- 1School of Biological Science and Medical Engineering, Southeast University, Nanjing, China
- 2Zhongda Hospital, The Affiliated Hospital of Southeast University, Nanjing, China
- 3First Affiliated Hospital, Nanjing Medical University, Nanjing, China
- 4Duke-NUS Medical School, National University of Singapore, Singapore, Singapore
- 5National Heart Centre Singapore, Singapore, Singapore
- 6School of Mechanical, Medical and Process Engineering, Queensland University of Technology (QUT), Brisbane, QLD, Australia
Left atrial appendage (LAA) is believed to be a common site of thrombus formation in patients with atrial fibrillation (AF). However, the commonly-applied stroke risk stratification model (such as. CHA2DS2-VASc score) does not include any structural or hemodynamic features of LAA. Recent studies have suggested that it is important to incorporate LAA geometrical and hemodynamic features to evaluate the risk of thrombus formation in LAA, which may better delineate the AF patients for anticoagulant administration and prevent strokes. This review focuses on the LAA-related factors that may be associated with thrombus formation and cardioembolic events.
Introduction
Atrial fibrillation (AF) is the most common sustained rhythm disorder worldwide (1–4), and the worldwide AF incidence is approximately 1–2% (3–6), and the prevalence increases with age. In general, patients with AF have higher morbidity and mortality rates due to increased risk of stroke (7–10). The risk of ischemic stroke related to AF, increases from 4.6% for ages 50 through 59 years to 20.2% for ages 80 through 89 years (11). Therefore, AF prevalence is expected to dramatically increase with an aging population in the coming years (12).
In AF patients, irregular heart rhythm disturbs blood flow, which can lead to blood stagnation and clot formation, with subsequent dislodgment and embolization in the brain resulting in thromboembolic events (13–15). There is an agreement that AF increases stroke risk by 5-fold (16), where AF-related strokes are associated with greater disability, higher medical cost, poorer functional outcomes, and a lower chance of being discharged to home compared to non-AF-related strokes (17).
In AF patients with thromboembolic event, studies have revealed that more than 90% of thrombi originate in the left atrial appendage (LAA) (18–20), which is the remnant of the embryonic left atrium and originates from the primordial pulmonary vein and its branches (21–24). However, the commonly applied stroke risk scoring model (viz.: CHA2DS2 or CHA2DS2-VASc, shown in the following Table 1) in the clinic incorporates only patient demographics and clinical data (25), but do not include any morphological or hemodynamic information about the LAA, and we think that with the LAA information added, some high stroke risk patients but with low scores would be identified.
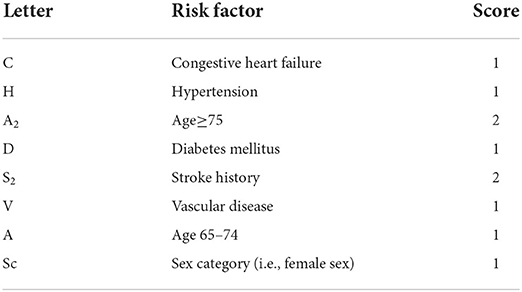
Table 1. Definition of CHA2DS2-VASc or CHADS2 (17).
Recent studies have shown extensive evidence that it is necessary to include some important LAA features to better evaluate the thromboembolic risk and prevent stroke risk. This study serves to overview of current concepts and recent developments in the relationship between stroke risk and LAA risk-related characteristics.
LAA characteristics related with stroke
A number of studies have focused on the stroke-related LAA characteristics. These characteristics can be classified into three groups: shape, morphology and hemodynamics. In the following paragraphs we elucidate the relationship between these characteristic groups and stroke risk.
LAA shape
Shape is an intuitive term for describing the external form, contours, or outline of the LAA, and various classification approaches have been proposed for describing LAA shape (26–29). In these classification schemes, a widely accepted classification, first proposed by Wang et al. (26), classifies LAA into 4 types: cactus, chicken wing, windsock and cauliflower (Figure 1). This classification was derived from 612 patients based on characteristics of bend, lobes and overall length. Where the characteristics were used as the guidance for LAA closure.
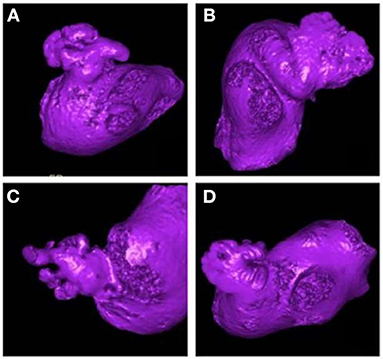
Figure 1. The shape classification of LAA. (A) Cactus, (B) Windsock, (C) Cauliflower, (D) Chicken wing. This picture is driven from the classification performed by the clinicians based on Wang's scheme (30).
Di Biase et al. (31) conducted an initial study to investigate the relationship between LAA shape and stroke risk. In their study, 932 AF patients were classified into four groups based on Wang's shape types obtained using computer tomography (CT)/magnetic resonance imaging (MRI), and they found that the chicken wing LAA type was less likely to have a stroke/transient ischemic attack (TIA), and the cauliflower was the highest stroke risk type. As the first study to focus on the association between stroke risk and LAA shape, Di Biase's research obtained considerable attention. Subsequent independent investigations conclusively established chicken wing as the “safe” LAA type (32, 33), and from that point to present, the consensus that chicken wing type LAA is least likely to result in stroke (i.e., is the “safe” LAA type) is widely accepted in clinical practice (34).
After recognition of chicken wing as the LAA shape with lowest risk, questions remained regarding high-risk LAA shapes, motivating a series of investigations to figure out the “unsafe” LAA types, which found that all the three non-chicken wing LAA shapes were “unsafe” (35–39). Specifically speaking, Lee et al. (39) conducted a case-control study on 255 patients based on the history of cardiovascular adverse events, and concluded that the cauliflower type is associated with an increased risk of stroke. Deng et al. (37) evaluated stroke risk via detection of spontaneous echo contrast (SEC) or thrombi using 3D transesophageal echocardiography (TOE) in 320 AF patients and found that the cauliflower LAA type was associated with a higher prevalence of SEC or thrombi. Adukauskaite et al. (35) found that windsock was the highest stroke-prone LAA type based on a stroke history assessment of 158 patients. Anselmino et al. (36) reached the conclusion that cauliflower and windsock are associated with a higher prevalence of silent cerebral ischemia based on a study of 348 AF patients. Kimura et al. (38) performed a case-control study investigating the high-risk LAA type in 80 AF patients with low CHADS2 score, and found cauliflower to be the most stroke-prone LAA type. Additionally, Smit et al. (40) developed a new 4-type classification scheme (chicken wing, swan, cauliflower and windsock) and evaluated stroke risk as a function of prior stroke history. Their results demonstrated that the swan LAA type (LAA presented a second sharp curve folding the structure back) was independently associated with the prior stroke history. Given the results of these studies, researchers subsequently combined the three “non-chicken wing” shapes into a single category and conducted studies to confirm an “unsafe” designation for risk of stroke on the “non-chicken wing” type (41–43). The simplification and efficiency of this two-type classification scheme is widely recognized in clinic (33, 34).
However, it is worth mentioning that several studies did not justify the origin of the emboli, as many studies considered that most strokes for AF patients are resulted from the cardioembolic origin. We think it is important to determine the embolic origin in future studies.
Detailed information on Wang's scheme of LAA shape types and the related studies on the association between stroke risk is summarized in Table 2.
Although Wang's scheme is widely accepted in clinical application, however, several studies have revealed marked variability in shape classification based on the scheme. Both Wu et al. (44) and Khurram et al. (45) pointed out the high inter-observer variability in the classification schemes based on Wang's shape description.
Table 3 illustrates the diversity in the prevalence among Wang LAA shape types observed by different investigators. Such disparity indicates that rigorous ascertainments based on these rather subjective descriptors is difficult and not reliably reproducible. The need for greater objectivity in defining LAA classification parameters enabling reliable assessment of associations with stroke risk led to the development of morphology parameters.
LAA morphology
The LAA morphology classification approach, with characteristics categorized by means of dimensional level, is superior to the LAA shape scheme in terms of objectivity and reproducibility and is widely studied.
Among these morphological characteristics, the LAA orifice (see Figure 2A) attracts a lot of focus, and a number of studies have revealed the correlation between orifice size and the stroke risk. In these studies, LAA orifice is consistently shown to be positively related to the stroke risk, both for its area (35, 46, 47) and diameter (41, 45, 48–50) (see Figure 2B). Although the positive relationship is widely accepted, the cut-off value of the orifice size for determining the high stroke risk is seldom calculated. Only Lee et al. (46, 47) gave the different criteria of >3.5 cm2 and >4 cm2 respectively in their two studies, with the criterion being larger for those with low CHA2DS2-VASc scores.
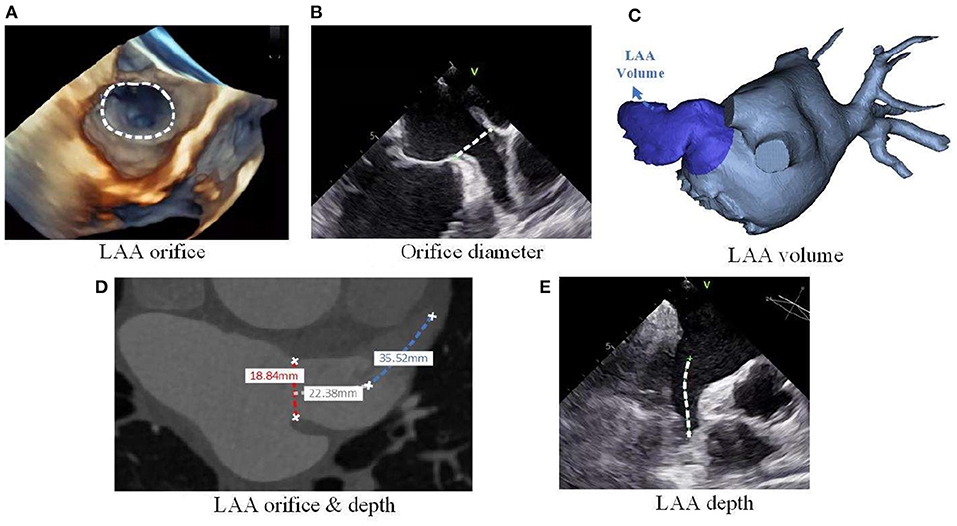
Figure 2. LAA morphological parameters. (A) LAA orifice measurement from 3D echo, (B) LAA orifice diameter measurement from planer echo, (C) LAA volume measurement from 3D reconstruction of CT images, (D) LAA orifice & depth measurement from planer CT images, and (E) LAA depth measurement from planar echocardiogram.
Though the large orifice is believed to relate to the high stroke risk, Khurram et al. (45) draw a contrary conclusion that a smaller LAA orifice diameter is associated with prevalent stroke. In seeking for the reasons for such different conclusions regarding the association between LAA orifice and stroke risk, we found that these studies used different definitions of LAA orifice (Figure 3).
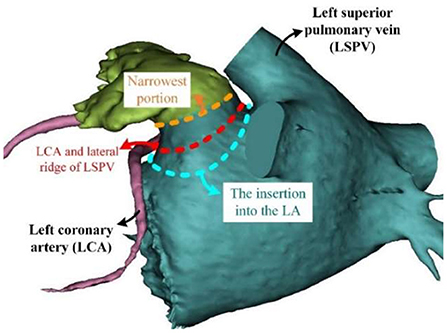
Figure 3. Different definition of LAA orifice. LA, left atrial; LAA, left atrial appendage; LCA, left coronary artery; LSPV, left superior pulmonary vein.
Intuitively speaking, the LAA orifice would be the section at the interface between the LAA and LA (Figure 3, blue dashed line). This intuitive definition was used by Khurram et al. (45). However, an anatomical definition of the LAA orifice exists, and it is usually specified as the section aligning with the left upper pulmonary vein ridge and the circumflex coronary (51) (Figure 3, red dashed line). This seems more accurate and less subjective compared to the intuitive definition, and is used as the measurement method for LAA orifice in (52). However, several studies have defined the LAA orifice as the narrowest portion at the entrance into the left atrium (49, 53). We deduced that this wide-spread definition had its origins in studies of LAA occlusion, as the location of occlusion is usually defined as the narrowest portion (Figure 3, orange dashed line), which would be defined as the LAA neck in our context. The differing definitions caused the diversity of conclusions. In this regard, a clear and unified definition of LAA orifice would be required in the future studies.
Apart from LAA orifice, LAA volume (see Figure 2C) is another prominent parameter. Several studies on the associations between LAA volume and stroke risk have shown that AF patients with a history of stroke/TIA tend to exhibit a larger LAA volume (48, 49, 54–57). However, the specific cardiac phase for defining the LAA volume was not clearly defined in most of the above-mentioned studies. Only Chen et al. (55) clearly stated their LAA volume was at the end-diastolic phase, and the results showed that LAA end-diastolic volume was positively associated with the stroke risk. Although consensus has been achieved regarding a qualitative relationship between the LAA volume and stroke risk, a cut-off value or quantitative relationship was not defined in most of the related studies. Chen et al. (55) and Burrell et al. (54) proposed cut-off values of 8.6 and 34 cm3, respectively, for judging stroke risk, although the two values reflect a large disparity which could be attributed to differences in cardiac cycle phase. In this regard, the specific cardiac cycle phase of LAA volume should be defined clearly in future studies, which will allow a standardized comparison among different studies. However, it is worth to mention that the LA will be dilated with the impact form AF, and so the volume of LAA will also be enlarged. Meanwhile, these morphology characteristics would be better compared with the normalizations to the other patient specific characteristics [e.g.,: body mass index (BMI), body surface area (BSA)], so that these morphology characteristics could be compared between different patients.
Different from the focus on LAA volume for a specific cardiac phase, Al-Issa et al. (58) focused on the changes in LAA volume during the whole cardiac cycle. In their study, the LAA area change ratio (known as regional function) was measured by CT and used to denote the volume change, and the result showed this parameter was significantly associated with stroke risk history, where stroke/TIA group tended to have a lower area change ratio compared with the control group.
In addition to the above-mentioned characteristics, some other characteristics were also studied, such as the angle of LAA bend (59), the depth (48, 53) (see Figures 2D,E) and the number of lobes (52, 60). In these studies, the acute angle, increased depth and large number of lobes was each considered as a high-risk morphology of LAA. All these morphological characteristics suggest that the stroke risk is increased with the increasing of LAA morphology complexity. Detailed information on the associations between stroke risk and LAA morphological parameters are summarized in Table 4.
To better quantify this complexity, Kaminski et al. (62) used different parameters to describe the whole LAA. In their study, different parametric schemes with various parameters were used to describe the different LAA types. In addition to Bieging et al. (63), where they assessed the LAA shape using statistical shape analysis on the “surface” of the LAA, Sanatkhani et al. (64) also performed an analysis to parametrize the LAA region's appearance based on principal components and eigen shapes. And also, Slipsager et al. (65) conducted the unsupervised clustering based on the LAA shapes, which result into two clusters: chicken wing and non-chicken wing shapes.
Although the morphology parameters are considered to be an objective approach for characterizing the LAA, the morphology parameter features are inherently isolated from the complex LAA geometry, and it lacks a comprehensive and mechanistic prospective for stroke evaluation. Under this circumstance, the hemodynamic analysis attracted the attention, for that the hemodynamic description utilizes the entire 3D dataset and is more comprehensive compared to the single or several morphological parameters. Furthermore, the study of hemodynamics is aligned with Virchow's triad used to characterize the relationship between blood flow and thrombus formation. In this regard, evaluating the stroke risk from the aspect of hemodynamics in the LAA may be an optimal approach for evaluation of stroke risk.
LAA hemodynamics
LAA flow velocity accounts for the majority of studies on the hemodynamic characteristics. Verhorst et al. (66) firstly evaluated the stroke risk using peak LAA velocity (both emptying and filling) and concluded that peak LAA velocity may potentially identify patients at high risk for systemic embolism. In subsequent studies, both peak LAA filling and emptying velocity were found to exhibit significant difference between the stroke and non-stroke patient groups (50). In a series of related studies, the peak LAA emptying velocity (pLAAev) was then used to quantify LAA flow velocity, revealing a correlation between a lower pLAAev and a higher stroke risk (32, 39, 67–69). However, the cut-off values for pLAAev were neglected in most studies, with only a few studies calculating this value. Generally speaking, the velocity of 20 cm/s is widely accepted as the threshold for determining the stroke risk (70–72). Chen et al. (73) concluded a slightly higher cut-off value of 21.5 cm/s based on study of 307 AF patients. However, Lee et al. (47) found that 37 cm/s could be used as a prognostic threshold for stroke risk in AF patients, with a higher value of 40 cm/s in patients with low CHA2DS2-VASc scores (46). The large difference for the aforementioned values (~20 vs. ~40 cm/s) may attribute to the different placement of sample volume in the TEE measurement. In Lee et al.'s (46, 47) measurements, the sample volume was placed at the middle portion of the LAA, while the other measurements placed 1–2 cm inside of LAA.
In addition to LAA flow velocity, other hemodynamic parameters such as flow pattern and LAA ejection fraction (LAAef) have also been studied. For LAA flow pattern, Garciafernandez et al. (74) identified three different flow patterns and studied their association with stroke risk. Their results revealed that the flow pattern characterized by the absence of identifiable flow waves was associated with a higher prevalence of LAA spontaneous contrast (higher stroke risk). Regarding LAAef, consensus was reached that the LAAef is negatively related with the stroke risk (75, 76). Specifically speaking, Park et al. (76) retrospectively studied 176 paroxysmal AF patients, and the result revealed that LAAef was significantly lower in the stroke group compared with the non-stroke group. LAA emptying fraction assessed by the feature-tracking echocardiographic method was put forward by Iwama et al. (75) who found that LAA emptying fraction was significantly reduced in AF patients with thrombus compared to those without thrombus. However, the cut-off values for LAAef were not determined in the studies, which should be considered in the future research. Studies on association of stroke risk and LAA hemodynamic parameters are summarized in Table 5.
Although evaluation of stroke risk by means of LAA hemodynamic parameters is particularly convincing, the availability of direct clinical measurements of these parameters is often limited. Alternatively, computational fluid dynamics (CFD) method has been used to obtain these parameters. CFD is a numerical analysis approach for predicting the behavior of fluid flows whereby the hemodynamic details of LA and LAA can be obtained and visualized. Utilizing the CFD method, Gracia-Isla et al. (77) not only obtained the velocity profile located at the LAA orifice, but also calculated the other hemodynamic descriptors such as time-averaged wall shear stress (TAWSS), oscillatory shear index (OSI), endothelial cell activation potential (ECAP), relative residence time (RRT), and vortex structures in four different LAA models.
Based on CFD, some other studies applied more intuitive and quantitative methods in evaluating thrombus formation. Bosi et al. (78) used contrast dye to simulate blood stasis in the LAA and then evaluated the risk of thrombus formation in the LAA (Figure 4A). Additionally, Masci et al. (79, 80) and Fang et al. (81) adopted a fluid particle distribution simulation in analyzing thrombus formation risk (Figure 4B). Similarly, Sanatkhani et al. put forward a mean residence time of blood-borne particles (82) and asymptotic concentration remaining (83) inside LAA for evaluating thrombus formation. In a further study, Yan et al. (84) applied a numerical thrombus model presented by Menichini et al. (85) to predict thrombus formation risk. This thrombus model included not only the flow characteristics, but also the biomedical characteristics of platelets and coagulation.
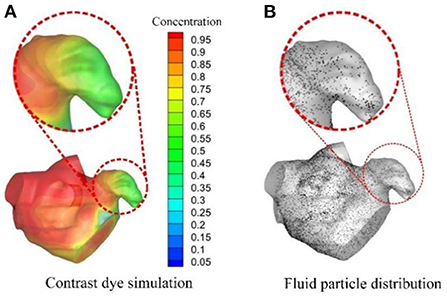
Figure 4. CFD analysis for evaluating thromboembolic risk in LAA. (A) Contrast dye simulations and (B) Fluid particle distributions.
In addition to studies assumed the boundary walls to be rigid to simulate the idealized AF conditions (77, 78, 86–88). Different ways were applied to realize the wall motion, several studies applied the immersed boundary analysis to fulfill the fluid-structure interactions, where the walls were moved with the impact from the fluid flows (89–91). Followingly, with the lack of knowledge in the material and mechanical properties for heart walls, several other studies used a four-dimensional displacement vector field directly generated from the medical images, and the displacement filed was then applied to the LA structure to move the walls (80, 92–94). Meanwhile, with the lack in the dynamic medical images, several other studies also fulfill the wall motion with the displacements generated form literature (95, 96). To better compare the difference between the rigid walls and moving walls simulation, Duenas-Pamplona et al. (97) performed a comprehensive comparation between the two type simulations, and they found that the rigid wall model can lead to a poor approximation in some cases.
Although several hemodynamic parameters proposed by the CFD models rely on numerical simulations to evaluate thrombus formation risk. further research is required to validate their potential application in stroke risk evaluation in patients.
Discussion
Intra-group relationship
As illustrated in the former section, shape, morphology and hemodynamic parameters of the LAA are all validated to be highly associated with the risk of stroke. Several studies have pointed out the subjectivity of the shape classifications and the advantages of morphology and hemodynamic descriptors as objective classifiers, which have led to studies of relationships among these parameters. A number of these studies have established that the non-chicken wing type LAA is usually associated with a larger LAA orifice (32, 33) and higher lobe count (60). Studies on the relationships between shape and hemodynamic parameters revealed that peak LAA flow velocity exhibited significant difference among LAA shapes (72, 78) with a low LAA flow velocity associated with the non-chicken wing LAA type (98) and vice versa (33, 99). In addition, several other studies have identified the relationships between the hemodynamic and morphological parameters. In a multi-linear regression analysis, LAA morphology was found to be the significant determinant of LAA flow velocity (99). In other studies, flow velocity was shown to be negatively correlated with orifice size, and many studies found that a greater orifice size usually resulted in decreased flow velocity (32, 47, 53). CFD was also applied in studies relevant to morphology parameters in which LAA length, orifice area, tortuosity, etc. were studied for their relationship with hemodynamic parameters. Masci et al. (79) concluded that even qualitatively simple LAA morphology could lead to a high probability of thrombus formation. The summarized intra-group relationships are illustrated in the Figure 5.
The relationships among the shape, morphology and hemodynamic parameters highlight the importance of LAA in stroke evaluation, which motivates further studies to find and evaluate the key LAA features.
Imaging modalities
As shown in the Tables 2, 4, 5, different imaging modalities were used for the evaluation of LAA shape, morphology and hemodynamic parameters, namely the TEE, cardiac CT and MRI modalities.
TEE
Compared with other imaging modalities, echocardiography can obtain the image at low cost and with high efficiency, so it is a widely used method in clinics. During the imaging procedure, the probe of TEE is close to the heart with high frequency, and therefore TEE can be used not only to assess the LAA morphology, but also to screen out the thrombus and spontaneous echo contrast (SEC) in the LA/LAA (100), and it is considered as the gold standard modality for detecting the LAA thrombi (100, 101). Additionally, TEE is superior in time resolution, and so it can obtain the LAA hemodynamic parameters (e.g.,: LAA flow profile) within the continuous cardiac cycles (102). Beside the above-mentioned applications, TEE is also applied in the guidance and evaluation during the LAA occlusion operation in most centers, for that TEE allows the real-time imaging, without ionizing radiation or contrast medium. Under this circumstance, TEE is considered as the conventional gold-standard imaging modality for LAA occlusion (103). However, TEE may not be applicable for some cases and it is considered to be a semi-invasive test that carries a small risk to the patient, although very rare, some complications can be life threatening (104, 105).
All in all, TEE is widely applied in the clinical practice related with LAA with its efficient, clear and low-cost imaging procedure. And this imaging modality with high time-resolution is applicable in the shape, morphology and especially the hemodynamic evaluations for the LAA.
Cardiac CT
Compared with TEE, cardiac CT offers superior spatial resolution. Its high-quality multi-planar and 3D reconstruction would do much help in the characterization of the LAA anatomy and accurate sizing (31). Therefore, CT has been considered as the gold standard for visualizing the LAA (106, 107), and it is widely applied in the evaluation of shape and morphology parameters. Meanwhile, some studies have also considered cardiac CT with a delayed imaging as a useful modality for the detection of LAA thrombi (108), and therefore it is routinely performed to screen the LA/LAA thrombi before the AF ablation procedure in clinics. However, compared to TEE, CT imaging modality is limited in the time resolution, it cannot get the real-time visualization of the hemodynamics and LAA structure with the cardiac cycle. To strike the deficiency in the time resolution, it is worth mentioning a new CT-based method proposed by Morelos-Guzman (109), which can calculate the flow velocity with the scans. It is a novel method that can expend the CT application to the hemodynamic parameter evaluation.
In general, cardiac CT is limited in the time resolution, but its high spatial resolution contributes to a wide application in the shape and morphology evaluation for LAA.
Cardiac MRI
Cardiac MRI is a noninvasive imaging modality for the structure and hemodynamic evaluation. It has excellent tissue characterization capacity but a lower spatial resolution when compared to the cardiac CT (110). Meanwhile, it is often used to delineate the anatomy of the left atrium but conferring no ionizing radiation to patients. Additionally, MRI can measure the LAA flow velocity and shows a good correlation with TEE (111), and MRI shows the good sensitivity and specificity in evaluating the LAA thrombi (110), thus, it has the potential to be a useful imaging modality for the detection of LAA thrombi. However, similar with CT, the real-time visualization of LAA is not permitted and it is not applicable for patients with pacemakers.
Generally speaking, though the MRI modality is noninvasive and can obtain the shape, morphology and hemodynamic parameters of the LAA, the present number of its application scenarios in LAA are small, most for the patients unsuitable for TEE and CT (110). Its potential capabilities in LAA related evaluation will be strongly stimulated with the high-resolution MRI in the future applications.
Perspectives
Stroke prevention is the cornerstone of AF management and the anticoagulation decision is currently based on the CHA2DS2-VASc score scheme. This scheme first became part of guideline medical therapy as part of the 2012 European Society of Cardiology updated guidelines (112) as well as the 2014 American Heart Association/American College of Cardiology/Heart Rhythm Society (AHA/ACC/HRS) guideline for the management of patients with AF (113). Both the European and US guidelines specifically recommend risk stratification with the CHA2DS2-VASc score, and delineate that anticoagulation may be considered for males with a score of 1 and females with a score of 2 (113, 114). However, CHA2DS2-VASc score does not take into account paroxysmal vs. non-paroxysmal AF as well as duration of paroxysmal AF episodes. Even patients with low CHA2DS2-VASc score may suffer a stroke. The score also fails to take into consideration the structure (31, 115) and the hemodynamic characteristics for the LAA (37, 116, 117) in addition to other clinical markers (118–120). Thus, additional LAA features and clinical markers may further risk stratify stroke prevention for patients with AF. These two additional predictors may improve the sensitivity and specificity of LAA-related stroke risk.
Meanwhile, with the requirement of large data bank processing, several potential tools (or algorithms) could be utilized for the LAA related studies:
Computer aided pre-processing
The pre-processing of image segmentation and reconstruction are the foundation for shape and morphology evaluation, while the manual segmentation and reconstruction for a patient-specific data is time-consuming. In this regard, some auto or semi-auto segmentation and reconstruction algorithms were put forward (121–124) to improve the efficiency. Besides the study for the efficient segmentation and reconstruction algorithms, there is also a need for the image-based measurement algorithms. With the measurement algorithms, not only the time of the measurement can be greatly reduced, but also the reproducibility will be improved. In this regard, studies that focus on the auto or semi-auto measurement, like Leventic et al.'s (125) work, should be encouraged in future studies. Clinical studies are also needed to validate the developed algorithms.
CFD analysis
Blood stasis is one of the three conditions in Virchow's triad, the study of blood stasis requires a comprehensive characterization of the local hemodynamics, and the clinical measurement of echocardiography is currently the main tool to explore the blood flow patterns and velocity profiles. While the echocardiography cannot fully characterize the complexity of 4D blood flow patterns, new imaging techniques like 4D flow MRI or blood speckle tracking are promising but their capability to capture the low flow velocities related to thrombus formation is still unclear. As an alternative, CFD poses a great potential to thoroughly assess the hemodynamics inside the LA/LAA, and several studies have been performed to analyze the hemodynamic parameters in the LAA in relation to thrombogenic risk (72, 80–82, 84).
However, these CFD analysis uses various settings for boundary conditions, LA wall behavior, mesh resolution and etc., and different modeling strategies were used. It has to admit that the optimal strategy is difficult to identify due to the absence of joint benchmark studies with reliable ground truth (126). Meanwhile, there is no consensus on the most appropriate metrics for the quantification of the CFD result. Therefore, the consensus for the appropriate metrics needed to be reached in the future studies. Based on the consensus, the modeling pipelines would be proposed for improving the capacity to process the increased number specific cases. Moreover, these CFD analysis should be validated with reliable ground truth to form the benchmark studies for optimal strategy in future work, and Duenas-Pamplona et al. (127) proposed an in-vitro flow equipment to validate there CFD models. Finally, it is worth to mention the work performed by Morales et al. (96), where they performed a deep learning surrogate models of CFD analysis for thrombus formation risk evaluation in the LAA.
Conclusion
Stroke prevention remains a major goal in patients in AF. Significant evidence indicates that the assessment of LAA structural and hemodynamic parameters have meaningful implications for the stroke risk evaluation. Future work is needed to understand the role of LAA structural (shape and morphology) and hemodynamic parameters on stratification of stroke risk, especially in patients with low CHA2DS2-VASc scores. Computer-aided tools will become instrumental in the stroke evaluation with these LAA-related parameters.
Author contributions
ZL and LZ designed and supervised the study. RF and YL reviewed the literature and wrote the original manuscript. JW and ZW helped with the literature search. JA and CC modified the article. All authors contributed to the article and approved the submitted version.
Funding
This work is partially supported by the National Natural Science Foundation of China (11972118, 61821002, 12172089), Postgraduate Research & Practice Innovation Program of Jiangsu Province (No.SJCX20_0012) and the Fundamental Research Funds for the Central Universities (3207032103D).
Conflict of interest
The authors declare that the research was conducted in the absence of any commercial or financial relationships that could be construed as a potential conflict of interest.
Publisher's note
All claims expressed in this article are solely those of the authors and do not necessarily represent those of their affiliated organizations, or those of the publisher, the editors and the reviewers. Any product that may be evaluated in this article, or claim that may be made by its manufacturer, is not guaranteed or endorsed by the publisher.
References
1. Go AS, Hylek EM, Phillips KA, Chang YC, Henault LE, Selby JV, et al. Prevalence of diagnosed atrial fibrillation in adults - National implications for rhythm management and stroke prevention: the Anticoagulation and Risk Factors in Atrial Fibrillation (ATRIA) study. JAMA. (2001) 285:2370–5. doi: 10.1001/jama.285.18.2370
2. Andrade J, Khairy P, Dobrev D, Nattel S. The clinical profile and pathophysiology of atrial fibrillation relationships among clinical features, epidemiology, and mechanisms. Circ Res. (2014) 114:1453–68. doi: 10.1161/CIRCRESAHA.114.303211
3. Camm AJ, Kirchhof P, Lip GYH, Schotten U, Savelieva IS, et al. Guidelines for the management of atrial fibrillation The Task Force for the Management of Atrial Fibrillation of the European Society of Cardiology (ESC). Eur Heart J. (2010) 31:2369–429. doi: 10.1093/eurheartj/ehq278
4. McManus DD, Rienstra M, Benjamin EJ. An update on the prognosis of patients with atrial fibrillation. Circulation. (2012) 126:E143–6. doi: 10.1161/CIRCULATIONAHA.112.129759
5. January CT, Wann LS, Alpert JS, Calkins H, Cigarroa JE, Cleveland JC, et al. 2014 AHA/ACC/HRS guideline for the management of patients with atrial fibrillation: executive summary. J Am Coll Cardiol. (2014) 64:2246–80. doi: 10.1016/j.jacc.2014.03.021
6. Virani SS, Alonso A, Benjamin EJ, Bittencourt MS, Callaway CW, Carson AP, et al. Heart Disease and Stroke Statistics-2020 Update: A Report From the American Heart Association. Circulation. (2020) 141:E139–E596. doi: 10.1161/CIR.0000000000000746
7. Pistoia F, Sacco S, Tiseo C, Degan D, Ornello R, Carolei A. The epidemiology of atrial fibrillation and stroke. Cardiol Clin. (2016) 34:255–63. doi: 10.1016/j.ccl.2015.12.002
8. Wilke T, Groth A, Mueller S, Pfannkuche M, Verheyen F, Linder R, et al. Incidence and prevalence of atrial fibrillation: an analysis based on 8.3 million patients. Europace. (2013) 15:486–93. doi: 10.1093/europace/eus333
9. Morin DP, Bernard ML, Madias C, Rogers PA, Thihalolipavan S, Estes NAM. The State of the Art: Atrial Fibrillation Epidemiology, Prevention, and Treatment. Mayo Clin Proc. (2016) 91:1778–810. doi: 10.1016/j.mayocp.2016.08.022
10. Staerk L, Sherer JA, Ko D, Benjamin EJ, Helm RH. Atrial fibrillation epidemiology, pathophysiology, and clinical outcomes. CircRes. (2017) 120:1501–17. doi: 10.1161/CIRCRESAHA.117.309732
11. Bjorck S, Palaszewski B, Friberg L, Bergfeldt L. Atrial fibrillation, stroke risk, and warfarin therapy revisited a population-based study. Stroke. (2013) 44:3103–8. doi: 10.1161/STROKEAHA.113.002329
12. Naccarelli GV, Varker H, Lin J, Schulman KL. Increasing prevalence of atrial fibrillation and flutter in the United States. Am J Cardiol. (2009) 104:1534–9. doi: 10.1016/j.amjcard.2009.07.022
13. Hart RG, Pearce LA, Halperin JL, Hylek EM, Albers GW, Anderson DC, et al. Comparison of 12 risk stratification schemes to predict stroke in patients with nonvalvular atrial fibrillation. Stroke. (2008) 39:1901–10. doi: 10.1161/STROKEAHA.107.501825
14. Ganjehei L, Massumi A, Razavi M, Rasekh A. Stroke prevention in nonvalvular atrial fibrillation. Texas Heart Inst J. (2011) 38:350–2.
15. Petersen P. Thromboembolic complications in atrial-fibrillation. Stroke. (1990) 21:4–13. doi: 10.1161/01.STR.21.1.4
16. Wolf PA, Abbott RD, Kannel WB. Atrial-fibrillation as an independent risk factor for stroke - the framingham-study. Stroke. (1991) 22:983–8. doi: 10.1161/01.STR.22.8.983
17. Lip GYH. Stroke and bleeding risk assessment in atrial fibrillation: when, how, and why? Eur Heart J. (2013) 34:1041–9. doi: 10.1093/eurheartj/ehs435
18. Okuyama H, Hirono O, Liu L, Takeishi Y, Kayama T, Kubota I. Higher levels of serum fibrin-monomer reflect hypercoagulable state and thrombus formation in the left atrial appendage in patients with acute ischemic stroke. Circ J. (2006) 70:971–6. doi: 10.1253/circj.70.971
19. Yaghi S, Song C, Gray WA, Furie KL, Elkind MS, Kamel H. Left atrial appendage function and stroke risk. Stroke. (2015) 46:3554–9. doi: 10.1161/STROKEAHA.115.011273
20. Beigel R, Wunderlich NC, Ho SY, Arsanjani R, Siegel RJ. The left atrial appendage: anatomy, function, and noninvasive evaluation. JACC Cardiovasc Imaging. (2014) 7:1251–65. doi: 10.1016/j.jcmg.2014.08.009
21. Sharma S, Devine W, Anderson RH, Zuberbuhler JR. The determination of atrial arrangement by examination of appendage morphology in 1842 heart specimens. Br Heart J. (1988) 60:227–31. doi: 10.1136/hrt.60.3.227
22. Ucerler H, Ikiz ZAA, Ozgur T. Human left atrial appendage anatomy and overview of its clinical significance. Anat J Cardiol. (2013) 13:566–72. doi: 10.5152/akd.2013.181
23. Reddy VY, Sievert H, Halperin J, Doshi SK, Buchbinder M, Neuzil P, et al. Percutaneous left atrial appendage closure vs warfarin for atrial fibrillation a randomized clinical trial. JAMA. (2014) 312:1988–98. doi: 10.1001/jama.2014.15192
24. Tuccillo B, Stumper O, Hess J, Vansuijlen RJ, Bos E, Roelandt J, et al. Transesophageal echocardiographic evaluation of atrial morphology in children with congenital heart-disease. Eur Heart J. (1992) 13:223–31. doi: 10.1093/oxfordjournals.eurheartj.a060151
25. Boyle PM, del Alamo JC, Akoum N. Fibrosis, atrial fibrillation and stroke: clinical updates and emerging mechanistic models. Heart. (2021) 107:99–105. doi: 10.1136/heartjnl-2020-317455
26. Wang Y, Biase LDi, Horton RP, Nguyen T, Morhanty P, Natale A. Left atrial appendage studied by computed tomography to help planning for appendage closure device placement. J Cardiovasc Electrophysiol. (2010) 21:973–82. doi: 10.1111/j.1540-8167.2010.01814.x
27. Koplay M, Erol C, Paksoy Y, Kivrak AS, Ozbek S. An investigation of the anatomical variations of left atrial appendage by multidetector computed tomographic coronary angiography. Eur J Radiol. (2012) 81:1575–80. doi: 10.1016/j.ejrad.2011.04.060
28. Shi AW, Chen ML, Yang B, Cao KJ, Kong XQ. A morphological study of the left atrial appendage in chinese patients with atrial fibrillation. J Int Med Res. (2012) 40:1560–7. doi: 10.1177/147323001204000436
29. Beutler DS, Gerkin RD, Loli AI. The morphology of left atrial appendage lobes: a novel characteristic naming scheme derived through three-dimensional cardiac computed tomography. World J Cardiovasc Surg. (2014) 4:17–24. doi: 10.4236/wjcs.2014.43004
30. Jun W, Yi X, Xiaomei Z, Xin T, Weiwei H, Haibin S. The value of left atrial parameters measured on cardiac CT in predicting stroke in patients with atrial fibrillation (in chinese). Nat Sci. (2019) 39:136–40. doi: 10.7655/NYDXBNS20190128
31. Biase LDi, Santangeli P, Anselmino M, Mohanty P, Salvetti I, Gili S, et al. Does the left atrial appendage morphology correlate with the risk of stroke in patients with atrial fibrillation? Results from a multicenter study. J Am Coll Cardiol. (2012) 60:531–8. doi: 10.1016/j.jacc.2012.04.032
32. Lee JM, Seo J, Uhm JS, Kim YJ, Lee HJ, Kim JY, et al. Why is left atrial appendage morphology related to strokes? An analysis of the flow velocity and orifice size of the left atrial appendage. J Cardiovasc Electrophysiol. (2015) 26:922–7. doi: 10.1111/jce.12710
33. Lupercio F, Ruiz JC, Briceno DF, Romero J, Villablanca PA, Berardi C, et al. Left atrial appendage morphology assessment for risk stratification of embolic stroke in patients with atrial fibrillation: a meta-analysis. Heart Rhythm. (2016) 13:1402–9. doi: 10.1016/j.hrthm.2016.03.042
34. Basu-Ray I, Sudhakar D, Schwing G, Monlezun D, Zhang L, Shah SK, et al. Complex left atrial appendage morphology is an independent risk factor for cryptogenic ischemic stroke. Front Cardiovasc Med. (2018) 5:8. doi: 10.3389/fcvm.2018.00131
35. Adukauskaite A, Barbieri F, Senoner T, Plank F, Knoflach M, Boehme C, et al. Left atrial appendage morphology and left atrial wall thickness are associated with cardio-embolic stroke. J Clin Med. (2020) 9:9. doi: 10.3390/jcm9123944
36. Anselmino M, Scaglione M, Biase LD, Gili S, Santangeli P, Corsinovi L, et al. Left atrial appendage morphology and silent cerebral ischemia in patients with atrial fibrillation. Heart Rhythm. (2014) 11:2–7. doi: 10.1016/j.hrthm.2013.10.020
37. Deng BQ, Nie RQ, Qiu Q, Wei YL, Liu YM, Lv HL, et al. 3D transesophageal echocardiography assists in evaluating the morphology, function, and presence of thrombi of left atrial appendage in patients with atrial fibrillation. Ann Transl Med. (2021) 9:13. doi: 10.21037/atm-21-1981
38. Kimura T, Takatsuki S, Inagawa K, Katsumata Y, Nishiyama T, Nishiyama N, et al. Anatomical characteristics of the left atrial appendage in cardiogenic stroke with low CHADS2 scores. Heart Rhythm. (2013) 10:921–5. doi: 10.1016/j.hrthm.2013.01.036
39. Lee Y, Park HC, Lee Y, Kim SG. Comparison of morphologic features and flow velocity of the left atrial appendage among patients with atrial fibrillation alone, transient ischemic attack, and cardioembolic stroke. Am J Cardiol. (2017) 119:1596–604. doi: 10.1016/j.amjcard.2017.02.016
40. Smit JM, Simon J, El Mahdiui M, Szaraz L, van Rosendael PJ, Kolassvary M, et al. Anatomical characteristics of the left atrium and left atrial appendage in relation to the risk of stroke in patients with vs. without atrial fibrillation. Circ Arrhythm Electrophysiol. (2021) 14:10. doi: 10.1161/CIRCEP.121.009777
41. Kong B, Liu Y, Hu H, Wang L, Fan Y, Mei Y, et al. Left atrial appendage morphology in patients with atrial fibrillation in China: implications for stroke risk assessment from a single center study. Chin Med J. (2014) 127:4210–4. doi: 10.3760/cma.j.issn.0366-6999.20141520
42. Du H, Bi K, Xu LS, Chen F, Xiong WF, Wang Y. Analysis of risk factors for thrombosis of the left atrium/left atrial appendage in patients with non-valvular atrial fibrillation. Cardiovasc J Afr. (2021) 32:116–22. doi: 10.5830/CVJA-2019-071
43. Yaghi S, Chang AD, Hung P, Mac Grory B, Collins S, Gupta A, et al. Left atrial appendage morphology and embolic stroke of undetermined source: a cross-sectional multicenter pilot study. J Stroke Cerebrovasc Dis. (2018) 27:1497–501. doi: 10.1016/j.jstrokecerebrovasdis.2017.12.036
44. Wu LM, Liang EP, Fan SY, Zheng LH, Du ZP, Liu SY, et al. Relation of left atrial appendage morphology determined by computed tomography to prior stroke or to increased risk of stroke in patients with atrial fibrillation. Am J Cardiol. (2019) 123:1283–6. doi: 10.1016/j.amjcard.2019.01.024
45. Khurram IM, Dewire J, Mager M, Maqbool F, Zimmerman SL, Zipunnikov V, et al. Relationship between left atrial appendage morphology and stroke in patients with atrial fibrillation. Heart Rhythm. (2013) 10:1843–9. doi: 10.1016/j.hrthm.2013.09.065
46. Lee JM, Kim JB, Uhm JS, Pak HN, Lee MH, Joung B. Additional value of left atrial appendage geometry and hemodynamics when considering anticoagulation strategy in patients with atrial fibrillation with low CHA(2)DS(2)-VASc scores. Heart Rhythm. (2017) 14:1297–301. doi: 10.1016/j.hrthm.2017.05.034
47. Lee JM, Shim J, Uhm JS, Kim YJ, Lee HJ, Pak HN, et al. Impact of increased orifice size and decreased flow velocity of left atrial appendage on stroke in nonvalvular atrial fibrillation. Am J Cardiol. (2014) 113:963–9. doi: 10.1016/j.amjcard.2013.11.058
48. Beinart R, Heist EK, Newell JB, Holmvang G, Ruskin JN, Mansour M. Left atrial appendage dimensions predict the risk of stroke/TIA in patients with atrial fibrillation. J Cardiovasc Electrophysiol. (2011) 22:10–5. doi: 10.1111/j.1540-8167.2010.01854.x
49. Jeong WK, Choi JH, Son JP, Lee S, Lee MJ, Choe YH, et al. Volume and morphology of left atrial appendage as determinants of stroke subtype in patients with atrial fibrillation. Heart Rhythm. (2016) 13:820–7. doi: 10.1016/j.hrthm.2015.12.026
50. Sakr SA, El-Rasheedy WA, Ramadan MM, El-Menshawy I, Mahfouz E, Bayoumi M. Association between left atrial appendage morphology evaluated by trans-esophageal echocardiography and ischemic cerebral stroke in patients with atrial fibrillation. Int Heart J. (2015) 56:329–34. doi: 10.1536/ihj.14-374
51. Korsholm K, Berti S, Iriart X, Saw J, Wang DD, Cochet H, et al. Expert recommendations on cardiac computed tomography for planning transcatheter left atrial appendage occlusion. JACC. (2020) 13:277–92. doi: 10.1016/j.jcin.2019.08.054
52. Yamamoto M, Seo Y, Kawamatsu N, Sato K, Sugano A, Machino-Ohtsuka T, et al. Complex left atrial appendage morphology and left atrial appendage thrombus formation in patients with atrial fibrillation. Circ Cardiovasc Imaging. (2014) 7:337–43. doi: 10.1161/CIRCIMAGING.113.001317
53. Chen L, Xu CJ, Chen WS, Zhang CQ. Left atrial appendage orifice area and morphology is closely associated with flow velocity in patients with nonvalvular atrial fibrillation. BMC Cardiovasc Disord. (2021) 21:13. doi: 10.1186/s12872-021-02242-9
54. Burrell LD, Horne BD, Anderson JL, Muhlestein JB, Whisenant BK. Usefulness of left atrial appendage volume as a predictor of embolic stroke in patients with atrial fibrillation. Am J Cardiol. (2013) 112:1148–52. doi: 10.1016/j.amjcard.2013.05.062
55. Chen ZX, Bai WJ, Li C, Wang H, Tang H, Qin YP, et al. Left atrial appendage parameters assessed by real-time three dimensional transesophageal echocardiography predict thromboembolic risk in patients with nonvalvular atrial fibrillation. J Ultrasound Med. (2017) 36:1119–28. doi: 10.7863/ultra.16.05070
56. Korhonen M, Muuronen A, Arponen O, Mustonen P, Hedman M, Jakala P, et al. Left atrial appendage morphology in patients with suspected cardiogenic stroke without known atrial fibrillation. PLoS ONE. (2015) 10:12. doi: 10.1371/journal.pone.0118822
57. Taina M, Vanninen R, Hedman M, Jakala P, Karkkainen S, Tapiola T, et al. Left atrial appendage volume increased in more than half of patients with cryptogenic stroke. PLoS ONE. (2013) 8:8. doi: 10.1371/journal.pone.0079519
58. Al-Issa A, Inoue Y, Cammin J, Tang Q, Nazarian S, Calkins H, et al. Regional function analysis of left atrial appendage using motion estimation CT and risk of stroke in patients with atrial fibrillation. Eur Heart J Cardiovasc Imaging. (2016) 17:788–96. doi: 10.1093/ehjci/jev207
59. Yaghi S, Chang AR, Ignacio G, Scher E, Panda N, Chu AT, et al. Left atrial appendage morphology improves prediction of stagnant flow and stroke risk in atrial fibrillation. Circ Arrhythm Electrophysiol. (2020) 13:3. doi: 10.1161/CIRCEP.119.008074
60. Wang F, Zhu MY, Wang XY, Zhang W, Su Y, Lu YY, et al. Predictive value of left atrial appendage lobes on left atrial thrombus or spontaneous echo contrast in patients with non-valvular atrial fibrillation. BMC Cardiovasc Disord. (2018) 18:11. doi: 10.1186/s12872-018-0889-y
61. Dudzińska-Szczerba K, Michałowska I, Piotrowski R, Sikorska A, Paszkowska A, Stachnio U, et al. Assessment of the left atrial appendage morphology in patients after ischemic stroke — The ASSAM study. Int J Cardiol. (2021) 330:65–72. doi: 10.1016/j.ijcard.2021.01.001
62. Kaminski R, Kosinski A, Brala M, Piwko G, Lewicka E, Dabrowska-Kugacka A, et al. Variability of the left atrial appendage in human hearts. PLoS ONE. (2015) 10:9. doi: 10.1371/journal.pone.0141901
63. Bieging ET, Morris A, Chang L, Dagher L, Marrouche NF, Cates J. Statistical shape analysis of the left atrial appendage predicts stroke in atrial fibrillation. Int J Cardiovasc Imaging. (2021) 37:2521–7. doi: 10.1007/s10554-021-02262-8
64. Sanatkhani S, Menon PG. Generative statistical modeling of left atrial appendage appearance to substantiate clinical paradigms for stroke risk stratification. In: Conference on Medical Imaging - Image Processing. Houston, TX. (2018).
65. Slipsager JM, Juhl KA, Sigvardsen PE, Kofoed KF, De Backer O, Olivares AL, et al. Statistical shape clustering of left atrial appendages. In: Pop M, Sermesant M, Zhao J, Li S, McLeod K, Young A, et al., editors. Statistical Atlases and Computational Models of the Heart. Atrial Segmentation and LV Quantification Challenges. Shenzhen: Springer International Publishing. (2019), p. 32–39.
66. Verhorst PMJ, Kamp O, Visser CA, Verheugt FWA. Left Atrial Appendage Flow Velocity Assessment Using Transesophageal Echocardiography In Nonrheumatic Atrial-Fibrillation And Systemic Embolism. Am J Cardiol. (1993) 71:192–6. doi: 10.1016/0002-9149(93)90737-W
67. Bernhardt P, Schmidt H, Hammerstingl C, Luderitz B, Omran H. Patients with atrial fibrillation and dense spontaneous echo contrast at high risk - a prospective and serial follow-up over 12 months with transesophageal echocardiography and cerebral magnetic resonance imaging. J Am Coll Cardiol. (2005) 45:1807–12. doi: 10.1016/j.jacc.2004.11.071
68. Goldman ME, Pearce LA, Hart RG, Zabalgoitia M, Asinger RW, Safford R., et al. Stroke Prevention Atrial, Pathophysiologic correlates of thromboembolism in nonvalvular atrial fibrillation: I Reduced flow velocity in the left atrial appendage (The Stroke Prevention in Atrial Fibrillation SPAF-III study). J Am Soc Echocardiogr. (1999) 12:1080–7. doi: 10.1016/S0894-7317(99)70105-7
69. Taguchi Y, Takashima S, Hirai T, Fukuda N, Ohara K, Nakagawa K, et al. Significant impairment of left atrial function in patients with cardioembolic stroke caused by paroxysmal atrial fibrillation. Intern Med. (2010) 49:1727–32. doi: 10.2169/internalmedicine.49.3580
70. Kamp O, Verhorst PMJ, Welling RC, Visser CA. Importance of left atrial appendage flow as a predictor of thromboembolic events in patients with atrial fibrillation. Eur Heart J. (1999) 20:979–85. doi: 10.1053/euhj.1998.1453
71. Miyazaki S, Ito T, Suwa M, Nakamura T, Kobashi A, Kitaura Y. Role of transesophageal echocardiography in the prediction of thromboembolism in patients with chronic nonvalvular atrial fibrillation. Jpn Circ J Engl Ed. (2001) 65:874–8. doi: 10.1253/jcj.65.874
72. Negrotto SM, Lugo RM, Metawee M, Kanagasundram AN, Chidsey G, Baker MT, et al. Left atrial appendage morphology predicts the formation of left atrial appendage thrombus. J Cardiovasc Electrophysiol. (2021) 32:1044–52. doi: 10.1111/jce.14922
73. Chen L, Zinda A, Rossi N, Han XJ, Sprankle S, Bullock-Palmer R, et al. A new risk model of assessing left atrial appendage thrombus in patients with atrial fibrillation - Using multiple clinical and transesophageal echocardiography parameters. Int J Cardiol. (2020) 314:60–3. doi: 10.1016/j.ijcard.2020.04.039
74. Garciafernandez MA, Torrecilla EC, Roman DS, Azevedo J, Bueno H, Moreno MM, et al. Left atrial appendage doppler flow patterns - implications on thrombus formation. Am Heart J. (1992) 124:955–61. doi: 10.1016/0002-8703(92)90978-5
75. Iwama M, Kawasaki M, Tanaka R, Ono K, Watanabe T, Hirose T, et al. Left atrial appendage emptying fraction assessed by a feature-tracking echocardiographic method is a determinant of thrombus in patients with nonvalvular atrial fibrillation. J Cardiol. (2012) 59:329–36. doi: 10.1016/j.jjcc.2012.01.002
76. Park HC, Shin J, Ban JE, Choi JI, Park SW, Kim YH. Left atrial appendage: morphology and function in patients with paroxysmal and persistent atrial fibrillation. Int J Cardiovasc Imaging. (2013) 29:935–44. doi: 10.1007/s10554-012-0161-y
77. Garcia-Isla G, Olivares AL, Silva E, Nunez-Garcia M, Butakoff C, Sanchez-Quintana D, et al. Sensitivity analysis of geometrical parameters to study haemodynamics and thrombus formation in the left atrial appendage. Int J Numer Methods Biomed Eng. (2018) 34:14. doi: 10.1002/cnm.3100
78. Bosi GM, Cook A, Rai R, Menezes LJ, Schievano S, Torii R, et al. Computational fluid dynamic analysis of the left atrial appendage to predict thrombosis risk. Front Cardiovasc Med. (2018) 5:8. doi: 10.3389/fcvm.2018.00034
79. Masci A, Barone L, Dedè L, Fedele M, Tomasi C, Quarteroni A, et al. The impact of left atrium appendage morphology on stroke risk assessment in atrial fibrillation: a computational fluid dynamics study. Front Physiol. (2019) 9:11. doi: 10.3389/fphys.2018.01938
80. Masci A, Alessandrini M, Forti D, Menghini F, Dedé L, Tomasi C, et al. A proof of concept for computational fluid dynamic analysis of the left atrium in atrial fibrillation on a patient-specific basis. J Biomech Eng. (2020) 142:11. doi: 10.1115/1.4044583
81. Fang RX, Li Y, Zhang YJ, Chen Q, Liu QJ, Li ZY. Impact of left atrial appendage location on risk of thrombus formation in patients with atrial fibrillation. Biomech Model Mechanobiol. (2021) 20:1431–43. doi: 10.1007/s10237-021-01454-4
82. Sanatkhani S, Nedios S, Menon PG, Bollmann A, Hindricks G, Shroff SG. Subject-specific calculation of left atrial appendage blood-borne particle residence time distribution in atrial fibrillation. Front Physiol. (2021) 12:12. doi: 10.3389/fphys.2021.633135
83. Sanatkhani S, Menon PG. Relating atrial appendage flow stasis risk from computational fluid dynamics to imaging based appearance paradigms for cardioembolic risk. In: International Workshop on Bio-Imaging and Visualization for Patient-Customized Simulations (BIVPCS) / International Workshop on Point-of-Care Ultrasound - Algorithms, Hardware, and Applications (POCUS). Quebec, Canada. (2017), pp. 86–93.
84. Yan W, Qiao YH, Mao YK, Jiang CY, Fan JR, Luo K. Numerical prediction of thrombosis risk in left atrium under atrial fibrillation. Math Biosci Eng. (2020) 17:2348–60. doi: 10.3934/mbe.2020125
85. Menichini C, Xu XY. Mathematical modeling of thrombus formation in idealized models of aortic dissection: initial findings and potential applications. J Math Biol. (2016) 73:1205–26. doi: 10.1007/s00285-016-0986-4
86. Aguado AM, Olivares AL, Yague C, Silva E, Nunez-Garcia M, Femandez-Quilez A, et al. In silico optimization of left atrial appendage occluder implantation using interactive and modeling tools. Front Physiol. (2019) 10:237. doi: 10.3389/fphys.2019.00237
87. Wang LF, Wang ZD, Fang RX, Li ZY. Evaluation of stroke risk in patients with atrial fibrillation using morphological and hemodynamic characteristics. Front Cardiovasc Med. (2022) 9:8. doi: 10.3389/fcvm.2022.842364
88. D'Alessandro N, Masci A, Andalo A, Dede L, Tomasi C, Quarteroni A, et al. Simulation of the Hemodynamic Effects of the Left Atrial Appendage Occlusion in Atrial Fibrillation: Preliminary Results, Computing in Cardiology Conference (CinC). Rimini, Italy. (2020).
89. Zhang LT, Gay M. Characterizing left atrial appendage functions in sinus rhythm and atrial fibrillation using computational models. J Biomech. (2008) 41:2515–23. doi: 10.1016/j.jbiomech.2008.05.012
90. Feng LY, Gao H, Griffith B, Niederer S, Luo XY. Analysis of a coupled fluid-structure interaction model of the left atrium and mitral valve. Int J Numer Methods Biomed Eng. (2019) 35:e3254. doi: 10.1002/cnm.3254
91. Gonzalo M, Garcia-Villalba, Rossini L, Duran E, Vigneault D, Martinez-Legazpi P, et al. Non-newtonian blood rheology impacts left atrial stasis in patient-specific simulations. Int J Numer Methods Biomed Eng. (2022) 38:e3597. doi: 10.1002/cnm.3597
92. Otani T, Al-Issa A, Pourmorteza A, McVeigh ER, Wada S, Ashikaga H. A computational framework for personalized blood flow analysis in the human left atrium. Ann Biomed Eng. (2016) 44:3284–94. doi: 10.1007/s10439-016-1590-x
93. Qureshi A, Darwish O, Dillon-Murphy D, Chubb H, Williams S, Nechipurenko D, et al. Modelling left atrial flow and blood coagulation for risk of thrombus formation in atrial fibrillation. In: Computing in Cardiology Conference (CinC). Rimini, Italy. (2020).
94. Garcia-Villalba M, Rossini L, Gonzalo A, Vigneault D, Martinez-Legazpi P, Duran E, et al. Demonstration of patient-specific simulations to assess left atrial appendage thrombogenesis risk. Front Physiol. (2021) 12:596596. doi: 10.3389/fphys.2021.596596
95. Vella D, Monteleone A, Musotto G, Bosi GM, Burriesci G. Effect of the alterations in contractility and morphology produced by atrial fibrillation on the thrombosis potential of the left atrial appendage. Front Bioeng Biotechnol. (2021) 9:586041. doi: 10.3389/fbioe.2021.586041
96. Morales X, Mill J, Juhl KA, Olivares A, Jimenez-Perez G, Paulsen RR, et al. Deep learning surrogate of computational fluid dynamics for thrombus formation risk in the left atrial appendage. In: 10th International Workshop on Statistical Atlases and Computational Modelling of the Heart (STACOM). Shenzhen, Peoples R China. (2019), pp. 157–66.
97. Duenas-Pamplona J, Garcia Garcia J, Sierra-Pallares J, Ferrera C, Agujetas R, Lopez-Minguez JR. A comprehensive comparison of various patient-specific CFD models of the left atrium for atrial fibrillation patients. Comput Biol Med. (2021) 133:104423. doi: 10.1016/j.compbiomed.2021.104423
98. Kishima H, Mine T, Ashida K, Sugahara M, Kodani T, Masuyama T. Does left atrial appendage morphology influence left atrial appendage flow velocity? Circ J. (2015) 79:1706–11. doi: 10.1253/circj.CJ-14-1380
99. Fukushima K, Fukushima N, Kato K, Ejima K, Sato H, Fukushima K, et al. Correlation between left atrial appendage morphology and flow velocity in patients with paroxysmal atrial fibrillation. Eur Heart J Cardiovasc Imaging. (2016) 17:59–66. doi: 10.1093/ehjci/jev117
100. Manning WJ, Weintraub RM, Waksmonski CA, Haering JM, Rooney PS, Maslow AD, et al. Accuracy of transesophageal echocardiography for identifying left atrial thrombi - a prospective, intraoperative study. Ann Intern Med. (1995) 123:817-22. doi: 10.7326/0003-4819-123-11-199512010-00001
101. Bhagirath P, van der Graaf AWM, Karim R, van Driel V, Ramanna H, et al. Multimodality imaging for patient evaluation and guidance of catheter ablation for atrial fibrillation - current status and future perspective. Int J Cardiol. (2014) 175:400–8. doi: 10.1016/j.ijcard.2014.06.047
102. Romero J, Cao JJ, Garcia MJ, Taub CC. Cardiac imaging for assessment of left atrial appendage stasis and thrombosis. Nat Rev Cardiol. (2014) 11:470–80. doi: 10.1038/nrcardio.2014.77
103. Farkowski MM, Jubele K, Marin F, Gandjbakhch E, Ptaszynski P, Merino JL, et al. Diagnosis and management of left atrial appendage thrombus in patients with atrial fibrillation undergoing cardioversion or percutaneous left atrial procedures: results of the European Heart Rhythm Association survey. Europace. (2020) 22:162–9. doi: 10.1093/europace/euz257
104. Romero J, Husain SA, Kelesidis I, Sanz J, Medina HM, Garcia MJ. Detection of left atrial appendage thrombus by cardiac computed tomography in patients with atrial fibrillation a meta-analysis. Circ Cardiovasc Imaging. (2013) 6:185–94. doi: 10.1161/CIRCIMAGING.112.000153
105. Nakajima H, Seo Y, Ishizu T, Yamamoto M, Machin T, Harimura Y, et al. Analysis of the left atrial appendage by three-dimensional transesophageal echocardiography. Am J Cardiol. (2010) 106:885–92. doi: 10.1016/j.amjcard.2010.05.014
106. Morcos R, Al Taii H, Bansal P, Casale J, Manam R, Patel V, et al. Accuracy of commonly-used imaging modalities in assessing left atrial appendage for interventional closure: review article. J Clin Med. (2018) 7:13. doi: 10.3390/jcm7110441
107. Holmes DR, Reddy VY, Turi ZG, Doshi SK, Sievert H, Buchbinder M, et al. Investigators, percutaneous closure of the left atrial appendage vs. warfarin therapy for prevention of stroke in patients with atrial fibrillation: a randomised non-inferiority trial. Lancet. (2009) 374:534–42. doi: 10.1016/S0140-6736(09)61343-X
108. Yu SD, Zhang HP, Li HW. Cardiac computed tomography vs. transesophageal echocardiography for the detection of left atrial appendage thrombus: a systemic review and meta-analysis. J Am Heart Assoc. (2021) 10:23. doi: 10.1161/JAHA.121.022505
109. Morelos-Guzman M, Minero-Garcia L, Jaramillo-Almaguer JE, Chavez-Carbajal JF, Arean-Martinez CA, Vargas-Espinosa JM, et al. A novel, non-invasive, integral imaging assessment in atrial fibrillation by cardiac tomography. Arch Cardiol Mex. (2021) 91:41–8. doi: 10.24875/ACME.M21000174
110. Vira T, Pechlivanoglou P, Connelly K, Wijeysundera HC, Roifman I. Cardiac computed tomography and magnetic resonance imaging vs. transoesophageal echocardiography for diagnosing left atrial appendage thrombi. Europace. (2019) 21:E1–E10. doi: 10.1093/europace/euy142
111. Muellerleile K, Sultan A, Groth M, Steven D, Hoffmann B, Adam G, et al. Velocity encoded cardiovascular magnetic resonance to assess left atrial appendage emptying. J Cardiov Magn Reson. (2012) 14:39. doi: 10.1186/1532-429X-14-39
112. Camm AJ, Lip GYH, De Caterina R, Savelieva I, Atar D, Hohnloser SH, et al. 2012 focused update of the ESC guidelines for the management of atrial fibrillation an update of the 2010 ESC guidelines for the management of atrial fibrillation developed with the special contribution of the European Heart Rhythm Association. Europace. (2012) 14:1385–413. doi: 10.1093/europace/eus305
113. January CT, Wann LS, Calkins H, Chen LY, Cigarroa JE, Cleveland JC, et al. 2019 AHA/ACC/HRS Focused Update of the 2014 AHA/ACC/HRS guideline for the management of patients with atrial fibrillation a report of the American College of Cardiology/American Heart Association Task Force on Clinical Practice Guidelines and the Heart Rhythm Society. J Am Coll Cardiol. (2019) 74:104–32. doi: 10.1016/j.jacc.2019.01.011
114. Kirchhof P, Benussi S, Kotecha D, Ahlsson A, Atar D, Casadei B, et al. 2016 ESC Guidelines for the management of atrial fibrillation developed in collaboration with EACTS. Eur Heart J. (2016) 37:2893-962. doi: 10.5603/KP.2016.0172
115. Marsico F, Cecere M, Parente A, Paolillo S, de Martino F, Dellegrottaglie S, et al. Effects of novel oral anticoagulants on left atrial and left atrial appendage thrombi: an appraisal. J Thromb Thrombolysis. (2017) 43:139–48. doi: 10.1007/s11239-016-1421-9
116. Schnieder M, Siddiqui T, Karch A, Bahr M, Hasenfuss G, Schroeter MR, et al. Low flow in the left atrial appendage assessed by transesophageal echocardiography is associated with increased stroke severity-Results of a single-center cross-sectional study. Int J Stroke. (2019) 14:423–9. doi: 10.1177/1747493018816511
117. Gawalko M, Budnik M, Uzieblo-Zyczkowska B, Krzesinski P, Scislo P, Kochanowski J, et al. Decreased left atrial appendage emptying velocity as a link between atrial fibrillation type, heart failure and older age and the risk of left atrial thrombus in atrial fibrillation. Int J Clin Pract. (2020) 74:9. doi: 10.1111/ijcp.13609
118. Yaggi HK, Concato J, Kernan WN, Lichtman JH, Brass LM, Mohsenin V. Obstructive sleep apnea as a risk factor for stroke and death. N Engl J Med. (2005) 353:2034–41. doi: 10.1056/NEJMoa043104
119. Pereg D, Rozenbaum Z, Vorobeichik D, Shlomo N, Gilad R, Bloch S, et al. Prevalence and significance of unrecognized renal dysfunction in patients with stroke. Am J Med. (2016) 129:1074–81. doi: 10.1016/j.amjmed.2016.05.003
120. Hayashi K, Tsuda T, Nomura A, Fujino N, Nohara A, Sakata K, et al. Impact of B-type natriuretic peptide level on risk stratification of thromboembolism and death in patients with nonvalvular atrial fibrillation - the hokuriku-plus AF registry. Circ J. (2018) 82:L1271-8. doi: 10.1253/circj.CJ-17-1085
121. Jin C, Feng JJ, Wang L, Yu H, Liu J, Lu JW, et al. Left atrial appendage segmentation using fully convolutional neural networks and modified three-dimensional conditional random fields. IEEE J Biomed Health Inf. (2018) 22:1906–16. doi: 10.1109/JBHI.2018.2794552
122. Jin C, Feng JJ, Wang L, Yu H, Liu J, Lu JW, et al. Left atrial appendage segmentation and quantitative assisted diagnosis of atrial fibrillation based on fusion of temporal-spatial information. Comput Biol Med. (2018) 96:52–68. doi: 10.1016/j.compbiomed.2018.03.002
123. Morais P, Queiros S, De Meester P, Budts W, Vilaca JL, Tavares J, et al. Fast segmentation of the left atrial appendage in 3-D transesophageal echocardiographic images. IEEE Trans Ultrason Ferroelectr Freq Control. (2018) 65:2332–42. doi: 10.1109/TUFFC.2018.2872816
124. Qiao MY, Wang YY, Berendsen FF, van der Geest RJ, Tao Q. Fully automated segmentation of the left atrium, pulmonary veins, and left atrial appendage from magnetic resonance angiography by joint-atlas-optimization. Med Phys. (2019) 46:2074–84. doi: 10.1002/mp.13475
125. Leventic H, Babin D, Velicki L, Devos D, Galic I, Zlokolica V, et al. Left atrial appendage segmentation from 3D CCTA images for occluder placement procedure. Comput Biol Med. (2019) 104:163–74. doi: 10.1016/j.compbiomed.2018.11.006
126. Mill J, Agudelo V, Olivares AL, Pons MI, Silva E, Nunez-Garcia M, et al. Sensitivity analysis of in silico fluid simulations to predict thrombus formation after left atrial appendage occlusion. Mathematics. (2021) 9:1–14. doi: 10.3390/math9182304
Keywords: left atrial appendage, atrial fibrillation, stroke, association, risk evaluation
Citation: Fang R, Li Y, Wang J, Wang Z, Allen J, Ching CK, Zhong L and Li Z (2022) Stroke risk evaluation for patients with atrial fibrillation: Insights from left atrial appendage. Front. Cardiovasc. Med. 9:968630. doi: 10.3389/fcvm.2022.968630
Received: 14 June 2022; Accepted: 27 July 2022;
Published: 22 August 2022.
Edited by:
Nicola Mumoli, ASST Ovest Milanese, ItalyReviewed by:
Soroosh Sanatkhani, Columbia University, United StatesCristiana Corsi, University of Bologna, Italy
Christian Urbanek, Klinikum Ludwigshafen, Germany
Copyright © 2022 Fang, Li, Wang, Wang, Allen, Ching, Zhong and Li. This is an open-access article distributed under the terms of the Creative Commons Attribution License (CC BY). The use, distribution or reproduction in other forums is permitted, provided the original author(s) and the copyright owner(s) are credited and that the original publication in this journal is cited, in accordance with accepted academic practice. No use, distribution or reproduction is permitted which does not comply with these terms.
*Correspondence: Zhiyong Li, zylicam@gmail.com