- 1Cardiovascular Research Center, The Affiliated Suzhou Hospital of Nanjing Medical University, Suzhou Municipal Hospital, Gusu School, Nanjing Medical University, Suzhou, China
- 2Department of Cardiology, The First Affiliated Hospital of Nanjing Medical University, Nanjing Medical University, Nanjing, China
- 3Department of Genetics, University Medical Center Groningen, University of Groningen, Groningen, Netherlands
Background: Genetic, observational, and clinical intervention studies indicate that circulating levels of remnant cholesterol (RC) are associated with cardiovascular diseases. However, the predictive value of RC for cardiovascular mortality in the general population remains unclear.
Methods: Our study population comprised 19,650 adults in the United States from the National Health and Nutrition Examination Survey (NHANES) (1999–2014). RC was calculated from non-high-density lipoprotein cholesterol (non-HDL-C) minus low-density lipoprotein cholesterol (LDL-C) determined by the Sampson formula. Multivariate Cox regression, restricted cubic spline analysis, and subgroup analysis were applied to explore the relationship of RC with cardiovascular mortality.
Results: The mean age of the study cohort was 46.4 ± 19.2 years, and 48.7% of participants were male. During a median follow-up of 93 months, 382 (1.9%) cardiovascular deaths occurred. In a fully adjusted Cox regression model, log RC was significantly associated with cardiovascular mortality [hazard ratio (HR) 2.82; 95% confidence interval (CI) 1.17–6.81]. The restricted cubic spline curve indicated that log RC had a linear association with cardiovascular mortality (p for non-linearity = 0.899). People with higher LDL-C (≥130 mg/dL), higher RC [≥25.7/23.7 mg/dL in males/females corresponding to the LDL-C clinical cutoff point (130 mg/dL)] and abnormal HDL-C (<40/50 mg/dL in males/females) levels had a higher risk of cardiovascular mortality (HR 2.18; 95% CI 1.13–4.21 in males and HR 2.19; 95% CI 1.24–3.88 in females) than the reference group (lower LDL-C, lower RC and normal HDL-C levels).
Conclusions: Elevated RC levels were associated with cardiovascular mortality independent of traditional risk factors.
Introduction
Abnormal lipid metabolism plays a key role in atherosclerosis, causing adverse cardiovascular events (1–4). A reduction in the level of low-density lipoprotein cholesterol (LDL-C) was thought to diminish the morbidity and mortality of cardiovascular events. However, it is apparent that there is a residual risk not attributable to LDL-C or other known risk factors (5).
Remnant cholesterol (RC), which is defined as the cholesterol present in triglyceride-rich remnant lipoproteins (TRLs) (6), has been demonstrated to be associated with atherosclerosis development and total mortality in several observational studies (7–9). About 1/3 of the cholesterol load carried by apolipoprotein B (apoB) containing lipoprotein particles is transported via remnant particles in non-fasting conditions. And, cardiovascular risk remains high in statin-treated individuals, even with low LDL-C levels (10, 11). These suggest that RC, rather than LDL-C, is an independent risk factor for cardiovascular diseases (CVDs).
However, there are significant hurdles in investigating and quantifying the contribution of RC to atherogenesis (12). The spectrum of TRLs in the circulation is heterogenous, and there is no definitive biomarker that permits the unequivocal quantitation of remnant levels. This is partly because TRLs in the circulation are modified by metabolic processes (13). Accordingly, it is reasonable to calculate RC by using other available lipid data. The calculation of LDL-C is an essential step. Recently, various methods have been used for determining LDL-C. However, direct methods of LDL-C measurement, such as the β-quantification procedure, require specialized equipment and a large volume of serum. In contrast to ultracentrifugation, other methods of isolating LDL-C use proprietary chemicals that lack standardization, adding time and expense to the process (14).
The National Health and Nutrition Examination Survey (NHANES) is a continuous program administered by the National Center for Health Statistics (NCHS), which is responsible for the nation's health and vital statistics. However, the National Death Index record linked to NHANES is updated only to December 31, 2015, and LDL-C was determined by the Friedewald equation instead of direct tests. By this method, the RC value is essentially one-fifth of the triglyceride (TG) value, and other components are not counted (15). Thus, estimating LDL-C accurately is imperative. Indirect methods for estimating LDL-C, such as the Martin equation, are recommended by guidelines (16). Unfortunately, LDL-C values derived from the Martin equation are inaccurate with TG values ≥400 mg/dL (17). In 2020, Sampson et al. developed a new formula for calculating LDL-C, the Sampson formula. Even when the TG level reached 800 mg/dL, the measurement results were almost consistent with direct methods and had superior accuracy to other indirect methods (18).
To our knowledge, the association of RC and long-term cardiovascular deaths has not yet been studied in the general population. Therefore, using clinical data from NHANES, we assessed the association of RC with cardiovascular mortality in the U.S. adult population.
Methods
Study design and population
Using a complex sampling design, the NHANES sample represents the non-institutionalized civilian population of the U.S. There were 82,091 participants in the survey cycles from 1999 to 2014. We included participants with available data on total cholesterol (TC), high-density lipoprotein cholesterol (HDL-C) and triglycerides (TGs) (n = 55,242). After the exclusion of participants aged <18 years (n = 6,274), those with cancer at baseline (n = 903) and those with missing follow-up data (n = 22), 19,650 individuals were enrolled for further analysis (Figure 1). All participants provided informed consent, and the program passed ethical review.
Assessment of exposure
TC and TG were measured enzymatically, while HDL-C was quantified via the heparin-manganese precipitation method or direct immunoassay technique (19). LDL-C was estimated by the Sampson formula (18): LDL—C (mg/dL) = (TC/0.948) — (HDL—C/0.971) — [(TG/8.56) + (TG × non- HDL—C/ 2,140) — (TG × TG/16,100)] — 9.44.RC = non- HDL—C — LDL—C = TC — HDL—C — LDL—C (10, 11). All blood samples were collected using standardized procedures, and lipid concentrations were measured utilizing a Hitachi 704 Analyzer (20).
Covariates
Demographic data, including age, sex and race, were collected by questionnaires. People who had smoked over 100 cigarettes during their lifetime were regarded as smokers (21). A history of present illness (e.g., heart failure, coronary artery disease and stroke) and medication treatment were obtained from self-reported personal interview data. Body mass index (BMI) was calculated using the following formula: weight (kg)/height squared (m2). Blood pressure (BP) was calculated by averaging three consecutive BP readings after the participants rested calmly for 5 min. Hypertension was defined as systolic BP ≥140 mmHg and/or diastolic BP ≥90 mmHg, a history of hypertension or use of antihypertensive drugs (22). Diabetes was defined as fasting blood glucose ≥7.0 mmol/L, hemoglobin A1c (HbA1c) ≥6.5% or a history of diabetes (23).
Ascertainment of outcomes
The outcome of interest was cardiovascular mortality, defined as death caused by CVD or cerebrovascular disease. The death status and cause of death were identified by linking the National Death Index record to NHANES (reference for death registry). Follow-up lasted from the date of survey participation to cardiovascular mortality, the drop-out date, or December 31, 2015, whichever came first.
Statistical analysis
Continuous and categorical variables were expressed as the mean ± standard deviation and numbers with percentages, respectively. Cox proportional hazards models were constructed to explore the association between the RC level (log10-transformed) and cardiovascular death. Model 1 was a crude model without adjustment for confounders. Age, sex, race, and use of medications were adjusted in model 2. Model 3 was additionally adjusted by several traditional cardiovascular risk factors, including SBP, coronary heart disease, stroke and smoking status. Considering that LDL-C and HDL-C are also important predictors of cardiovascular mortality, we constructed additional models. Models 4 and 5 were further adjusted by HDL-C and LDL-C, respectively. We incorporated both in model 6. Variables such as obesity (i.e., BMI) and diabetes, which are implicated in the causal pathway in rising RC levels, were not included in the models, nor were variables closely associated with the diagnosis of diabetes, including fasting blood glucose and HbA1c. For subgroup analysis, the results stratified by age, sex, diabetes, hypertension, and smoking status from the fully adjusted regression models were tested. Restricted cubic splines were used to explore the potential non-linear relationship of log RC with cardiovascular mortality in the total population and different subgroups. Multiple imputation was used to replace missing values. Statistical analyses were performed via R version 3.5.3. A P-value < 0.05 was regarded as statistically significant.
Results
Baseline characteristics
A total of 19,650 participants were ultimately included in this study (Table 1). The mean age was 46.4 ± 19.2 years, and 48.7% of participants were male. The median follow-up was 93 months, and 382 (1.9%) incident cardiovascular deaths occurred. Individuals with cardiovascular events were older, had higher SBP, HbA1c and fasting blood glucose levels, and accounted for a larger proportion of the diabetes, hypertension and antihypertensive and lipid-lowering drug use groups (P < 0.001) than those without cardiovascular events. In addition, they had higher TG and TC levels but lower HDL-C levels. Significantly, people who died of cardiovascular events had higher RC levels than other participants (P < 0.001).
Association of RC with cardiovascular mortality
After full adjustment for confounders as well as several traditional cardiovascular risk factors, our continuous analysis revealed a significant association between log RC levels (HR 2.82; 95% CI 1.17–6.81) and cardiovascular mortality. By comparison, the association of HDL-C and cardiovascular mortality was attenuated after adjustment for the same factors along with LDL-C (Table 2). Notably, RC was still associated with incident cardiovascular deaths despite adjustment for the risk factors directly related to the Sampson equation, including both HDL-C and LDL-C (all p < 0.05), although the significance was attenuated after additional adjustment (Table 3). The restricted cubic spline curve showed that log RC was linearly associated with cardiovascular mortality in the general population (p for non-linearity = 0.899) (Figure 2A).
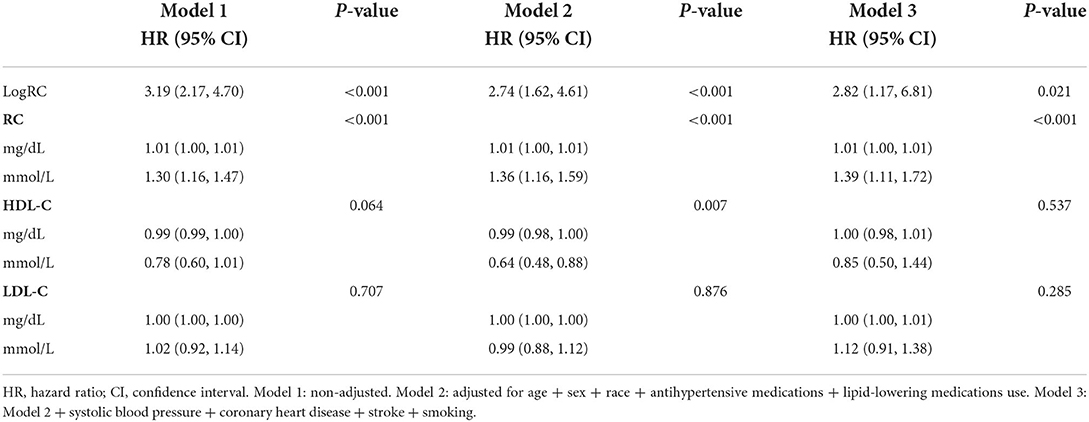
Table 2. Cox models for cardiovascular mortality for logRC, RC, HDL-C, LDL-C (continuous variables) in the pooled cohort.
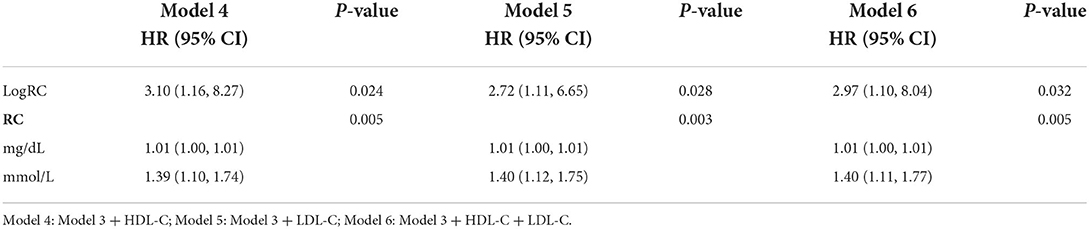
Table 3. Cox models for cardiovascular mortality for logRC, RC (continuous variables) after additional adjustment in the pooled cohort.
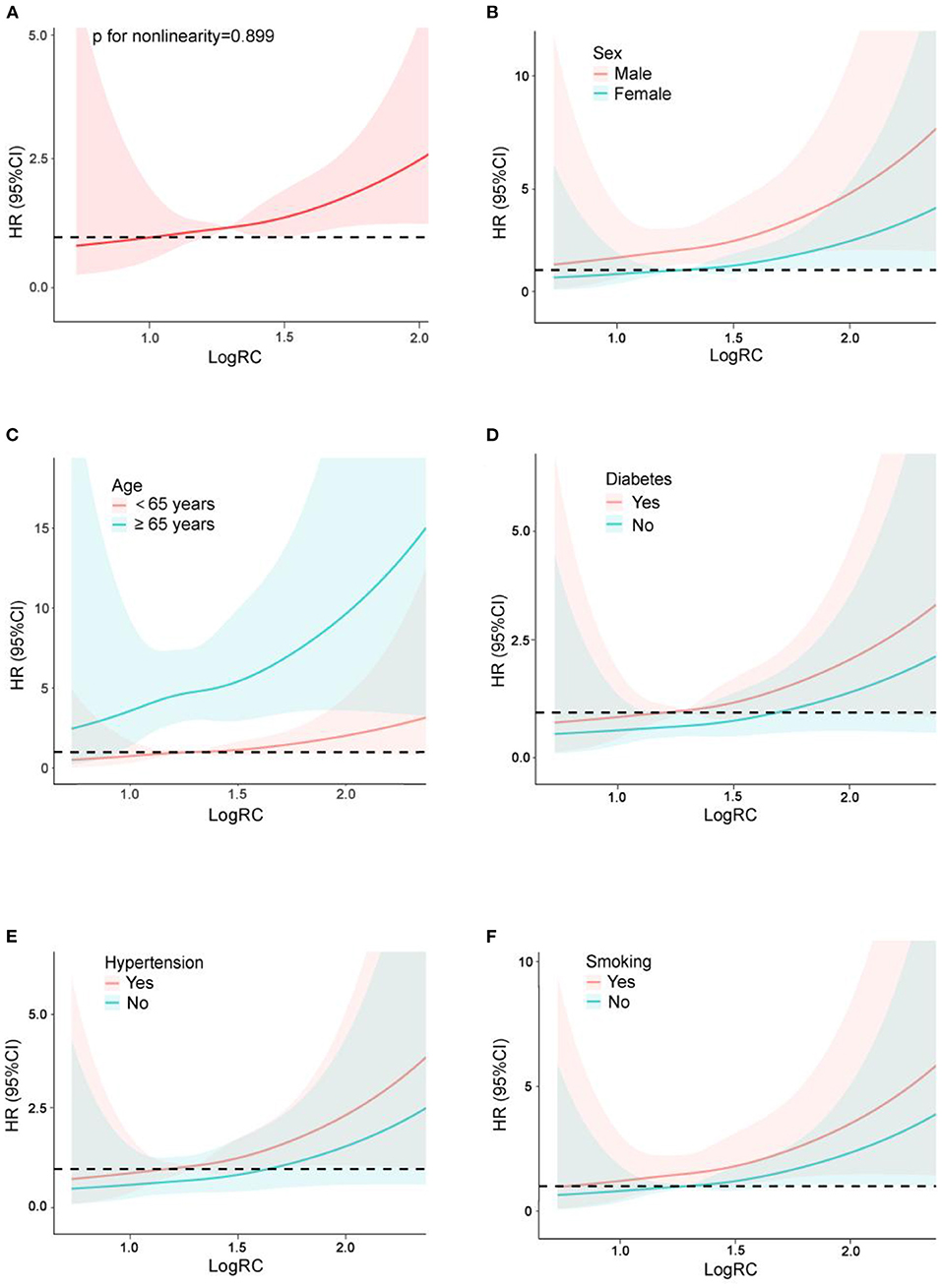
Figure 2. Restricted cubic spline plots of the association between log10-transformed RC and cardiovascular mortality in the general population (A) and subgroup analysis by sex (B), age (C), diabetes (D), hypertension (E) and smoking (F). Analysis was adjusted for age + sex + race + antihypertensive medications + lipid-lowering medications use + systolic blood pressure + coronary heart disease + stroke + smoking. HR, hazard ratio; CI, confidence interval.
Subgroup analysis
Analysis stratified by sex, age, diabetes, hypertension and smoking showed further evidence of a relationship between RC and cardiovascular mortality (Table 4). Males, participants over 65 years old, individuals with diabetes and hypertension, and smokers had a higher risk for cardiovascular death after full adjustment for several traditional cardiovascular risk factors (all p < 0.01). In addition, there were significant effects of the interactions of RC (p for interaction = 0.025) and log RC (p for interaction < 0.001) with age on cardiovascular mortality, and the population over 65 years old had a stronger association between log RC and cardiovascular mortality than that age 65 and younger. As shown by restricted cubic spline plots, there was a linear relationship between log RC and cardiovascular mortality in the subgroups stratified by sex, age, diabetes, hypertension and smoking (Figures 2B–F). This linear relationship was also found in population over 65 years old by sex (Figure 3A), but was not significant in those below 65 years old (Figure 3B).
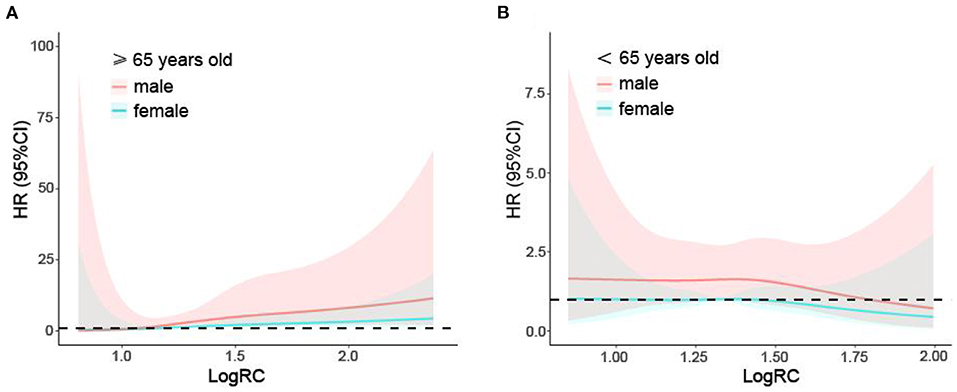
Figure 3. Restricted cubic spline plots of the association between log10-transformed RC and cardiovascular mortality in the general population over (A) and below 65 years (B) by sex. Analysis was adjusted for age + sex + race + antihypertensive medications + lipid-lowering medications use + systolic blood pressure + coronary heart disease + stroke + smoking. HR, hazard ratio; CI, confidence interval.
Hazard ratios for cardiovascular events in different lipid groups
Corresponding to the LDL-C clinical cutoff point (130 mg/dL), the respective RC cutoff points were identified using equivalent percentiles and found to be 25.7 and 23.7 mg/dL in the male and female groups. The HDL-C clinical cutoff points were 40 and 50 mg/dL in the male and female groups. Compared with the reference group (lower LDL-C, lower RC and normal HDL-C levels), people with higher LDL-C, higher RC and abnormal HDL-C levels had a higher risk of cardiovascular mortality (HR 2.18; 95% CI 1.13–4.21 in men and HR 2.19; 95% CI 1.24–3.88 in women) (Table 5). In the case of normal HDL-C levels, the hazard of incident cardiovascular deaths of the male group with lower LDL-C and higher RC levels was also significantly different from that of the reference group (HR 2.34; 95% CI 1.29–4.24), as well as that of the female group with higher LDL-C and higher RC levels (HR 1.92; 95% CI 1.07–3.44). Notably, the female group with lower LDL-C, abnormal HDL-C and higher RC levels also had an increased hazard of cardiovascular mortality (HR 2.49; 95% CI 1.49–4.17). However, a larger sample size and more events in each category would improve precision.
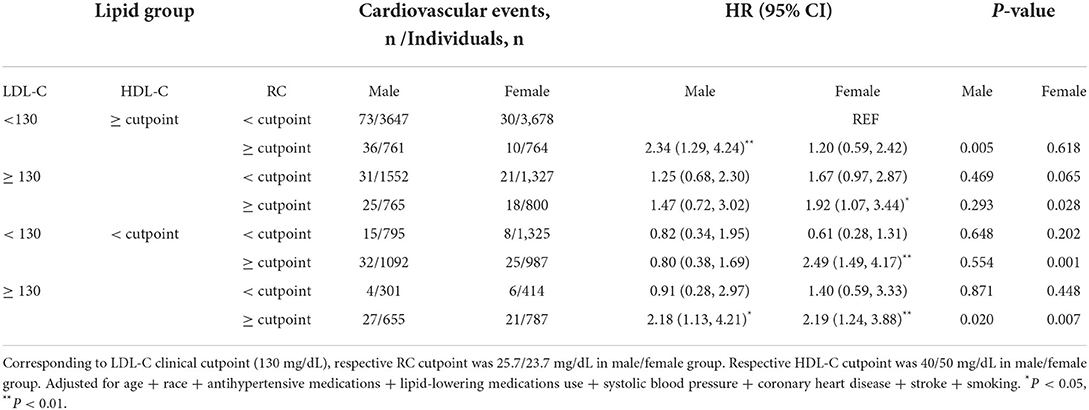
Table 5. Hazard ratios (95% confidence interval) and P-value for cardiovascular events of different lipid groups.
Discussion
This is the first study using NHANES to reveal an independent association between RC and cardiovascular deaths in the general U.S. adult population, according to our results. In addition, RC showed a linear relationship with cardiovascular mortality that was more pronounced in males. Our results for patients with diabetes and hypertension, individuals over 65 years old, and smokers confirmed previous evidence on the association between RC and incident cardiovascular deaths, especially in people with higher LDL-C, higher RC and abnormal HDL-C levels.
After being remodeled in the systemic circulation, very low-density lipoproteins (VLDLs) undergo hydrolysis by the enzyme lipoprotein lipase (LPL), transforming into LDL, as well as intermediate-density lipoproteins (IDLs). RC represents the cholesterol content of the TRLs, which are made up of VLDL and IDL in the fasting state and chylomicron remnants in the non-fasting state. Overproduction of TRLs (24) (chylomicrons from the gut and VLDL from the liver) reduces the activity of lipolysis by LPL, which results in an accumulation of partially metabolized remnant particles. Particle for particle, these remnants exhibit an atherogenic potential similar to that of LDL particles (25), but they contain 40 times (26) more cholesterol. It can be suggested that the increased cardiovascular mortality risk associated with RC is caused by the mechanisms described before, which is related to local inflammation and plaque formation (27, 28). A higher concentration of RC in serum is more likely to penetrate into the arterial wall, where macrophages easily trap and absorb the RC, causing foam cells to form faster than they do with LDL (29). By hydrolyzing TRLs, RC induces the production of cytokines and interleukins, and proatherogenic adhesion molecules are released, activating inflammation and the coagulation cascade (30, 31). The combination of all these factors above may result in plaque rupture and ultimately, cardiovascular mortality.
Observational (3, 32) and genetic studies (27, 33, 34) have demonstrated an association between RC and cardiovascular outcomes. The fact that RC is causally associated with CVD and low-grade inflammation is now clear, regardless of fasting status, definition, and form of assessment (35, 36). Notably, however, the outcome in this study reflected cardiovascular mortality rather than just incident CVD, indicating that fatal rather than all cardiovascular events differed from those of previous studies. One interpretation for this is that our study included subjects with TG ≥400 mg/dL, and incident cardiovascular deaths in this population were reasonably counted. It would be more logical to estimate the RC value with the Sampson formula rather than Friedewald's equation because the LDL-C value computed by the latter is missing or inaccurate in this case (16). Another interpretation could be that most observational studies tended to miss a certain amount of cardiovascular deaths due to limited follow-up time or confined populations.
In previous cohort studies of obese elderly participants, RC ≥30 mg/dL distinguished individuals with a higher hazard of major adverse cardiovascular events (MACEs) than those with lower RC concentrations, irrespective of LDL-C levels (10). In this study, similar analyses were carried out. Those with higher RC and LDL-C and lower HDL-C concentrations faced the highest risk. This implied a complex interaction among RC, LDL-C and HDL-C. In addition to reversing cholesterol transport, HDL-C is also antioxidative and anti-inflammatory (37). However, recent epidemiological studies have demonstrated that the relationship between HDL-C and all-cause mortality and cardiovascular mortality is U-shaped in some specific populations (38–40). This could also partly explain why a similar situation occurred in our study when comparing the cardiovascular death risk of different lipid groups.
Various methods have been proposed for measuring and defining RC, including ultracentrifugation and immunoseparation (41). These methods, however, have been questioned for their accuracy, as remnant lipoproteins contain different apolipoprotein and lipid compositions. It has been proven difficult to measure RC directly because it takes a long time and requires special equipment. In 1990, NMRS (nuclear magnetic resonance spectroscopy, NMRS) was first used for the measuring of blood lipid components, and was subsequently standardized and used for the measuring of clinical blood lipid subgroup profiles. The principle is to obtain blood lipid profile levels by analyzing the specific amplitudes of different lipid methyl groups. NMRS can determine the number, density, size and composition of lipoproteins, and the results of NMRS are not easily affected by changes in lipoprotein composition (42). However, this method still has limitations. First, since NMRS is sensitive to drastic changes in the matrix, changes in ionic strength and pH can both affect the chemical shifts of lipid signals. Therefore, the detection should be completed within 4 h after blood collection (43). Secondly, if the laboratory does not have the conditions for testing, the plasma must be separated within the specified time and sent for inspection after cryopreservation. The freezing and thawing of the samples cannot be guaranteed during the storage and transportation of the plasma samples, and the accuracy of the test results may be affected (44), and finally, the NMR instrument is expensive, and it is still difficult to promote clinically. As a result, these methods cannot be used routinely (36). Comparatively, RC can be calculated as TC−HDL−C−LDL−C, as has been done in several large cohort studies (10, 11). Thus, accurately estimating LDL-C is crucial. The new Sampson formula reported by Sampson et al. is a better alternative for estimating LDL-C than conventional methods (18). Quite a few studies proved that Sampson's formula is more accurate than Friedewald's formula, even when TG is above 800 mg/dL (45, 46). Given the above reasons, it is reasonable to estimate RC using the Sampson formula. We found that RC significantly increased the risks of cardiovascular mortality, independent of both LDL-C and HDL-C, even after adjusting for lipid-lowering drugs and other confounders, and as a result, it would probably be more beneficial to use RC-targeted therapy than to further reduce LDL-C levels in high-risk subjects for whom statin treatment is not appropriate or who are already taking high- or moderate-dose statins (47–49). According to a recent study, lowering RC by 32 mg/dl can reduce recurrent MACEs by 20% in secondary prevention (50). There are certain therapeutic strategies available to lower RC levels by reducing TRL levels (12). Treatment with high-intensity statins limits triglyceride levels, whereas fibrates are more effective in lowering triglyceride levels and reducing cardiovascular hazards in people with atherogenic dyslipidemia (51–53). When statins and ezetimibe doses are optimized or intolerable, PCSK9 inhibitors may be used, but their triglyceride-lowering ability is modest (54, 55). On the other hand, high doses of omega-3 fatty acids, notably icosapent ethyl (54), and newer medications, such as RNA-based antisense-oligonucleotide inhibitors of apolipoprotein C-III and angiopoietin-like 3 genes (55, 56), markedly reduce TRL levels. Nonetheless, it must be determined whether this approach is superior to a more intensive LDL-C lowering strategy for CVD prevention, especially when risk-specific LDL-C targets are achieved in subjects with elevated TG levels at high CVD risk.
There are several limitations of our study. First, some variables were self-reported, which might cause recall bias. Second, despite adjusting for covariates, we cannot exclude the residual confounding effects from variables that were not measured or included, such as lipoprotein (a), apoA, apoB and apoE, due to limited data from the NHANES. Third, there may still be competing risks with other causes of mortality such as car accidents, although we have excluded cancer patients. Fourth, since the data were retrieved from a database of individuals from the U.S., they might not apply to other populations or regions. Fifth, this study was observational, and the predictive value of RC for cardiovascular mortality needs to be evaluated further in clinical studies. Finally, compared with direct measurement, our study might overestimate the value of RC due to the indirect method. Nevertheless, the indirect calculation of RC can provide valuable information for clinical management since it is affordable and inexpensive.
Conclusions
We found that elevated RC levels increased cardiovascular mortality in the general population, independent of traditional cardiovascular risk factors, HDL-C and LDL-C. It may be more pragmatic to identify RC residual risk, as HDL-C boosting therapies have failed over the past few years and the era of targeted RC-lowering therapies is coming. A consensus on the most viable and cost-effective measurement method must be reached before RC is widely used in routine clinical practice. In the future, it will be necessary to demonstrate the mechanisms underlying the association between RC and cardiovascular mortality in addition to the total atherogenic particle concentration and to clarify the cardiovascular benefits of RC-targeted therapy.
Data availability statement
The original contributions presented in the study are included in the article/supplementary material, further inquiries can be directed to the corresponding author/s.
Ethics statement
The studies involving human participants were reviewed and approved by the Ethics Review Board of National Center for Health Statistics. The patients/participants provided their written informed consent to participate in this study.
Author contributions
KZ contributed to formal analysis and writing-original draft of the manuscript. XQ and FZ were responsible for software and data curation. QD performed visualization. ZG, FW, and LX were responsible for methodology and investigation. ML contributed to validation. LC and YW contributed discussion and edited manuscript. HZ revised manuscript. YS and KZ contributed to conceptualization. XK designed and supervised the project. All authors contributed to the article and approved the submitted version.
Funding
This paper was funded by the Science Foundation of Gusu School (GSKY20210105) and the Natural Science Foundation of Jiangsu Province (BK2012648). The funding body played no role in the design, writing, or decision to publish this paper.
Acknowledgments
The authors would like to thank the workforce and participants of the NHANES study for their valuable contributions.
Conflict of interest
The authors declare that the research was conducted in the absence of any commercial or financial relationships that could be construed as a potential conflict of interest.
Publisher's note
All claims expressed in this article are solely those of the authors and do not necessarily represent those of their affiliated organizations, or those of the publisher, the editors and the reviewers. Any product that may be evaluated in this article, or claim that may be made by its manufacturer, is not guaranteed or endorsed by the publisher.
References
1. Varbo A, Nordestgaard BG. Nonfasting triglycerides, low-density lipoprotein cholesterol, and heart failure risk: two cohort studies of 113 554 individuals. Arterioscler Thromb Vasc Biol. (2018) 38:464–72. doi: 10.1161/ATVBAHA.117.310269
2. Joshi PH, Khokhar AA, Massaro JM, Lirette ST, Griswold ME, Martin SS, et al. Remnant lipoprotein cholesterol and incident coronary heart disease: the jackson heart and framingham offspring cohort studies. J Am Heart Assoc. (2016) 5:e002765. doi: 10.1161/JAHA.115.002765
3. Chapman MJ, Ginsberg HN, Amarenco P, Andreotti F, Borén J, Catapano AL, et al. Triglyceride-rich lipoproteins and high-density lipoprotein cholesterol in patients at high risk of cardiovascular disease: evidence and guidance for management. Eur Heart J. (2011) 32:1345–61. doi: 10.1093/eurheartj/ehr112
4. Brunzell JD, Davidson M, Furberg CD, Goldberg RB, Howard BV, Stein JH, et al. Lipoprotein management in patients with cardiometabolic risk: consensus conference report from the American diabetes association and the American college of cardiology foundation. J Am Coll Cardiol. (2008) 51:1512–24. doi: 10.1016/j.jacc.2008.02.034
5. Shapiro MD, Fazio S. From lipids to inflammation: new approaches to reducing atherosclerotic risk. Circ Res. (2016) 118:732–49. doi: 10.1161/CIRCRESAHA.115.306471
6. Varbo A, Nordestgaard BG. Remnant lipoproteins. Curr Opin Lipidol. (2017) 28:300–7. doi: 10.1097/MOL.0000000000000429
7. Jepsen AM, Langsted A, Varbo A, Bang LE, Kamstrup PR, Nordestgaard BG. Increased remnant cholesterol explains part of residual risk of all-cause mortality in 5414 patients with ischemic heart disease. Clin Chem. (2016) 62:593–604. doi: 10.1373/clinchem.2015.253757
8. Varbo A, Benn M, Tybjærg-Hansen A, Nordestgaard BG. Elevated remnant cholesterol causes both low-grade inflammation and ischemic heart disease, whereas elevated low-density lipoprotein cholesterol causes ischemic heart disease without inflammation. Circulation. (2013) 128:1298–309. doi: 10.1161/CIRCULATIONAHA.113.003008
9. Elshazly MB, Mani P, Nissen S, Brennan DM, Clark D, Martin S, et al. Remnant cholesterol, coronary atheroma progression and clinical events in statin-treated patients with coronary artery disease. Eur J Prev Cardiol. (2020) 27:1091–100. doi: 10.1177/2047487319887578
10. Castañer O, Pintó X, Subirana I, Amor AJ, Ros E, Hernáez Á, et al. Remnant cholesterol, Not LDL cholesterol, is associated with incident cardiovascular disease. J Am Coll Cardiol. (2020) 76:2712–24. doi: 10.1016/j.jacc.2020.10.008
11. Quispe R, Martin SS, Michos ED, Lamba I, Blumenthal RS, Saeed A, et al. Remnant cholesterol predicts cardiovascular disease beyond LDL and ApoB: a primary prevention study. Eur Heart J. (2021) 42:4324–32. doi: 10.1093/eurheartj/ehab432
12. Laufs U, Parhofer KG, Ginsberg HN, Hegele RA. Clinical review on triglycerides. Eur Heart J. (2020) 41:99–109c. doi: 10.1093/eurheartj/ehz785
13. Borén J, Packard CJ. Keeping remnants in perspective. Eur Heart J. (2021) 42:4333–5. doi: 10.1093/eurheartj/ehab531
14. Brownstein AJ, Martin SS. More accurate LDL-C calculation: externally validated, guideline endorsed. Clin Chim Acta. (2020) 506:149–53. doi: 10.1016/j.cca.2020.03.030
15. Szili-Torok T, Tietge UJF. Remnant cholesterol is just another word for triglycerides when calculating LDL-C using the friedewald formula. J Am Coll Cardiol. (2021) 77:1841. doi: 10.1016/j.jacc.2021.01.053
16. Wilson PWF, Jacobson TA, Martin SS, Jackson EJ, Le NA, Davidson MH, et al. Lipid measurements in the management of cardiovascular diseases: practical recommendations a scientific statement from the national lipid association writing group. J Clin Lipidol. (2021) 15:629–48. doi: 10.1016/j.jacl.2021.09.046
17. Martin SS, Blaha MJ, Elshazly MB, Toth PP, Kwiterovich PO, Blumenthal RS, et al. Comparison of a novel method vs the friedewald equation for estimating low-density lipoprotein cholesterol levels from the standard lipid profile. JAMA. (2013) 310:2061–8. doi: 10.1001/jama.2013.280532
18. Sampson M, Ling C, Sun Q, Harb R, Ashmaig M, Warnick R, et al. A new equation for calculation of low-density lipoprotein cholesterol in patients with normolipidemia and/or hypertriglyceridemia. JAMA Cardiol. (2020) 5:540–8. doi: 10.1001/jamacardio.2020.0013
19. Bucholz EM, Rodday AM, Kolor K, Khoury MJ, de Ferranti SD. Prevalence and predictors of cholesterol screening, awareness, and statin treatment among US adults with familial hypercholesterolemia or other forms of severe dyslipidemia (1999-2014). Circulation. (2018) 137:2218–30. doi: 10.1161/CIRCULATIONAHA.117.032321
20. Doran B, Guo Y, Xu J, Weintraub H, Mora S, Maron DJ, et al. Prognostic value of fasting versus nonfasting low-density lipoprotein cholesterol levels on long-term mortality: insight from the national health and nutrition examination survey III (NHANES-III). Circulation. (2014) 130:546–53. doi: 10.1161/CIRCULATIONAHA.114.010001
21. Jiang M, Sun J, Zou H, Li M, Su Z, Sun W, et al. Prognostic role of neutrophil to high-density lipoprotein cholesterol ratio for all-cause and cardiovascular mortality in the general population. Front Cardiovasc Med. (2022) 9:807339. doi: 10.3389/fcvm.2022.807339
22. Williams B, Mancia G, Spiering W, Agabiti Rosei E, Azizi M, Burnier M, et al. 2018 ESC/ESH guidelines for the management of arterial hypertension. Eur Heart J. (2018) 39:3021–104. doi: 10.1097/HJH.0000000000001940
23. American Diabetes Association. Classification and diagnosis of diabetes: standards of medical care in diabetes-2020. Diabetes Care. (2020) 43:S14–31. doi: 10.2337/dc20-S002
24. Nordestgaard BG, Varbo A. Triglycerides and cardiovascular disease. Lancet. (2014) 384:626–35. doi: 10.1016/S0140-6736(14)61177-6
25. Ference BA, Kastelein JJP, Ray KK, Ginsberg HN, Chapman MJ, Packard CJ, et al. Association of triglyceride-lowering LPL variants and LDL-C-lowering LDLR variants with risk of coronary heart disease. JAMA. (2019) 321:364–73. doi: 10.1001/jama.2018.20045
26. Borén J, Williams KJ. The central role of arterial retention of cholesterol-rich apolipoprotein-B-containing lipoproteins in the pathogenesis of atherosclerosis: a triumph of simplicity. Curr Opin Lipidol. (2016) 27:473–83. doi: 10.1097/MOL.0000000000000330
27. Thomsen M, Varbo A, Tybjærg-Hansen A, Nordestgaard BG. Low nonfasting triglycerides and reduced all-cause mortality: a mendelian randomization study. Clin Chem. (2014) 60:737–46. doi: 10.1373/clinchem.2013.219881
28. Varbo A, Nordestgaard BG. Remnant cholesterol and ischemic heart disease. Curr Opin Lipidol. (2014) 25:266–73. doi: 10.1097/MOL.0000000000000093
29. Miller YI, Choi SH, Fang L, Tsimikas S. Lipoprotein modification and macrophage uptake: role of pathologic cholesterol transport in atherogenesis. Subcell Biochem. (2010) 51:229–51. doi: 10.1007/978-90-481-8622-8_8
30. Olufadi R, Byrne CD. Effects of VLDL and remnant particles on platelets. Pathophysiol Haemost Thromb. (2006) 35:281–91. doi: 10.1159/000093221
31. Shin HK, Kim YK, Kim KY, Lee JH, Hong KW. Remnant lipoprotein particles induce apoptosis in endothelial cells by NAD(P)H oxidase-mediated production of superoxide and cytokines via lectin-like oxidized low-density lipoprotein receptor-1 activation: prevention by cilostazol. Circulation. (2004) 109:1022–8. doi: 10.1161/01.CIR.0000117403.64398.53
32. Varbo A, Freiberg JJ, Nordestgaard BG. Extreme nonfasting remnant cholesterol vs. extreme LDL cholesterol as contributors to cardiovascular disease and all-cause mortality in 90000 individuals from the general population. Clin Chem. (2015) 61:533–43. doi: 10.1373/clinchem.2014.234146
33. Helgadottir A, Gretarsdottir S, Thorleifsson G, Hjartarson E, Sigurdsson A, Magnusdottir A, et al. Variants with large effects on blood lipids and the role of cholesterol and triglycerides in coronary disease. Nat Genet. (2016) 48:634–9. doi: 10.1038/ng.3561
34. Do R, Willer CJ, Schmidt EM, Sengupta S, Gao C, Peloso GM, et al. Common variants associated with plasma triglycerides and risk for coronary artery disease. Nat Genet. (2013) 45:1345–52. doi: 10.1038/ng.2795
35. Varbo A, Benn M, Tybjærg-Hansen A, Jørgensen AB, Frikke-Schmidt R, Nordestgaard BG. Remnant cholesterol as a causal risk factor for ischemic heart disease. J Am Coll Cardiol. (2013) 61:427–36. doi: 10.1016/j.jacc.2012.08.1026
36. Varbo A, Benn M, Nordestgaard BG. Remnant cholesterol as a cause of ischemic heart disease: evidence, definition, measurement, atherogenicity, high risk patients, and present and future treatment. Pharmacol Ther. (2014) 141:358–67. doi: 10.1016/j.pharmthera.2013.11.008
37. Yang Y, Han K, Park SH, Kim MK, Yoon KH, Lee SH. High-Density lipoprotein cholesterol and the risk of myocardial infarction, stroke, and cause-specific mortality: a nationwide cohort study in Korea. J Lipid Atheroscl. (2021) 10:74–87. doi: 10.12997/jla.2021.10.1.74
38. Bowe B, Xie Y, Xian H, Balasubramanian S, Zayed MA. Al-Aly Z. High density lipoprotein cholesterol and the risk of all-cause mortality among US veterans. Clin J Am Soc Nephrol. (2016) 11:1784–93. doi: 10.2215/CJN.00730116
39. Chen CL, Liu XC, Liu L, Lo K, Yu YL, Huang JY, et al. U-shaped association of high-density lipoprotein cholesterol with all-cause and cardiovascular mortality in hypertensive population. Risk Manag Healthc Policy. (2020) 13:2013–25. doi: 10.2147/RMHP.S272624
40. Li ZH, Lv YB, Zhong WF, Gao X, Byers Kraus V, Zou MC, et al. High-density lipoprotein cholesterol and all-cause and cause-specific mortality among the elderly. J Clin Endocrinol Metab. (2019) 104:3370–8. doi: 10.1210/jc.2018-02511
41. Faridi KF, Quispe R, Martin SS, Hendrani AD, Joshi PH, Brinton EA, et al. Comparing different assessments of remnant lipoprotein cholesterol: the very large database of lipids. J Clin Lipidol. (2019) 13:634–44. doi: 10.1016/j.jacl.2019.06.001
42. Blake GJ, Otvos JD, Rifai N, Ridker PM. Low-density lipoprotein particle concentration and size as determined by nuclear magnetic resonance spectroscopy as predictors of cardiovascular disease in women. Circulation. (2002) 106:1930–7. doi: 10.1161/01.CIR.0000033222.75187.B9
43. Tsai MY, Georgopoulos A, Otvos JD, Ordovas JM, Hanson NQ, Peacock JM, et al. Comparison of ultracentrifugation and nuclear magnetic resonance spectroscopy in the quantification of triglyceride-rich lipoproteins after an oral fat load. Clin Chem. (2004) 50:1201–4. doi: 10.1373/clinchem.2004.032938
44. Wang F, Debik J, Andreassen T, Euceda LR, Haukaas TH, Cannet C, et al. Effect of repeated freeze-thaw cycles on NMR-measured lipoproteins and metabolites in biofluids. J Proteome Res. (2019) 18:3681–8. doi: 10.1021/acs.jproteome.9b00343
45. Li J, Xin Y, Li J, Meng M, Zhou L, Qiu H, et al. Evaluation of Sampson equation for LDL-C in acute coronary syndrome patients: a Chinese population-based cohort study. Lipids Health Dis. (2022) 21:39. doi: 10.1186/s12944-022-01648-4
46. Sajja A, Park J, Sathiyakumar V, Varghese B, Pallazola VA, Marvel FA, et al. Comparison of methods to estimate low-density lipoprotein cholesterol in patients with high triglyceride levels. JAMA Network Open. (2021) 4:e2128817. doi: 10.1001/jamanetworkopen.2021.28817
47. Schwartz GG, Abt M, Bao W, DeMicco D, Kallend D, Miller M, et al. Fasting triglycerides predict recurrent ischemic events in patients with acute coronary syndrome treated with statins. J Am Coll Cardiol. (2015) 65:2267–75. doi: 10.1016/j.jacc.2015.03.544
48. Jørgensen AB, Frikke-Schmidt R, West AS, Grande P, Nordestgaard BG, Tybjærg-Hansen A. Genetically elevated non-fasting triglycerides and calculated remnant cholesterol as causal risk factors for myocardial infarction. Eur Heart J. (2013) 34:1826–33. doi: 10.1093/eurheartj/ehs431
49. Vallejo-Vaz AJ, Fayyad R, Boekholdt SM, Hovingh GK, Kastelein JJ, Melamed S, et al. Triglyceride-rich lipoprotein cholesterol and risk of cardiovascular events among patients receiving statin therapy in the TNT trial. Circulation. (2018) 138:770–81. doi: 10.1161/CIRCULATIONAHA.117.032318
50. Langsted A, Madsen CM, Nordestgaard BG. Contribution of remnant cholesterol to cardiovascular risk. J Intern Med. (2020) 288:116–27. doi: 10.1111/joim.13059
51. Jun M, Foote C, Lv J, Neal B, Patel A, Nicholls SJ, et al. Effects of fibrates on cardiovascular outcomes: a systematic review and meta-analysis. Lancet. (2010) 375:1875–84. doi: 10.1016/S0140-6736(10)60656-3
52. Lee M, Saver JL, Towfighi A, Chow J, Ovbiagele B. Efficacy of fibrates for cardiovascular risk reduction in persons with atherogenic dyslipidemia: a meta-analysis. Atherosclerosis. (2011) 217:492–8. doi: 10.1016/j.atherosclerosis.2011.04.020
53. Kim NH, Han KH, Choi J, Lee J, Kim SG. Use of fenofibrate on cardiovascular outcomes in statin users with metabolic syndrome: propensity matched cohort study. BMJ. (2019) 366:l5125. doi: 10.1136/bmj.l5125
54. Bhatt DL, Steg PG, Miller M, Brinton EA, Jacobson TA, Ketchum SB, et al. Cardiovascular risk reduction with icosapent ethyl for hypertriglyceridemia. N Engl J Med. (2019) 380:11–22. doi: 10.1056/NEJMoa1812792
55. Mäkinen P, Ruotsalainen AK, Ylä-Herttuala S. Nucleic acid-based therapies for atherosclerosis. Curr Atheroscler Rep. (2020) 22:10. doi: 10.1007/s11883-020-0826-2
Keywords: remnant cholesterol, Sampson formula, cardiovascular mortality, NHANES, correlational analysis
Citation: Zhang K, Qi X, Zhu F, Dong Q, Gou Z, Wang F, Xiao L, Li M, Chen L, Wang Y, Zhang H, Sheng Y and Kong X (2022) Remnant cholesterol is associated with cardiovascular mortality. Front. Cardiovasc. Med. 9:984711. doi: 10.3389/fcvm.2022.984711
Received: 02 July 2022; Accepted: 29 August 2022;
Published: 20 September 2022.
Edited by:
Nathalie Pamir, Oregon Health and Science University, United StatesReviewed by:
Matti Sakari Jauhiainen, Minerva Foundation Institute for Medical Research, FinlandPeter Penson, Liverpool John Moores University, United Kingdom
Copyright © 2022 Zhang, Qi, Zhu, Dong, Gou, Wang, Xiao, Li, Chen, Wang, Zhang, Sheng and Kong. This is an open-access article distributed under the terms of the Creative Commons Attribution License (CC BY). The use, distribution or reproduction in other forums is permitted, provided the original author(s) and the copyright owner(s) are credited and that the original publication in this journal is cited, in accordance with accepted academic practice. No use, distribution or reproduction is permitted which does not comply with these terms.
*Correspondence: Yanhui Sheng, yhsheng@njmu.edu.cn; Xiangqing Kong, kongxq@njmu.edu.cn
†These authors have contributed equally to this work