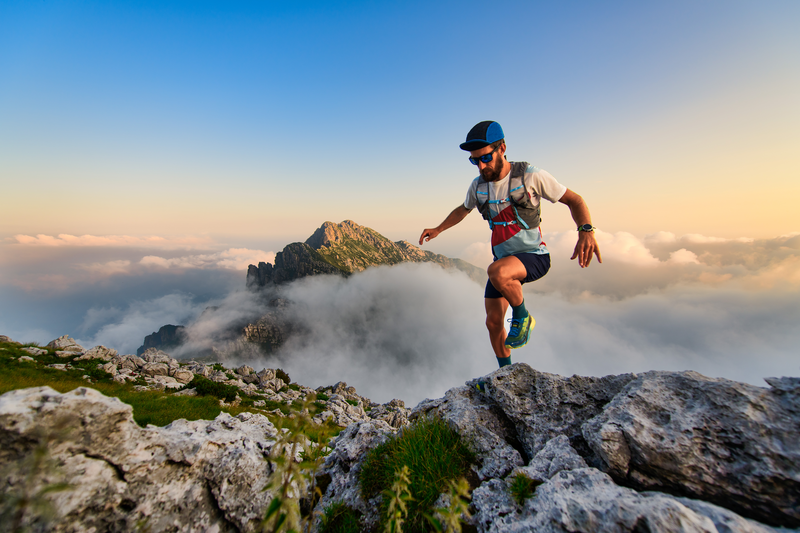
94% of researchers rate our articles as excellent or good
Learn more about the work of our research integrity team to safeguard the quality of each article we publish.
Find out more
SYSTEMATIC REVIEW article
Front. Cardiovasc. Med. , 08 September 2022
Sec. Coronary Artery Disease
Volume 9 - 2022 | https://doi.org/10.3389/fcvm.2022.949494
This article is part of the Research Topic Reviews in Bioresorbable Scaffold View all 6 articles
Background: While current concerns about bioresorbable scaffolds (BRS) are centered on late or very late scaffold thrombosis, less attention had been paid to short- and mid-term clinical outcomes. This review aimed to compare the short- and mid-term outcomes between BRS and drug-eluting stents (DES).
Methods: A systematic review of randomized controlled trials (RCTs) that compared BRS vs. DES was conducted by searching PubMed, Cochrane Library, Web of Science, CNKI, WanFang, and VIP databases from inception until 19 April 2022 (language limited to English or Chinese). The primary outcome was target lesion failure (TLF) within 12 months, defined as a composite of target lesion revascularization (TLR), target vessel myocardial infarction (TVMI), and cardiac death. The secondary outcomes were in-stent diameter stenosis (DS%) provided by intraluminal imaging.
Results: A total of 13 studies were eligible and were included in this review (N = 9,702 patients). The follow-up duration ranged from 6 months to 1 year. A significantly higher rate of TLF [RR, 1.22, 95% CI (1.03, 1.44)] driven by the higher rate of TVMI [RR, 1.39, 95% CI (1.09, 1.76)] was observed in the BRS group than in the DES group. The risk of TLR and cardiac death was similar between the groups. Also, compared with the DES group, the BRS group had a significantly higher in-stent DS% within 1 year [MD = 5.23, 95%CI (3.43, 7.04); I2 = 97%; p < 0.00001].
Conclusion: Bioresorbable scaffolds were associated with an increased risk of target lesion failure within 1 year as compared with DES, driven by the increased rates of target vessel myocardial infarction. Also, the in-stent DS% seemed to be higher with BRS. Therefore, BRS was inferior to DES in terms of target lesion outcomes at short- or mid-term follow-up.
Systematic review registration: https://www.crd.york.ac.uk/PROSPERO/display_record.php?RecordID=327966, PROSPERO (CRD42022327966).
Since the time drug-eluting stents (DES) were discovered, many trials and studies have shown their superiority to bare-metal stents (1). In addition, among DES, second-generation devices have substantially improved long-term safety and efficacy outcomes compared with first-generation devices (1, 2). Therefore, second-generation DES have been widely recommended for PCI (3). Nevertheless, studies also showed that DES was linked to neoatherosclerosis and fracture-related adverse pathological events (4, 5). Due to the presence of a permanent metallic stent, numerous deleterious long-term effects may occur, including vessel straightening, loss of compliance, vasoregulation, adaptive remodeling, and the potential for late inflammation and mechanical failure (6, 7). Therefore, the concept of bioresorbable scaffolds emerged. BRS was designed with the aim of reducing long-term adverse events stemming from permanent materials (8). To date, multiple trials evaluating clinical outcomes with BRS have been performed, including observational, non-comparative/single-arm studies, and large-scale random controlled trials (9, 10). Based on those studies, many prior meta-analyses focusing on long-term efficacy and safety (i.e., beyond 1 year) suggested that BRS was associated with worse clinical outcomes and a higher risk of adverse events, including device thrombosis, compared with DES (9, 11–14). However, much less emphasis has been placed on short- and mid-term clinical outcomes of BRS. Although several meta-analyses showed that BRS was inferior to DES at 1-year follow-up, these reviews did not include all the randomized controlled trials (RCTs) so far (15, 16). As more results of large-scale RCTs have been presented in recent years, we aimed to conduct a meta-analysis involving all the RCTs up to now. Moreover, compared with other previous reviews, this review emphasized more in terms of neointimal hyperplasia and lumen loss.
This review was performed according to the Preferred Reporting Items for Systematic Reviews and Meta-Analyses Statement 2020 (17). We systematically searched PubMed, Cochrane Library, Web of Science, CNKI, WanFang database, and VIP databases from inception until 19 April 2022. The language was limited to Chinese or English. References of the included studies were also searched manually to supplement relevant articles. Searches were conducted using a combination of subject terms and free words. English keywords included “bioresorbable vascular stent,” “target lesion failure,” and “randomized controlled trial.” See Appendix 1 in the Supplementary material for search strategies in detail.
Studies were considered eligible for inclusion if they were RCTs performed in patients undergoing PCI with both a clinical and an imaging diagnosis of CAD (including SCAD and ACS). Patients were randomized to treatment with either BRS or DES, regardless of the brands, types, lengths, and material parameters of stents and scaffolds. Duplicate publications were excluded (e.g., publications using the same data in different languages or partial data from a large-scale study).
The setting of composite endpoints for this review was based on the standardized definitions from the Academic Research Consortium (ARC) (18). Our primary outcome was target lesion failure (TLF), defined as target lesion revascularization (TLR), target vessel myocardial infarction (TVMI), and cardiac death. The RCTs included should provide at least one of the outcomes mentioned above at 6–12 months of follow-up after PCI. The secondary outcome was in-stent diameter stenosis (in-stent DS%). Studies enrolled should provide in-stent DS% as reported by coronary angiography, IVUS, or optical coherence tomography (OCT) at 6 to 12-month follow-up after PCI.
Searches and study selection were conducted independently by two reviewers (Yan-di Wan and Da-yang Wang). The process advanced as follows: (I) read study titles and exclude the studies not related to bioresorbable scaffolds, (II) read the abstracts and exclude non-RCTs (cohort comparison studies and nested case-control studies), (III) read the original text and exclude the studies that did not report the outcomes mentioned in the Outcomes section, and (IV) further read the full text and exclude duplicate publications. Finally, the results of searches and selections were cross-checked. Any discrepancy regarding searches and selection was discussed in consultation with and resolved by a third reviewer (Wen-qi Deng).
After identifying the included studies, two reviewers independently conducted data extraction. The data included the following: (I) basic information: study name, year of publication, country of study, sample size, and eligibility criteria; (II) baseline information: gender, age, follow-up visit, material of stent, and brand of stent; (III) outcome indicators: TLF, TLR, TVMI, cardiac death, and in-stent DS%; and (IV) assessment on the risk of bias: randomization, blinding, and allocation concealment. Data were checked after the extraction; Discrepancy, if any, was verified and resolved by a third reviewer (Wen-qi Deng). Literature was managed with the Endnote X9 software (19).
In this review, the management of missing values followed the processing method reported in the original studies. Last observation carried forward (LOCF) was a very common approach employed in trials to impute values for missing outcomes (20).
Statistical analysis was performed using Revman5.4. Data extracted were entered in Revman5.4 (21). Meanwhile, the mean ± standard deviation (mean ± SD) was adopted as the effect analysis statistic for measures, and the risk ratio (RR) with corresponding 95% confidence intervals (CIs) was adopted as the effect analysis statistic for binary variables.
The assessment of study quality was performed based on the Cochrane Collaboration's tool for assessing the risk of bias (22). Each entry from the tool was adjusted according to the review. To assess the risk of bias in the included studies, the tool was adjusted based on the seven criteria, namely, random sequence generation, allocation concealment, blinding of participants and personnel, blinding of outcome assessment, incomplete outcome data, selective reporting, and other biases. The points mentioned above stood for selection, performance, detection, attrition, reporting, and other biases, respectively. According to the extracted data, each study included was rated as “low risk of bias,” “unclear risk of bias,” or “high risk of bias” based on those seven points correspondingly. Summary graphs of the risk of bias were also generated with Revman5.4 based on the rating of the studies in terms of those points.
The quality of evidence was also evaluated according to the GRADE (Grading of Recommendations Assessment, Development, and Evaluation) system. Based on the type of studies, the GRADE system graded four levels of quality (high, moderate, low, and very low). RCTs started with a high rating, and the rating was modified downward in the following situations: (1) study limitations, (2) imprecision, (3) inconsistency of results, (4) indirectness of evidence, and (5) publication bias likely. Finally, the quality of each outcome was rated as high, moderate, low, or very low (23).
Between-study heterogeneity was evaluated using the χ2 test. Meanwhile, the heterogeneity was evaluated quantitatively with the Higgins I2 index (24). Evaluation for risk of publication bias was performed using Revman5.4, with funnel plots being generated. Also, the risk of publication bias was evaluated quantitatively using Stata 12.0 (25).
If there was no statistical between-study heterogeneity, a meta-analysis was performed with the fixed-effects model. If statistical heterogeneity existed, the source of heterogeneity was further analyzed. Then, the random-effects model was adopted after the effect of significant clinical heterogeneity was excluded (α = 0.05 was adopted as the test level of meta-analysis). The summary RRs were constructed using the DerSimonian–Laird random-effects model (26). Significant clinical heterogeneity was coped using subgroup analysis and sensitivity analysis, or descriptive analysis alone.
In this review, subgroup analysis was based on the brand of the stents in order to determine how this factor influenced the short- and mid-term outcomes of patients with CAD treated with BRS. When there was high between-study heterogeneity, each of the included studies in this review was excluded one after another in order to analyze the sensitivity of the overall results.
The electronic search yielded 1,337 articles. According to the eligibility criteria, a final number of 13 trials with 9,702 patients (with 5,328 patients in the BRS arm and 4,374 patients in the DES arm) were included in this meta-analysis (see Figure 1 for the specific selection process). All the trials were multicenter, except for the EVERBIO II trial, which was a single-center trial (27). Both the TROFI II trial and the ISAR-Absorb MI trial reported outcomes at 6 months (28, 29), while the EVEROBIO II trial reported outcomes at 9 months (27). The other 10 trials all reported outcomes at 1 year (10, 30–38). Among all the included trials, the BRS could be divided into four brands, including ABSORB BVS, NEOVAS, XINSORB, and MAGMARIS. The former three brands were PLLA-based scaffolds, and the latter one was magnesium-based. The follow-up duration reported in the studies ranged from 6 months to 1 year. The mean age of participants was 57.4–67.0 years. The percentage of women was 10.6–32.7% in those studies. The proportion of participants diagnosed with diabetes was 16.0–35.35%. Six included studies applied the “adequate pre-dilation, accurate sizing, and adequate post-dilation” (PSP) technique to PCI (refer to Table 1 for summarized baseline characteristics of the studies included).
The risk of bias for each included study was evaluated using the Cochrane Collaboration's tool for assessing the risk of bias. The results are shown in Figure 2. Out of the 13 included trials, 10 were rated as low risk of bias, while 2 were found to have a high risk of bias and the rest 1 was unclear in terms of risk of bias. The overall risk of bias was low. Funnel plots (Figure 3) were generated using STATA to make a qualitative analysis for publication bias. According to those figures, no significant publication bias was found. Also, Egger's test (39) and Begg's test were performed using STATA. The results showed p > 0.05 for each outcome indicator. Therefore, publication bias was not found.
Figure 2. Assessment on risk of bias. (A) The overall risk of bias. (B) Risk of bias for specific studies.
Figure 3. Funnel plots for outcomes. (A) TLF. (B) TLR. (C) TVMI. (D) TVR. (E) Cardiac death. (F) Follow-up DS.
A total of 12 included studies reported the TLF rate within 1 year, involving 9,361 participants. Out of 5,157 participants treated with BRS, 330 turned out to have TLF, while 223 had TLF among the 4,204 participants in the DES group. The results of the heterogeneity test showed p = 0.93 and I2 = 0; thus, the fixed-effects model was adopted. According to the results of the meta-analysis, the incidence of TLF in the BRS group was greater than that in the DES group [RR = 1.22, 95% CI (1.03, 1.44); I2 = 0%; p = 0.02], mainly driven by the higher rate of TVMI [RR, 1.39, 95% CI (1.09, 1.76); I2 = 0%; p = 0.008] (see Figure 4 for forest plots). Also, a subgroup analysis was additionally conducted among the studies that applied ABSORB BVS. Still, the results showed that the incidence of TLF was greater in the BRS group, with RR = 1.22, 95% CI (1.02, 1.46); I2 = 0%; p = 0.03. Since the heterogeneity was high among the studies applying other brands of stents, further subgroup analysis was not performed.
A total of 11 included studies reported in-stent DS% within 1 year. The total between-study heterogeneity was high (I2 = 97%). A subgroup analysis was additionally conducted among the studies that applied ABSORB BVS and other types, respectively. However, the results showed high heterogeneity. We also performed subgroup analyses according to the sample size and AMI population, but they showed similar results. Because there were no more available data of in-stent DS% on detailed subgroups such as poststent dilation, ACS, and DM population, we were unable to further analyze the reasons for such high heterogeneity. Therefore, a random effect model was used (refer to Figure 5 for details). Compared with the DES group, the BRS group had a significantly higher in-stent DS% within 1 year [MD = 5.23, 95%CI (3.43, 7.04); I2 = 97%; p < 0.00001]. A sensitivity analysis was also conducted. We removed one study at a time and recalculated the combined estimate on the remaining studies. The sensitivity analysis did not identify the apparent difference.
Other outcomes in this review included TLR and cardiac death. Compared with DES, BRS had a similar risk of TLR [RR = 1.19, 95% CI (0.92, 1.54); I2 = 0%; p = 0.18] and cardiac death [RR = 1.31, 95% CI (0.72, 2.38); I2 = 0%; p = 0.37]. The results of the meta-analysis on those outcomes are summarized in Table 2.
In this meta-analysis, a total of 13 RCTs were included, involving 9,702 patients with coronary artery disease undergoing PCI. The result indicated a higher target lesion failure in BRS compared with DES, mainly driven by a higher risk of TVMI. Also, in the BRS group, a significantly increased risk for in-stent DS% within 1 year was observed as compared to DES. The incidence of TLR and cardiac death was not significantly different between the two groups.
This review mainly focused on the outcomes of BRS implantation at short- and mid-term follow-up. While the current concerns about BRS centered on late/very late scaffold thrombosis (40) and late lumen loss (41), less attention had been paid to short- and mid-term follow-up. Since the peak of DS rate after stenting showed within 1 year, the incidence of TLF within 1 year was defined as the short- and mid-term outcome in this review. Generally speaking, the peak rate of neointimal hyperplasia occurs in 3–6 months (42) after BMS implantation, whereas in the case of DES, the peak rate was delayed (43) due to the application of anti-proliferative drugs. No related data on BRS were available. However, since its drug release rate is similar to that of DES, we suppose that the peak time of neointimal hyperplasia in the case of BRS may be similar to that of DES. Therefore, the follow-up was set at 6 months to 1 year in this review.
Most clinical research on coronary stents tended to adopt TLF as the primary outcome. TLF included all the events related to the target lesion directly, namely, TLR, TVMI, and cardiac death (33). They were also referred to as device-oriented composite endpoint (DoCE) in that those events reflected the safety associated with device implantation. In addition to TLF, lumen gain or loss within the stented segment was another important outcome, as it provided a more visualized comparison of the safety among different stents. In general, it was obtained through coronary angiography, OCT, or IVUS at follow-up. In this review, it was adopted as another important outcome.
Results of the meta-analysis in this review showed a significantly greater incidence of TLF within 1 year for BRS as compared with that for DES. This was in line with the hypothesis of this review that BRS was inferior to DES in terms of improvements in short- and mid-term prognosis. Significant statistical differences were not found in any of the included studies alone (shown in Figure 4). Hence, this result could not be observed without a meta-analysis.
When analyzing the reasons for the increased risk of TVMI, we found that there was also a higher risk of definite or probable scaffold thrombosis for BRS [RR = 2.11, 95% CI (1.36, 3.28); I2 = 0%; p = 0.0009]. Prior data from meta-analyses and real-world studies had already shown a trend of a higher risk of definite or probable scaffold thrombosis with BRS (44–46), including early scaffold thrombosis and late/very late scaffold thrombosis (9, 11, 12), which contributed to the potentially higher risk of TLR, TVMI, and cardiac death. This was in line with the results of this meta-analysis.
Further analyzing the inferiority of BRS to DES, we found that the potential reasons may lie in three aspects, including thicker scaffold struts, poorer radial support and ductility, and the stimuli to tunica intima caused by degradation products of coating. Currently, a variety of potential matrices for BRS have been explored. Moreover, many in vitro and animal experiments have been completed (47) for them. However, the majority of BRS that had passed the clinical tests in humans were made of PLLA. Although PLLA had a good balance of degradation, radial support, and biocompatibility, it could not rival metallic materials. For instance, to provide sufficient radial support, the PLLA scaffold struts needed to reach a thickness of ~150 μm (34). Consequently, the scaffold strut area was nearly four times as big as a metal one after implantation, resulting in greater thrombosis risk as demonstrated in this review and other similar studies (48). Even though the thickness of the scaffold struts for BRS had reached 150 μm, BRS was still inferior to DES in terms of support and ductility. Therefore, the prognosis may be affected by stent malapposition and under-expansion, which occurred afterward. In addition to that, the acid environment created by the degradation products of PLLA itself also stimulated local inflammatory responses in the tunica intima. In brief, all the potential factors mentioned above may contribute to PLLA-based BRS being inferior to metal-based DES.
As far as we know, this is the largest meta-analysis of RCTs up till now evaluating the short- and mid-term outcomes of BRS compared with DES. Although this meta-analysis was conducted with a robust methodology, it was not without limitations. As is known to cardiologists and clinical researchers, stenting is a special way of intervention in which the blinding of operators is almost impossible. Additionally, a certain degree of bias may occur during the implementation of intervention because the incidence of restenosis and scaffold thrombosis is closely related to the implantation technique. As regards the outcome assessors, although they were mentioned to be blinded in most included studies, the effect of blinding on them was limited in actual evaluation. The reason may lie in the fact that compared with DES, BRS had thicker stent struts and they were not visible under radiation, which made it easy for assessors to distinguish them through angiography, OCT, and IVUS. Thus, there was a certain degree of detection bias in the assessment of stenosis degree within the stent. However, most of the studies included in this review were sponsored by BRS manufacturers. In other words, the bias may be directional. Consequently, the result of BRS leading to poor prognosis at short- and mid-term follow-up may be weakened. According to the results from this review, the adverse effect of BRS on short- and mid-term prognosis may have been underestimated. In terms of publication bias, the funnel plots and Egger's and Begg's tests have been performed and no publication bias was found.
Bioresorbable scaffolds, compared with DES, had an increased risk of TLF, which was mainly driven by the higher incidence of TVMI. Also, in-stent DS% was higher with BRS. Therefore, BRS was inferior to DES at short- or mid-term follow-up in terms of target lesion outcomes. Further data of short-term follow-up from randomized trials are needed to fully evaluate and analyze the clinical outcomes with BRS before resorption of the scaffold.
The original contributions presented in the study are included in the article/Supplementary material, further inquiries can be directed to the corresponding author/s.
Y-dW and D-yW drafted the initial manuscript, registered the protocol at PROSPERO, and conducted the search, study selection, data extraction, and risk of bias assessment. D-yW developed the search strategy and rechecked and analyzed the outcomes. W-qD and S-jL were responsible for checking references. All authors contributed to the review drafting and approved the final manuscript.
The research was supported by the National Natural Science Foundation of China (81774058).
The authors declare that the research was conducted in the absence of any commercial or financial relationships that could be construed as a potential conflict of interest.
All claims expressed in this article are solely those of the authors and do not necessarily represent those of their affiliated organizations, or those of the publisher, the editors and the reviewers. Any product that may be evaluated in this article, or claim that may be made by its manufacturer, is not guaranteed or endorsed by the publisher.
The Supplementary Material for this article can be found online at: https://www.frontiersin.org/articles/10.3389/fcvm.2022.949494/full#supplementary-material
1. Palmerini T, Benedetto U, Biondi-Zoccai G, Della Riva D, Bacchi-Reggiani L, Smits PC, et al. Long-term safety of drug-eluting and bare-metal stents: evidence from a comprehensive network meta-analysis. J Am Coll Cardiol. (2015) 65:2496–507. doi: 10.1016/j.jacc.2015.04.017
2. Sarno G, Lagerqvist B, Frobert O, Nilsson J, Olivecrona G, Omerovic E, et al. Lower risk of stent thrombosis and restenosis with unrestricted use of 'new-generation' drug-eluting stents: a report from the nationwide Swedish Coronary Angiography and Angioplasty Registry (SCAAR). Eur Heart J. (2012) 33:606–13. doi: 10.1093/eurheartj/ehr479
3. Neumann FJ, Sousa-Uva M, Ahlsson A, Alfonso F, Banning AP, Benedetto U, et al. 2018 ESC/EACTS Guidelines on myocardial revascularization. Eur Heart J. (2019) 40:87–165. doi: 10.1093/eurheartj/ehy394
4. Lee SY, Hur SH, Lee SG, Kim SW, Shin DH, Kim JS, et al. Optical coherence tomographic observation of in-stent neoatherosclerosis in lesions with more than 50% neointimal area stenosis after second-generation drug-eluting stent implantation. Circul Cardiovasc Interv. (2015) 8:e001878. doi: 10.1161/CIRCINTERVENTIONS.114.001878
5. Otsuka F, Vorpahl M, Nakano M, Foerst J, Newell JB, Sakakura K, et al. Pathology of second-generation everolimus-eluting stents versus first-generation sirolimus- and paclitaxel-eluting stents in humans. Circulation. (2014) 129:211–23. doi: 10.1161/CIRCULATIONAHA.113.001790
6. Finn AV, Joner M, Nakazawa G, Kolodgie F, Newell J, John MC, et al. Pathological correlates of late drug-eluting stent thrombosis: strut coverage as a marker of endothelialization. Circulation. (2007) 115:2435–41. doi: 10.1161/CIRCULATIONAHA.107.693739
7. Finn AV, Nakazawa G, Joner M, Kolodgie FD, Mont EK, Gold HK, et al. Vascular responses to drug eluting stents: importance of delayed healing. Arterioscler Thromb Vasc Biol. (2007) 27:1500–10. doi: 10.1161/ATVBAHA.107.144220
8. Katagiri Y, Stone GW, Onuma Y, Serruys PW. State of the art: the inception, advent and future of fully bioresorbable scaffolds. EuroIntervention. (2017) 13:734–50. doi: 10.4244/EIJ-D-17-00499
9. Toyota T, Morimoto T, Shiomi H, Yoshikawa Y, Yaku H, Yamashita Y, et al. Very late scaffold thrombosis of bioresorbable vascular scaffold: systematic review and a meta-analysis. JACC Cardiovasc Interv. (2017) 10:27–37. doi: 10.1016/j.jcin.2016.10.027
10. Stone GW, Ellis SG, Gori T, Metzger DC, Stein B, Erickson M, et al. Blinded outcomes and angina assessment of coronary bioresorbable scaffolds: 30-day and 1-year results from the ABSORB IV randomised trial. Lancet (London, England). (2018) 392:1530–40. doi: 10.1016/S0140-6736(18)32283-9
11. Nairooz R, Saad M, Sardar P, Aronow WS. Two-year outcomes of bioresorbable vascular scaffold versus drug-eluting stents in coronary artery disease: a meta-analysis. Heart (British Cardiac Society). (2017) 103:1096–103. doi: 10.1136/heartjnl-2016-310886
12. Mahmoud AN, Barakat AF, Elgendy AY, Schneibel E, Mentias A, Abuzaid A, et al. Long-term efficacy and safety of everolimus-eluting bioresorbable vascular scaffolds versus everolimus-eluting metallic stents: a meta-analysis of randomized trials. Circul Cardiovasc Interv. (2017)10:5286. doi: 10.1161/CIRCINTERVENTIONS.117.005286
13. Ali ZA, Gao R, Kimura T, Onuma Y, Kereiakes DJ, Ellis SG, et al. Three-year outcomes with the absorb bioresorbable scaffold: individual-patient-data meta-analysis from the ABSORB randomized trials. Circulation. (2018) 137:464–79. doi: 10.1161/CIRCULATIONAHA.117.031843
14. Elias J, van Dongen IM, Kraak RP, Tijssen RYG, Claessen B, Tijssen JGP, et al. Mid-term and long-term safety and efficacy of bioresorbable vascular scaffolds versus metallic everolimus-eluting stents in coronary artery disease: a weighted meta-analysis of seven randomised controlled trials including 5577 patients. Neth Heart J. (2017) 25:429–38. doi: 10.1007/s12471-017-1008-x
15. Stone GW, Gao R, Kimura T, Kereiakes DJ, Ellis SG, Onuma Y, et al. 1-year outcomes with the absorb bioresorbable scaffold in patients with coronary artery disease: a patient-level, pooled meta-analysis. Lancet (London, England). (2016) 387:1277–89. doi: 10.1016/S0140-6736(15)01039-9
16. Kang SH, Chae IH, Park JJ, Lee HS, Kang DY, Hwang SS, et al. Stent thrombosis with drug-eluting stents and bioresorbable scaffolds: evidence from a network meta-analysis of 147 trials. JACC Cardiovasc Interv. (2016) 9:1203–12. doi: 10.1016/j.jcin.2016.03.038
17. Page MJ, McKenzie JE, Bossuyt PM, Boutron I, Hoffmann TC, Mulrow CD, et al. The PRISMA 2020 statement: an updated guideline for reporting systematic reviews. BMJ. (2021) 372:n71. doi: 10.1136/bmj.n71
18. Cutlip DE, Windecker S, Mehran R, Boam A, Cohen DJ, van Es GA, et al. Clinical end points in coronary stent trials: a case for standardized definitions. Circulation. (2007) 115:2344–51. doi: 10.1161/CIRCULATIONAHA.106.685313
20. Mavridis D, Chaimani A, Efthimiou O, Leucht S, Salanti G. Addressing missing outcome data in meta-analysis. Evid Based Ment Health. (2014) 17:85–9. doi: 10.1136/eb-2014-101900
22. Higgins JP, Altman DG, Gotzsche PC, Juni P, Moher D, Oxman AD, et al. The Cochrane Collaboration's tool for assessing risk of bias in randomised trials. BMJ. (2011) 343:d5928. doi: 10.1136/bmj.d5928
23. Guyatt G, Oxman AD, Akl EA, Kunz R, Vist G, Brozek J, et al. GRADE guidelines: 1. Introduction-GRADE evidence profiles and summary of findings tables. J Clin Epidemiol. (2011) 64:383–94. doi: 10.1016/j.jclinepi.2010.04.026
24. Higgins JP, Thompson SG, Deeks JJ, Altman DG. Measuring inconsistency in meta-analyses. BMJ. (2003) 327:557–60. doi: 10.1136/bmj.327.7414.557
26. DerSimonian R, Laird N. Meta-analysis in clinical trials. Control Clin Trials. (1986) 7:177–88. doi: 10.1016/0197-2456(86)90046-2
27. Puricel S, Arroyo D, Corpataux N, Baeriswyl G, Lehmann S, Kallinikou Z, et al. Comparison of everolimus- and biolimus-eluting coronary stents with everolimus-eluting bioresorbable vascular scaffolds. J Am College Cardiol. (2015) 65:791–801. doi: 10.1016/j.jacc.2014.12.017
28. Sabaté M, Windecker S, Iñiguez A, Okkels-Jensen L, Cequier A, Brugaletta S, et al. Everolimus-eluting bioresorbable stent vs. durable polymer everolimus-eluting metallic stent in patients with ST-segment elevation myocardial infarction: results of the randomized ABSORB ST-segment elevation myocardial infarction-TROFI II trial. Eur Heart J. (2016) 37:229–40. doi: 10.1093/eurheartj/ehv500
29. Byrne RA, Alfonso F, Schneider S, Maeng M, Wiebe J, Kretov E, et al. Prospective, randomized trial of bioresorbable scaffolds vs. everolimus-eluting stents in patients undergoing coronary stenting for myocardial infarction: the Intracoronary Scaffold Assessment a Randomized evaluation of Absorb in Myocardial Infarction (ISAR-Absorb MI) trial. Eur Heart J. (2019) 40:167–76. doi: 10.1093/eurheartj/ehy710
30. Ellis SG, Kereiakes DJ, Metzger DC, Caputo RP, Rizik DG, Teirstein PS, et al. Everolimus-eluting bioresorbable scaffolds for coronary artery disease. New Engl J Med. (2015) 373:1905–15. doi: 10.1056/NEJMoa1509038
31. Gao R, Yang Y, Han Y, Huo Y, Chen J, Yu B, et al. Bioresorbable vascular scaffolds versus metallic stents in patients with coronary artery disease: ABSORB China trial. J Am Coll Cardiol. (2015) 66:2298–309. doi: 10.1016/j.jacc.2015.09.054
32. Kimura T, Kozuma K, Tanabe K, Nakamura S, Yamane M, Muramatsu T, et al. A randomized trial evaluating everolimus-eluting Absorb bioresorbable scaffolds vs. everolimus-eluting metallic stents in patients with coronary artery disease: ABSORB Japan. Eur Heart J. (2015) 36:3332–42. doi: 10.1093/eurheartj/ehv435
33. Serruys PW, Chevalier B, Dudek D, Cequier A, Carrié D, Iniguez A, et al. A bioresorbable everolimus-eluting scaffold versus a metallic everolimus-eluting stent for ischaemic heart disease caused by de-novo native coronary artery lesions (ABSORB II): an interim 1-year analysis of clinical and procedural secondary outcomes from a randomised controlled trial. Lancet (London, England). (2015) 385:43–54. doi: 10.1016/S0140-6736(14)61455-0
34. Han Y, Xu B, Fu G, Wang X, Xu K, Jin C, et al. A randomized trial comparing the NeoVas sirolimus-eluting bioresorbable scaffold and metallic everolimus-eluting stents. JACC Cardiovasc Interv. (2018) 11:260–72. doi: 10.1016/j.jcin.2017.09.037
35. Sabaté M, Alfonso F, Cequier A, Romaní S, Bordes P, Serra A, et al. Magnesium-based resorbable scaffold versus permanent metallic sirolimus-eluting stent in patients with ST-segment elevation myocardial infarction: the MAGSTEMI randomized clinical trial. Circulation. (2019) 140:1904–16. doi: 10.1161/CIRCULATIONAHA.119.043467
36. Wu Y, Shen L, Yin J, Chen J, Qian J, Ge L, et al. Twelve-month angiographic and clinical outcomes of the XINSORB bioresorbable sirolimus-eluting scaffold and a metallic stent in patients with coronary artery disease. Int J Cardiol. (2019) 293:61–6. doi: 10.1016/j.ijcard.2019.06.053
37. Seo J, Ahn JM, Hong SJ, Kang DY, Hong SJ, Her AY, et al. Bioresorbable vascular scaffolds versus drug-eluting stents for diffuse long coronary narrowings. Am J Cardiol. (2020) 125:1624–30. doi: 10.1016/j.amjcard.2020.02.031
38. Smits PC, Chang CC, Chevalier B, West NEJ, Gori T, Barbato E, et al. Bioresorbable vascular scaffold versus metallic drug-eluting stent in patients at high risk of restenosis: the COMPARE-ABSORB randomised clinical trial. Eur Soc Cardiol. (2020) 16:645–53. doi: 10.4244/EIJ-D-19-01079
39. Egger M, Davey Smith G, Schneider M, Minder C. Bias in meta-analysis detected by a simple, graphical test. BMJ. (1997) 315:629–34. doi: 10.1136/bmj.315.7109.629
40. Chevalier B, Cequier A, Dudek D, Haude M, Carrie D, Sabaté M, et al. Four-year follow-up of the randomised comparison between an everolimus-eluting bioresorbable scaffold and an everolimus-eluting metallic stent for the treatment of coronary artery stenosis (ABSORB II Trial). EuroIntervention. (2018) 13:1561–4. doi: 10.4244/EIJ-D-17-00873
41. Serruys PW, Chevalier B, Sotomi Y, Cequier A, Carrié D, Piek JJ, et al. Comparison of an everolimus-eluting bioresorbable scaffold with an everolimus-eluting metallic stent for the treatment of coronary artery stenosis (ABSORB II): a 3 year, randomised, controlled, single-blind, multicentre clinical trial. Lancet (London, England). (2016) 388:2479–91. doi: 10.1016/S0140-6736(16)32050-5
42. Omeh DJ, Shlofmitz E. Restenosis. 1th edition. Treasure Island (FL): StatPearls Publishing (2019).
43. Pleva L, Kukla P, Hlinomaz O. Treatment of coronary in-stent restenosis: a systematic review. J Geriatr Cardiol. (2018) 15:173–84. doi: 10.11909/j.issn.1671-5411.2018.02.007
44. Cassese S, Byrne RA, Ndrepepa G, Kufner S, Wiebe J, Repp J, et al. Everolimus-eluting bioresorbable vascular scaffolds versus everolimus-eluting metallic stents: a meta-analysis of randomised controlled trials. Lancet (London, England). (2016) 387:537–44. doi: 10.1016/S0140-6736(15)00979-4
45. Bangalore S, Toklu B, Bhatt DL. Outcomes with bioabsorbable vascular scaffolds versus everolimus eluting stents: Insights from randomized trials. Int J Cardiol. (2016) 212:214–22. doi: 10.1016/j.ijcard.2016.03.070
46. Lipinski MJ, Escarcega RO, Baker NC, Benn HA, Gaglia MA Jr, Torguson R, et al. Scaffold thrombosis after percutaneous coronary intervention with ABSORB bioresorbable vascular scaffold: a systematic review and meta-analysis. JACC Cardiovasc Interv. (2016) 9:12–24. doi: 10.1016/j.jcin.2015.09.024
47. Lipinski MJ, Escarcega RO, Lhermusier T, Waksman R. The effects of novel, bioresorbable scaffolds on coronary vascular pathophysiology. J Cardiovasc Transl Res. (2014) 7:413–25. doi: 10.1007/s12265-014-9571-7
48. Brugaletta S, Gori T, Low AF, Tousek P, Pinar E, Gomez-Lara J, et al. Absorb bioresorbable vascular scaffold versus everolimus-eluting metallic stent in ST-segment elevation myocardial infarction: 1-year results of a propensity score matching comparison: the BVS-EXAMINATION Study (bioresorbable vascular scaffold-a clinical evaluation of everolimus eluting coronary stents in the treatment of patients with ST-segment elevation myocardial infarction). JACC Cardiovasc Interv. (2015) 8(1 Pt B):189–97. doi: 10.1016/j.jcin.2014.10.005
Keywords: bioresorbable scaffolds, drug-eluting stents, systematic review, meta-analysis, outcomes
Citation: Wan Y-d, Wang D-y, Deng W-q, Lai S-j and Wang X (2022) Bioresorbable scaffolds vs. drug-eluting stents on short- and mid-term target lesion outcomes in patients after PCI: A systematic review and meta-analysis. Front. Cardiovasc. Med. 9:949494. doi: 10.3389/fcvm.2022.949494
Received: 21 May 2022; Accepted: 01 August 2022;
Published: 08 September 2022.
Edited by:
Yohei Sotomi, Osaka University, JapanReviewed by:
Yao-Jun Zhang, Xuzhou Medical University, ChinaCopyright © 2022 Wan, Wang, Deng, Lai and Wang. This is an open-access article distributed under the terms of the Creative Commons Attribution License (CC BY). The use, distribution or reproduction in other forums is permitted, provided the original author(s) and the copyright owner(s) are credited and that the original publication in this journal is cited, in accordance with accepted academic practice. No use, distribution or reproduction is permitted which does not comply with these terms.
*Correspondence: Xian Wang, d3g2NTA1MTVAMTYzLmNvbQ==
†These authors have contributed equally to this work
Disclaimer: All claims expressed in this article are solely those of the authors and do not necessarily represent those of their affiliated organizations, or those of the publisher, the editors and the reviewers. Any product that may be evaluated in this article or claim that may be made by its manufacturer is not guaranteed or endorsed by the publisher.
Research integrity at Frontiers
Learn more about the work of our research integrity team to safeguard the quality of each article we publish.