- 1Department of Radiation Oncology, Nanfang Hospital, Southern Medical University, Guangzhou, China
- 2Department of Cardiology, Union Hospital, Tongji Medical College, Huazhong University of Science and Technology, Wuhan, China
Background: Increasing incidences of both atrial fibrillation (AF) and cancer have been observed in recent years. However, the casual association of both serious conditions has been scarcely evaluated and is considered to be a blank slate in cardio-oncology. Thus, we introduced Mendelian randomization (MR) methods to estimate the effects of AF on cancer risks.
Methods: We performed univariable and multivariable two-sample MR analyses to evaluate the effects of AF on the risk of 19 site-specific types of cancer. This MR study was conducted based on 111 independent AF-associated genetic instruments from genome-wide association studies and summarized-level data from corresponding cancer consortia. Multiple sensitivity analyses, including the leave-one-out analysis, MR-Egger regression, and MR-PRESSO tests, were further performed to examine the potential directional pleiotropic effects. Functional annotation was performed for common differentially expressed genes of AF and prostate cancer (PCA).
Results: A total of 6,777,155 European-descent people, including 533,725 cases and 6,243,430 controls, were included in the present MR analysis. Univariable MR analyses demonstrated a causal effect of AF on the incidence of PCA [odds ratio (OR): 0.96; 95% confidence interval (CI) 0.92–0.99, p = 0.01], and the causal effect remained significant (OR: 0.65; 95% CI 0.47–0.90, p = 0.01) after adjusting for potential confounders through the multivariable MR approach. However, no casual associations between AF and the other 18 site-specific cancer risks were observed (all p-values were > 0.05). The consistency of outcomes across complementary sensitivity MR methods further supported the causality. The functional analysis emphasized the essential role of antioxidant and xenobiotic catabolic processes in AF and PCA.
Conclusion: Contrary to the findings of several previous observational studies, our comprehensive MR analyses did not corroborate a causal role for AF in increasing the risk of various types of cancer. They did, however, demonstrate that AF may decrease the risk of PCA. Studies from larger sample sizes and individuals with different ethnic backgrounds are required to further support our conclusions.
1. Introduction
Atrial fibrillation (AF) is the most common cardiac arrhythmia (1) that imposes a substantial risk of death from many cardiovascular diseases and huge societal healthcare burdens. It is presumed that 6–12 million people will develop AF in the USA by 2050 and 17.9 million citizens in Europe by 2060 (2). Interestingly, a recent prospective cohort study has reported that cancer is the leading causes of death in high-income countries, accounting for two times as many deaths as cardiovascular diseases (3). Moreover, abundant evidence based on large populations shows that patients with cancer are related to an increased incidence of AF (4). However, the effect of AF on cancer risks is an uncharted field in cardio-oncology (5). Given the unacceptably high prevalence and treatment costs of both serious conditions, cardio-oncology should not be only concerned with the cardiac side effects of antineoplastic drugs in this case (6). Thus, a specific study is urgently required to find the potential associations between AF and cancer risks, which may provide new insights into the possible mechanisms and therapeutic targets.
Several observational studies have described the ambiguous effects of AF on types of cancer (7–12) due to shared risk factors and predisposing biological processes (13, 14). Prior evidence shows that patients with new-onset AF would have a noticeably increased risk of a malignant diagnosis (7), which was consistent with those of population-based cohort studies that showed that AF was related to a higher malignant incidence (8, 9, 11). However, several intrinsic methodological limitations in prior study designs may impact the observed results, thus resulting in contradictory conclusions. It is difficult, for example, for observational studies to rule out several important lifestyle differences or residual confounders (e.g., smoking, alcohol consumption, diabetes, or hypertension) in both AF and types of cancer (14). Moreover, observational results suggest that an observed association might be attributable to instances associated with a cancer diagnosis and detection bias instead of a causal relationship (9). Consequently, there is an urgent need for a reliable study design that will assess the exact causal association between AF and cancer risks.
Mendelian randomization (MR) analysis has recently become a promising and novel epidemiological approach to assess the causal relationship between exposures and outcomes, using genetic variants as instrumental variables (IVs). Adopting genetic variants as the IVs in the MR analysis can make it less susceptible to reverse causality and hypothetical confounders. A two-sample MR analysis can be performed with robust statistical power using summary-level data from large genome-wide association studies (GWAS) (15). Hence, to solve the aforementioned issue regarding AF and types of cancer, we aimed to evaluate the causal relationship between AF and the risks of 19 site-specific types of cancer with univariable and multivariable two-sample MR methods. Given the tight association between AF and heart failure (HF) (16), we also evaluated the causality between HF and cancer risks.
2. Method
2.1. Mendelian randomization assumptions
To enable a valuable interpretation, all analyses in our MR study were based on the following three core hypotheses or study designs (17): (i) the IVs were convincingly correlated with exposures, (ii) the IVs influenced tumors only through their effects on exposures, and (iii) the IVs were independent of any confounders from the AF/HF cancer association (Figure 1A).
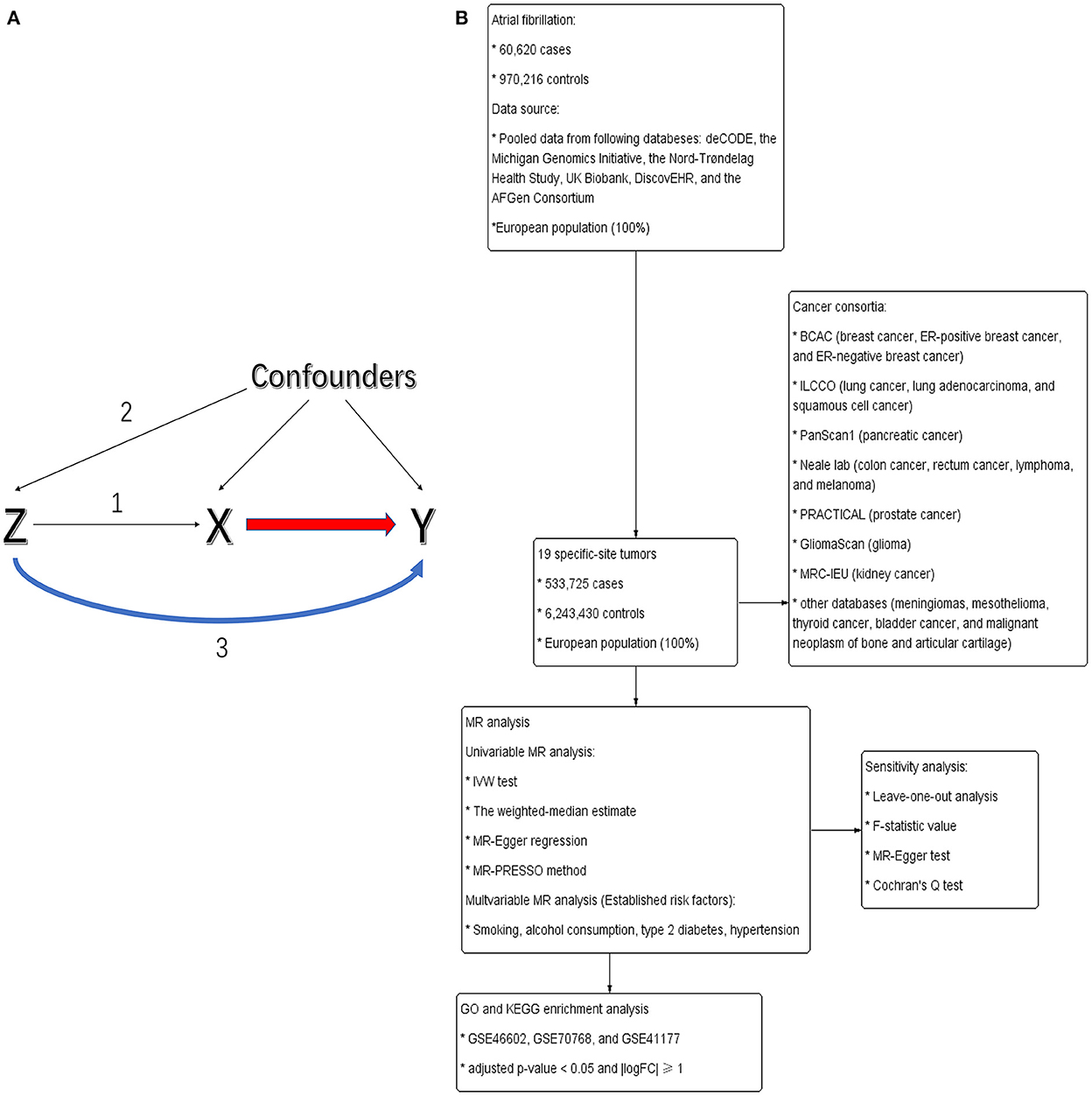
Figure 1. (A) Directed acyclic graph portraying the design of Mendelian randomization and potential pleiotropy. Genetic variants (Z) served as genetic instruments to evaluate whether exposures (X) were causally related to outcomes (Y). Numbers 1–3 represent assumptions 1–3. (B) The flowchart of this study.
2.2. Selection of genetic instruments for exposures
The flowchart of this study is shown in Figure 1B. Exposures considered in this analysis included AF and HF. Variant AF/HF relationships were derived and manually extracted from the available summary-level GWAS. Nielsen et al. (18) carried out a GWAS with 60,620 AF cases and 970,216 controls to distinguish genetic variations. This study was mainly derived from six databases [deCODE, the Michigan Genomics Initiative (MGI), the Nord-Trøndelag Health Study (HUNT), UK Biobank, DiscovEHR, and the AFGen Consortium]. All enrolled patients were of European descent and generally elder people (the median age at first AF diagnosis: MGI: 65; deCODE: 74; HUNT: 76). The genetic variation of HF was derived from a GWAS meta-analysis of HF comprising 47,309 European-descent cases and 930,014 controls from the Heart Failure Molecular Epidemiology for Therapeutic Targets Consortium (19).
Given that the violation of three MR assumptions may lead to unreliable conclusions, the following steps would help choose the best IVs. First, we extracted accessible summary-level data from Nielsen et al. (18) and Sonia et al. (19) and set up a significance threshold of p < 5 × 10−8. Detailed information about AF/HF-related single-nucleotide polymorphisms (SNPs) is shown in Supplementary Table 1. To measure genetic correlation, we further conducted a linkage disequilibrium (LD) clumping test at an R2 < 0.001 and 10,000 kb window to preserve the SNPs that were most robustly associated with the AF/HF for downstream analysis. To exclude bias from weak instruments, the F-statistic value was assessed on the bias of the formula , where R2 is the proportion of variance explained by the IVs, k and N are the number of SNPs and enrolled patients, respectively. F-statistic values > 10 were robust enough to avoid weak instrument bias. Eventually, several SNPs were excluded to eradicate the genetic bias created by palindromes with intermediate allele frequencies (20), and we created a total of 111 and 12 SNPs as the original AF- and HF-IVs, respectively.
2.3. Study participants of various types of cancer
To obtain genetic data for 19 site-specific tumors, we analyzed the following European cancer consortia: Breast Cancer Association Consortium (BCAC) (21) (breast cancer: 122,977 patients and 105,974 controls; ER-positive breast cancer: 69,501 patients and 105,974 controls; and ER-negative breast cancer: 21,468 patients and 105,974 controls), Prostate Cancer Association Group to Investigate Cancer Associated Alterations in the Genome (PRACTICAL) (22) [prostate cancer (PCA): 79,148 patients and 61,106 controls], International Lung Cancer Consortium (ILCCO) (23) (lung cancer: 11,348 patients and 15,861 controls, lung adenocarcinoma: 3,442 patients and 14,895 controls; and squamous cell cancer: 3,275 patients and 15,038 controls), PanScan1 (24) (pancreatic cancer: 1,896 patients, 1,939 controls), GliomaScan (25) (glioma: 6,811 patients, 1,856 controls), the Medical Research Council-Integrative Epidemiology Unit (MRC-IEU) consortium (kidney cancer: 1,114 patients, 461,896 controls; pancreatic cancer: 1,114 patients, 461,896 controls; rectum cancer: 1,470 patients, 461,540 controls), Neale lab (colon cancer: 2,437 patients, 358,757 controls; lymphoma: 1,752 patients, 359,442 controls; melanoma: 3,598 patients, 459,335 controls), and other databases (malignant neoplasm of bone and articular cartilage: 119 patients, 174,006 controls; thyroid cancer: 989 patients, 174,006 controls; bladder cancer: 1,279 patients, 372,016 controls; mesothelioma: 133 patients, 174,006 controls; meningiomas: 455 patients, 86,713 controls) (Table 1).
2.4. Statistical analysis of MR estimates
All MR analyses were performed in R 4.0.5 using the package TwoSampleMR (version 0.5.0).
2.4.1. Two-sample MR method
We performed the inverse-variance weighted (IVW) test, which can provide a coherent estimation of the causality between genetically determined exposures and outcomes. It is made up of a meta-analysis of a single SNP's Wald ratio between the exposures and outcomes using a random-effects inverse-variance method, which can weigh every single Wald ratio according to its standard error to judge potential measurable heterogeneity (20). The causal effects were calculated and presented in the form of odds ratios (ORs) with 95% confidence intervals (CIs) for 14 site-specific types of cancer. Two-sided p-values < 0.05 were considered to be statistically significant. Of note, the results of IVW tests might be biased given the horizontal pleiotropy in invalid instrumental variables. Hence, the MR-Egger regression and the weighted-median estimate were conducted to predominantly assess the MR outcomes (20, 26). The MR-Egger regression can amend the IVW test by allowing a nonzero intercept that can provide an exploration of pleiotropy and an evaluation of the causality adjusted for pleiotropy (20). The weighted-median analysis is used to pool the median effects of all SNPs and can return an unbiased estimate once 50% of the SNPs are valid instruments (20). Finally, the MR Pleiotropy Residual Sum and Outlier (MR-PRESSO) test was conducted using the “MRPRESSO” R package to distinguish outlying SNPs that may result in horizontal pleiotropy and causal effects.
2.4.2. Multivariable MR analysis
To support the univariate MR results and the third assumption, multivariable MR analyses adjusted for confounders, including smoking (trait ID: ukb-a-225), alcohol consumption (trait ID: ukb-d-20117_2), type 2 diabetes (trait ID: ebi-a-GCST006867), and hypertension (trait ID: ukb-b-14057) were introduced. Multivariable MR showed that the SNPs used in univariate MR analyses were also related to these confounders. Then, multivariable MR estimated the effects of each exposure on a single outcome. That is, this can simultaneously assess the effects of all risk factors that share a set of overlapping SNPs and make sure that the direct effects of each exposure on outcomes will not be mediated by other factors (27). As we included MR analyses of 19 site-specific types of cancer, a Bonferroni-adjusted p-value less than the threshold (i.e., 0.05/19 = 0.0026) was deemed as a significant causality to adjust for multiple-comparison tests. A potential relationship was considered significant if a p-value is between 0.05 and 0.0026.
2.5. Pleiotropy and sensitivity analysis
We conducted the leave-one-out analyses to assess whether the results of the IVW tests would be biased by single-sensitive SNPs (26). The aforementioned Egger intercept analysis was then performed to estimate the horizontal pleiotropy. The MR-heterogeneity analysis was ultimately performed to single out SNPs that were responsible for heterogeneity in casual estimation by means of Cochran's Q-test (28).
2.6. Identification and enrichment analyses of DEGs
Two PCA-related microarray datasets [GSE46602 (29) and GSE70768 (30)] and one AF-related dataset [GSE41177 (31)] were downloaded from the GEO (http://www.ncbi.nlm.nih.gov/geo) database to select differentially expressed genes (DEGs). Herein, genes with an adjusted p-value of < 0.05 and |logFC| ≥ 1 were considered DEGs. Gene Ontology (GO) and Kyoto Encyclopedia of Genes and Genomes (KEGG) pathway were performed to explore the potential biological functions of DEGs.
3. Results
In general, this MR study included a total of 6,334,916 European-descent people, with 463,502 cases and 5,871,414 controls (Table 1). Considering the variation in sample sizes of different cancers, F-statistics values in this study ranged from 273.8 to 369.1. The instruments (F > 100) used in our MR analyses were very strong to avoid bias (Table 1). All the MR evaluations for multi-polymorphism scores are shown in Tables 2, 3. Our results indicated that the genetically predicted AF was associated with a decreased risk of cancers of PCA and found no detrimental effects of AF/HF on the other 18 site-specific cancer risks (Tables 2, 3). The estimated effect sizes of the SNPs on both exposure (AF) and outcomes (PCA, breast cancer, lung cancer, and kidney cancer) are displayed in scatterplots (Figure 2).
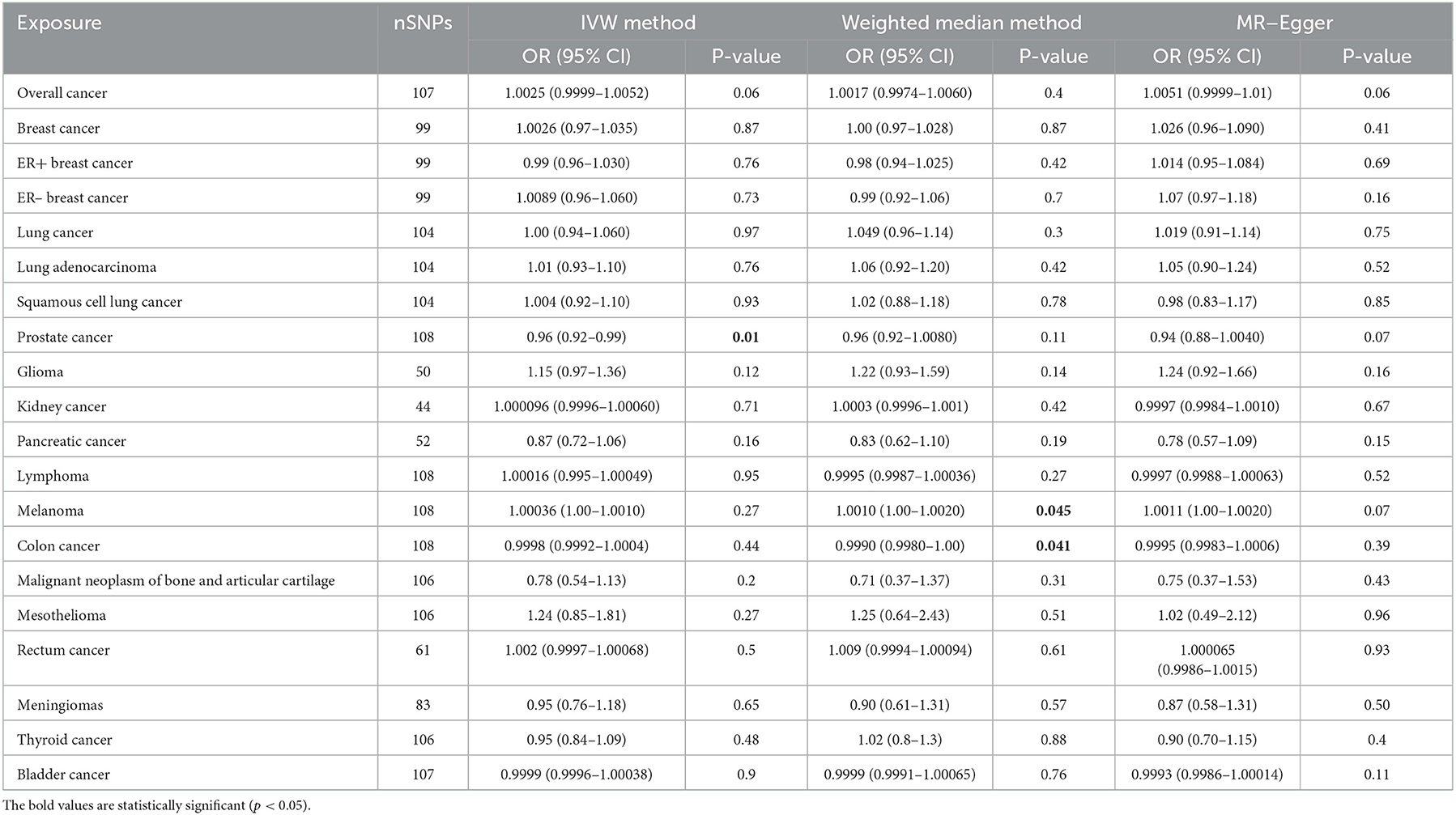
Table 2. Mendelian randomization estimates of the casual relationships between atrial fibrillation and cancer risks.
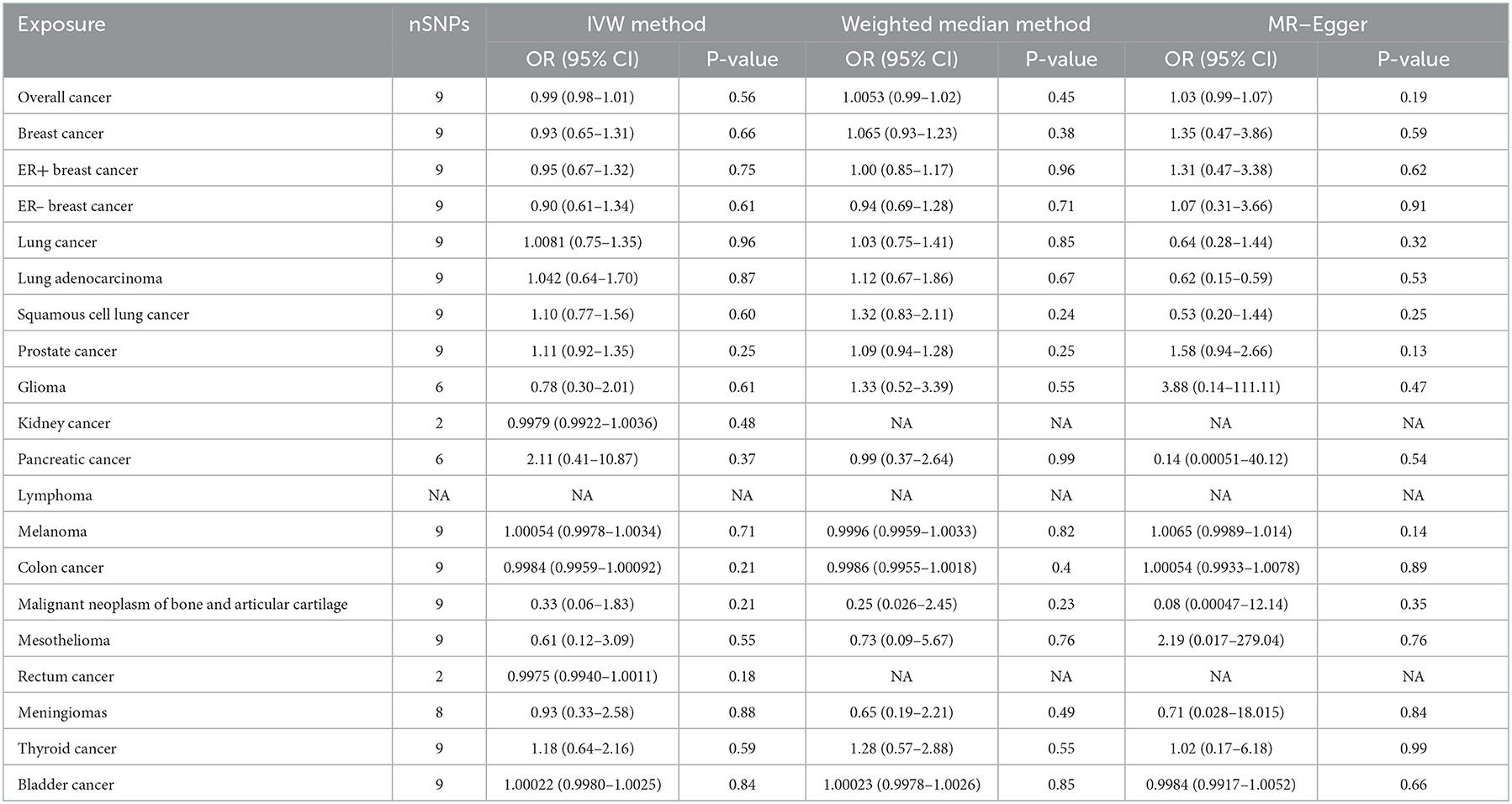
Table 3. Mendelian randomization estimates of the associations between heart failure and cancer risks.
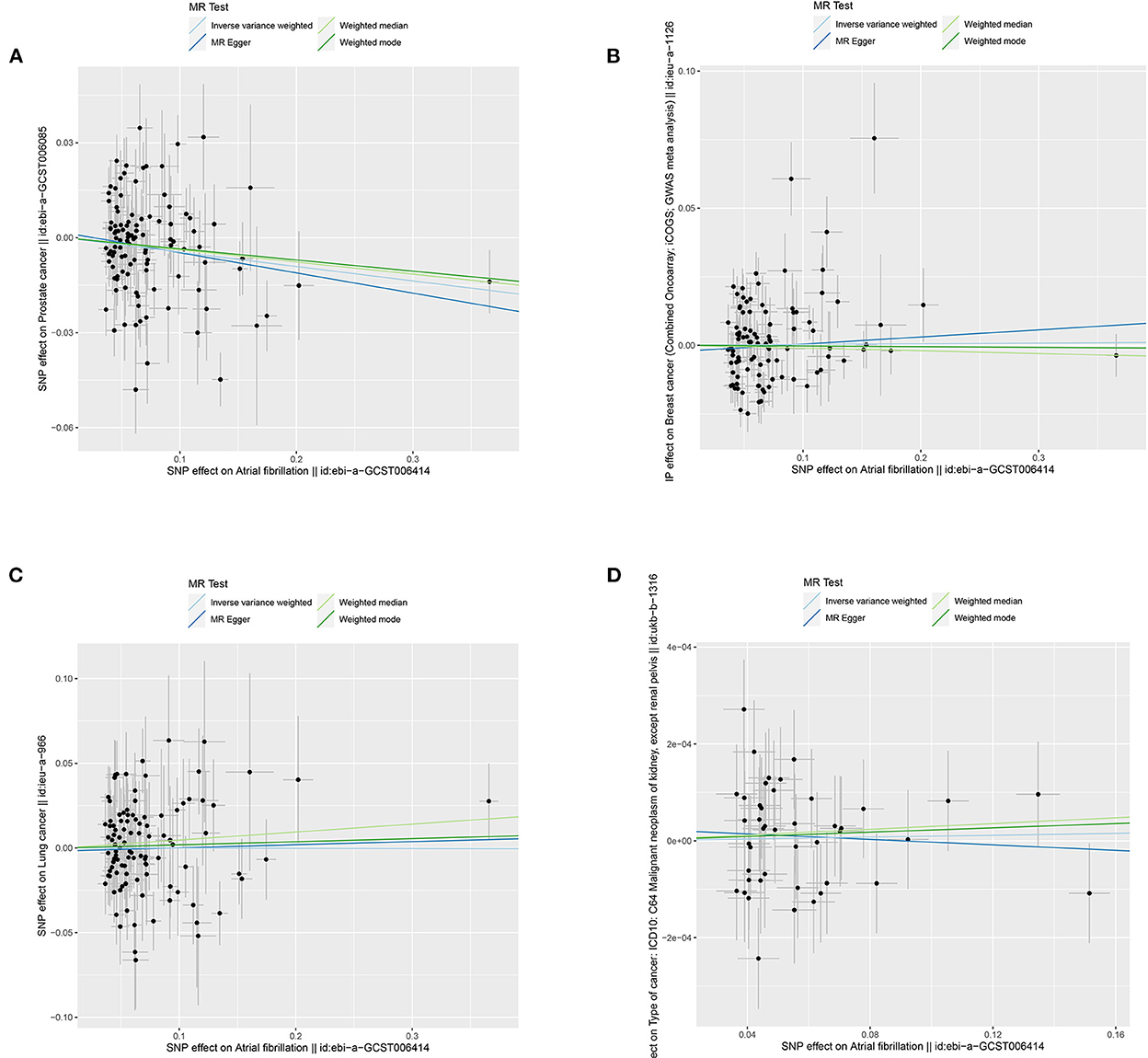
Figure 2. Scatterplots for MR analyses of the causal effect of atrial fibrillation on cancer risks: (A) prostate cancer; (B) breast cancer; (C) lung cancer; and (D) kidney cancer. The x-axis represents the previously published β-estimate for the association of each SNP with atrial fibrillation. The y-axis outlines the β-estimate for the relationship of each SNP with cancer risks by means of the multivariate logistic regression model. Lines represent causal estimates from the different methods. The slope of each line indicates the estimated MR effect per method. Circles correspond to marginal genetic associations with atrial fibrillation and risk of outcome for each variant. Error bars indicate 95% confidence intervals. SD, standard error; MR, Mendelian randomization.
3.1. Association of genetic liability to exposures with cancer risks
3.1.1. Univariable MR results
The results of the IVW test revealed a suggestive association of genetic liability to AF and PCA (OR = 0.96; 95% CI 0.92–0.99, p = 0.01) (Table 2). However, no relationships of genetic liability to AF with lower odds of breast cancer (OR = 1.003; 95% CI 0.97–1.035, p = 0.87), ER-negative breast cancer (OR = 1.009; 95% CI 0.96–1.06, p = 0.7), ER-positive breast cancer (OR = 0.99; 95% CI 0.96–1.03, p = 0.76), lung cancer (OR = 1.00; 95% CI 0.94–1.06, p = 0.97), lung adenocarcinoma (AF: OR = 1.01; 95% CI 0.93–1.10, p = 0.76), and squamous cell lung cancer (OR = 1.004; 95% CI 0.92–1.10, p = 0.93) were observed. Regarding other 12 site-specific types of cancer, limited evidence validated a causal association of genetic liability to AF with the risk of kidney cancer (OR = 1.0001; 95% CI 0.9996–1.0006, p = 0.71), melanoma (OR = 1.0003; 95% CI 0.9999–1.0011, p = 0.93), lymphoma (OR = 1.00016; 95% CI 0.995–1.00049, p = 0.95), glioma (OR = 1.15; 95% CI 0.97–1.36, p = 0.12), colon cancer (OR = 0.9998; 95% CI 0.9992–1.0004, p = 0.44), rectum cancer (OR = 1.0002; 95% CI 0.9997–1.00068, p = 0.27), meningiomas (OR = 0.95; 95% CI 0.76–1.18, p = 0.65), thyroid cancer (OR = 0.95; 95% CI 0.84–1.09, p = 0.48), and bladder cancer (OR = 0.9999; 95% CI 0.9996–1.00038, p = 0.9); malignant neoplasm of bone and articular cartilage (OR = 0.78; 95% CI 0.54–1.13, p = 0.2); and mesothelioma (OR = 1.24; 95% CI 0.85–1.81, p = 0.27) (Table 2). Some outliers were observed with the MR-PRESSO analysis, and the results remained in line with the original ones after removing these outliers (Supplementary Table 2). Additionally, we also found no associations between HF and 19 site-specific cancer risks (Table 3).
3.1.2. Multivariable MR analysis
As illustrated in Figure 3 and Supplementary Table 3, after adjusting for potential pleiotropic or mediating effects, multivariable MR still expounded strong independent associations between genetic predisposition to AF and PCA (OR = 0.94; 95% CI 0.90–0.98, p = 0.0048) and yielded similar results that AF was not associated with the increased risk of other site-specific cancer types.
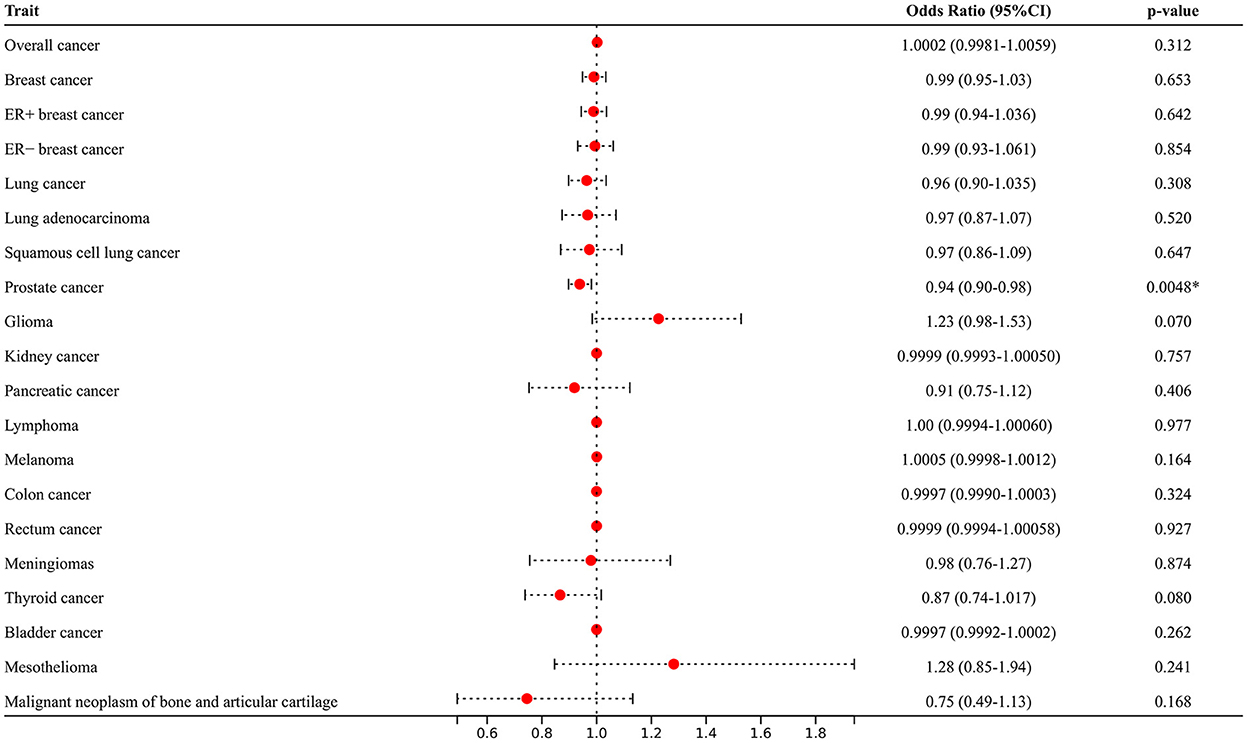
Figure 3. Multivariable Mendelian randomization analyses adjusted for smoking, alcohol consumption, diabetes, and hypertension. *Means statistically significance.
3.2. Assessment of MR assumptions
The first assumption was met because our included SNPs were selected at the genome-wide significance threshold of p < 5 × 10−8 and F-statistics values ranged from 273.8 to 369.1 (F > 100). Leave-one-out analysis suggested that individual SNPs had no impact on the overall effect of AF on cancer risks. Moreover, the MR-Egger regression analysis suggested that the impact of pleiotropy was negligible because intercepts were not statistically significant (all p-values>0.05) (Supplementary Table 4). Sensitive analyses demonstrated that the second MR assumption was not violated. Although the Cochrane Q-tests showed certain horizontal pleiotropy, little influence affected the overall results because no pleiotropy biased the results of the MR-Egger and MR-PRESSO tests (32). With regard to the third MR assumption, multivariable MR and MR-PRESSO analyses eliminated pleiotropic effects, which abided by the third MR assumption.
3.3. Analysis of the functional characteristics of common DEGs
In total, 51 common DEGs between AF-related and PCA-related datasets were identified (Figure 4A). Results of the KEGG pathway demonstrated that several significant enrichment pathways were noted, such as glutathione metabolism and metabolism of xenobiotics by cytochrome P450 (all p-values were <0.05) (Figure 4B). Regarding GO analysis, these DEGs were mainly enriched in cellular detoxification, xenobiotic metabolic process, glutathione derivative metabolic process, cellular response to xenobiotic stimulus, glutathione binding, and antioxidant activity (all p-values were <0.05) (Figures 4C, D). These outcomes firmly revealed that the antioxidant activity, xenobiotic catabolic process, and cytochrome P450 metabolism were involved in the development of AF and PCA.
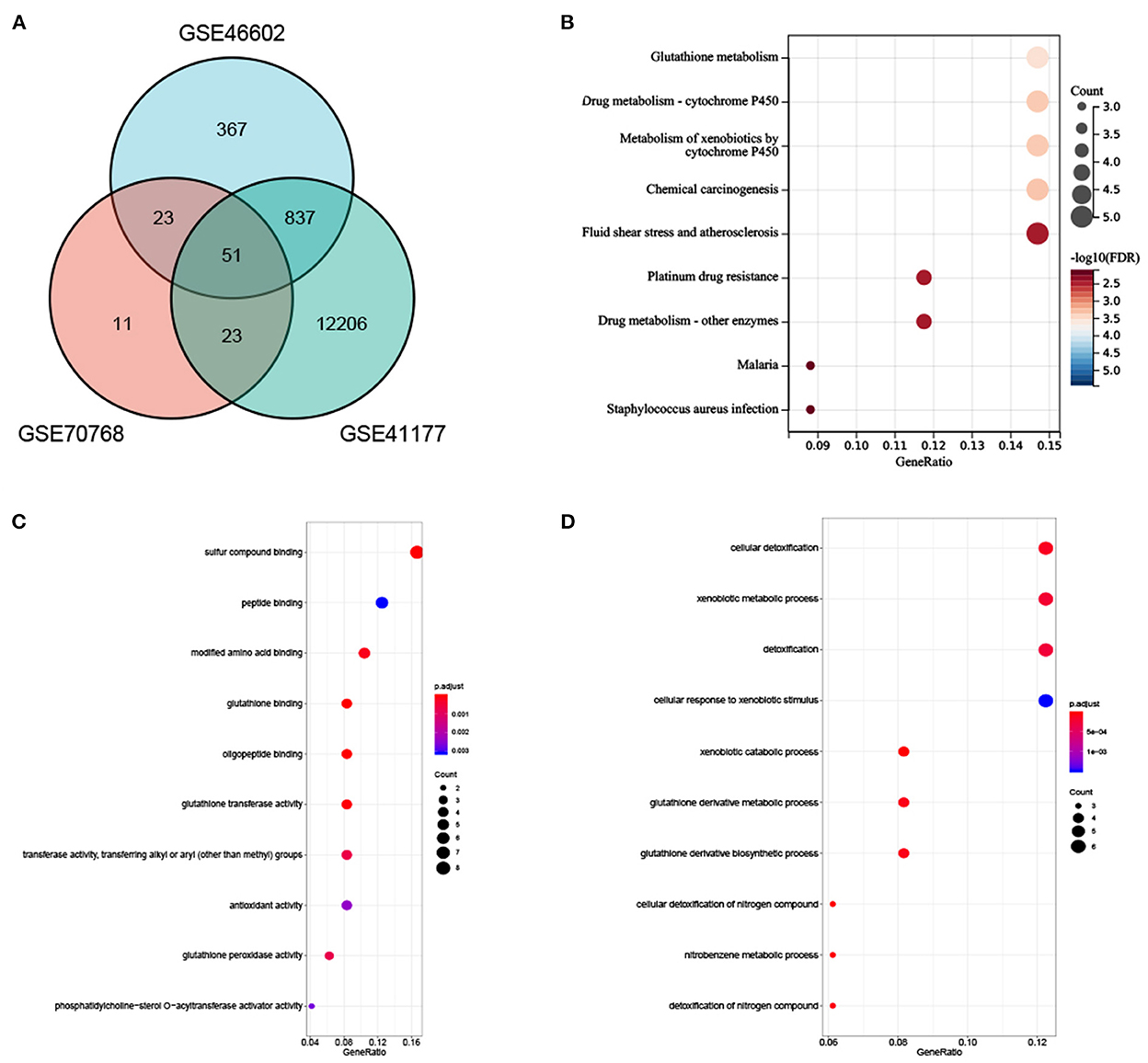
Figure 4. (A) Venn diagram showed an overlap of 51 DEGs. (B) The enrichment analysis results of KEGG Pathway. (C, D) The enrichment analysis results of GO terms.
4. Discussion
4.1. Principal findings
In this study, we performed MR analyses to evaluate whether genetic evidence supported a causal association between AF and the risk of 19 site-specific types of cancer. To the best of our knowledge, this is the first cardio-oncology MR study involving 6,334,916 people to explicate that AF may casually reduce the risk of PCA. Moreover, little evidence demonstrated that AF and closely related HF were casually related to the increased risks of lung cancer, breast cancer, PCA, kidney cancer, glioma, pancreatic cancer, colon cancer, rectum cancer, meningiomas, thyroid cancer, bladder cancer, lymphoma, or melanoma, with less susceptibility to potential confounders and inverse causation. Based on our findings, cancer screening beyond standard routine healthcare may not be currently merited with a new diagnosis of AF. Nonetheless, a tight collaboration between cardiologists and oncologists is still essential to improve the management of patients, which may provide crucial mechanistic and therapeutic insights with regard to both serious conditions.
4.2. Previous research
The present MR findings do not support previous observational studies, suggesting that the manifestation of AF was a marker of occult cancer. Several sporadic epidemiological trials have described the underlying effect of AF on site-specific malignancies, but their findings were very controversial. An observational cohort study by Conen et al. (12) included 34,691 women and revealed that women with new-onset AF may have an elevated cancer risk beyond 1 year of AF diagnosis. This questionnaire-derived study also indicated that new-onset AF was statistically significant for the risk of colon cancer, whereas significant multivariable-adjusted relationships for breast cancer were not observed (12). These findings were consistent with a Danish population-based cohort study enrolling 26,222 men and 28,879 women free of AF (8). In this cohort trial, AF was not related to breast cancer or PCA (8), but the risk of colorectal cancer and lung cancer was paradoxically remarkably high within the initial 90 days following the diagnosis of AF (8). A retrospective cohort of 5,130 patients, however, demonstrated that the standard incidence ratio of lung and colon cancer was significantly high in patients with AF, although there was no significant increase in the risks of liver or breast cancer (11). Interestingly, evidence from a prospective cohort study even reached a contrasting conclusion that AF was related to decreased odds of the new diagnosis of breast cancer and colorectal cancer, indicating that an association noted in a previous study may be caused by potential detection bias instead of a causal relationship (9).
Atrial fibrillation and HF are often presented together with each other (16), and a similar scenario also holds for the relationship between HF and cancer risks. Retrospective research has suggested that the prevalence of malignancy in established HF ranges from 18.9 to 33.7 per 1,000 person-years (33–35). Conversely, a study with larger cohorts and longer follow-up durations explicated that HF was neither associated with an increased risk of cancer nor cancer-specific deaths (36). Overall, according to previous studies, an association between AF and malignant tumors has been reported but is largely controversial.
4.3. The interpretation of the observed results
The potential limitations in previous epidemiological studies may bias the observed outcomes. The conflicting outcomes regarding cancer risks and AF in prior studies may be mediated by lots of possibilities, and some of the potential relationships are complex.
First, it was not surprising to see elevated cancer risks in patients with AF because related treatments render clinically overt cancer that could be otherwise asymptomatic. Several cardiovascular drugs, including spironolactone (37) and aspirin (38), have been shown to lower the carcinogenesis of certain types of cancer. In addition to the effect of related treatments, inherent drawbacks in observational study design, such as shorter follow-up duration, possible selection/surveillance bias (8, 12), and a lack of comprehensive data on shared risk factors (14, 39), could explain the perplexing results. The median time from AF/HF to malignancy diagnosis in some prior investigations was <3 years (33, 35), which might be too short of a period to expound a causal association. With regard to selection or surveillance bias, some questionnaire-based studies may be unable to accurately determine whether patients underwent the examination before or after AF diagnosis (12). Besides, patients with screen-detected AF are more possible to have cancer screenings at an early stage, which is usually missed in the general population. For instance, if silent malignancies stay undetected, AF-related antithrombotic (40) or anticoagulant agents (8, 12) could increase the positivity rate of intestinal hemorrhage or hematuria, thus followed by several cancer detections (41). Third, AF and cancer are complex conditions and share many common risk factors, including alcohol consumption, smoking, hypertension, and diabetes (42). The risk of malignancy in patients with AF will naturally increase with the presence of the number of these risk factors. Hence, minimizing the effects of confounders and limitations of study designs is necessary for the evaluation of causality.
4.4. Possible mechanisms
In the present MR analysis, we found that AF may lower the risk of PCA using univariable and multivariable MR methods. The observed findings may be first attributed to an atrial natriuretic peptide (ANP), which may provide meaningful information about the underlying mechanism. AF is an independent determinant of ANP that exerts an important role in restraining tumor growth (43, 44). The inhibition of malignant cell proliferation by ANP is mediated by both intracellular acidity and Wnt/β-catenin signaling (45). ANP might also hinder the adhesion of malignant cells to microvascular endothelial cells by suppressing the E-selectin expression, which is regulated by inflammation (46). Second, AF-related hypercoagulability would alter cancer cell adhesion and tumor progression by decreasing matrix metalloproteinases (MMPs) in tissue and increasing circulating levels of inhibitors of matrix metalloproteinases (TIMPs) (5). TIMPs could control MMP that could lead to the degradation of the extracellular matrix and, consequently, organize the path for malignant cells to progress and spread to distant secondary areas (47). Third, certain immune-related genes identified in AF have recently been linked to the prognosis and immune infiltration in several tumor types (48). Herein, present KEGG and GO enrichment analyses of 51 common DEGs also revealed that these genes were significantly enriched in antioxidant activity, xenobiotic catabolic processes, and cytochrome P450 metabolism pathways. It has been expounded that the cause of PCA occurrence might be the outcome of an imbalance of antioxidants (49). Antioxidant defenses might be notably attenuated in patients with PCA (50). Moreover, environmental xenobiotics are largely involved in PCA development and are metabolized by cytochrome P450 in the human organism (51).
4.5. Strengths and limitations
The present MR study has several notable strengths. First, this is the first MR study conducted to evaluate the causal association between AF and cancer risks. MR analysis is deemed a reliable epidemiological method to evaluate the causality between exposures and outcomes. Residual confounding from unmeasured variations of baseline information may not ascertain cause–effect associations in previous studies (7, 8, 11, 12). The MR analysis, however, may better diminish the interference of confounders and inverse causation. Moreover, we were more likely to portray a relatively independent causal inference from AF to cancer risks with the multivariable MR approach adjusted for confounders. Second, the included AF-associated SNPs as IVs were gained from all documented GWASs, which may better explicate the variation of AF. Third, the present genetic summary data of certain types of cancer were obtained from large-scale consortia (namely, ILCCO, BCAC, and PRACTICAL), including millions of cases, which were far more than some previous studies (7, 11, 12). Compared with the low-occurrence rates of certain tumors in the previous studies (7, 11, 12), the present results from a relatively large sample size and strongly related IVs could present sufficient statistical power and a precise assessment of causal effects.
Some drawbacks should be taken into account to better elucidate the present findings. The participants in our study were of European descent. Thus, the results of our analysis were less likely to be biased by population stratification, but whether our assertion could be generalizable to other populations for different genetic backgrounds needs to be verified. Besides, the sample size of several site-specific cancer types in our analysis was small. For example, the consortium of malignant plasma cell neoplasms consisted of only 180 patients compared with its vast number of 87,061 controls. The statistical power may not estimate their causality accurately. Finally, the association between AF and PCA was not maintained in the results of MR weighted-median and MR-Egger analyses. However, the direction of MR estimates was consistent among IVW, weighted median, and MR–Egger methods in this study. Moreover, MR-PRESSO and multivariable MR tests were conducted to distinguish possible horizontal pleiotropy and supported the original IVW results.
5. Conclusion
This large cardio-oncology study revealed that AF may reduce the risk of PCA. Despite the lack of a causal relationship between AF and increased cancer risks, we should not ignore the two diseases' shared risk factors and pathophysiological mechanisms. Numerous studies still investigate the complicated interrelations between AF and cancer stay and, with an aging population, it represents a valuable field for future investigation. A multidisciplinary approach is still needed to better understand the underlying mechanisms regarding the links between AF and cancer risks.
Data availability statement
The datasets presented in this study can be found in online repositories. The names of the repository/repositories and accession number(s) can be found in the article/Supplementary material.
Ethics statement
Ethical review and approval was not required for the study on human participants. Written informed consent was not required to participate in this study.
Author contributions
WL, MH, and WW conceived and designed the study, collected, analyzed, and interpreted the data. The first version of the manuscript was written by WL, RW, and WW. All authors contributed to the article and approved the submitted version.
Funding
This study was supported by the National Natural Science Foundation of China Grants (81172243 and 81572966 to WW) and the Natural Science Foundation of Guangdong Province (2017A030313883 to WW).
Acknowledgments
The authors would like to appreciate the efforts of the consortia in providing high-quality GWAS resources for scholars. Data and material can be available from the corresponding GWAS consortium.
Conflict of interest
The authors declare that the research was conducted in the absence of any commercial or financial relationships that could be construed as a potential conflict of interest.
Publisher's note
All claims expressed in this article are solely those of the authors and do not necessarily represent those of their affiliated organizations, or those of the publisher, the editors and the reviewers. Any product that may be evaluated in this article, or claim that may be made by its manufacturer, is not guaranteed or endorsed by the publisher.
Supplementary material
The Supplementary Material for this article can be found online at: https://www.frontiersin.org/articles/10.3389/fcvm.2022.974402/full#supplementary-material
Abbreviations
AF, atrial fibrillation; ANP, atrial natriuretic peptide; CIs, confidence intervals; DEG, differentially expressed gene; GWAS, genome-wide association study; GO, Gene Ontology; HF, heart failure; IVW, inverse-variance weighted; KEGG, Kyoto Encyclopedia of Genes and Genomes; LD, linkage disequilibrium; MR, Mendelian randomization; OR, odds ratio; PCA, prostate cancer; MR-PRESSO, MR Pleiotropy Residual Sum and Outlier; SNP, single-nucleotide polymorphism.
References
1. Rahman F, Kwan G, Benjamin E. Global epidemiology of atrial fibrillation. Nat Rev. Cardiol. (2014) 11:639–54. doi: 10.1038/nrcardio.2014.118
2. Lippi G, Sanchis-Gomar F, Cervellin G. Global epidemiology of atrial fibrillation: an increasing epidemic and public health challenge. Int J Stroke Off. (2021) 16:217–21. doi: 10.1177/1747493019897870
3. Walli-Attaei M, Joseph P, Rosengren A, Chow C, Rangarajan S, Lear S, et al. Variations between women and men in risk factors, treatments, cardiovascular disease incidence, and death in 27 high-income, middle-income, and low-income countries (Pure): a prospective cohort study. Lancet. (2020) 396:97–109. doi: 10.1016/S0140-6736(20)30543-2
4. Erichsen R, Christiansen C, Mehnert F, Weiss N, Baron J, Sørensen H. Colorectal cancer and risk of atrial fibrillation and flutter: a population-based case-control study. Intern Emerg Med. (2012) 7:431–8. doi: 10.1007/s11739-011-0701-9
5. Chu G, Versteeg H, Verschoor A, Trines S, Hemels M, Ay C, et al. Atrial fibrillation and cancer - an unexplored field in cardiovascular oncology. Blood Rev. (2019) 35:59–67. doi: 10.1016/j.blre.2019.03.005
6. Roderburg C, Loosen S, Jahn J, Gänsbacher J, Luedde T, Kostev K, et al. Heart failure is associated with an increased incidence of cancer diagnoses. ESC Heart Failure. (2021) 8:3628–33. doi: 10.1002/ehf2.13421
7. Ostenfeld E, Erichsen R, Pedersen L, Farkas D, Weiss N, Sørensen H. Atrial Fibrillation as a marker of occult cancer. PLoS ONE. (2014) 9:e102861. doi: 10.1371/journal.pone.0102861
8. Vinter N, Christesen A, Fenger-Grøn M, Tjønneland A, Frost L. Atrial fibrillation and risk of cancer: a Danish population-based cohort study. J Am Heart Assoc. (2018) 7:e009543. doi: 10.1161/JAHA.118.009543
9. Saliba W, Rennert H, Gronich N, Gruber S, Rennert G. Association of atrial fibrillation and cancer: analysis from two large population-based case-control studies. PLoS ONE. (2018) 13:e0190324. doi: 10.1371/journal.pone.0190324
10. Karnik A, Benjamin E, Trinquart L. Breast cancer and atrial fibrillation-a malignant combination? Heart Rhythm. (2019) 16:349–50. doi: 10.1016/j.hrthm.2018.11.016
11. Hung C, Chang C, Lin J, Ho Y, Chen M. The association between new onset atrial fibrillation and incident cancer-a nationwide cohort study. PLoS ONE. (2018) 13:e0199901. doi: 10.1371/journal.pone.0199901
12. Conen D, Wong J, Sandhu R, Cook N, Lee I, Buring J, et al. Risk of malignant cancer among women with new-onset atrial fibrillation. JAMA Cardiol. (2016) 1:389–96. doi: 10.1001/jamacardio.2016.0280
13. Conen D, Ridker P, Everett B, Tedrow U, Rose L, Cook N, et al. A multimarker approach to assess the influence of inflammation on the incidence of atrial fibrillation in women. Eur Heart J. (2010) 31:1730–6. doi: 10.1093/eurheartj/ehq146
14. Koene R, Prizment A, Blaes A, Konety S. Shared risk factors in cardiovascular disease and cancer. Circulation. (2016) 133:1104–14. doi: 10.1161/CIRCULATIONAHA.115.020406
15. Burgess S, Scott R, Timpson N, Davey Smith G, Thompson S. Using published data in mendelian randomization: a blueprint for efficient identification of causal risk factors. Eur J Epidemiol. (2015) 30:543–52. doi: 10.1007/s10654-015-0011-z
16. Carlisle M, Fudim M, DeVore A, Piccini J. Heart failure and atrial fibrillation, like fire and fury. JACC Heart Failure. (2019) 7:447–56. doi: 10.1016/j.jchf.2019.03.005
17. VanderWeele T, Tchetgen Tchetgen E, Cornelis M, Kraft P. Methodological challenges in Mendelian randomization. Epidemiology. (2014) 25:427–35. doi: 10.1097/EDE.0000000000000081
18. Nielsen J, Thorolfsdottir R, Fritsche L, Zhou W, Skov M, Graham S, et al. Biobank-driven genomic discovery yields new insight into atrial fibrillation biology. Nat Genet. (2018) 50:1234–9. doi: 10.1038/s41588-018-0171-3
19. Shah S, Henry A, Roselli C, Lin H, Sveinbjörnsson G, Fatemifar G, et al. Genome-wide association and mendelian randomisation analysis provide insights into the pathogenesis of heart failure. Nat Commun. (2020) 11:163. doi: 10.1038/s41467-019-13690-5
20. Hemani G, Zheng J, Elsworth B, Wade K, Haberland V, Baird D, et al. The Mr-base platform supports systematic causal inference across the human phenome. Elife. (2018) 7:34408. doi: 10.7554/eLife.34408
21. Michailidou K, Lindström S, Dennis J, Beesley J, Hui S, Kar S, et al. Association analysis identifies 65 new breast cancer risk loci. Nature. (2017) 551:92–4. doi: 10.1038/nature24284
22. Schumacher F, Al Olama A, Berndt S, Benlloch S, Ahmed M, Saunders E, et al. Association analyses of more than 140,000 men identify 63 new prostate cancer susceptibility loci. Nat Genet. (2018) 50:928–36. doi: 10.1038/s41588-018-0142-8
23. Wang Y, McKay J, Rafnar T, Wang Z, Timofeeva M, Broderick P, et al. Rare variants of large effect in brca2 and chek2 affect risk of lung cancer. Nat Genet. (2014) 46:736–41.
24. Amundadottir L, Kraft P, Stolzenberg-Solomon R, Fuchs C, Petersen G, Arslan A, et al. Genome-wide association study identifies variants in the abo locus associated with susceptibility to pancreatic cancer. Nat Genet. (2009) 41:986–90. doi: 10.1038/ng.429
25. Rajaraman P, Melin B, Wang Z, McKean-Cowdin R, Michaud D, Wang S, et al. Genome-wide association study of glioma and meta-analysis. Hum Genet. (2012) 131:1877–88. doi: 10.1007/s00439-012-1212-0
26. Verbanck M, Chen C, Neale B, Do R. Detection of widespread horizontal pleiotropy in causal relationships inferred from Mendelian randomization between complex traits and diseases. Nat Genet. (2018) 50:693–8. doi: 10.1038/s41588-018-0099-7
27. Burgess S, Thompson S. Multivariable mendelian randomization: the use of pleiotropic genetic variants to estimate causal effects. Am J Epidemiol. (2015) 181:251–60. doi: 10.1093/aje/kwu283
28. Bowden J, Del Greco M F, Minelli C, Davey Smith G, Sheehan N, Thompson J, et al. Framework for the investigation of pleiotropy in two-sample summary data Mendelian randomization. Stat Med. (2017) 36:1783–802. doi: 10.1002/sim.7221
29. Mortensen M, Høyer S, Lynnerup A, Ørntoft T, Sørensen K, Borre M, et al. Expression profiling of prostate cancer tissue delineates genes associated with recurrence after prostatectomy. Sci Rep. (2015) 5:16018. doi: 10.1038/srep16018
30. Ross-Adams H, Lamb A, Dunning M, Halim S, Lindberg J, Massie C, et al. Integration of copy number and transcriptomics provides risk stratification in prostate cancer: a discovery and validation cohort study. EBioMedicine. (2015) 2:1133–44. doi: 10.1016/j.ebiom.2015.07.017
31. Yeh Y, Kuo C, Lee Y, Lin Y, Nattel S, Tsai F, et al. Region-specific gene expression profiles in the left atria of patients with valvular atrial fibrillation. Heart Rhythm. (2013) 10:383–91. doi: 10.1016/j.hrthm.2012.11.013
32. Burgess S, Thompson S. Interpreting findings from mendelian randomization using the Mr-egger method. Eur J Epidemiol. (2017) 32:377–89. doi: 10.1007/s10654-017-0255-x
33. Hasin T, Gerber Y, Weston S, Jiang R, Killian J, Manemann S, et al. Heart failure after myocardial infarction is associated with increased risk of cancer. J Am Coll Cardiol. (2016) 68:265–71. doi: 10.1016/j.jacc.2016.04.053
34. Hasin T, Gerber Y, McNallan S, Weston S, Kushwaha S, Nelson T, et al. Patients with heart failure have an increased risk of incident cancer. J Am Coll Cardiol. (2013) 62:881–6. doi: 10.1016/j.jacc.2013.04.088
35. Banke A, Schou M, Videbaek L, Møller J, Torp-Pedersen C, Gustafsson F, et al. Incidence of cancer in patients with chronic heart failure: a long-term follow-up study. Eur J Heart Fail. (2016) 18:260–6. doi: 10.1002/ejhf.472
36. Selvaraj S, Bhatt D, Claggett B, Djoussé L, Shah S, Chen J, et al. Lack of association between heart failure and incident cancer. J Am Coll Cardiol. (2018) 71:1501–10. doi: 10.1016/j.jacc.2018.01.069
37. Mackenzie I, Morant S, Wei L, Thompson A, MacDonald T. Spironolactone use and risk of incident cancers: a retrospective, matched cohort study. Br J Clin Pharmacol. (2017) 83:653–63. doi: 10.1111/bcp.13152
38. Fu B, Wang K, Mucci L, Clinton S, Giovannucci E. Aspirin use and prostate tumor angiogenesis. Cancer Causes Control. (2021) 33:149–51. doi: 10.1007/s10552-021-01501-6
39. Meijers W, De Boer R. Common risk factors for heart failure and cancer. Cardiovasc Res. (2019) 115:844–53. doi: 10.1093/cvr/cvz035
40. Ameri P, Canepa M, Anker M, Belenkov Y, Bergler-Klein J, Cohen-Solal A, et al. Cancer diagnosis in patients with heart failure: epidemiology, clinical implications and gaps in knowledge. Eur J Heart Fail. (2018) 20:879–87. doi: 10.1002/ejhf.1165
41. Papanastasiou A, Morsi-Yeroyannis A, Karagiannidis E, Kartas A, Doundoulakis I, Karvounis H, et al. Association of anticoagulant-related bleeding events with cancer detection in atrial fibrillation: a systematic review and meta-analysis. Hellenic J Cardiol. (2020) 5:359–65. doi: 10.1016/j.hjc.2020.11.007
42. Menichelli D, Vicario T, Ameri P, Toma M, Violi F, Pignatelli P, et al. Cancer and atrial fibrillation: epidemiology, mechanisms, and anticoagulation treatment. Prog Cardiovasc Dis. (2021) 66:28–36. doi: 10.1016/j.pcad.2021.04.004
43. Rossi A, Enriquez-Sarano M, Burnett J, Lerman A, Abel M, Seward J. Natriuretic peptide levels in atrial fibrillation: a prospective hormonal and Doppler-echocardiographic study. J Am Coll Cardiol. (2000) 35:1256–62. doi: 10.1016/S0735-1097(00)00515-5
44. Vesely D. Cardiac and renal hormones: anticancer effects in vitro and in vivo. J Invest Med. (2009) 57:22–8. doi: 10.2310/JIM.0b013e3181948b25
45. Serafino A, Moroni N, Psaila R, Zonfrillo M, Andreola F, Wannenes F, et al. Anti-proliferative effect of atrial natriuretic peptide on colorectal cancer cells: evidence for an Akt-mediated cross-talk between Nhe-1 activity and Wnt/B-catenin signaling. Biochim Biophys Acta. (2012) 1822:1004–18. doi: 10.1016/j.bbadis.2012.02.016
46. Nojiri T, Hosoda H, Tokudome T, Miura K, Ishikane S, Otani K, et al. Atrial natriuretic peptide prevents cancer metastasis through vascular endothelial cells. Proc Natl Acad Sci U S A. (2015) 112:4086–91. doi: 10.1073/pnas.1417273112
47. Bourboulia D, Stetler-Stevenson W. Matrix metalloproteinases (Mmps) and tissue inhibitors of metalloproteinases (Timps): positive and negative regulators in tumor cell adhesion. Semin Cancer Biol. (2010) 20:161–8. doi: 10.1016/j.semcancer.2010.05.002
48. Yan T, Zhu S, Shi Y, Xie C, Zhu M, Zhang Y, et al. Anxa4pan-cancer analysis of atrial-fibrillation-related innate immunity gene. Front Cardiovas Med. (2021) 8:713983. doi: 10.3389/fcvm.2021.713983
49. Ahmed Amar S, Eryilmaz R, Demir H, Aykan S, Demir C. Determination of oxidative stress levels and some antioxidant enzyme activities in prostate cancer. J Int Soc Stud Aging Male. (2019) 22:198–206. doi: 10.1080/13685538.2018.1488955
50. Sajjaboontawee N, Supasitthumrong T, Tunvirachaisakul C, Nantachai K, Snabboon T, Reiche E, et al. Lower thiol, glutathione, and glutathione peroxidase levels in prostate cancer: a meta-analysis study. J Int Soc Stud Aging Male. (2020) 23:1533–44. doi: 10.1080/13685538.2020.1858048
Keywords: cancer, Mendelian randomization, single-nucleotide polymorphism, atrial fibrillation, prevention
Citation: Li W, Huang M, Wang R and Wang W (2023) Impact of genetically predicted atrial fibrillation on cancer risks: A large cardio-oncology Mendelian randomization study using UK biobank. Front. Cardiovasc. Med. 9:974402. doi: 10.3389/fcvm.2022.974402
Received: 21 June 2022; Accepted: 07 December 2022;
Published: 05 January 2023.
Edited by:
Feng Cao, People's Liberation Army General Hospital, ChinaReviewed by:
Zhenyu Huo, Guangzhou Medical University, ChinaDanilo Menichelli, Sapienza University of Rome, Italy
Alice Giontella, University of Verona, Italy
Copyright © 2023 Li, Huang, Wang and Wang. This is an open-access article distributed under the terms of the Creative Commons Attribution License (CC BY). The use, distribution or reproduction in other forums is permitted, provided the original author(s) and the copyright owner(s) are credited and that the original publication in this journal is cited, in accordance with accepted academic practice. No use, distribution or reproduction is permitted which does not comply with these terms.
*Correspondence: Wei Wang, d2FuZ3dlaTk1MDBAaG90bWFpbC5jb20=;
d3dlaTk1MDBAc211LmVkdS5jbg==
†These authors share co-first authorship