- 1Section of Pharmacology, Department of Medicine and Surgery, University of Perugia, Perugia, Italy
- 2Department of Life Sciences, University of Trieste, Trieste, Italy
- 3Section of Pharmacology, Department of Pharmacy-Drug Sciences, University of Bari, Bari, Italy
COVID-19, the severe acute respiratory syndrome, is one of the major emergencies that have affected health care systems. Drugs and oxygen are only partially effective in saving lives in patients with severe COVID-19, and the most important protection from death is vaccination. The widespread use of COVID-19 adenovirus-based vaccines has provided evidence for the occurrence of rare venous thrombotic events including cerebral venous thrombosis and splanchnic venous thrombosis in recipients of Vaxzevria and Jcovden vaccines and the review focus on them. One year ago, thromboses in Vaxzevria recipients have been associated with thrombocytopenia in the presence of antibodies to platelet factor 4 and have been called vaccine-induced immune thrombotic thrombocytopenia (VITT). The incidence of VITT is equal to 9-31 events per one million doses of vaccines as evaluated by health agencies worldwide and is higher in female and young vaccine recipients. More recently, by using the European EudraVigilance database, it has been demonstrated that the incidence of thrombosis in recipients of adenovirus-based vaccines is 5–10 fold higher than that of VITT and 7–12 fold higher than observed in the recipients of Comirnaty, an mRNA-based vaccine, suggesting that adenovirus-based vaccines cause not only VITT but also thrombosis without thrombocytopenia (non-VITT thrombosis). The incidence of the vaccine-dependent non-VITT thrombosis is different in the adenovirus-based vaccines and the VITT/non-VITT incidence ratio depends on the severity of thrombosis and is inversely related to the age of the recipients. The possible causes and clinical implications of non-VITT thrombosis in vaccine recipients are discussed.
Efficacy and adverse events of COVID-19 vaccines
COVID-19 vaccines protect against severe COVID-19
COVID-19, the severe acute respiratory syndrome caused by the SARS-CoV-2 virus, is one of the major emergencies that have affected health care systems and society in recent decades. The clinical signs of COVID-19 depend on the severity of the disease and can be classified as mild, severe, and critical (1). Severe COVID-19 is characterized by oxygen saturation <90% on room air, signs of pneumonia, and trouble breathing. Exacerbation of the disease is defined by hypoxemic respiratory failure, sepsis, septic shock, and impairment of multiple organs, particularly the lungs (pneumonia and acute respiratory distress syndrome), heart (arrhythmias, chest pain), brain (dizziness, headache, impaired consciousness), kidneys, and liver. Many symptoms derive from the inflammatory response to the SARS-CoV-2 virus and the activation of coagulation. The Spike (S) protein may be implicated in the development of both phenomena (2–5).
In patients with a critical disease, drugs and life-sustaining treatments are only partially effective in saving lives (1, 6, 7), and the most important protection from death is represented by vaccination (8–14).
The mRNA-based vaccines Comirnaty (BNT162b2) and Spikevax (mRNA1273) and the adenovirus-based vaccines Vaxzevria (ChadOx1 nCoV-19) and Jcovden (AD26.COV2.S) were the first approved vaccines. Several studies have shown that these vaccines provide important protection against infection, severe COVID-19, and death (11–15). With the advent of omicron, protection against symptomatic disease is relatively poor, ranging from 20 to 80% after the primary vaccine series, but rapidly decreasing over time (0–30% of effectiveness 4–5 months after the last dose) (16–18). Despite the presence of major differences in efficacy following vaccination, as shown by several studies evaluating the same vaccine, it seems reasonable to conclude that protection is slightly lower after vaccination with adenovirus-based vaccines (18). On the contrary, the effectiveness of the vaccines against severe disease and hospitalization is high, ranging from 80 to 100% after the booster dose and remaining constant over time (18).
Adverse events of COVID-19 vaccines
The adverse events (AEs) observed following vaccination are largely mild or moderate in severity and include fever, chills, headache, flu-like symptoms, muscle pain, and lymphadenopathy (19–22). These events are observed soon after the injection, are not life-threatening and, except for extremely rare anaphylactic reactions, do not require additional treatments. On the other hand, severe AEs (SAEs), although rare, may occur even several days after vaccination and can be life-threatening.
Some SAEs were described for all vaccines, such as myocarditis and pericarditis; in a recently published study (23), the number of these events in young vaccinated males was 11.5, 11.7, and 15.4 events per million doses administered (OMD) of Comirnaty, Spikevax, and Vaxzevria, respectively. These events were reversible and no deaths were observed in under-40 vaccine recipients (23).
Some SAEs occur more frequently with only one/some vaccines, such as Bell’s palsy, caused by Comirnaty and Spikevax (24), and Guillain-Barré syndrome, caused by Jcovden (25). Other SAEs associated with specific COVID-19 vaccines are the venous and arterial thromboses observed in recipients of adenovirus-based vaccines. Our review focuses on these SAEs and their association with thrombocytopenia.
Venous thrombosis in recipients of adenovirus-based vaccines
Vaxzevria causes vaccine-induced immune thrombotic thrombocytopenia
The widespread use of COVID-19 vaccines has provided evidence for the occurrence of rare venous thrombotic events in recipients of the Vaxzevria vaccine (26). After two studies demonstrated that, in Vaxzevria recipients, venous thrombotic events [cerebral venous thrombosis (CVT), splanchnic venous thrombosis, and/or other thromboses] are observed in association with platelet aggregation, thrombocytopenia and antibodies to platelet factor 4 (PF4) (27, 28), such event was called vaccine-induced immune thrombotic thrombocytopenia (VITT). VITT is similar to heparin-induced thrombocytopenia (HIT) and is considered an adverse event caused by Vaxzevria (26). Vaccine-induced anti-PF4 antibodies are thought to be responsible for these thrombotic complications because anti-PF4 antibodies were rarely found in CVT patients in the past (i.e., before VITT) (29, 30).
The role of anti-PF4 antibodies in adenovirus-based vaccines was recently confirmed (31, 32). Baker et al. performed a computational simulation showing the electrostatic interaction of adenoviruses with PF4, which was experimentally confirmed by surface plasmon resonance. Greinacher et al. showed that vaccine components can form antigenic complexes with PF4 on platelet surfaces, which become targets of anti-PF4 antibodies in patients with VITT. In addition, they demonstrated that approximately 50% of the protein content of the Vaxzevria vaccine belongs to T-REx-293, the cells in which it is produced, and that EDTA, an excipient of the Vaxzevria vaccine, causes local vascular leakage that favors the systemic dissemination of the vaccine components (32). Finally, a confirmation of the pathogenesis of VITT comes from a washed platelet aggregation-based assay (32).
Incidence of vaccine-induced immune thrombotic thrombocytopenia in Vaxzevria recipients
After the discovery that Vaxzevria causes VITT, the incidence of VITT was classified as very rare by the European Medicines Agency (EMA) (26) but with the accumulation of data, regulatory agencies stated that in Vaxzevria recipients, 1-3 SAEs per 100,000 vaccinations are VITT. In particular, EMA, the Medicines and Healthcare products Regulatory Agency (MHRA, UK), Health Canada, and the Australian Technical Advisory Group on Immunisation (ATAGI) reported 9.3, 15.1, 21.9, and 27–31 VITT/OMD, respectively (33–36).
By evaluating SAEs from each patient report and considering only patients in whom both thrombosis and thrombocytopenia were reported, we showed that the VITT incidence in the European EudraVigilance database (EEd) was 19.4 SAEs/OMD in Vaxzevria recipients (37). The frequency of VITT reports is about 40 folds greater than that shown in Comirnaty recipients. Similar results were found in South Korea (38) even if the incidence of VITT in Vaxzevria recipients was lower than that observed in EEd.
Study findings and the variable incidence of VITT observed worldwide by regulatory agencies may be due to several factors, including genetics and co-morbidity of vaccinated populations, besides the fact that the sex and age of Vaxzevria recipients may also play a role. For example, we found that the incidence of CVT in Vaxzevria recipients was much higher in young people and women of childbearing age than in adult males and the elderly (39). In particular, a sixfold difference in CVT incidence was found between 18 and 24 years and 60–69 years Vaxzevria recipients (39). Thus, the difference in the incidence of VITT after the Vaxzevria vaccine in South Korea compared with Europe may be due, at least in part, to the age of vaccinee. In fact, in South Korea only 18.3% of the vaccinated people were under 50 years of age, whereas in Europe, these were 37.1% of the population (38, 39).
The incidence of thrombosis after Vaxzevria is much higher than that of vaccine-induced immune thrombotic thrombocytopenia
In Vaxzevria recipients, the incidence of thrombosis including CVTs, splanchnic vein thromboses, thromboembolic diseases, and “other” venous thrombotic events (with or without thrombocytopenia) was about 5 fold more (110.6 SAEs/OMD) than the above-reported incidence of VITT (37). Moreover, in the same recipients, the incidence of thromboses and thrombosis-related deaths was much higher than that observed in Comirnaty recipients, used as a baseline reference (37). Two more studies demonstrated an increased risk of venous thrombosis in Vaxzevria than in Comirnaty and Spikevax recipients (40, 41).
Given clinicians’ knowledge of VITT and the recommendation of regulatory authorities to check platelet count in the presence of thrombosis (42), underreporting of low platelet count is unlikely, and data suggest that most thromboses observed in Vaxzevria recipients, despite being caused by vaccination, occur without thrombocytopenia (non-VITT thrombosis). Non-VITT thrombosis following Vaxzevria vaccination has been reported by case studies and shows a persistently normal platelet count and can occur both in the presence and in the absence of anti-PF4 antibodies (43–46).
In Vaxzevria recipients, the VITT/non-VITT thrombosis incidence ratio is severity-dependent and inversely correlated with age
In Vaxzevria recipients, relevant differences in the association between thrombosis and thrombocytopenia are observed depending on the severity of thrombosis. Indeed, thrombocytopenia is associated with 18% of thrombotic SAEs and 48% of thrombosis-related deaths (37). Therefore, the non-VITT/VITT thrombosis incidence ratio is equal to 4.6 in thrombotic SAEs and 1.1 in thrombosis-related deaths. Moreover, thrombocytopenia is associated with 49% of CVT, one of the most severe thrombosis, and 77% of CVT-related deaths (37).
In addition, the incidence of the thrombocytopenia/CVT association is even higher in young-adults representing 83% of Vaxzevria recipients who died because of CVT (37) (Figure 1A). Data are consistent with the results published by Greinacher et al. that considered deadly Vaxzevria-dependent CVT to be almost always associated with thrombocytopenia (27). Therefore, thrombocytopenia is more frequent in life-threatening thromboses than in non-fatal ones and in young-adults than in the elderly.
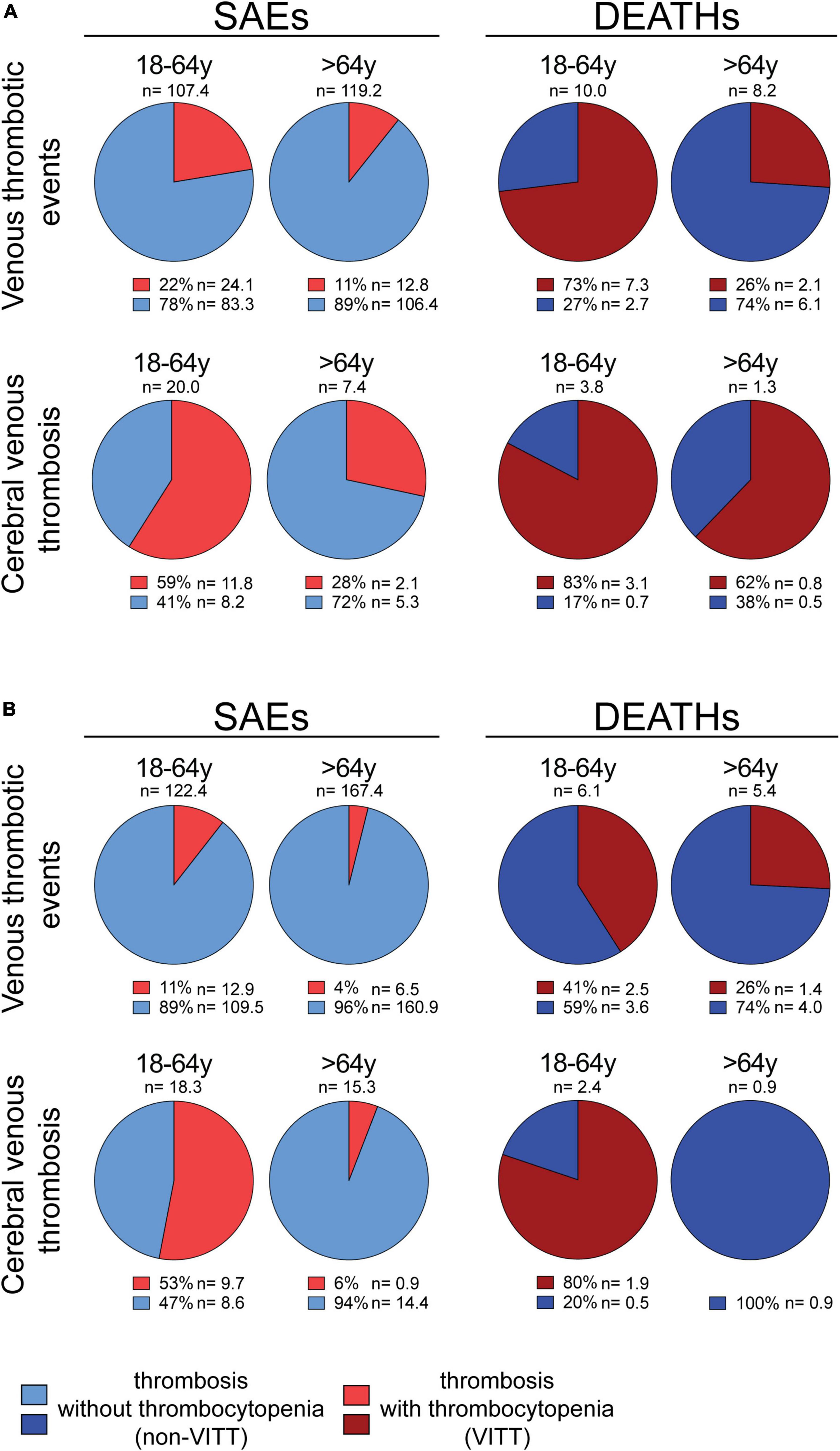
Figure 1. Percentage of thrombotic events associated or not with thrombocytopenia in Vaxzevria and Jcovden recipients. (A, left panel) All venous thrombotic events (cerebral venous thromboses, splanchnic vein thromboses, thromboembolic diseases, and “other” venous thrombotic events) or only cerebral venous thromboses, reported as SAEs in the EudraVigilance database following the Vaxzevria vaccine are shown. The number of events/OMD (n) and the percentage of individual SAEs associated (light red) or not (light blue) with thrombocytopenia are reported [data from (37)]. (A, right panel) The deaths due to all venous thrombotic events (cerebral venous thromboses, splanchnic vein thromboses, thromboembolic diseases, and “other” venous thrombotic events) or cerebral venous thromboses in the EudraVigilance database following the Vaxzevria vaccine are shown. The number of events/OMD (n) and the percentage of deaths associated (dark red) or not (dark blue) with thrombocytopenia are reported [data from (37)]. (B, left panel) All venous thrombotic events (cerebral venous thromboses, splanchnic vein thromboses, thromboembolic diseases, and “other” venous thrombotic events) or only cerebral venous thromboses, reported as SAEs in the EudraVigilance database following the Jcovden vaccine are shown. The number of events/OMD (n) and the percentage of individual SAEs associated (light red) or not (light blue) with thrombocytopenia are reported [data from (37)]. (B, right panel) The deaths due to all venous thrombotic events (cerebral venous thromboses, splanchnic vein thromboses, thromboembolic diseases, and “other” venous thrombotic events) or only cerebral venous thrombosis in the EudraVigilance database following the Jcovden vaccine are shown. The number of events/OMD (n) and the percentage of deaths associated (dark red) or not (dark blue) with thrombocytopenia are reported [data from (37)].
Incidence of vaccine-induced immune thrombotic thrombocytopenia in Jcovden recipients
Cerebral venous thrombosis (CVT) has also been observed in Jcovden recipients (47, 48), making VITT one of the severe AEs of Jcovden (49). Notably, the incidence of VITT in Jcovden recipients is lower than that observed in Vaxzevria recipients (37). The lower incidence of VITT in Jcovden as compared to Vaxzevria recipients is observed both in young-adults (12.9 vs. 24.1 SAEs/OMD) and in the elderly (6.5 vs. 12.7 SAEs/OMD) (37). Even VITT-related deaths are less frequent in Jcovden as compared to Vaxzevria recipients (37).
The incidence of thrombosis after Jcovden is much higher than that of vaccine-induced immune thrombotic thrombocytopenia
As in the case of Vaxzevria, the incidence of Jcovden-dependent thrombosis is much higher (131 SAEs/OMD) than the incidence of VITT (11.2 SAEs/OMD) (37), suggesting that most vaccine-dependent thromboses are unrelated to thrombocytopenia. The incidence of VITT decreases with the age of the vaccinee (37). Indeed, thrombocytopenia is associated with 11% and 4% of thrombotic SAEs in young-adults and elderly, respectively (Figure 1B) (39) and, in those who die, thrombocytopenia is associated with about 41 and 26% of the thrombotic SAEs in young-adults and elderly, respectively (Figure 1B) (39). Moreover, the incidence of VITT increases with the severity of SAEs (Figure 1B) (37). An exception to the trend of VITT incidence is the virtual absence of VITT in the elderly with CVT or dying of CVT (Figure 1B) (37). One case report described the occurrence of non-VITT thrombosis in Jcovden recipients (50).
Differences between Vaxzevria- and Jcovden-dependent thrombosis
The VITT/non-VITT incidence ratio in Vaxzevria and Jcovden recipients is different, although both are adenovirus-based vaccines. While Vaxzevria recipients show a twice higher incidence of VITT than Jcovden recipients, the incidence of non-VITT thrombosis is higher in Jcovden recipients than in Vaxzevria recipients (120 and 91 SAEs/OMD, respectively) (37). In addition, the incidence of thrombosis and thromboembolic diseases is similar in Vaxzevria and Jcovden young-adult recipients and is higher in Jcovden than Vaxzevria elderly recipients (37).
Arterial thrombosis in recipients of adenovirus-based vaccines
The incidence of arterial thrombotic events [myocardial infarction, ischemic stroke, and non-cardiac, non-cerebral arterial thrombotic events (37)] in Vaxzevria and Jcovden recipients has been compared with that in Comirnaty, used as baseline control (37). The incidence of myocardial infarction reported in EEd in young-adult recipients of adenovirus-based vaccines is about sixfold higher, compared with Comirnaty, and an even higher incidence was observed in other arterial SAEs (Figure 2A), suggesting that Vaxzevria and Jcovden vaccines not only cause venous thrombosis events but also cause arterial thrombosis. Specifically, in Jcovden elderly recipients, the excess of ischemic stroke over Comirnaty is 106 SAEs/OMD, causing an excess of 10.5 deaths/OMD (37). A recent study, confirms that Vaxzevria and Jcovden vaccines are associated with myocardial infarction (relative incidence, 1.29 and 1.75, respectively), even if significance concerning the SAE due to Jcovden was not reached, probably due to the low number of vaccinations with Jcovden (50).
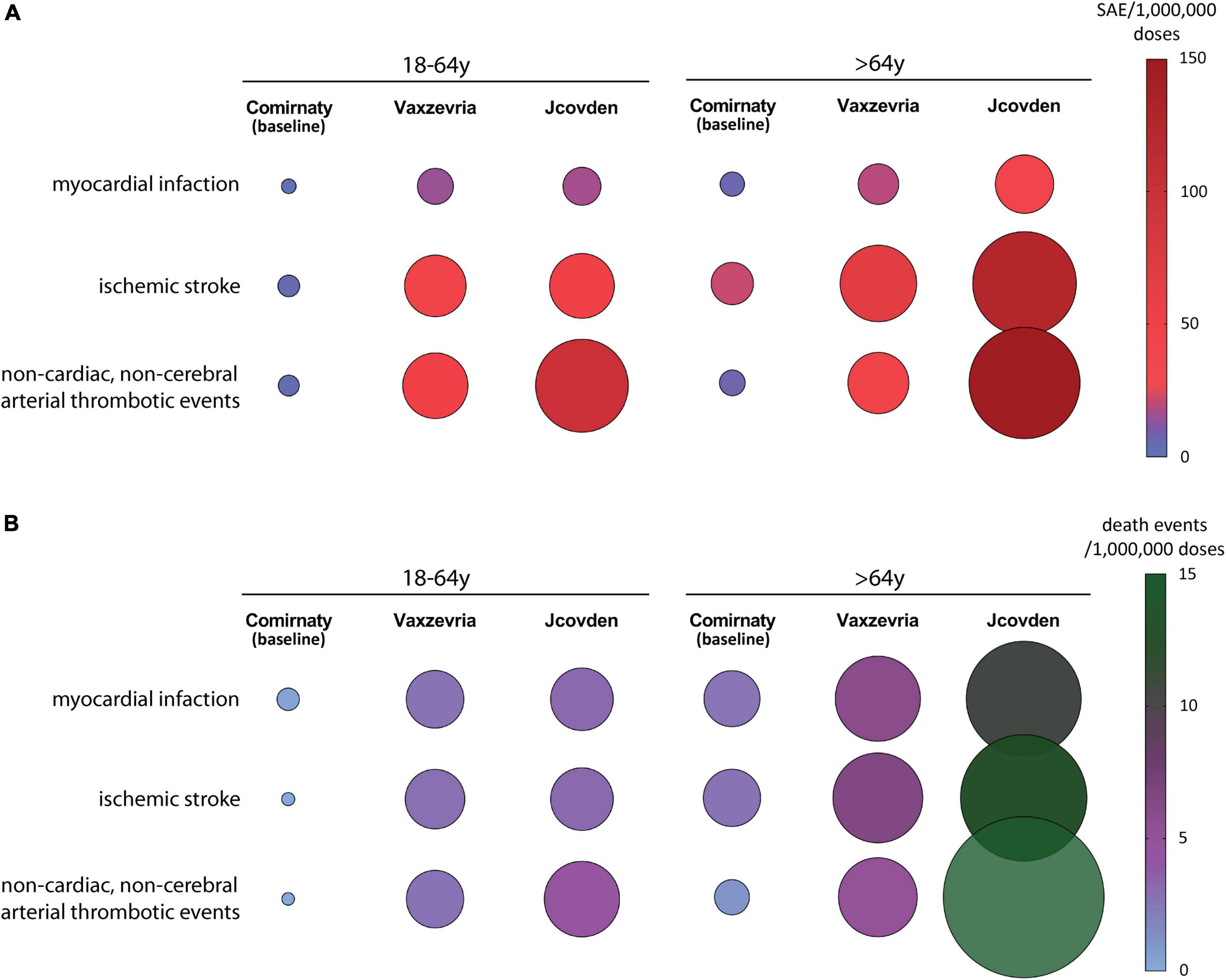
Figure 2. The incidence of arterial thrombotic events in the EudraVigilance database following Vaxzevria and Jcovden vaccines are shown. The incidence of events following Comirnaty is reported for comparison. (A) Incidence of individual SAEs/OMD related to arterial thrombotic events in young-adult (left panel) and elderly (right panel) vaccine recipients [data from (37)]. (B) Incidence of death events/OMD related to arterial thrombotic events in young-adult (left panel) and elderly (right panel) vaccine recipients [data from (37)].
Regarding arterial SAEs, Jcovden causes more SAEs and SAE-dependent deaths than Vaxzevria (Figure 2B).
Discussion
Are VITT and non-VITT thromboses caused by different mechanisms?
It can be hypothesized that non-VITT thrombosis can occur in two ways. First, thrombosis is caused by anti-PF4 antibodies that activate platelets only at specific sites and do not cause thrombocytopenia [thrombosis with anti-PF4 antibodies without thrombocytopenia syndrome (T4-noTS)]. T4-noTS would mean that platelets, activated by low concentrations of anti-PF4 antibodies, aggregate mainly at sites where predisposing factors (e.g., pro-inflammatory environment, blood stasis) are present without causing massive consumption of platelets and thrombocytopenia. According to this hypothesis, T4-noTS would be caused by the same mechanisms leading to VITT. The hypothesis seems to be confirmed by the higher incidence of thrombocytopenia in vaccine recipients who die due to thrombosis as compared to those who survive.
The second way to envision non-VITT thrombosis is to hypothesize that anti-PF4 antibodies are not involved when thrombosis is not associated with thrombocytopenia [thrombosis without anti-PF4 antibodies without thrombocytopenia syndrome (T-no4TS)]. T-no4TS could be caused by factors promoting platelet aggregation, such as an inflammatory response favored by adenovirus-based vaccines and local predisposing factors. The hypothesis seems to be confirmed by the higher incidence of arterial thrombotic events in adenovirus-based vaccine recipients, the different incidence of thrombosis and VITT between Vaxzevria and Jcovden recipients, and the different ages at which VITT and non-VITT thrombosis are observed.
How can vaccines cause pro-thrombotic effects independent of anti-PF4 antibodies?
Azzarone et al. hypothesized that in vaccine recipients suffering from local inflammatory reaction, an early event is represented by the production of interleukin 6 (IL-6) (3) which favors endothelial cells reactivity and induces plasminogen activator inhibitor-1 (51, 52), the main physiological inhibitor of the plasminogen activators in the bloodstream (53). A confirmation of the hypothesis came from an observational study demonstrating that 24-48h following Vaxzevria vaccination, the IL-6 levels were almost doubled (54).
Interestingly, another observational study demonstrated that in healthy young-adults vaccinated with Vaxzevria, hemostatic changes were still present after about one month from vaccination (55). In particular, the levels of Von Willebrand Factor (VWF) and active VWF, indicating endothelial activation, were increased 39% and 24%, respectively. Moreover, faster thrombin generation (i.e., more active coagulation system) and lower coagulations levels (use of coagulation factors) were observed, suggesting that the hemostatic system of vaccinee is shifted to a more procoagulant state compared to unvaccinated controls. Therefore, non-VITT thrombo-embolic events may be the clinical expression of procoagulant coagulation profile, consequent to a pro-inflammatory trigger, represented by vaccination with adenovirus-based vaccines.
How do vaccine components determine pro-inflammatory/pro-thrombotic events?
In search of potential pro-inflammatory and pro-thrombotic triggering factors present in virus-based vaccines, we found three factors: the S protein (2, 4, 56, 57), the adenovirus particles (3, 31), and the cellular debris present in vaccine preparation (32).
The presence of S protein in blood vessels and tissues other than the muscle into which the vaccine is injected can be hypothesized. The S protein may induce pro-inflammatory cytokines and chemokines in macrophages (56, 57) and T cells (58), directly damage endothelium (4), activate platelets (5), and autoimmune response, the latter triggered by antigenic epitopes of S protein shared with molecular chaperones (59). However, given the lower incidence of thrombosis and arterial SAEs in Comirnaty recipients, S protein appears to be not sufficient to cause such events, at least at the concentration determined by the Comirnaty vaccine.
Viral blood dissemination was detected either in patients treated with infusions of adenoviral vectors in the tumor or the right hepatic artery (51, 60). Indeed, after the injection of Vaxzevria into mice, sporadic small amounts of the virus were found in other tissues (61). Adenoviral particles seem to not promote platelet aggregation (3), but the receptors mediating the binding of adenoviral particles to cells are expressed by human endothelial cells, platelets, and erythrocytes (3, 31).
Finally, it should also be considered that the Vaxzevria and Jcovden vaccines contain residual cellular proteins from the cell lines by which adenoviruses are produced likely causing platelet activation (32).
If the reasons why adenovirus-based vaccines cause non-VITT thrombosis and arterial events might be those mentioned above, it is currently impossible to say which is the main culprit. Their synergism is likely crucial.
Why is the incidence of severe adverse events different in recipients of Vaxzevria and Jcovden?
The different incidences of VITT, non-VITT thromboses, and arterial events in recipients of Vaxzevria and Jcovden may be explained by the differences between the two vaccines, which can be summarized as follows. First, the type of adenovirus is different and it has been demonstrated that chimpanzee adenovirus (Vaxzevria) has a stronger negative charge than the human Ad26 virus (Jcovden) (62). Moreover, molecular simulations suggest that the Vaxzevria’s adenovirus charge and shape could allow it to bind to the positively charged PF4 protein (31). Second, the amount of infectious units in the Jcovden vaccine is 3.3-fold higher than that in the Vaxzevria vaccine (49, 63), possibly implying a higher S protein and adenoviral protein burden. Third, the human cell lines by which adenoviruses are produced are different: T-REx-293 cells for Vaxzevria and PER.C6 TetR cells for Jcovden (64). Furthermore, approximately 50% of the protein content of the Vaxzevria vaccine belongs to T-REx-293 (32), while Jcovden seems to be less contaminated by cell line debris (64). Finally, EDTA is present in the Vaxzevria vaccine but not in the Jcovden vaccine (49, 63) and may favor the development of inflammation at the vaccine inoculation site.
Concluding remarks
The incidence of VITT, non-VITT thrombosis, and arterial events, although rare, is much higher in recipients of Vaxzevria and Jcovden vaccines than in recipients of the Comirnaty vaccine (37, 38), suggesting a causal relationship between these events and the adenovirus-based vaccines. We hypothesize that the venous and arterial thromboses observed with adenovirus-based vaccines and observed in absence of thrombocytopenia are due to the combination of at least three triggering factors, all of which may be involved in vascular inflammation and coagulation, and several arguments suggest that it is independent of anti-PF4 antibodies (T-no4TS). However, further studies are needed to confidently exclude that non-VITT thromboses are due to low levels of anti-PF4 antibodies.
In Vaxzevria recipients with thrombosis, the incidence of VITT is very high when they die due to CVT. However, in the presence of thrombosis other than CVT, the incidence of VITT is lower, and it is further reduced in the elderly and young adults who do not die. Therefore, in recently vaccinated individuals, the possibility that a thrombosis or arterial event is caused by adenovirus-based vaccines should be considered even in the absence of thrombocytopenia, favoring a correct diagnosis and providing important information for the management of treatment.
Author contributions
GN with the contribution of LC, MNA, AB, SPa, SPi, and GS: conceptualization. GN, LC, and GS: writing—original draft. All authors contributed to the article and approved the submitted version.
Funding
This article was funded by Ministero dell’Università e della Ricerca (MUR) (grant number: FISR2020IP_03103 and granted to AB, GN, and SPi).
Conflict of interest
The authors have no relevant affiliation or financial involvement with any organization or entity with a financial interest in or financial conflict with the subject matter or material discussed in the manuscript. All this includes employment, consultancies, honoraria, stock ownership or options, expert testimony, grants or patents received or pending, and royalties.
Publisher’s note
All claims expressed in this article are solely those of the authors and do not necessarily represent those of their affiliated organizations, or those of the publisher, the editors and the reviewers. Any product that may be evaluated in this article, or claim that may be made by its manufacturer, is not guaranteed or endorsed by the publisher.
References
1. World Health Organization [WHO]. Therapeutics and COVID-19: Living Guideline. Geneva: WHO (2022).
2. Kircheis R. Coagulopathies after vaccination against SARS-CoV-2 may be derived from a combined effect of SARS-CoV-2 spike protein and adenovirus vector-triggered signaling pathways. Int J Mol Sci. (2021) 22:10791. doi: 10.3390/ijms221910791
3. Azzarone B, Veneziani I, Moretta L, Maggi E. Pathogenic mechanisms of vaccine-induced immune thrombotic thrombocytopenia in people receiving anti-COVID-19 adenoviral-based vaccines: a proposal. Front Immunol. (2021) 12:728513. doi: 10.3389/fimmu.2021.728513
4. Lei Y, Zhang J, Schiavon CR, He M, Chen L, Shen H, et al. SARS-CoV-2 spike protein impairs endothelial function via downregulation of ACE 2. Circ Res. (2021) 128:1323–6. doi: 10.1161/CIRCRESAHA.121.318902
5. Zhang S, Liu Y, Wang X, Yang L, Li H, Wang Y, et al. SARS-CoV-2 binds platelet ACE2 to enhance thrombosis in COVID-19. J Hematol Oncol. (2020) 13:120. doi: 10.1186/s13045-020-00954-7
6. Wiersinga WJ, Rhodes A, Cheng AC, Peacock SJ, Prescott HC. Pathophysiology, transmission, diagnosis, and treatment of coronavirus disease 2019 (COVID-19). JAMA. (2020) 324:782. doi: 10.1001/jama.2020.12839
7. Harrison AG, Lin T, Wang P. Mechanisms of SARS-CoV-2 transmission and pathogenesis. Trends Immunol. (2020) 41:1100–15. doi: 10.1016/j.it.2020.10.004
8. Vasileiou E, Simpson CR, Shi T, Kerr S, Agrawal U, Akbari A, et al. Interim findings from first-dose mass COVID-19 vaccination roll-out and COVID-19 hospital admissions in Scotland: a national prospective cohort study. Lancet. (2021) 397:1646–57. doi: 10.1016/S0140-6736(21)00677-2
9. Lopez Bernal J, Andrews N, Gower C, Robertson C, Stowe J, Tessier E, et al. Effectiveness of the Pfizer-BioNTech and Oxford-AstraZeneca vaccines on covid-19 related symptoms, hospital admissions, and mortality in older adults in England: test negative Case-Control Study. BMJ. (2021) 373:n1088. doi: 10.1136/bmj.n1088
10. Dagan N, Barda N, Kepten E, Miron O, Perchik S, Katz MA, et al. BNT162b2 mRNA Covid-19 vaccine in a nationwide mass vaccination setting. N Engl J Med. (2021) 384:1412–23. doi: 10.1056/NEJMoa2101765
11. Polack FP, Thomas SJ, Kitchin N, Absalon J, Gurtman A, Lockhart S, et al. Safety and efficacy of the BNT162b2 mRNA Covid-19 vaccine. N Engl J Med. (2020) 383:2603–15. doi: 10.1056/NEJMoa2034577
12. Baden LR, El Sahly HM, Essink B, Kotloff K, Frey S, Novak R, et al. Efficacy and safety of the mRNA-1273 SARS-CoV-2 vaccine. N Engl J Med. (2021) 384:403–16. doi: 10.1056/NEJMoa2035389
13. Voysey M, Clemens SAC, Madhi SA, Weckx LY, Folegatti PM, Aley PK, et al. Safety and efficacy of the ChAdOx1 nCoV-19 vaccine (AZD1222) against SARS-CoV-2: an interim analysis of four randomised controlled trials in Brazil, South Africa, and the UK. Lancet. (2021) 397:99–111. doi: 10.1016/S0140-6736(20)32661-1
14. Sadoff J, Gray G, Vandebosch A, Cárdenas V, Shukarev G, Grinsztejn B, et al. Final analysis of efficacy and safety of single-dose Ad26.COV2.S. N Engl J Med. (2022) 386:847–60. doi: 10.1056/NEJMoa2117608
15. Dan JM, Mateus J, Kato Y, Hastie KM, Yu ED, Faliti CE, et al. Immunological memory to SARS-CoV-2 assessed for up to 8 months after infection. Science. (2021) 371:eabf4063. doi: 10.1126/science.abf4063
16. Bar-On YM, Goldberg Y, Mandel M, Bodenheimer O, Amir O, Freedman L, et al. Protection by a fourth dose of BNT162b2 against Omicron in Israel. N Engl J Med. (2022) 386:1712–20. doi: 10.1056/NEJMoa2201570
17. Hein S, Mhedhbi I, Zahn T, Sabino C, Benz NI, Husria Y, et al. Quantitative and qualitative difference in antibody response against omicron and ancestral SARS-CoV-2 after third and fourth vaccination. Vaccines. (2022) 10:796. doi: 10.3390/vaccines10050796
18. Higdon MM, Baidya A, Walter KK, Patel MK, Issa H, Espié E, et al. Duration of effectiveness of vaccination against COVID-19 caused by the omicron variant. Lancet Infect Dis. (2022) 22:1114–6. doi: 10.1016/S1473-3099(22)00409-1
19. European Medicines Agency [EMA]. Comirnaty: EPAR - Medicine Overview. Amsterdam: European Medicines Agency (2022).
20. European Medicines Agency [EMA]. Spikevax (Previously COVID-19 Vaccine Moderna): EPAR - Medicine Overview. Amsterdam: European Medicines Agency (2022).
21. European Medicines Agency [EMA]. Jcovden (Previously COVID-19 Vaccine Janssen): EPAR - Medicine Overview. Amsterdam: European Medicines Agency (2022).
22. European Medicines Agency [EMA]. Vaxzevria (Previously COVID-19 Vaccine AstraZeneca): EPAR - Medicine Overview. Amsterdam: European Medicines Agency (2022).
23. Karlstad Ø, Hovi P, Husby A, Härkänen T, Selmer RM, Pihlström N, et al. SARS-CoV-2 vaccination and myocarditis in a Nordic Cohort Study of 23 million residents. JAMA Cardiol. (2022) 7:600. doi: 10.1001/jamacardio.2022.0583
24. Ozonoff A, Nanishi E, Levy O. Bell’s palsy and SARS-CoV-2 vaccines. Lancet Infect Dis. (2021) 21:450–2. doi: 10.1016/S1473-3099(21)00076-1
25. Hanson KE, Goddard K, Lewis N, Fireman B, Myers TR, Bakshi N, et al. Incidence of guillain-barré syndrome after COVID-19 vaccination in the vaccine safety datalink. JAMA Netw Open. (2022) 5:e228879. doi: 10.1001/jamanetworkopen.2022.8879
26. European Medicines Agency [EMA]. AstraZeneca’s COVID-19 Vaccine: EMA Finds Possible Link to Very Rare Cases of Unusual Blood Clots with Low Blood Platelets. Amsterdam: European Medicines Agency (2021).
27. Greinacher A, Thiele T, Warkentin TE, Weisser K, Kyrle PA, Eichinger S. Thrombotic thrombocytopenia after ChAdOx1 nCov-19 vaccination. N Engl J Med. (2021) 384:2092–101. doi: 10.1056/NEJMoa2104840
28. Schultz NH, Sørvoll IH, Michelsen AE, Munthe LA, Lund-Johansen F, Ahlen MT, et al. Thrombosis and thrombocytopenia after ChAdOx1 nCoV-19 vaccination. N Engl J Med. (2021) 384:2124–30. doi: 10.1056/NEJMoa2104882
29. Krzywicka K, Heldner MR, Sánchez van Kammen M, van Haaps T, Hiltunen S, Silvis SM, et al. Post-SARS-CoV-2-vaccination cerebral venous sinus thrombosis: an analysis of cases notified to the European Medicines Agency. Eur J Neurol. (2021) 28:3656–62. doi: 10.1111/ene.15029
30. Sánchez van Kammen M, Heldner MR, Brodard J, Scutelnic A, Silvis S, Schroeder V, et al. Frequency of thrombocytopenia and platelet factor 4/heparin antibodies in patients with cerebral venous sinus thrombosis prior to the COVID-19 pandemic. JAMA. (2021) 326:332. doi: 10.1001/jama.2021.9889
31. Baker AT, Boyd RJ, Sarkar D, Teijeira-Crespo A, Chan CK, Bates E, et al. ChAdOx1 interacts with CAR and PF4 with implications for thrombosis with thrombocytopenia syndrome. Sci Adv. (2021) 7:eabl8213. doi: 10.1126/sciadv.abl8213
32. Greinacher A, Selleng K, Palankar R, Wesche J, Handtke S, Wolff M, et al. Insights in ChAdOx1 nCoV-19 vaccine-induced immune thrombotic thrombocytopenia. Blood. (2021) 138:2256–68. doi: 10.1182/blood.2021013231
33. European Medicines Agency [EMA]. COVID-19 Vaccine Safety Update for Vaxzevria (Previously COVID-19 Vaccine AstraZeneca): 14 July 2021. Amsterdam: European Medicines Agency (2021).
34. Medicines and Healthcare products Regulatory Agency [MHRA]. Coronavirus Vaccine - Weekly Summary of Yellow Card Reporting - Updated 30 September 2021. London: Medicines and Healthcare products Regulatory Agency (2021).
35. Health Canada. Reported side Effects following COVID-19 Vaccination in Canada - September 24, 2021. Ottawa, ON: Health Canada (2021).
36. Australian Government Department of Health. ATAGI Statement on Revised Recommendations on the Use of COVID-19 Vaccine AstraZeneca, 17 June 2021. Canberra, ACT: Australian Government Department of Health (2021).
37. Cari L, Alhosseini MN, Fiore P, Pierno S, Pacor S, Bergamo A, et al. Cardiovascular, neurological, and pulmonary events following vaccination with the BNT162b2, ChAdOx1 nCoV-19, and Ad26.COV2.S vaccines: an analysis of European data. J Autoimmun. (2021) 125:102742. doi: 10.1016/j.jaut.2021.102742
38. Lee DS, Kim JW, Lee KL, Jung YJ, Kang HW. Adverse events following COVID-19 vaccination in South Korea between February 28 and August 21, 2021: a nationwide observational study. Int J Infect Dis. (2022) 118:173–82. doi: 10.1016/j.ijid.2022.03.007
39. Cari L, Fiore P, Naghavi Alhosseini M, Sava G, Nocentini G. Blood clots and bleeding events following BNT162b2 and ChAdOx1 nCoV-19 vaccine: an analysis of European data. J Autoimmun. (2021) 122:102685. doi: 10.1016/j.jaut.2021.102685
40. Pottegård A, Lund LC, Karlstad Ø, Dahl J, Andersen M, Hallas J, et al. Arterial events, venous thromboembolism, thrombocytopenia, and bleeding after vaccination with Oxford-AstraZeneca ChAdOx1-S in Denmark and Norway: population based cohort study. BMJ. (2021) 373:n1114. doi: 10.1136/bmj.n1114
41. Dag Berild J, Bergstad Larsen V, Myrup Thiesson E, Lehtonen T, Grøsland M, Helgeland J, et al. Analysis of thromboembolic and thrombocytopenic events after the AZD1222, BNT162b2, and MRNA-1273 COVID-19 vaccines in 3 Nordic Countries. JAMA Netw Open. (2022) 5:e2217375. doi: 10.1001/jamanetworkopen.2022.17375
42. European Medicines Agency [EMA]. COVID-19 Vaccine Safety Update for Vaxzevria (Previously COVID-19 Vaccine AstraZeneca): 21 May 2021. Amsterdam: European Medicines Agency (2021).
43. Page D, Zhu N, Sawler D, Sun HW, Turley E, Pai M, et al. Vaccine-induced immune thrombotic thrombocytopenia presenting with normal platelet count. Res Pract Thromb Haemost. (2021) 5:e12596. doi: 10.1002/rth2.12596
44. Gabarin N, Patterson S, Pai M, Afzaal T, Nazy I, Sheppard J-AI, et al. Venous thromboembolism and mild thrombocytopenia after ChAdOx1 nCoV-19 vaccination. Thromb Haemost. (2021) 121:1677–80. doi: 10.1055/a-1585-6182
45. Walter U, Fuchs M, Grossmann A, Walter M, Thiele T, Storch A, et al. Adenovirus-vectored COVID-19 vaccine–induced immune thrombosis of carotid artery. Neurology. (2021) 97:716–9. doi: 10.1212/WNL.0000000000012576
46. Greinacher A, Langer F, Makris M, Pai M, Pavord S, Tran H, et al. Vaccine-induced immune thrombotic thrombocytopenia (VITT): update on diagnosis and management considering different resources. J Thromb Haemost. (2022) 20:149–56. doi: 10.1111/jth.15572
47. U.S. Food and Drug Administration [FDA]. Joint CDC and FDA Statement on Johnson & Johnson COVID-19 Vaccine. Silver Spring, MD: U.S. Food and Drug Administration (2021).
48. See I, Su JR, Lale A, Woo EJ, Guh AY, Shimabukuro TT, et al. US case reports of cerebral venous sinus thrombosis with thrombocytopenia after Ad26.COV2.S vaccination, March 2 to April 21, 2021. JAMA. (2021) 325:2448. doi: 10.1001/jama.2021.7517
49. European Medicines Agency [EMA]. COVID-19 Vaccine Janssen?: EPAR - Product Information. Amsterdam: European Medicines Agency (2022).
50. Botton J, Jabagi MJ, Bertrand M, Baricault B, Drouin J, Le Vu S, et al. Risk for myocardial infarction, stroke, and pulmonary embolism following COVID-19 vaccines in adults younger than 75 years in France. Ann Intern Med. (2022): [Epub ahead of print]. doi: 10.7326/M22-0988
51. Raper SE, Yudkoff M, Chirmule N, Gao G-P, Nunes F, Haskal ZJ, et al. A pilot study of in vivo liver-directed gene transfer with an adenoviral vector in partial ornithine transcarbamylase deficiency. Hum Gene Ther. (2002) 13:163–75. doi: 10.1089/10430340152712719
52. Kang S, Tanaka T, Inoue H, Ono C, Hashimoto S, Kioi Y, et al. IL-6 trans-signaling induces plasminogen activator inhibitor-1 from vascular endothelial cells in cytokine release syndrome. Proc Natl Acad Sci USA. (2020) 117:22351–6. doi: 10.1073/pnas.2010229117
53. Westrick R, Eitzman D. Plasminogen activator inhibitor-1 in vascular thrombosis. Curr Drug Targets. (2007) 8:996–1002. doi: 10.2174/138945007781662328
54. Willems LH, Nagy M, Ten Cate H, Spronk HMH, Jacobs LMC, Kranendonk J, et al. ChAdOx1 vaccination, blood coagulation, and inflammation: no effect on coagulation but increased interleukin-6. Res Pract Thromb Haemost. (2021) 5:e12630. doi: 10.1002/rth2.12630
55. de Laat B, Stragier H, de Laat-Kremers R, Ninivaggi M, Mesotten D, Thiessen S, et al. Population-wide persistent hemostatic changes after vaccination with ChAdOx1-S. Front Cardiovasc Med. (2022) 9:966028. doi: 10.3389/fcvm.2022.966028
56. Khan S, Shafiei MS, Longoria C, Schoggins JW, Savani RC, Zaki H. SARS-CoV-2 spike protein induces inflammation via TLR2-dependent activation of the NF-κB pathway. Elife. (2021) 10:e68563. doi: 10.7554/eLife.68563
57. Olajide OA, Iwuanyanwu VU, Lepiarz-Raba I, Al-Hindawi AA. Induction of exaggerated cytokine production in human peripheral blood mononuclear cells by a recombinant SARS-CoV-2 spike glycoprotein S1 and its inhibition by dexamethasone. Inflammation. (2021) 44:1865–77. doi: 10.1007/s10753-021-01464-5
58. Scaglioni V, Soriano ER. Are superantigens the cause of cytokine storm and viral sepsis in severe COVID-19? Observations and hypothesis. Scand J Immunol. (2020) 92:e12944. doi: 10.1111/sji.12944
59. Paladino L, Vitale AM, Caruso Bavisotto C, Conway de Macario E, Cappello F, Macario AJL, et al. The role of molecular chaperones in virus infection and implications for understanding and treating COVID-19. J Clin Med. (2020) 9:3518. doi: 10.3390/jcm9113518
60. Seiradake E, Henaff D, Wodrich H, Billet O, Perreau M, Hippert C, et al. The cell adhesion molecule “CAR” and sialic acid on human erythrocytes influence adenovirus in vivo biodistribution. PLoS Pathog. (2009) 5:e1000277. doi: 10.1371/journal.ppat.1000277
61. European Medicines Agency [EMA]. Vaxzevria (Previously COVID-19 Vaccine AstraZeneca)?: EPAR - Public Assessment Report. Amsterdam: European Medicines Agency (2021).
62. Ledford H. COVID vaccines and blood clots: what researchers know so far. Nature. (2021) 596:479–81. doi: 10.1038/d41586-021-02291-2
63. European Medicines Agency [EMA]. Vaxzevria (Previously COVID-19 Vaccine AstraZeneca)?: EPAR - Product Information. Amsterdam: European Medicines Agency (2021).
Keywords: COVID-19 vaccines, adenovirus-based vaccines, VITT, thrombosis, thrombocytopenia, inflammatory response
Citation: Cari L, Naghavi Alhosseini M, Bergamo A, Pacor S, Pierno S, Sava G and Nocentini G (2022) Thrombotic events with or without thrombocytopenia in recipients of adenovirus-based COVID-19 vaccines. Front. Cardiovasc. Med. 9:967926. doi: 10.3389/fcvm.2022.967926
Received: 13 June 2022; Accepted: 06 September 2022;
Published: 29 September 2022.
Edited by:
Hugo Ten Cate, Maastricht University Medical Centre, NetherlandsReviewed by:
Gayathri Viswanathan, Duke University, United StatesHendrik Stragier, East Limburg Hospital, Belgium
Copyright © 2022 Cari, Naghavi Alhosseini, Bergamo, Pacor, Pierno, Sava and Nocentini. This is an open-access article distributed under the terms of the Creative Commons Attribution License (CC BY). The use, distribution or reproduction in other forums is permitted, provided the original author(s) and the copyright owner(s) are credited and that the original publication in this journal is cited, in accordance with accepted academic practice. No use, distribution or reproduction is permitted which does not comply with these terms.
*Correspondence: Giuseppe Nocentini, Z2l1c2VwcGUubm9jZW50aW5pQHVuaXBnLml0