- 1Department of Anesthesiology and Perioperative Medicine, Xijing Hospital, Air Force Medical University, Xi’an, China
- 2Department of Anesthesiology, Tianjin Chest Hospital, Tianjin, China
- 3Department of Anesthesiology and Perioperative Medicine, Henan Provincial People’s Hospital, People’s Hospital of Zhengzhou University, Zhengzhou, China
Background: The neuroprotective effect of remote ischemic preconditioning (RIPC) in patients undergoing elective cardiopulmonary bypass (CPB)-assisted coronary artery bypass graft (CABG) or valvular cardiac surgery remains unclear.
Methods: A randomized, double-blind, placebo-controlled superior clinical trial was conducted in patients undergoing elective on-pump coronary artery bypass surgery or valve surgery. Before anesthesia induction, patients were randomly assigned to RIPC (three 5-min cycles of inflation and deflation of blood pressure cuff on the upper limb) or the control group. The primary endpoint was the changes in S-100 calcium-binding protein β (S100-β) levels at 6 h postoperatively. Secondary endpoints included changes in Neuron-specific enolase (NSE), Mini-mental State Examination (MMSE), and Montreal Cognitive Assessment (MoCA) levels.
Results: A total of 120 patients [mean age, 48.7 years; 36 women (34.3%)] were randomized at three cardiac surgery centers in China. One hundred and five patients were included in the modified intent-to-treat analysis (52 in the RIPC group and 53 in the control group). The primary result demonstrated that at 6 h after surgery, S100-β levels were lower in the RIPC group than in the control group (50.75; 95% confidence interval, 67.08 to 64.40 pg/ml vs. 70.48; 95% CI, 56.84 to 84.10 pg/ml, P = 0.036). Compared to the control group, the concentrations of S100-β at 24 h and 72 h and the concentration of NSE at 6 h, 24 h, and 72 h postoperatively were significantly lower in the RIPC group. However, neither the MMSE nor the MoCA revealed significant between-group differences in postoperative cognitive performance at 7 days, 3 months, and 6 months after surgery.
Conclusion: In patients undergoing CPB-assisted cardiac surgery, RIPC attenuated brain damage as indicated with the decreased release of brain damage biomarker S100-β and NSE.
Clinical trial registration: [ClinicalTrials.gov], identifier [NCT01231789].
Introduction
Neurological and neurobehavioral disorders are common complications after cardiopulmonary bypass (CPB)-assisted cardiac surgeries, including delirium, postoperative cognitive decline (POCD), and dementia. Postoperative cognitive dysfunction (POCD) is a common clinical complication with impacts on a wide array of cognitive domains, including attention, memory, executive function, and information processing speed (1). According to a recent meta-analysis, the prevalence of POCD following cardiac surgery was 28% between the first and fourth months postoperatively and 22% between the sixth and twelve months postoperatively (2), which is associated with disability and a poor postoperative quality of life (3–5). In addition, early cognitive decline following cardiac surgery may persist or become permanent, posing a significant risk of morbidity and mortality (6–8). Nonetheless, how to mitigate early-phase postoperative cognitive decline after cardiac surgery remains a major clinical challenge.
Remote ischemic preconditioning (RIPC) is a low-cost, non-invasive, and easy-to-use technique for attenuating ischemic organ injury by inducing transient sublethal episodes of ischemia and reperfusion in non-vital tissues (e.g., skeletal muscles), thereby enabling remote vital organs to tolerate a subsequent prolonged ischemic event (9–11). Some proof-of-concept trials demonstrated the cardiac and renal protective effects of RIPC in patients undergoing cardiovascular surgeries (12–15). RIPC is clearly a systemic phenomenon (11), which involves the activation of humoral and neuronal signaling pathways (16) and induces the release of circulating protective cells (17) and protective soluble factors (18–23). RIPC was reported to effectively delay the onset of cognitive decline in individuals with neurological comorbidities such as subcortical ischemic vascular dementia, cerebral small-vessel disease, and ischemic stroke (24–26). However, the efficacy of RIPC on neuroprotection in patients undergoing cardiovascular surgery remains controversial. Two studies failed to verify the neuroprotective effects of remote ischemic preconditioning in attenuating postoperative cognitive impairment in patients undergoing heart surgeries (27, 28), whether on CPB or not. In contrast, Hudetz et al. reported in a pilot study that RIPC prevented short-term deterioration of cognitive function after cardiac surgery (29).
Peripheral blood biomarkers convey information on a variety of pathological states (30). An elevated serum S-100 calcium-binding protein β (S100-β) level was associated with the severity of the brain injury (31), while the elevation of Neuron-specific enolase (NSE) indicated acute ischemic brain injury (32). Serum NSE and S100-β levels were also reported to be elevated in patients with neurological complications following cardiac surgeries (33). The 2 most commonly studied blood-based biomarkers of brain injury after cardiac arrest are S100-β and neuron-specific enolase (NSE) (34–36).
Therefore, the objective of the current study was to test the hypothesis that RIPC, as compared to standard care, attenuates brain injury as measured by the dynamic changes of S100-β and NSE in patients undergoing CPB-assisted CABG or valve surgery. In addition, we sought to determine if RIPC ameliorated postoperative neurocognitive dysfunction as assessed with Mini-mental State Examination (MMSE) and the Montreal Cognitive Assessment (MoCA).
Materials and methods
Trial design
A multi-center, parallel-group, superior, randomized controlled clinical trial was conducted in 3 cardiac surgery centers in China. The Department of Anesthesiology and Perioperative Medicine at Xijing Hospital was the trial coordinating center, which was responsible for trial design, generating randomization sequence, data verification, maintenance, and analysis. The trial protocol was approved by the Ethics Committee or Institutional Review Board of the coordinating center and the participating centers (Tianjin Chest Hospital and Henan provincial People’s Hospital). The investigation was conducted according to the Declaration of Helsinki and relevant Chinese laws. After approval by Institutional Review Board, the trial was registered on ClinicalTrials.gov (Identifier: NCT01231789). The trial was conducted in accordance with the principles of Good Clinical Practice. Data were monitored and audited by independent investigators from the coordinating center. Reporting follows the guidance of CONSORT recommendations. The full protocol with the statistical plan was attached in Supplementary material. All of the authors verify the accuracy and completeness of the data and the analysis.
Participants
Patients were included when they were 18–75 years old and scheduled for CPB-assisted CABG or valve surgery. We excluded patients with prior cardiac surgery history or those who underwent urgent or emergent surgery. Patients with hepatic dysfunction (Child-Pugh Score: Class C), pulmonary disease (forced expiratory volume in 1 s < 40% the predicted value), renal failure (estimated glomerular filtration rate < 30 ml/min/1.73 m2), or cardiac dysfunction [ejection fraction (EF) < 40%] were excluded. Patients with severe comorbidities that interfere with outcome measurement or RIPC implementation (i.e., an episode of stroke within 3 months or peripheral vascular disease affecting the upper limb) were excluded. In addition, patients with any risk factors that could influence the cognitive function evaluation were excluded. These included a lack of formal education (be educated for less than 7 years); visual or auditory impairment; preoperative cognitive dysfunction (MMSE score < 24 or MoCA score < 26); or a history of mental illness. Eligible patients were enrolled in the trial after written informed consent was provided.
Randomization, intervention, and blinding
Patients were randomized with a 1:1 ratio into RIPC or Control group. Randomization was performed centrally at the coordinating center and was stratified according to participating center. The generated randomization sequence was sealed in envelopes and sent to each site. To conceal allocation, investigators were allowed to open the envelope right before implementing of RIPC after the enrolled patient entered the pre-anesthesia preparation room. After catheterization in the left radial artery under local anesthesia for baseline blood pressure measurement, RIPC was induced by three cycles of right upper limb ischemia and reperfusion (To ensure the effect of remote ischemic preconditioning, the cuff should be inflated to high enough pressure to induce limb ischemia. Therefore, for patients with baseline systolic pressure lower than 150 mmHg, it was inflated to 200 mmHg; while for patients with comorbidities of hypertension (baseline systolic pressure higher than 150 mmHg), the cuff was inflated to 50 mmHg higher than the baseline systolic blood pressure for 5 min) by an appointed investigator in each center who was aware of the study-group allocation. For patients allocated to the control group, the blood pressure measurement cuff was inflated to a baseline diastolic pressure level for 5 min to generate a non-ischemic upper-limb compression, thus blinding the patient to the greatest extent possible. During the entire RIPC procedure, the surgical drapes were covered to blind the clinicians. Therefore, the anesthesiologist, surgeons, intensive care unit physicians, nurses, central lab personnel, and other study investigators were blinded to the treatment allocations.
Procedures
Anesthesia management, surgical procedures and perioperative management followed the institutional routine at each site. Briefly, arterial blood pressure, central venous pressure, electrocardiographic tracings, and nasopharyngeal temperature were monitored continuously. Anesthesia was induced with bolus injection of midazolam, etomidate and sufentanil and maintained with sevoflurane, sufentanil and rocuronium. Rocuronium was used to facilitate endotracheal intubation. During surgery, the bispectral index was maintained at 40–60. Surgery was performed using standard non-pulsatile CPB under mild hypothermia (core temperature range at 28–30°C). The blood cardioplegia was adopted for myocardial protection. During cardiopulmonary bypass (CPB), mean arterial pressure was maintained between 50 and 70 mmHg, and mixed venous oxygen saturation was > 65%. After the procedure and wean off the CPB, the protamine was administrated to neutralize the effect of heparin. Blood samples were collected before anesthesia induction (Time Point 1, TP1), before cardiopulmonary bypass (TP2), at the end of the surgery (TP3) and 6 h (TP4), 24 h (TP5), 48 h (TP6), 72 h (TP7) after surgery. Blood samples were inverted gently several times, allowed to clot for 30 min at room temperature, and centrifuged at 1,500 rpm for 15 min to obtain serum. Serum samples were then stored at −80°C before analysis. The S100-β and NSE were quantified in batches at the clinical central laboratory of Xijing Hospital using an electrochemiluminescence-based one-step enzyme immunoassay (Elecsys 2010; Roche Diagnostics, United Kingdom).
Outcomes
The primary endpoint was S100-β concentrations at the 6-h post-surgery. Secondary endpoints included S100-β levels at the remaining time points, the NSE levels, and postoperative neurocognitive function scores assessed with the Chinese version Mini-mental State Examination (MMSE) and Montreal Cognitive Assessment (MoCA) (37, 38). The MMSE test includes simple questions and problems in several areas: the time and place of the test, repeating lists of words, arithmetic such as the serial sevens, language use and comprehension, and basic motor skills. The MoCA test was characterized by good concurrent validity and could detect cognitive impairment in different neurological disorders, which was used to assess different types of cognitive abilities, including orientation, short-term memory or delayed recall, executive function or visuospatial ability, language abilities, abstraction, animal naming, attention and clock-drawing test. The baseline cognitive function was evaluated 1 day before the surgery. The postoperative cognitive function assessment was conducted at 7 days, 3 months, and 6 months post-surgery. When postoperative MMSE and MoCA scores were reduced more than 1 standard deviation (SD) as compared to the baseline value, POCD was diagnosed. The clinical indicators of postoperative quality of recovery (length of intensive cardiac care unit stay, emergence time, extubation time, and ventilation time) were compared between RIPC and the control group and considered an exploratory analysis.
Statistical analysis
According to our preliminary experiments (30 cases), the serum S100-β level at 6 h post-surgery was 48.12 (mean) pg/ml in the RIPC group and 66.42 (mean) pg/ml in the control group, with a pooled standard deviation of 32.5. The sample size was calculated using PASS (Product Application and Support Software, PASS 15.0). At a power of 0.8 and a two-sided significance level of 0.05, at least 51 patients were required in each intervention group. Finally, 120 patients were enrolled for randomization to account for possible dropouts. The continuous variables were expressed as mean and standard deviation (SD) or median (interquartile range) as appropriate. The discrete variables were presented as frequencies and percentages. Baseline characteristics and the clinical outcome of the patients in both RIPC and control groups were compared via the independent t test, chi-square test or Fisher exact tests as appropriate. Analysis of covariance was adopted for the primary outcome analysis with the treatment group and randomized stratification parameters were used as factors, with baseline values serving as covariates. The primary analysis was based on a modified intention-to-treat (mITT) principle. No imputation was performed for missing data.
For the secondary outcomes, considering that the measures were taken repetitively, the effects of the intervention on S100-β, NSE, MMSE and MoCA, respectively, were analyzed with linear mixed effect models. The response variables were S100-β, NSE, MMSE and MoCA, respectively, with the grouping information, measurement times and their interactions as explanatory factors. The log-transformation was applied to S100-β, NSE, MMSE and MoCA to adjust for the skewness in variable distributions. The impact of different interventions on the primary endpoint (S100-β level at the 6 h post-surgery) was revealed on the adjustment of random variations for different study subjects and time points. Similar logic was followed in interpretation of secondary responses including S100-β at all times measured, NSE, MMSE and MoCA. Adjusting for temporal trends and subject random intercepts, the grouping is shown to affect both the S100-β and NSE levels. The measurements for MMSE and MoCA are majorly different among individuals and fluctuate with times considered. The inclusion of fixed effects and random effects is determined upon comparison of nested models. This is in line with the step-wise variable selection procedure with the most optimal fit indicated by the Akaike Information Criterion (AIC). Missing data for secondary endpoints were imputed using the Last Observation Carried Forward method.
Post hoc analyses for the primary endpoint were performed in subgroups according to the types of cardiac surgery and age. No adjustments were conducted for the multiple comparisons in the post hoc analyses. Thus, they were considered exploratory. No imputation was performed for missing data in post hoc analyses. Two-sided hypothesis tests at a 5% significance level are carried out throughout this study. All statistical analyses were performed using R software (version 4.1.2, R Foundation for Statistical Computing, Vienna, Austria).
Results
Study population
From June 2009 to August 2013, 150 patients were screened. Among them, 30 patients were ineligible due to factors such as a prior cardiac surgery history (n = 11), peripheral vascular disease (n = 12), hepatic dysfunction (n = 1), visual or auditory disorder (n = 2), or refusal refused to participate (n = 4). Final randomization of 120 patients scheduled for elective on-pump CABG or valvular surgery. Fifteen patients were excluded from the final data analysis based on the modified ITT, due to unplanned repetition of CPB, change of surgery procedure, the withdrawal of informed consent, and contaminated blood samples. Ultimately, 53 patients in the control group and 52 in the RIPC group completed the follow-up evaluation (Figure 1). The clinical and baseline characteristics of the 105 patients were presented in Table 1. There were no statistically significant differences in all baseline characteristics. The mean age was 48.6 years (SD 11.3) in the RIPC group and 49.0 years (SD 12.7) in the control group. Female patients accounted for 37.3% in the RIPC and 32.1% in the control group. The rates of comorbidities, medication history, operation type, CPB duration, and aortic cross-clamping time were comparable between the two groups.
Primary outcomes
Elevated serum level of brain-derived proteins S-100 calcium-binding protein B (S100-β) was used as an early marker of cerebral damage. In this study, the preoperative baseline S100-β levels were within the normal range: 13.95 [95% confidence interval (CI), 11.64–16.26] pg/ml in the RIPC group and 12.40 (95% CI, 10.09–14.71) pg/ml in the control group (p = 0.333). At 6 h after surgery, S100-β was significantly lower in the RIPC group than that in the control group [43.17 (95% CI, 37.07–49.27) pg/ml in the RIPC group vs 77.92 (95% CI, 46.85–108.99) pg/ml in the control group, p = 0.035]. After adjusting for S100-β baseline levels, the least squares means of the two groups remained statistically different[50.75 (95% CI, 37.08–64.40) pg/ml in the RIPC group and 70.48 (95% CI, 56.84–84.10) pg/ml in the control group, p = 0.036] (Table 2).
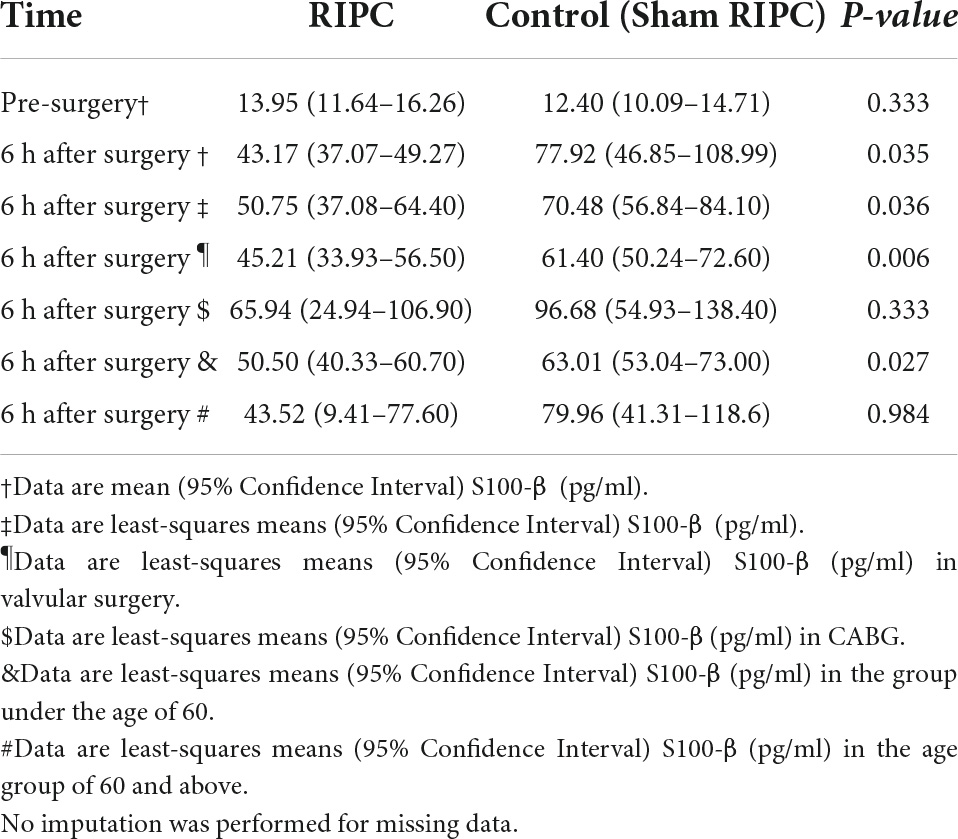
Table 2. Differences in S100-β at 6 h post-surgery between remote ischemic preconditioning and control groups.
Secondary outcomes
In both groups, there was a transient increase in S100-β at the end of surgery (TP3). However, the serum level of S100-β in the RIPC group was significantly lower at the end of the surgery, 6 h and 24 h compared to that of the control group (P < 0.05) (Table 3 and Figure 2A). After adjusting for the variation in different time points and individual participants, the concentration of S100-β in the control group remained greater than that of the RIPC group. Similarly, a temporary raise in NSE was detected in both groups shortly following surgery. Subsequently, the NSE in the RIPC group was significantly lower from 6 h to 72 h following surgery (Table 3 and Figure 2B). After adjusting for the variation of time points and individual participants, the level of NSE in the RIPC remained significantly lower than that of the control group. There was an interaction between intervention and time in the change of NSE, whereas the interaction effects were not observed for the change of S100-β concentration (Table 4). Patients were followed up for 6 months, and their MoCA and MMSE scores were obtained at 4-time points. MoCA and MMSE exhibited significant temporal patterns (Table 4), but there is no indication of between-group differences (Table 5 and Figure 3). MMSE and MoCA scores at baseline had standard deviations of 1.545 and 2.605, respectively. Figure 3 depicted cognitive function differences between RIPC and control groups. Four patients in the control group and five in the RIPC group experienced postoperative cognitive dysfunction (POCD) within 7 days following surgery. Fortunately, all nine patients recovered cognitive function at 3 or 6 months. Figure 4 depicted other clinical outcomes (cardiac intensive care unit length of stay, time to emergence and extubation, and duration of mechanic ventilation) in each treatment arm. No significant differences were found between these two groups. Additional post hoc analysis revealed significantly reduced S100-β concentration at 6 h postoperatively in the RIPC group compared to that in the control group in the valvular surgery subgroup, but not in the CABG subgroup (Table 2).
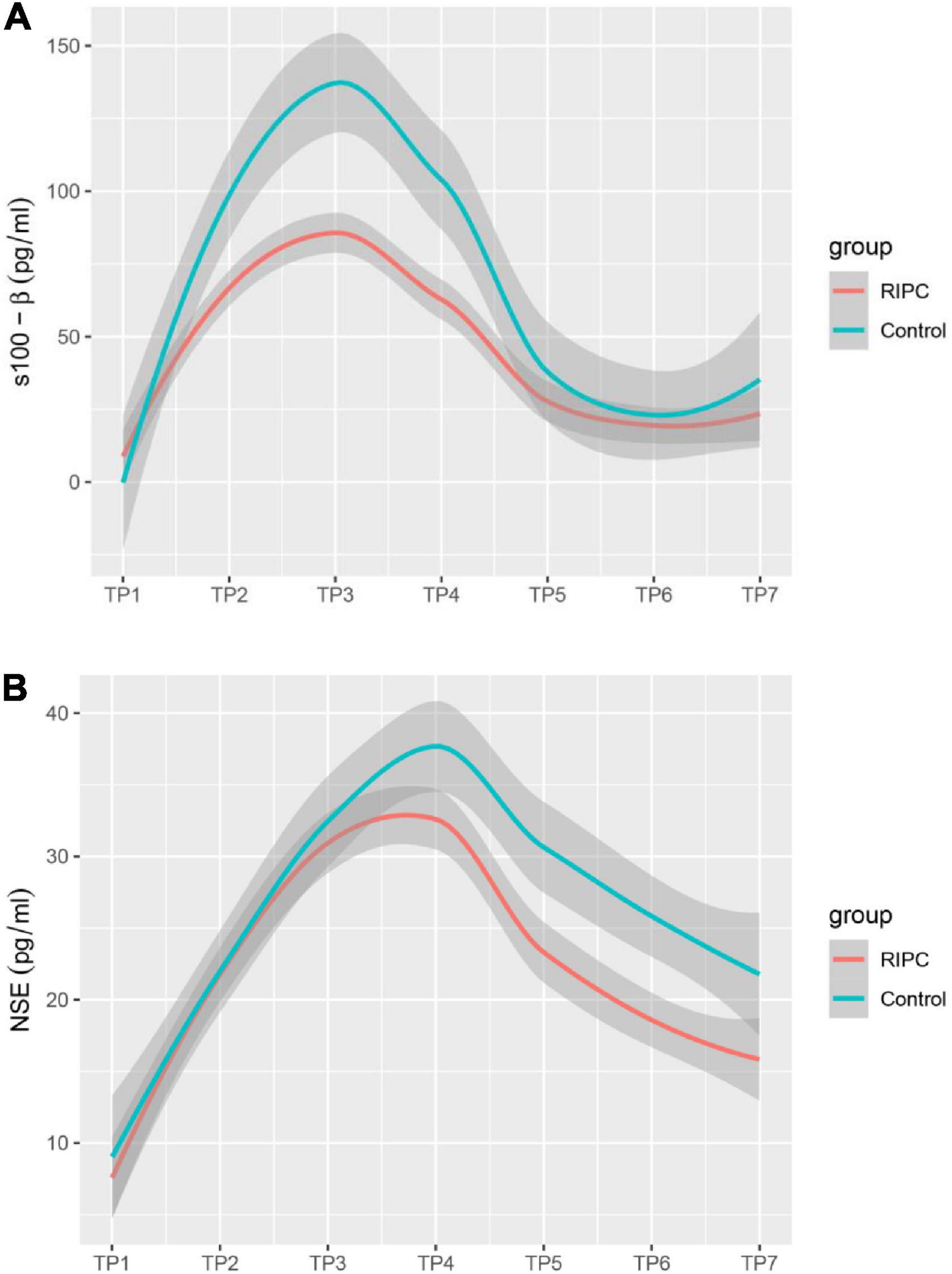
Figure 2. Estimated change trend in brain injury markers between RIPC group and control group across time points. S100-β (A); S-100 calcium-binding protein B. NSE (B); neuron specific enolase. Shaded areas indicate 95% confidence interval.
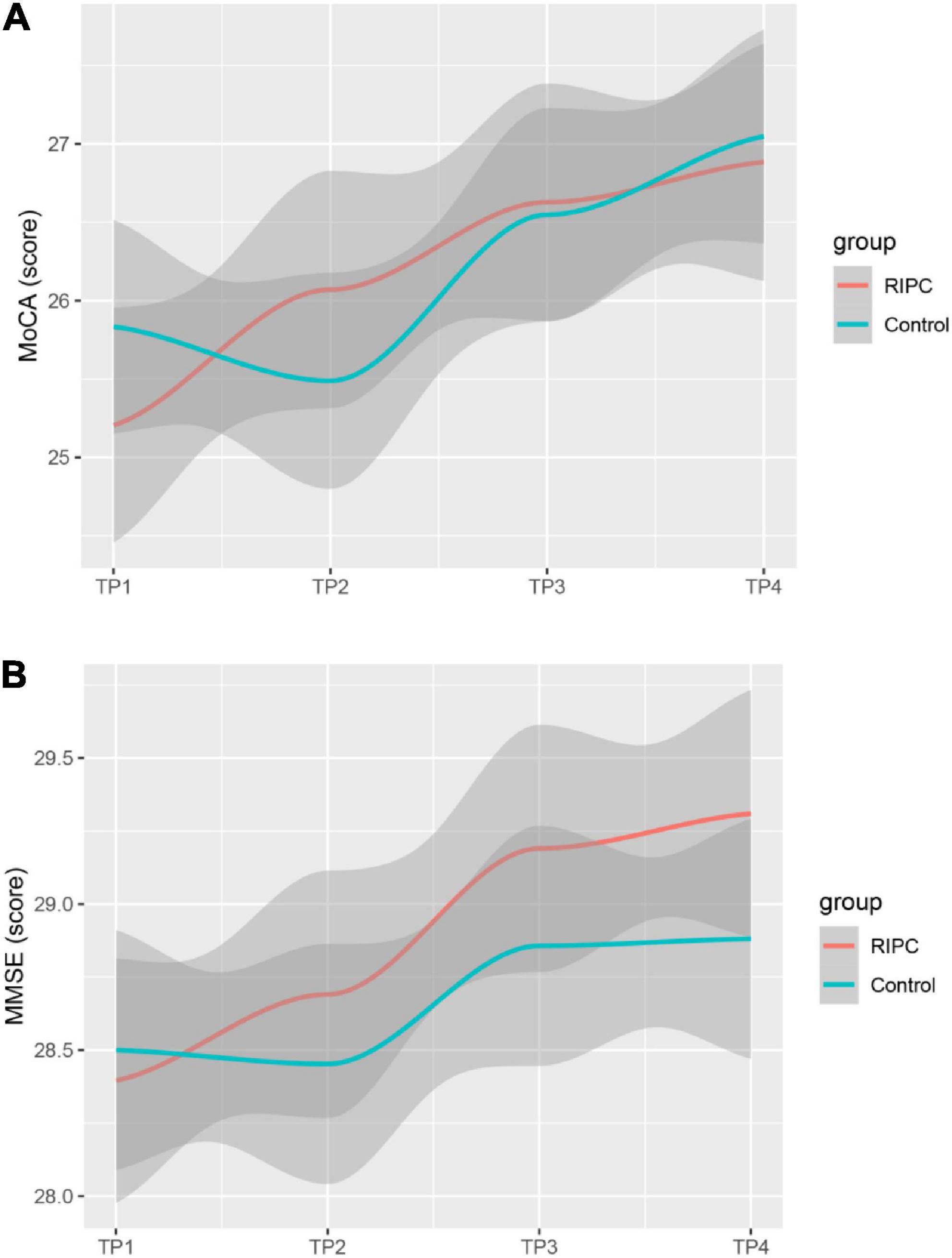
Figure 3. Estimated change trend in postoperative cognitive function between RIPC group and Control group across time points. Shaded areas indicate 95% confidence interval. MoCA (A); Montreal Cognitive Assessment. MMSE (B); Mini-mental State Examination.
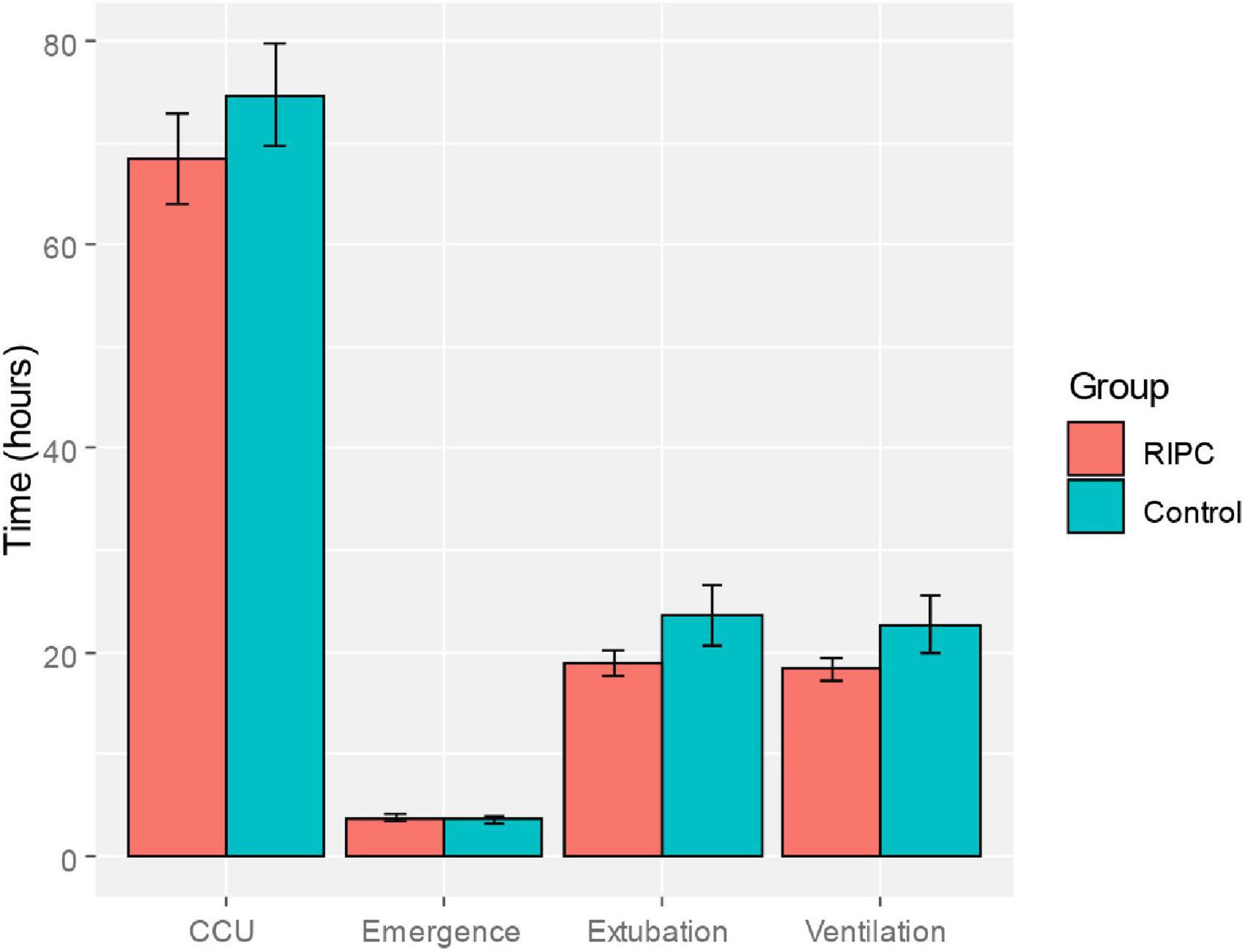
Figure 4. Comparison of clinical indicators of postoperative recovery (length of intensive cardiac care unit stay, emergence time, extubation time and ventilation time) between the RIPC group and the control group.
Discussion
In high-risk patients undergoing on-pump CABG and valve surgery, remote ischemic preconditioning applied prior to cardiac surgery attenuated neurological damage following surgery compared to the sham preconditioning, as indicated by the serum biomarkers. However, remote ischemic preconditioning did not affect the postoperative cognitive function and other clinical outcomes as compared with sham preconditioning.
RIPC exhibits neuroprotective effects in animal models of cerebral ischemia, it was reported that RIPC enhanced the recovery of cortical neuronal activity, preserved cerebral oxygen pressure and reduced cortical damage following hypothermic circulatory arrest (39–41). Nonetheless, two previous clinical trials exploring the neuroprotective effects of RIPC in patients undergoing cardiac surgery revealed disappointing results (27, 28). Using established test batteries, Meybohm et al. (28) tested cognitive function in patients undergoing cardiac surgery. The diagnosis of postoperative cognitive dysfunction was based on the standard deviation or composite z score in two or more cognitive domains in order to evaluate the changes in cognitive function of patients before and after surgery. This study failed to demonstrate that remote ischemic preconditioning had a protective effect on cognitive function after cardiac surgery. However, a summarized z-score analysis revealed a trend toward a greater decline in cognitive function in the sham control group. In addition, in this study (28), the demographics were significantly different in the remote ischemic preconditioning group and the control group. The participants allocated in the RIPC group were more likely to be elderly, female, had diabetes or hypertension, or received beta-blockers. Unbalanced baseline data may be one of the possible explanations for these unfavorable results. By using 6 neuropsychometric tests, including (language learning, forward and reverse digit span, digit sign substitution, trajectory making parts A and B), Joung et al. (27) assessed the protective effect of remote ischemic preconditioning on postoperative cognitive function in patients undergoing off-pump CABG. Cognitive impairment was defined as a decrease of more than 20% in scores on two or more tests 1 week after surgery relative to preoperative scores. According to these criteria, there was no significant difference between the RIPC group and the control group in the incidence of postoperative cognitive impairment. Why did RIPC fail to provide neuroprotective effects for patients undergoing cardiac surgery in these two clinical trials? It is possible that regular perioperative management is facilitated by availability guidelines and has been refined in cardiac surgery, yet certain protective measures, such as cardiopulmonary bypass, hypothermia and bispectral index monitoring are clinical routine. Therefore, additional protection exerted from RIPC is difficult to detect. In addition, co-morbidities and co-medication as confounders for cardioprotection may interfere with the effect of RIPC, aortic cross-clamp time duration to make the efficacy of protection more evident and propofol to interfere with RIPC in patients undergoing cardiovascular surgery. However, the capacity of clinical investigations to identify a confounding impact of a single co-morbidity or co-medication is poor, and the amount of clinical data available for ischemic and pharmacological conditioning is limited (42).
The secondary endpoints of our study were consistent with the above-mentioned two trials, the incidence of POCD and long-term cognitive function were not significantly different between the RIPC and the control group. However, early postoperative moderate neurological damage was difficult to detect and was associated with poor long-term outcomes (6–8). As a result, we chose biomarkers of brain injury as the surrogate primary outcome measure. Serum and cerebral-spinal fluid levels of neuron-specific or astrocyte-specific proteins can be used to gauge the severity of central nervous system injury. S100-β and NSE are two of the most powerful diagnostic markers for brain injury among the various injury biomarkers. S100-β and NSE levels were elevated in the early phase of neuronal damage and were linked with subsequent cognitive impairment (43). Consistent with our findings, RIPC significantly limited the release of serum S100-β and NSE at 6 h and 1 day after elective cervical decompression surgery (44). However, there was no difference between RIPC and control groups in terms of S100-β and NSE levels in patients with aneurysmal subarachnoid hemorrhage or severe Carotid artery stenosis following surgery (45, 46). We then speculated that the neuroprotective effect of RIPC is surgery-type dependent. The severity of pre-existing brain injury, such as more invasive cerebrovascular surgery, may disguise the neuroprotective effect of RIPC. Additional post hoc analysis found that RIPC was ineffective at reducing S100-β levels 6 h after surgery in the CABG subgroup (p = 0.333). Similar to our findings, two large-scale trials have shown no benefits of applying RIPC before CABG surgery (47, 48).
The translation of remote ischemic conditioning to patients remains challenging (49). Experimental studies on the long-term effects of adjunctive cardioprotection beyond reducing infarct size, namely on repair, remodeling, and mortality are lacking. Adequate phase II dosing and timing studies are required when rushing from promising proof-of-concept trials to larger clinical outcome trials. Severe flaws in the design and conduct of clinical trials have also largely contributed to the failure to translate cardioprotection into clinical practice (50, 51). In addition, the clinical translation of RIPC to improve cognitive function after cardiac surgery also faces difficulties. In the present study, there was no significant difference in long-term postoperative cognitive function and other clinical outcomes between the RIPC and control groups. Contrary to expectations, although lowering the release of neurological damage biomarkers, RIPC appeared to have little effect on the clinical outcomes after cardiac surgery. Similarly, two adequately powered clinical trials failed to demonstrate any beneficial effect of RIPC on clinical outcomes in pediatric (52) and adult (53) patients. In our trial, we revealed that RIPC dramatically lowered the rise of S100-β and NSE, these two prognostic markers have been shown to correlate with the severity of cognitive dysfunction after cardiac surgery. Consequently, it is reasonable to conclude that RIPC can ameliorate postoperative cognitive dysfunction to some extent; however, the effect of RIPC may not be strong enough to alter the long-term cognitive outcomes. Additionally, age is a leading risk factor associated with postoperative cognitive dysfunction after cardiac surgery, older patients are more susceptible to postoperative cognitive dysfunction than younger patients (54, 55). In the current study, the average age of the participants in the current study was 48 years, indicating a relatively lower-risk patient population. Patients were separated into two strata: non-geriatric patients (age < 60 years) and geriatric patients (age ≥ 60 years) in the post hoc subgroup analysis. The effect modification was assessed by including an interaction term between age and trial group in the analysis. It was demonstrated that RIPC lowered the level of S100 β at 6 h after surgery in both age subgroups. The decrease in S100 β was not statistically different between the age ≥ 60 years subgroup. However, spurious negative effect modification effects of age may arise regrading the relatively small sample size.
Our study has some limitations. Firstly, only 105 patients were included in the final analysis, which is a relatively small sample size despite having sufficient statistical power. In the post hoc analysis, RIPC was ineffective at reducing S100-β levels at 6 h after surgery in the CABG subgroup, which was likely due to the small sample size and insufficient power of this subgroup. Second, the incidence of delirium was not assessed in our study. The symptoms of delirium may occur prior and are closely related to POCD. Third, as the RIPC was performed prior to anesthesia, the patient could perceive the cuff’s inflation and pressure. We did our utmost to blind the patient, but we cannot rule out the possibility that he or she could figure out the difference in inflation pressure through communications with other enrolled patients. However, since the primary endpoint was the S100-β concentration, which was measured by the blinded clinical central lab personnel and was relatively objective, thus the concerns regarding placebo effects were considerably reduced. Fourth, the choice of serum biomarkers as the primary endpoint may affect the extrapolation of study results. As the beneficial effect of RIPC on surrogate outcomes may not immediately translate into the clinical outcomes that are important to patients (56, 57). Lastly, the included patients are a historical collective, beyond that, there are no follow-up data beyond the 6 months.
Conclusion
In conclusion, in our multicenter randomized clinical trial, upper-limb RIPC reduced the postoperative elevation of brain injury biomarkers S100-β and NSE in patients undergoing elective CPB-assisted coronary artery bypass grafting or valve surgery. However, there was no significant difference in postoperative cognitive performance between RIPC and the control group at 7 days, 3 months, and 6 months after surgery.
Data availability statement
The original contributions presented in this study are included in the article/Supplementary material, further inquiries can be directed to the corresponding author/s.
Ethics statement
The studies involving human participants were reviewed and approved by the Ethics Committee of Xijing Hospital (No: XJIRB-20090625-2). The patients/participants provided their written informed consent to participate in this study.
Author contributions
SZ contributed to the drafting the article. CL and HD contributed to the conception and design of the study. WL, PO, JH, and JZ contributed to the data acquisition. SZ and ZZ contributed to the analysis and interpretation of data. CL contributed to the reviewing final manuscript. All authors contributed to the article and approved the submitted version.
Funding
This work was supported by the National Natural Science Foundation of China (NNSFC, No. 81970448), the National Key Research and Development Program of China, the Ministry of Science and Technology of China (2017YFC1308402 and 2018YFC1106504), and the Special Fund for Clinical Medical Research, Chinese Medical Association.
Acknowledgments
We acknowledge the operating room staff who contributed to this research. We would like to thank Professor Lize Xiong (Department of Anesthesiology and Perioperative Medicine, Shanghai Fourth People’s Hospital Affiliated to Tongji University School of Medicine, Translational Research Institute of Brain and Brain-Like Intelligence Affiliated to Tongji University School of Medicine, Shanghai, China) for constructive suggestions.
Conflict of interest
The authors declare that the research was conducted in the absence of any commercial or financial relationships that could be construed as a potential conflict of interest.
Publisher’s note
All claims expressed in this article are solely those of the authors and do not necessarily represent those of their affiliated organizations, or those of the publisher, the editors and the reviewers. Any product that may be evaluated in this article, or claim that may be made by its manufacturer, is not guaranteed or endorsed by the publisher.
Supplementary material
The Supplementary Material for this article can be found online at: https://www.frontiersin.org/articles/10.3389/fcvm.2022.952033/full#supplementary-material
References
1. Phillips-Bute B, Mathew JP, Blumenthal JA, Grocott HP, Laskowitz DT, Jones RH, et al. Association of neurocognitive function and quality of life 1 year after coronary artery bypass graft (CABG) surgery. Psychosom Med. (2006) 68:369–75. doi: 10.1097/01.psy.0000221272.77984.e2
2. Greaves D, Psaltis PJ, Ross TJ, Davis D, Smith AE, Boord MS, et al. Cognitive outcomes following coronary artery bypass grafting: a systematic review and meta-analysis of 91,829 patients. Int J Cardiol. (2019) 289:43–9. doi: 10.1016/j.ijcard.2019.04.065
3. Brown CHT, Probert J, Healy R, Parish M, Nomura Y, Yamaguchi A, et al. Cognitive Decline after Delirium in Patients Undergoing Cardiac Surgery. Anesthesiology. (2018) 129:406–16. doi: 10.1097/aln.0000000000002253
4. Li HC, Wei YC, Hsu RB, Chi NH, Wang SS, Chen YS, et al. Surviving and thriving 1 year after cardiac surgery: frailty and delirium matter. Ann Thorac Surg. (2021) 111:1578–84. doi: 10.1016/j.athoracsur.2020.07.015
5. Labaste F, Porterie J, Bousquet P, Marcheix B, Sanchez-Verlaan P, Frances B, et al. Postoperative delirium is a risk factor of poor evolution three years after cardiac surgery: an observational cohort study. Clin Interv Aging. (2020) 15:2375–81. doi: 10.2147/cia.S265797
6. Roach GW, Kanchuger M, Mangano CM, Newman M, Nussmeier N, Wolman R, et al. Adverse cerebral outcomes after coronary bypass surgery. Multicenter Study of Perioperative Ischemia Research Group and the Ischemia Research and Education Foundation Investigators. N Engl J Med. (1996) 335:1857–63. doi: 10.1056/nejm199612193352501
7. Newman MF, Kirchner JL, Phillips-Bute B, Gaver V, Grocott H, Jones RH, et al. Longitudinal assessment of neurocognitive function after coronary-artery bypass surgery. N Engl J Med. (2001) 344:395–402. doi: 10.1056/nejm200102083440601
8. Dijkstra JB, Houx PJ, Jolles J. Cognition after major surgery in the elderly: test performance and complaints. Br J Anaesth. (1999) 82:867–74. doi: 10.1093/bja/82.6.867
9. Heusch G. Myocardial ischaemia-reperfusion injury and cardioprotection in perspective. Nat Rev Cardiol. (2020) 17:773–89. doi: 10.1038/s41569-020-0403-y
10. Heusch G, Bøtker HE, Przyklenk K, Redington A, Yellon D. Remote ischemic conditioning. J Am Coll Cardiol. (2015) 65:177–95. doi: 10.1016/j.jacc.2014.10.031
11. Kleinbongard P, Skyschally A, Heusch G. Cardioprotection by remote ischemic conditioning and its signal transduction. Pflugers Arch. (2017) 469:159–81. doi: 10.1007/s00424-016-1922-6
12. Bøtker HE, Kharbanda R, Schmidt MR, Bøttcher M, Kaltoft AK, Terkelsen CJ, et al. Remote ischaemic conditioning before hospital admission, as a complement to angioplasty, and effect on myocardial salvage in patients with acute myocardial infarction: a randomised trial. Lancet. (2010) 375:727–34. doi: 10.1016/s0140-6736(09)62001-8
13. Gaspar A, Lourenço AP, Pereira M, Azevedo P, Roncon-Albuquerque R Jr., Marques J, et al. Randomized controlled trial of remote ischaemic conditioning in ST-elevation myocardial infarction as adjuvant to primary angioplasty (RIC-STEMI). Basic Res Cardiol. (2018) 113:14. doi: 10.1007/s00395-018-0672-3
14. Kleinbongard P, Peters J, Jakob H, Heusch G, Thielmann M. Persistent survival benefit from remote ischemic pre-conditioning in patients undergoing coronary artery bypass surgery. J Am Coll Cardiol. (2018) 71:252–4. doi: 10.1016/j.jacc.2017.10.083
15. Thielmann M, Kottenberg E, Kleinbongard P, Wendt D, Gedik N, Pasa S, et al. Cardioprotective and prognostic effects of remote ischaemic preconditioning in patients undergoing coronary artery bypass surgery: a single-centre randomised, double-blind, controlled trial. Lancet. (2013) 382:597–604. doi: 10.1016/s0140-6736(13)61450-6
16. Lieder HR, Kleinbongard P, Skyschally A, Hagelschuer H, Chilian WM, Heusch G. Vago-splenic axis in signal transduction of remote ischemic preconditioning in pigs and rats. Circ Res. (2018) 123:1152–63. doi: 10.1161/circresaha.118.313859
17. Lieder HR, Tsoumani M, Andreadou I, Schrör K, Heusch G, Kleinbongard P. Platelet-mediated transfer of cardioprotection by remote ischemic conditioning and its abrogation by aspirin but not by ticagrelor. Cardiovasc Drugs Ther. (2022). [Epub ahead of print]. doi: 10.1007/s10557-022-07345-9
18. Shimizu M, Tropak M, Diaz RJ, Suto F, Surendra H, Kuzmin E, et al. Transient limb ischaemia remotely preconditions through a humoral mechanism acting directly on the myocardium: evidence suggesting cross-species protection. Clin Sci. (2009) 117:191–200. doi: 10.1042/cs20080523
19. Jensen RV, Støttrup NB, Kristiansen SB, Bøtker HE. Release of a humoral circulating cardioprotective factor by remote ischemic preconditioning is dependent on preserved neural pathways in diabetic patients. Basic Res Cardiol. (2012) 107:285. doi: 10.1007/s00395-012-0285-1
20. Hildebrandt HA, Kreienkamp V, Gent S, Kahlert P, Heusch G, Kleinbongard P. Kinetics and Signal Activation Properties of Circulating Factor(s) From Healthy Volunteers Undergoing Remote Ischemic Pre-Conditioning. JACC Basic Transl Sci. (2016) 1:3–13. doi: 10.1016/j.jacbts.2016.01.007
21. Skyschally A, Kleinbongard P, Lieder H, Gedik N, Stoian L, Amanakis G, et al. Humoral transfer and intramyocardial signal transduction of protection by remote ischemic perconditioning in pigs, rats, and mice. Am J Physiol Heart Circ Physiol. (2018) 315:H159–72. doi: 10.1152/ajpheart.00152.2018
22. Lieder HR, Skyschally A, Heusch G, Kleinbongard P. Plasma from remotely conditioned pigs reduces infarct size when given before or after ischemia to isolated perfused rat hearts. Pflugers Arch. (2019) 471:1371–9. doi: 10.1007/s00424-019-02314-y
23. Lieder HR, Tüller P, Braczko F, Zandi A, Kamler M, Thielmann M, et al. Bioassays of humoral cardioprotective factors released by remote ischemic conditioning in patients undergoing coronary artery bypass surgery. J Cardiovasc Pharmacol Ther. (2022) 27:10742484221097273. doi: 10.1177/10742484221097273
24. Liao Z, Bu Y, Li M, Han R, Zhang N, Hao J, et al. Remote ischemic conditioning improves cognition in patients with subcortical ischemic vascular dementia. BMC Neurol. (2019) 19:206. doi: 10.1186/s12883-019-1435-y
25. Wang Y, Meng R, Song H, Liu G, Hua Y, Cui D, et al. Remote ischemic conditioning may improve outcomes of patients with cerebral small-vessel disease. Stroke. (2017) 48:3064–72. doi: 10.1161/strokeaha.117.017691
26. Landman TRJ, Schoon Y, Warlé MC, de Leeuw FE, Thijssen DHJ. Remote ischemic conditioning as an additional treatment for acute ischemic stroke. Stroke. (2019) 50:1934–9. doi: 10.1161/strokeaha.119.025494
27. Joung KW, Rhim JH, Chin JH, Kim WJ, Choi DK, Lee EH, et al. Effect of remote ischemic preconditioning on cognitive function after off-pump coronary artery bypass graft: a pilot study. Korean J Anesthesiol. (2013) 65:418–24. doi: 10.4097/kjae.2013.65.5.418
28. Meybohm P, Renner J, Broch O, Caliebe D, Albrecht M, Cremer J, et al. Postoperative neurocognitive dysfunction in patients undergoing cardiac surgery after remote ischemic preconditioning: a double-blind randomized controlled pilot study. PLoS One. (2013) 8:e64743. doi: 10.1371/journal.pone.0064743
29. Hudetz JA, Patterson KM, Iqbal Z, Gandhi SD, Pagel PS. Remote ischemic preconditioning prevents deterioration of short-term postoperative cognitive function after cardiac surgery using cardiopulmonary bypass: results of a pilot investigation. J Cardiothorac Vasc Anesth. (2015) 29:382–8. doi: 10.1053/j.jvca.2014.07.012
30. Onatsu J, Vanninen R, JÄkÄlÄ P, Mustonen P, Pulkki K, Korhonen M, et al. Tau, S100B and NSE as blood biomarkers in acute cerebrovascular events. In Vivo. (2020) 34:2577–86. doi: 10.21873/invivo.12075
31. He M, Qian K, Zhang Y, Huang XF, Deng C, Zhang B, et al. Olanzapine-induced activation of hypothalamic astrocytes and toll-like receptor-4 signaling via endoplasmic reticulum stress were related to olanzapine-induced weight gain. Front Neurosci. (2020) 14:589650. doi: 10.3389/fnins.2020.589650
32. Ehrenreich H, Kästner A, Weissenborn K, Streeter J, Sperling S, Wang KK, et al. Circulating damage marker profiles support a neuroprotective effect of erythropoietin in ischemic stroke patients. Mol Med. (2011) 17:1306–10. doi: 10.2119/molmed.2011.00259
33. Johnsson P, Lundqvist C, Lindgren A, Ferencz I, Alling C, Ståhl E. Cerebral complications after cardiac surgery assessed by S-100 and NSE levels in blood. J Cardiothorac Vasc Anesth. (1995) 9:694–9. doi: 10.1016/s1053-0770(05)80231-9
34. Rossetti AO, Rabinstein AA, Oddo M. Neurological prognostication of outcome in patients in coma after cardiac arrest. Lancet Neurol. (2016) 15:597–609. doi: 10.1016/s1474-4422(16)00015-6
35. Stammet P, Collignon O, Hassager C, Wise MP, Hovdenes J, Åneman A, et al. Neuron-specific enolase as a predictor of death or poor neurological outcome after out-of-hospital cardiac arrest and targeted temperature management at 33°C and 36°C. J Am Coll Cardiol. (2015) 65:2104–14. doi: 10.1016/j.jacc.2015.03.538
36. Stammet P, Dankiewicz J, Nielsen N, Fays F, Collignon O, Hassager C, et al. Protein S100 as outcome predictor after out-of-hospital cardiac arrest and targeted temperature management at 33 °C and 36 °C. Crit Care. (2017) 21:153. doi: 10.1186/s13054-017-1729-7
37. Folstein MF, Folstein SE, McHugh PR. “Mini-mental state”. A practical method for grading the cognitive state of patients for the clinician. J Psychiatr Res. (1975) 12:189–98. doi: 10.1016/0022-3956(75)90026-6
38. Nasreddine ZS, Phillips NA, Bédirian V, Charbonneau S, Whitehead V, Collin I, et al. The montreal cognitive assessment, MoCA: a brief screening tool for mild cognitive impairment. J Am Geriatr Soc. (2005) 53:695–9. doi: 10.1111/j.1532-5415.2005.53221.x
39. Jensen HA, Loukogeorgakis S, Yannopoulos F, Rimpiläinen E, Petzold A, Tuominen H, et al. Remote ischemic preconditioning protects the brain against injury after hypothermic circulatory arrest. Circulation. (2011) 123:714–21. doi: 10.1161/circulationaha.110.986497
40. Yannopoulos FS, Mäkelä T, Niemelä E, Tuominen H, Lepola P, Alestalo K, et al. Improved cerebral recovery from hypothermic circulatory arrest after remote ischemic preconditioning. Ann Thorac Surg. (2010) 90:182–8. doi: 10.1016/j.athoracsur.2010.03.058
41. Yannopoulos F, Mäkelä T, Arvola O, Haapanen H, Anttila V, Kiviluoma K, et al. Remote ischemic precondition preserves cerebral oxygen tension during hypothermic circulatory arrest. Scand Cardiovasc J. (2012) 46:245–50. doi: 10.3109/14017431.2012.661874
42. Kleinbongard P, Bøtker HE, Ovize M, Hausenloy DJ, Heusch G. Co-morbidities and co-medications as confounders of cardioprotection-does it matter in the clinical setting? Br J Pharmacol. (2020) 177:5252–69. doi: 10.1111/bph.14839
43. Sojka P, Stålnacke BM, Björnstig U, Karlsson K. One-year follow-up of patients with mild traumatic brain injury: occurrence of post-traumatic stress-related symptoms at follow-up and serum levels of cortisol, S-100B and neuron-specific enolase in acute phase. Brain Inj. (2006) 20:613–20. doi: 10.1080/02699050600676982
44. Hu S, Dong HL, Li YZ, Luo ZJ, Sun L, Yang QZ, et al. Effects of remote ischemic preconditioning on biochemical markers and neurologic outcomes in patients undergoing elective cervical decompression surgery: a prospective randomized controlled trial. J Neurosurg Anesthesiol. (2010) 22:46–52. doi: 10.1097/ANA.0b013e3181c572bd
45. Sangeetha RP, Venkatapura RJ, Kamath S, Christopher R, Bhat DI, Arvinda HR, et al. Effect of remote ischemic preconditioning on cerebral vasospasm, biomarkers of cerebral ischemia, and functional outcomes in aneurysmal subarachnoid hemorrhage (ERVAS): a randomized controlled pilot trial. Brain Circ. (2021) 7:104–10. doi: 10.4103/bc.bc_13_21
46. Zhao W, Meng R, Ma C, Hou B, Jiao L, Zhu F, et al. Safety and efficacy of remote ischemic preconditioning in patients with severe carotid artery stenosis before carotid artery stenting: a proof-of-concept, randomized controlled trial. Circulation. (2017) 135:1325–35. doi: 10.1161/circulationaha.116.024807
47. Hausenloy DJ, Candilio L, Evans R, Ariti C, Jenkins DP, Kolvekar S, et al. Remote ischemic preconditioning and outcomes of cardiac surgery. N Engl J Med. (2015) 373:1408–17. doi: 10.1056/NEJMoa1413534
48. Meybohm P, Bein B, Brosteanu O, Cremer J, Gruenewald M, Stoppe C, et al. A multicenter trial of remote ischemic preconditioning for heart surgery. N Engl J Med. (2015) 373:1397–407. doi: 10.1056/NEJMoa1413579
49. Heusch G. Critical issues for the translation of cardioprotection. Circ Res. (2017) 120:1477–86. doi: 10.1161/circresaha.117.310820
50. Heusch G, Gersh BJ. Is cardioprotection salvageable? Circulation. (2020) 141:415–7. doi: 10.1161/circulationaha.119.044176
51. Heusch G, Rassaf T. Time to give up on cardioprotection? a critical appraisal of clinical studies on ischemic pre-, post-, and remote conditioning. Circ Res. (2016) 119:676–95. doi: 10.1161/circresaha.116.308736
52. McCrindle BW, Clarizia NA, Khaikin S, Holtby HM, Manlhiot C, Schwartz SM, et al. Remote ischemic preconditioning in children undergoing cardiac surgery with cardiopulmonary bypass: a single-center double-blinded randomized trial. J Am Heart Assoc. (2014) 3:e000964. doi: 10.1161/jaha.114.000964
53. Hong DM, Lee EH, Kim HJ, Min JJ, Chin JH, Choi DK, et al. Does remote ischaemic preconditioning with postconditioning improve clinical outcomes of patients undergoing cardiac surgery? Remote ischaemic preconditioning with postconditioning outcome trial. Eur Heart J. (2014) 35:176–83. doi: 10.1093/eurheartj/eht346
54. Soenarto RF, Mansjoer A, Amir N, Aprianti M, Perdana A. Cardiopulmonary bypass alone does not cause postoperative cognitive dysfunction following open heart surgery. Anesth Pain Med. (2018) 8:e83610. doi: 10.5812/aapm.83610
55. Bhushan S, Li Y, Huang X, Cheng H, Gao K, Xiao Z. Progress of research in postoperative cognitive dysfunction in cardiac surgery patients: a review article. Int J Surg. (2021) 95:106163. doi: 10.1016/j.ijsu.2021.106163
56. Hausenloy DJ, Mwamure PK, Venugopal V, Harris J, Barnard M, Grundy E, et al. Effect of remote ischaemic preconditioning on myocardial injury in patients undergoing coronary artery bypass graft surgery: a randomised controlled trial. Lancet. (2007) 370:575–9. doi: 10.1016/s0140-6736(07)61296-3
Keywords: neuroprotective effect, remote ischemic preconditioning, cardiac surgery, surrogate, postoperative cognitive dysfunction
Citation: Zhu S, Zheng Z, Lv W, Ouyang P, Han J, Zhang J, Dong H and Lei C (2022) Neuroprotective effect of remote ischemic preconditioning in patients undergoing cardiac surgery: A randomized controlled trial. Front. Cardiovasc. Med. 9:952033. doi: 10.3389/fcvm.2022.952033
Received: 24 May 2022; Accepted: 15 August 2022;
Published: 06 September 2022.
Edited by:
Robert Jeenchen Chen, Stanford University, United StatesReviewed by:
Puneet Randhawa, University of Central Florida, United StatesHelmut Lieder, University of Duisburg-Essen, Germany
Copyright © 2022 Zhu, Zheng, Lv, Ouyang, Han, Zhang, Dong and Lei. This is an open-access article distributed under the terms of the Creative Commons Attribution License (CC BY). The use, distribution or reproduction in other forums is permitted, provided the original author(s) and the copyright owner(s) are credited and that the original publication in this journal is cited, in accordance with accepted academic practice. No use, distribution or reproduction is permitted which does not comply with these terms.
*Correspondence: Hailong Dong, aGxkb25nNkBob3RtYWlsLmNvbQ==; Chong Lei, Y3J5c3RhbGxlaWNob25nQDEyNi5jb20=