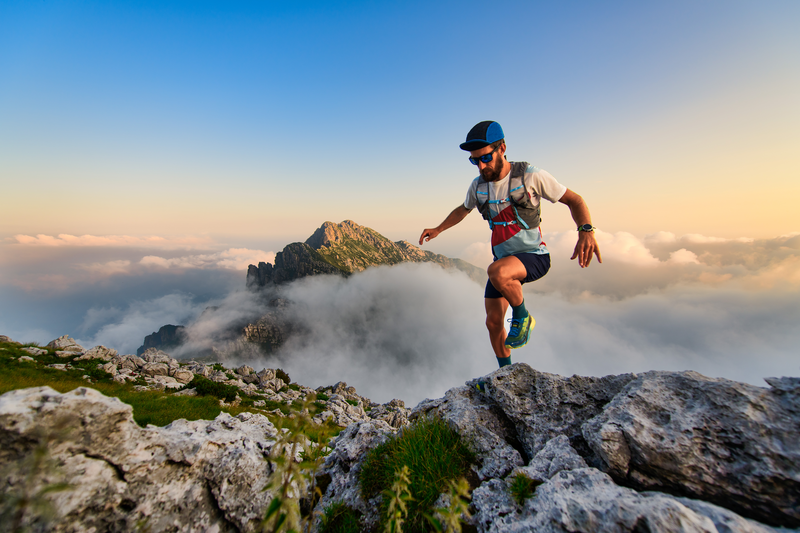
94% of researchers rate our articles as excellent or good
Learn more about the work of our research integrity team to safeguard the quality of each article we publish.
Find out more
ORIGINAL RESEARCH article
Front. Cardiovasc. Med. , 12 December 2022
Sec. Heart Failure and Transplantation
Volume 9 - 2022 | https://doi.org/10.3389/fcvm.2022.976730
Objective: Whether exercise-induced venous-to-systemic shunt (EIS) during cardiopulmonary exercise testing (CPET) has different manifestations or characteristics in idiopathic pulmonary arterial hypertension (IPAH) and chronic thromboembolic pulmonary hypertension (CTEPH) patients remains unknown. We explored the differences in hemodynamics, echocardiography, and prognosis between IPAH and CTEPH patients with and without EIS.
Methods: We conducted a retrospective cross-sectional cohort study and included 161 PH patients at Shanghai Pulmonary Hospital. Demographic, echocardiography, pulmonary hemodynamic, and CPET variables were compared between patients with and without EIS stratified by IPAH and CTEPH. EIS was determined by CPET. Binary logistic regression analyses were performed to explore independent influencing factors of EIS. Cox survival analysis was used to quantify the impact of EIS on the prognosis of patients.
Results: Exercise-induced venous-to-systemic shunt was found in approximately 17.4% of 86 IPAH patients and 20% of 75 CTEPH patients. All-cause mortality occurred in 43 (26.7%) patients during a median follow-up of 6.5 years. Compared with those without EIS, patients with EIS had higher peak end-tidal O2 and lower VO2/VE and tricuspid annular plane systolic excursion (TAPSE). Among the IPAH patients, EIS was associated with lower cardiac output, cardiac index, mixed venous oxygen saturation, VO2/VE, and TAPSE and higher VE/VCO2 and right ventricular end-diastolic transverse diameter. Logistic regression analysis indicated that VO2/VE was an independent factor influencing whether IPAH patients developed EIS during CPET. Cox logistic regression indicated that female IPAH patients or IPAH patients with higher VO2/VE and EIS had a better prognosis. Female IPAH patients had better 10-year survival. In IPAH patients without EIS, patients with higher VO2/VE had better 10-year survival. However, compared with CTEPH patients without EIS, those with EIS had similar echocardiographic, hemodynamic, CPET parameter results and 10-year survival.
Conclusion: Exercise-induced venous-to-systemic shunt exhibits different profiles among IPAH and CTEPH patients. Among IPAH patients, those with EIS had worse peak end-tidal O2, VO2/VE, and TAPSE than those without EIS. VO2/VE was an independent factor of EIS among IPAH patients. IPAH patients with EIS, female sex or higher VO2/VE had better survival. However, the association between EIS and PAH severity or prognosis in CTEPH patients needs to be further explored.
Pulmonary hypertension (PH) is a progressive and fatal disease that is characterized by an increase in pulmonary vascular resistance (PVR) and progressive structural remodeling of pulmonary arteries, ultimately leading to right ventricular failure and death (1, 2). According to clinical classification, it is divided into GROUP 1–5 PH (3). Pulmonary arterial hypertension (PAH) and chronic thromboembolic pulmonary hypertension (CTEPH) are GROUP 1 PH and GROUP 4 PH, respectively. CTEPH is caused by pulmonary vasculature obstruction and has a high mortality (4, 5). In addition, it is well-established that PAH is associated with abnormal cardiopulmonary exercise testing (CPET), such as decreased exercise tolerance, ventilation efficiency, and cardiac function and submaximal exercise tolerance (6–8). Changes in gas exchange patterns caused by exercise-induced right-to-left shunt (EIS) through the patent foramen ovale (PFO), which occurs during CPET, are frequently observed in PAH patients (9). The incidence of EIS in PAH patients is approximately 40% (7, 9). However, the relationship between EIS and prognosis in patients with PH is currently unclear. Some researchers have suggested that chronic PH increases the potential for shunting through the foramen ovale and that such shunting may favor increased survival (9). Nevertheless, others contend that the persistence or development of EIS strongly predicts death or transplantation independent of hemodynamics and all other exercise measures (10).
In the fetal stage, the foramen ovale is a physiological and anatomical structure required to maintain the normal development of the embryo. As the pressure of the left atrium gradually exceeds that of the right atrium, the foramen ovale generally undergoes functional closure before the first year of life (11). The PFO is found in approximately 25% of the adult population and can cause a significant right-to-left shunt when performing things that raise the thoracic pressure, such as Valsalva movements and coughing (11–13). With regard to PAH patients, the increase in exercise load further leads to an increase in PVR because flow-mediated dilatation of the lung circulation cannot increase appropriately, which eventually leads the right atrial pressure (RAP) to exceed the left atrial pressure, and then EIS occurs. The presence of an EIS can cause venous blood with low PaO2, high PaCO2 and H+ to enter the systemic circulation directly through the PFO, which stimulates the systemic circulation arterial chemoreceptors and causes hyperventilation of the lung (14).
The current literature on EIS during CPET has focused on WHO Group 1 PH, especially in idiopathic pulmonary arterial hypertension (IPAH) patients. The presence of EIS has proven to be associated with the severity of exercise limitation and poor hemodynamics and prognosis in patients with IPAH (7, 9, 10). We have previously compared the differences in CPET between IPAH and CTEPH patients. The oxygen uptake efficiency plateau and oxygen uptake efficiency at the anaerobic threshold are higher in IPAH patients than in CTEPH patients and are not in proportion to hemodynamics, probably due to differences in pulmonary vascular occlusion (15). In addition to microarterial lesions similar to those in IPAH patients, CTEPH patients have thrombi in the main trunk, leaves, segments, subsegments and other parts. In addition, whether there are EIS differences between IPAH and CTEPH patients or whether EIS is associated with severity and prognosis in CTEPH patients remains unknown.
Therefore, our purpose was (1) to compare the different profiles of the hemodynamics, echocardiography and CPET in PH patients and subpopulations with or without EIS; (2) to explore the risk factors associated with EIS; and (3) to assess the impact of EIS on the prognosis of IPAH and CTEPH patients.
A total of 200 patients diagnosed with IPAH or CTEPH from 2010 to 2015 were screened from our center database, and 161 patients with available CPET results were finally included (Figure 1). The diagnosis of IPAH and CTEPH was based on the diagnostic criteria of PH (16, 17). The exclusion criteria were (1) patients with other causes of PH, such as chronic lung disease or left heart disease-associated PH (16); (2) patients who received pulmonary endarterectomy (PEA) or balloon pulmonary angioplasty (BPA) treatment; and (3) patients with no right heart catheterization (RHC) or CPET records. RHC was performed within 1 week of each patient’s CPET examination. Demographic information, 6-min walk distance (6MWD), WHO functional class, echocardiographic parameters, PAH risk score (low, intermediate, or high), and hemodynamic features were determined at baseline during hospitalization. This study was conducted in accordance with the amended Declaration of Helsinki. The Institutional Ethics Committee of Shanghai Pulmonary Hospital approved the protocol.
Figure 1. Flow diagram for the cohort. CTEPH, chronic thromboembolic pulmonary hypertension; IPAH, idiopathic pulmonary arterial hypertension; RHC, right heart catheterization; CPET, cardiopulmonary exercise testing.
Right heart catheterization was performed in all patients during hospitalization. RHC was performed as described previously (18, 19). Mean pulmonary arterial pressure (mPAP), right atrial pressure (RAP), pulmonary arterial wedge pressure (PAWP), and cardiac output (CO) were measured. CO was measured in triplicate by the thermodilution technique with iced normal saline. The cardiac index (CI) was calculated by dividing CO by body surface area. Pulmonary vascular resistance (PVR) was calculated by dividing the mPAP minus the PAWP by CO.
All the patients and controls exercised on a cycle ergometer using a breath-by-breath system according to the American Thoracic Society/American College of Chest Physicians statement on CPET (20). Before each test, the equipment was calibrated in accordance with the manufacturer’s specifications using reference and calibration gases. The protocol comprised 3 min of rest, 3 min of unloaded cycling at 55–65 revolutions per minute (rpm), followed by a progressively increasing work rate of 5–15 watts (W)/min for PH patients to the maximum tolerance, and 4 min of recovery (8, 21). Direct measurements of oxygen uptake (O2), carbon dioxide output (CO2), minute ventilation (E), end-tidal CO2 (PETCO2), end-tidal O2 (PETO2) and several derived parameters, such as the heart rate (HR), oxygen pulse (O2/HR), and respiratory exchange ratio (RER), were obtained. Peak O2 was defined as the highest 30-s average of oxygen uptake in the last minute of exercise, and other peak parameters were calculated at the same time. The E-CO2 slope was determined by linear regression analysis of the relationship between E and CO2 during exercise, excluding data above the ventilatory compensation point (22). The lowest E/CO2 was determined by averaging the lowest consecutive 90-s data points (22). O2/E, known as oxygen uptake efficiency (OUE), was computed by linear least squares regression of the oxygen uptake on the logarithm of the minute ventilation according to the following equation: O2 = a × log E + b, where the constant a is the OUE (7, 8, 23). The oxygen uptake efficiency plateau (OUEP) was the highest 90-s stretch of O2 (mL/min)/E (L/min) (7, 8, 23).
EIS was determined by two investigators who were blinded to all the patients’ clinical courses. Nine-panel CPET plots were independently reviewed to identify EIS during exercise using the following criteria as described in detail previously: an abrupt and sustained increase in PETO2, RER, E/O2, and E/CO2 with a simultaneous, sustained decrease in PETCO2 and pulse oximetry (SpO2) (9).
Using the above criteria, two investigators independently graded the 161 PH patients as either EIS positive (+) or EIS negative (−). Patients who were graded consistently as EIS (+) by all three graders were placed in the shunt group, and those who were graded consistently as EIS (−) by all three graders were placed in the no-shunt group.
The process of transthoracic echocardiography was as previously described (24, 25). Right ventricular end-diastolic transverse dimension (RVEDTD), right ventricular end-diastolic longitudinal dimension (RVEDLD), right atrial transverse dimension (RATD), right atrial longitudinal dimension (RALD), end-systolic stage left ventricular eccentricity index (ENDSEI), pulmonary arterial systolic pressure (PASP), and tricuspid annular plane systolic excursion (TAPSE) were measured.
Clinical, demographic, echocardiographic, CPET, and RHC parameters were collected from hospital records. The primary endpoint was all-cause mortality. Mortality information during follow-up was obtained from chart review, outpatient clinic visits or telephone interviews. The follow-up lasted from the date of CPET until the patient died or was censored at the end of the study (31 January 2021).
Data are described as the mean ± standard deviation or median and interquartile and counts (proportions), as appropriate. Spearman’s or Pearson’s correlation coefficient was calculated, as appropriate. Binary logistic regression was applied to find the variables associated with EIS (+) among the IPAH or CTEPH patients separately. Univariate and multivariate Cox proportional hazards regression analyses were applied to quantify the relationships between all-cause mortality and variables of interest. After correction by collinearity analysis, the variables significant in univariate analysis were included in the multivariate Cox regression model. Wald chi-square statistics and 95% confidence intervals (CIs) were used to calculate the significance of the estimates at the level of 0.05. Event-free survival was estimated from the time of CPET, with all-cause mortality as the endpoint. The best separation cutoff values of the parameters, as judged from the log-rank χ2 statistics, were identified by the survminer package of R software. Kaplan–Meier survival curves and log-rank tests were used to assess EIS and other variables for survival outcomes. Statistical analysis was performed using R software (version 4.1.3).1 In all univariate analyses, P < 0.05 was considered statistically significant.
None of the 200 patients ultimately enrolled in this study had discordant evaluations. The medical records of 161 patients with IPAH (n = 86) or CTEPH (n = 75) who systematically underwent RHC and CPET for clinical evaluation at Shanghai Pulmonary Hospital between January 2010 and December 2015 were retrospectively studied (Figure 1). Among them, 15 IPAH and 15 CTEPH patients had EIS. The PFO was found in approximately 14.7 and 20% of IPAH and CTEPH patients, respectively. A comparison between demographic, hemodynamic, CPET, and echocardiography characteristics was made between EIS (+) and EIS (−) patients. Compared with EIS (−) PH patients, EIS (+) PH patients had worse peak PETO2, VO2/VE, and TAPSE despite having comparable pulmonary hemodynamics (Table 1). These results indicate that EIS (+) PH patients had impaired ventilation efficiency and submaximal exercise tolerance and right ventricular function (Table 1). All echocardiographs were performed at rest, and the PFO was found by echocardiography at rest. Table 2 shows the detailed comparisons of hemodynamics, CPET and echocardiography, stratified by PH category, namely, IPAH and CTEPH.
Table 1. Demographic, hemodynamic, cardiopulmonary exercise testing, and echocardiographic data in patients with or without EIS.
Table 2. Comparisons of demographic, hemodynamic, cardiopulmonary exercise testing, and echocardiographic variables in IPAH or CTEPH patients with vs. without EIS.
With regard to hemodynamics, CO, CI, and SvO2 decreased among EIS (+) IPAH patients, while PVR increased significantly. No differences were found in CO, CI, SvO2, or PVR between the CTEPH patients, irrespective of the presence of EIS (Table 2 and Figure 2). Table 2 and Figure 3 depict the differences in exercise tolerance, ventilation efficiency, right heart function and submaximal exercise tolerance among EIS (+) or EIS (−) IPAH and CTPEH patients. Among IPAH patients, neither peak VO2 nor peak VO2/HR, which represented exercise tolerance and heart function, respectively, distinguished EIS (+) from EIS (−) patients. In terms of ventilator efficiency, VE/VCO2 was higher in EIS (+) IPAH patients than in EIS (−) IPAH patients, suggesting more impaired ventilation efficiency (65.8 ± 29.1 L/min/L/min vs. 49.3 ± 15.1 L/min/L/min, P < 0.05). However, other parameters reflecting ventilation efficiency, such as peak PETO2, peak PETCO2, and lowest VE/VCO2, did not exhibit differences. With regard to submaximal exercise tolerance, VO2/VE was lower in EIS (+) IPAH patients than in EIS (−) IPAH patients (15.9 ± 5.3 ml/L vs. 20.8 ± 5.3 ml/L, P < 0.05), while OUEP was not different. Among the echocardiography parameters, TAPSE decreased, while RVEDTD increased significantly among both EIS (+) and EIS (−) IPAH patients (4.6 ± 0.8 cm vs. 4.0 ± 0.8 cm, P < 0.05). Neither echocardiography nor CPET overall mean parameters could distinguish CTEPH patients with and without EIS.
Figure 2. Comparison of hemodynamic parameters in IPAH and CTEPH patients stratified by EIS (+). *P < 0.05. IPAH, idiopathic pulmonary arterial hypertension; CTEPH, chronic thromboembolic pulmonary hypertension; EIS, exercise-induced right-to-left shunt; CO, cardiac output; CI, cardiac index; PVR, pulmonary vessel resistance; SvO2, mixed venous oxygen saturation. (A,C,E,F), IPAH; (B,D,G,H), CTEPH.
Figure 3. Comparison of echocardiographic parameters in IPAH (A,C,E,F) and CTEPH (B,D,G,H) patients stratified by EIS (+). **P < 0.01, ***P < 0.001. IPAH, idiopathic pulmonary arterial hypertension; CTEPH, chronic thromboembolic pulmonary hypertension; EIS, exercise-induced right-to-left shunt; RVEDTD, right ventricular end-diastolic transverse diameter; TAPSE, tricuspid annular plane systolic excursion; VCO2, carbon dioxide output; VE, minute ventilation; VO2, oxygen uptake.
First, stratified by PH category, the risk, CI, CO, peak PETO2, peak VO2/HR, VO2/VE, VE/VCO2, and RVEDTD achieved statistical significance in binary univariate logistical analysis. Then, excluding the influence of collinearity between variables, binary multivariate logistical analyses with the stepwise method were performed, which revealed that VO2/VE was significantly associated with EIS (+) in IPAH patients (odds ratio: 0.833, 95% CI: 0.736–0.943, P < 0.004) (Table 3). No significant variable was found to be associated with EIS (+) CTEPH patients.
All-cause mortality occurred in 43 (26.7%) patients during a median follow-up of 6.5 (4.7, 7.4) years. The follow-up rate was 100%. In 86 IPAH patients, all-cause mortality occurred in 22 patients. Based on high correlation coefficients (Figure 4), we excluded those parameters with strong collinearity. In addition, considering that the low, intermediate and high simplified PH risk stratifications were grouped by NT-proBNP, WHO FC, RAP, CI, SvO2, and 6MWD, we retained the simplified risk stratification and excluded the abovementioned dimensional parameters. Moreover, based on clinical significance and significant differences, EIS, risk, sex, VO2/VE, VE/VCO2, TAPSE, RVEDTD, and ENDSEI were selected for multivariate Cox regression (Figure 5). The predictors of all-cause mortality in the IPAH cohort were EIS, sex, VO2/VE, and RVEDTD with a cutoff of 20.99 ml/L. In CTEPH patients, none of the above parameters were independent influencing factors of EIS (Supplementary Figure 1).
Figure 4. Correlation analysis of demographics, CPET, hemodynamics and echocardiography in patients with IPAH. CO, cardiac output; CI, cardiac index; PVR, pulmonary vessel resistance; SvO2, mixed venous oxygen saturation; RAP, right atrial pressure; Peak PETCO2, peak end–tidal partial pressure of CO2, Peak PETO2, peak end–tidal partial pressure of O2; VCO2, carbon dioxide output; VE, minute ventilation; VO2, oxygen uptake; RVEDTD, right ventricular end-diastolic transverse diameter; TAPSE, tricuspid annular plane systolic excursion; OUEP, oxygen uptake efficiency plateau.
Figure 5. Cox univariate regression analyses for all-cause mortality among IPAH patients. RVEDTD, right ventricular end-diastolic transverse dimension; TAPSE, tricuspid annular plane systolic excursion; EIS, exercise-induced right-to-left shunt; VCO2, carbon dioxide output; VE, minute ventilation; VO2, oxygen uptake; IPAH, idiopathic pulmonary arterial hypertension.
Kaplan–Meier survival curves and log-rank tests were used to identify scores that would predict survival outcomes. Figure 6A shows no differences in 10-year survival between EIS (+) and EIS (−) IPAH patients; however, EIS (+) IPAH patients had better 6-year survival than EIS (−) patients (Figure 6B). Figures 6C,D show the Kaplan–Meier survival estimates for EIS with sex and VO2/VE, respectively. Female EIS (+) IPAH patients had a better prognosis than male EIS (−) IPAH patients; IPAH patients with VO2/VE ≥ 20.99 ml + EIS (+) had a better prognosis than those with VO2/VE < 20.99 ml and EIS (−).
Figure 6. Kaplan–Meier analysis for all-cause mortality in IPAH patients, separated by (A) 10-year survival; (B) 6-year survival; (C) sex and EIS; and (D) VO2/VE and EIS. EIS, exercise-induced right-to-left shunt; VE, minute ventilation; VO2, oxygen uptake; IPAH, idiopathic pulmonary arterial hypertension.
For EIS (−) IPAH patients, the prognosis of females was significantly better than that of males (Figure 7A); the prognosis of patients with VO2/VE ≥ 20.99 ml/L was much better than that of those with VO2/VE < 20.99 ml/L (Figure 7B). For EIS (+) IPAH patients, there were no significant differences regardless of sex or VO2/VE (Figures 7C,D).
Figure 7. Kaplan–Meier analysis for all-cause mortality in IPAH patients, separated by (A) sex in the EIS (–) subgroup; (B) VO2/VE (<20.99 ml/L vs. ≥20.99 ml/L) in the EIS (–) subgroup; (C) sex in the EIS (+) subgroup; (D) VO2/VE (<20.99 ml/L vs. ≥20.99 ml/L) in the EIS (+) subgroup. EIS, exercise-induced right-to-left shunt; VE, minute ventilation; VO2, oxygen uptake; IPAH, idiopathic pulmonary arterial hypertension.
However, for CTEPH patients, there were no significant prognoses regardless of 10-year survival, 1-year survival, 5-year survival, and 6-year survival (Supplementary Figures 2A–D, respectively).
Our study found that the relationship between EIS and disease severity or prognosis was significantly different in IPAH and CTEPH patients. EIS (+) IPAH patients had worse peak end-tidal O2, VO2/VE and TAPSE than EIS (−) IPAH patients. OUE, namely VO2/VE, was an independent factor of EIS among IPAH patients. IPAH patients with EIS, higher VO2/VE and female sex had better survival. Both EIS (+) and EIS (−) CTEPH patients had similar PAH severity and prognosis.
In our study, the incidence of EIS in patients with PH was 18.6%, while the incidence of EIS in the IPAH and CTEPH groups was 17.4 and 20.0%, respectively, which were lower than the rates previously reported (7, 9). There may be some reasons. We did not include discordant EIS patients in our study. In addition, in our study, some patients had undergone targeted-PAH treatment, which reduced their PVR compared with the baseline level, thus reducing the likelihood of EIS during CPET. The incidence of EIS in IPAH and CTEPH patients was similar, but the correlation with disease severity and prognosis varied. We infer that the different pathophysiological mechanisms of CTEPH and IPAH might be one of the causes. In addition to microarterial lesions similar to those in IPAH patients, CTEPH patients have thrombi in the main trunk, leaves, segments, subsegments and other parts.
In our study, peak PETO2 significantly increased in patients with EIS (+) PH, while VO2/VE and TAPSE significantly decreased in EIS (+) PH patients. When EIS occurs, oxygen-deficient blood with high concentrations of CO2 and H+ is shunted into the systemic circulation, which stimulates chemoreceptors in the arteries to maintain the arterial homeostasis of H+ and PaCO2 (26). During this process, alveolar ventilation increases acutely, resulting in a rapid increase in PaO2 and a rapid decrease in PaCO2 in the alveoli, which is reflected in the increase in peak PETO2 and the decrease in peak PETCO2 (9). Unfortunately, we did not find a significant difference in peak PETCO2 between EIS (+) and EIS (−) patients. In addition, VO2/VE represents the OUE of the pulmonary artery to a certain extent (27). Therefore, the decrease in VO2/VE in EIS patients indicates that such patients have lower OUE. OUE has been widely used to evaluate the severity and prognosis of cardiopulmonary diseases such as chronic heart failure, coronary heart disease and PH (28–30), and also be used to evaluate submaximal exercise endurance in patients with PH (31). EIS (+) patients have lower OUE, which we speculate may be explained as follows. First, patients with shunts have poorer hemodynamics, and experience anaerobic exercise earlier (7), which can lead to lactate buildup. The acid and hypoxia in the shunt blood can significantly stimulate ventilation to maintain homeostasis in the arteries, which can cause patients to experience unbearable dyspnea and to eventually be forced to stop exercising, resulting in a decrease in oxygen uptake. Second, a combination of acute alveolar hyperventilation and reduced right ventricular blood flow into the pulmonary vasculature in EIS (+) patients can lead to severe V/Q dysregulation that ultimately hinders the patient’s gas exchange. Furthermore, TAPSE reflects the longitudinal systolic function of the right ventricular myocardium (32). Thus, a lower TAPSE may predict reduced right ventricular function in EIS (+) patients.
We found the differences in clinical data between EIS (+) and EIS (−) IPAH and CTEPH patients and the influencing factors of EIS according to different PH classifications. We found that there was no significant difference in hemodynamics, CPET or echocardiography for the occurrence of EIS in CTEPH patients, but the situation was significantly different in IPAH patients. In terms of hemodynamics, EIS (+) IPAH patients had lower CO, CI, and SvO2 and higher PVR levels than EIS (−) IPAH patients, suggesting that EIS (+) patients may have more severe PH, which would be in line with the results of Guo et al. (7). In addition, EIS (+) IPAH patients had lower TAPSE and higher RVTDED than EIS (−) patients on echocardiography, which further indicated that such patients had worse right heart function. The higher VE/VCO2 indicated that the EIS (+) IPAH patients had a more impaired ventilation efficiency, with a sharp increase in lung ventilation during CPET. The VE/VCO2 ratio is the result of both the dead space to tidal volume ratio (VD/VT) and the PaCO2 set point. When EIS occurs, diverting deoxygenated, acidemic, and CO2-rich blood stimulate arterial chemoreceptors, causing an immediate increase in ventilation, as manifested by rapid increases in alveolar PaO2 and decreases in PaCO2.
The remodeling of the pulmonary vasculature in IPAH patients and the occlusion of small pulmonary vessels in CTEPH patients both lead to an increase in PVR. Moreover, EIS (+) patients have reduced blood flow from the right ventricle to the pulmonary vasculature, which further results in alveolar hypoperfusion and an increase in VE/VCO2 (28, 33). Notably, we found that VO2/VE in EIS (+) IPAH patients was significantly lower than that in EIS (−) IPAH patients, and VO2/VE was an independent factor influencing whether IPAH patients developed EIS. This suggests that OUE may indicate a more serious disease in IPAH patients and that paying attention to patients’ OUE may be helpful for the timely detection of such IPAH patients.
In the follow-up, all-cause mortality stratified by EIS was assessed in the IPAH group. We found that male sex and lower OUE predicted worse survival in IPAH patients, especially in those with EIS (+). In EIS (−) IPAH patients, although male sex and lower OUE represented a trend of lower survival, they were not statistically significant, which we speculate might be related to the sample size. Interestingly, despite more severe hemodynamic characteristics (lower CO, CI, and SvO2), patients with EIS and IPAH had better survival at 6 years. This might be because the major cause of death in patients with PAH and CTEPH was right heart failure, and the possibility of a right-to-left shunt allowed the unloading of the right side of the heart. Consistent with our study, Ronald et al. (10) noted that reduced aerobic capacity and ventilation efficiency were associated with increased mortality in PAH patients. In addition, Tang et al. (34) confirmed that OUE has good prognostic value in IPAH patients. The reasons may be as follows: on the one hand, hyperventilation during the occurrence of EIS causes OUE (namely, VO2/VE) to decline; on the other hand, OUE is related to the patient’s cardiac function. Poor cardiac function affects the pumping ability of the heart, namely, cardiac output, leading to a decrease in OUE (35). In addition, VO2 is dependent both on the cardiac output and the delivery of oxygen (QxCaO2) as well as oxygen extraction in the tissue (CaO2–CvO2), which is of importance since in EIS, there is oxygen desaturation and impaired oxygen delivery (QxCaO2). Therefore, OUE can better reflect the physiological changes in the disease and the severity of the harm caused by the disease. We also found that male EIS (+) IPAH patients had lower survival rates, which suggests that sex differences in changes in venous-to-systemic circulation shunts may contribute to further analysis of independent clinical outcomes.
Interestingly, by stratifying IPAH or CTEPH by EIS and comparing the relevant clinical data and outcomes, we found that EIS seems to have a greater impact on IPAH patients, which has not been previously studied. The reasons for this finding may be as follows: First, this may be related to the low incidence of EIS in this study, which resulted in some comparisons having clinically relevant trends but no significant differences. Second, the two classifications of disease are treated differently, and the clinical orientation of targeted drugs for IPAH and CTEPH may be inconsistent, which results in different pulmonary vascular responses to exercise-induced elevated PVR in the two PH classifications. Third, it could be associated with the pathophysiological manifestations of the two types of PH. The pathological changes in IPAH mainly involve the distal pulmonary arterioles, while CTEPH involves both the pulmonary arterioles and the larger pulmonary arteries, which may lead to the different reactions to EIS.
There are several limitations to our study. First, it only included patients with IPAH and CTEPH, while ignoring other classifications of PH, which added some bias to the study. Future studies should include various types of PH. Second, we did not compare changes in CPET parameters from rest to the end of unloading, as previous studies have done, which may also be related to PH severity. Finally, this was a small, single-center, retrospective study. A large, multicenter, prospective study may be needed to better evaluate the condition and prognosis of PH through relevant indicators.
EIS exhibits different profiles among IPAH and CTEPH patients. The association between EIS and disease severity or prognosis is quite different in IPAH and CTEPH patients, which may be related to the pathophysiological characteristics of the two diseases and requires special attention in clinical practice. IPAH patients with EIS have worse peak end-tidal O2, VO2/VE and TAPSE than those without EIS. VO2/VE is an independent factor of EIS among IPAH patients. IPAH patients with EIS, higher VO2/VE and female sex have better survival, which indicates that there is a greater association between EIS and OUE, but no such association is found in CTEPH patients. However, further large-sample randomized controlled trials are needed to confirm and explore the specific mechanisms involved. In addition, this study may provide new ideas for further research on the pathophysiological mechanisms of the two types of PH.
The raw data supporting the conclusions of this article will be made available by the authors, without undue reservation.
The studies involving human participants were reviewed and approved by the Institutional Ethics Committee of Shanghai Pulmonary Hospital Local Committees. The patients/participants provided their written informed consent to participate in this study.
RJ, JG, and J-ML contributed to the conception and design of the study. PY, X-XS, and Z-YG organized the database. H-LQ, C-JL, and Q-HZ performed the statistical analysis. RJ and SW wrote the first draft of the manuscript. S-GG, LW, JH, RZ, H-TL, W-HW, and LW wrote sections of the manuscript. All authors contributed to manuscript revision, read, and approved the submitted version.
This work was funded by the Program of the National Natural Science Foundation of China (81700045 and 81870042), the Three-year Action Plan to Promote Clinical Skills and Clinical Innovation in Municipal Hospitals (SHDC2020CR4021), and the Program of Shanghai Pulmonary Hospital (FKLY20011). This work was also supported by the Department Support Fund of Shanghai Pulmonary Hospital.
The authors declare that the research was conducted in the absence of any commercial or financial relationships that could be construed as a potential conflict of interest.
All claims expressed in this article are solely those of the authors and do not necessarily represent those of their affiliated organizations, or those of the publisher, the editors and the reviewers. Any product that may be evaluated in this article, or claim that may be made by its manufacturer, is not guaranteed or endorsed by the publisher.
The Supplementary Material for this article can be found online at: https://www.frontiersin.org/articles/10.3389/fcvm.2022.976730/full#supplementary-material
Supplementary Figure 1 | Cox univariate regression analyses for all-cause mortality among CTEPH patients. RVEDTD, right ventricular end-diastolic transverse dimension; TAPSE, tricuspid annular plane systolic excursion; EIS, exercise-induced right-to-left shunt; VCO2, carbon dioxide output; VE, minute ventilation; VO2, oxygen uptake.
Supplementary Figure 2 | Kaplan–Meier analysis for all-cause mortality in CTEPH patients, separated by (A) 10-year survival, (B) 1-year survival, (C) 5-year survival, and (D) 6-year survival. CTEPH, chronic thromboembolic pulmonary hypertension.
1. Al-Omary M, Sugito S, Boyle A, Sverdlov A, Collins N. Pulmonary hypertension due to left heart disease: diagnosis, pathophysiology, and therapy. Hypertension. (2020) 75:1397–408. doi: 10.1161/HYPERTENSIONAHA.119.14330
2. Medvedev R, Sanchez-Alonso J, Alvarez-Laviada A, Rossi S, Dries E, Schorn T, et al. Nanoscale study of calcium handling remodeling in right ventricular cardiomyocytes following pulmonary hypertension. Hypertension. (2021) 77:605–16. doi: 10.1161/HYPERTENSIONAHA.120.14858
3. Humbert M, Kovacs G, Hoeper M, Badagliacca R, Berger R, Brida M, et al. 2022 ESC/ERS Guidelines for the diagnosis and treatment of pulmonary hypertension. Eur Heart J. (2022) 43:3618–731. doi: 10.1183/13993003.00879-2022
4. Ruaro B, Confalonieri P, Caforio G, Baratella E, Pozzan R, Tavano S, et al. Chronic thromboembolic pulmonary hypertension: an observational study. Medicina. (2022) 58:1094. doi: 10.3390/medicina58081094
5. Sharma M, Levine D. Revisiting a distinct entity in pulmonary vascular disease: chronic thromboembolic pulmonary hypertension (CTEPH). Medicina. (2021) 57:355. doi: 10.3390/medicina57040355
6. Riley M, Porszasz J, Engelen M, Shapiro S, Brundage B, Wasserman K. Responses to constant work rate bicycle ergometry exercise in primary pulmonary hypertension: the effect of inhaled nitric oxide. J Am Coll Cardiol. (2000) 36:547–56. doi: 10.1016/S0735-1097(00)00727-0
7. Guo J, Shi X, Yang W, Gong S, Zhao Q, Wang L, et al. Exercise physiology and pulmonary hemodynamic abnormality in PH patients with exercise induced venous-to-systemic shunt. PLoS One. (2014) 10:e0121690. doi: 10.1371/journal.pone.0121690
8. Jiang R, Liu H, Pudasaini B, Zhang R, Xu J, Wang L, et al. Characteristics of cardiopulmonary exercise testing of patients with borderline mean pulmonary artery pressure. Clin Respir J. (2019) 13:148–58. doi: 10.1111/crj.12996
9. Sun X, Hansen J, Oudiz R, Wasserman K. Gas exchange detection of exercise-induced right-to-left shunt in patients with primary pulmonary hypertension. Circulation. (2002) 105:54–60. doi: 10.1161/hc0102.101509
10. Oudiz R, Midde R, Hovenesyan A, Sun X, Roveran G, Hansen J, et al. Usefulness of right-to-left shunting and poor exercise gas exchange for predicting prognosis in patients with pulmonary arterial hypertension. Am J Cardiol. (2010) 105:1186–91. doi: 10.1016/j.amjcard.2009.12.024
11. Saver J. Cryptogenic stroke in patients with patent foramen ovale. Curr Atheroscler Rep. (2007) 9:319–25. doi: 10.1007/s11883-007-0039-y
12. Sharma A, Gheewala N, Silver P. Role of patent foramen ovale in migraine etiology and treatment: a review. Echocardiography. (2011) 28:913–7. doi: 10.1111/j.1540-8175.2011.01460.x
13. Homma S, Messé S, Rundek T, Sun Y, Franke J, Davidson K, et al. Patent foramen ovale. Nat Rev Dis Primers. (2016) 2:15086. doi: 10.1038/nrdp.2015.86
14. Mojadidi M, Zaman M, Elgendy I, Mahmoud A, Patel N, Agarwal N, et al. Cryptogenic stroke and patent foramen ovale. J Am Coll Cardiol. (2018) 71:1035–43. doi: 10.1016/j.jacc.2017.12.059
15. Sun X, Shi X, Cao Y, Zhu H, Pudasaini B, Yang W, et al. Variation of PetCO2 during incremental exercise and severity of IPAH and CTEPH. BMC Pulm Med. (2022) 22:249. doi: 10.1186/s12890-022-02045-4
16. Simonneau G, Gatzoulis M, Adatia I, Celermajer D, Denton C, Ghofrani A, et al. Updated clinical classification of pulmonary hypertension. J Am Coll Cardiol. (2013) 62:D34–41. doi: 10.1016/j.jacc.2013.10.029
17. Wilkens H, Konstantinides S, Lang I, Bunck A, Gerges M, Gerhardt F, et al. Chronic thromboembolic pulmonary hypertension (CTEPH): updated recommendations from the cologne consensus conference 2018. Int J Cardiol. (2018) 272S:69–78. doi: 10.1016/j.ijcard.2018.08.079
18. Jiang R, Ai Z, Jiang X, Yuan P, Liu D, Zhao Q, et al. Intravenous fasudil improves in-hospital mortality of patients with right heart failure in severe pulmonary hypertension. Hypertens Res. (2015) 38:539–44. doi: 10.1038/hr.2015.33
19. Jiang X, Wang Y, Zhao Q, Jiang R, Wu Y, Peng F, et al. Acute hemodynamic response of infused fasudil in patients with pulmonary arterial hypertension: a randomized, controlled, crossover study. Int J Cardiol. (2014) 177:61–5. doi: 10.1016/j.ijcard.2014.09.101
20. American Thoracic Society, American College of Chest Physicians. ATS/ACCP Statement on cardiopulmonary exercise testing. Am J Respir Crit Care Med. (2003) 167:211–77. doi: 10.1164/rccm.167.2.211
21. Sue D, Wasserman K. Impact of integrative cardiopulmonary exercise testing on clinical decision making. Chest. (1991) 99:981–92. doi: 10.1378/chest.99.4.981
22. Sun X, Hansen J, Garatachea N, Storer T, Wasserman K. Ventilatory efficiency during exercise in healthy subjects. Am J Respir Crit Care Med. (2002) 166:1443–8. doi: 10.1164/rccm.2202033
23. Bentley D, McNaughton L. Comparison of W(peak), VO2(peak) and the ventilation threshold from two different incremental exercise tests: relationship to endurance performance. J Sci Med Sport. (2003) 6:422–35. doi: 10.1016/S1440-2440(03)80268-2
24. Rudski L, Lai W, Afilalo J, Hua L, Handschumacher M, Chandrasekaran K, et al. Guidelines for the echocardiographic assessment of the right heart in adults: a report from the American society of echocardiography endorsed by the European association of echocardiography, a registered branch of the European society of cardiology, and the Canadian society of echocardiography. J Am Soc Echocardiogr. (2010) 23:685–713. doi: 10.1016/j.echo.2010.05.010
25. Jiang R, Wu C, Pudasaini B, Wang L, Zhao Q, Zhang R, et al. A novel scoring index by doppler echocardiography for predicting severe pulmonary hypertension due to chronic lung diseases: a cross-sectional diagnostic accuracy study. Int J Chron Obstruct Pulmon Dis. (2017) 12:1741–51. doi: 10.2147/COPD.S133854
26. Elliott J, Laurie S, Kern J, Beasley K, Goodman R, Kayser B, et al. AltitudeOmics: impaired pulmonary gas exchange efficiency and blunted ventilatory acclimatization in humans with patent foramen ovale after 16 days at 5,260 m. J Appl Physiol. (2015) 118:1100–12. doi: 10.1152/japplphysiol.00879.2014
27. Hossri C, Souza I, de Oliveira J, Mastrocola L. Assessment of oxygen-uptake efficiency slope in healthy children and children with heart disease: generation of appropriate reference values for the OUES variable. Eur J Prev Cardiol. (2019) 26:177–84. doi: 10.1177/2047487318807977
28. Valentim Goncalves A, Pereira-da-Silva T, Soares R, Feliciano J, Abreu A, Rio P, et al. Prognostic value of VE/VCO2 slope in overweight heart failure patients. Am J Cardiovasc Dis. (2020) 10:578–84.
29. Wang Y, Yao S, Liu D, Shen T, Zhao W, Gao W, et al. [Relationships between percentage of skeletal muscle mass and cardiorespiratory fitness in elderly patients with coronary heart disease]. Zhonghua Yi Xue Za Zhi. (2018) 98:831–6.
30. Antoine-Jonville S, Pichon A, Vazir A, Polkey M, Dayer M. Oxygen uptake efficiency slope, aerobic fitness, and V(E)-VCO2 slope in heart failure. Med Sci Sports Exerc. (2012) 44:428–34. doi: 10.1249/MSS.0b013e31822f8427
31. Shi X, Guo J, Gong S, Sapkota R, Yang W, Liu H, et al. Oxygen uptake is more efficient in idiopathic pulmonary arterial hypertension than in chronic thromboembolic pulmonary hypertension. Respirology. (2016) 21:149–56. doi: 10.1111/resp.12648
32. Koestenberger M, Avian A, Cantinotti M, Hansmann G. Tricuspid annular plane systolic excursion (TAPSE) in pediatric pulmonary hypertension: integrating right ventricular ejection efficiency (RVEe) into advanced multi-parametric imaging. Int J Cardiol. (2019) 274:296–8. doi: 10.1016/j.ijcard.2018.07.013
33. Klaassen S, Liu L, Hummel Y, Damman K, van der Meer P, Voors A, et al. Clinical and hemodynamic correlates and prognostic value of VE/VCO2 slope in patients with heart failure with preserved ejection fraction and pulmonary hypertension. J Card Fail. (2017) 23:777–82. doi: 10.1016/j.cardfail.2017.07.397
34. Tang Y, Luo Q, Liu Z, Ma X, Zhao Z, Huang Z, et al. Oxygen uptake efficiency slope predicts poor outcome in patients with idiopathic pulmonary arterial hypertension. J Am Heart Assoc. (2017) 6:e005037. doi: 10.1161/JAHA.116.005037
Keywords: pulmonary hypertension, cardiopulmonary exercise test, venous-to-systemic shunt, right heart catheterization, idiopathic pulmonary arterial hypertension, chronic thromboembolic pulmonary hypertension
Citation: Wang S, Guo Z-Y, Sun X-X, Yuan P, Zhao Q-H, Wu W-H, Qiu H-L, Luo C-J, Gong S-G, Li H-T, Zhang R, He J, Wang L, Liu J-M, Guo J and Jiang R (2022) Differences in disease severity and prognosis of exercise-induced right-to-left shunt between idiopathic pulmonary arterial hypertension and chronic thromboembolic pulmonary hypertension patients. Front. Cardiovasc. Med. 9:976730. doi: 10.3389/fcvm.2022.976730
Received: 23 June 2022; Accepted: 21 November 2022;
Published: 12 December 2022.
Edited by:
Zhihong Liu, Chinese Academy of Medical Sciences and Peking Union Medical College, ChinaCopyright © 2022 Wang, Guo, Sun, Yuan, Zhao, Wu, Qiu, Luo, Gong, Li, Zhang, He, Wang, Liu, Guo and Jiang. This is an open-access article distributed under the terms of the Creative Commons Attribution License (CC BY). The use, distribution or reproduction in other forums is permitted, provided the original author(s) and the copyright owner(s) are credited and that the original publication in this journal is cited, in accordance with accepted academic practice. No use, distribution or reproduction is permitted which does not comply with these terms.
*Correspondence: Rong Jiang, bGlzdGVuaW5nMzlAMTYzLmNvbQ==; Jian Guo, amlhbmppYW4xMTEyMTk3OEAxNjMuY29t
†These authors have contributed equally to this work and share first authorship
Disclaimer: All claims expressed in this article are solely those of the authors and do not necessarily represent those of their affiliated organizations, or those of the publisher, the editors and the reviewers. Any product that may be evaluated in this article or claim that may be made by its manufacturer is not guaranteed or endorsed by the publisher.
Research integrity at Frontiers
Learn more about the work of our research integrity team to safeguard the quality of each article we publish.