- 1Division of Cardiology, Department of Internal Medicine, MacKay Memorial Hospital, Taipei, Taiwan
- 2Department of Medicine, Mackay Medical College, New Taipei City, Taiwan
- 3Mackay Medicine, Nursing and Management College, New Taipei City, Taiwan
- 4Division of Cardiology, Department of Internal Medicine, MacKay Memorial Hospital, Hsinchu, Taiwan
- 5Department of Gastroenterology, MacKay Memorial Hospital, Taipei, Taiwan
- 6Department of Radiology, MacKay Memorial Hospital, Taipei, Taiwan
Background: Epicardial adipose tissue (EAT) as a marker of metabolic disorders has been shown to be closely associated with a variety of unfavorable cardiovascular events and cardiac arrhythmias. Data on regional-specific visceral adiposity outside the heart and its modulation on autonomic dysfunction, particularly heart rate recovery after exercise, remain obscure.
Methods: We studied 156 consecutive subjects (mean age: 49.3 ± 8.0 years) who underwent annual health surveys and completed treadmill tests. Multi-detector computed tomography-based visceral adiposity, including EAT and peri-aortic fat (PAF) tissue, was quantified using dedicated software (Aquarius 3D Workstation, TeraRecon, San Mateo, CA, USA). We further correlated EAT and PAF with blood pressure and heart rate (HR) recovery information from an exercise treadmill test. Metabolic abnormalities were scored by anthropometrics in combination with biochemical data.
Results: Increased EAT and PAF were both associated with a smaller reduction in systolic blood pressure during the hyperventilation stage before exercise compared to supine status (β-coefficient (coef.): −0.19 and −0.23, respectively, both p < 0.05). Both visceral adipose tissue mediated an inverted relationship with heart rate recovery at 3 (EAT: β-coef.: −0.3; PAF: β-coef.: −0.36) and 6 min (EAT: β-coef.: −0.32; PAF: β-coef.: −0.34) after peak exercise, even after adjusting for baseline clinical variables and body fat composition (all p < 0.05).
Conclusion: Excessive visceral adiposity, whether proximal or distal to the heart, may modulate the autonomic response by lowering the rate of HR recovery from exercise after accounting for clinical metabolic index. Cardiac autonomic dysfunction may partly explain the increase in cardiovascular morbidity and mortality related to both visceral fats.
Introduction
Epicardial adipose tissue (EAT) and periaortic fat (PAF) are white adipose tissue but also have highly protective functions for the adjacent myocardium and vessels through brown fat-like features (1, 2). However, both adipose tissue, estimated by multi-detector computed tomography (MDCT), have been correlated with inflammatory biochemical makers, aortic calcification, the severity of coronary artery disease (CAD), myocardial remodeling, cardiac arrhythmia, and even cardiovascular mortality (3–5). The pathogenesis of both EAT and PAF has also been associated with paracrine function. Local toxic effects of fat-related inflammatory cytokines on contacting tissues, such as coronary arteries and aorta, may promote atherogenesis and lead to an increase in adverse cardiovascular events (6, 7). Additionally, intrinsic cardiac autonomic ganglia and axons are concentrated within both EAT and PAF pads (8, 9); modulation of these autonomic ganglia can induce cardiac arrhythmia, which further the potential for cardiovascular morbidity and mortality. It has also been found that local inflammatory cytokines may influence the function of nearby nerve ganglia (10, 11). However, the exact relationship between both visceral fats and the cardiac autonomic system remains unclear. Heart rate (HR) recovery is a simple and easily available diagnostic tool that reflects the cardiac autonomic tone and has been discovered to be an important predictor of cardiovascular events (12–14). Attenuated HR recovery after exercise termination indicates either a decrease in vagal tone or an increase in sympathetic activity. In this study, we aim to evaluate the relationship between EAT, PAF, and the autonomic nervous system by using MDCT and assessing HR recovery after exercise.
Materials and methods
Study population
From 2005 to 2006, MDCT was performed for the assessment of coronary disease in 156 subjects who attended an annual cardiovascular health survey in a tertiary medical center in Taipei, Taiwan (Mackay Memorial Hospital). A thorough review of baseline anthropometrics and medical history was conducted by a structured questionnaire along with a detailed physical examination. The presence of cardiovascular disease history included coronary arterial disease, stroke, or prior hospitalization for heart failure. Hypertension was defined as either systolic blood pressure (SBP) of more than 140 mmHg or diastolic blood pressure (DBP) of more than 90 mmHg. Diabetes mellitus (DM) was defined as a fasting plasma glucose level of more than 126 mg/dL or a casual blood glucose level of more than 200 mg/dL. Complete body surface electrocardiograms from 12-leads and routine chest films were performed for all subjects. No subject showed significant coronary abnormality or obvious stenosis (<50%).
Treadmill test
A standardized Bruce exercise protocol was adopted for an exercise treadmill study. HR and blood pressure during supine, standing, and standing with hyperventilation status were recorded using digitalized software. The treadmill began at 2.74 km/h (1.7 mph) at a gradient of 10%. At 3-min intervals, the incline and speed of the treadmill were increased by 2%. The maximum HR achieved was recorded and calculated as the percentage of target HR [220 beats per minute minus age in years], referenced to a value based on participant age. Participants who could not achieve more than 85% of the target HR were excluded. HR during standing and hyperventilation status were compared to that during the supine posture. We calculated the changes in HR from the peak exercise stage to those at 3- and 6-min post-exercise. SBP and DBP at 3- and 6-min post-exercise were also measured and compared to the data at peak exercise stage-derived maximal HR. All participants completed the treadmill protocol and most of the participants achieved the target heart rate and presented no strong positive treadmill results.
Baseline anthropometric measure
All baseline characteristics and anthropometric measures, including age, body height, body weight, body mass index (BMI), and waist and buttock circumferences, were collected. The standardized sphygmomanometer cuff-defined resting blood pressure was measured under resting status by medical staff blinded to the other test results. Body fat analysis was conducted by measuring bioelectrical impedance from foot to foot, performed by a Tanita-305 body-fat analyzer (Tanita Corp., Tokyo, Japan) that provided a print-out estimate of impedance and calculation of body fat. Metabolic scores were calculated from the number of abnormal items defined by the NCEP Panel III criteria (ATP III) based on measures of waist circumference (Female ≥80 cm or Male ≥90 cm), fasting glucose (≥100 mg/dL), high-density lipoprotein cholesterol (Male <40 mg/dL or Female <50 mg/dL), triglyceride (>150 mg/dL), and blood pressure (>130/85 mmHg). A 0 score represented the absence of any abnormal metabolic components, whereas 5 represented subjects with all 5 abnormal metabolic components based on the Taiwanese population (15, 16).
Laboratory data acquisition and analysis
A Hitachi 7170 Automatic Analyzer (Hitachi Corp. Hitachinaka Ibaraki, Japan) was used to measure glucose level (hexokinase method), total cholesterol, triglyceride, and uric acid. Lipid profiles including low- and high-density lipoprotein cholesterol (homogenous enzymatic colorimetric assay), blood urea nitrogen, and creatinine level (kinetic colorimetric assay) were obtained by a Hitachi 7170 Automatic Analyzer (Hitachi Corp. Hitachinaka Ibaraki, Japan). High-sensitivity C-reactive protein (Hs-CRP) levels were determined by highly sensitive, latex particle-enhanced immunoassay using Elecsys 2010 (Roche, Mannheim, Germany).
Measurements of EAT and thoracic PAF by using MDCT
Cardiac computed tomography was performed by using a 16-slice MDCT scanner (Sensation 16, Siemens Medical Solutions, Forchheim, Germany) as demonstrated in a previous study (5). In brief, images were acquired from above the level of tracheal bifurcation to below the base of the heart using prospectively ECG triggering with the center of the acquisition at 70% of the R-R interval during one breath-hold period. From the raw data, the images were reconstructed with a standard kernel in 3 mm thick axial, non-overlapping slices, and a 25 cm field of view. Visceral adipose tissue of EAT and PAF was quantified by MDCT using a dedicated workstation (Aquarius 3D Workstation, TeraRecon, San Mateo, CA, USA). We traced the region of interest manually and defined fat tissue as pixels within a window of −195 to −45 Hounsfield unit (HU) and a window center of −120 HU. EAT was defined as any adipose tissue located within the pericardial sac (Figures 1A,B). Thoracic PAF was defined as the adipose tissue surrounding the thoracic aorta, which extended 67.5 mm from the level of the bifurcation of pulmonary arteries (Figures 1C,D) with cranial-caudal coverage of the thoracic aorta. Detailed methods conducted in our laboratory for similar measures have been published elsewhere (5, 17, 18).
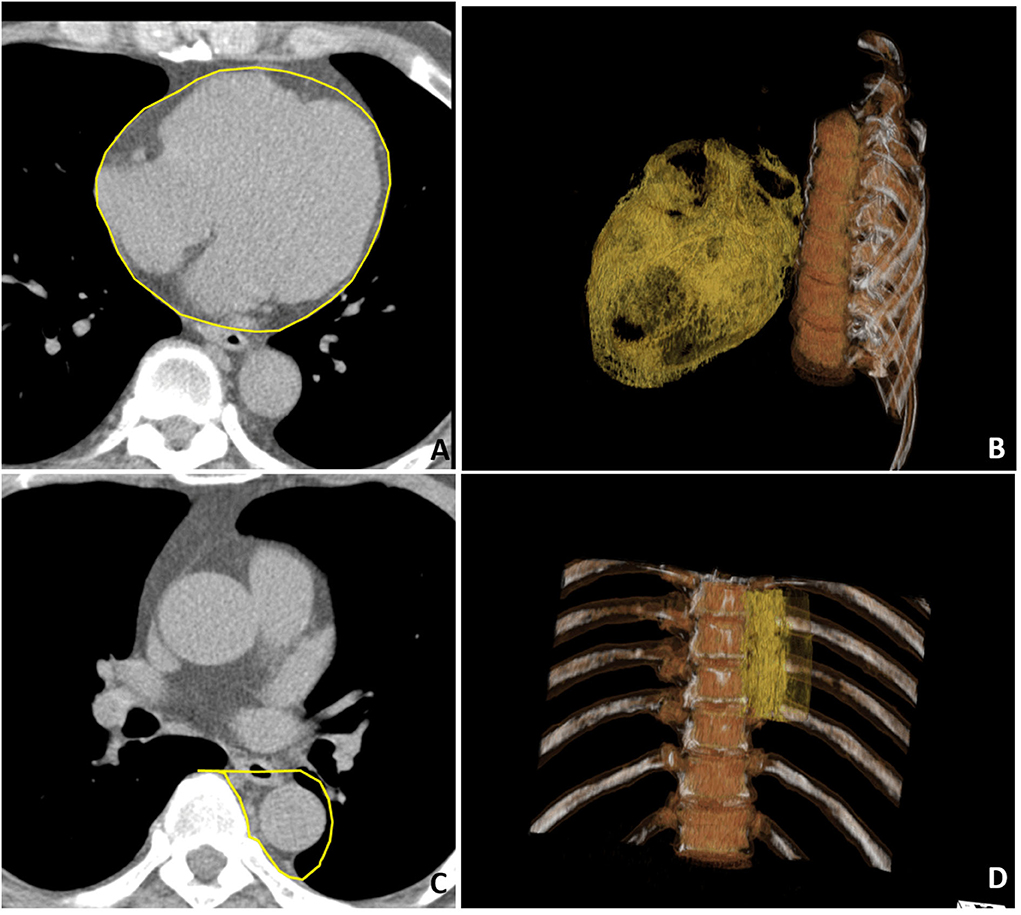
Figure 1. Multidetector computed tomography (MDCT) demonstrated regional-specific adiposity measures including (A,B) epicardial adipose tissue (EAT) and (C,D) peri-aortic fat (PAF) in (A,C) axial view and (B,D) three-dimensional (3D) reconstruction. (A) The EAT was defined as the fat inside the pericardial sac with pericardium labeled in yellow color. (C) The PAF was defined as the fat surrounding the thoracic descending aorta with the region of interest labeled in yellow. In the 3D reconstruction of the (B) EAT and (D) PAF, where orange regions indicate visceral fat tissue.
Statistical analysis
Continuous data were expressed as the mean and standard deviation and compared using a non-parametric trend test (Wilcoxon rank-sum test) across ordered age groups with categorical data expressed as the frequency and proportion of occurrence in all subjects. Differences in baseline demographics between groups were tested with a one-way analysis of variance (ANOVA) test with categorical data analyzed by chi-square or Fisher's exact test as appropriate. The Wilcoxon rank-sum test was used to estimate the trend of all continuous data and ordinal variables across all ordered groups. Uni- and multi-variable logistic regression models were used to determine the significant factors in the prediction of HR recovery with individual odds ratios, and significance (p-value) and 95% confidence intervals were reported. All data were analyzed by the commercialized software STATA 8.0 package (Stata Corp., College Station, Texas). An α-value of significance for all analyses was two-sided with 0.05 was considered to be statistically significant.
Results
Baseline information
Among 156 consecutively enrolled participants (mean age: 49.3±8.0 years), two of them were excluded from the assessment of the EAT because of the inadequate quality of the images. The average EAT and PAF were 75.8 ± 29.1 and 7.5 ± 3.9 ml, respectively. Tables 1, 2 list the baseline demographic data based on EAT and PAF of the participants in this study. With increasing EAT amount, the age and female gender was increasing in a significant trend (p < 0.001). Anthropometric data including body height, weight, body mass index, buttock or waist circumferences, and waist-to-hip ratio all increased in a significant trend across the EAT and PAF tertile groups (all trends p < 0.001). An ordered increase in baseline SBPs and DBPs and HRs was also observed across the tertiles (all trends p < 0.001). While the history of DM showed a non-significant association, hypertension increased in a significant fashion with increasing EAT and PAF in our study (both p < 0.01).
We also demonstrated the association between baseline biochemistry and PAF burden. Elevated fasting blood glucose level, insulin, homeostatic model assessment for insulin resistance, and glycated hemoglobin product were observed to correlate with higher PAF burden (all trends p < 0.001). In addition, both EAT and PAF volume also positively correlated with serum triglyceride (both p < 0.05) and showed an inverse relationship with high-density lipoprotein cholesterol level (both p < 0.001). Serum uric acid as well as systemic inflammatory marker Hs-CRP were observed to increase across the EAT and PAF tertiles (both p < 0.05).
The relationship between standard treadmill parameters and both visceral fat amount
Resting status HRs including those taken while supine and standing as well as hyperventilation showed an increasing trend, with a general trend toward higher heart rates in the third EAT and PAF tertile groups (without statistical significance) (Figure 2). In Table 3, we showed that maximal HR was significantly reduced in the third EAT and PAF tertiles (both p < 0.05). In Table 4, we showed that increasing both types of visceral fats was associated with less of a reduction in SBP during the hyperventilation stage compared to that during the supine status stage (β-coefficient [coef.]: −0.23 and −0.19 for PAF and EAT, respectively, both p < 0.05). In addition, there were significantly inverted associations between PAF and HR increase (β-coef.: −0.2, p= 0.014), with a borderline inverse relationship between a larger EAT and HR increase (β-coef.: −0.15, p = 0.061) during the hyperventilation stage compared with that during baseline status. Finally, we also observed that both higher visceral fats were associated with significantly attenuated HR recovery at 3 (β-coef.: −0.32 and −0.28 for PAF & EAT, respectively, both p ≤ 0.001) and 6 min (β-coef.: −0.40 and −0.34 for PAF and EAT, respectively, both p < 0.001) from maximal heart rate after maximal exercise (Figure 3).
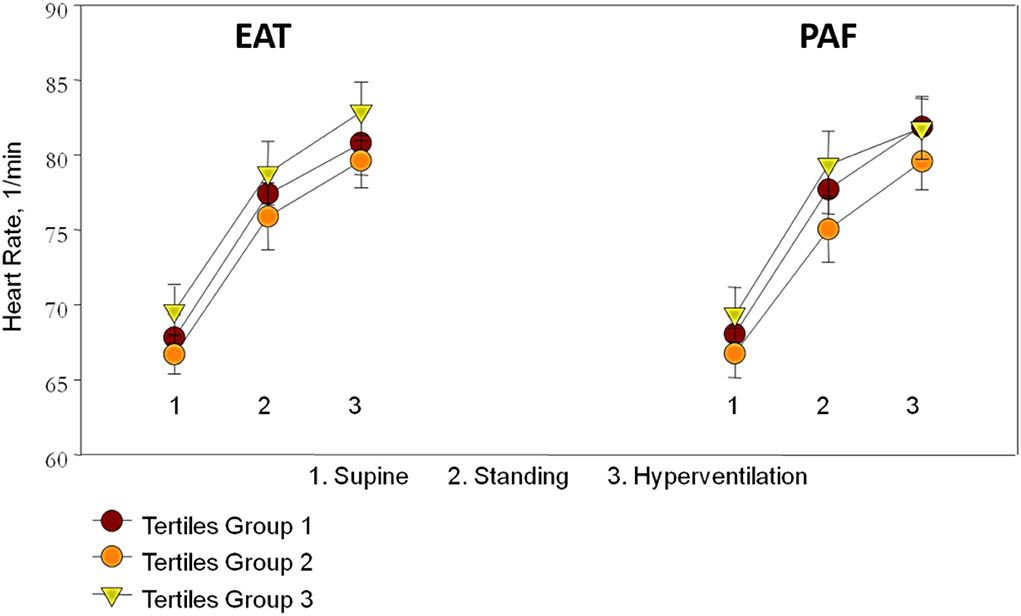
Figure 2. The association between resting status heart rate (HR) including sequential supine, standing postures, hyperventilation, and the tertile groups of epicardial adipose tissue (EAT) and peri-aortic fat (PAF) showed an increased trend though not reaching statistical significance.
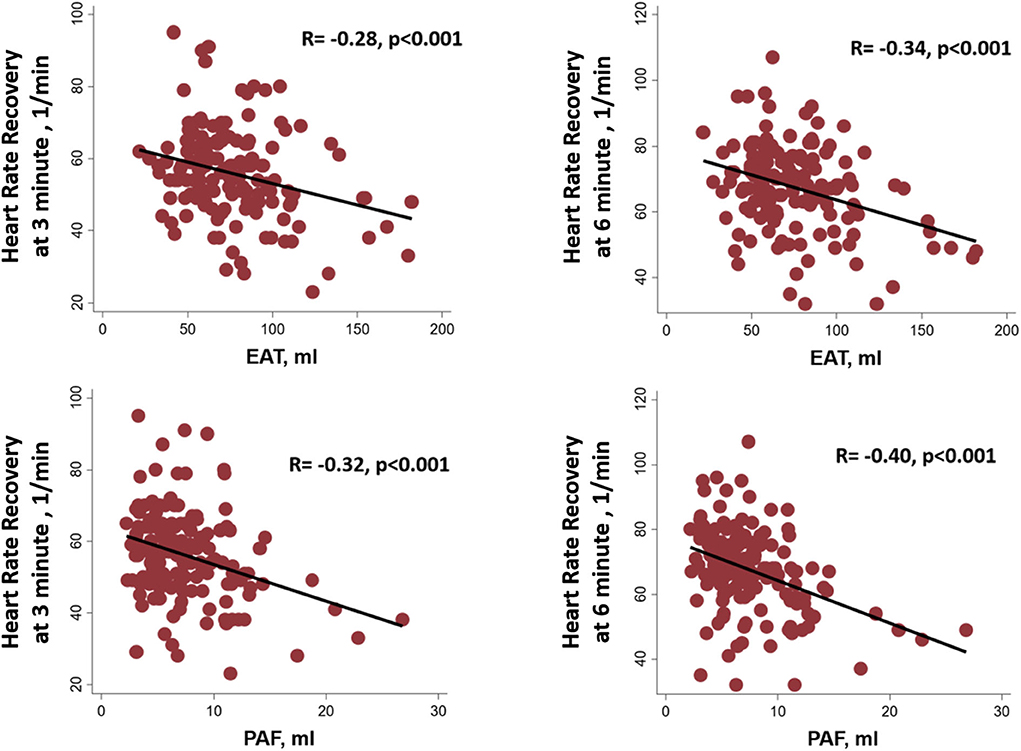
Figure 3. Linear relationships among the epicardial adipose tissue (EAT), peri-aortic fat (PAF), and heart rate recovery at 3 and 6 min from maximal heart rate after maximal exercise.
Multi-variable models relating treadmill parameters and both visceral fat amount
Table 5 further demonstrated the multi-variable models with anthropometrics based on BMI between various treadmill parameters and both types of visceral fat burden. We observed that HR changes during standing and hyperventilation from resting status had borderline associations with PAF and EAT. There was a consistent inverse relationship between the visceral fat amount and HR recovery at 3 (β-coef.: −0.36 and −0.30 for PAF and EAT, respectively, both p < 0.05) and 6 min (β-coef.: −0.34 and −0.32 for PAF and EAT, respectively, both p < 0.05) after adjusting for baseline clinical variables and body fat composition.
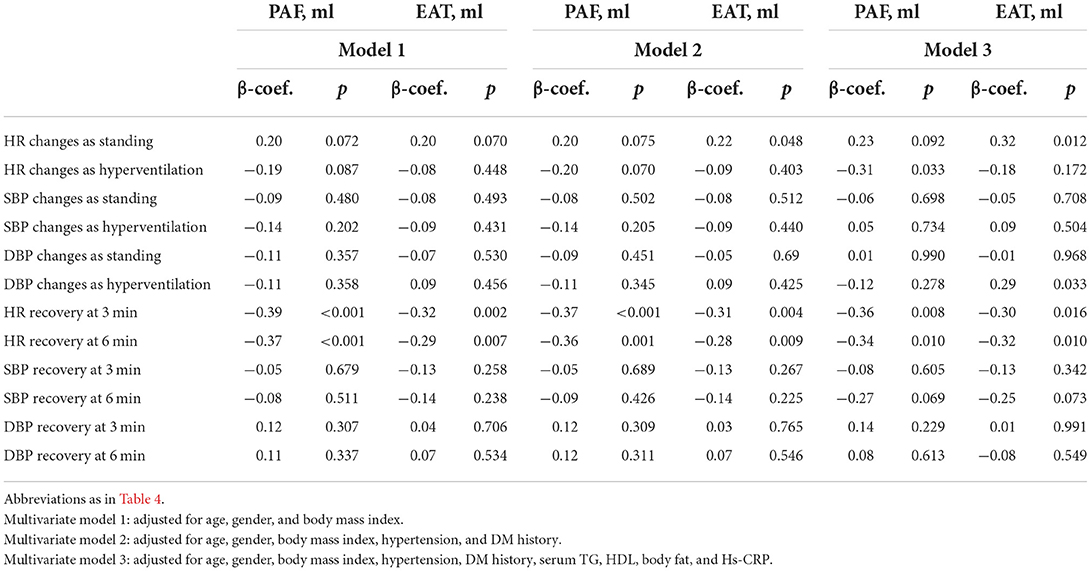
Table 5. The association of visceral adipose tissue and standard treadmill parameters on multi-variable regression models.
Discussion
To the best of our knowledge, this was the first study to examine the association between PAF and EAT and cardiac autonomic function in terms of HR recovery from the peak exercise treadmill program. We found that both EAT and PAF volumes exert an inverted relationship with HR recovery, indicating the potential adverse effects of both visceral fats on cardiac autonomic function. The associations persisted even after adjustment for clinical variables, anthropometric data, and BMI.
Visceral fat, cardiovascular risk factors, and cardiac autonomic function
HR is modulated by both the vagal and sympathetic nerve systems. HR recovery within a few minutes after exercise termination is a simple and easily available measure of cardiac autonomic function. The period of HR recovery after maximum exercise results from a combination of parasympathetic reactivation and sympathetic withdrawal. An attenuated HR recovery, indicating a decrease in the parasympathetic nervous system activity and/or increase in the sympathetic tone, predicts poor cardiovascular outcomes (12, 14, 19, 20). Previous studies have revealed an association between HR recovery and cardiovascular diseases, including atrial fibrillation, CAD, left ventricular diastolic dysfunction, and endothelial dysfunction (12, 21–23). The association between HR recovery and clinical cardiovascular risk factors, including hypertension, insulin resistance, dyslipidemia, and obesity, was also noted (24, 25). These conditions often coexist with increased EAT and PAF volume as shown in this and other studies (3, 5, 26–28). Previous reports have suggested that epicardial fat thickness measured on echocardiography is associated with blunted HR recovery (21, 22). However, their study groups were restricted to either men or patients with metabolic syndrome, and only epicardial fat over the free wall of the right ventricular was estimated (29, 30). Epicardial fat thickness measured using echocardiography has been suggested to provide less accurate data than epicardial fat volume measured using cardiac computed tomography (31). In this study, using a previously validated MDCT technique, we were able to estimate the total volume of both EAT and PAF. We also extended the findings by demonstrating strong correlations between both visceral fat burden and attenuated HR recovery in a cohort of the general population who answered a cardiovascular health survey even after adjustment for age, gender, BMI, waist, total body fat, cholesterol, triglyceride, and history of hypertension and DM. Our findings suggest that both EAT and PAF are more influential than systemic adiposity in cardiac autonomic function. Additionally, cardiac autonomic dysfunction, indicated by blunted HR recovery, may partly explain the increase in cardiovascular morbidity and mortality related to both visceral fats.
Potential mechanism
Although the volume is small, EAT is a rich source of free fatty acids (FFA) because the rate of FFA synthesis and release in response to catecholamine is higher than that in other adipose tissues (32). Elevated plasma FFA level is known to alter the autonomic system by stimulating cardiac sympathetic nervous activity in both healthy subjects and DM patients (33, 34). Both EAT and PAF are also metabolically active tissues that secret cytokines and have effects on other organs through their paracrine function (6, 7). Local toxic effects of fat-related inflammatory cytokines on contact with tissues, such as coronary arteries, aorta, and myocardium, have been found to promote atherogenesis and fibrosis and lead to CAD, aortic calcification, and myocardial dysfunction (3, 5, 7). Both EAT and PAF embed intrinsic cardiac autonomic ganglia and axons, which innervate atria, ventricles, and conduction system and modulate the HR (8, 9). A previous animal study has demonstrated that interleukin-1β, an inflammatory cytokine known to increase pericardial fat (6), could modulate the excitability of ganglion neurons through a paracrine mechanism (10). Tumor necrosis factor-α, another cytokine released from pericardial fat (6), alters the cellular function of sympathetic neurons (11). Adiponectin, a protein secreted by adipocytes, can decrease the activity of autonomic ganglia (35). Thereafter, the abnormality of cardiac autonomic response manifesting reduced heart rate recovery after exercise may also result from the pathogenic effect of local inflammatory cytokines secreted from nearby visceral fats.
Study limitations
First, this study design was cross-sectional and limited inferences of causality. Second, the study sample was comprised of Asian participants only, and thus it is uncertain if the findings may be applied to other ethnic groups. Third, we did not have complete HR recovery at 1 or 2 min after exercise in our database. However, a previous study demonstrated that a decreased HR recovery, even measured 5 min after exercise recovery, was still predictive of cardiovascular mortality (13). Our findings demonstrated that there were strong correlations between both visceral fats and HR recovery at 3 and 6 min after maximum exercise. Fourth, we did not show how the cytokines that are released by the adipose tissue are in the different tertiles. The mechanism of increased visceral adipose tissue impaired cardiac autonomic function needs further study. Finally, we do not have complete data on medications. However, the medicines that could influence the heart rate or autonomic system, e.g., beta-blockers or calcium-channel blockers, had been demonstrated to be independent of the abnormal value for HR recovery in a previous study (12). Angiotensin-converting enzyme inhibitors may also have vagomimetic activity but were proved to have no effect on HR recovery values from 3 to 10 min after maximum exercise (36). Sodium–glucose cotransporter type-2 inhibitors could potentially improve cardiac autonomic function but were not available in the period of analysis. Furthermore, we adjusted the HR recovery data for hypertension and DM, which should help reduce any potential effect modification by medications.
Conclusion
The association between both visceral fats and blunted HR recovery was independent of traditional cardiovascular risk factors and stronger than more systemic measures of adipose tissues. Our findings showed that cardiac autonomic dysfunction, indicated by blunted HR recovery, may partly explain the increase in cardiovascular morbidity and mortality related to both visceral fats.
Data availability statement
The raw data supporting the conclusions of this article will be made available by the authors, without undue reservation.
Ethics statement
The studies involving human participants were reviewed and approved by Local Ethical Institutional Committee (Mackay Memorial Hospital), IRB No. 09MMHIS036. The patients/participants provided their written informed consent to participate in this study.
Author contributions
J-YK and S-HC collected data, analyzed data, and wrote the article. C-LH, P-HC, and C-TT conceived, designed the study, and edited the article. C-LH performed the statistical analysis. J-YK and J-PT acquisition of data, analysis, and interpretation of data. T-CH, CH, Y-HL, C-YL, W-MH, and C-LH conceptual frame work. C-HY, H-IY, and C-LH reviewed article. H-IY and C-LH have given final approval to the version to be published. All authors read and approved the final manuscript.
Funding
This research was supported by the Ministry of Science and Technology (Taiwan) (Grants NSC-101-2314-B-195-020, NSC103-2314-B-010-005-MY3, 103-2314-B-195-001-MY3, 101-2314-B-195-020–MY1, MOST 103-2314-B-195-006-MY3, NSC102-2314-B-002-046-MY3, 106-2314-B-195-008-MY2, 108-2314-B-195-018-MY2, MOST 108-2314-B-195-018-MY2, MOST 109-2314-B-715-008, and MOST 110-2314-B-715-009-MY1), MacKay Memorial Hospital (10271, 10248, 10220, 10253, 10375, 10358, and E-102003), and the Taiwan Foundation for geriatric emergency and critical care.
Acknowledgments
We thank C-HY for his assistance with CT data measurement and imaging analysis.
Conflict of interest
The authors declare that the research was conducted in the absence of any commercial or financial relationships that could be construed as a potential conflict of interest.
Publisher's note
All claims expressed in this article are solely those of the authors and do not necessarily represent those of their affiliated organizations, or those of the publisher, the editors and the reviewers. Any product that may be evaluated in this article, or claim that may be made by its manufacturer, is not guaranteed or endorsed by the publisher.
References
1. Sacks HS, Fain JN, Bahouth SW, Ojha S, Frontini A, Budge H, et al. Human epicardial fat exhibits beige features. J Clin Endocrinol Metab. (2013) 98:E1448–55. doi: 10.1210/jc.2013-1265
2. Vargas D, Camacho J, Duque J, Carreño M, Acero E, Pérez M, et al. Functional characterization of preadipocytes derived from human periaortic adipose tissue. Int J Endocrinol. (2017) 2017:9. doi: 10.1155/2017/2945012
3. Rosito GA, Massaro JM, Hoffmann U, Ruberg FL, Mahabadi AA, Vasan RS, et al. Pericardial fat, visceral abdominal fat, cardiovascular disease risk factors, and vascular calcification in a community-based sample: the framingham heart study. Circulation. (2008)117:605–13. doi: 10.1161/CIRCULATIONAHA.107.743062
4. Cheng VY, Dey D, Tamarappoo B, Nakazato R, Gransar H, Miranda-Peats R, et al. Pericardial fat burden on ecg-gated noncontrast ct in asymptomatic patients who subsequently experience adverse cardiovascular events. JACC Cardiovasc Imaging. (2010) 3:352–60. doi: 10.1016/j.jcmg.2009.12.013
5. Yun CH, Lin TY, Wu YJ, Liu CC, Kuo JY, Yeh HI, et al. Pericardial and thoracic peri-aortic adipose tissues contribute to systemic inflammation and calcified coronary atherosclerosis independent of body fat composition, anthropometric measures and traditional cardiovascular risks. Eur J Radiol. (2012) 81:749–56. doi: 10.1016/j.ejrad.2011.01.035
6. Mazurek T, Zhang L, Zalewski A, Mannion JD, Diehl JT, Arafat H, et al. Human epicardial adipose tissue is a source of inflammatory mediators. Circulation. (2003) 108:2460–6. doi: 10.1161/01.CIR.0000099542.57313.C5
7. Henrichot E, Juge-Aubry CE, Pernin A, Pache JC, Velebit V, Dayer JM, et al. Production of chemokines by perivascular adipose tissue: a role in the pathogenesis of atherosclerosis? Arterioscler Thromb Vasc Biol. (2005) 25:2594–9. doi: 10.1161/01.ATV.0000188508.40052.35
8. Singh S, Johnson PI, Lee RE, Orfei E, Lonchyna VA, Sullivan HJ, et al. Topography of cardiac ganglia in the adult human heart. J Thorac Cardiovasc Surg. (1996) 112:943–53. doi: 10.1016/S0022-5223(96)70094-6
9. Hou Y, Scherlag BJ, Lin J, Zhang Y, Lu Z, Truong K, et al. Ganglionated plexi modulate extrinsic cardiac autonomic nerve input: Effects on sinus rate, atrioventricular conduction, refractoriness, and inducibility of atrial fibrillation. J Am Coll Cardiol. (2007) 50:61–8. doi: 10.1016/j.jacc.2007.02.066
10. Takeda M, Takahashi M, Matsumoto S. Contribution of activated interleukin receptors in trigeminal ganglion neurons to hyperalgesia via satellite glial interleukin-1beta paracrine mechanism. Brain Behav Immun. (2008) 22:1016–23. doi: 10.1016/j.bbi.2008.03.004
11. Soliven B, Albert J. Tumor necrosis factor modulates Ca2+ currents in cultured sympathetic neurons. J Neurosci. (1992) 12:2665–71. doi: 10.1523/JNEUROSCI.12-07-02665.1992
12. Cole CR, Blackstone EH, Pashkow FJ, Snader CE, Lauer MS. Heart-rate recovery immediately after exercise as a predictor of mortality. N Engl J Med. (1999) 341:1351–7. doi: 10.1056/NEJM199910283411804
13. Cheng YJ, Lauer MS, Earnest CP, Church TS, Kampert JB, Gibbons LW, et al. Heart rate recovery following maximal exercise testing as a predictor of cardiovascular disease and all-cause mortality in men with diabetes. Diabetes Care. (2003) 26:2052–7. doi: 10.2337/diacare.26.7.2052
14. Vivekananthan DP, Blackstone EH, Pothier CE, Lauer MS. Heart rate recovery after exercise is a predictor of mortality, independent of the angiographic severity of coronary disease. J Am Coll Cardiol. (2003) 42:831–8. doi: 10.1016/s0735-1097(03)00833-7
15. Alberti KG, Eckel RH, Grundy SM, Zimmet PZ, Cleeman JI, Donato KA, et al. Harmonizing the metabolic syndrome: a joint interim statement of the international diabetes federation task force on epidemiology and prevention; national heart, lung, and blood institute; american heart association; world heart federation; international atherosclerosis society; and international association for the study of obesity. Circulation. (2009) 120:1640–5. doi: 10.1161/CIRCULATIONAHA.109.192644
16. Fan JG, Saibara T, Chitturi S, Kim BI, Sung JJ, Chutaputti A. What are the risk factors and settings for non-alcoholic fatty liver disease in Asia-Pacific. J Gastroenterol Hepatol. (2007) 22:794–800. doi: 10.1111/j.1440-1746.2007.04952.x
17. Sung KT, Kuo R, Sun JY, Hung TC, Chang SC, Liu CC, et al. Associations between CT-determined visceral fat burden, hepatic steatosis, circulating white blood cell counts and neutrophil-to-lymphocyte ratio. PLoS ONE. (2018) 13:e0207284. doi: 10.1371/journal.pone.0207284
18. Yang FS, Yun CH, Wu TH, Hsieh YC, Bezerra HG, Liu CC, et al. High pericardial and peri-aortic adipose tissue burden in pre-diabetic and diabetic subjects. BMC Cardiovasc Disord. (2013) 13:98. doi: 10.1186/1471-2261-13-98
19. Arena R, Guazzi M, Myers J, Peberdy MA. Prognostic value of heart rate recovery in patients with heart failure. Am Heart J. (2006) 151:851–13. doi: 10.1016/j.ahj.2005.09.012
20. Kannankeril PJ, Le FK, Kadish AH, Goldberger JJ. Parasympathetic effects on heart rate recovery after exercise. J Investig Med. (2004) 52:394–401. doi: 10.1136/jim-52-06-34
21. Gera N, Taillon LA, Ward RP. Usefulness of abnormal heart rate recovery on exercise stress testing to predict high-risk findings on single-photon emission computed tomography myocardial perfusion imaging in men. Am J Cardiol. (2009) 103:611–4. doi: 10.1016/j.amjcard.2008.11.004
22. Maddox TM, Ross C, Ho PM, Magid D, Rumsfeld JS. Impaired heart rate recovery is associated with new-onset atrial fibrillation: a prospective cohort study. BMC Cardiovasc Disord. (2009) 9:11. doi: 10.1186/1471-2261-9-11
23. Huang PH, Leu HB, Chen JW, Cheng CM, Huang CY, Tuan TC, et al. Usefulness of attenuated heart rate recovery immediately after exercise to predict endothelial dysfunction in patients with suspected coronary artery disease. Am J Cardiol. (2004) 93:10–3. doi: 10.1016/j.amjcard.2003.09.004
24. Spies C, Otte C, Kanaya A, Pipkin SS, Schiller NB, Whooley MA. Association of metabolic syndrome with exercise capacity and heart rate recovery in patients with coronary heart disease in the heart and soul study. Am J Cardiol. (2005) 95:1175–9. doi: 10.1016/j.amjcard.2005.01.045
25. Yeckel CW, Gulanski B, Zgorski ML, Dziura J, Parish R, Sherwin RS. Simple exercise recovery index for sympathetic overactivity is linked to insulin resistance. Med Sci Sports Exerc. (2009) 41:505–15. doi: 10.1249/mss.0b013e31818afa2f
26. Al Chekakie MO, Welles CC, Metoyer R, Ibrahim A, Shapira AR, Cytron J, et al. Pericardial fat is independently associated with human atrial fibrillation. J Am Coll Cardiol. (2010) 56:784–8. doi: 10.1016/j.jacc.2010.03.071
27. Aydin H, Toprak A, Deyneli O, Yazici D, Tarcin O, Sancak S, et al. Epicardial fat tissue thickness correlates with endothelial dysfunction and other cardiovascular risk factors in patients with metabolic syndrome. Metab Syndr Relat Disord. (2010) 8:229–34. doi: 10.1089/met.2009.0080
28. Iacobellis G, Leonetti F, Singh N. Relationship of epicardial adipose tissue with atrial dimensions and diastolic function in morbidly obese subjects. Int J Cardiol. (2007) 115:272–3. doi: 10.1016/j.ijcard.2006.04.016
29. Kim MK, Tanaka K, Kim MJ, Matsuo T, Tomita T, Ohkubo H, et al. Epicardial fat tissue: Relationship with cardiorespiratory fitness in men. Med Sci Sports Exerc. (2010) 42:463–9. doi: 10.1249/mss.0b013e3181b8b1f0
30. Sengul C, Duman D. The association of epicardial fat thickness with blunted heart rate recovery in patients with metabolic syndrome. Tohoku J Exp Med. (2011) 224:257–62. doi.org/10.1620/tjem.224.257
31. Greif M, Becker A, von Ziegler F, Lebherz C, Lehrke M, Broedl UC, et al. Pericardial adipose tissue determined by dual source ct is a risk factor for coronary atherosclerosis. Arterioscler Thromb Vasc Biol. (2009) 29:781–6. doi: 10.1161/ATVBAHA.108.180653
32. Marchington JM, Mattacks CA, Pond CM. Adipose tissue in the mammalian heart and pericardium: Structure, foetal development and biochemical properties. Comp Biochem Physiol B. (1989) 94:225–32. doi: 10.1016/0305-0491(89)90337-4
33. Paolisso G, Manzella D, Rizzo MR, Ragno E, Barbieri M, Varricchio G, et al. Elevated plasma fatty acid concentrations stimulate the cardiac autonomic nervous system in healthy subjects. Am J Clin Nutr. (2000) 72:723–30. doi: 10.1093/ajcn/72.3.723
34. Manzella D, Barbieri M, Rizzo MR, Ragno E, Passariello N, Gambardella A, et al. Role of free fatty acids on cardiac autonomic nervous system in noninsulin-dependent diabetic patients: Effects of metabolic control. J Clin Endocrinol Metab. (2001) 86:2769–74. doi: 10.1210/jcem.86.6.7553
35. Zhou Z, Li SY, Sheng X, Liu ZH, Lai YQ, Wang ML, et al. Interactions between metabolism regulator adiponectin and intrinsic cardiac autonomic nervous system: a potential treatment target for atrial fibrillation. Int J Cardiol. (2020) 302:59–66. doi: 10.1016/j.ijcard.2019.12.031
Keywords: epicardial adipose tissue, peri-aortic adipose tissue, multidetector computed tomography, heart rate recovery, visceral adiposity measures
Citation: Chang S-H, Chu P-H, Tsai C-T, Kuo J-Y, Tsai J-P, Hung T-C, Hou CJ-Y, Lai Y-H, Liu C-Y, Huang W-M, Yun C-H, Yeh H-I and Hung C-L (2022) Both epicardial and peri-aortic adipose tissue blunt heart rate recovery beyond body fat mass. Front. Cardiovasc. Med. 9:939515. doi: 10.3389/fcvm.2022.939515
Received: 09 May 2022; Accepted: 12 August 2022;
Published: 21 September 2022.
Edited by:
Carolina Restini, Michigan State University, United StatesReviewed by:
Milene Tavares Fontes, University of South Carolina, United StatesFernanda Priviero, University of South Carolina, United States
Copyright © 2022 Chang, Chu, Tsai, Kuo, Tsai, Hung, Hou, Lai, Liu, Huang, Yun, Yeh and Hung. This is an open-access article distributed under the terms of the Creative Commons Attribution License (CC BY). The use, distribution or reproduction in other forums is permitted, provided the original author(s) and the copyright owner(s) are credited and that the original publication in this journal is cited, in accordance with accepted academic practice. No use, distribution or reproduction is permitted which does not comply with these terms.
*Correspondence: Chun-Ho Yun, bWVkMjAyNjU3QGdtYWlsLmNvbQ==; Chung-Lieh Hung, am90YXJvMzc5MUBnbWFpbC5jb20=