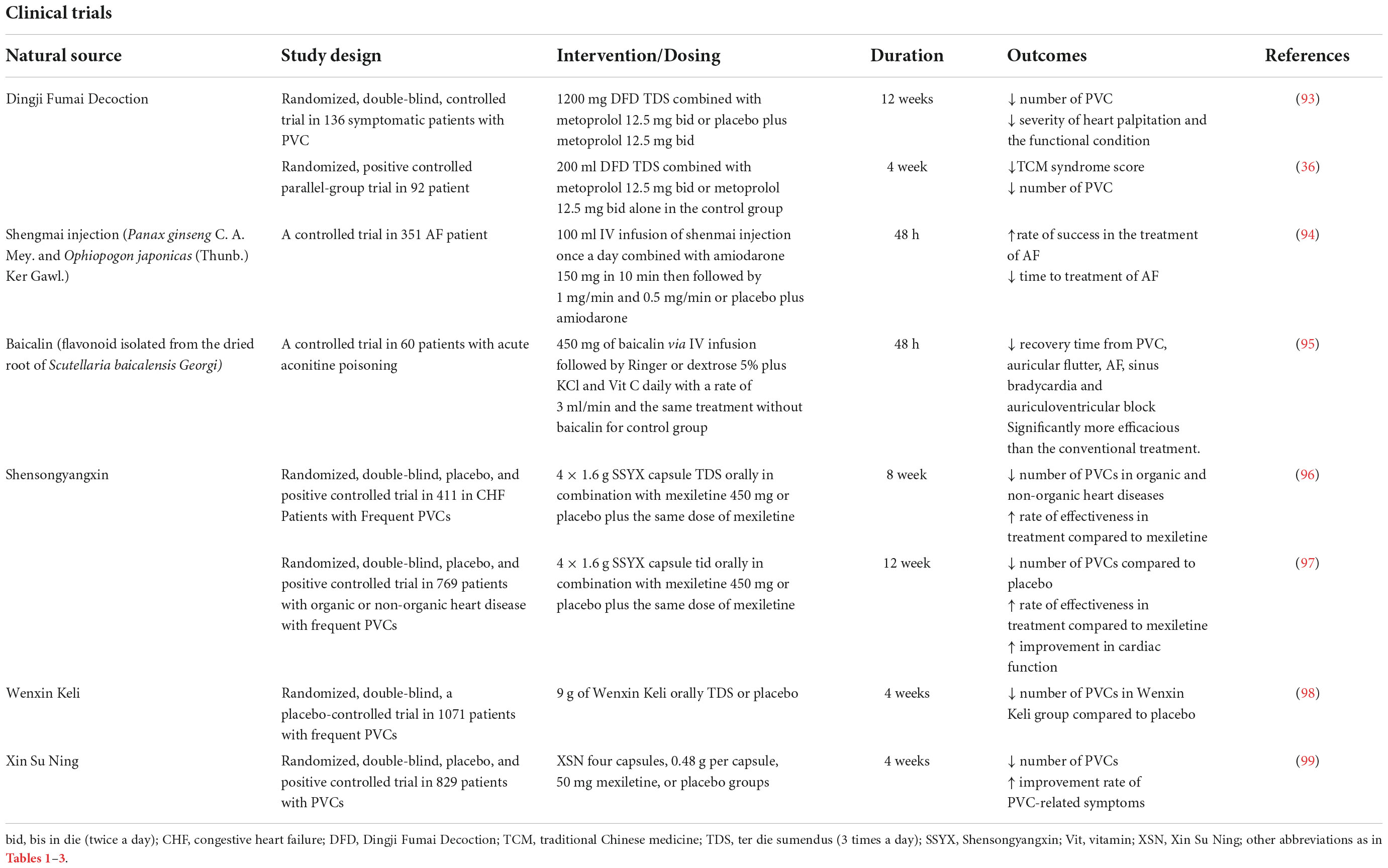
Table 4. Summary of the clinical studies investigating the efficacy and safety of phytochemicals or multi-component herbal preparations on cardiac arrhythmias.
Figure 2A presents the quality assessment of the preclinical studies based on SYRCLE’s RoB tool. Twenty-eight studies (53.0%) mentioned “random” in sequence generation, but none of the studies explained the randomization method. The bias risk assessment results of “baseline characteristics” in six studies were unclear because there was no information about animal samples in the experimental and control groups. No study reported the “allocation concealment” clearly. In the experiment implementation stage, almost all of the studies did not mention “random housing to animals,” “blinding in the study design or assessments of outcomes,” and “random selection of animals for outcome assessment.” All studies reported low bias in “addressing the incomplete outcome data,” “selective outcome reporting,” and other biases. Figure 2B presents the quality assessments of the clinical studies based on the Jadad score. Five studies involved a double-blind process and were judged to be of high quality (Jadad score = 5). Two studies were not randomized clinical trials and were judged to be of poor quality (Jaded score = 1). One study with a Jaded score of 3 had moderate quality.
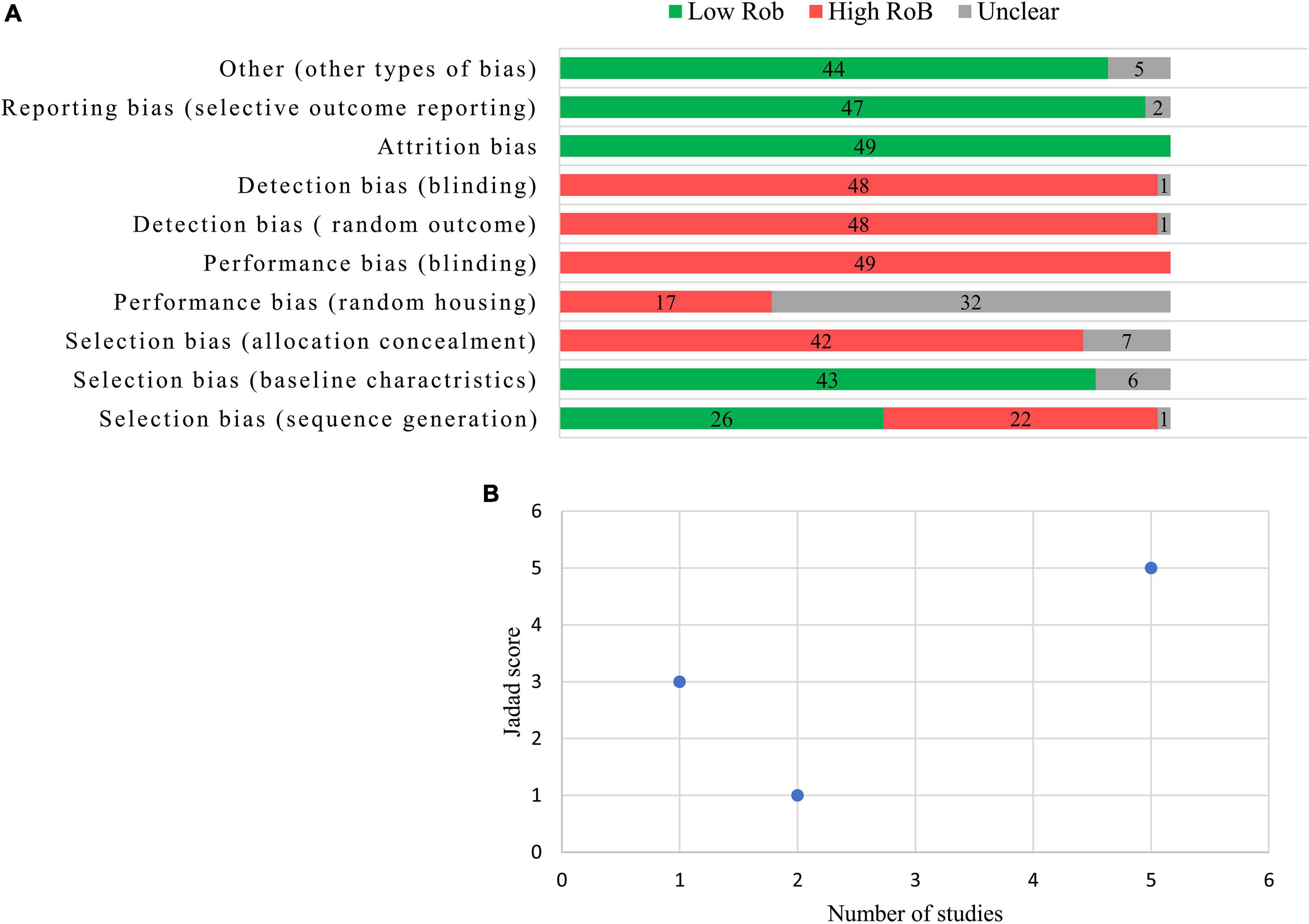
Figure 2. Results from the risk of bias assessment according to the Systematic Review Centre for Laboratory Animal Experimentation (SYRCLE) authors’ judgment about each risk of bias item (A) and the quality assessment of clinical trials according to the Jadad score (B).
Antiarrhythmic mechanisms of medicinal plants: preclinical studies
Medicinal plants found in our review exerted their antiarrhythmic effects via different mechanisms. Here, we discussed the experimental studies in which antiarrhythmic effects of plant extract, phytochemicals, and multi-component herbal preparations have been investigated alongside their underlying antiarrhythmic mechanisms. Several other plants with antiarrhythmic effects whose probable mechanisms of action have been explored in another independent study on physiologic models were not discussed in the body of the article and merely summarized in the Supplementary Tables 1–3. Figures 3, 4 illustrate a schema of the mechanisms affected by such compounds to manage cardiac arrhythmias.
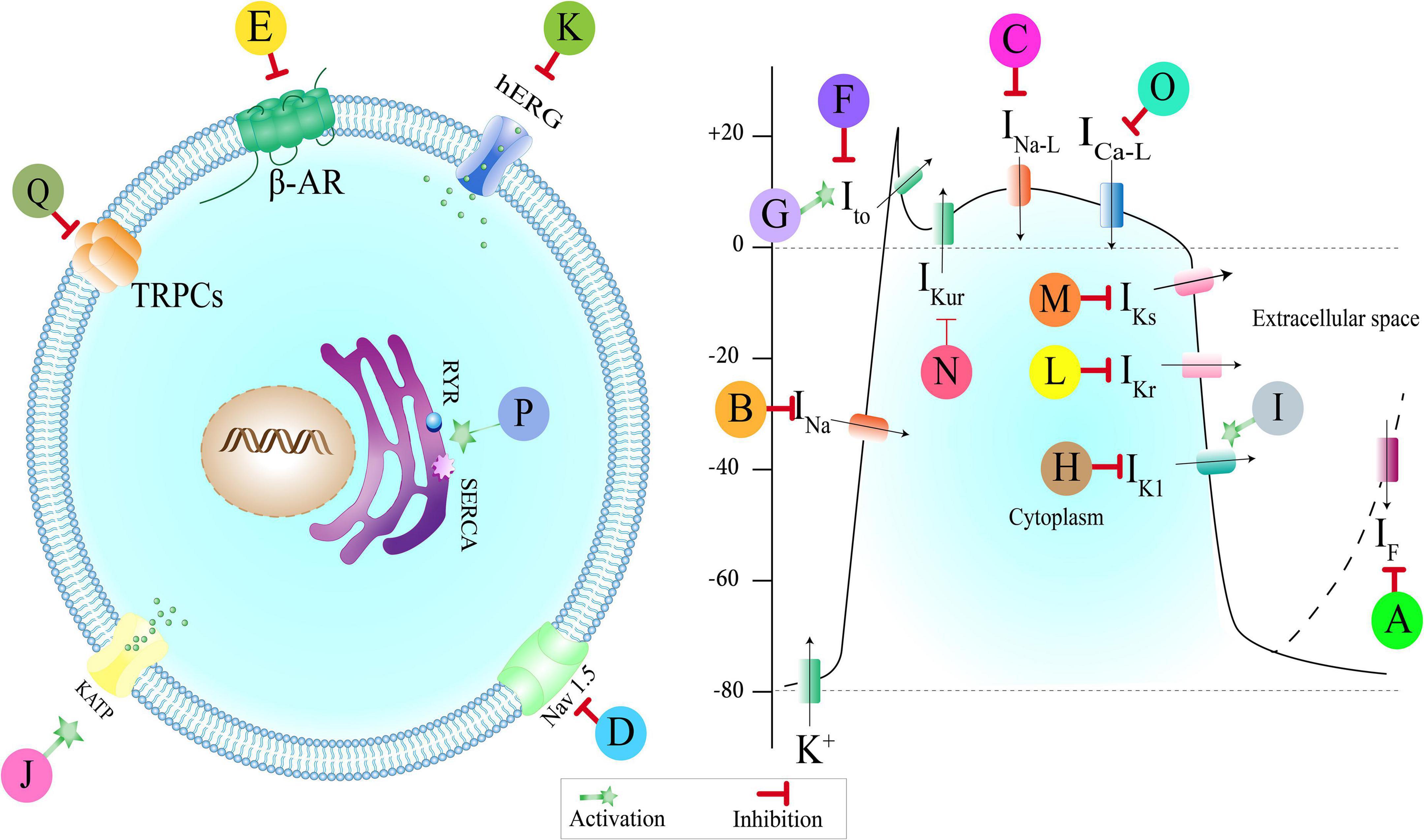
Figure 3. Medicinal plants, multi-component herbal preparations, and phytochemicals with an antiarrhythmic effect based on their effect on ionic channels. Some of them have more than one mechanism. Class (0): A: Shensong Yangxin capsule, Ginkgo biloba. Class (I): B: Andrographolide, Oxymatrine, Liriodenine, Arctigenin, Resveratrol, C: Wenxin Keli, Curcumin, Barbaloin, D: Dingji Fumai decoction, Xin Su Ning. Class (II): E: olea europaea. Class (III): F: Wenxin Keli, Shensong Yangxin capsule, Allicin, Resveratrol, Cinnamophilin, Acacetin, G: Oxymatrine, H: Berberin, Shensong Yangxin capsule, Oxymatrine, I: Tanshinone IIa, Rhodiola spp., J: Sasanquasaponin, Total flavonoids from Hypericum attenuatum, K: Xin Su Ning, L: curcumin, M: Resveratrol, N: Acacetin. Class (IV): O: Wenxin Keli, Allicin, Total flavonoids from Hypericum attenuatum, flavonoid extract of Viscum coloratum, Ginsenoside Rb1, Oxymatrine, Curcumin, Arctigenin, Cinnamophilin, Barbaloin, Resveratrol, Rhodiola spp., P: Rhodiola spp. Class (V): Q: Salvianolic acid-B. IF: Pacemaker current, INa: Sodium currents, INa–L: Late sodium currents, Nav: voltage-gated sodium channel, Ito: Transient outward current, IK1: Inward rectifier current, KATP: ATP activated K+ channels, hERG: Human Ether-a-go-go-related Gene (hERG) Potassium Channel, IKr: Rapid delayed rectifier current, IKs: Slow delayed rectifier current, IKur: Ultrarapid delayed rectifier currents, ICa–L: L-type-calcium current, RYR: Ryanodine receptors, SERCA: Sarcoendoplasmic reticulum calcium transport ATPase, TRPCs: Transient receptor potential canonical channels, β-AR: Beta-adrenergic receptor.
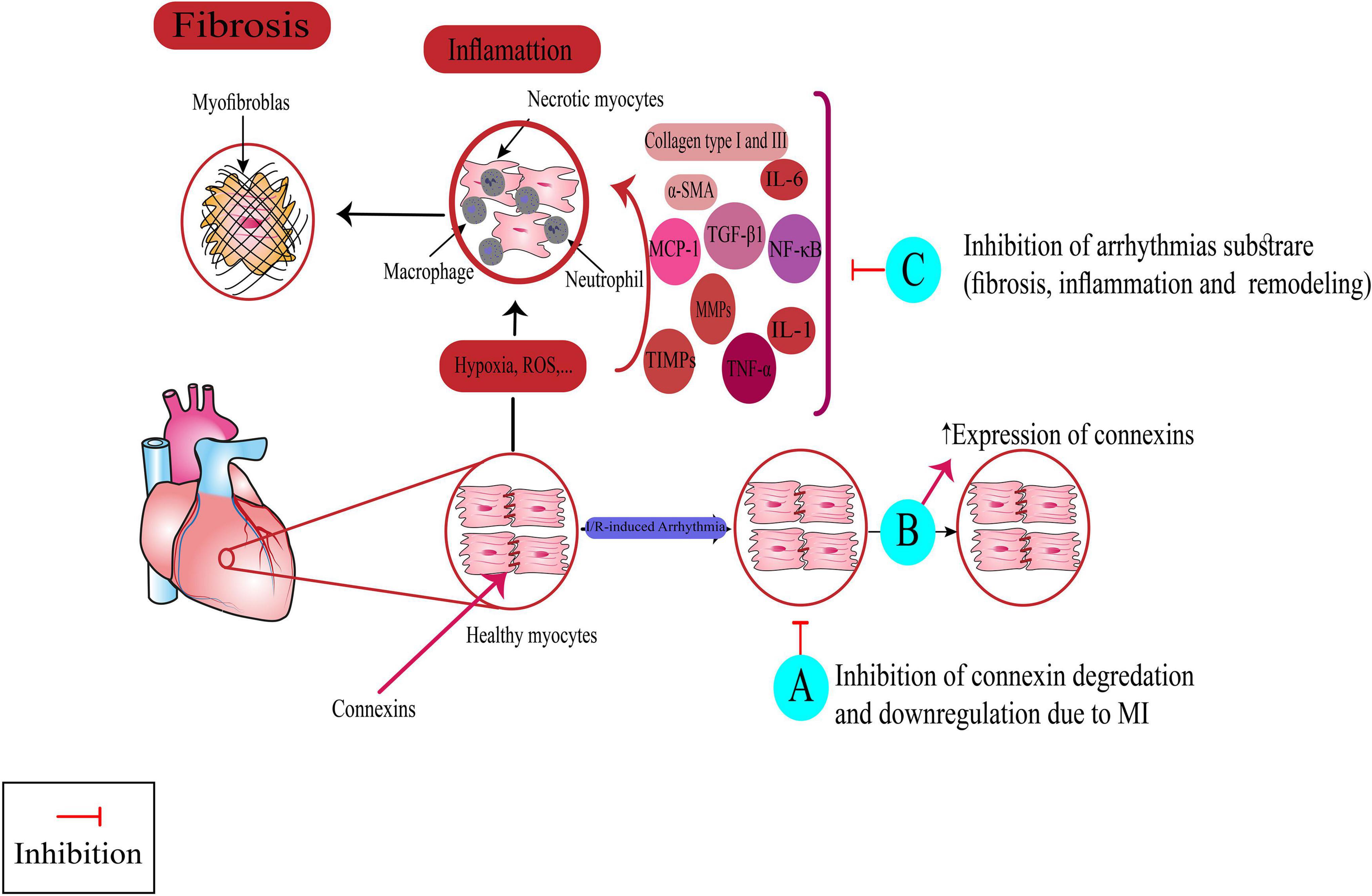
Figure 4. Targeting non-ionic mechanism for suppressing arrhythmias by medicinal plants, multi-component herbal preparations, and phytochemicals. Some of them have more than one mechanism. Class (VI): A: Nardostachys chinensis, Linalool, B: Danqi soft capsule, Fumai granule, Tongguan capsule, Grape seed proanthocyanidin. Class (VII): C: Arnebiae euchroma, Rhodiola spp., Danqi soft capsule, Ping-Lv-Mixture, Shensong Yangxin capsule, Fumai granule, Bilberry Anthocyanins, Hesperidin, Troxerutin, Magnolol, Panax notoginseng saponins, Arctigenin, Yindanxinnaotong capsule. Nrf2: Nuclear factor erythroid 2-related factor 2, TGF-β1: transforming growth factor-beta1, MCP-1: monocyte chemoattractant protein-1, TNF-α: tumor necrosis factor-α: TNF-α, TIMPs: Tissue inhibitors of metalloproteinases, MMPs: Matrix metalloproteinases, IL: interleukin, α-SMA: Smooth muscle alpha-actin, NF-κB: nuclear factor- κB, I/R: Ischemia-reperfusion, ROS: reactive oxygen species.
Class (0): HCN channel-mediated pacemaker current blocker
The hyperpolarization-activated cyclic nucleotide-gated (HCN) channel family is composed of transmembrane channels with a single pore, among which HCN2 and HCN4 are the predominant isoforms of the heart. The HCN channel-mediated pacemaker current (If) is essential for the generation and conduction of cardiac impulses. They are highly expressed in the sinoatrial node (SAN), and their inhibition decreases the spontaneous activity (automaticity) of the sinoatrial node (SAN) by affecting phase 4 of depolarization, thereby controlling the increased heart rate (7, 10). Although the expression level of HCN channels is very low in normal myocardium, it seems to be increased in pathologic conditions such as ischemic cardiomyopathy and congestive heart failure. In such pathologic settings, If may be responsible for inappropriate automaticity in the ventricular cardiomyocytes, leading to ventricular premature complexes that may generate ventricular arrhythmias. Therefore, it appears that inhibiting this current with medications in this class, like ivabradine, can diminish arrhythmia in such pathologic conditions, as preclinical studies have indicated. In the context of ischemia, the antiarrhythmic effects may be the result of reduced heart rate, resulting in energy preservation and mitigation of ischemia-induced electrophysiological consequences. In the setting of congestive heart failure, the antiarrhythmic effects may be attributable to the inhibition of HCN channels, which consequently reduces the proarrhythmic pathological automaticity in ventricular cardiomyocytes (11, 12).
Regarding this class of AADs, our review found no study that, besides the antiarrhythmic effects of the plant, also examined concurrently such mechanism of action for that. However, we found a number of plant extracts and multi-component herbal preparations, as described below and summarized in Supplementary Table 1, whose inhibitory effects on If have been identified in an in vitro model of pacemaker cells in some studies, while their antiarrhythmic effects have been evaluated in some other separate studies.
Ginkgo biloba L. extract and one of its main active ingredients, namely bilobalide, were shown to have antiarrhythmic effects in rat models of ischemia-reperfusion (I/R) injury (13, 14). One study by Satoh et al. (15) indicated that this plant diminishes the pacemaker activity in SAN, thereby exerting a negative chronotropic effect in a concentration-dependent manner. This study also found that modulating multiple currents in SAN, including but not limited to If, is probably responsible for such an effect. Thus, blockade of If might be one of the antiarrhythmic mechanisms for this plant, probably exerting through reducing heart rate, which leads to energy preservation and a reduction in ischemia-induced electrophysiological consequences. However, further studies in experimental models of cardiac arrhythmias are warranted to know the exact effect of this plant on If in pathologic conditions.
Shensong Yangxin (SSYX) capsule is a traditional Chinese medicine (TCM) consisting of 12 medicinal plants, approved clinically for its antiarrhythmic effects in China through affecting multiple channels in cardiac pacemaker cells (16). One study by Sun et al. (17) found that SSYX can inhibit the If via reducing the expression of HCN channel genes. Also, our literature search found that one of the active ingredients in the SSYX capsule, namely berberine, can act as an If blocker, which might contribute to its antiarrhythmic efficacy (18). Thus, we can expect that the antiarrhythmic effects of SSYX might be, at least in part, due to the inhibition of If, similar to that seen in class (0) AADs. However, there is no study that has examined this mechanism in an arrhythmia model, and this issue should be considered in future studies.
Class (I): Voltage-gated Na+ channel blockers
Voltage-gated Na+ (Nav) channels are transmembrane proteins composed of a pore-forming subunit and auxiliary subunits that are extensively expressed throughout the atrial, Purkinje conducting, and ventricular cardiomyocytes, where they are responsible for the rapid upstroke of the cardiac action potential (AP) and the rapid conduction of impulses through cardiac tissue. Consequently, dysfunction in Nav channel current (INa) plays an important role in the formation of cardiac arrhythmias (19, 20). Nav channel blockers comprise the class (I) AADs, which itself is divided into four subclasses (Ia, Ib, Ic, and Id), all of which generally influence the upstroke and duration of AP (7). Below, we described several phytochemicals and multi-component herbal preparations whose antiarrhythmic effects are exerted through inhibiting Nav channels like the action of class (I) AADs.
Andrographolide is a labdane diterpenoid isolated from the leaves and roots of Andrographis paniculata (Burm.f.) Nees with several cardioprotective effects. Zeng et al. (21) conducted an in vivo experiment with an aconitine-induced arrhythmia animal model as well as in vitro assays, using rabbit left ventricular and left atrial myocytes to confirm their hypothesis that andrographolide has antiarrhythmic effects through modulation of cardiac ion channels. They showed that 20 μM andrographolide suppressed the aconitine-induced arrhythmias in the in vivo rabbit model. In the in vitro analyses, Andrographolide shifted the INa and ICa inactivation curves toward negative membrane potential and expedited the inactivation process, thereby inhibiting the entry of Na+ and Ca2+ into the cardiomyocyte. In addition, an electrophysiological evaluation showed a significant decrease in AP duration and maximal upstroke velocity (Vmax) of phase 0 depolarizarion as well as the cellular mechanisms underlying cardiac arrhythmias, including delayed afterdepolarizations (DADs)-induced triggered activities induced by isoproterenol and high Ca2+ administration. Thus, the authors indicated that andrographolide suppressed aconitine-induced arrhythmias via the inhibition of INa and ICa, similar to that seen in class (I) and class (IV) of AADs.
Oxymatrine is an alkaloid compound isolated from Sophora flavescens Aiton roots and demonstrated to have cardioprotective effects. Runtao et al. (22) investigated the effect of oxymatrine on aconitine-induced and coronary artery ligation-induced arrhythmias in rats and its fundamental mechanisms. They indicated that oxymatrine (3, 10, and 30 mg.kg–1) could prevent these arrhythmias in a concentration-dependent manner. In in vitro analysis of isolated rat cardiomyocytes, oxymatrine significantly inhibited INa and ICa, similar to the action of class (I) and class (IV) of AADs, which could explain the observed antiarrhythmic effects in this study.
Liriodenine is an aporphine-type alkaloid extracted from Fissistigma glaucescens (Hance) Merr and shown to have beneficial effects on the cardiovascular system (23). The antiarrhythmic potential of liriodenine was investigated by Chang et al. (24), who assessed the effect of this active compound in an ex vivo model of I/R-induced arrhythmias in the Langendorff perfused rat heart. They found that liriodenine with a half maximal effective concentration (EC50) of 0.3 μM suppressed the I/R-induced arrhythmias in a dose-dependent manner. Additionally, in a concentration-dependent manner, liriodenine increased AP duration and reduced the Vmax of phase 0 depolarization in isolated ventricular myocytes from rats. They also showed in the whole-cell patch-clamp recording that such electrophysiological effects were due to the inhibition of INa and transient outward K+ current (Ito), respectively. Such effects can be likened to the actions of class (I) and class (III) of AADs, respectively.
Curcumin, a flavonoid found in Curcuma longa L rhizomes, has shown to have a wide range of cardioprotective effects (25). Song et al. (26) evaluated the antiarrhythmic effects of curcumin (30 mM) and indicated its ability to reduce the incidence and duration of I/R-induced ventricular tachyarrhythmias (VT) and ventricular fibrillation (VF) in Langendorff-perfused rabbit hearts. In in vitro analysis, curcumin reduced the AP duration and suppressed early afterdepolarization (EAD) and Ca2+-induced DADs through inhibiting multiple ion channel currents, specifically late Na+ channel current (INa–L) and, to some extent, voltage-gated L-type Ca2+current (ICa–L) and transient Na+ current (INa–T). Moreover, in this study, curcumin inhibited rapid delayed rectifier K+ current (IKr), which although such inhibition can cause the AP duration to lengthen, because of concurrent inhibition of INa–L and ICa–L, curcumin shortened the AP duration altogether. Thus, curcumin exerts its antiarrhythmic effects like the action of class (I), class (III), and class (IV) of AADs.
Arctigenin (ATG) is a lignan isolated from the dried fruit of Arctium lappa L., shown to be effective in combating cardiac arrhythmias (27, 28). Zhao et al. (27) showed that 100 μM ATG markedly delayed the onset of aconitine-induced arrhythmia in the rat model. In the in vitro analysis, ATG shortened the AP duration, increased the Ito, and produced inhibitory effects on INa and ICa–L. Thus, the authors suggested that the antiarrhythmic effect of ATG may be mediated by modulating multiple ion channels.
Cinnamophilin, a lignan extracted from Cinnamomum philippinense (Merr.) C. E. Chang was found to have protective effects against cardiac arrhythmias. Su et al. (29) demonstrated that administration of cinnamophilin (1 to 10 μM) reversed VTs induced by I/R into the sinus rhythm in isolated heart rat preparations. They also found that such an effect may be partly related to the inhibition of INa, leading to a reduction in Vmax, similar to the action of class (I) AADs. Cinnamophilin was also found to have inhibitory effects on Ito and ICa, which may exert antiarrhythmic effects like the action of class (III) and class (IV) of AADs. Ito inhibition leads to an increase in AP duration, which may generate proarrhythmic effects similar to those found with class (III) AADs. However, concurrent inhibition of the ICa by cinnamophilin may reduce this prolongation, resulting in fewer proarrhythmic effects.
Barbaloin is an anthraquinone-type phenolic compound with multiple biological activities, extracted from Aloe spp. Using isolated rabbit ventricular myocytes as an in vitro animal model, Cao et al. (30) discovered that after the treatment with barbaloin (200 μM), the onset time of aconitine-induced VT and VF was prolonged, and their incidence was decreased. The cellular electrophysiological evaluation showed that barbaloin (100 and 200 μM) could markedly shorten the AP duration and terminate Anemonia sulcata toxin II-induced EADs and Ca2+-induced DADs in isolated myocytes in a dose-dependent manner. These electrophysiological changes, together with inhibition of INa–L and ICa–L discovered in this study, may explain the antiarrhythmic effects of barbaloin, which are similar to the action of class (I) and class (IV) of AADs.
Resveratrol is a phytoalexin isolated from some plants such as Vitis and Vaccinium species. Several animal studies have proved the protective effects of resveratrol against cardiac arrhythmias (31–36). Among them, Chen et al. (36) evaluated the antiarrhythmic effects of resveratrol and its ability to change INa in a rat model of isolated myocytes. In this study, resveratrol eliminated the I/R-induced ventricular arrhythmias, reduced Vmax and prolonged the AP duration and effective refractory period (ERP), all of which were shown to be related to the inhibition of INa, Ito and sustained outward K+ currents (Iss) in cellular electrophysiological recordings. Such effects are similar to the actions of class (I) and class III of AADs. Resveratrol was also shown to have a partial inhibitory effect on ICa–L, which can explain the prolonged atrioventricular (AV) nodal conduction observed in this study.
Wenxin Keli (WXKL) is a TCM composed of a mixture of five natural compounds (37). Burashnikov et al. (37) investigated the antiarrhythmic effects of WXKL in an ex vivo model of isolated canine arterially perfused right atrial preparations. They found that WXKL can effectively suppress acetylcholine (ACh)-induced atrial fibrillation (AF) and prevent its induction via affecting the electrophysiological properties of Nav channels. In this study, WXKL increased the ERP, induced rate-dependent post-repolarization refractoriness, and decreased Vmax, predominantly in the atria. These atrial-selective properties are similar to those seen in Ranolazine, an antiarrhythmic drug belonging to class Id, shown to be effective in the management of AF and INa–L-related tachyarrhythmias (7, 38).
Dingji Fumai decoction (DFD), a herbal mixture composed of nine different herbs, has been traditionally prescribed for treating palpitation (39). Liang et al. (40) investigated the effect of DFD on BaCl2-induced and aconitine-induced arrhythmias in rats. They found that pretreatment with DFD (17.6 g.kg–1. once a day) for 2 weeks could reduce the duration of ventricular arrhythmias and delay their initiation mainly through four mechanisms: (1) dose-dependent inhibition of Nav1.5 current, (2) modulating Na+-K+-ATPase activity, (3) modulating gap junctions, and (4) antioxidant properties; The former is similar to that effect exerted by class (I) AADs (7).
Class (II): Autonomic inhibitors and activators
Autonomic imbalance is known as a key player in the development of CVDs. The parasympathetic and sympathetic branches of the autonomic nervous system exert various effects on the human myocardium through the activation of different G protein-coupled receptors that mediate the interplay between autonomic nervous system function and the cardiovascular system and can be targeted by multiple drugs with the purpose of preventing and treating CVDs (41). According to the modernized classification of AADs, autonomic activators and inhibitors belong to class II, and are categorized into five subclasses (IIa, IIb, IIc, IId, and IIe) (7).
Olea europaea L., commonly known as olive, has demonstrated antiarrhythmic effects by affecting various ion channels (42). In one study, Somova et al. (42) showed that two triterpenoids, namely oleanolic acid and ursolic acid, isolated from O. europaea leaves, can behave as β-adrenergic antagonists in three models of CaCl2, adrenaline, and I/R—induced arrhythmias. This is similar to that seen in class IIa, where the non-selective β- and selective β1-adrenergic receptor blockers inhibit adrenergically induced Gs protein-mediated effects of increased adenylyl kinase activity, thereby suppressing SAN pacing rate (7).
Class (III): K+ channel blockers and openers
In cardiac tissue, K+ channels include inwardly rectifying K+ channels (Kir), transient outward K+ channels, delayed outward rectifying K+ channels, Ca2+-activated K+ channels, and two-pore domain K+ channels. They are primarily responsible for maintaining the resting potential, regulating electrical excitation, forming the peak of the AP, and repolarizing the cell (43). Any dysfunction in these channels may result in cardiac arrhythmia. Class III of AADs, including K+ channel blockers and openers, suppress arrhythmias by influencing AP phase 3 repolarization and ERP. This class is further subdivided into three subclasses comprising voltage-gated K+ (Kv) channel blockers (IIIa), metabolically dependent K+ channel openers (IIIb), and transmitter dependent K+ channel blockers (IIIc). Below are several plants or their active ingredients that have been found to have antiarrhythmic efficacy similar to this class of AADs in experimental studies (7).
Hypericum attenuatum Fisch. ex Choisy, commonly known as St. John’s wort, has been shown to have the potential to combat cardiac arrhythmias. In one study, the rats were treated with total flavonoids extracted from H. attenuatum (100 mg.kg–1.day–1) for 7 days, and then the I/R model was carried out to induce arrhythmias. They also evaluated the cellular mechanism behind the probable cardioprotective effects of this extract. Results showed that the flavonoid extract could prevent I/R-induced arrhythmias through two mechanisms: (1) increasing the expression of the ATP-sensitive K+ (KATP) channels subunit Kir 6.1, leading to increase their open probability, similar to the action seen in subclass IIIb of AADs, (2) reducing the expression of CaL-α1C, the most important subunit of L-type Ca2+ channels, which in turn leads to inhibiting the Ca+2 entry, similar to the class IV of AADs (44).
Rhodiola crenulata (Hook.f. and Thomson) H. Ohba, a Tibetan medicinal plant, has been found to be effective in suppressing cardiac arrhythmias (45, 46). In one study, Liu et al. (46) evaluated the antiarrhythmic effects of this plant in a rabbit model of heart failure (HF). The APD and ERP were found to be greater in the HF group than in the control group once AF was induced in both groups. This finding is consistent with previous evidence indicating that anatomical and structural remodeling in HF causes an increase in the ERP and that such an electrophysiological change is linked to the EAD- and DAD-induced triggered activity, thereby possibly initiating AF (47–49) found that animals treated with R. crenulata (270 mg.kg–1.day–1 orally) for 2 weeks had lower AF inducibility and shorter ERP and AP duration than the HF group, which received no treatment. Molecular analysis by this study showed the increased mRNA expression of Kv1.5, contributing to ultra-rapid rectifier K+ current (IKur) following treatment with R. crenulata, an action which may be likened to AADs with K+ channel activating effects. Moreover, R. crenulata increased the expression of Ca2+ -ATPase pump which restores the reduced Ca+2 concentration in sarcoplasmic reticulum in HF model, that might be likened to the action of subclass IVc of AADs, known Ca2+ channel activators Liu et al. (46).
Acacetin is a natural flavone extracted from Saussurea tridactyla Sch.Bip. ex Hook.f. Intraduodenal administration of this compound at doses of 2.5, 5, and 10 mg.kg–1 could remarkedly prevent AF in dogs through atrial selectively inhibition of IKur, Ito and acetylcholine-activated K+ current (IKACh), represented by atrial ERP prolongation and no alteration in the corrected QT interval (50).
Berberine is a natural alkaloid found in some plants, such as Berberis spp. and Coptis chinensis Franch., shown to have the potential to suppress cardiac arrhythmias in several experimental studies (51, 52). The antiarrhythmic effects of berberine on the streptozotocin-induced model of type 2 diabetes mellitus (T2DM) in ischemic conditions have been proved by Wang and colleagues (53). They tested the antiarrhythmic effects of berberine (180 mg.kg–1 intragastrically for 2 weeks) in four groups: group 1: normal control rats; group 2: normal rats with myocardial infarction (MI); group 3: T2DM rats associated with MI; and group 4: T2DM rats associated with MI treated with Berberine. Berberine significantly restored the protein expression of Kir 2.1, which is impaired in diabetic and ischemic conditions, compared to untreated groups, leading to a shortening of AP duration and subsequently preventing the triggered activity in ischemic tissues. This effect can be likened to the action of subclass IIIb AADs, specifically inward rectifier K+ current (IK1) agonists, which speeds up the late phase 3 AP repolarization and stabilizes phase 4 diastolic resting potentials (7). Some mechanistic studies further studied the pharmacological targets and electrophysiological effects of berberine on isolated cardiac myocytes and found its inhibitory effects on IK1, slow delayed rectifier K+ current (IKs), and IKr, which subsequently could prolong the repolarization phase of AP duration and ERP, similar to the action of subclass IIIa AADs (54–56). However, these studies have been designed in non-pathologic conditions, so further studies are needed to assess these mechanisms alongside the outcomes related to arrhythmias.
Oxymatrine and its metabolites like matrine are isoquinoline alkaloids found in Sophora flavescens root, shown to be effective in suppressing arrhythmias (22, 57). Yong-Gang et al. (57) evaluated the effects of oxymatrine on coronary ligation-induced arrhythmias in Wistar rats. They discovered that intravenous (IV) injection of oxymatrine (10 and 20 mg.kg–1) prior to occlusion could decrease the VT duration, delay the onset of VT, and reduce the severity score of arrhythmias. It was shown that such protective effects are mediated by inhibition of IK1, reduction of ICa–L, and enhancement of Ito. The IK1 inhibition by this phytochemical is likened to the action of subclass IIIa AADs; However, the AP duration was shortened following oxymatrine injection, and this is contrary to what is expected from subclass IIIa AADs and may be due to simultaneous effects on ICa–L and Ito. Another study by Li et al. (51) explored the antiarrhythmic effects of matrine in a rat model of MI and confirmed the results obtained in the Gang et al. study. They found that long-term oral administration of matrine could reduce the rate of ligation-induced arrhythmias and mortality through restoring Ito, IK1, and ICa impaired by MI induction, represented electrophysiologically by shortening the AP duration.
Sasanquasaponin is a triterpenoid isolated from Camellia oleifera Abel seeds, and its antiarrhythmic activity has been scarcely evaluated. Lai et al. (58) designed one study under both in vitro and in vivo conditions and showed that IV injection of sasanquasaponin (0.1, 0.2, and 0.4 mg.kg–1), 10 min prior to ischemia significantly reduced the incidence of I/R-induced VT and VF, mediated by reducing AP duration, probably through the activation of KATP channels, similar to that seen in subclass IIIb AADs.
Tanshinone IIA is a diterpene constituent isolated from the roots and rhizomes of Salvia miltiorrhiza Bunge and found to be effective in suppressing arrhythmias. Shan et al. (59) found that pretreatment of rats with tanshinone IIA (10 mg.kg–1.day–1) for 7 days before left anterior descending artery ligation could significantly reduce the duration, incidence, and severity score of arrhythmias such as VT and VF in a rat model of MI. In this study, tanshinone IIA significantly restored the protein expression of Kir2.1, which was impaired due to the overexpression of microRNA-1 during MI. Tanshinone IIA also improved IK1 which may be as a result of increased Kir 2.1 expression. Increased IK1 could preserve resting membrane potential in rats that received tanshinone IIA, which is probably the main underlying mechanism for protecting against ventricular arrhythmias (59). Moreover, previous evidence shows that the increased IK1 could decrease AP duration, which subsequently prevents the EAD- and DAD-induced triggered activity in ischemic tissues. Thus, tanshinone IIA seems to exert its antiarrhythmic activity in ischemic conditions by increasing IK1, resulting in preserving resting membrane potential and, to some extent, preventing prolongation of AP duration and subsequent triggered activity. Such effects are similar to those exerted by IK1 agonists in subclass III b AADs (7).
Allicin is an organosulfur compound with multiple cardiometabolic effects, isolated from Allium sativum L. An experimental study by Huang et al. (60) found that 28-day pretreatment with allicin could delay the onset time of BaCl2-induced arrhythmias and decrease the arrhythmia score. Their results showed that such beneficial effects are produced by reducing AP duration through two main mechanisms, including enhancement of IK1 and inhibition of ICa–L. As previously explained, the former mechanism is likened to the action of IK1 agonists in subclass III b AADs (7). One other mechanistic study found the inhibitory effects of allicin on Ito in isolated ventricular myocytes similar to the action of subclass IIIa; however, this study has not evaluated the arrhythmia outcomes (61), and this issue should be clarified in future studies.
We discussed the antiarrhythmic effects of some active ingredients, including liriodenine, curcumin, cinnamophilin, and resveratrol, through inhibiting Nav channels in previous subsections. Studies have shown that these phytochemicals also behave like class III AADs, as they can modulate the K+ channel currents and affect AP duration, thereby protecting the heart against I/R-induced arrhythmias (24, 26, 29, 32, 33, 36). Note that the inhibition of Ca2+ channels by curcumin, cinnamophilin, and resveratrol might alleviate the prolonged AP duration exerted by inhibiting K+ channels.
The antiarrhythmic effects of WXKL through inhibiting Na+ current were discussed in previous subsections. One study looked at the other mechanisms of antiarrhythmic action of WXKL and showed that 3-week administration of WXKL (8 g.kg–1.day–1) before ischemia induction could significantly reduce the incidence and number of VT, VF, and ventricular ectopic beats in a rat model of I/R-induced injury via inhibiting the ICa–L and Ito (62). The latter is similar to the action subclass IIIa AADs.
As we mentioned above, the SSYX capsule, as a mixture formulation of herbal drugs, has been traditionally used for treating cardiac arrhythmias in China by targeting multiple ion channels in the heart (16). In one study, Zhao et al. (63) revealed that pretreatment of rats with SSYX capsule (1.8 g.kg–1.day–1) for 7 days could significantly reduce the incidence of VT, VF, and arrhythmias severity scores and delay the initiation of coronary occlusion-induced arrhythmias. In this study, SSYX significantly prolonged AP and slowed cardiac repolarization by inhibiting IK1 and Ito, which subsequently changed the myocardial refractoriness and conductivity and exerted a therapeutic effect on ischemic arrhythmias. Such an effect is similar to that exerted by subclass IIIa, which has been shown to be effective in combating reentry-induced cardiac arrhythmias.
Class (IV): Ca2+ handling modulators
The main Ca2+ channels in cardiac cells are transmembrane L-type and T-type, as well as intracellular channels. Calcium channels in cardiac cells are responsible for regulating cardiac pacemaking, AV conduction, and heart rate determination (64). Ca2+ handling modulators are known as Class IV antiarrhythmics. According to modernized classification, this class of AADs is divided into five subclasses (IVa–IVe), the first two of which, surface membrane Ca2+ channel blockers (IVa) and intracellular Ca2+ channel blockers (IVb), have been clinically approved for the treatment of certain types of cardiac arrhythmias. The agents in the IVa subclass are the most commonly used drugs. They are primarily used to treat supraventricular arrhythmias caused by reentry or triggered activity by reducing AV nodal conduction (7).
Panax notoginseng (Burkill) F. H. Chen is a Chinese medicinal plant that contains multiple saponins shown to be protective against CVDs (65). Guan et al. (66) tested the antiarrhythmic effects and mechanism of action of ginsenoside-Rb1, a component of P. notoginseng saponins, through in vivo and in vitro investigations. They found that Rb1 (30 and 50 mg.Kg–1) reduced BaCl2-induced VTs in the rat model and CaCl2-ACh-induced AF and chloroform-induced VF in the mouse model. In vitro study on heart cells showed that Rb1 restored the isoprenaline-induced increased intracellular Ca2+ concentration by inhibiting its entry through Ca2+ channels in a concentration-dependent manner (66).
As mentioned in the previous subsections, WXKL, allicin, and total flavonoid from Hypericum perforatum have protective effects against arrhythmias induced by the I/R model or BaCl2 injection. Regarding WXKL, in vitro investigations of isolated ventricular myocytes showed that one other mechanism responsible for such beneficial effects is the inhibition of ICa–L (62) similar to that exerted by L-type voltage-gated Ca2+ (Cav) channel blockers in subclass IVa AADs, such as verapamil and diltiazem (7). Regarding Allicin, as we earlier stated, Huang et al. (60) study proved its antiarrhythmic effects associated with shortening of AP duration, mechanistically caused by ICa–L inhibition, resemble the action of subclass IVa AADs. In the case of total flavonoid from H. attenuatum, Ma et al. (44) found that 7-day pretreatment of rats with this extract could reduce I/R-induced arrhythmias via a mechanism similar to the action of class III and class IV AADs.
Flavonoid extract from Viscum coloratum (Kom.) Nakai is thought to have cardioprotective effects. Wen-Feng et al. (67) investigated the antiarrhythmic effect of V. coloratum on aconitine-induced arrhythmias in rats. They found that pretreatment of rats with an IV injection of V. coloratum could significantly alleviate the susceptibility to premature ventricular contraction (PVC), VT, and VF. In this study, V. coloratum significantly produced an inhibitory effect on ICa–L and shortened the AP duration of ventricular myocytes, similar to that seen in subclass IVa AADs.
As discussed in previous subsections, oxymatrine and its metabolites like matrine found in S. flavescens root have a protective role against ischemia-induced and aconitine-induced arrhythmias. Evidence shows that these alkaloids, in addition to their inhibitory action on Na+ and K+ channels, can also block the ICa–L, resembling the class IV of AADs (22, 57).
We discussed in previous subsections that some phytochemicals, including curcumin, cinnamophilin, barbaloin, and resveratrol, exert their antiarrhythmic effects through modulating multiple ion channels. One of the mechanisms behind their beneficial effects involves blocking the ICa–L, similar to the action of class IV AADs (26, 27, 29, 30, 32, 33, 36). As mentioned above, inhibiting ICa–L in such agents with multiple actions can moderate the AP prolongation that occurs by concurrent inhibition of K+ and Na+ channels, which could be considered as a starting point for developing future AADs without serious side effects like QT prolongation. Also, as we stated above, Rhodiola crenulata has protective effects against arrhythmias through affecting the expression of multiple ion channels, among which the increased expression of sarcoplasmic reticulum Ca2+ ATPase is likened to the action of subclass IVc of AADs (46).
Class (V): Mechanosensitive channel blockers
Transient receptor potential channels are mechanosensitive ion channels that have several subfamilies, among which TRPC1, TRPC3, and TRPC6 are related to cardiac function and are involved in intracellular Ca2+ signaling and the regulation of heart function. They are closely linked to the development of cardiac hypertrophy, fibrosis, arrhythmias, and myocardial infarction (68, 69). Inhibition of such channels has recently been considered to be a promising therapeutic target for treating some types of cardiac arrhythmias by reducing EAD-/DAD-induced TAs, known as Class (V) AADs according to modernized classification (7).
In the case of this class of AADs, our review did not find any study that concurrently evaluated the antiarrhythmic effects and such a mechanism of action of herbal medicine. However, we found a small numbers of plant extracts and multi-component herbal preparations, as described below and summarized in Table 2, whose inhibitory effects on mechanosensitive ion channels have been identified in in vitro studies, while their antiarrhythmic effects have been evaluated in some other separate studies. For instance, S. miltiorrhiza, known as Danshen in China, is one of the main components of a Chinese multi-component herbal preparation, namely Danqi soft capsule (DQ), found to be effective in suppressing cardiac arrhythmias (70). Salvianolic acid B, a bioactive compound found in the roots of S. miltiorrhiza, has been shown to be capable of inhibiting TRPC3 and TRPC6 mediated Ca2+ overload in rat cardiomyocytes (71). Thus, the antiarrhythmic effects of S. miltiorrhiza might be, at least in part, due to the inhibitory effects of Salvianolic acid B on TRPC channels. However, further studies are warranted to concurrently evaluate the efficacy and mechanism of action of this medicinal plant.
Class (VI): Gap junction channel modulators
Gap junctions are intercellular junctions composed of different subunits, namely connexin (Cx) proteins. Three major connexin isotypes are expressed in the heart: Cx43, Cx45, and Cx40. Connexins play an essential role in electrical coupling by modulating intercellular currents; they also influence AP conduction, cardiomyocyte death, and survival (72). The instability of gap junctions in response to pathophysiological signals, either by disrupting or over-expressing their subunits, can increase susceptibility to cardiac arrhythmias (73). Several agents acting as Cx openers or blockers have shown promising results in suppressing cardiac arrhythmias, known as Class VI in the modernized classification of AADs (7). Below, we summarized the results of experimental studies that investigated the antiarrhythmic effects of herbal medicine and concurrently determined the mechanism of antiarrhythmic action.
Nardostachys chinensis Batalin is a TCM shown to have potential cardioprotective effects in some studies (74, 75). One study by Zhang et al. (75) evaluated the protective effects of N. chinensis against spontaneous ventricular arrhythmias in a rat model of hyper-acute MI. Their results showed that pretreatment of rats with N. chinensis rhizome extract (600 mg.kg–1.day–1) for 30 days could reduce the incidence of VF, VT, and PVCs induced by I/R injury (75). The molecular analysis of ventricular myocyte tissues showed a reduced degradation rate of Cx43 in the treatment group, which might be the possible mechanism underlying the observed antiarrhythmic effects.
Grape seed proanthocyanidin extract (GSPE), a polyphenol derived from grape seeds, has been found to protect against cardiac arrhythmias (76, 77). Liang et al. (77) found that pretreatment of rabbits with GSPE (100 or 200 mg.kg–1) for 21 days significantly lowered the incidence of I/R-induced VF. The immunohistochemistry results showed that the expression of Cx43, impaired by I/R injury, was significantly improved in the GSPE group, which may provide a mechanism for antiarrhythmic effects similar to the effect of Gap junction channel modulators in class VI of AADs. Another study on a rat model of I/R injury showed that GSPE could reduce the incidence of I/R-induced ventricular arrhythmias through increasing the expression of Na+/K+-ATPase α1-subunit, confirmed by western blot analysis (76). Evidence shows that Na+/K+-ATPase α1 activity is necessary for the formation of gap junction proteins (78) and can modulate the cardiac remodeling that occurs in the failing heart (79). Hence, the increased expression of Cx43 and improving gap junctional communication observed in the Liang et al. study might be, at least in part, due to the increase in Na+/K+-ATPase activity by GSPE administration.
Linalool is a natural monoterpene extracted from aromatic plant-derived essential oils. Cardioprotective effects of Linalool against I/R injury have been found in several experimental studies (80, 81). Ke et al. (81) found that pretreatment of rats with linalool (50 or 100 mg.kg–1.day–1) for 7 days before LAD occlusion significantly decreased the prevalence, duration, and severity score of ventricular arrhythmias. Through molecular analysis, they proved the preventive effect of linalool against Cx43 degradation occurred by ischemic injury, which may be an underlying mechanism for the observed antiarrhythmic effects.
Tongguan capsule is a patented TCM consisting of four natural compounds, shown to be effective in CVDs (82). The antiarrhythmic effect of the Tongguan capsule has been proven only by one experimental study. Ma et al. (83) found that 4-week treatment with Tongguan capsule could significantly reduce susceptibility to arrhythmias in the post-MI rat model, probably through two main underlying mechanisms: (1) increasing the expression of Cx43, (2) reducing interstitial fibrosis, hypertrophy and left ventricular remodeling. These mechanisms are similar to those seen in classes VI and VII of AADs, respectively.
Danqi soft capsule is a combination of S. miltiorrhiza and P. notoginseng, two herbal medicines with cardioprotective effects (70). Ma et al. (70) showed the antiarrhythmic effect of low, middle, and high doses of DQ on MI-induced arrhythmia in rats. They found that all doses of DQ could significantly decrease cardiac remodeling, VT score and VT inducibility. They also found that DQ could reverse the downregulation of Cx43 and inhibit cardiac fibrosis formation via inhibiting the transforming growth factor-beta1 (TGF-β1)/Smad3 pathway. These are similar to those seen in class VI and VII of AADs that decrease arrhythmia by modulating gap junctions and preventing cardiac remodeling and hypertrophy, respectively.
Fumai granule is a Shengmai San-derived TCM containing a mixture of six medicinal herbs that have protective effects against arrhythmias. One study by Ma et al. (84) has tested the efficacy of this multi-component herbal preparation in preventing AF in a rat model of ischemic HF. They found that Fumai granule (500 mg.kg–1.day–1) for 4 weeks following the induction of ischemic HF could significantly reduce the inducibility and duration of electrical stimulation-induced AF. They also described two mechanisms for such effects, which include: (1) increasing the expression of Cx43 and Cx40 to resemble the action of class VI AADs, and (2) inhibiting cardiac fibrosis and inflammation through affecting the TGF-β1 pathways and reducing inflammatory markers such as interleukin-6 (IL-6), monocyte chemoattractant protein-1, tumor necrosis factor-α, and B-type natriuretic peptide.
Class (VII): Upstream target modulators
Evidence shows that inflammation and myocardial fibrosis can increase the risk of developing arrhythmias (85, 86). Several anti-fibrotic and anti-inflammatory agents were shown to have antiarrhythmic action through limiting pro-arrhythmia substrates such as post-infarct myocardial fibrosis and remodeling (87, 88). These drugs mostly have other cardioprotective effects, and though their antiarrhythmic efficacy has not been approved yet, they might be considered as potential therapeutic choices (88). Such cardioprotective non-antiarrhythmic drugs with potential antiarrhythmic action are classified as upstream target modulators in the modernized AADs classification (7). Below we described medicinal herbs or their active constituents with antiarrhythmic action like upstream target modulators.
Arnebia euchroma (Royle) I. M. Johnst. Radix and its active ingredients have been shown to be effective against CVDs (89, 90). Zhou et al. (89) have investigated the antiarrhythmic effects of this plant and found that 1-week pretreatment of rats with A. euchroma Radix (0.18 g.mL–1) could significantly reduce the incidence and duration of ACh-CaCl2-induced AF similar to that exerted by amiodarone as the positive control. Through an in vitro assay, A. euchroma Radix diminished atrial fibrosis and enlargement induced by ACh-CaCl2, which suggests that this mechanism may be responsible for the anti-AF effects exerted by this plant (89). Such a mechanism is similar to the action of upstream target modulators in the class VII AADs.
The antiarrhythmic effects of R. crenulata and its ability to act like class III and class IV of AADs were stated in previous subsections. Another mechanism proposed for the antiarrhythmic effects of R. crenulata is the regulation of inflammatory cellular signaling pathways. Hsiao et al. (45) found that R. crenulata and its main active ingredient, salidroside, exerted their antiarrhythmics effects by reducing the expression of inflammatory markers and inhibiting fibrosis, collagen genesis, and cellular pathways leading to apoptosis.
Hesperidin is a bioflavonoid mainly found in medicinal plants belonging to the Citrus spp. Gandhi et al. (91) investigated the antiarrhythmic effects of hesperidin (100 mg.kg–1) for 15 days in an in vivo model of I/R myocardial injury and found a considerable reduction in the incidence and duration of VT and VF and the severity score of the arrhythmias. Hesperidin significantly increased the endogenous antioxidant enzymes such as SOD and CAT, decreased oxidative stress and infarct size, and also exhibited anti-inflammatory action. These effects are likened to the action of upstream target modulators. Similarly, Pashai et al. (92) confirmed the protective effects of Hesperidin against I/R-induced arrhythmias and found that such effects are mediated by activating signaling pathways, leading to the inhibition of proinflammatory conditions.
Troxerutin is a flavonol-type flavonoid isolated from Styphnolobium japonicum (L.) Schott and Dimorphandra gardneriana Tul., and has been studied for its antiarrhythmic effect in one study. Najafi et al. (93) investigated the effect of troxerutin on I/R-induced arrhythmia in diabetic and healthy rat-isolated hearts. They showed that pretreatment of rats with troxerutin (150 mg.kg–1.day–1) for 4 weeks decreased the duration and incidence of PVC, VT, and suppressed VF. Moreover, troxerutin decreased the arrhythmias severity score in diabetic rats more than the control group. They further evaluated the mechanism behind such antiarrhythmic effects and found that troxerutin could remarkably reduce pro-inflammatory cytokines, similar to that described for upstream target modulators (93).
Magnolol, a lignan compound isolated from Magnolia officinalis Rehder and E. H. Wilson, has been found to be effective in preventing and suppressing cardiac arrhythmias (94, 95). Lee et al. (95) surveyed the effect of magnolol on I/R-induced arrhythmias in rats and concurrently evaluated its mechanism of action. They showed that pretreatment of rats with magnolol (0.2 and 0.5 μg.kg–1) before coronary ligation significantly abolished the VF development and reduced the duration of VT and VF. Through in vitro investigation, magnolol showed anti-inflammatory effects, indicated by reduced neutrophil migration in ischemic myocardium, which may explain its antiarrhythmic effects.
As we described in previous subsections, saponin compounds found in P. notoginseng have antiarrhythmic effects. In one study by Li et al. (96), these saponins were found to be effective in reducing the duration of CaCl2-ACh mixture-induced AF when administrated 1 h before model induction at the doses of 100 and 150 mg.kg–1 daily for 7 days. In this study, further insights into the mechanism showed the antioxidant and anti-inflammatory properties of this compound, which may explain, at least in part, its antiarrhythmic effects.
Anthocyanins are colored pigments of some flavonoid compounds found in several plants, such as bilberry (Vaccinium myrtillus L.) (97). Ziberna et al. (97) studied the antiarrhythmic effect of bilberry anthocyanins on I/R induced arrhythmia in rats isolated hearts. Their study showed that the perfusion of hearts with low concentration of bilberry anthocyanins (0.1, 1, 5, and 10 mg.L–1) could significantly reduce the incidence and duration of arrhythmias such as VF. The mechanism behind the antiarrhythmic effects of anthocyanins was found to be its antioxidant and anti-inflammatory properties, similar to the action of upstream target modulators.
Arctigenin is a lignan derived from A. lappa, shown to have anti-inflammatory and cardioprotective effects (28, 98). Yang et al. (28) have evaluated the antiarrhythmic and antioxidant effects of ATG in a rat model of I/R injury. They showed that pretreatment with ATG (50 mg.kg–1.day–1 and 200 mg.kg–1.day–1) could significantly prevent ventricular arrhythmias and reduce their duration during ischemia and reperfusion phases. Molecular analysis showed that ATG could remarkably increase the expression of nuclear factor erythroid 2-related factor 2 (Nrf2), a transcription factor modulating the cellular response to oxidative stress and inflammation, and some other antioxidant molecules. Considering the key role of activating Nrf2 signaling pathways in suppressing inflammatory processes (99), increasing the expression of Nrf2 following ATG administration seems to be an underlying mechanism for protecting the myocardium from cellular injuries and subsequent arrhythmias.
As described in the previous subsections, SSYX, DQ, and Fumai granule are multi-component herbal preparations demonstrated to be useful antiarrhythmic agents in experimental studies. One study by Ma et al. (16) showed that 4-week administration of SSYX (600 mg.kg–1.day–1) could significantly reduce the duration and vulnerability of programmed electrical stimulation-induced AF in a rat model of MI. This study also found that such effects might occur by inhibiting the expression of inflammatory factors and reducing fibrosis, similar to the effects exerted by upstream target modulators. Regarding the antiarrhythmic effects of the DQ capsule, Ma et al. (70) found the protective effects against MI-induced arrhythmia via inhibiting cardiac fibrosis formation in the rat model of MI, similar to the action of upstream target modulators. In the case of Fumai granule, the protective effects against electrical stimulation-induced AF were thought to be mainly related to the inhibition of cardiac fibrosis and inflammatory statues (84).
Ping-Lv-Mixture (PLM) is a Chinese multi-component herbal preparation consisting of a mixture of five medicinal plants. An and Yang found that 7-day pretreatment of rats with PLM (0.04, 0.2, and 1 g.kg–1.day–1) could significantly reduce the incidence and duration of VT, VF, and PVCs in the rat model of myocardial I/R injury (100). This study also showed that such protective effects might be mediated by antioxidative and anti-inflammatory mechanisms, assembling the action of upstream target modulators.
Yindanxinnaotong capsule is a Chinese patented multi-component herbal preparation, shown to have antiarrhythmic effects through affecting inflammatory pathways. One study by Wang et al. (101) found that the types of Yindanxinnaotong formulations which contain G. biloba and S. miltiorrhiza extracts could reduce the rate of I/R-induced ventricular arrhythmias in a rat model. In vitro investigation showed a reduction in myocardial injury markers and inflammatory factors, which may be responsible for the observed antiarrhythmic effects.
Clinical trial studies
Here we reviewed the available clinical studies which evaluate the efficacy and safety of multi-component herbal preparations and bioactive compounds for the treatment of cardiac arrhythmias in humans. The results are summarized in Table 4.
Dingji Fumai decoction
Dingji Fumai Decoction is a mixture of several herbal medicines developed to treat palpitations (39). Liang et al. (39) conducted a double-blind, randomized, placebo-controlled trial including 92 patients to investigate the safety and efficacy of adding DFD to metoprolol for treating PVCs and compare the results with the metoprolol alone group as the positive control group. The number of PVCs was significantly reduced in DFD combined with the metoprolol group compared to the metoprolol alone group. Moreover, no side effects were reported in the DFD treatment group. These researchers performed another double-blinded, randomized, placebo-controlled trial to investigate the impact of DFD on PVC in a larger population (102). In this study, 136 patients (71 in the DFD group and 65 in the control group) completed the study. The results revealed that after 12 weeks of treatment with DFD or placebo, the number of PVCs and the severity of palpitations remarkably decreased in the DFD arm. Also, patients had good compliance with the treatment, and DFD was well tolerated with no adverse drug reactions in the DFD arm.
Shenmai injection
Shenmai is a traditional Chinese herbal mixture mainly consisting of Panax ginseng C. A. Mey. and Ophiopogon japonicas (Thunb.) Ker Gawl. Shenmai injection is a Chinese patent injection that has been effective as adjunctive therapy for coronary heart disease. An open-label, non-randomized controlled trial by Deng et al. (103) studied the efficacy and safety of shenmai injection combined with amiodarone in suppressing paroxysmal AF. A total of 351 patients were enrolled in the study: 223 patients in the treatment group (shenmai injection + amiodarone) and 128 patients in the control group (amiodarone alone). The results of this study showed that the total efficacy rate had no significant difference between the two groups; however, in the treatment group, the ventricular rate was significantly lower than in the control group. Also, in the treatment group, the cardioversion rate was considerably higher, and it took less time to reach normal rhythm compared to the control group. Patients in both groups only experienced mild adverse drug reactions and there were no further adverse effects.
Baicalin
Baicalin is a flavone-type flavonoid isolated from the dried root of Scutellaria baicalensis Georgi. Xiao et al. (104), in an open-label, randomized controlled trial, investigated the efficacy of baicalin in patients with aconitine toxicity. A total of 60 patients were included in the study, divided into the treatment group (baicalin + conventional therapy) and the control group (conventional treatment alone). The study showed that patients in the baicalin group had much faster relief from symptoms of premature beats, auricular flutter, and auricular fibrillation and had a shorter recovery time compared to the control group.
Shensong Yangxin
Shensong Yangxin capsule is an approved herbal medicine for treating arrhythmias in China (105). Wang et al. (105) investigated the efficacy and safety of the SSYX capsule in treating PVCs in a double-blinded, randomized, multicenter clinical trial in 411 patients. Results showed that 12-week treatment with SSYX remarkably reduced the total number of PVCs and improved left ventricular ejection fraction compared to the placebo group. In another study, Zou et al. (106) conducted a double-blinded, placebo-controlled multicenter randomized clinical trial to evaluate the efficacy and safety of SSYX capsules in the treatment of PVCs in 677 patients with organic and 188 patients with non-organic heart diseases. They found that 8-week therapy with SSYX capsules significantly decreased the number of PVCs compared to the control group. Only one patient in the non-organic group and four in the organic group reported mild adverse effects with SSYX, which all disappeared after a few days.
Wenxin Keli
Wenxin Keli, a mixture of herbal medicines, as earlier described, has been reported to have therapeutic effects on cardiac arrhythmias (37). Hua et al. (107) conducted a randomized, double-blind, controlled clinical trial to determine the efficacy and safety of WXKL in treating patients with frequent PVCs. A total of 1071 patients completed the study (536 cases in the treatment arm and 535 cases in the placebo arm). The results of the study showed that a 4-week treatment with WXKL (9 g three times daily) significantly lowered the number of PVCs and their related symptoms compared to the placebo. Only three patients in the WXKL reported mild side effects, probably unrelated to the WXKL.
Xin Su Ning
Xin Su Ning (XSN) is a Chinese multi-component herbal preparation that has been patented for treating ventricular arrhythmia. Ma et al. (108) investigated the efficacy and safety of XSN in treating PVCs in a three-armed, double-blind, randomized controlled trial on 861 patients. A total of 779 patients completed the study, and results revealed that a 4-week treatment with XSN or mexiletine significantly lowered the number of PVCs and their related symptoms. Xin Su Ning had a higher rate of significantly effective responses than the placebo. Only mild side effects were reported, which probably were not related to the XSN. This study also reported the results of an animal study conducted to investigate the antiarrhythmic mechanism of this multi-herbal component. They found that XSN dose-dependently inhibited the human Nav1.5 channel at its inactivation state, suggesting a post-repolarization refractoriness period, which provides a mechanistic explanation for the XSN effect on the premature heart beat in patients. Xin Su Ning also inhibited the hERG K+ channel, which subsequently prolonged APD and increased the ERP of the heart, leading to PVC inhibition.
Discussion
This review targeted the antiarrhythmic effects and their underlying mechanisms of plant extracts, phytochemicals, and multi-component herbal preparations in preclinical and clinical studies. Below, we summarize the important results from reviewed studies and discuss the information gaps and research priorities.
In the present study, three plants extracts, 26 phytochemicals, and eight multi-component herbal preparations were found to be encouraging compounds for developing new antiarrhythmic drugs. The phytochemicals reviewed in this study were mostly polyphenol compounds (flavonoids and non-flavonoids), alkaloids, and terpenoids. The multi-component herbal preparations consisted of several herbal medicines; some were studied individually for their antiarrhythmic effects. Four multi-component herbal preparations were patented for their antiarrhythmic and cardioprotective effects.
The reviewed plants, multi-component herbal preparations, and phytochemicals had different electrophysiological properties and mechanisms of action, as shown in Figures 3, 4. Most of them exhibited their antiarrhythmic effects by activation or inhibition of ionic current or by affecting the expression of the cardiac ion channels. The modulation of gap junction proteins (Cx43 and Cx40), and elimination of the arrhythmia’s substrate, as upstream targets (e.g., fibrosis, remodeling, and inflammation) were the other investigated antiarrhythmic mechanisms. In some reviewed studies, the exact mechanism of action was not investigated, and the authors discussed some therapeutic mechanism hypotheses based on previous studies’ results. Although most plants demonstrated anti-oxidant properties, only some of them were clinically relevant for controlling cardiac arrhythmias. Also, the lack of information on cellular electrophysiological effects is another gap in reviewed studies. Understanding these information gaps will certainly develop the application of medicinal plants to prevent and treat diseases. Thus, further work to identify the therapeutic mechanisms and electrophysiological effects of plants, in parallel with exploring their pharmacological activity, is needed.
Most of the reviewed studies did not determine the phytochemical composition of the medicinal plants and just focused on previous studies to justify their pharmacological claims. The concurrent study of phytochemicals and mechanism of action should be investigated further to determine the main chemical compounds responsible for therapeutic effects. Also, some studies did not introduce the origin of medicinal plants, influencing the active constituents probably due to the various environmental factors. Many studies have proved that environmental differences, such as climate, soil parameters, and altitude, in different places of origin influence the content of active ingredients and, therefore, the pharmacological activity of medicinal plants (109). Thus, this issue should be a matter of interest in future research.
There are also some other information gaps in the method of the reviewed studies that need to be explored in future studies. Based on our quality assessment, as shown in Figure 2, the most detected biases were attributed to blinding and randomization, which are given little attention in animal studies. Also, the majority of studies had a short duration, which might weaken the level of evidence. Moreover, a high level of uncertainty in these studies, including inadequate information on optimal dose, the minimal active concentration, the frequency of administration, toxicity evaluation, the methods of specimen collection, sampling, and statistical analysis, may lead to reduced reproducibility and replicability, according to “Guidelines for submission in peer-reviewed pharmacological literature” (110).
This review emphasizes the importance of research on the cardioprotective of medicinal plants and their bioactive compounds to provide guidance for the future development of new antiarrhythmic drugs. The question is, what approach can bridge the gap between herbal medicine and modern pharmacology, and how can medicinal plants be viewed through the lens of modern pharmacology? Medicinal plants typically contain hundreds of active compounds, the identification of which is essential for comprehending the mechanism of the entire plant. Although discovering an active compound and describing its effects on pharmacological targets is useful in identifying lead compounds for drug discovery, investigating the effects of multiple compounds together and their synergistic effects may be more valuable. Multiple active ingredients of a single plant can simultaneously act on different cellular targets and together show a beneficial cumulative effect on a specific disease that may be superior to using each isolated component separately (111, 112). In other words, these compounds can potentially act as a “polypill,” a concept in modern medicine that was first introduced by Wald and Low in 2003 as a single pill combining several drugs with fixed doses for the prevention of CVDs (113). Combination therapies in modern medicine may be preferred in some chronic diseases such as cardiovascular disease and cancer due to their greater efficacy, lower resistance, and higher compliance rate over monotherapy. Cardiac arrhythmias are also complex diseases that are linked to the interacting results of multiple molecular, cellular, and physiological pathways. Investigating multiple compounds which simultaneously target multiple arrhythmogenic pathways may help to identify more effective and lower toxicity multi-target AADs. For instance, one of the issues in atrial arrhythmia management relates to the use of AADs that, in addition to their beneficial effect, lengthen the AP of ventricular cells, represented by QT prolongation in the electrocardiogram, which can itself trigger hazardous ventricular arrhythmias. The solution of modern medicine is to find drugs that selectively act on the atria and have minimal effect on the ventricles. Through this review, we also found some phytochemicals and multiple-component herbal formulations, like WXKL, that have selective antiarrhythmic effects against atrial arrhythmias without having a major effect on the AP of the ventricles. Regarding WXKL, evidence shows its inhibitory effect on multiple ion channel currents (7, 38), possibly indicating that different active ingredients of such herbal formulations have targeted desirable ion channels, which have led to its acting as an atrial-selective agent. Investigating active ingredients of such herbs using scientific methods to clarify their mechanisms individually and their pooled effects and interactions when used together, and subsequently optimizing combinations in terms of dose-response, can be a starting point for developing future atrial-selective AADs with a minimum adverse effect on ventricules. This is precisely what “Network Pharmacology” does; a new paradigm in drug discovery that examines the underlying pathologic pathways of diseases as a network in order to combine different synergistic agents to create an optimal formula with the greatest efficacy and the fewest side effects for a specific disease (112).
Overall, this review tried to find plant-derived natural agents, including herbal extracts, phytochemicals, and herbal formulations which potentially could be considered as lead compounds for future drug discovery in the field of cardiac arrhythmias. Classification of agents based on the modernized classification of AADs may help researchers design comparative studies of better quality and identify the therapeutic potential of these medicinal plants for their use as a new medicine. Gaps in study design, phytochemistry, pharmacology, and toxicology information can dispraise the reproducibility of these pieces of evidence. Thus, more experimental and clinical studies of better quality should be designed to bridge these gaps and validate the relationship between their uses in traditional medicine and modern pharmacology.
Author contributions
DS, BA, and AV-F contributed to the conception and design of the study. DS and BA contributed to search and data collection. DS, AV-F, and RR supervised the research. DS, BA, AV-F, RR, AT, and HR wrote the original draft of the manuscript. BA prepared the figures for the manuscript. All authors contributed to manuscript revision and read and approved the submitted version.
Funding
This research was funded by the Tehran University of Medical Science (Grant no. 56614). The funders had no role in the study design, data collection and analysis, decision to publish, or preparation of the manuscript.
Conflict of interest
The authors declare that the research was conducted in the absence of any commercial or financial relationships that could be construed as a potential conflict of interest.
Publisher’s note
All claims expressed in this article are solely those of the authors and do not necessarily represent those of their affiliated organizations, or those of the publisher, the editors and the reviewers. Any product that may be evaluated in this article, or claim that may be made by its manufacturer, is not guaranteed or endorsed by the publisher.
Supplementary material
The Supplementary Material for this article can be found online at: https://www.frontiersin.org/articles/10.3389/fcvm.2022.990063/full#supplementary-material
Abbreviations
AADs, antiarrhythmic drugs; CVDs, cardiovascular disease; If, pacemaker current; SAN, sinoatrial node; SSYX, Shensong Yangxin; AP, action potential; Nav, voltage-gated Na+; INa, sodium currents; Cav, voltage-gated Ca2+; WXKL, Wenxin Keli; ACh, acetylcholine; AF, atrial fibrillation; ERP, effective refractory period; Vmax, action potential upstroke; INa–L, late sodium channel current; INa–T, transient sodium currents; DFD, Dingji Fumai decoction; ICa, calcium currents; ICa–L, L-type calcium currents; DADs, delayed afterdepolarization; I/R, ischemia-reperfusion; EC50, Half maximal effective concentration; Ito, transient outward potassium current; VT, ventricular tachyarrhythmias; VF, ventricular fibrillation; EAD, early afterdepolarization; IKr, rapid delayed rectifier potassium currents; ISS, sustained outward potassium currents; Kv, voltage-gated K+; hERG, Human Ether-a-go-go-related Gene mediated potassium currents; IK1, inward rectifier potassium current; T2DM, type 2 diabetes mellitus; MI, myocardial infarction; KATP, ATP-sensitive potassium channel; Kir, Inwardly rectifying potassium channel subunit; IK, outward delayed potassium current; IKs, slow delayed rectifier potassium current; AV, atrioventricular; PVC, premature ventricular contraction; IV, intravenous; TRPC, Transient Receptor Potential Channel; DQ, Danqi soft capsule; Cx, connexin; TGF- β 1/Smad3 pathway, transforming growth factor-beta1; TCM, traditional Chinese medicine; IL, interleukin; GSPE, Grape seed proanthocyanidin extract; PLM, Ping-Lv-Mixture; Nrf2, nuclear factor erythroid 2-related factor 2.
References
1. Nattel S, Harada M. Atrial remodeling and atrial fibrillation: recent advances and translational perspectives. J Am Coll Cardiol. (2014) 63:2335–45. doi: 10.1016/j.jacc.2014.02.555
2. Williams EVA. Classification of antiarrhythmic actions reassessed after a decade of new drugs. J Clin Pharmacol. (1984) 24:129–47. doi: 10.1002/j.1552-4604.1984.tb01822.x
3. Tavolinejad H, Soltani D, Zargaran A, Rezaeizadeh H, Vasheghani-Farahani A. The story of amiodarone: discovery of an anti-arrhythmic drug by exploring a medicinal plant, from history to clinical practice. Eur Heart J. (2019) 40:2758–9. doi: 10.1093/eurheartj/ehz583
4. Stout CW, Weinstock J, Homoud MK, Wang PJ, Estes NM, Link MS. Herbal medicine: beneficial effects, side effects, and promising new research in the treatment of arrhythmias. Curr Cardiol Rep. (2003) 5:395–401. doi: 10.1007/s11886-003-0097-x
5. Bahramsoltani R, Farzaei MH, Ram M, Nikfar S, Rahimi R. Bioactive foods and medicinal plants for cardiovascular complications of type ii diabetes: current clinical evidence and future perspectives. Evid Based Complement Alternat Med. (2021) 2021:6681540. doi: 10.1155/2021/6681540
6. Bahramsoltani R, Ebrahimi F, Farzaei MH, Baratpourmoghaddam A, Ahmadi P, Rostamiasrabadi P, et al. Dietary polyphenols for atherosclerosis: a comprehensive review and future perspectives. Crit Rev Food Sci Nutr. (2019) 59:114–32. doi: 10.1080/10408398.2017.1360244
7. Lei M, Wu L, Terrar DA, Huang CL-H. Modernized classification of cardiac antiarrhythmic drugs. Circulation. (2018) 138:1879–96. doi: 10.1161/CIRCULATIONAHA.118.035455
8. Hooijmans CR, Rovers MM, de Vries R, Leenaars M, Ritskes-Hoitinga M, Langendam MW. Syrcle’s risk of bias tool for animal studies. BMC Med Res Methodol. (2014) 14:43. doi: 10.1186/1471-2288-14-43
9. Jadad AR, Moore RA, Carroll D, Jenkinson C, Reynolds DJM, Gavaghan DJ, et al. Assessing the quality of reports of randomized clinical trials: is blinding necessary? Control Clin Trials. (1996) 17:1–12. doi: 10.1016/0197-2456(95)00134-4
10. Biel M, Wahl-Schott C, Michalakis S, Zong X. Hyperpolarization-activated cation channels: from genes to function. Physiol Rev. (2009) 89:847–85. doi: 10.1152/physrev.00029.2008
11. Hoppe UC, Jansen E, Südkamp M, Beuckelmann DJ. Hyperpolarization-activated inward current in ventricular myocytes from normal and failing human hearts. Circulation. (1998) 97:55–65. doi: 10.1161/01.CIR.97.1.55
12. Oknińska M, Paterek A, Zambrowska Z, Mackiewicz U, Ma̧czewski M. Effect of ivabradine on cardiac ventricular arrhythmias: friend or foe? J Clin Med. (2021) 10:4732. doi: 10.3390/jcm10204732
13. Zhao X, Yao H, Yin H-L, Zhu Q-L, Sun J-L, Ma W, et al. Ginkgo Biloba extract and ginkgolide antiarrhythmic potential by targeting Herg and Ica-L channel. J Pharmacol Sci. (2013) 123:318–27. doi: 10.1254/jphs.13118FP
14. Koltai M, Tosaki A, Hosford D, Braquet P. Ginkgolide B protects isolated hearts against arrhythmias induced by ischemia but not reperfusion. Eur J Pharmacol. (1989) 164:293–302. doi: 10.1016/0014-2999(89)90470-6
15. Satoh H. Suppression of pacemaker activity by Ginkgo Biloba extract and its main constituent, bilobalide in rat sino-atrial nodal cells. Life Sci. (2005) 78:67–73. doi: 10.1016/j.lfs.2005.04.081
16. Ma J, Yin C, Ma S, Qiu H, Zheng C, Chen Q, et al. Shensong Yangxin capsule reduces atrial fibrillation susceptibility by inhibiting atrial fibrosis in rats with post-myocardial infarction heart failure. Drug Design Dev Ther. (2018) 12:3407–18. doi: 10.2147/DDDT.S182834
17. Sun L-P, Li N, Wu Y-l, Pu J-L. Effects of Shensong Yangxin capsule on pacemaker channels encoded by human Hcn4 gene. Chin Med J. (2010) 123:3148–50.
18. Chen H, Chen Y, Tang Y, Yang J, Wang D, Yu T, et al. Berberine attenuates spontaneous action potentials in sinoatrial node cells and the currents of human Hcn4 channels expressed in Xenopus laevis oocytes. Mol Med Rep. (2014) 10:1576–82. doi: 10.3892/mmr.2014.2377
19. Balser JR. Structure and function of the cardiac sodium channels. Cardiovasc Res. (1999) 42:327–8. doi: 10.1016/S0008-6363(99)00031-0
20. Zimmer T, Haufe V, Blechschmidt S. Voltage-gated sodium channels in the mammalian heart. Glob Cardiol Sci Pract. (2015) 2014:58. doi: 10.5339/gcsp.2014.58
21. Zeng M, Jiang W, Tian Y, Hao J, Cao Z, Liu Z, et al. Andrographolide inhibits arrhythmias and is cardioprotective in rabbits. Oncotarget. (2017) 8:61226. doi: 10.18632/oncotarget.18051
22. Runtao G, Guo D, Jiangbo Y, Xu W, Shusen Y. Oxymatrine, the main alkaloid component of sophora roots, protects heart against arrhythmias in rats. Planta Med. (2011) 77:226–30. doi: 10.1055/s-0030-1250256
23. Chen C-Y, Wu H-M, Chao W-Y, Lee C-H. Review on pharmacological activities of liriodenine. Afr J Pharm Pharmacol. (2013) 7:1067–70. doi: 10.5897/AJPP2013.3477
24. Chang GJ, Wu MH, Wu YC, Su MJ. Electrophysiological mechanisms for antiarrhythmic efficacy and positive inotropy of liriodenine, a natural aporphine alkaloid from fissistigma glaucescens. Br J Pharmacol. (1996) 118:1571–83. doi: 10.1111/j.1476-5381.1996.tb15577.x
25. Wongcharoen W, Phrommintikul A. The protective role of curcumin in cardiovascular diseases. Int J Cardiol. (2009) 133:145–51. doi: 10.1016/j.ijcard.2009.01.073
26. Song L, Zhang Z-F, Hu L-K, Zhang P-H, Cao Z-Z, Liu Z-P, et al. Curcumin, a multi-ion channel blocker that preferentially blocks Late Na+ current and prevents I/R-induced arrhythmias. Front Physiol. (2020) 11:978. doi: 10.3389/fphys.2020.00978
27. Zhao Z, Yin Y, Wu H, Jiang M, Lou J, Bai G, et al. Arctigenin, a potential anti-arrhythmic agent, inhibits aconitine-induced arrhythmia by regulating multi-ion channels. Cell Physiol Biochem. (2013) 32:1342–53. doi: 10.1159/000354532
28. Yang J, Yin H-S, Cao Y-J, Jiang Z-A, Li Y-J, Song M-C, et al. Arctigenin attenuates ischemia/reperfusion induced ventricular arrhythmias by decreasing oxidative stress in rats. Cell Physiol Biochem. (2018) 49:728–42. doi: 10.1159/000493038
29. Su M-J, Chen W-P, Lo T-Y, Wu T-S. Ionic mechanisms for the antiarrhythmic action of cinnamophilin in rat heart. J Biomed Sci. (1999) 6:376–86. doi: 10.1007/BF02253669
30. Cao Z-Z, Tian Y-J, Hao J, Zhang P-H, Liu Z-P, Jiang W-Z, et al. Barbaloin inhibits ventricular arrhythmias in rabbits by modulating voltage-gated ion channels. Acta Pharmacol Sin. (2018) 39:357–70. doi: 10.1038/aps.2017.93
31. Hung L-M, Chen J-K, Huang S-S, Lee R-S, Su M-J. Cardioprotective effect of resveratrol, a natural antioxidant derived from grapes. Cardiovasc Res. (2000) 47:549–55. doi: 10.1016/S0008-6363(00)00102-4
32. Chen Y-R, Yi F-F, Li X-Y, Wang C-Y, Chen L, Yang X-C, et al. Resveratrol attenuates ventricular arrhythmias and improves the long-term survival in rats with myocardial infarction. Cardiovasc Drugs Ther. (2008) 22:479. doi: 10.1007/s10557-008-6141-8
33. Zhang Y, Liu Y, Wang T, Li B, Li H, Wang Z, et al. Resveratrol, a natural ingredient of grape skin: antiarrhythmic efficacy and ionic mechanisms. Biochem Biophys Res Commun. (2006) 340:1192–9. doi: 10.1016/j.bbrc.2005.12.124
34. Liew R, Stagg MA, MacLeod KT, Collins P. The red wine polyphenol, resveratrol, exerts acute direct actions on guinea-pig ventricular myocytes. Eur J Pharmacol. (2005) 519:1–8. doi: 10.1016/j.ejphar.2005.06.017
35. Zhang L-P, Yin J-X, Liu Z, Zhang Y, Wang Q-S, Zhao J. Effect of resveratrol on L-type calcium current in rat ventricular myocytes. Acta Pharmacol Sin. (2006) 27:179–83. doi: 10.1111/j.1745-7254.2006.00250.x
36. Chen W-P, Su M-J, Hung L-M. In vitro electrophysiological mechanisms for antiarrhythmic efficacy of resveratrol, a red wine antioxidant. Eur J Pharmacol. (2007) 554:196–204. doi: 10.1016/j.ejphar.2006.10.016
37. Burashnikov A, Petroski A, Hu D, Barajas-Martinez H, Antzelevitch C. Atrial-selective inhibition of sodium-channel current by Wenxin Keli is effective in suppressing atrial fibrillation. Heart Rhythm. (2012) 9:125–31. doi: 10.1016/j.hrthm.2011.08.027
38. Burashnikov A, Di Diego JM, Zygmunt AC, Belardinelli L, Antzelevitch C. Atrium-selective sodium channel block as a strategy for suppression of atrial fibrillation: differences in sodium channel inactivation between atria and ventricles and the role of ranolazine. Circulation. (2007) 116:1449–57. doi: 10.1161/CIRCULATIONAHA.107.704890
39. Liang B, Fu L, Zou F, Liao H. The efficacy and safety of Dingji Fumai decoction combined with metoprolol in premature ventricular contractions: a randomized controlled clinical trial. J Mol Cell Cardiol. (2020) 140:27. doi: 10.1016/j.yjmcc.2019.11.062
40. Liang B, Zhou Y, Fu L, Liao H-L. Antiarrhythmic mechanisms of Chinese herbal medicine Dingji Fumai decoction. Evid Based Compl Altern Med. (2020) 2020:9185707. doi: 10.1155/2020/9185707
41. Herring N, Kalla M, Paterson DJ. The autonomic nervous system and cardiac arrhythmias: current concepts and emerging therapies. Nat Rev Cardiol. (2019) 16:707–26. doi: 10.1038/s41569-019-0221-2
42. Somova LI, Shode FO, Mipando M. Cardiotonic and antidysrhythmic effects of oleanolic and ursolic acids, methyl maslinate and uvaol. Phytomedicine. (2004) 11:121–9. doi: 10.1078/0944-7113-00329
43. Thomsen MB. Potassium channels in the heart. Channelopath Heart Dis. (2018) 6:47–75 doi: 10.1007/978-3-319-77812-9_3
44. Ma Y, Wang Y, Gao Y, Fu Y, Li J. Total flavonoids from ganshanbian (herba hyperici attenuati) effect the expression of Cal-A 1c and Katp-Kir6.1 Mrna of the myocardial cell membrane in myocardial ischemia-reperfusion arrhythmia rats. J Tradit Chin Med. (2014) 34:357–61. doi: 10.1016/S0254-6272(14)60102-3
45. Hsiao Y-W, Tsai Y-N, Huang Y-T, Liu S-H, Lin Y-J, Lo L-W, et al. Rhodiola crenulata reduces ventricular arrhythmia through mitigating the activation of Il-17 and Inhibiting the Mapk signaling pathway. Cardiovasc Drugs Ther. (2021) 35:889–900. doi: 10.1007/s10557-020-07072-z
46. Liu SH, Hsiao YW, Chong E, Singhal R, Fong MC, Tsai YN, et al. Rhodiola inhibits atrial arrhythmogenesis in a heart failure model. J Cardiovasc Electrophysiol. (2016) 27:1093–101. doi: 10.1111/jce.13026
47. Pandit SV, Workman AJ. Atrial electrophysiological remodeling and fibrillation in heart failure. Clin Med Insights Cardiol. (2016) 10:41–6. doi: 10.4137/CMC.S39713
48. Sanders P, Morton JB, Davidson NC, Spence SJ, Vohra JK, Sparks PB, et al. Electrical remodeling of the atria in congestive heart failure: electrophysiological and electroanatomic mapping in humans. Circulation. (2003) 108:1461–8. doi: 10.1161/01.CIR.0000090688.49283.67
49. Uhm JS, Mun HS, Wi J, Shim J, Joung B, Lee MH, et al. Prolonged atrial effective refractory periods in atrial fibrillation patients associated with structural heart disease or sinus node dysfunction compared with lone atrial fibrillation. Pacing Clin Electrophysiol. (2013) 36:163–71. doi: 10.1111/pace.12037
50. Li GR, Wang HB, Qin GW, Jin MW, Tang Q, Sun HY, et al. Acacetin, a natural flavone, selectively inhibits human atrial repolarization potassium currents and prevents atrial fibrillation in dogs. Circulation. (2008) 117:2449–57. doi: 10.1161/CIRCULATIONAHA.108.769554
51. Chen X, Guo H, Li Q, Zhang Y, Liu H, Zhang X, et al. Protective effect of berberine on aconite-induced myocardial injury and the associated mechanisms. Mol Med Rep. (2018) 18:4468–76. doi: 10.3892/mmr.2018.9476
52. Wang L-H, Li X-L, Li Q, Fu Y, Yu H-J, Sun Y-Q, et al. Berberine alleviates ischemic arrhythmias via recovering depressed ito and ica currents in diabetic rats. Phytomedicine. (2012) 19:206–10. doi: 10.1016/j.phymed.2011.11.002
53. Wang LH, Yu CH, Fu Y, Li Q, Sun YQ. Berberine elicits anti-arrhythmic effects via Ik1/Kir2.1 in the rat type 2 diabetic myocardial infarction model. Phytother Res. (2011) 25:33–7. doi: 10.1002/ptr.3097
54. Li B-X, Yang B-F, Zhou J, Xu C, Li Y-R. Inhibitory effects of berberine on Ik1, Ik, and Herg channels of cardiac myocytes. Acta Pharmacol Sin. (2001) 22:125–31.
55. Neto FR. Electropharmacological effects of berberine on canine cardiac purkinje fibres and ventricular muscle and atrial muscle of the rabbit. Br J Pharmacol. (1993) 108:534. doi: 10.1111/j.1476-5381.1993.tb12836.x
56. Wang Y-X, Zheng Y-M. Ionic mechanism responsible for prolongation of cardiac action-potential duration by berberine. J Cardiovasc Pharmacol. (1997) 30:214–22. doi: 10.1097/00005344-199708000-00010
57. Yong-Gang C, Shan J, Lei L, Jing-Quan G, Zhi-Ying S, Yan L, et al. Antiarrhythmic effects and ionic mechanisms of oxymatrine from Sophora flavescens. Phytother Res. (2010) 24:1844–9. doi: 10.1002/ptr.3206
58. Lai ZF, Shao Z, Chen YZ, He M, Huang Q, Nishi K. Effects of sasanquasaponin on ischemia and reperfusion injury in mouse hearts. J Pharmacol Sci. (2004) 94:313–24. doi: 10.1254/jphs.94.313
59. Shan H, Li X, Pan Z, Zhang L, Cai B, Zhang Y, et al. Tanshinone IIA protects against sudden cardiac death induced by lethal arrhythmias via repression of microrna-1. Br J Pharmacol. (2009) 158:1227–35. doi: 10.1111/j.1476-5381.2009.00377.x
60. Huang W, Wang Y, Cao Y-G, Qi H-P, Li L, Bai B, et al. Antiarrhythmic effects and ionic mechanisms of allicin on myocardial injury of diabetic rats induced by streptozotocin. Naunyn Schmiedeberg Arch Pharmacol. (2013) 386:697–704. doi: 10.1007/s00210-013-0872-1
61. Cao H, Huang C, Wang X. Allicin inhibits transient outward potassium currents in mouse ventricular myocytes. Exp Ther Med. (2016) 11:1896–900. doi: 10.3892/etm.2016.3116
62. Wang X, Wang X, Gu Y, Wang T, Huang C. Wenxin Keli attenuates ischemia-induced ventricular arrhythmias in rats: involvement of L-type calcium and transient outward potassium currents. Mol Med Rep. (2013) 7:519–24. doi: 10.3892/mmr.2012.1195
63. Zhao Y, Gao F, Zhang Y, Wang H, Zhu J, Chang L, et al. Shensong Yangxin capsules prevent ischemic arrhythmias by prolonging action potentials and alleviating Ca2+ overload. Mol Med Rep. (2016) 13:5185–92. doi: 10.3892/mmr.2016.5203
64. Mesirca P, Torrente AG, Mangoni ME. Functional role of voltage gated Ca2+ channels in heart automaticity. Front Physiol. (2015) 6:19. doi: 10.3389/fphys.2015.00019
65. Yang X, Xiong X, Wang H, Wang J. Protective effects of Panax notoginseng saponins on cardiovascular diseases: a comprehensive overview of experimental studies. Evid Based Compl Altern Med. (2014) 2014:204840. doi: 10.1155/2014/204840
66. Guan YY, Miao LY, Sun JJ. Effects of Rb1 from the Panax notoginseng saponins on arrhythmia and Ca2+ movement in rat heart cells. Drug Dev Res. (1996) 39:179–83. doi: 10.1002/(SICI)1098-2299(199610)39:2<179::AID-DDR11>3.0.CO;2-7
67. Wen-Feng C, Guo-Fen Q, Yan-Jie L, Zhen-Wei P, Xian-Mei P, Yun-Long B, et al. Flavonoids from Chinese Viscum coloratum: antiarrhythmic efficacy and ionic mechanisms. Phytother Res. (2006) 20:1100–2. doi: 10.1002/ptr.2006
68. Nishida M, Hara Y, Yoshida T, Inoue R, Mori Y. TRP channels: molecular diversity and physiological function. Microcirculation. (2006) 13:535–50. doi: 10.1080/10739680600885111
69. Wen H, Gwathmey JK, Xie L-H. Role of transient receptor potential canonical channels in heart physiology and pathophysiology. Front Cardiovasc Med. (2020) 7:24. doi: 10.3389/fcvm.2020.00024
70. Ma S, Ma J, Mai X, Zhao X, Guo L, Zhang M. Danqi soft capsule prevents infarct border zone remodelling and reduces susceptibility to ventricular arrhythmias in post-myocardial infarction rats. J Cell Mol Med. (2019) 23:5454–65. doi: 10.1111/jcmm.14428
71. Chen R-C, Sun G-B, Ye J-X, Wang J, Sun X-B. Salvianolic acid B attenuates doxorubicin-induced Er stress by inhibiting Trpc3 and Trpc6 mediated Ca2+ overload in rat cardiomyocytes. Toxicol Lett. (2017) 276:21–30. doi: 10.1016/j.toxlet.2017.04.010
72. Lo CW. Role of gap junctions in cardiac conduction and development: insights from the connexin knockout mice. Am Heart Assoc. (2000) 87:346–8. doi: 10.1161/01.RES.87.5.346
73. King JH, Huang CL, Fraser JA. Determinants of myocardial conduction velocity: implications for arrhythmogenesis. Front Physiol. (2013) 4:154. doi: 10.3389/fphys.2013.00154
74. Li M, Xu X, Yang X, Kwong JS, Shang H. The cardioprotective and antiarrhythmic effects of Nardostachys chinensis in animal and cell experiments. BMC Compl Altern Med. (2017) 17:398. doi: 10.1186/s12906-017-1910-1
75. Zhang J, Qiang CC, Li WJ, Liu LJ, Lin XX, Cheng YJ, et al. Effects of Nardostachys chinensis on spontaneous ventricular arrhythmias in rats with acute myocardial infarction. J Cardiovasc Pharmacol. (2014) 64:127–33. doi: 10.1097/FJC.0000000000000096
76. Zhao G, Gao H, Qiu J, Lu W, Wei X. The molecular mechanism of protective effects of grape seed proanthocyanidin extract on reperfusion arrhythmias in rats in vivo. Biol Pharm Bull. (2010) 33:759–67. doi: 10.1248/bpb.33.759
77. Liang Y, Qiu J, Gao HQ, Li BY. Protective effect of grape seed proanthocyanidins extracts on reperfusion arrhythmia in rabbits. J Nutr Sci Vitaminol. (2009) 55:223–30. doi: 10.3177/jnsv.55.223
78. Rajamanickam GD, Kastelic JP, Thundathil JC. The ubiquitous isoform of Na/K-atpase (Atp1a1) regulates junctional proteins, connexin 43 and Claudin 11 Via Src-Egfr-Erk1/2-Creb pathway in rat sertoli cells†. Biol Reprod. (2017) 96:456–68. doi: 10.1095/biolreprod.116.141267
79. Liu L, Wu J, Kennedy DJ. Regulation of cardiac remodeling by cardiac Na+/K+-Atpase isoforms. Front Physiol. (2016) 7:382. doi: 10.3389/fphys.2016.00382
80. Zheng X-H, Liu C-P, Hao Z-G, Wang Y-F, Li X-L. Protective effect and mechanistic evaluation of linalool against acute myocardial ischemia and reperfusion injury in rats. RSC Adv. (2017) 7:34473–81. doi: 10.1039/C7RA00743D
81. Ke J, Zhu C, Zhang Y, Zhang W. Anti-arrhythmic effects of linalool Via Cx43 expression in a rat model of myocardial infarction. Front Pharmacol. (2020) 11:926. doi: 10.3389/fphar.2020.00926
82. Qi J, Yu J, Wang L, Guo L, Ma S, Huang D, et al. Tongguan capsule protects against myocardial ischemia and reperfusion injury in mice. Evid Based Complement Altern Med. (2013) 2013:159237. doi: 10.1155/2013/159237
83. Ma S, Ma J, Zhou Y, Guo L, Bai J, Zhang M. Tongguan capsule derived-herb ameliorates remodeling at infarcted border zone and reduces ventricular arrhythmias in rats after myocardial infarction. Biomed Pharmacother. (2019) 120:109514. doi: 10.1016/j.biopha.2019.109514
84. Ma J, Ma S, Yin C, Wu H. Shengmai San-derived herbal prevents the development of a vulnerable substrate for atrial fibrillation in a rat model of ischemic heart failure. Biomed Pharmacother. (2018) 100:156–67. doi: 10.1016/j.biopha.2018.02.013
85. Li N, Brundel BJ. Inflammasomes and proteostasis novel molecular mechanisms associated with atrial fibrillation. Circ Res. (2020) 127:73–90. doi: 10.1161/CIRCRESAHA.119.316364
86. De Jong S, van Veen TA, van Rijen HV, de Bakker JM. Fibrosis and cardiac arrhythmias. J Cardiovasc Pharmacol. (2011) 57:630–8. doi: 10.1097/FJC.0b013e318207a35f
87. Lopaschuk GD, Verma S. Mechanisms of cardiovascular benefits of sodium glucose co-transporter 2 (Sglt2) inhibitors: a state-of-the-art review. Basic Transl Sci. (2020) 5:632–44. doi: 10.1016/j.jacbts.2020.02.004
88. Andelova K, Bacova BS, Sykora M, Hlivak P, Barancik M, Tribulova N. Mechanisms underlying antiarrhythmic properties of cardioprotective agents impacting inflammation and oxidative stress. Int J Mol Sci. (2022) 23:1416. doi: 10.3390/ijms23031416
89. Zhou Q, Chen B, Chen X, Wang Y, Ji J, Kizaibek M, et al. Arnebiae radix prevents atrial fibrillation in rats by ameliorating atrial remodeling and cardiac function. J Ethnopharmacol. (2020) 248:112317. doi: 10.1016/j.jep.2019.112317
90. Yang J, Wang Z, Chen D-L. Shikonin ameliorates isoproterenol (Iso)-induced myocardial damage through suppressing fibrosis, inflammation, apoptosis and Er stress. Biomed Pharmacother. (2017) 93:1343–57. doi: 10.1016/j.biopha.2017.06.086
91. Gandhi C, Upaganalawar A, Balaraman R. Protection against in vivo focal myocardial ischemia/reperfusion injury-induced arrhythmias and apoptosis by hesperidin. Free Radic Res. (2009) 43:817–27. doi: 10.1080/10715760903071656
92. Pashai M, Toutounchi SNS, Rameshrad M, Vaez H, Fathiazad F, Garjani A. The effects of hesperidin on ischemia/reperfusion induced arrhythmias and infarct size in isolated rat heart. Pharm Sci. (2016) 22:68–75. doi: 10.15171/PS.2016.12
93. Najafi M, Noroozi E, Javadi A, Badalzadeh R. Anti-arrhythmogenic and anti-inflammatory effects of troxerutin in ischemia/reperfusion injury of diabetic myocardium. Biomed Pharmacother. (2018) 102:385–91. doi: 10.1016/j.biopha.2018.03.047
94. Hong C, Huang S, Tsai S. Magnolol reduces infarct size and suppresses ventricular arrhythmia in rats subjected to coronary ligation. Clin Exp Pharmacol Physiol. (1996) 23:660–4. doi: 10.1111/j.1440-1681.1996.tb01753.x
95. Lee Y-M, Hsiao G, Chen H-R, Chen Y-C, Sheu J-R, Yen M-H. Magnolol reduces myocardial ischemia/reperfusion injury via neutrophil inhibition in rats. Eur J Pharmacol. (2001) 422:159–67. doi: 10.1016/S0014-2999(01)01069-X
96. Li D, Zeng X, Zheng B, Li W, Dai R. Therapeutic effects of Panax notoginseng saponins against atrial fibrillation in rats. Int J Clin Exp Med. (2019) 12:9146–51.
97. Ziberna L, Lunder M, Moze S, Vanzo A, Tramer F, Passamonti S, et al. Acute cardioprotective and cardiotoxic effects of bilberry anthocyanins in ischemia–reperfusion injury: beyond concentration-dependent antioxidant activity. Cardiovasc Toxicol. (2010) 10:283–94. doi: 10.1007/s12012-010-9091-x
98. Ni S-H, Sun S-N, Zhou Z, Li Y, Huang Y-S, Li H, et al. Arctigenin alleviates myocardial infarction injury through inhibition of the Nfat5-related inflammatory phenotype of cardiac macrophages/monocytes in mice. Lab Investig. (2020) 100:527–41. doi: 10.1038/s41374-019-0340-8
99. Ahmed SMU, Luo L, Namani A, Wang XJ, Tang X. Nrf2 signaling pathway: pivotal roles in inflammation. Biochim Biophys Acta Mol Basis Dis. (2017) 1863:585–97. doi: 10.1016/j.bbadis.2016.11.005
100. An W, Yang J. Protective effects of ping-Lv-mixture (Plm), a medicinal formula on arrhythmias induced by myocardial ischemia-reperfusion. J Ethnopharmacol. (2006) 108:90–5. doi: 10.1016/j.jep.2006.04.009
101. Wang W, Wang L, Yang H, Wang J, Yin X, Xu H, et al. Protective effects of Yindanxinnaotong capsule in a rat model of myocardial ischemia/reperfusion injury. J Tradit Chin Med. (2014) 34:699–709. doi: 10.1016/S0254-6272(15)30085-6
102. Liang B, Zou FH, Fu L, Liao HL. Chinese herbal medicine Dingji Fumai decoction for ventricular premature contraction: a real-world trial. Biomed Res Int. (2020) 2020:5358467. doi: 10.1155/2020/5358467
103. Deng M, Sui XQ, Zhu SB, Ma W, Xu Y, Chen ZM. Clinical observation on the treatment of atrial fibrillation with amiodarone combined with Shenmai injection (参麦注射液). Chin J Integr Med. (2010) 16:453–6. doi: 10.1007/s11655-010-0540-y
104. Xiao GL, Zhang CH, Liu GD, Liu FY, Liu ZY, Hu SY, et al. Clinical study of the effects of baicalin on arrhythmia induced by aconitine poisoning. J Med Plants Res. (2011) 5:88–92.
105. Wang X, Hu D, Dang S, Huang H, Huang CX, Yuan MJ, et al. Effects of traditional Chinese medicine Shensong Yangxin capsules on heart rhythm and function in congestive heart failure patients with frequent ventricular premature complexes: a randomized, double-blind, multicenter clinical trial. Chin Med J (Engl). (2017) 130:1639–47. doi: 10.4103/0366-6999.209906
106. Zou JG, Zhang J, Jia ZH, Cao KJ. Evaluation of the traditional Chinese medicine Shensongyangxin capsule on treating premature ventricular contractions: a randomized, double-blind, controlled multicenter trial. Chin Med J (Engl). (2011) 124:76–83.
107. Hua W, Gao RL, Zhao BC, Wang J, Chen XH, Cai C, et al. The efficacy and safety of Wenxin Keli in patients with frequent premature ventricular contractions: a randomized, double-blind, placebo-controlled, parallel-group, multicenter trial. Chin Med J (Engl). (2015) 128:2557–64. doi: 10.4103/0366-6999.166026
108. Ma Y-L, Hu R-M, Yang X, Wang T, Noble PJ, Wilkins R, et al. Investigation of the cellular pharmacological mechanism and clinical evidence of the multi-herbal antiarrhythmic Chinese medicine Xin Su Ning. Front Pharmacol. (2020) 11:600. doi: 10.3389/fphar.2020.00600
109. Dong J, Ma X, Wei Q, Peng S, Zhang S. Effects of growing location on the contents of secondary metabolites in the leaves of four selected superior clones of Eucommia ulmoides. Ind Crops Products. (2011) 34:1607–14. doi: 10.1016/j.indcrop.2011.06.007
110. Mullane K, Enna S, Piette J, Williams M. Guidelines for Manuscript Submission in the Peer-Reviewed Pharmacological Literature. London: Elsevier (2015). doi: 10.1016/j.bcp.2015.06.023
111. Wagner H, Ulrich-Merzenich G. Synergy research: approaching a new generation of phytopharmaceuticals. Phytomedicine. (2009) 16:97–110. doi: 10.1016/j.phymed.2008.12.018
112. Yuan H, Ma Q, Cui H, Liu G, Zhao X, Li W, et al. How can synergism of traditional medicines benefit from network pharmacology? Molecules. (2017) 22:1135. doi: 10.3390/molecules22071135
Keywords: cardiac arrhythmias, antiarrhythmic agents, plant extracts, phytochemicals, herbal medicine
Citation: Soltani D, Azizi B, Rahimi R, Talasaz AH, Rezaeizadeh H and Vasheghani-Farahani A (2022) Mechanism-based targeting of cardiac arrhythmias by phytochemicals and medicinal herbs: A comprehensive review of preclinical and clinical evidence. Front. Cardiovasc. Med. 9:990063. doi: 10.3389/fcvm.2022.990063
Received: 09 July 2022; Accepted: 14 September 2022;
Published: 29 September 2022.
Edited by:
Mirza Muhammad Faran Ashraf Baig, The University of Hong Kong, Hong Kong SAR, ChinaReviewed by:
Antonio Zaza, University of Milano-Bicocca, ItalyMaria Sancho, University of Vermont, United States
Copyright © 2022 Soltani, Azizi, Rahimi, Talasaz, Rezaeizadeh and Vasheghani-Farahani. This is an open-access article distributed under the terms of the Creative Commons Attribution License (CC BY). The use, distribution or reproduction in other forums is permitted, provided the original author(s) and the copyright owner(s) are credited and that the original publication in this journal is cited, in accordance with accepted academic practice. No use, distribution or reproduction is permitted which does not comply with these terms.
*Correspondence: Roja Rahimi, cm9qYXJhaGltaUBnbWFpbC5jb20=; Ali Vasheghani-Farahani, YXZhc2hlZ2hhbmlAdHVtcy5hYy5pcg==
†These authors have contributed equally to this work