- 1Division of Pediatric Cardiology, Department of Pediatrics, Medical University Graz, Graz, Austria
- 2Institute for Medical Informatics, Statistics and Documentation, Medical University of Graz, Graz, Austria
- 3Department of Pediatric Cardiology and Critical Care, Hannover Medical School, Hanover, Germany
- 4European Pediatric Pulmonary Vascular Disease Network, Berlin, Germany
Background: An accurate assessment of the right and left ventricle and their interaction is important in pediatric pulmonary hypertension (PH). Our objective was to provide normal reference values for the right ventricular to left ventricular endsystolic (RV/LVes) ratio and the LV endsystolic eccentricity index (LVes EI) in healthy children and in children with PH.
Methods: We conducted an echocardiographic study in 769 healthy children (median age: 3.36 years; range: 1 day—18 years) and validated abnormal values in 44 children with PH (median age: 2.1 years; range: 0.1 months—17.7 years). We determined the effects of gender, age, body length, body weight, and body surface area (BSA) on RV/LVes ratio and LVes EI values. The RV/LVes ratio and LVes EI were measured from the parasternal short axis view between papillary muscle from the endocardial to endocardial surfaces.
Results: Both, the RV/LVes ratio and the LVes EI were highly age-dependent: (i) neonates RV/LVes ratio [median 0.83 (range 0.53–1.37)], LVes EI [1.21 (0.92–1.45)]; (ii) 12–24 months old: RV/LVes ratio: [0.55 (0.35–0.80)], LVes EI: [1.0 (0.88–1.13)]; iii) 18th year of life RV/LVes ratio: [0.53 (0.32–0.74)], LVes EI: [1.0 (0.97–1.07)]. Healthy neonates had high LVes EI and RV/LVes ratios, both gradually decreased within the first year of life and until BSA values of about 0.5 m2, body weight to about 15 kg and body length to about 75 cm, but were almost constant thereafter. Children (>1 year) and adolescents with PH had significantly higher RV/LVes ratio (no PH: median 0.55, IQR 0.49–0.60; PH: 1.02, 0.87–1.26; p < 0.001) and higher LVes EI values (no PH: 1.00, 0.98–1.00; PH: 1.53, 1.26–1.71; p < 0.001) compared to those without PH. To predict the presence of PH in children > 1 year, we found the following best cutoff values: RV/LVes ratio ≥ 0.67 (sensitivity: 1.00, specificity: 0.95) and LVes EI ≥ 1.06 (sensitivity: 1.00, specificity: 0.97).
Conclusion: We provide normal echocardiographic reference values of the RV/LVes ratio and LVes EI in healthy children, as well as statistically determined cutoffs for the increased values in children with PH.
Introduction
Right ventricular (RV) and left ventricular (LV) size and function are important determinants for diagnosis, treatment and follow-up in a number of pediatric cardiovascular conditions, including pulmonary hypertension (PH) and congenital heart disease (CHD) (1–7). Because of growth throughout childhood, interpretation of these measurements requires normalization of the according variables to the size of the body. In pediatric PH research, awareness of the importance of biventricular size and function variables and ventricular-ventricular interaction (VVI) is increasing (8–16). The impact of the RV “under pressure” is also related to underfilling and dysfunction of the compressed left ventricle, which reduces LV output (13, 17). During direct interaction, the LV cavity is compressed owing to leftward bowing of the ventricular septum, causing impaired LV filling, and low cardiac output (8, 18). The echocardiographically derived RV/LV endsystolic ratio (RV/LVes ratio) and the LV endsystolic eccentricity index (LVes EI), both measured in the parasternal short axis view, are potentially useful diagnostic variables for patients with suspected PH. The RV/LVes ratio and LVes EI are the most important surrogates for disease severity when tricuspid regurgitation velocity jet is not interpretable (in about 30–54% of pediatric PH echocardiograms (15, 19–21).
The aim of the study was to determine normal reference values for RV/LVes ratios and LVes EI, and possible associations of these variables with age, body length (BL), body weight (BW), body surface area (BSA), and gender in a large healthy pediatric cohort, including neonates. This is the first study to provide data on representative, normal, pediatric RV/LVes ratios and LVes EI with according reference values and to compare such data with values obtained in a small but representative pediatric PH cohort. We hypothesized that higher RV/LVes ratios and LVes EI may become sufficient markers for the diagnosis and follow-up of children with PH when compared to normative values.
Materials and Methods
Primary Aim of the Study
We aim to establish RV/LVes ratios and LVes EI age specific normative values and reference values for healthy children (age: 1 day up to 18 years).
Healthy Study Group
The study group consisted of 769 healthy children. The study subjects were recruited from healthy children referred to the Department of Pediatrics; Medical University Graz, Austria for evaluation of a heart murmur or a family history of heart disease.
Inclusion Criteria
Age from first day of life to 18 years, male or female, echocardiograms with an official reading of “normal cardiovascular anatomy and function.” Only subjects whose physical examination was judged as being normal were included.
Exclusion Criteria
Patients with an acquired heart diseases, chest and thoracic spine deformities, chromosomal syndromes, patients with paradoxical septum motion including patients with right bundle branch block, as well as patients with hemodynamically significant patent ductus arteriosus, significant ventricular septal defect or atrial septum defect, pulmonary artery stenosis or insufficiency were excluded. Patients were examined in a resting state.
For children of the “healthy cohort,” we confirmed a normal left ventricular ejection fraction, measured using the Simpsons formula, as well as a normal RV size (22) and RV systolic function (23) by including only patients with normal age-related RV size parameters compared to respective available normative values (5, 22, 24).
Pulmonary Hypertension Study Group
The PH study group (Table 1) consisted of 44 children, including children with PH associated with congenital heart disease (PH-CHD) 48%, PH associated with bronchopulmonary dysplasia (PH-BPD) 41%, and patients with idiopathic pulmonary arterial hypertension (iPAH) 11%. The respective CHDs were surgically repaired in all patients at a mean age of 5.6 months (range: 0.6–15.3). All VSD, AVSD, and complex CHDs patients had a partial/complete bundle branch block after operation (varying from 0.10to 0.15 s QRS duration), while patients after ASD closure had narrow QRS. Patients with hemodynamic relevant conduit regurgitation or stenosis, right ventricular outflow tract obstruction, relevant intracardiac shunts, Fontan physiology, Eisenmenger Syndrome, active pacing, were excluded from the study. At time of enrolment, all patients were clinically stable without change of medications within the preceding 4 months.
Echo Protocol
Echocardiograms were performed using a commercially available echocardiographic system (Sonos iE33, Philips, Andover, Mass, United States) using transducers of 5–1, 8–3, and 12–4 MHz depending on patient age, size, and weight. Images were recorded digitally and analyzed using off-line software (Xcelera Echo; Philips Medical Systems, The Netherlands).
The RV/LVes ratios were measured from the parasternal short axis (PSAX) view at mid LV level between papillary muscle, from the endocardial to endocardial surfaces at endsystole (Figures 1A,B) as described by Jone et al. (12). The LVes EI were calculated similar to the method, first described by Ryan et al. (25) as the ratio of LV diameter parallel to the septum divided by diameter perpendicular to the septum (Figures 1A,B). However, we measured both (RV/LVes ratio and LVes EI) between the papillary muscle levels. Es was defined similar to Burkett et al. (26) as the final frame of free-wall contraction, immediately prior to outward motion (relaxation) of the LV free-wall. In PH, systole and diastole between the RV and LV can occur at different times, with RV diastolic inflow significantly delayed beyond LV inflow due to prolonged systole and/or isovolumic relaxation; this delay is related to increased afterload (8). Thus, endsystole and end-diastole can be different for each ventricle in PH, leading to confusion (8). To simplify, we used the contractile pattern of the LV to define endsystole like Vonk Noordegraaf et al. (4). In early LV diastole (outward motion of the free-wall), while the RV is still under increased pressure due to PH, there is often more profound leftward septal displacement (14). To minimize variability, a strict institutional protocol for image acquisition was used for this study. Age, BW, BL, BSA, and non-invasive blood pressure were measured at time of echocardiography, and the BSA was calculated using the Mosteller formula (27).
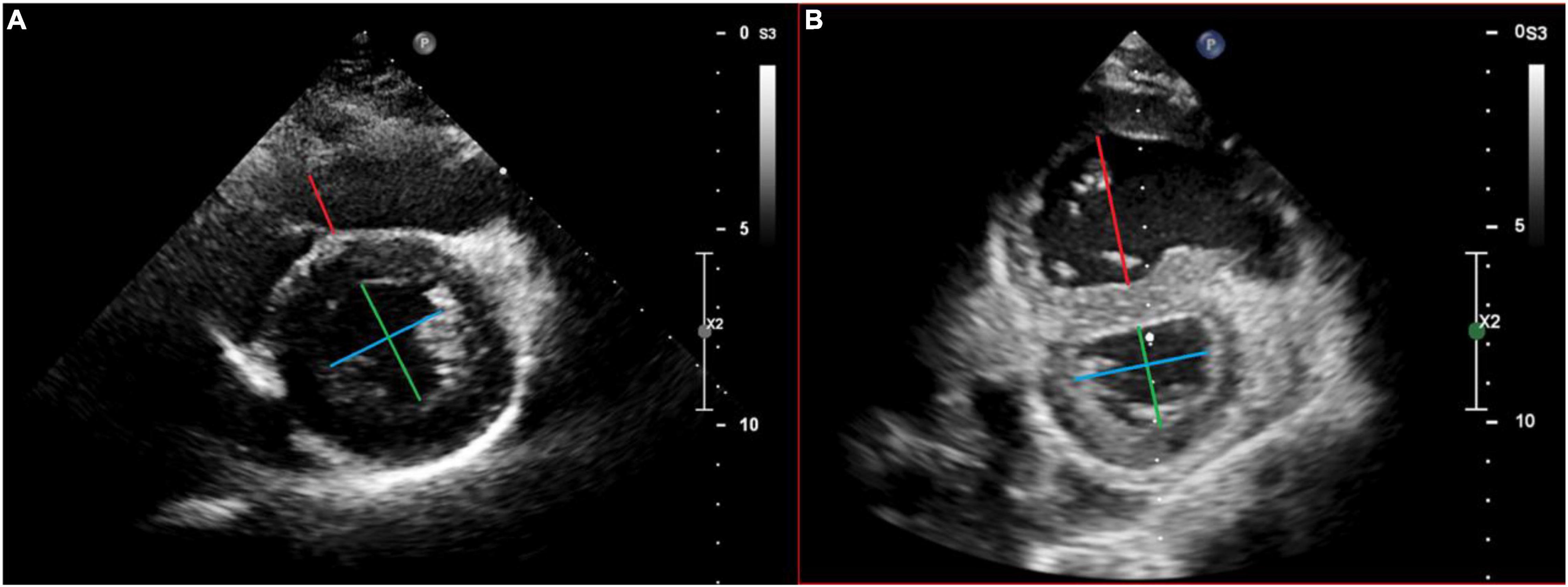
Figure 1. Echocardiographic measurement of the right ventricular to left the ventricular endsystolic (RV/LVes) ratio and the left ventricular endsystolic eccentricity index (LVes EI) in a healthy subject (A) and in a patient with pulmonary hypertension (B). Measurement of the RV/LVes ratio (RV = red/LV = green line) and the LVes EI (blue/green line) in the apical parasternal short axis view between papillary muscle, from the endocardial to endocardial surfaces at endsystole.
Additional Measurements for Pulmonary Hypertension Children
NYHA FC/modified ROSS score were determined by 2 independent pediatric cardiologists, who are responsible for the medical care of the patients. The tricuspid annular plane systolic excursion (TAPSE) reflects the longitudinal excursion of the tricuspid annulus toward the apex and was measured with M-mode in the apical 4-chamber view as described before (23). The pulmonary artery acceleration time (PAAT) was measured as the interval between the onset of ejection and the peak flow velocity, defined as the time from the onset to maximal velocity (28) and is an inverse surrogate of pulmonary artery pressure and pulmonary vascular resistance index (PVRi).
Ethics
This study complies with the institutional guidelines related to patient confidentiality and research ethics including the institutional review board approval of the Ethics Board of Graz Medical University (Ethics committee Number 33–320 ex 20/21.).
Statistics
Data are presented as median and range (minimum–maximum) for continuous variables or absolute and relative numbers for categorical data. Associations of RV/LVes ratio and LVes EI with age, body length, weight, BSA, and clinical parameters were analyzed using Spearman’s rank correlation coefficient. Differences in RV/LVes ratio and LVes EI values between patients of different NYHA classes were analyzed using Kruskal Wallis test (Dunn’s post hoc test with the Bonferroni correction). To determine age-, body length-, weight-, and BSA-specific reference values, generalized additive models were used. Therefore, growth curve centile estimations were performed using either normal distribution or Reverse Gumbel distribution for the response variable. The decision regarding whether to use normal or Reverse Gumbel distribution was based on residual distribution. To define a “normal” range between 2.5 and 97.5% percentiles, the data are given in Supplementary Tables 3A–D, 4A–D and Figures 2A–D, 3A–D. For children older than 1 year, BSA > 0.5 m2, body length > 75 cm or weight > 15 kg, ROC analysis was performed to calculate AUC with 95% confidence intervals and the best cutoff to identify PH-patients. The best cutoff was defined by Youden-Index. For data analysis R (Version 4.1.1; R Foundation for Statistical Computing) was used. Interobserver and intraobserver variability was examined with an intraclass correlation coefficient (ICC) and corresponding 95% confidence intervals (95%CI).
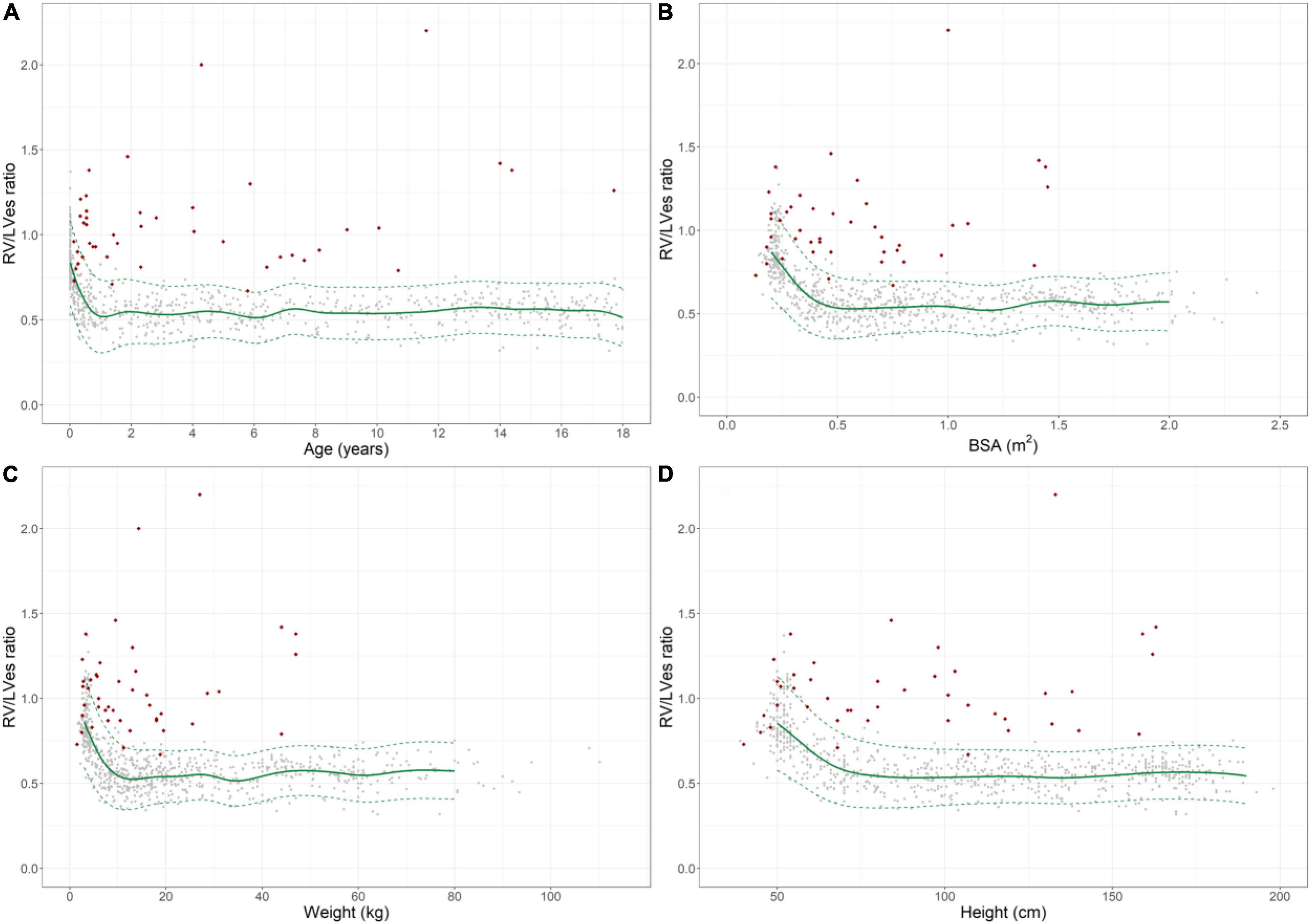
Figure 2. Normal reference values for right ventricular to left ventricular endsystolic (RV/LVes) ratio in healthy children and individual levels of pulmonary hypertension patients (red diamonds). Normal change of RV/LVes ratio with increasing (A) age, (B) BSA, (C) weight and (D) height (gray circles: individual values; green solid line: median, dashed green line: 2.5 and 97.5% percentile).
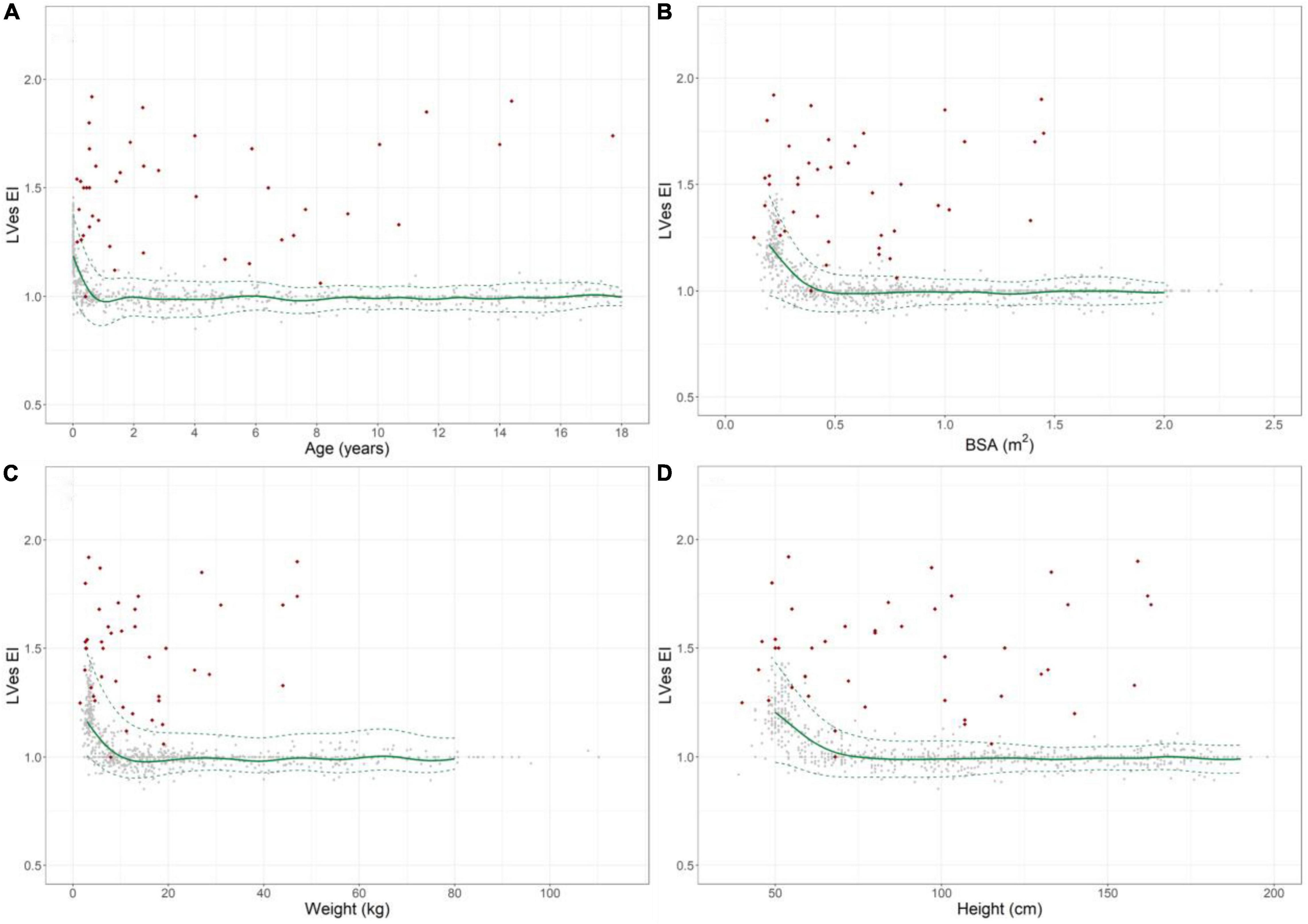
Figure 3. Normal reference values for LVes EI (LV endsystolic eccentricity index) in healthy children and individual levels of pulmonary hypertension patients (red diamonds). Normal change of LVes EI with increasing (A) age, (B) BSA, (C) weight, and (D) height (gray circles: individual values; green solid line: median, dashed green line: 2.5 and 97.5% percentile).
Results
Healthy Children
In the 769 healthy neonates, children, and adolescents studied, no difference between males (50.7%) and females was observed in baseline characteristics (Supplementary Table 1).
Right Ventricular to Left Ventricular Endsystolic Ratio
The RV/LVes ratio values were highly age dependent: Healthy neonates [median 0.83 (range 0.53–1.37)], healthy 12–24 months old [0.55 (0.35–0.80)], 18th year of life [0.53 (0.32–0.74)] (Figure 2A and Supplementary Table 2).
The RV/LVes ratio was high in healthy neonates 0.83 (range: 0.53–1.37) and gradually decreased within the first year of life (Figure 2A) and until increases in BSA (Figure 2B) to about 0.5 m2, body weight to about 15 kg (Figure 2C), and BL to about 75 cm (Figure 2D). The RV/LVes ratio was almost constant thereafter and did not further change with increasing age, BSA, body length and weight (18th year of life: median: 0.53, range: 0.32–0.74). The RV/LVes ratio negatively correlated with age (p < 0.001, rs = –0.498;p < 0.001, rs = –0.744 in infants < 1 year), BSA (p < 0.001, rs = –0.484; p < 0.001, rs = –0.740 in children with BSA < 0.5 m2), BW (p < 0.001, rs = –0.484; p < 0.001, rs = –0.764 in children with BW < 15 kg) and BL (p < 0.001, rs = –0.486; p < 0.001, rs = –0.701 in children with BL < 75 cm) (Figures 2A–D). Age-, BSA-, BL-, and BW-related normal RV/LVes ratio values are shown in Supplementary Tables 3A–D).
Left Ventricular Endsystolic Eccentricity Index
LVes EI values were highly age dependent: Healthy neonates: [median 1.21 (range 0.92–1.45)], healthy 12–24 months old [1.0 (0.88–1.13)], 18th year of life: ratio: [1.0 (0.97–1.07)], Figure 3A and Supplementary Table 2.
The LVes EI was high in healthy neonates (median: 1.21, range: 0.92–1.45, Supplementary Table 2) and gradually decreased within the first year of life (Figure 3A) and until increases in BSA (Figure 3B) to about 0.5 m2, body weight to about 15 kg (Figure 3C) and BL to about 75 cm (Figure 3D), but were almost constant thereafter and did not further change with increasing age, BSA, body length, and weight (18th year of life: median: 1.00, range: 0.97–1.07), Figures 3A–D. The LVes EI negatively correlated with age (p < 0.001, rs = –0.499; p < 0.001, rs = –0.758 in infants < 1 year), BSA (p < 0.001, rs = -0.493; p < 0.001, rs = –0.696 in children with BSA < 0.5 m2), BW (p < 0.001, rs = –0.493; p < 0.001, rs = –0.722 in children with BW < 15 kg) and BL (p < 0.001, rs = –0.494; p < 0.001, rs = –0.642 in children with BL < 75 cm). Age-, BSA-, BL-, and BW-related normal LVes EI values are shown in Supplementary Tables 4A–D).
Pulmonary Hypertension Children
In the 44 PH patients studied (median age: 2.1 years; range: 0.1 months to 17.7 years; 32% female) the RV/LVes ratio (median: 1.01, range: 0.67–2.20) and the LVes EI values (1.50, 1.06–2.30) did not change with age (RV/LVes: p = 0.257, rs = 0.18; LVes EI p = 0.229, rs = 0.19), body length (RV/LVes: p = 0.530, rs = 0.10; LVes EI p = 0.544, rs = 0.10), body weight (RV/LVes: p = 0.734, rs = 0.05; LVes EI p = 0.799, rs = 0.04), or BSA (RV/LVes: p = 0.732, rs = 0.05; LVes EI p = 0.781, rs = 0.04). Children (> 1 year) and adolescents with PH had significantly higher RV/LVes ratios (no PH: median 0.55, IQR 0.49–0.60; PH: 1.02, 0.87–1.26; p < 0.001) and higher LVes EI values (no PH: 1.00, 0.98–1.00; PH: 1.53, 1.26–1.71; p < 0.001) compared to children and adolescents without PH (Figures 4, 5).
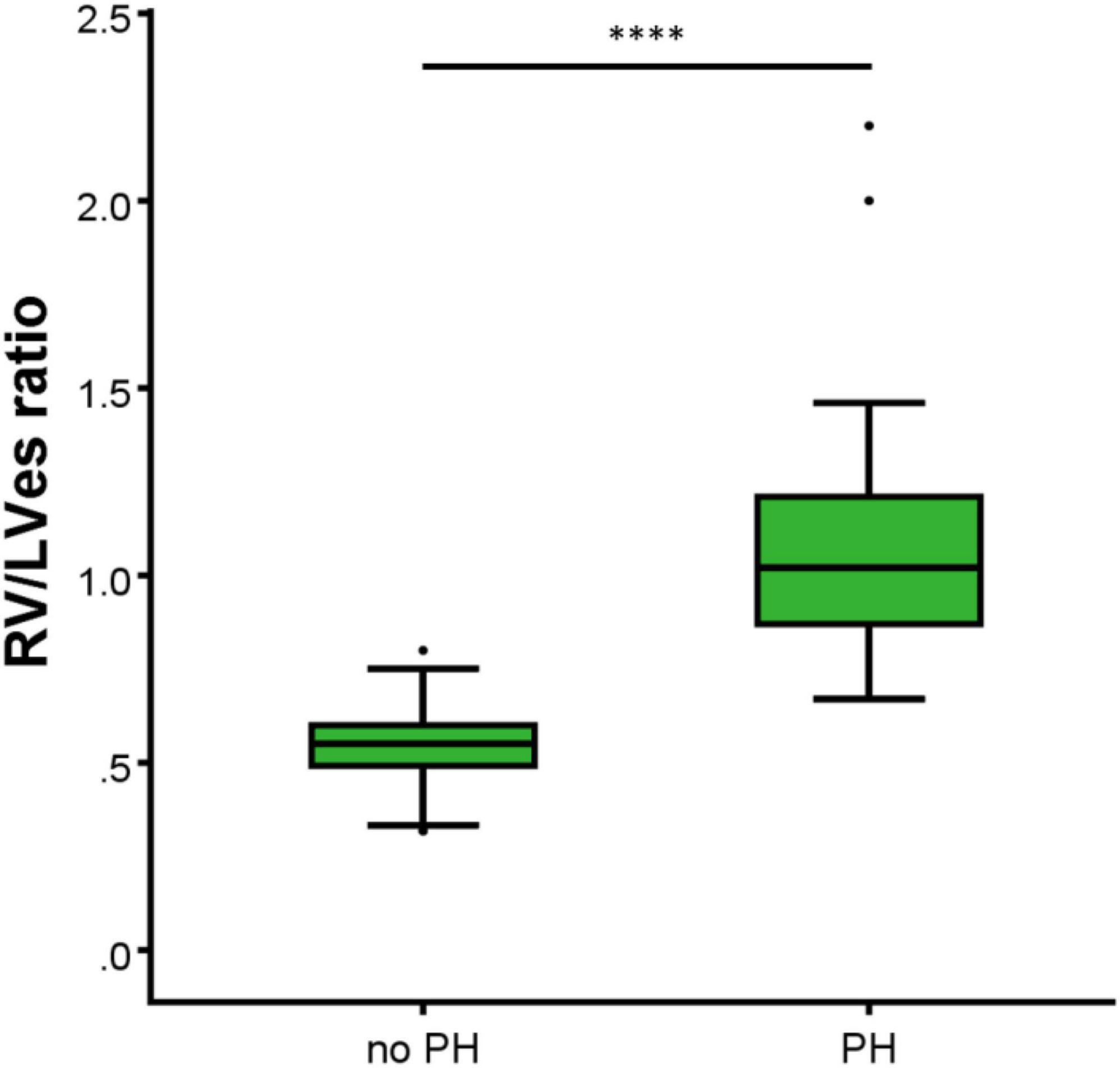
Figure 4. Differences between the healthy study group (no PH) and pulmonary hypertension (PH) study group in RV/LVes ratio (right ventricular to left ventricular endsystolic ratio). The box plot graphs show the median, IQR and range (Tukey method 1.5*IQR). ****p < 0.0001.
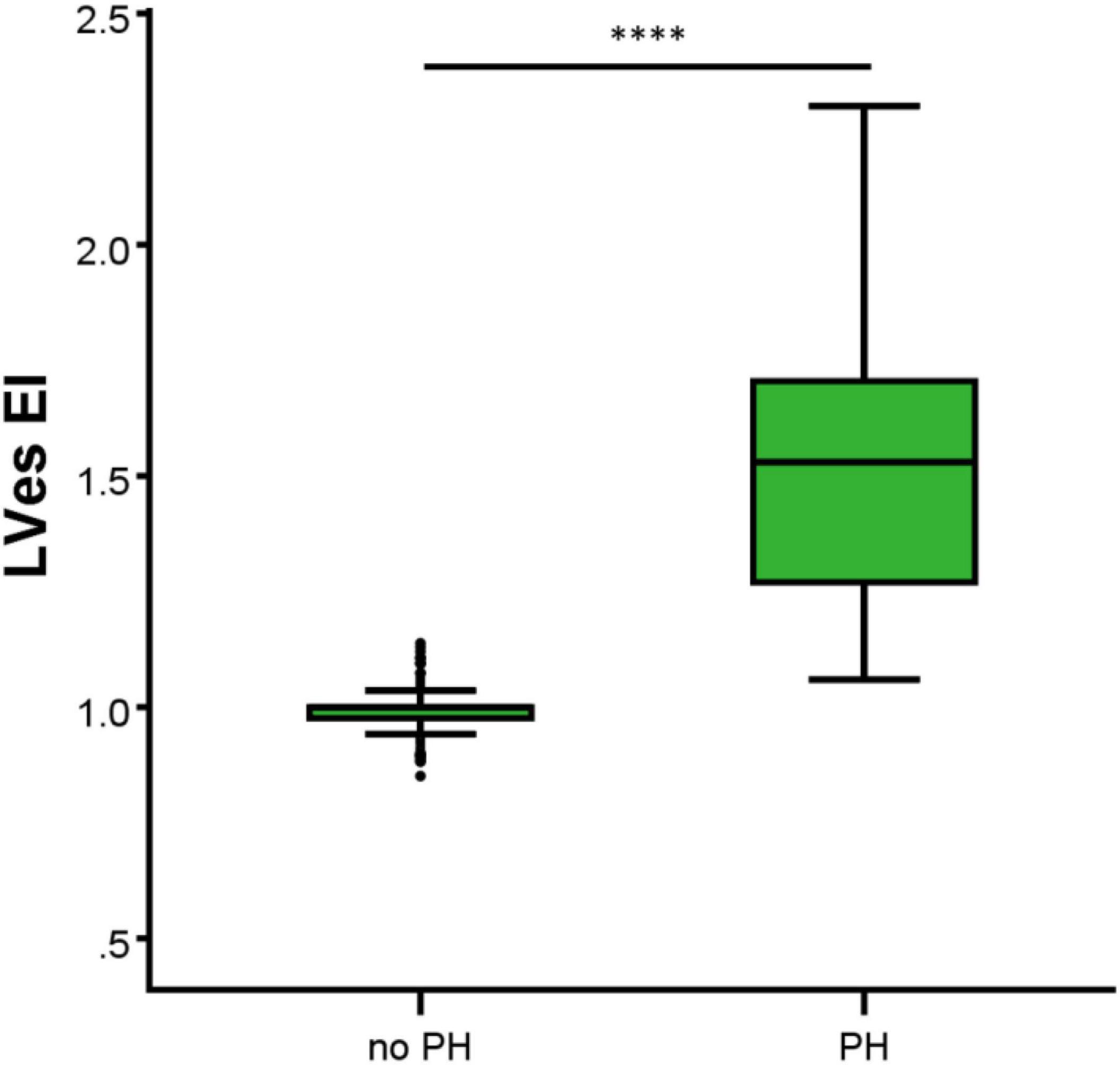
Figure 5. Differences between the healthy study group (no PH) and pulmonary hypertension (PH) study group in LVes EI (left ventricular endsystolic eccentricity index). The box plot graphs show the median, IQR and range (Tukey method 1.5*IQR). ****p < 0.0001.
No difference between males and females were observed in LVes EI (median, range: female: 1.37, 1.06–1.87; male: 1.53, 1.15–2.30; p = 0.198) or RV/LVes ratio (female: 0.90, 0.67–2.20; male: 1.02, 0.81–2.00; p = 0.147). The best cutoff to differentiate children with PH from children without PH for RV/LVes ratio (Table 2) is ≥ 0.67 in children older than 1 year, > 0.5 m2 or > 75 cm (sensitivity: 1.00, specificity: 0.95, Figure 6A), for children with a weight of > 15 kg the best cutoff is ≥ 0.78 (sensitivity: 0.96, specificity: 1.00). For LVes EI the best cutoff (Table 2) is ≥ 1.06 for children older than 1 year, > 0.5 m2, > 75 cm (sensitivity: 1.00, specificity: 0.97) or > 15 kg (sensitivity: 1.00, specificity: 0.96, Figure 6B). In our cohort with a prevalence of 5.2% for RV/LVes ratio the positive predictive value was 50% and the negative predictive value 100% and for LVes EI the positive predictive value was 61.4% and the negative predictive value 100%. The RV/LVes ratio values (Supplementary Table 7) in the PH group showed a significantly negative correlation with PAAT, but no correlation was found between PAAT and LVes EI (Supplementary Table 8).
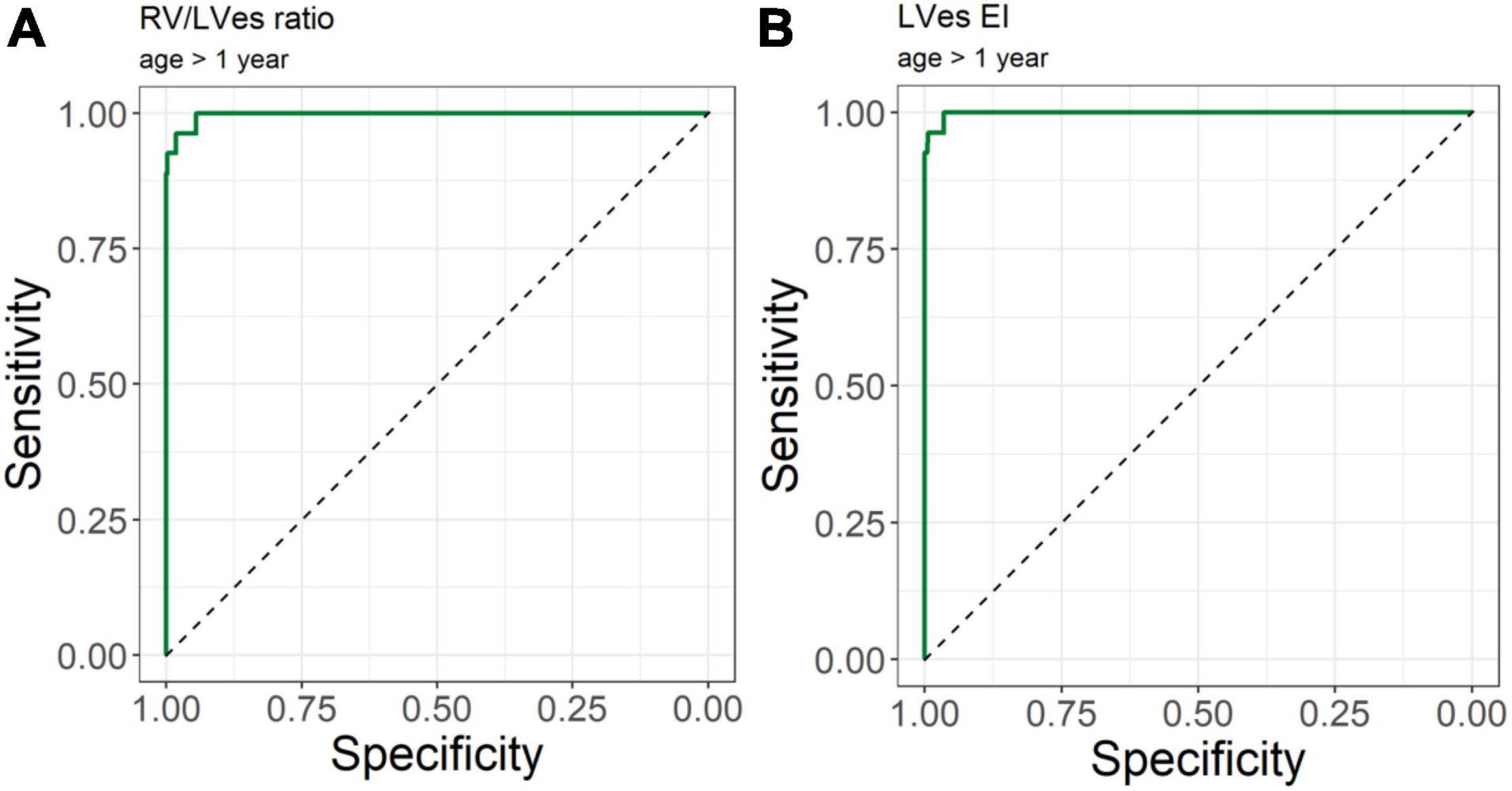
Figure 6. Receiver operating curve (ROC) analysis for the detection of pulmonary hypertension patients. Using (A) RV/LVes Ratio (AUC = area under the curve: 0.997, 95%CI: 0.993–1.000) and (B) LVes EI (AUC: 0.999, 95% CI: 0.996–1.000) in children older 1 year.
There was no correlation between TAPSE and RV/LVes ratio (Supplementary Table 7) or TAPSE and LVes EI (Supplementary Table 8). The RV/LVes ratio was significantly lower in patients with NYHA class 1 compared to class 2 or class 3 (class 1: 0.88, 0.67–1.09; class 2: 1.09, 0.71–1.46; p = 0.035; class 3: 1.10, 0.73–2.20). Patients with NYHA class 1 had significantly lower LVes EI values compared to class 2 (class 1: 1.28, 1.06–1.60; class 2: 1.52, 1.12–1.92; p = 0.039) and class 3 (1.68, 1.25–2.30; p = 0.009).
Reproducibility
Interobserver and intraobserver variability was examined with an intraclass correlation coefficient (ICC) and corresponding 95% confidence intervals (95%CI).
Intraobserver ICC for the healthy LVes EI and RV/LVes ratio were as follows: ICC: 0.93, 95%CI: 0.88–0.96; ICC: 0.98, 95%CI: 0.96–0.99.
Interobserver ICC for the healthy LVes EI and RV/LVes ratio were as follows: 0.81, 95%CI: 0.70–0.88; ICC: 0.95, 95%CI: 0.92–0.97.
Intraobserver ICC for the PH LVes EI and RV/LVes ratio were as follows: ICC: 0.98, 95%CI: 0.97–0.99; ICC: 0.99, 95%CI: 0.99–1.00.
Interobserver ICC for the PH LVes EI and RV/LVes ratio were as follows: ICC: 0.97, 95%CI: 0.94–0.98, ICC: 0.96, 95%CI: 0.93–0.98.
Discussion
We provide Age-, BSA-, BL-, and BW-related normal RV/LVes ratio (Figures 2A–D) and LVes EI (Figures 3A–D) reference values and percentiles (2.5, 50, and 97.5% percentile, Supplementary Tables 3A–D, 4A–D) in a large cohort of healthy neonates, children, and adolescents, as well as validated abnormal values in children with PH (median age: 2.1 years; range: 0.1 months to 17.7 years).
The physiology of ventricular-ventricular interactions is based on the in-series circulation and common pericardium, interventricular septum, and myocardial tracts running between the ventricles (29). A potential LV compression can be visualized by parasternal echocardiography in the short axis as a D-shaped LV, most simply quantified with the LV eccentricity index (EI) (25). This index measures the LV lateral dimension as a ratio over the anterior-posterior dimension in the parasternal short axis. In adults a LV EI > 1.1 is considered abnormal (25, 30). Ryan et al. (25) and Lammers et al. (31) demonstrated that children with PH have a more prominent systolic bowing of the interventricular septum than in diastole in their LV EI. The LV EI measures the deformity in the left ventricle, but does not take the altered RV dimension into account. The RV/LV ratio is measured from a parasternal short axis image at the LV papillary muscle level as the ratio of the anterior-posterior dimension of the RV- and LV diameters at end-systole (12, 26) or at end-diastole (26, 32, 33). The RV/LV ratio was shown to correlate with invasive measurements of PH (12, 26) and incorporates leftward septal shift and RV dilatation, thereby incorporating RV failure, remodeling, and adverse hemodynamics. An RV/LV ratio > 1 has been associated with increased mortality risk and thus is clinically useful (12).
In 1992 Louie et al. (34) reported of 11 adult PH patients (age 33 ± 10 years) with a LVes EI of 1.64 ± 0.48 and 11 healthy controls with a LVes EI of 1 ± 0.05. Averin et al. (21) reported of 29 PH subjects [3.8 years (0.9, 11.5), 72% IPAH] with a mean LV EI of 1.6 ± 0.5. Burkett et al. (26) reported of 78 healthy children/young adults (0–23 years) with a normal LVes EI of 1 (0.97–1.04) vs. 1.27 (1.1–1.5) in 78 PH patients (0–23 years; 92.3% under PH medication) investigated. We found that children (> 1 year) and adolescents with PH had significantly higher LVes EI values (no PH: median 1.00, IQR 0.98–1.00; PH: 1.53, 1.26–1.71) compared to children and adolescents without PH. In our study, healthy neonates had high LVes EI (median: 1.21, range: 0.92–1.45) and the RV/LVes ratio (median:0.83, range:0.53–1.37), both of which gradually decreased within the first year of life (Figures 2A, 3A) and until increases in BSA to about 0.5 m2 (Figures 2B, 3B), BW to about 15 kg (Figures 2C, 3C) and BL to about 75 cm (Figures 2D, 3D), but were almost constant thereafter and did not further change with increasing age, BSA, BW, and BL (LVes EI: 18 year of life: median: 1.00, range: 0.97–1.07; RV/LVes ratio: median: 0.53, range: 0.32–0.74).
The RV/LVes ratio and LVes EI values decreased significantly (p < 0.001) with increasing age, body weight, body length, and BSA in a non-linear way in the healthy patients. This highlights the importance of creating normal values for the pediatric age group, since indexed measurements to age, BSA, BL, and BW—due to the high variability of age-dependent growth—are highly warranted in this particular population. In contrast to toddlers, children, and adolescents with PH, the LVes EI and the RV/LVes ratio values were within the normative values in a substantial proportion of infants with PH. Therefore, the LVes EI and the RV/LVes ratio data in neonates and young infants should be interpreted with caution, due to the high variability in body size, weight, and pulmonary blood flow in this particular age group. While this phenomenon was not investigated within the last 30 years, Ichida et al. (35) reported in 1988 that the RV diameter in diastole is relatively large on the 1st day of life in healthy children (parasternal long axis RV: 1.05 ± 0.08) and the diastolic RV/LV ratio was found to be high (0.61 ± 0.05). While their data cannot be directly compared to our data (we investigated the ratio and LVes EI in the PSAX and not in the PLAX and at endsystole but not diastole), they give us a hint that this observed phenomenon is quite physiologic. Ichida et al. further found that the RV/LV ratio decreases gradually until 3 months of life, as well as the diastolic RV dimension. After the first months of life, a steady increase in RV dimension related to age is observed, while in contrast, the LV dimension was found to be gradually increased with age (35). Therefore, echocardiographic determination of the RV/LVes ratio and the LVes EI represents a promising approach for monitoring children with PH during standard echocardiographic examinations. The LVes EI turned out to be a useful variable of RV mechanics in infants with bronchopulmonary dysplasia and may be incorporated in routine protocols when there is a concern for PH in infants (19).
We (10) recently found that pediatric PH patients with increased LV EI values have significantly smaller LA areas. In the same study we found that the LV EI increased with an increasing systolic pulmonary artery pressure/systolic systemic arterial pressure ratio (sPAP/sSAP ratio) and also with an increasing pulmonary vascular resistance index, showing that the degree of LV compression due to significant RV afterload can be visualized. In a pediatric PH cohort, the RV/LV ratio was found to be positively correlated with an increasing sPAP/sSAP ratio and with an increasing NYHA FC (10). This suggests that these VVI variables (RV/LV ratio and the LV EI) potentially reflect the disease severity in children suffering from PH (10). Hansmann et al. (36) reported that the RV/LVes ratio and the LVes EI were greatly abnormal pre-lung transplantation (LuTx), with median values of 2.6 and 2.0, respectively. In contrast to the RV end-diastolic diameter that showed similar relative diameter reduction post-LuTx in most patients, the RV/LVes ratio and LVes EI had a “hand fan” type reduction pattern: Even PAH patients with very high ratios (RV dilation, LV compression/underfilling) pre-LuTx rapidly normalized both indices post-LuTx to indices of 0.62 and 1.0, respectively. These data demonstrate that readily available transthoracic echocardiography (2, 37) can sufficiently document the post-LuTx improvements of RV-hypertrophy, RV dilation, and LV filling. In this regard, the RV/LVes ratio (10, 38), the LVes EI (26, 39), and RV endsystolic remodeling index (9) are robust and useful echocardiographic markers (2, 11, 37, 38). Moreover, the LV EI and RV/LV dimension ratio were associated with a lower WHO functional class, and worse hemodynamics (26). This is in agreement with our findings; children with NYHA class 1 had significantly lower LVes EI and RV/LVes ratio values compared to class 2 and class 3.
Burkett et al. (26) showed that the systolic-, diastolic-, and maximum LV EI and the endsystolic and end-diastolic RV/LV ratio correlated with invasive hemodynamics (e.g., mean pulmonary arterial pressure, mPAP). An LVes EI of 1.16 yielded the largest AUC to define PH (LVes EI PH: 1.27 [1.1–1.49] vs. healthy subjects: 0.99 [0.97–1.04]). Only LVes EI and RV/LVes ratio correlated with the need to escalate therapy (26) suggesting that these VVI variables might be able to reflect the disease severity of PH also in children suffering from PH (26). Jone et al. (12) investigated the RV/LVes ratio retrospectively in 80 matched normal controls and 84 PH patients without shunts. Of their PH patients, 49 children underwent 94 echocardiographic examinations and cardiac catheterizations within 48 h (13 patients had simultaneous measurements). The RV/LVes ratio was correlated with hemodynamic variables. Twenty-two PH patients with RV/LV ratios > 1 had adverse events within a median of 1.1 years from their earliest echocardiographic studies. Increasing RV/LV ratio was associated with an increasing hazard for a clinical event. The RV/LVes ratios were lower in controls compared to patients with PH (mean, 0.51 [95% confidence interval, 0.48–0.54] vs. 1.47 [95% confidence interval, 1.25–1.70]), (12). We observed that children (> 1 year) and adolescents with PH had significantly higher RV/LVes ratio (no PH: median 0.55, IQR 0.49–0.60; PH: 1.02, 0.87–1.26; Figure 4) and higher LVes EI values (no PH: 1.00, 0.98–1.00; PH: 1.53, 1.26–1.71; Figure 5) compared to children and adolescents without PH. In children older than 1 year, the best cutoff to differentiate children with PH from children without PH for RV/LVes ratio was ≥ 0.67 (sensitivity: 1.00, specificity: 0.95) and for LVes EI ≥ 1.06 (sensitivity: 1.00, specificity: 0.97), Table 2 and Figure 6. The RV/LVes ratio values (Supplementary Table 7) in the PH group showed a significantly negative correlation with PAAT, but no correlation was found between PAAT and LVes EI (Supplementary Table 8). There was no correlation between TAPSE and RV/LVes ratio (Supplementary Table 7) or between TAPSE and LVes EI (Supplementary Table 8).
Conclusion
Taken together, we provide normal reference values and percentiles (of the RV/LVes ratio and LVes EI in healthy children and adolescents (1 day–18 years) that will assist to identify children with abnormal values. The RV/LVes ratio and the LVes EI have been shown to be useful, simple, reliable, and non-invasive markers of right ventricular hypertension in infants with PH (11, 12, 19, 36, 40) and may be incorporated in routine protocols when there is a concern for PH in children.
Study Limitations
The LV EI has limited power in postoperative CHD-PH patients due to an unpredictable effect of, e.g., a VSD repair on the long-time performance of the interventricular septum. In our postoperative CHD-PH patients, the effect of a bundle branch block on the dimensions (LVes EI and RV/LVes ratio) was not investigated. A bundle branch block may influence LVes EI and RV/LVes ratio. However, to what extent a bundle branch block may alter these values remains speculative. We do not provide more sophisticated indicators of RV remodeling and RV-LV interactions, such as 3D echocardiography.
Data Availability Statement
The datasets presented in this article are not readily available. Requests to access the datasets should be directed to corresponding author.
Ethics Statement
The studies involving human participants were reviewed and approved by the Ethics Board of Graz Medical University (Ethics committee Number 33–320 ex 20/21.). Written informed consent to participate in this study was provided by the participants or their legal guardian/next of kin.
Author Contributions
SS, GH, and MK: conception and design and manuscript writing. SK-K, PC, and AG: administrative support. GG, AB, AF, and NN: provision of study materials or patients. SS and MK: collection and assembly of data. AA: data analysis and interpretation. All authors final approval of manuscript.
Funding
This work was supported by the German Research Foundation (DFG KFO311 HA4348/6-2 to GH) and the European Pediatric Pulmonary Vascular Disease Network (EPPVDN), Berlin, Germany.
Conflict of Interest
The authors declare that the research was conducted in the absence of any commercial or financial relationships that could be construed as a potential conflict of interest.
Publisher’s Note
All claims expressed in this article are solely those of the authors and do not necessarily represent those of their affiliated organizations, or those of the publisher, the editors and the reviewers. Any product that may be evaluated in this article, or claim that may be made by its manufacturer, is not guaranteed or endorsed by the publisher.
Supplementary Material
The Supplementary Material for this article can be found online at: https://www.frontiersin.org/articles/10.3389/fcvm.2022.950765/full#supplementary-material
Abbreviations
BL, body lengths; BSA, body surface area; BW, body weight; CHD, congenital heart disease; EI, eccentricity index; Es, endsystolic; iPAH, idiopathic pulmonary arterial hypertension; LuTx, lung transplantation; LV, left ventricular; LVes EI, LV endsystolic eccentricity index; NYHA FC, New York Heart Association Functional class; PAAT, pulmonary artery acceleration time; PH, pulmonary hypertension; PH-BPD, PH associated with bronchopulmonary dysplasia; PH-CHD, PH associated with congenital heart disease; PSAX, parasternal short axis; RV, right ventricular; RV/LV ratio, right ventricular to left ventricular ratio; RV/LVes ratio, right ventricular to left ventricular endsystolic ratio; sPAP/sSAP ratio, systolic pulmonary artery pressure/systolic systemic arterial pressure ratio; TAPSE, tricuspid annular plane systolic excursion; VVI, ventricular-ventricular interaction.
References
1. Hansmann G. Pulmonary hypertension in infants, children, and young adults. J Am Coll Cardiol. (2017) 69:2551–69. doi: 10.1016/j.jacc.2017.03.575
2. Koestenberger M, Apitz C, Abdul-Khaliq H, Hansmann G. Transthoracic echocardiography for the evaluation of children and adolescents with suspected or confirmed pulmonary hypertension. Expert consensus statement on the diagnosis and treatment of paediatric pulmonary hypertension. The European paediatric pulmonary vascular disease network, endorsed by ISHLT and D6PK. Heart. (2016) 102(Suppl. 2):ii14–22. doi: 10.1136/heartjnl-2014-307200
3. Hansmann G, Koestenberger M, Alastalo TP, Apitz C, Austin ED, Bonnet D, et al. 2019 updated consensus statement on the diagnosis and treatment of pediatric pulmonary hypertension: the European pediatric pulmonary vascular disease network (EPPVDN), endorsed by AEPC, ESPR and ISHLT. J Heart Lung Transplant. (2019) 38:879–901. doi: 10.1016/j.healun.2019.06.022
4. Vonk Noordegraaf A, Haddad F, Bogaard HJ, Hassoun PM. Non-invasive imaging in the assessment of the cardiopulmonary vascular unit. Circulation. (2015) 131:899–913. doi: 10.1161/CIRCULATIONAHA.114.006972
5. Cantinotti M, Giordano R, Scalese M, Murzi B, Assanta N, Spadoni I, et al. Nomograms for two-dimensional echocardiography derived valvular and arterial dimensions in Caucasian children. J Cardiol. (2017) 69:208–15. doi: 10.1016/j.jjcc.2016.03.010
6. Cantinotti M, Kutty S, Franchi E, Paterni M, Scalese M, Iervasi G, et al. Pediatric echocardiographic nomograms: what has been done and what still needs to be done. Trends Cardiovasc Med. (2017) 27:336–49. doi: 10.1016/j.tcm.2017.01.006
7. Cantinotti M, Scalese M, Giordano R, Assanta N, Marchese P, Franchi E, et al. A statistical comparison of reproducibility in current pediatric two-dimensional echocardiographic nomograms. Pediatr Res. (2021) 89:579–90. doi: 10.1038/s41390-020-0900-z
8. Burkett DA, Slorach C, Patel SS, Redington AN, Ivy DD, Mertens L, et al. Impact of pulmonary hemodynamics and ventricular interdependence on left ventricular diastolic function in children with pulmonary hypertension. Circ Cardiovasc Imaging. (2016) 9:e004612. doi: 10.1161/CIRCIMAGING.116.004612
9. Koestenberger M, Avian A, Chouvarine P, Gamillscheg A, Cvirn G, Schweintzger S, et al. Right ventricular end-systolic remodeling index in the assessment of pediatric pulmonary arterial hypertension. The European pediatric pulmonary vascular disease network (EPPVDN). Pediatr Res. (2020) 88:285–92. doi: 10.1038/s41390-020-0748-2
10. Koestenberger M, Sallmon H, Avian A, Cantinotti M, Gamillscheg A, Kurath-Koller S, et al. Ventricular-ventricular interaction variables correlate with surrogate variables of clinical outcome in children with pulmonary hypertension. Pulm Circ. (2019) 9:2045894019854074. doi: 10.1177/2045894019854074
11. Meinel K, Koestenberger M, Sallmon H, Hansmann G, Pieles GE. Echocardiography for the assessment of pulmonary hypertension and congenital heart disease in the young. Diagnostics (Basel). (2020) 11:49. doi: 10.3390/diagnostics11010049
12. Jone PN, Hinzman J, Wagner BD, Ivy DD, Younoszai A. Right ventricular to left ventricular diameter ratio at end-systole in evaluating outcomes in children with pulmonary hypertension. J Am Soc Echocardiogr. (2014) 27:172–8. doi: 10.1016/j.echo.2013.10.014
13. Friedberg MK. Imaging right-left ventricular interactions. JACC Cardiovasc Imaging. (2018) 11:755–71. doi: 10.1016/j.jcmg.2018.01.028
14. Okumura K, Slorach C, Mroczek D, Dragulescu A, Mertens L, Redington AN, et al. Right ventricular diastolic performance in children with pulmonary arterial hypertension associated with congenital heart disease: correlation of echocardiographic parameters with invasive reference standards by high-fidelity micromanometer catheter. Circ Cardiovasc Imaging. (2014) 7:491–501. doi: 10.1161/CIRCIMAGING.113.001071
15. Ploegstra MJ, Roofthooft MT, Douwes JM, Bartelds B, Elzenga NJ, van de Weerd D, et al. Echocardiography in pediatric pulmonary arterial hypertension: early study on assessing disease severity and predicting outcome. Circ Cardiovasc Imaging. (2015) 8:e000878. doi: 10.1161/CIRCIMAGING.113.000878
16. Lammers AE, Apitz C, Michel-Behnke I, Koestenberger M. A guide to echocardiographic assessment in children and adolescents with pulmonary hypertension. Cardiovasc Diagn Ther. (2021) 11:1160–77. doi: 10.21037/cdt-21-119
17. Marcus JT, Gan CT, Zwanenburg JJ, Boonstra A, Allaart CP, Gotte MJ, et al. Interventricular mechanical asynchrony in pulmonary arterial hypertension: left-to-right delay in peak shortening is related to right ventricular overload and left ventricular underfilling. J Am Coll Cardiol. (2008) 51:750–7. doi: 10.1016/j.jacc.2007.10.041
18. Schafer M, Collins KK, Browne LP, Ivy DD, Abman S, Friesen R, et al. Effect of electrical dyssynchrony on left and right ventricular mechanics in children with pulmonary arterial hypertension. J Heart Lung Transplant. (2018) 37:870–8. doi: 10.1016/j.healun.2018.01.1308
19. Abraham S, Weismann CG. Left ventricular end-systolic eccentricity index for assessment of pulmonary hypertension in infants. Echocardiography. (2016) 33:910–5. doi: 10.1111/echo.13171
20. Amsallem M, Sternbach JM, Adigopula S, Kobayashi Y, Vu TA, Zamanian R, et al. Addressing the controversy of estimating pulmonary arterial pressure by echocardiography. J Am Soc Echocardiogr. (2016) 29:93–102. doi: 10.1016/j.echo.2015.11.001
21. Averin K, Michelfelder E, Sticka J, Cash M, Hirsch R. Changes in ventricular geometry predict severity of right ventricular hypertension. Pediatr Cardiol. (2016) 37:575–81. doi: 10.1007/s00246-015-1317-z
22. Koestenberger M, Nagel B, Ravekes W, Avian A, Burmas A, Grangl G, et al. Reference values and calculation of Z-scores of echocardiographic measurements of the normal pediatric right ventricle. Am J Cardiol. (2014) 114:1590–8. doi: 10.1016/j.amjcard.2014.08.028
23. Koestenberger M, Ravekes W, Everett AD, Stueger HP, Heinzl B, Gamillscheg A, et al. Right ventricular function in infants, children and adolescents: reference values of the tricuspid annular plane systolic excursion (TAPSE) in 640 healthy patients and calculation of Z score values. J Am Soc Echocardiogr. (2009) 22:715–9. doi: 10.1016/j.echo.2009.03.026
24. Pettersen MD, Du W, Skeens ME, Humes RA. Regression equations for calculation of Z scores of cardiac structures in a large cohort of healthy infants, children, and adolescents: an echocardiographic study. J Am Soc Echocardiogr. (2008) 21:922–34. doi: 10.1016/j.echo.2008.02.006
25. Ryan T, Petrovic O, Dillon JC, Feigenbaum H, Conley MJ, Armstrong WF. An echocardiographic index for separation of right ventricular volume and pressure overload. J Am Coll Cardiol. (1985) 5:918–27. doi: 10.1016/s0735-1097(85)80433-2
26. Burkett DA, Patel SS, Mertens L, Friedberg MK, Ivy DD. Relationship between left ventricular geometry and invasive hemodynamics in pediatric pulmonary hypertension. Circ Cardiovasc Imaging. (2020) 13:e009825. doi: 10.1161/CIRCIMAGING.119.009825
27. Mosteller RD. Simplified calculation of body-surface area. N Engl J Med. (1987) 317:1098. doi: 10.1056/NEJM198710223171717
28. Koestenberger M, Grangl G, Avian A, Gamillscheg A, Grillitsch M, Cvirn G, et al. Normal reference values and Z scores of the pulmonary artery acceleration time in children and its importance for the assessment of pulmonary hypertension. Circ Cardiovasc Imaging. (2017) 10:e005336. doi: 10.1161/CIRCIMAGING.116.005336
29. Friedberg MK, Redington AN. Right versus left ventricular failure: differences, similarities, and interactions. Circulation. (2014) 129:1033–44. doi: 10.1161/CIRCULATIONAHA.113.001375
30. Galie N, Humbert M, Vachiery JL, Gibbs S, Lang I, Torbicki A, et al. 2015 ESC/ERS guidelines for the diagnosis and treatment of pulmonary hypertension: the joint task force for the diagnosis and treatment of pulmonary hypertension of the European society of cardiology (ESC) and the European respiratory society (ERS): endorsed by: association for European paediatric and congenital cardiology (AEPC), international society for heart and lung transplantation (ISHLT). Eur Heart J. (2016) 37:67–119. doi: 10.1093/eurheartj/ehv317
31. Lammers AE, Haworth SG, Riley G, Maslin K, Diller GP, Marek J. Value of tissue doppler echocardiography in children with pulmonary hypertension. J Am Soc Echocardiogr. (2012) 25:504–10. doi: 10.1016/j.echo.2012.01.017
32. Hashimoto I, Watanabe K. Geometry-related right ventricular systolic function assessed by longitudinal and radial right ventricular contractions. Echocardiography. (2016) 33:299–306. doi: 10.1111/echo.13039
33. Hashimoto I, Watanabe K, Ichida F. Right to left ventricular diameter ratio >/=0.42 is the warning flag for suspecting atrial septal defect in preschool children: age- and body surface area-related reference values determined by m-mode echocardiography. Pediatr Cardiol. (2016) 37:704–13. doi: 10.1007/s00246-015-1334-y
34. Louie EK, Rich S, Levitsky S, Brundage BH. Doppler echocardiographic demonstration of the differential effects of right ventricular pressure and volume overload on left ventricular geometry and filling. J Am Coll Cardiol. (1992) 19:84–90. doi: 10.1016/0735-1097(92)90056-s
35. Ichida F, Denef B, Dumoulin M, Van der Hauwaert LG. Cardiac chamber growth pattern determined by two-dimensional echocardiography. Heart Vessels. (1988) 4:26–33. doi: 10.1007/BF02058684
36. Hansmann G, Diekmann F, Chouvarine P, Ius F, Carlens J, Schwerk N, et al. Full recovery of right ventricular systolic function in children undergoing bilateral lung transplantation for severe PAH. J Heart Lung Transplant. (2021) 41:187–98. doi: 10.1016/j.healun.2021.10.014
37. Koestenberger M, Friedberg MK, Ravekes W, Nestaas E, Hansmann G Non-invasive imaging for congenital heart disease: recent innovations in transthoracic echocardiography. J Clin Exp Cardiol. (2012) 8:2. doi: 10.4172/2155-9880.S8-002
38. Koestenberger M, Friedberg MK, Nestaas E, Michel-Behnke I, Hansmann G. Transthoracic echocardiography in the evaluation of pediatric pulmonary hypertension and ventricular dysfunction. Pulm Circ. (2016) 6:15–29. doi: 10.1086/685051
39. Koestenberger M, Hansmann G. Left ventricular geometry and near-simultaneous invasive hemodynamics in pediatric pulmonary hypertension. Circ Cardiovasc Imaging. (2020) 13:e010787. doi: 10.1161/CIRCIMAGING.120.010787
Keywords: normative values, pediatric, endsystolic right to left ventricular ratios, left ventricular endsystolic eccentricity index, pulmonary hypertension
Citation: Schweintzger S, Kurath-Koller S, Burmas A, Grangl G, Fandl A, Noessler N, Avian A, Gamillscheg A, Chouvarine P, Hansmann G and Koestenberger M (2022) Normal Echocardiographic Reference Values of the Right Ventricular to Left Ventricular Endsystolic Diameter Ratio and the Left Ventricular Endsystolic Eccentricity Index in Healthy Children and in Children With Pulmonary Hypertension. Front. Cardiovasc. Med. 9:950765. doi: 10.3389/fcvm.2022.950765
Received: 23 May 2022; Accepted: 20 June 2022;
Published: 14 July 2022.
Edited by:
Hannes Sallmon, Deutsches Herzzentrum Berlin, GermanyReviewed by:
Massimiliano Cantinotti, Gabriele Monasterio Tuscany Foundation (CNR), ItalyColin McMahon, University College Dublin, Ireland
Copyright © 2022 Schweintzger, Kurath-Koller, Burmas, Grangl, Fandl, Noessler, Avian, Gamillscheg, Chouvarine, Hansmann and Koestenberger. This is an open-access article distributed under the terms of the Creative Commons Attribution License (CC BY). The use, distribution or reproduction in other forums is permitted, provided the original author(s) and the copyright owner(s) are credited and that the original publication in this journal is cited, in accordance with accepted academic practice. No use, distribution or reproduction is permitted which does not comply with these terms.
*Correspondence: Sabrina Schweintzger, c2FicmluYS5zY2h3ZWludHpnZXJAbWVkdW5pZ3Jhei5hdA==