- 1Stanford Cardiovascular Institute, Stanford University School of Medicine, Stanford, CA, United States
- 2Division of Cardiovascular Medicine, Department of Medicine, Stanford University, Stanford, CA, United States
Immunosuppressive medications are widely used to treat patients with neoplasms, autoimmune conditions and solid organ transplants. Key drug classes, namely calcineurin inhibitors, mammalian target of rapamycin (mTOR) inhibitors, and purine synthesis inhibitors, have direct effects on the structure and function of the heart and vascular system. In the heart, immunosuppressive agents modulate cardiac hypertrophy, mitochondrial function, and arrhythmia risk, while in vasculature, they influence vessel remodeling, circulating lipids, and blood pressure. The aim of this review is to present the preclinical and clinical literature examining the cardiovascular effects of immunosuppressive agents, with a specific focus on cyclosporine, tacrolimus, sirolimus, everolimus, mycophenolate, and azathioprine.
Introduction
Medications that target and downregulate the immune system are utilized for the prevention and treatment of a variety of conditions, including neoplasms, autoimmune diseases, and acute rejection after solid organ transplantation (1). In a recent cohort, 2.8% of the adult population was treated with long-term immunosuppressive medications, consistent with prior self-reported estimates (2, 3). In addition to the well described increased risk of infection and malignancy in chronically immunosuppressed patients, many of these agents exhibit direct effects on the cardiovascular system including risk of left ventricular (LV) hypertrophy, myocardial fibrosis, arrhythmia, hypertension, dyslipidemia, and coronary atherosclerosis (4). Herein, we focus on the cardiovascular effects and mechanistic underpinnings of calcineurin inhibitors (CNI), mammalian target of rapamycin (mTOR) inhibitors, and purine synthesis inhibitors (Figure 1).
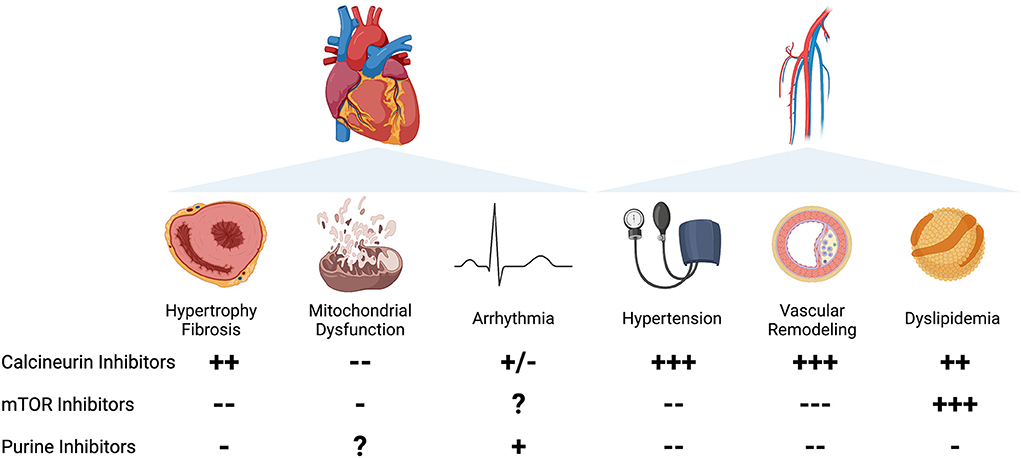
Figure 1. Left Panel: Cardiac effects of immunosuppression. Column A: Calcineurin inhibitors are associated with increased hypertrophy in clinical studies, with mixed preclinical evidence. mTOR inhibitors are associated with a decrease in cardiac hypertrophy in patients and animal studies. Purine synthesis inhibitors prevent cardiac remodeling in limited evidence in preclinical studies. Column B: Calcineurin inhibitors, particularly CsA, prevent mitochondrial dysfunction and mPTP opening. mTOR inhibitors may prevent mitochondrial dysfunction in preclinical studies. The effects of purine synthesis inhibitors on mitochondrial function in the heart are unknown. Column C: Calcineurin inhibitors are associated with arrhythmia in limited clinical case reports, with mixed effects in animal studies. The effects of mTOR inhibitors on arrhythmia are unknown. Purine synthesis inhibitors, particularly azathioprine, are weakly associated with increased atrial arrhythmias in clinical case reports. Right Panel: Vascular effects of immunosuppression. Column A: Hypertension. Calcineurin inhibitors are strongly associated with an increased incidence of hypertension in preclinical and clinical studies. mTOR inhibitors and purine synthesis inhibitors have a vasodilatory effect in animal models and limited clinical studies. Column B: Vascular remodeling. Calcineurin inhibitors are strongly associated with proliferative vasculopathy and vascular inflammation. mTOR inhibitors protect against vascular damage in clinical studies and preclinical models. Purine synthesis inhibitors are associated with improvement in vascular remodeling in preclinical studies and limited clinical reports. Column C: Dyslipidemia. Calcineurin inhibitors are associated with increased total serum cholesterol and LDL. mTOR inhibitors, particularly sirolimus, are strongly associated with an increase in serum cholesterol and triglycerides. Purine synthesis inhibitors are weakly associated with improvement in serum lipids.
Hypertrophy and fibrosis
Cardiac hypertrophy is a feature of adverse cardiac remodeling that may be driven by genetic or acquired factors. Hypertrophy is frequently seen in association with diastolic dysfunction and represents an important marker for adverse remodeling (5, 6). Much of the focus on immunosuppression-induced cardiac remodeling has been on the effects on cardiac hypertrophy in native or transplanted hearts (7–9) (Table 1).
Calcineurin inhibitors
Calcineurin, a calcium and calmodulin-dependent phosphatase, plays a pivotal role in cardiac hypertrophy by translocating to the nucleus and dephosphorylating NFAT, allowing it to transcribe genes to activate hypertrophy in cardiomyocytes. Cyclosporine (CsA) binds to cyclophilin A, forming a complex with high affinity for calcineurin, which in turn inhibits its nuclear translocation. This is hypothesized to inhibit activation of NFAT-mediated hypertrophy (59). Tacrolimus binds to FK506-binding protein (FKBP12) to inhibit calcineurin activity driving reduced NFAT-mediated transcription of hypertrophic genes.
In early animal experiments, CsA successfully prevented or attenuated cardiac hypertrophy in mice overexpressing contractile elements (10, 29), genetic predispositions to hypertrophy (19), and treatment with exogenous chemical signals promoting hypertrophy (11, 15, 60). However, these data were challenged by the failure of CsA to prevent hypertrophy in several models of hypertension or pressure overload (16, 35, 61). Tacrolimus has also yielded mixed results. In murine models of genetic hypertrophic cardiomyopathy, tacrolimus exacerbated cardiac hypertrophy (37). In animal models of hypertrophy induced by phenylephrine stimulation, spontaneously hypertensive rats, or aortic banding, tacrolimus treatment had variable effects, with exacerbation or amelioration of the hypertrophic phenotype (16, 38, 61, 62).
Some hypothesized that the mixed results were driven by variability in hypertrophic signaling from genetic/sarcomeric-driven hypertrophic signaling vs. adaptive chemical or afterload-driven hypertrophy (37, 59). This hypothesis is somewhat weakened by mixed data for transverse aortic constriction rodent models.
Subsequent investigations suggested that CsA-induced effects on hypertrophic remodeling may be driven by increased fibrosis. Multiple studies have shown that CsA treatment led to increases in MMP2, MMP9, and Collagen I in dose dependent manner (20–22, 63). Rat hearts treated with CsA exhibited increased fibrosis/collagen content (64). Similar data of increased collagen deposition in response to tacrolimus treatment was observed in human induced pluripotent stem cell-derived cardiac organoids treated with tacrolimus (65). The in vitro findings suggest that increased fibrosis is not a result of calcineurin-induced hypertension.
Notwithstanding some of the conflicting data in animal models, the data from humans have been fairly consistent as to the effects of CsA and tacrolimus on human hearts. Endomyocardial biopsies from heart or liver transplant patients treated with CsA showed structural distortion, increased fibrosis, and increased collagen levels (25, 26). Furthermore, patients treated with CsA and tacrolimus had hypertrophy or increased LV mass on autopsy or imaging (8, 26, 27, 39, 40). A clinical trial investigating the effect of CsA in patients with hypertrophic cardiomyopathy was initiated, but it is unclear if the study was completed and findings, if any, were not published (66).
Despite some earlier reports of amelioration of cardiac hypertrophy by CNI, there is no clear evidence in humans to corroborate this finding. Supported by in vitro and human data, a consistent signal of increased hypertrophy and fibrosis associated with CNI treatment is observed (23, 28). Cellular data highlight that the increase in LV mass may be driven primarily by CNI-induced increase in fibrosis and collagen deposition rather than cardiomyocyte remodeling.
mTOR inhibitors
mTOR inhibitors, such as sirolimus and everolimus, inhibit mammalian target of rapamycin complex I, thereby inhibiting downstream pathways driving cell growth, proliferation, and survival. There are notable differences between sirolimus and everolimus (67). Everolimus is the 40-O-(2-hydroxyethyl) derivative of sirolimus, and differs in its subcellular distribution, pharmacokinetics and binding affinity. Compared to sirolimus, everolimus has higher bioavailability and shorter half-life. Both drugs form a complex with FKBP-12, which binds mTOR. However, everolimus binding to FKBP-12 is ~3-fold weaker than that of sirolimus, leading to significant differences in inhibition of mTORC2 activation and downstream effects (68, 69). Clinically this has translated into differences in side effect profile and potency of each drug.
This class of drugs has garnered significant interest in solid organ transplantation owing to salutary effects on renal function, allograft vasculopathy and malignancy risk (70). Sirolimus has been shown to reduce cardiac hypertrophy and fibrosis in animal models of pressure overload, uremia, and adriamycin induced cardiomyopathy (42, 43, 71). In a rat model of myocardial infarction, everolimus improved post-infarct remodeling (72) although in the recently published CLEVER-ACS trial of patients with myocardial infarction, there everolimus treatment had no effect on myocardial remodeling (73). Cellular data suggest that attenuation of adverse cardiac remodeling by mTOR inhibitors may be related in part to reduced cardiac fibroblast proliferation and collagen secretion (65).
The favorable signal for sirolimus has been validated in human studies, which largely compared outcomes to subjects treated with CNI. Sirolimus has been associated with improvement in diastolic dysfunction and filling pressures, possibly through attenuation of fibrosis (47–49). In patients with heart transplantation, everolimus treatment was associated with less myocardial fibrosis than mycophenolate treatment by biopsy and imaging (50, 51). The data in kidney transplant patients has been more mixed with some suggesting less LV hypertrophy with the use of everolimus (74), while a number of randomized trials showed no difference in LV mass index after conversion from CsA to everolimus post-kidney transplant (52–55). The incidence of adverse cardiovascular events from these studies was mixed with the majority showing no differences in outcomes (75–77). This discordant signal may be related to the fact that kidney transplant recipients often have concomitant hypertension and activation of the renin-angiotensin system that may have already contributed to significant adverse cardiac remodeling prior to kidney transplant—making it less likely to observe differences following kidney transplantation (75, 78). Additionally, most of the studies may have been underpowered to detect differences in cardiovascular outcomes.
Purine synthesis inhibitors
Purine synthesis inhibitors block cell proliferation by preventing the synthesis of DNA and RNA during S phase of the cell-cycle. Mycophenolate mofetil (MMF) treatment has been shown to prevent or attenuate ischemic injury and autoimmune myocarditis in animal models, with reduced secretion of inflammatory markers such as TLR4, NFκB, BAX expression, and TNFα (57, 58). There are no human studies suggesting a link between cardiac hypertrophy or fibrosis in association with MMF or azathioprine use.
Mitochondrial dysfunction
Mitochondria constitute a third of cardiomyocyte volume, and the heart, as a metabolically active organ, relies heavily on mitochondrial ATP production (79). Mitochondrial dysfunction is a feature of multiple types of cardiomyopathy, as it confers oxidative stress and changes in energetics to drive adverse cardiac remodeling. Immunosuppressive agents can exert direct effects on mitochondrial health to modulate cardiac remodeling and this has been subject of much investigation (Table 2).
Calcineurin inhibitors
Cyclophilin D is a protein in the inner mitochondrial matrix involved in opening of the mitochondrial permeability transition pore (mPTP) (94). mPTP opening results in mitochondrial calcium overload, release of cytochrome C, a process involved in apoptosis and implicated in myocardial ischemia-reperfusion (IR) injury (80). CsA interacts with cyclophilin D thereby preventing mPTP opening and protecting the mitochondria from calcium overload. Tacrolimus does not bind cyclophilin D and the effects of tacrolimus on mitochondrial function and mPTP opening are less defined. Multiple animal studies have sought to define the effect of both drugs on mitochondrial function.
CsA prevented mitochondrial-mediated injury and improved myocardial recovery in models of hypothermia, IR injury, and inborn errors of mitochondrial DNA polymerase (81–86, 95). In addition, CsA and/or tacrolimus have been associated with a favorable mitochondrial phenotype in the face of adriamycin treatment, hypoxia or endotoxemia (88–90).
Clinical data on the implications of these findings have been scant. In a single study of patients presenting with ST elevation myocardial infarction, CsA treatment decreased myocardial scar burden, which in combination with pre-clinical evidence provided promise for CsA as a “post-conditioning agent” during myocardial infarction (24, 30). However, follow-up studies failed to show any benefit to CsA treatment in regards to LV function, arrhythmia, or mortality (31–34). The discordance suggests that CsA protection from mitochondrial injury is largely a short term or acute benefit. No human studies to date have evaluated the effect of CNI on mitochondrial structure and function in light of associated cardiac remodeling.
mTOR inhibitors
Sirolimus has been associated with a reduction in respiration and cellular energetics in cardiomyocytes (92). This effect has been attributed to the observation that mTOR may activate AMP-activated protein kinase to regulate cellular bioenergetics (96). In a mouse model of cardiac IR injury, sirolimus inhibited apoptosis and improved cardiac performance via interaction with the mitochondrial ATP-sensitive potassium channel (93) and appears to reduce ER stress and cytochrome C release (97). In brain, sirolimus enhances the distribution of CsA into mitochondria, accentuating its effects of decreasing mitochondrial metabolism, whereas everolimus appears to antagonize the effects of CsA in mitochondria to increase energy metabolism (67, 98). At therapeutically relevant concentrations, everolimus, but not sirolimus, distributes into brain mitochondria (99, 100). As cited above clinical studies have suggested a favorable effect for mTOR inhibitors on cardiac remodeling—but data examining mitochondrial function is lacking.
Purine synthesis inhibitors
There are no reports of direct effects of MMF and Azathioprine on mitochondrial function in cardiomyocytes or heart tissue.
Arrhythmia
With described effects on myocardial structural remodeling and intracellular ion transporter function, immunosuppressive therapies may modulate the risk of arrhythmia. This poses significant short- and long-term risks, especially in patients with underlying structural heart disease and heart transplant recipients (Table 3).
Calcineurin inhibitors
Calcineurin affects intracellular calcium transients in cardiomyocytes via modulation of the ryanodine receptor and activation of the NFAT pathway, which drives transcriptional changes in proteins regulating intracellular calcium (124). Calcineurin inhibitors in turn can play a role in mediating changes in calcium transients impacting the electrical phenotype of the heart.
Delineating the precise effect of CNI on calcium regulation in human cardiomyocytes has proven elusive. In some models CsA appeared to reduce sarcoplasmic reticulum (SR) calcium release and cytosolic levels of Ca2+ (106). However, other models showed that both CsA and tacrolimus result in increased Ca2+ release events and an increase in QT prolongation. A possible mechanism of QT prolongation may be an increase in the duration of Ca2+ transients due to blockade of Na2+/Ca2+ exchanger. It is possible that CsA and tacrolimus exert different electrical phenotypes owing to their differential role in mitochondrial Ca2+ regulation and mPTP opening. Nonetheless the results in animal models of both drugs have been equally mixed; in some animal models, the cellular phenotypes of CNI appeared to translate to a reduced propensity to arrhythmia (103, 105, 106), but not in other models (101, 102).
Clinically, in case reports, CsA and tacrolimus induced atrial fibrillation and tacrolimus induced QT prolongation and atrial arrhythmias (107, 116). However, neither signal was seen in clinical trials with either drug suggesting that the arrhythmic risk is low (125, 126).
mTOR inhibitors
There are no published reports of mTOR inhibitors modulating risk of arrhythmias. The recently published CLEVER-ACS trial showed no difference in atrial arrhythmias in patients treated with everolimus after myocardial infarction (73).
Purine synthesis inhibitors
Azathioprine use is associated with increased incidence of atrial arrhythmias. In a 3-year randomized controlled trial of azathioprine vs. MMF, heart transplant patients treated with azathioprine had a higher rate of atrial arrhythmias than those on MMF (119). The mechanism for this phenomenon is unknown. There are no published reports of MMF modulating arrhythmia risk.
Hypertension
Hypertension is a well described side effect of immunosuppressive medication use, particularly CNI, and is associated with increased risk of coronary artery disease, cerebrovascular events, renal dysfunction, and adverse cardiovascular remodeling (Table 4).
Calcineurin inhibitors
CNI are known to cause hypertension, with 50–80% of patients reported to have hypertension with chronic use. CsA is associated with a higher incidence compared to tacrolimus (130). CNI are implicated in afferent arteriole vasoconstriction and activation of the renin-angiotensin system, promoting sodium retention and volume expansion (127, 149). Furthermore, CsA and tacrolimus are associated with promoting direct vasoconstriction by one or more of the following mechanisms: increased tone of vascular smooth muscle (128, 150, 151), reduced nitric oxide production (129), and activation of endothelin-1 receptor (129). In cultured murine endothelial and vascular smooth muscle cells, both CsA and tacrolimus were associated with production of proinflammatory cytokines and endothelial activation, with increased superoxide production and NF-kB regulated synthesis of proinflammatory factors, which were prevented by pharmacological inhibition of TLR4. This raises the possibility that a proinflammatory milieu drives chronic endothelial dysfunction, contributing to CNI-induced hypertension (152).
There is some controversy as to whether the clinical hypertrophic phenotype is related to direct myocardial effects or is in fact due an increase in the incidence of hypertension associated with CsA use. Observations that rats treated with CsA develop hypertension prior to myocardial hypertrophy (4, 101, 153–155) supported the notion that perhaps the clinical hypertrophic phenotype is purely related to CNI-induced hypertension rather than direct myocardial effects. While hypertension may be a contributor to the hypertrophic phenotype observed, multiple animal and cellular models have supported a direct effect of CNI on myocardial remodeling.
mTOR inhibitors
mTOR inhibitors have been associated with a lower risk of hypertension compared to calcineurin inhibitors when used in solid organ transplant recipients (137, 156). The difference between effects of CNI and mTOR inhibitors is likely driven by multiple mechanisms with an overall vasodilatory effect of mTOR inhibitors (157, 158). Sirolimus and everolimus appear to increase nitric oxide production preventing endothelial hyperplasia and dysfunction (133, 134). This promising anti-hypertensive profile has led to the consideration of mTOR inhibitors as a primary therapy for specialized difficult-to-treat populations with hypertension including pulmonary arterial hypertension and primary hyperaldosteronism (138, 139).
Purine synthesis inhibitors
Purine synthesis inhibitors are not associated with hypertension and may in fact have an antihypertensive effect. In comparison to patients treated with CsA after heart transplantation, those treated with azathioprine were less likely to develop hypertension (148). Lower blood pressures have been reported in patients taking MMF for psoriasis and rheumatoid arthritis (145). Possible mechanisms for the favorable hypertensive profile include: lower pro-inflammatory signaling that drives endothelial dysfunction and hyperplasia, decreased circulating levels of endothelin-1, and reduced sodium reabsorption and neuro-hormonal activation leading to hypertension (142–144). Taken together, these data suggest that purine synthesis inhibitors carry a lower risk of systemic hypertension, and may in fact contribute to favorable mechanisms to reduce hypertension in pulmonary hypertension and renal dysfunction-associated hypertension.
Vascular remodeling
In addition to effects on hypertension, immunosuppressive agents may directly contribute to abnormal vascular remodeling to drive cardiovascular adverse events, independent of hypertension or dyslipidemia. Defining this risk and the contributing mechanisms for each drug is important in order to ensure appropriate follow up and identify potential actionable targets to modify the risk profile (Table 5).
Calcineurin inhibitors
CNI, particularly tacrolimus, have been associated with increased risk of allograft vasculopathy (167–169, 191). This notable complication of transplanted hearts represents a major driver of graft dysfunction and has significant implications for quality of life and longevity of heart transplant recipients (163–165). This has been replicated in animal models using both tacrolimus and CsA with adverse remodeling features of vascular stiffness, thickening, inflammation and fibrosis noted in treated animals (159, 160). The mechanisms for these include: decreased fibrinolytic activity in vessel walls, increased oxidative stress in endothelial cells, and possibly increased intracellular calcium in vascular smooth muscle cells (161, 162, 192).
mTOR inhibitors
Both sirolimus and everolimus have been associated with a more favorable vascular profile and their clinical efficacy in reducing the rate of progression of cardiac allograft vasculopathy has led to widespread use in heart transplant recipients (175, 176, 183). In addition to reducing signaling associated with endothelial dysfunction, mTOR inhibitors have been shown to reduce vascular smooth muscle proliferation, intimal hyperplasia, and infiltration by inflammatory cells (170–173, 193, 194). Everolimus, in particular, was shown to reduce pro-inflammatory signaling by decreasing IL-9, VEGF release, and TNFα induced adhesion of endothelial cells (184). These effects have led to wide adoption of everolimus- and sirolimus-eluting stents in the treatment of coronary artery disease (179, 195, 196).
In several trials of kidney transplant patients, a switch from CsA to mTOR inhibitor was associated with stabilization or improvement in parameters of arterial stiffness, including pulse wave velocity (PWV), carotid systolic blood pressure, pulse pressure, and augmentation index (177, 178). One notable exception was a secondary analysis of the ELEVATE trial, where no difference in PWV was found with switch from CsA to everolimus, which was attributed to significant variation in baseline PWV in the study population (75).
In addition to reducing allograft vasculopathy, the anti-vascular proliferation signal conferred by mTOR inhibitors has made the drug class of substantial interest in oncology to suppress tumor neovascularization. Nonetheless, while this anti-proliferation profile offers a substantial benefit, it carries some drawbacks; Namely, both mTOR inhibitor drugs are associated with an increased incidence of lymphedema, which is thought to be driven by inhibition of lymphatic endothelial cell proliferation (197, 198). The incidence of such side effects must be considered in oncologic therapy, where drug dosage is typically higher than that used in transplant immunosuppression (199).
Purine synthesis inhibitors
Purine synthesis inhibitors appear to confer a beneficial vascular remodeling profile. MMF has been associated with reduced atherosclerosis progression and CAV in patients and animal models (119, 142, 185). Animal models point to a signal of decreased vascular oxidative stress and inflammation as the driving mechanism of that benefit (187, 200, 201). Reduced endothelial and smooth muscle proliferation in association with MMF have also been proposed as a possible mechanism, although the evidence is more limited than for mTOR inhibitors (190).
Dyslipidemia
Immunosuppressive medications are associated with dyslipidemia. Each drug class is associated with individual variations in affected lipid particles and more importantly in the conferred risk of atherosclerosis (Table 6).
Calcineurin inhibitors
CsA use is associated with a dose-dependent increase in total cholesterol and low-density lipoprotein (LDL) cholesterol, a decrease in high-density lipoprotein (HDL) cholesterol, and an increase in serum triglycerides (202, 203). These changes are driven by a decrease in lipoprotein lipase and an increase in activity of cholesteryl ester transfer protein (204, 229). Additionally, CsA may reduce expression of the LDL receptor, thereby impairing LDL clearance (230–232). Tacrolimus is associated with a similar, but milder, dyslipidemia profile compared to CsA (130, 209–212). CsA appears to be associated with an increase in oxidized LDL, which confers a higher risk of atherosclerosis, while the data for tacrolimus effect on LDL oxidation are mixed (206–208).
mTOR inhibitors
Sirolimus is a stronger inducer of hyperlipidemia than CNI, associated clinically with an increase in serum LDL and triglyceride levels (70, 218, 233). The mechanism remains unclear, although it may be due to a combination of reduced catabolism, an increase in the free fatty acid pool, increased hepatic production of triglycerides, and secretion of very low density lipoprotein (VLDL) (204, 217). In addition, sirolimus is associated with an increase in serum PCSK9 levels, which acts as a post-transcriptional regulator of LDL receptor expression (234). Clinical data on the risk of dyslipidemia associated with everolimus has been mixed. In clinical studies, everolimus was not associated with an increased risk of dyslipidemia compared to CNI (220, 222, 223, 235–237). However, a meta-analysis comparing mTOR inhibitors to CNI adverse events has noted no difference between sirolimus and everolimus in the incidence of dyslipidemia (238). This suggests that everolimus may contribute to dyslipidemia, but at an intensity that is between CNI and sirolimus.
Interestingly, despite the increase in serum lipids, mTOR inhibitors are associated with an overall lower risk of atherosclerosis (195). Sirolimus reduces oxidized-LDL adhesion and uptake to endothelial cells, and can promote its autophagic degradation (214, 215). Additionally, sirolimus reduces intracellular lipid accumulation in vascular smooth muscle cells, and increases cholesterol efflux via increased expression of the ATP binding cassette protein ABCA1 (216). Similarly everolimus treatment in LDL receptor knockout mice, everolimus increased VLDL/LDL levels but reduced the rate of atherosclerosis. Thus, regardless of dyslipidemia profile, mTOR inhibitors appear to result in a net reduction in the rate of atherosclerosis, which may explain the overall clinical benefit observed.
Purine synthesis inhibitors
Both MMF and azathioprine appear to have a neutral effect on lipids with no significant changes observed in lipid profile in clinical studies (131, 226–228). In vitro studies suggest that MMF increases cholesterol efflux, but another study demonstrated inhibition of lipoprotein lipase activity—the opposing effects may explain the net neutral profile conferred by the drug.
Drug exposure and bioavailability
It is important to note that the bioavailability and exposure levels of the immunosuppression drugs have varied tremendously across clinic and scientific studies in the field. This may explain the differences observed between pre-clinical and clinical studies or even discrepancies between different clinical studies. Part of this variation is not simply investigator mediated, but is driven by variability in clinical practice by geographic area and changes in clinical practice over time. Early CsA trough concentrations in kidney transplant patients ranged 200–500 μg/ml, whereas in Europe, they were typically lower (100–200 μg/ml). Similarly, tacrolimus trough levels ranged 12–20 ηg/ml in the US, and lower in Europe (8–15 ηg/ml). There were also variations in sirolimus and everolimus levels when used in combination with CNI. MMF was previously prescribed at higher doses than is typically used now (2–3 g twice daily to 1 g twice daily) (12, 55, 107, 131).
Conclusions
Immunosuppressive agents exert significant effects on the heart and vasculature. Mechanistic studies point toward immunosuppression drug-specific influences on changes in cell proliferation, mitochondrial function, inflammatory cytokines, and altered calcium handling as potential mediators of these phenotypes. Calcineurin inhibitors promote cardiac hypertrophy, hypertension, dyslipidemia, and vascular remodeling, while mTOR inhibitors have an anti-proliferative effect with attenuation of cardiac hypertrophy and vascular remodeling despite promoting dyslipidemia. Purine synthesis inhibitor are less well studied, but may have a neutral to mildly positive effect on hypertension and vascular remodeling. These phenotypes are associated with significant morbidity in patients taking immunosuppressive medications, carrying increased risks of heart failure, cardiovascular disease, and kidney dysfunction. While preclinical studies have provided invaluable insight into mechanisms of cardiovascular remodeling, the discordance with clinical data, such as in the case of CNI and hypertrophy, highlights the importance of caution in generalizing the results of cell-based and animal models. Further translational research is needed to identify actionable targets to treat associated cardiovascular side effects of immunosuppression drugs.
Data availability statement
The original contributions presented in the study are included in the article/supplementary material, further inquiries can be directed to the corresponding author.
Author contributions
AE, RD, and KS contributed to the writing and figures presented in the manuscript. All authors contributed to the article and approved the submitted version.
Funding
NIH T32 HL09427411A1 (AE), NIH K08 HL135343 (KS), and American Heart Association and Enduring Hearts Grant # 924127/Sallam and Hollander/2022 (KS).
Acknowledgments
Figures were created using Biorender.com.
Conflict of interest
The authors declare that the research was conducted in the absence of any commercial or financial relationships that could be construed as a potential conflict of interest.
Publisher's note
All claims expressed in this article are solely those of the authors and do not necessarily represent those of their affiliated organizations, or those of the publisher, the editors and the reviewers. Any product that may be evaluated in this article, or claim that may be made by its manufacturer, is not guaranteed or endorsed by the publisher.
References
1. Goldraich LA, Leitão SAT, Scolari FL, Marcondes-Braga FG, Bonatto MG, Munyal D, et al. A comprehensive and contemporary review on immunosuppression therapy for heart transplantation. Curr Pharm Des. (2020) 26:3351–84. doi: 10.2174/1381612826666200603130232
2. Wallace BI, Kenney B, Malani PN, Clauw DJ, Nallamothu BK, Waljee AK. Prevalence of immunosuppressive drug use among commercially insured US adults, 2018-2019. JAMA Netw Open. (2021) 4:e214920. doi: 10.1001/jamanetworkopen.2021.4920
3. Harpaz R, Dahl RM, Dooling KL. Prevalence of immunosuppression among US adults. JAMA. (2016) 316:2547–8. doi: 10.1001/jama.2016.16477
4. Miller LW. Cardiovascular toxicities of immunosuppressive agents. Am J Transplant Off J Am Soc Transplant Am Soc Transpl Surg. (2002) 2:807–18. doi: 10.1034/j.1600-6143.2002.20902.x
5. Levy D, Garrison RJ, Savage DD, Kannel WB, Castelli WP. Prognostic implications of echocardiographically determined left ventricular mass in the Framingham Heart Study. N Engl J Med. (1990) 322:1561–6. doi: 10.1056/NEJM199005313222203
6. Mottram PM, Marwick TH. Assessment of diastolic function: what the general cardiologist needs to know. Heart Br Card Soc. (2005) 91:681–95. doi: 10.1136/hrt.2003.029413
7. Luo Z, Shyu KG, Gualberto A, Walsh K. Calcineurin inhibitors and cardiac hypertrophy. Nat Med. (1998) 4:1092–3. doi: 10.1038/2578
8. Nakata Y, Yoshibayashi M, Yonemura T, Uemoto S, Inomata Y, Tanaka K, et al. Tacrolimus and myocardial hypertrophy. Transplantation. (2000) 69:1960–2. doi: 10.1097/00007890-200005150-00039
9. Paoletti E. mTOR inhibition and cardiovascular diseases: cardiac hypertrophy. Transplantation. (2018) 102:S41. doi: 10.1097/TP.0000000000001691
10. Sussman MA, Lim HW, Gude N, Taigen T, Olson EN, Robbins J, et al. Prevention of cardiac hypertrophy in mice by calcineurin inhibition. Science. (1998) 281:1690–3. doi: 10.1126/science.281.5383.1690
11. Meguro T, Hong C, Asai K, Takagi G, McKinsey TA, Olson EN, et al. Cyclosporine attenuates pressure-overload hypertrophy in mice while enhancing susceptibility to decompensation and heart failure. Circ Res. (1999) 84:735–40. doi: 10.1161/01.RES.84.6.735
12. Eto Y, Yonekura K, Sonoda M, Arai N, Sata M, Sugiura S, et al. Calcineurin is activated in rat hearts with physiological left ventricular hypertrophy induced by voluntary exercise training. Circulation. (2000) 101:2134–7. doi: 10.1161/01.CIR.101.18.2134
13. Lim HW, De Windt LJ, Steinberg L, Taigen T, Witt SA, Kimball TR, et al. Calcineurin expression, activation, and function in cardiac pressure-overload hypertrophy. Circulation. (2000) 101:2431–7. doi: 10.1161/01.CIR.101.20.2431
14. Hill JA, Karimi M, Kutschke W, Davisson RL, Zimmerman K, Wang Z, et al. Cardiac hypertrophy is not a required compensatory response to short-term pressure overload. Circulation. (2000) 101:2863–9. doi: 10.1161/01.CIR.101.24.2863
15. Ding B, Price RL, Borg TK, Weinberg EO, Halloran PF, Lorell BH. Pressure overload induces severe hypertrophy in mice treated with cyclosporine, an inhibitor of calcineurin. Circ Res. (1999) 84:729–34. doi: 10.1161/01.RES.84.6.729
16. Zhang W, Kowal RC, Rusnak F, Sikkink RA, Olson EN, Victor RG. Failure of calcineurin inhibitors to prevent pressure-overload left ventricular hypertrophy in rats. Circ Res. (1999) 84:722–8. doi: 10.1161/01.RES.84.6.722
17. Mervaala E, Müller DN, Park JK, Dechend R, Schmidt F, Fiebeler A, et al. Cyclosporin A protects against angiotensin II-induced end-organ damage in double transgenic rats harboring human renin and angiotensinogen genes. Hypertens Dallas Tex. (2000) 35:360–6. doi: 10.1161/01.HYP.35.1.360
18. Lassila M, Finckenberg P, Pere AK, Krogerus L, Ahonen J, Vapaatalo H, et al. Comparison of enalapril and valsartan in cyclosporine A-induced hypertension and nephrotoxicity in spontaneously hypertensive rats on high-sodium diet. Br J Pharmacol. (2000) 130:1339–47. doi: 10.1038/sj.bjp.0703422
19. Mende U, Kagen A, Cohen A, Aramburu J, Schoen FJ, Neer EJ. Transient cardiac expression of constitutively active Galphaq leads to hypertrophy and dilated cardiomyopathy by calcineurin-dependent and independent pathways. Proc Natl Acad Sci U S A. (1998) 95:13893–8. doi: 10.1073/pnas.95.23.13893
20. Eleftheriades EG, Ferguson AG, Samarel AM. Cyclosporine A has no direct effect on collagen metabolism by cardiac fibroblasts in vitro. Circulation. (1993) 87:1368–77. doi: 10.1161/01.CIR.87.4.1368
21. Chi J, Wang L, Zhang X, Fu Y, Liu Y, Chen W, et al. Cyclosporin A induces autophagy in cardiac fibroblasts through the NRP-2/WDFY-1 axis. Biochimie. (2018) 148:55–62. doi: 10.1016/j.biochi.2018.02.017
22. Rezzani R, Angoscini P, Rodella L, Bianchi R. Alterations induced by cyclosporine A in myocardial fibers and extracellular matrix in rat. Histol Histopathol. (2002) 17:761–6. doi: 10.14670/HH-17.761
23. Kolár F, Papousek F, MacNaughton C, Pelouch V, Milerová M, Korecky B. Myocardial fibrosis and right ventricular function of heterotopically transplanted hearts in rats treated with cyclosporin. Mol Cell Biochem. (1996) 163–164:253–60. doi: 10.1007/BF00408666
24. Minners J, van den Bos EJ, Yellon DM, Schwalb H, Opie LH, Sack MN. Dinitrophenol, cyclosporin A, and trimetazidine modulate preconditioning in the isolated rat heart: support for a mitochondrial role in cardioprotection. Cardiovasc Res. (2000) 47:68–73. doi: 10.1016/S0008-6363(00)00069-9
25. Stovin PG, English TA. Effects of cyclosporine on the transplanted human heart. J Heart Transplant. (1987) 6:180–5.
26. Roberts CA, Stern DL, Radio SJ. Asymmetric cardiac hypertrophy at autopsy in patients who received FK506 (tacrolimus) or cyclosporine A after liver transplant. Transplantation. (2002) 74:817–21. doi: 10.1097/00007890-200209270-00015
27. Espino G, Denney J, Furlong T, Fitzsimmons W, Nash RA. Assessment of myocardial hypertrophy by echocardiography in adult patients receiving tacrolimus or cyclosporine therapy for prevention of acute GVHD. Bone Marrow Transplant. (2001) 28:1097–103. doi: 10.1038/sj.bmt.1703304
28. Karch SB, Billingham ME. Cyclosporine induced myocardial fibrosis: a unique controlled case report. J Heart Transplant. (1985) 4:210–2.
29. Choudhary R, Sastry BKS, Subramanyam C. Positive correlations between serum calcineurin activity and left ventricular hypertrophy. Int J Cardiol. (2005) 105:327–31. doi: 10.1016/j.ijcard.2005.04.006
30. Piot C, Croisille P, Staat P, Thibault H, Rioufol G, Mewton N, et al. Effect of cyclosporine on reperfusion injury in acute myocardial infarction. N Engl J Med. (2008) 359:473–81. doi: 10.1056/NEJMoa071142
31. Ghaffari S, Kazemi B, Toluey M, Sepehrvand N. The effect of prethrombolytic cyclosporine-A injection on clinical outcome of acute anterior ST-elevation myocardial infarction. Cardiovasc Ther. (2013) 31:e34–39. doi: 10.1111/1755-5922.12010
32. Cung TT, Morel O, Cayla G, Rioufol G, Garcia-Dorado D, Angoulvant D, et al. Cyclosporine before PCI in patients with acute myocardial infarction. N Engl J Med. (2015) 373:1021–31. doi: 10.1056/NEJMoa1505489
33. Ottani F, Latini R, Staszewsky L, La VL, Locuratolo N, Sicuro M, et al. Cyclosporine A in reperfused myocardial infarction. J Am Coll Cardiol. (2016) 67:365–74. doi: 10.1016/j.jacc.2015.10.081
34. Chen -Scarabelli Carol, Scarabelli TM. Cyclosporine a prior to primary PCI in STEMI patients. J Am Coll Cardiol. (2016) 67:375–8. doi: 10.1016/j.jacc.2015.11.024
35. Sakata Y, Masuyama T, Yamamoto K, Nishikawa N, Yamamoto H, Kondo H, et al. Calcineurin inhibitor attenuates left ventricular hypertrophy, leading to prevention of heart failure in hypertensive rats. Circulation. (2000) 102:2269–75. doi: 10.1161/01.CIR.102.18.2269
36. Shimoyama M, Hayashi D, Zou Y, Takimoto E, Mizukami M, Monzen K, et al. [Calcineurin inhibitor attenuates the development and induces the regression of cardiac hypertrophy in rats with salt-sensitive hypertension]. J Cardiol. (2001) 37:114–8.
37. Fatkin D, McConnell BK, Mudd JO, Semsarian C, Moskowitz IG, Schoen FJ, et al. An abnormal Ca(2+) response in mutant sarcomere protein-mediated familial hypertrophic cardiomyopathy. J Clin Invest. (2000) 106:1351–9. doi: 10.1172/JCI11093
38. Kanazawa K, Iwai-Takano M, Kimura S, Ohira T. Blood concentration of tacrolimus and age predict tacrolimus-induced left ventricular dysfunction after bone marrow transplantation in adults. J Med Ultrason. (2001) 47:97–105. doi: 10.1007/s10396-019-00990-y
39. Atkison P, Joubert G, Barron A, Grant D, Paradis K, Seidman E, et al. Hypertrophic cardiomyopathy associated with tacrolimus in paediatric transplant patients. Lancet Lond Engl. (1995) 345:894–6. doi: 10.1016/S0140-6736(95)90011-X
40. Mano A, Nakatani T, Yahata Y, Kato T, Hashimoto S, Wada K, et al. Reversible myocardial hypertrophy induced by tacrolimus in a pediatric heart transplant recipient: case report. Transplant Proc. (2009) 41:3831–4. doi: 10.1016/j.transproceed.2009.05.040
41. Boluyt MO, Zheng JS, Younes A, Long X, O'Neill L, Silverman H, et al. Rapamycin Inhibits α1-Adrenergic Receptor–Stimulated Cardiac Myocyte Hypertrophy but Not Activation of Hypertrophy-Associated Genes. Circ Res. (1997) 81:176–86. doi: 10.1161/01.RES.81.2.176
42. Gao XM, Wong G, Wang B, Kiriazis H, Moore XL, Su YD, et al. Inhibition of mTOR reduces chronic pressure-overload cardiac hypertrophy and fibrosis. J Hypertens. (2006) 24:1663–70. doi: 10.1097/01.hjh.0000239304.01496.83
43. Gu J, Hu W, Song ZP, Chen YG, Zhang DD, Wang CQ. Rapamycin Inhibits Cardiac Hypertrophy by Promoting Autophagy via the MEK/ERK/Beclin-1 Pathway. Front Physiol [Internet]. (2016). Available online at: https://www.frontiersin.org/article/10.3389/fphys.2016.00104 doi: 10.3389/fphys.2016.00104 (accessed April 28, 2022).
44. Yu SY, Liu L, Li P, Li J. Rapamycin inhibits the mTOR/p70S6K pathway and attenuates cardiac fibrosis in adriamycin-induced dilated cardiomyopathy. Thorac Cardiovasc Surg. (2013) 61:223–8. doi: 10.1055/s-0032-1311548
45. Reifsnyder PC, Ryzhov S, Flurkey K, Anunciado-Koza RP, Mills I, Harrison DE, et al. Cardioprotective effects of dietary rapamycin on adult female C57BLKS/J-Leprdb mice. Ann N Y Acad Sci. (2018) 1418:106–17. doi: 10.1111/nyas.13557
46. Luck C, DeMarco VG, Mahmood A, Gavini MP, Pulakat L. Differential Regulation of Cardiac Function and Intracardiac Cytokines by Rapamycin in Healthy and Diabetic Rats. Oxid Med Cell Longev. (2017) 2017:5724046. doi: 10.1155/2017/5724046
47. Raichlin E, Chandrasekaran K, Kremers WK, Frantz RP, Clavell AL, Pereira NL, et al. Sirolimus as primary immunosuppressant reduces left ventricular mass and improves diastolic function of the cardiac allograft. Transplantation. (2008) 86:1395–400. doi: 10.1097/TP.0b013e318189049a
48. Alnsasra H, Asleh R, Oh JK, Maleszewski JJ, Lerman A, Toya T, et al. Impact of sirolimus as a primary immunosuppressant on myocardial fibrosis and diastolic function following heart transplantation. J Am Heart Assoc. (2021) 10:e018186. doi: 10.1161/JAHA.120.018186
49. Kushwaha SS, Raichlin E, Sheinin Y, Kremers WK, Chandrasekaran K, Brunn GJ, et al. Sirolimus affects cardiomyocytes to reduce left ventricular mass in heart transplant recipients. Eur Heart J. (2008) 29:2742–50. doi: 10.1093/eurheartj/ehn407
50. Anthony C, Imran M, Pouliopoulos J, Emmanuel S, Iliff JW, Moffat KJ, et al. Everolimus for the prevention of calcineurin-inhibitor-induced left ventricular hypertrophy after heart transplantation (RADTAC Study). JACC Heart Fail. (2021) 9:301–13. doi: 10.1016/j.jchf.2021.01.007
51. Imamura T, Kinugawa K, Nitta D, Kinoshita O, Nawata K, Ono M. Everolimus attenuates myocardial hypertrophy and improves diastolic function in heart transplant recipients. Int Heart J. (2016) 57:204–10. doi: 10.1536/ihj.15-320
52. Krishnan A, Teixeira-Pinto A, Chan D, Chakera A, Dogra G, Boudville N, et al. Impact of early conversion from cyclosporin to everolimus on left ventricular mass index: a randomized controlled trial. Clin Transplant. (2017) 31:10. doi: 10.1111/ctr.13043
53. Murbraech K, Holdaas H, Massey R, Undset LH, Aakhus S. Cardiac response to early conversion from calcineurin inhibitor to everolimus in renal transplant recipients: an echocardiographic substudy of the randomized controlled CENTRAL trial. Transplantation. (2014) 97:184–8. doi: 10.1097/TP.0b013e3182a92728
54. Murbraech K, Massey R, Undset LH, Midtvedt K, Holdaas H, Aakhus S. Cardiac response to early conversion from calcineurin inhibitor to everolimus in renal transplant recipients–a three-yr serial echocardiographic substudy of the randomized controlled CENTRAL trial. Clin Transplant. (2015) 29:678–84. doi: 10.1111/ctr.12565
55. de Fijter JW, Holdaas H, Øyen O, Sanders JS, Sundar S, Bemelman FJ, et al. Early conversion from Calcineurin inhibitor- to Everolimus-based therapy following kidney transplantation: results of the randomized ELEVATE trial. Am J Transplant Off J Am Soc Transplant Am Soc Transpl Surg. (2017) 17:1853–67. doi: 10.1111/ajt.14186
56. Uchinaka A, Yoneda M, Yamada Y, Murohara T, Nagata K. Effects of mTOR inhibition on cardiac and adipose tissue pathology and glucose metabolism in rats with metabolic syndrome. Pharmacol Res Perspect. (2017) 5:4. doi: 10.1002/prp2.331
57. Li T, Yu J, Chen R, Wu J, Fei J, Bo Q, et al. Mycophenolate mofetil attenuates myocardial ischemia-reperfusion injury via regulation of the TLR4/NF-κB signaling pathway. Pharm. (2014) 69:850–5. doi: 10.1691/ph.2014.4598
58. Kamiyoshi Y, Takahashi M, Yokoseki O, Yazaki Y, Hirose SI, Morimoto H, et al. Mycophenolate mofetil prevents the development of experimental autoimmune myocarditis. J Mol Cell Cardiol. (2005) 39:467–77. doi: 10.1016/j.yjmcc.2005.04.004
59. Molkentin JD. Calcineurin and beyond: cardiac hypertrophic signaling. Circ Res. (2000) 87:731–8. doi: 10.1161/01.RES.87.9.731
60. Zhu W, Zou Y, Shiojima I, Kudoh S, Aikawa R, Hayashi D, et al. Ca2+/calmodulin-dependent kinase II and calcineurin play critical roles in endothelin-1-induced cardiomyocyte hypertrophy. J Biol Chem. (2000) 275:15239–45. doi: 10.1074/jbc.275.20.15239
61. Zhang W. Old and new tools to dissect calcineurin's role in pressure-overload cardiac hypertrophy. Cardiovasc Res. (2002) 53:294–303. doi: 10.1016/S0008-6363(01)00451-5
62. Taigen T, De Windt LJ, Lim HW, Molkentin JD. Targeted inhibition of calcineurin prevents agonist-induced cardiomyocyte hypertrophy. Proc Natl Acad Sci U S A. (2000) 97:1196–201. doi: 10.1073/pnas.97.3.1196
63. Pan B, Li J, Parajuli N, Tian Z, Wu P, Lewno MT, et al. The calcineurin-TFEB-p62 pathway mediates the activation of cardiac macroautophagy by proteasomal malfunction. Circ Res. (2020) 127:502–18. doi: 10.1161/CIRCRESAHA.119.316007
64. Nayler WG, Gu XH, Casley DJ, Panagiotopoulos S, Liu J, Mottram PL. Cyclosporine increases endothelin-1 binding site density in cardiac cell membranes. Biochem Biophys Res Commun. (1989) 163:1270–4. doi: 10.1016/0006-291X(89)91115-7
65. Sallam K, Thomas D, Gaddam S, Lopez N, Beck A, Beach L, et al. Modeling effects of immunosuppressive drugs on human hearts using induced pluripotent stem cell-derived cardiac organoids and single-cell RNA sequencing. Circulation. (2022) 145:1367–9. doi: 10.1161/CIRCULATIONAHA.121.054317
66. National Heart, Lung, and Blood Institute (NHLBI). Double Blind Placebo Controlled Study of Cyclosporin A in Patients With Left Ventricular Hypertrophy Caused by Sarcomeric Gene Mutations [Internet]. clinicaltrials. (2008). Available online at: https://clinicaltrials.gov/ct2/show/NCT00001965 (accessed May 16, 2022).
67. Klawitter J, Nashan B, Christians U. Everolimus and sirolimus in transplantation-related but different. Expert Opin Drug Saf. (2015) 14:1055–70. doi: 10.1517/14740338.2015.1040388
68. Mabasa VH, Ensom MHH. The role of therapeutic monitoring of everolimus in solid organ transplantation. Ther Drug Monit. (2005) 27:666–76. doi: 10.1097/01.ftd.0000175911.70172.2e
69. Stenton SB, Partovi N, Ensom MHH. Sirolimus: the evidence for clinical pharmacokinetic monitoring. Clin Pharmacokinet. (2005) 44:769–86. doi: 10.2165/00003088-200544080-00001
70. Kahan BD, Podbielski J, Napoli KL, Katz SM, Meier-Kriesche HU, Van Buren CT. Immunosuppressive effects and safety of a sirolimus/cyclosporine combination regimen for renal transplantation. Transplantation. (1998) 66:1040–6. doi: 10.1097/00007890-199810270-00013
71. Haller ST, Yan Y, Drummond CA, Xie J, Tian J, Kennedy DJ, et al. Rapamycin Attenuates Cardiac Fibrosis in Experimental Uremic Cardiomyopathy by Reducing Marinobufagenin Levels and Inhibiting Downstream Pro-Fibrotic Signaling. J Am Heart Assoc. (2016) 5:e004106. doi: 10.1161/JAHA.116.004106
72. Buss SJ, Muenz S, Riffel JH, Malekar P, Hagenmueller M, Weiss CS, et al. Beneficial effects of mammalian target of rapamycin inhibition on left ventricular remodeling after myocardial infarction. J Am Coll Cardiol. (2009) 54:2435–46. doi: 10.1016/j.jacc.2009.08.031
73. Klingenberg R, Stähli BE, Heg D, Denegri A, Manka R, Kapos I, et al. Controlled-Level EVERolimus in Acute Coronary Syndrome (CLEVER-ACS)—A phase II, randomized, double-blind, multi-center, placebo-controlled trial. Am Heart J. (2022) 247:33–41. doi: 10.1016/j.ahj.2022.01.010
74. Pascual J. Everolimus in clinical practice—renal transplantation. Nephrol Dial Transplant. (2006) 21:iii18–23. doi: 10.1093/ndt/gfl300
75. Holdaas H, de Fijter JW, Cruzado JM, Massari P, Nashan B, Kanellis J, et al. Cardiovascular parameters to 2 years after kidney transplantation following early switch to Everolimus without Calcineurin inhibitor therapy: an analysis of the randomized ELEVATE study. Transplantation. (2017) 101:2612–20. doi: 10.1097/TP.0000000000001739
76. Pipeleers L, Abramowicz D, Broeders N, Lemoine A, Peeters P, Van Laecke S, et al. 5-Year outcomes of the prospective and randomized CISTCERT study comparing steroid withdrawal to replacement of cyclosporine with everolimus in de novo kidney transplant patients. Transpl Int. (2021) 34:313–26. doi: 10.1111/tri.13798
77. van Dijk M, van Roon AM, Said MY, Bemelman FJ, Homan van der Heide JJ, de Fijter HW, et al. Long-term cardiovascular outcome of renal transplant recipients after early conversion to everolimus compared to calcineurin inhibition: results from the randomized controlled MECANO trial. Transpl Int Off J Eur Soc Organ Transplant. (2018) 31:1380–90. doi: 10.1111/tri.13322
78. Jennings DL, Taber DJ. Use of renin-angiotensin-aldosterone system inhibitors within the first eight to twelve weeks after renal transplantation. Ann Pharmacother. (2008) 42:116–20. doi: 10.1345/aph.1K471
79. Gustafsson AB, Gottlieb RA. Heart mitochondria: gates of life and death. Cardiovasc Res. (2008) 77:334–43. doi: 10.1093/cvr/cvm005
80. Yarana C, Sripetchwandee J, Sanit J, Chattipakorn S, Chattipakorn N. Calcium-induced cardiac mitochondrial dysfunction is predominantly mediated by cyclosporine A-dependent mitochondrial permeability transition pore. Arch Med Res. (2012) 43:333–8. doi: 10.1016/j.arcmed.2012.06.010
81. Rajesh KG, Sasaguri S, Ryoko S, Maeda H. Mitochondrial permeability transition-pore inhibition enhances functional recovery after long-time hypothermic heart preservation. Transplantation. (2003) 76:1314–20. doi: 10.1097/01.TP.0000085660.93090.79
82. Xie JR, Yu LN. Cardioprotective effects of cyclosporine A in an in vivo model of myocardial ischemia and reperfusion. Acta Anaesthesiol Scand. (2007) 51:909–13. doi: 10.1111/j.1399-6576.2007.01342.x
83. Griffiths EJ, Halestrap AP. Protection by Cyclosporin A of ischemia/reperfusion-induced damage in isolated rat hearts. J Mol Cell Cardiol. (1993) 25:1461–9. doi: 10.1006/jmcc.1993.1162
84. Mott JL, Zhang D, Freeman JC, Mikolajczak P, Chang SW, Zassenhaus HP. Cardiac disease due to random mitochondrial DNA mutations is prevented by cyclosporin A. Biochem Biophys Res Commun. (2004) 319:1210–5. doi: 10.1016/j.bbrc.2004.05.104
85. Nathan M, Friehs I, Choi YH, Cowan DB, Cao-Danh H, McGowan FX, et al. Cyclosporin a but not FK-506 protects against dopamine-induced apoptosis in the stunned heart. Ann Thorac Surg. (2005) 79:1620–6. doi: 10.1016/j.athoracsur.2004.10.030
86. Gao L, Hicks M, Villanueva JE, Doyle A, Chew HC, Qui MR, et al. Cyclosporine A as a cardioprotective agent during donor heart retrieval, storage, or transportation: benefits and limitations. Transplantation. (2019) 103:1140–51. doi: 10.1097/TP.0000000000002629
87. Hiemstra JA, Gutiérrez-Aguilar M, Marshall KD, McCommis KS, Zgoda PJ, Cruz-Rivera N, et al. A new twist on an old idea part 2: cyclosporine preserves normal mitochondrial but not cardiomyocyte function in mini-swine with compensated heart failure. Physiol Rep. (2014) 2:e12050. doi: 10.14814/phy2.12050
88. Marechal X, Montaigne D, Marciniak C, Marchetti P, Hassoun SM, Beauvillain JC, et al. Doxorubicin-induced cardiac dysfunction is attenuated by ciclosporin treatment in mice through improvements in mitochondrial bioenergetics. Clin Sci. (2011) 121:405–13. doi: 10.1042/CS20110069
89. Joshi MS, Julian MW, Huff JE, Bauer JA, Xia Y, Crouser ED. Calcineurin regulates myocardial function during acute endotoxemia. Am J Respir Crit Care Med. (2006) 173:999–1007. doi: 10.1164/rccm.200411-1507OC
90. Nishinaka Y, Sugiyama S, Yokota M, Saito H, Ozawa T. Protective effect of FK506 on ischemia/reperfusion-induced myocardial damage in canine heart. J Cardiovasc Pharmacol. (1993) 21:448–54. doi: 10.1097/00005344-199303000-00015
91. Sharp WW, Fang YH, Han M, Zhang HJ, Hong Z, Banathy A, et al. Dynamin-related protein 1 (Drp1)-mediated diastolic dysfunction in myocardial ischemia-reperfusion injury: therapeutic benefits of Drp1 inhibition to reduce mitochondrial fission. FASEB J Off Publ Fed Am Soc Exp Biol. (2014) 28:316–26. doi: 10.1096/fj.12-226225
92. Albawardi A, Almarzooqi S, Saraswathiamma D, Abdul-Kader HM, Souid AK, Alfazari AS. The mTOR inhibitor sirolimus suppresses renal, hepatic, and cardiac tissue cellular respiration. Int J Physiol Pathophysiol Pharmacol. (2015) 7:54–60.
93. Khan S, Salloum F, Das A, Xi LW, Vetrovec G, Cukreja R. Rapamycin confers preconditioning-like protection against ischemia–reperfusion injury in isolated mouse heart and cardiomyocytes. J Mol Cell Cardiol. (2006) 41:256–64. doi: 10.1016/j.yjmcc.2006.04.014
94. Johnson N, Khan A, Virji S, Ward JM, Crompton M. Import and processing of heart mitochondrial cyclophilin D. Eur J Biochem. (1999) 263:353–9. doi: 10.1046/j.1432-1327.1999.00490.x
95. Hausenloy DJ, Boston-Griffiths EA, Yellon DM. Cyclosporin A and cardioprotection: from investigative tool to therapeutic agent. Br J Pharmacol. (2012) 165:1235–45. doi: 10.1111/j.1476-5381.2011.01700.x
96. Tennant DA, Durán RV, Gottlieb E. Targeting metabolic transformation for cancer therapy. Nat Rev Cancer. (2010) 10:267–77. doi: 10.1038/nrc2817
97. Zhu J, Hua X, Li D, Zhang J, Xia Q. Rapamycin attenuates mouse liver ischemia and reperfusion injury by inhibiting endoplasmic reticulum stress. Transplant Proc. (2015) 47:1646–52. doi: 10.1016/j.transproceed.2015.05.013
98. Klawitter J, Gottschalk S, Hainz C, Leibfritz D, Christians U, Serkova NJ. Immunosuppressant neurotoxicity in rat brain models: oxidative stress and cellular metabolism. Chem Res Toxicol. (2010) 23:608–19. doi: 10.1021/tx900351q
99. Serkova N, Christians U. Transplantation: toxicokinetics and mechanisms of toxicity of cyclosporine and macrolides. Curr Opin Investig Drugs Lond Engl. (2003) 4:1287–96.
100. Serkova N, Jacobsen W, Niemann CU, Litt L, Benet LZ, Leibfritz D, et al. Sirolimus, but not the structurally related RAD (everolimus), enhances the negative effects of cyclosporine on mitochondrial metabolism in the rat brain. Br J Pharmacol. (2001) 133:875–85. doi: 10.1038/sj.bjp.0704142
101. Tavares P, Reis F, Ribeiro CAF, Teixeira F. Cardiovascular effects of cyclosporin treatment in an experimental model. Rev Port Cardiol Orgao Of Soc Port Cardiol Port J Cardiol Off J Port Soc Cardiol. (2002) 21:141–55.
102. Biary N, Xie C, Kauffman J, Akar FG. Biophysical properties and functional consequences of reactive oxygen species (ROS)-induced ROS release in intact myocardium. J Physiol. (2011) 589:5167–79. doi: 10.1113/jphysiol.2011.214239
103. Oropeza-Almazán Y, Blatter LA. Mitochondrial calcium uniporter complex activation protects against calcium alternans in atrial myocytes. Am J Physiol Heart Circ Physiol. (2020) 319:H873–81. doi: 10.1152/ajpheart.00375.2020
104. Huang Y, Lu CY, Yan W, Gao L, Chen Q, Zhang YJ. [Influence of cyclosporine A on atrial L-type calcium channel alpha1c subunit in a canine model of atrial fibrillation]. Zhonghua Xin Xue Guan Bing Za Zhi. (2009) 37:112–4.
105. Schreiner KD, Kelemen K, Zehelein J, Becker R, Senges JC, Bauer A, et al. Biventricular hypertrophy in dogs with chronic AV block: effects of cyclosporin A on morphology and electrophysiology. Am J Physiol Heart Circ Physiol. (2004) 287:H2891–2898. doi: 10.1152/ajpheart.01051.2003
106. Gordan R, Fefelova N, Gwathmey JK, Xie LH. Iron overload, oxidative stress and calcium mishandling in cardiomyocytes: role of the mitochondrial permeability transition pore. Antioxidants. (2020) 9:758. doi: 10.3390/antiox9080758
107. Ko WJ, Lin FL, Wang SS, Chu SH. Hypomagnesia and arrhythmia corrected by replacing cyclosporine with FK506 in a heart transplant recipient. J Heart Lung Transplant Off Publ Int Soc Heart Transplant. (1997) 16:980–2.
108. Mayer AD, Dmitrewski J, Squifflet JP, Besse T, Grabensee B, Klein B, et al. Multicenter randomized trial comparing tacrolimus (FK506) and cyclosporine in the prevention of renal allograft rejection: a report of the European Tacrolimus Multicenter Renal Study Group. Transplantation. (1997) 64:436–43. doi: 10.1097/00007890-199708150-00012
109. Minematsu T, Ohtani H, Sato H, Iga T. Sustained QT prolongation induced by tacrolimus in guinea pigs. Life Sci. (1999) 65:PL197–202. doi: 10.1016/S0024-3205(99)00396-3
110. Minematsu T, Ohtani H, Yamada Y, Sawada Y, Sato H, Iga T. Quantitative relationship between myocardial concentration of tacrolimus and QT prolongation in guinea pigs: pharmacokinetic/pharmacodynamic model incorporating a site of adverse effect. J Pharmacokinet Pharmacodyn. (2001) 28:533–54.
111. McCall E, Li L, Satoh H, Shannon TR, Blatter LA, Bers DM. Effects of FK-506 on contraction and Ca2+ transients in rat cardiac myocytes. Circ Res. (1996) 79:1110–21. doi: 10.1161/01.RES.79.6.1110
112. DuBell WH, Wright PA, Lederer WJ, Rogers TB. Effect of the immunosupressant FK506 on excitation—contraction coupling and outward K+ currents in rat ventricular myocytes. J Physiol. (1997) 501:509–16. doi: 10.1111/j.1469-7793.1997.509bm.x
113. Bell WH, Gaa ST. Lederer WJ, Rogers TB. Independent inhibition of calcineurin and K+ currents by the immunosuppressant FK-506 in rat ventricle. Am J Physiol. (1998) 275:H2041–2052. doi: 10.1152/ajpheart.1998.275.6.H2041
114. Katanosaka Y, Iwata Y, Kobayashi Y, Shibasaki F, Wakabayashi S, Shigekawa M. Calcineurin inhibits Na+/Ca2+ exchange in phenylephrine-treated hypertrophic cardiomyocytes. J Biol Chem. (2005) 280:5764–72. doi: 10.1074/jbc.M410240200
115. Li X, Bilali A, Qiao R, Paerhati T, Yang Y. Association of the PPARγ/PI3K/Akt pathway with the cardioprotective effects of tacrolimus in myocardial ischemic/reperfusion injury. Mol Med Rep. (2018) 17:6759–67.
116. Hodak SP, Moubarak JB, Rodriguez I, Gelfand MC, Alijani MR, Tracy CM, et al. prolongation and near fatal cardiac arrhythmia after intravenous tacrolimus administration: a case report. Transplantation. (1998) 66:535–7. doi: 10.1097/00007890-199808270-00021
117. Kim BR, Shin HS, Jung YS, Rim H. A case of tacrolimus-induced supraventricular arrhythmia after kidney transplantation. Sáo Paulo Med J Rev Paul Med. (2013) 131:205–7. doi: 10.1590/1516-3180.2013.1313472
118. Nishimura M, Kim K, Uchiyama A, Fujino Y, Nishimura S, Taenaka N, et al. Tacrolimus-induced life-threatening arrhythmia in a pediatric liver-transplant patient. Intensive Care Med. (2002) 28:1683–4. doi: 10.1007/s00134-002-1479-z
119. Eisen HJ, Kobashigawa J, Keogh A, Bourge R, Renlund D, Mentzer R, et al. Three-year results of a randomized, double-blind, controlled trial of mycophenolate mofetil vs. azathioprine in cardiac transplant recipients. J Heart Lung Transplant Off Publ Int Soc Heart Transplant. (2005) 24:517–25. doi: 10.1016/j.healun.2005.02.002
120. Cassinotti A, Massari A, Ferrara E, Greco S, Bosani M, Ardizzone S, et al. New onset of atrial fibrillation after introduction of azathioprine in ulcerative colitis: case report and review of the literature. Eur J Clin Pharmacol. (2007) 63:875–8. doi: 10.1007/s00228-007-0328-y
121. Riccioni G, Bucciarelli V, Di Ilio E, Scotti L, Aceto A, D'Orazio N, et al. Recurrent atrial fibrillation in a patient with ulcerative colitis treated with azathioprine: case report and review of the literature. Int J Immunopathol Pharmacol. (2011) 24:247–9. doi: 10.1177/039463201102400131
122. Dodd HJ, Tatnall FM, Sarkany I. Fast atrial fibrillation induced by treatment of psoriasis with azathioprine. Br Med J Clin Res Ed. (1985) 291:706. doi: 10.1136/bmj.291.6497.706
123. Murphy G, Fulton RA, Keegan DJ. Fast atrial fibrillation induced by azathioprine. Br Med J Clin Res Ed. (1985) 291:1049–1049. doi: 10.1136/bmj.291.6501.1049-a
124. Saygili E, Rana OR, Günzel C, Rackauskas G, Saygili E, Noor-Ebad F, et al. Rate and irregularity of electrical activation during atrial fibrillation affect myocardial NGF expression via different signalling routes. Cell Signal. (2012) 24:99–105. doi: 10.1016/j.cellsig.2011.08.007
125. Sommerer C, Suwelack B, Dragun D, Schenker P, Hauser IA, Witzke O, et al. An open-label, randomized trial indicates that everolimus with tacrolimus or cyclosporine is comparable to standard immunosuppression in de novo kidney transplant patients. Kidney Int. (2019) 96:231–44. doi: 10.1016/j.kint.2019.01.041
126. U.S. Multicenter FK506 Liver Study Group. A comparison of tacrolimus (FK 506) and cyclosporine for immunosuppression in liver transplantation. N Engl J Med. (1994) 331:1110–5. doi: 10.1056/NEJM199410273311702
127. Hošková L, Málek I, Kautzner J, Honsová E, van Dokkum RPE, Husková Z, et al. Tacrolimus-induced hypertension and nephrotoxicity in Fawn-Hooded rats are attenuated by dual inhibition of renin–angiotensin system. Hypertens Res. (2014) 37:724–32. doi: 10.1038/hr.2014.79
128. Grześk E, Malinowski B, Wiciński M, Szadujkis-Szadurska K, Sinjab TA, Manysiak S, et al. Cyclosporine-A, but not tacrolimus significantly increases reactivity of vascular smooth muscle cells. Pharmacol Rep PR. (2016) 68:201–5. doi: 10.1016/j.pharep.2015.08.012
129. Tekes E, Soydan G, Tuncer M. The role of endothelin in FK506-induced vascular reactivity changes in rat perfused kidney. Eur J Pharmacol. (2005) 517:92–6. doi: 10.1016/j.ejphar.2005.05.029
130. Taylor DO, Barr ML, Radovancevic B, Renlund DG, Mentzer RM, Smart FW, et al. A randomized, multicenter comparison of tacrolimus and cyclosporine immunosuppressive regimens in cardiac transplantation: decreased hyperlipidemia and hypertension with tacrolimus. J Heart Lung Transplant Off Publ Int Soc Heart Transplant. (1999) 18:336–45. doi: 10.1016/S1053-2498(98)00060-6
131. Stegall MD, Wachs ME, Everson G, Steinberg T, Bilir B, Shrestha R, et al. Prednisone withdrawal 14 days after liver transplantation with mycophenolate: a prospective trial of cyclosporine and tacrolimus. Transplantation. (1997) 64:1755–60. doi: 10.1097/00007890-199712270-00023
132. Canzanello VJ, Schwartz L, Taler SJ, Textor SC, Wiesner RH, Porayko MK, et al. Evolution of cardiovascular risk after liver transplantation: a comparison of cyclosporine A and tacrolimus (FK506). Liver Transplant Surg Off Publ Am Assoc Study Liver Dis Int Liver Transplant Soc. (1997) 3:1–9. doi: 10.1002/lt.500030101
133. Temiz-Resitoglu M, Guden DS, Senol SP, Vezir O, Sucu N, Kibar D, et al. Pharmacological inhibition of mammalian target of rapamycin attenuates deoxycorticosterone acetate salt–induced hypertension and related pathophysiology: regulation of oxidative stress, inflammation, and cardiovascular hypertrophy in male rats. J Cardiovasc Pharmacol. (2022) 79:355–67. doi: 10.1097/FJC.0000000000001187
134. Kim JA, Jang HJ, Martinez-Lemus LA, Sowers JR. Activation of mTOR/p70S6 kinase by ANG II inhibits insulin-stimulated endothelial nitric oxide synthase and vasodilation. Am J Physiol Endocrinol Metab. (2012) 302:E201–208. doi: 10.1152/ajpendo.00497.2011
135. He Y, Zuo C, Jia D, Bai P, Kong D, Chen D, et al. Loss of DP1 aggravates vascular remodeling in pulmonary arterial hypertension via mTORC1 signaling. Am J Respir Crit Care Med. (2020) 201:1263–76. doi: 10.1164/rccm.201911-2137OC
136. Trinh B, Burkard T. The mTOR-inhibitor everolimus reduces hypervolemia in patients with primary aldosteronism. Minerva Endocrinol. (2021) 20:1. doi: 10.23736/S2724-6507.21.03382-0
137. Andreassen AK, Broch K, Eiskjær H, Karason K, Gude E, Mølbak D, et al. Blood Pressure in De Novo Heart Transplant Recipients Treated With Everolimus Compared With a Cyclosporine-based Regimen: Results From the Randomized SCHEDULE Trial. Transplantation. (2019) 103:781–8. doi: 10.1097/TP.0000000000002445
138. Seyfarth HJ, Hammerschmidt S, Halank M, Neuhaus P, Wirtz HR. Everolimus in patients with severe pulmonary hypertension: a safety and efficacy pilot trial. Pulm Circ. (2013) 3:632–8. doi: 10.1086/674311
139. Bendtsen MAF, Grimm D, Bauer J, Wehland M, Wise P, Magnusson NE, et al. Hypertension Caused by Lenvatinib and everolimus in the treatment of metastatic renal cell carcinoma. Int J Mol Sci. (2017) 18:1736. doi: 10.3390/ijms18081736
140. Lewis MJ, D'Cruz D. Adhesion molecules, mycophenolate mofetil and systemic lupus erythematosus. Lupus. (2005) 14 Suppl 1:s17–26. doi: 10.1177/096120330501400105
141. Taylor EB, Ryan MJ. Immunosuppression with mycophenolate mofetil attenuates hypertension in an experimental model of autoimmune disease. J Am Heart Assoc. (2017) 6:e005394. doi: 10.1161/JAHA.116.005394
142. Bravo Y, Quiroz Y, Ferrebuz A, Vaziri ND, Rodríguez-Iturbe B. Mycophenolate mofetil administration reduces renal inflammation, oxidative stress, and arterial pressure in rats with lead-induced hypertension. Am J Physiol Renal Physiol. (2007) 293:F616–623. doi: 10.1152/ajprenal.00507.2006
143. Moes AD, Severs D, Verdonk K, van der Lubbe N, Zietse R, Danser AHJ, et al. Mycophenolate mofetil attenuates DOCA-salt hypertension: effects on vascular tone. Front Physiol. (2018) 9:578. doi: 10.3389/fphys.2018.00578
144. Quiroz Y, Pons H, Gordon KL, Rincón J, Chávez M, Parra G, et al. Mycophenolate mofetil prevents salt-sensitive hypertension resulting from nitric oxide synthesis inhibition. Am J Physiol Renal Physiol. (2001) 281:F38–47. doi: 10.1152/ajprenal.2001.281.1.F38
145. Herrera J, Ferrebuz A, MacGregor EG, Rodriguez-Iturbe B. Mycophenolate mofetil treatment improves hypertension in patients with psoriasis and rheumatoid arthritis. J Am Soc Nephrol JASN. (2006) 17:S218–225. doi: 10.1681/ASN.2006080918
146. Tinsley JH, Chiasson VL, South S, Mahajan A, Mitchell BM. Immunosuppression improves blood pressure and endothelial function in a rat model of pregnancy-induced hypertension. Am J Hypertens. (2009) 22:1107–14. doi: 10.1038/ajh.2009.125
147. Botros L, Szulcek R, Jansen SMA, Kurakula K, Goumans MJTH, van Kuilenburg ABP, et al. The effects of mercaptopurine on pulmonary vascular resistance and BMPR2 expression in pulmonary arterial hypertension. Am J Respir Crit Care Med. (2020) 202:296–9. doi: 10.1164/rccm.202003-0473LE
148. Thompson ME, Shapiro AP, Johnsen AM, Itzkoff JM, Hardesty RL, Griffith BP, et al. The contrasting effects of cyclosporin-A and azathioprine on arterial blood pressure and renal function following cardiac transplantation. Int J Cardiol. (1986) 11:219–29. doi: 10.1016/0167-5273(86)90181-6
149. Ventura HO, Mehra MR, Stapleton DD, Smart FW. Cyclosporine-induced hypertension in cardiac transplantation. Med Clin North Am. (1997) 81:1347–57. doi: 10.1016/S0025-7125(05)70587-3
150. Textor SC, Taler SJ, Canzanello VJ, Schwartz L, Augustine JE. Posttransplantation hypertension related to calcineurin inhibitors. Liver Transplant Off Publ Am Assoc Study Liver Dis Int Liver Transplant Soc. (2000) 6:521–30. doi: 10.1053/jlts.2000.9737
151. Tang Q, Zheng YM, Song T, Reyes-García J, Wang C, Wang YX. Inhibition of big-conductance Ca2+-activated K+ channels in cerebral artery (vascular) smooth muscle cells is a major novel mechanism for tacrolimus-induced hypertension. Pflugers Arch. (2021) 473:53–66. doi: 10.1007/s00424-020-02470-6
152. Rodrigues-Diez R, González-Guerrero C, Ocaña-Salceda C, Rodrigues-Diez RR, Egido J, Ortiz A, et al. Calcineurin inhibitors cyclosporine A and tacrolimus induce vascular inflammation and endothelial activation through TLR4 signaling. Sci Rep. (2016) 6:27915. doi: 10.1038/srep27915
153. Porter GA, Bennett WM, Sheps SG. Cyclosporine-associated hypertension. National High Blood Pressure Education Program Arch Intern Med. (1990) 150:280–3. doi: 10.1001/archinte.150.2.280
154. Blum A, Aravot D. Heart transplantation–an update. Clin Cardiol. (1996) 19:930–8. doi: 10.1002/clc.4960191206
155. Miller LW, Schlant RC, Kobashigawa J, Kubo S, Renlund DG. 24th Bethesda conference: Cardiac transplantation. Task Force 5: Complications J Am Coll Cardiol. (1993) 22:41–54. doi: 10.1016/0735-1097(93)90814-H
156. Oberbauer R, Kreis H, Johnson RWG, Mota A, Claesson K, Ruiz JC, et al. Long-term improvement in renal function with sirolimus after early cyclosporine withdrawal in renal transplant recipients: 2-year results of the Rapamune Maintenance Regimen Study. Transplantation. (2003) 76:364–70. doi: 10.1097/01.TP.0000074360.62032.39
157. Sessa A, Esposito A, Giliberti A, Iavicoli G, Costa C, Bergallo M, et al. Immunosuppressive Agents and Metabolic Factors of Cardiovascular Risk in Renal Transplant Recipients. Transplant Proc. (2009) 41:1178–82. doi: 10.1016/j.transproceed.2009.02.045
158. Boots JMM, Christiaans MHL, van Hooff JP. Effect of immunosuppressive agents on long-term survival of renal transplant recipients. Drugs. (2004) 64:2047–73. doi: 10.2165/00003495-200464180-00004
159. Mennander A, Tiisala S, Paavonen T, Halttunen J, Häyry P. Chronic rejection of rat aortic allograft. II Administration of cyclosporin induces accelerated allograft arteriosclerosis. Transpl Int Off J Eur Soc Organ Transplant. (1991) 4:173–9. doi: 10.1007/BF00335340
160. Mennander A, Paavonen T, Häyry P. Cyclosporine-induced endothelialitis and accelerated arteriosclerosis in chronic allograft rejection. Transplant Proc. (1992) 24:341.
161. Diederich D, Skopec J, Diederich A, Dai FX. Cyclosporine produces endothelial dysfunction by increased production of superoxide. Hypertens Dallas Tex. (1994) 23:957–61. doi: 10.1161/01.HYP.23.6.957
162. Redondo-Horcajo M, Romero N, Martínez-Acedo P, Martínez-Ruiz A, Quijano C, Lourenço CF, et al. Cyclosporine A-induced nitration of tyrosine 34 MnSOD in endothelial cells: role of mitochondrial superoxide. Cardiovasc Res. (2010) 87:356–65. doi: 10.1093/cvr/cvq028
163. Gao SZ, Schroeder JS, Alderman EL, Hunt SA, Valantine HA, Wiederhold V, et al. Prevalence of accelerated coronary artery disease in heart transplant survivors. comparison of cyclosporine and azathioprine regimens. Circulation. (1989) 80:III100–105.
164. Mehra MR, Ventura HO, Chambers RB, Ramireddy K, Smart FW, Stapleton DD. The prognostic impact of immunosuppression and cellular rejection on cardiac allograft vasculopathy: time for a reappraisal. J Heart Lung Transplant Off Publ Int Soc Heart Transplant. (1997) 16:743–51.
165. Gamba A, Mamprin F, Fiocchi R, Senni M, Troise G, Ferrazzi P, et al. The risk of coronary artery disease after heart transplantation is increased in patients receiving low-dose cyclosporine, regardless of blood cyclosporine levels. Clin Cardiol. (1997) 20:767–72. doi: 10.1002/clc.4960200911
166. De Lima JJ, Xue H, Coburn L, Andoh TF, McCarron DA, Bennett WM, et al. Effects of FK506 in rat and human resistance arteries. Kidney Int. (1999) 55:1518–27. doi: 10.1046/j.1523-1755.1999.00366.x
167. Klauss V, König A, Spes C, Meiser B, Rieber J, Siebert U, et al. Cyclosporine vs. tacrolimus (FK 506) for prevention of cardiac allograft vasculopathy. Am J Cardiol. (2000) 85:266–9. doi: 10.1016/S0002-9149(99)00631-1
168. Reichart B, Meiser B, Viganò M, Rinaldi M, Martinelli L, Yacoub M, et al. European multicenter tacrolimus (FK506) Heart Pilot Study: one-year results–European tacrolimus multicenter heart study group. J Heart Lung Transplant Off Publ Int Soc Heart Transplant. (1998) 17:775–81.
169. Koglin J, Muscholl M, Petrakopoulou P, Groetzner J, Weis M, Meiser B, et al. Tacrolimus excels cyclosporine a in prevention of cardiac allograft vasculopathy—a prospective IVUS, angiography and doppler study. J Heart Lung Transplant. (2002) 21:68. doi: 10.1016/S1053-2498(01)00471-5
170. Cheng C, Tempel D, Oostlander A, Helderman F, Gijsen F, Wentzel J, et al. Rapamycin modulates the eNOS vs. shear stress relationship. Cardiovasc Res. (2008) 78:123–9. doi: 10.1093/cvr/cvm103
171. Pham SM, Shears LL, Kawaharada N, Li S, Venkataramanan R, Sehgal S. High local production of nitric oxide as a possible mechanism by which rapamycin prevents transplant arteriosclerosis. Transplant Proc. (1998) 30:953–4. doi: 10.1016/S0041-1345(98)00107-9
172. Poon M, Marx SO, Gallo R, Badimon JJ, Taubman MB, Marks AR. Rapamycin inhibits vascular smooth muscle cell migration. J Clin Invest. (1996) 98:2277–83. doi: 10.1172/JCI119038
173. Marx SO, Jayaraman T, Go LO, Marks AR. Rapamycin-FKBP inhibits cell cycle regulators of proliferation in vascular smooth muscle cells. Circ Res. (1995) 76:412–7. doi: 10.1161/01.RES.76.3.412
174. Bruemmer D, Yin F, Liu J, Kiyono T, Fleck E, Van Herle AJ, et al. Rapamycin inhibits E2F-dependent expression of minichromosome maintenance proteins in vascular smooth muscle cells. Biochem Biophys Res Commun. (2003) 303:251–8. doi: 10.1016/S0006-291X(03)00343-7
175. Mancini D, Pinney S, Burkhoff D, LaManca J, Itescu S, Burke E, et al. Use of rapamycin slows progression of cardiac transplantation vasculopathy. Circulation. (2003) 108:48–53. doi: 10.1161/01.CIR.0000070421.38604.2B
176. Potena L, Fearon WF, Sydow K, Holweg C, Luikart H, Chin C, et al. Asymmetric dimethylarginine and cardiac allograft vasculopathy progression: modulation by sirolimus. Transplantation. (2008) 85:827–33. doi: 10.1097/TP.0b013e318166a3a4
177. Joannidès R, Monteil C, de Ligny BH, Westeel PF, Iacob M, Thervet E, et al. Immunosuppressant regimen based on sirolimus decreases aortic stiffness in renal transplant recipients in comparison to cyclosporine. Am J Transplant Off J Am Soc Transplant Am Soc Transpl Surg. (2011) 11:2414–22. doi: 10.1111/j.1600-6143.2011.03697.x
178. Seckinger J, Sommerer C, Hinkel UP, Hoffmann O, Zeier M, Schwenger V. Switch of immunosuppression from cyclosporine A to everolimus: impact on pulse wave velocity in stable de-novo renal allograft recipients. J Hypertens. (2008) 26:2213–9. doi: 10.1097/HJH.0b013e32830ef940
179. Sousa JE, Costa MA, Abizaid A, Abizaid AS, Feres F, Pinto IM, et al. Lack of neointimal proliferation after implantation of sirolimus-coated stents in human coronary arteries: a quantitative coronary angiography and three-dimensional intravascular ultrasound study. Circulation. (2001) 103:192–5. doi: 10.1161/01.CIR.103.2.192
180. Baetta R, Granata A, Canavesi M, Ferri N, Arnaboldi L, Bellosta S, et al. Everolimus inhibits monocyte/macrophage migration in vitro and their accumulation in carotid lesions of cholesterol-fed rabbits. J Pharmacol Exp Ther. (2009) 328:419–25. doi: 10.1124/jpet.108.144147
181. Beutner F, Brendel D, Teupser D, Sass K, Baber R, Mueller M, et al. Effect of everolimus on pre-existing atherosclerosis in LDL-receptor deficient mice. Atherosclerosis. (2012) 222:337–43. doi: 10.1016/j.atherosclerosis.2012.03.003
182. Mueller MA, Beutner F, Teupser D, Ceglarek U, Thiery J. Prevention of atherosclerosis by the mTOR inhibitor everolimus in LDLR–/– mice despite severe hypercholesterolemia. Atherosclerosis. (2008) 198:39–48. doi: 10.1016/j.atherosclerosis.2007.09.019
183. Eisen HJ, Tuzcu EM, Dorent R, Kobashigawa J, Mancini D., Valantine-von Kaeppler HA, et al. Everolimus for the prevention of allograft rejection and vasculopathy in cardiac-transplant recipients. N Engl J Med. (2003) 349:847–58. doi: 10.1056/NEJMoa022171
184. Norum HM, Michelsen AE, Lekva T, Arora S, Otterdal K, Olsen MB, et al. Circulating delta-like Notch ligand 1 is correlated with cardiac allograft vasculopathy and suppressed in heart transplant recipients on everolimus-based immunosuppression. Am J Transplant Off J Am Soc Transplant Am Soc Transpl Surg. (2019) 19:1050–60. doi: 10.1111/ajt.15141
185. Placebo-controlled study of mycophenolate mofetil combined with cyclosporin and corticosteroids for prevention of acute rejection. European Mycophenolate Mofetil cooperative study group. Lancet Lond Engl. (1995) 345:1321–5. doi: 10.1016/S0140-6736(95)92534-1
186. Kobashigawa J, Miller L, Renlund D, Mentzer R, Alderman E, Bourge R, et al. A randomized active-controlled trial of mycophenolate mofetil in heart transplant recipients. Mycophenolate Mofetil Investigators Transplantation. (1998) 66:507–15. doi: 10.1097/00007890-199808270-00016
187. Krötz F, Keller M, Derflinger S, Schmid H, Gloe T, Bassermann F, et al. Mycophenolate acid inhibits endothelial NAD(P)H oxidase activity and superoxide formation by a rac1-dependent mechanism. Hypertension. (2007) 49:201–8. doi: 10.1161/01.HYP.0000251162.14782.d4
188. Chang CZ, Wu SC, Kwan AL, Lin CL, Hwang SL. 6-Mercaptopurine reverses experimental vasospasm and alleviates the production of endothelins in NO-independent mechanism-a laboratory study. Acta Neurochir (Wien). (2011) 153:939–49. doi: 10.1007/s00701-010-0865-5
189. Pols TWH, Bonta PI, Pires NMM, Otermin I, Vos M, de Vries MR, et al. 6-mercaptopurine inhibits atherosclerosis in apolipoprotein e*3-leiden transgenic mice through atheroprotective actions on monocytes and macrophages. Arterioscler Thromb Vasc Biol. (2010) 30:1591–7. doi: 10.1161/ATVBAHA.110.205674
190. Weigel G, Griesmacher A, DeAbreu RA, Wolner E, Mueller MM. Azathioprine and 6-mercaptopurine alter the nucleotide balance in endothelial cells. Thromb Res. (1999) 94:87–94. doi: 10.1016/S0049-3848(98)00199-6
191. Valantine H. Cardiac allograft vasculopathy after heart transplantation: risk factors and management. J Heart Lung Transplant Off Publ Int Soc Heart Transplant. (2004) 23:S187–193. doi: 10.1016/j.healun.2004.03.009
192. Atkison PR, Joubert GI, Guiraudon C, Armstrong R, Wall W, Asfar S, et al. Arteritis and increased intracellular calcium as a possible mechanism for tacrolimus-related cardiac toxicity in a pediatric transplant recipient. Transplantation. (1997) 64:773–5. doi: 10.1097/00007890-199709150-00020
193. Autieri MV. Allograft-induced proliferation of vascular smooth muscle cells: potential targets for treating transplant vasculopathy. Curr Vasc Pharmacol. 1:1–9. doi: 10.2174/1570161033386772
194. Vitiello D, Neagoe PE, Sirois MG, White M. Effect of everolimus on the immunomodulation of the human neutrophil inflammatory response and activation. Cell Mol Immunol. (2015) 12:40–52. doi: 10.1038/cmi.2014.24
195. Martinet W, De Loof H, De Meyer GRY. mTOR inhibition: a promising strategy for stabilization of atherosclerotic plaques. Atherosclerosis. (2014) 233:601–7. doi: 10.1016/j.atherosclerosis.2014.01.040
196. Wessely R. New drug-eluting stent concepts. Nat Rev Cardiol. (2010) 7:194–203. doi: 10.1038/nrcardio.2010.14
197. Kobayashi S, Kishimoto T, Kamata S, Otsuka M, Miyazaki M, Ishikura H. Rapamycin, a specific inhibitor of the mammalian target of rapamycin, suppresses lymphangiogenesis and lymphatic metastasis. Cancer Sci. (2007) 98:726–33. doi: 10.1111/j.1349-7006.2007.00439.x
198. Huber S, Bruns CJ, Schmid G, Hermann PC, Conrad C, Niess H, et al. Inhibition of the mammalian target of rapamycin impedes lymphangiogenesis. Kidney Int. (2007) 71:771–7. doi: 10.1038/sj.ki.5002112
199. Verheijen RB, Atrafi F, Schellens JHM, Beijnen JH, Huitema ADR, Mathijssen RHJ, et al. Pharmacokinetic optimization of everolimus dosing in oncology: a randomized crossover trial. Clin Pharmacokinet. (2018) 57:637–44. doi: 10.1007/s40262-017-0582-9
200. Allison AC, Eugui EM. Preferential suppression of lymphocyte proliferation by mycophenolic acid and predicted long-term effects of mycophenolate mofetil in transplantation. Transplant Proc. (1994) 26:3205–10.
201. Voloshyna I, Teboul I, Kasselman LJ, Salama M, Carsons SE, DeLeon J, et al. Macrophage lipid accumulation in the presence of immunosuppressive drugs mycophenolate mofetil and cyclosporin A. Inflamm Res Off J Eur Histamine Res Soc Al. (2019) 68:787–99. doi: 10.1007/s00011-019-01262-8
202. Kasiske BL, Tortorice KL, Heim-Duthoy KL, Awni WM, Rao KV. The adverse impact of cyclosporine on serum lipids in renal transplant recipients. Am J Kidney Dis Off J Natl Kidney Found. (1991) 17:700–7. doi: 10.1016/S0272-6386(12)80355-6
203. Ballantyne CM, Podet EJ, Patsch WP, Harati Y, Appel V, Gotto AM, et al. Effects of cyclosporine therapy on plasma lipoprotein levels. JAMA. (1989) 262:53–6. doi: 10.1001/jama.262.1.53
204. Tory R, Sachs-Barrable K, Hill JS, Wasan KM. Cyclosporine A and Rapamycin induce in vitro cholesteryl ester transfer protein activity, and suppress lipoprotein lipase activity in human plasma. Int J Pharm. (2008) 358:219–23. doi: 10.1016/j.ijpharm.2008.03.026
205. Derfler K, Hayde M, Heinz G, Hirschl MM, Steger G, Hauser AC, et al. Decreased postheparin lipolytic activity in renal transplant recipients with cyclosporin A. Kidney Int. (1991) 40:720–7. doi: 10.1038/ki.1991.266
206. Apanay DC, Neylan JF, Ragab MS, Sgoutas DS. Cyclosporine increases the oxidizability of low-density lipoproteins in renal transplant recipients. Transplantation. (1994) 58:663–9. doi: 10.1097/00007890-199409000-00005
207. Cofan F, Cofan M, Campos B, Guerra R, Campistol JM, Oppenheimer F. Effect of calcineurin inhibitors on low-density lipoprotein oxidation. Transplant Proc. (2005) 37:3791–3. doi: 10.1016/j.transproceed.2005.10.068
208. Bai L, Gabriels K, Wijnands E, Rousch M, Daemen MJAP, Tervaert JWC, et al. Low- but not high-dose FK506 treatment confers atheroprotection due to alternative macrophage activation and unaffected cholesterol levels. Thromb Haemost. (2010) 104:143–50. doi: 10.1160/TH09-07-0502
209. Jiang Y, Xie XB, Peng LK, Peng FH, Lan GB, Wang Y, et al. Dyslipidemia in human kidney transplant recipients receiving cyclosporine and tacrolimus is associated with different expression of CD36 on peripheral blood monocytes. Transplant Proc. (2011) 43:1612–5. doi: 10.1016/j.transproceed.2011.03.015
210. Deleuze S, Garrigue V, Delmas S, Chong G, Swarcz I, Cristol JP, et al. New onset dyslipidemia after renal transplantation: is there a difference between tacrolimus and cyclosporine? Transplant Proc. (2006) 38:2311–3. doi: 10.1016/j.transproceed.2006.06.125
211. Steinmüller TM, Gräf KJ, Schleicher J, Leder K, Bechstein WO, Mueller AR, et al. The effect of FK506 vs. cyclosporine on glucose and lipid metabolism–a randomized trial. Transplantation. (1994) 58:669–74. doi: 10.1097/00007890-199409000-00006
212. Abouljoud MS, Levy MF, Klintmalm GB. Hyperlipidemia after liver transplantation: long-term results of the FK506/cyclosporine A US multicenter trial. US Multicenter Study Group Transplant Proc. (1995) 27:1121–3.
213. Claesson K, Mayer AD, Squifflet JP, Grabensee B, Eigler FW, Behrend M, et al. Lipoprotein patterns in renal transplant patients: a comparison between FK 506 and cyclosporine a patients. Transplant Proc. (1998) 30:1292–4. doi: 10.1016/S0041-1345(98)00246-2
214. Zhou YD, Cao XQ, Liu ZH, Cao YJ, Liu CF, Zhang YL, et al. Rapamycin inhibits oxidized low density lipoprotein uptake in human umbilical vein endothelial cells via mTOR/NF-κB/LOX-1 pathway. PLoS One. (2016) 11:e0146777. doi: 10.1371/journal.pone.0146777
215. Choi SK, Kwon Y, Byeon S, Lee YH. Stimulation of autophagy improves vascular function in the mesenteric arteries of type 2 diabetic mice. Exp Physiol. (2020) 105:192–200. doi: 10.1113/EP087737
216. Ma KL, Ruan XZ, Powis SH, Moorhead JF, Varghese Z. Anti-atherosclerotic effects of sirolimus on human vascular smooth muscle cells. Am J Physiol Heart Circ Physiol. (2007) 292:H2721–2728. doi: 10.1152/ajpheart.01174.2006
217. Ai D, Chen C, Han S, Ganda A, Murphy AJ, Haeusler R, et al. Regulation of hepatic LDL receptors by mTORC1 and PCSK9 in mice. J Clin Invest. (2012) 122:1262–70. doi: 10.1172/JCI61919
218. Brattström C, Wilczek H, Tydén G, Böttiger Y, Säwe J, Groth CG. Hyperlipidemia in renal transplant recipients treated with sirolimus (rapamycin). Transplantation. (1998) 65:1272–4. doi: 10.1097/00007890-199805150-00023
219. Tur MD, Garrigue V, Vela C, Dupuy AM, Descomps B, Cristol JP, et al. Apolipoprotein CIII is upregulated by anticalcineurins and rapamycin: implications in transplantation-induced dyslipidemia. Transplant Proc. (2000) 32:2783–4. doi: 10.1016/S0041-1345(00)01884-4
220. Levy G, Schmidli H, Punch J, Tuttle-Newhall E, Mayer D, Neuhaus P, et al. Safety, tolerability, and efficacy of everolimus in de novo liver transplant recipients: 12- and 36-month results. Liver Transplant Off Publ Am Assoc Study Liver Dis Int Liver Transplant Soc. (2006) 12:1640–8. doi: 10.1002/lt.20707
221. Kasiske BL, de Mattos A, Flechner SM, Gallon L, Meier-Kriesche HU, Weir MR, et al. Mammalian Target of Rapamycin Inhibitor Dyslipidemia in Kidney Transplant Recipients. Am J Transplant. (2008) 8:1384–92. doi: 10.1111/j.1600-6143.2008.02272.x
222. Rosing K, Fobker M, Kannenberg F, Gunia S., Dell'Aquila AM, Kwiecien R, et al. Everolimus therapy is associated with reduced lipoprotein-associated phospholipase A2 (Lp-Pla2) activity and oxidative stress in heart transplant recipients. Atherosclerosis. (2013) 230:164–70. doi: 10.1016/j.atherosclerosis.2013.07.007
223. Engelen MA, Amler S, Welp H, Vahlhaus C, Gunia S, Sindermann JR, et al. Prospective study of everolimus with calcineurin inhibitor-free immunosuppression in maintenance heart transplant patients: results at 2 years. Transplantation. (2011) 91:1159–65. doi: 10.1097/TP.0b013e31821774bd
224. Sato S, Akamine Y, Kagaya H, Saito M, Inoue T, Numakura K, et al. Changes in PCSK9 and LDL cholesterol concentrations by everolimus treatment and their effects on polymorphisms in PCSK9 and mTORC1. Pharmacol Rep PR. (2020) 72:622–30. doi: 10.1007/s43440-020-00090-6
225. Schreiber TC, Greenstein SM, Kim DY, Calderon TM, Sun S, Schechner RS, et al. Effect of Mycophenolate Mofetil on Atherosclerosis in a Rabbit Model: Initial Histologic and Immunohistochemical Analyses 11Supported by Roche, Inc (Nutley, NJ). Transplant Proc. (1998) 30:961–2. doi: 10.1016/S0041-1345(98)00111-0
226. Perrea DN, Moulakakis KG, Poulakou MV, Vlachos IS, Nikiteas N, Kostakis A. Correlation between lipid abnormalities and immunosuppressive therapy in renal transplant recipients with stable renal function. Int Urol Nephrol. (2008) 40:521–7. doi: 10.1007/s11255-007-9266-y
227. van den Dorpel MA, Ghanem H, Rischen-Vos J, Man in't Veld AJ, Jansen H, Weimar W. Conversion from cyclosporine A to azathioprine treatment improves LDL oxidation in kidney transplant recipients. Kidney Int. (1997) 51:1608–12. doi: 10.1038/ki.1997.221
228. Akman B, Uyar M, Afsar B, Sezer S, Ozdemir FN, Haberal M. Lipid profile during azathioprine or mycophenolate mofetil combinations with cyclosporine and steroids. Transplant Proc. (2007) 39:135–7. doi: 10.1016/j.transproceed.2006.10.210
229. de Groen PC. Cyclosporine, low-density lipoprotein, and cholesterol. Mayo Clin Proc. (1988) 63:1012–21. doi: 10.1016/S0025-6196(12)64916-7
230. Rayyes O, Wallmark A, Florén C. Cyclosporine inhibits catabolism of low-density lipoproteins in HepG2 cells by about 25%. Hepatology. (1996) 24:613–9. doi: 10.1002/hep.510240325
231. López-Miranda J, Vilella E, Pérez-Jiménez F, Espino A., Jiménez-Perepérez José A, Masana L, et al. Low-density lipoprotein metabolism in rats treated with cyclosporine. Metabolism. (1993) 42:678–83. doi: 10.1016/0026-0495(93)90232-D
232. Kockx M, Kritharides L. Chapter 25—Cyclosporin A-Induced Dyslipidemia and LDL Receptors. In: Patel VB, editor. The Molecular Nutrition of Fats [Internet]. Academic Pr. (2019). p. 323–33. Available online at: https://www.sciencedirect.com/science/article/pii/B9780128112977000251 (accessed June 18, 2022).
233. MacDonald AS. RAPAMUNE Global Study Group. A worldwide, phase III, randomized, controlled, safety and efficacy study of a sirolimus/cyclosporine regimen for prevention of acute rejection in recipients of primary mismatched renal allografts. Transplantation. (2001) 71:271–80. doi: 10.1097/00007890-200101270-00019
234. Simha V, Qin S, Shah P, Smith BH, Kremers WK, Kushwaha S, et al. Sirolimus therapy is associated with elevation in circulating PCSK9 levels in cardiac transplant patients. J Cardiovasc Transl Res. (2017) 10:9–15. doi: 10.1007/s12265-016-9719-8
235. Vitko S, Tedesco H, Eris J, Pascual J, Whelchel J, Magee JC, et al. Everolimus with optimized cyclosporine dosing in renal transplant recipients: 6-month safety and efficacy results of two randomized studies. Am J Transplant Off J Am Soc Transplant Am Soc Transpl Surg. (2004) 4:626–35. doi: 10.1111/j.1600-6143.2004.00389.x
236. Holdaas H, Potena L, Saliba F. mTOR inhibitors and dyslipidemia in transplant recipients: A cause for concern? Transplant Rev. (2015) 29:93–102. doi: 10.1016/j.trre.2014.08.003
237. Pascual J. Concentration-controlled everolimus (Certican): combination with reduced dose calcineurin inhibitors. Transplantation. (2005) 79:S76–79. doi: 10.1097/01.TP.0000162434.62591.F3
Keywords: immunosuppression, cardiovascular, hypertrophy, hypertension, mitochondria, fibrosis, toxicity
Citation: Elezaby A, Dexheimer R and Sallam K (2022) Cardiovascular effects of immunosuppression agents. Front. Cardiovasc. Med. 9:981838. doi: 10.3389/fcvm.2022.981838
Received: 29 June 2022; Accepted: 30 August 2022;
Published: 21 September 2022.
Edited by:
Dong Han, People's Liberation Army General Hospital, ChinaReviewed by:
Josep M. Grinyó, University of Barcelona, SpainLionel PE Rostaing, Université Grenoble Alpes, France
Copyright © 2022 Elezaby, Dexheimer and Sallam. This is an open-access article distributed under the terms of the Creative Commons Attribution License (CC BY). The use, distribution or reproduction in other forums is permitted, provided the original author(s) and the copyright owner(s) are credited and that the original publication in this journal is cited, in accordance with accepted academic practice. No use, distribution or reproduction is permitted which does not comply with these terms.
*Correspondence: Karim Sallam, sallam@stanford.edu