- 1New York Institute of Technology, College of Osteopathic Medicine, Academic Medicine Scholar Program, Old Westbury, NY, United States
- 2Department of Biomedical Sciences, New York Institute of Technology College of Osteopathic Medicine, Old Westbury, NY, United States
Background: Many patients treated with Vitamin K antagonists (VKA) for anticoagulation have concomitant vascular or valvular calcification. This meta-analysis aimed to evaluate a hypothesis that vascular and valvular calcification is a side-effect of VKA treatment.
Methods: We conducted a systematic literature search to identify studies that reported vascular or valvular calcification in patients treated with VKA. The associations between VKA use and calcification were analyzed with random-effects inverse variance models and reported as odds ratios (OR) and 95% confidence intervals (95% CI). In addition, univariate meta-regression analyses were utilized to identify any effect moderators.
Results: Thirty-five studies were included (45,757 patients; 6,251 VKA users). The median follow-up was 2.3 years [interquartile range (IQR) of 1.2–4.0]; age 66.2 ± 3.6 years (mean ± SD); the majority of participants were males [77% (IQR: 72–95%)]. VKA use was associated with an increased OR for coronary artery calcification [1.21 (1.08, 1.36), p = 0.001], moderated by the duration of treatment [meta-regression coefficient B of 0.08 (0.03, 0.13), p = 0.0005]. Extra-coronary calcification affecting the aorta, carotid artery, breast artery, and arteries of lower extremities, was also increased in VKA treated patients [1.86 (1.43, 2.42), p < 0.00001] and moderated by the author-reported statistical adjustments of the effect estimates [B: −0.63 (−1.19, −0.08), p = 0.016]. The effect of VKA on the aortic valve calcification was significant [3.07 (1.90, 4.96), p < 0.00001]; however, these studies suffered from a high risk of publication bias.
Conclusion: Vascular and valvular calcification are potential side effects of VKA. The clinical significance of these side effects on cardiovascular outcomes deserves further investigation.
Introduction
It is well-recognized that vascular calcification is an independent predictor of cardiovascular disease (CVD) and mortality (1). Studies have shown that calcification correlates with clinically-significant coronary artery disease (CAD) (2–4), acute cardiac and cerebrovascular events (5, 6), arterial stiffness and hypertension (7), and aortic valve disease (8). CVD is the leading cause of death, accounting for over 30% of mortality worldwide. Coronary artery calcium scoring has emerged as a non-invasive imaging platform for atherosclerotic CVD risk stratification and guiding lipid-lowering therapies for primary prevention (9, 10).
Warfarin, a vitamin K antagonist (VKA), was introduced into clinical practice as an anticoagulant in the 1950 s (11). Over the years, warfarin and other VKAs have been approved for the prophylaxis of thrombotic events in recurrent venous thrombosis, atrial fibrillation, valvular heart disease, and valve replacement (12). Although the use of VKA has declined in the past few years due to the introduction of safer non-vitamin K oral anticoagulants [NOAC or DOAC (direct oral anticoagulants)], VKAs remain widely prescribed and is the only guideline-recommended therapy for patients with prosthetic valves (13–16). Moreover, older patients and patients with comorbidities are more likely to receive warfarin for anticoagulation (17).
VKA inhibits coagulation factors II, VII, IX, X, and several other proteins by suppressing vitamin K-dependent post-translational gamma-carboxylation required for their function (18). Calcification is suppressed under normal physiologic conditions by several endogenous inhibitors, including matrix Gla protein (MGP), pyrophosphate, and plasma fetuin-A (19). MGP belongs to the same group of gamma-carboxylated proteins as coagulation factors and requires gamma-carboxylation for its inhibitory activity (18). Long-term use of VKA is associated with increased vascular calcification, presumably due to the reduction of vitamin K-dependent gamma-carboxylation of MGP (20, 21).
The role of VKA in vascular calcification is still poorly recognized, and the clinical significance is undefined (22). Here, we present the first meta-analysis of clinical studies on this topic. We aimed to provide objective evidence for the association between VKA use and cardiovascular calcification.
Methods
Search strategy
All clinical studies except case studies and case series were considered, and the inclusion was not limited to a specific indication for VKA use. Primary outcomes were coronary artery calcification, extra-coronary calcification (abdominal or thoracic aorta, carotid arteries, breast arteries, and arteries of the extremities), and valvular calcification. The core systematic literature search was conducted in PubMed with a stepwise keyword search strategy (Supplementary Table 1) up until March 29, 2022. The search results were filtered using pubmed filters to exclude reviews, case reports, guidelines, and study protocols. The reference lists of the relevant articles and “similar articles” suggested by pubmed were also considered. Other databases, including CINAHL, cochrane register of studies, and google scholar, were searched for additional references. Abstracts were screened for the inclusion criteria: (1) VKA treatment and (2) at least one vascular or valvular calcification outcome, e.g., calcium score or index, calcified plaque volume, presence/absence of calcification, calcification severity grade, or an annual rate of progression. Two investigators screened the abstracts, and any disagreements were resolved by finding a consensus.
Data extraction and management
Data were selected based on a full-text assessment. The extracted data included a study identifier, country, study design, sample size, mean or median age, percent of males, VKA treatment or exposure, duration of VKA therapy, calcification outcome(s), methods of assessment of calcification, effect size estimates, and a brief description of statistical models used for the effect estimates. The effect sizes were extracted as incidence, prevalence, odds ratios (OR), mean change from baseline, regression coefficients, ratios of expected counts (REC), and F statistics. The coronary outcomes were coronary artery calcium (CAC) score, measured via computed tomography (CT), calcified plaque volume determined by coronary CT angiography (CCTA), and CAC index obtained via intravascular ultrasound (IVUS). Extra-coronary outcomes were the presence or absence of calcification, severity grade, calcification score, or an annual rate of progression (detected by CT, X-ray, mammography, or histopathology). Lastly, the aortic valve calcification outcomes included the presence or absence of calcification on transthoracic ultrasound (US), the number of affected aortic valve leaflets, CT calcification score, or positive findings on histopathology.
Risk of bias assessment
The risks of bias were assessed using the Revised Cochrane risk of bias tool (RoB 2) for randomized trials (downloaded February 9, 2022, from https://sites.google.com/site/riskofbiastool/welcome/rob-2-0-tool/current-version-of-rob-2) (23) or the Newcastle—Ottawa quality scale (NOS) (24) for observations studies.
Statistical analysis
Data were analyzed using the Review Manager computer program, Version 5.4 (RevMan5, the Cochrane Collaboration, 2020). Effect sizes were expressed as OR and standard errors (SE) using the RevMan5 effect size calculator or an online effect size calculator tool [Practical Meta-Analysis Effect Size Calculator (25)]. Inverse-variance random-effects models were used for data synthesis. Studies were grouped according to the site of calcification, coronary, extra-coronary, and valvular. The combined estimates were calculated as OR and 95% confidence intervals (95% CI) for the presence of vascular or valvular calcification in VKA-treated patients compared with other patients (non-VKA), which included patients treated with non-VKA anticoagulants and those who had no indications for anticoagulation and were not treated with any anticoagulants. The statistical heterogeneity was evaluated using the I2 test calculated in RevMan5. The risk for publication bias was assessed by an Egger regression and Begg & Mazumdar rank correlation tests, using the Meta-Essentials tool (downloaded on February 9, 2022, from https://www.erim.eur.nl/research-support/meta-essentials) (26). The Meta-Essentials tool was also used for the univariate meta-regression analyses considering the year of publication, geographic region (continent), study design, sample size, patient characteristics, median age, a ratio of participants by sex, duration of VKA treatment, calcium imaging modality, and whether or not the effect estimates were adjusted for confounders. Sub-group analyses were conducted according to each significant modifier detected by meta-regression. Furthermore, the sensitivity analysis was performed by excluding one study at a time from the corresponding meta-analysis. The significance was accepted at p < 0.05.
Results
Search results and characteristics of the included studies
A total of 330 articles were identified via PubMed search, and five articles were retrieved from other sources. Of these 335 papers, 114 were reviews and editorials, 22 case studies, two study protocols, and two clinical guidelines. Another 152 were deemed irrelevant by consensus between two investigators (NDK and OVS) if articles did not pertain to human subjects, lacked VKA treatment or effect estimates, or had no standardized method of detecting and quantifying calcification. After a full-text review of the remaining 43 articles, an additional seven were excluded due to missing data (n = 1), not matching the inclusion criteria (n = 4), being a secondary analysis of an already included study (n = 2), or an ongoing study (n = 1, Figure 1). Thus, 35 studies and 45,757 participants were included in the analysis, and 6,251 were treated with VKA (27–61). Of these, three studies were randomized trials (4 independent analysis cohorts, 333 patients, 169 VKA users) (29, 32, 39), and 32 observational studies (38 cohorts; 45,424 participants; 6,082 VKA users) (27, 28, 30, 31, 33–38, 40–61). Thirteen studies investigated the effects of VKA on coronary artery calcification (15 cohorts, 23,768 participants, 2,625 received VKA) (27, 28, 30, 31, 33–38). Sixteen studies that reported extra-coronary (any artery but coronary) calcification included 18 cohorts, 4,740 participants, and 1,595 patients treated with VKA (40–54). Finally, nine studies investigated the effects of VKA treatment on aortic valve calcification (9 cohorts; 17,161 participants; 1,987 VKA-treated patients) (31, 49, 55–61).
Characteristics of the included studies are shown in Table 1. Studies were published between 2005 and 2022. Twenty studies were conducted in Europe (29–31, 34–36, 38, 41–43, 46–51, 53, 55, 57–59), thirteen in North America (27, 28, 32, 33, 37, 39, 40, 44, 45, 52, 56, 58, 60), and two in Asia (54, 61). As stated above, we identified three randomized trials (29, 32, 39), one meta-analysis of patient-level data from eight randomized trials (27), twenty one retrospective cohort studies (30, 31, 34–38, 40–42, 45, 48–52, 54, 55, 57, 59, 60), one prospective cohort (61), and nine cross-sectional studies (28, 33, 43, 44, 46, 47, 53, 56, 58). The sample size ranged from 37 to 17,254 participants. The median sample size was 207, interquartile range (IQR) of 108 to 387 patients. The age of participants was 66.2 ± 3.6 years (weighted mean ± SD); 77% (IQR 72–95%) of participants were males. The weighted median duration of VKA treatment in 33 groups of prospectively or retrospectively followed patients was 2.3 years (IQR 1.2–4.0) (27, 29–32, 34–42, 45, 48–52, 54, 55, 57, 59–61). Nine study cohorts were cross-sectional with an unspecified duration of treatment (28, 33, 43, 44, 46, 47, 53, 56, 58). The medical history of the patients included coronary artery disease (CAD) (27, 32, 39), chronic kidney disease CKD (including patients with ESRD) (29, 40–43, 46, 47, 53, 55), calcific aortic valve disease or aortic stenosis (CAVD/AS) (28, 56, 57, 60), atrial fibrillation (AF/NVAF) (28, 29, 32, 34, 38, 39, 49, 54, 55, 58, 61), metallic prosthetic valves (36), lower limb amputation (44), carotid atherectomy (48), non-traumatic cerebral hemorrhage (50) or underwent cardiac CT (33, 35) or mammography (40, 52) tests for diagnostic or screening purposes. Two studies included the general population from health registries (30, 59). For the remaining two studies, patients' medical history was not specified (37, 51).
Quality assessment
Among the three randomized trials, one “per-protocol” trial (29) had a high risk of bias due to missing outcome data, whereas two other “intention-to-treat” studies had concerns regarding missing outcome data (32) and selective reporting (32, 39) (Supplementary Table 2). Observational studies were assessed for the risk of bias on a 9-point Newcastle-Ottawa quality scale. The majority of studies were of at least moderate quality (27, 28, 30, 31, 34–38, 40–43, 45, 46, 48–59, 61) [median score 7 (IQR 5-9)], except five studies in which the risk of bias was considered low to moderate on the Newcastle-Ottawa quality scale (31, 33, 44, 47, 53) (Supplementary Table 3).
Effects of VKA use on the coronary, extra-coronary, and aortic valve calcification
VKA use was associated with increased vascular and valvular calcification. The OR for the coronary artery calcification in VKA-treated patients was 1.21 (95% CI 1.08, 1.36), p = 0.001 compared to patients not treated with VKA (Figure 2A). VKA use was also associated with extra-coronary vascular calcification in the aorta, carotid arteries, breast arteries, and arteries of lower extremities [OR 1.86 (1.43, 2.42), p < 0.00001, Figure 2B]. Furthermore, we found an association between VKA use and aortic valve calcification [OR 3.07 (1.90, 4.96), p < 0.00001, Figure 2C]. Between-study heterogeneity was significant at I2 of 69, 78, and 90% in the coronary (n = 15), extra-coronary vascular (n = 18), and aortic valve studies (n = 9), respectively.
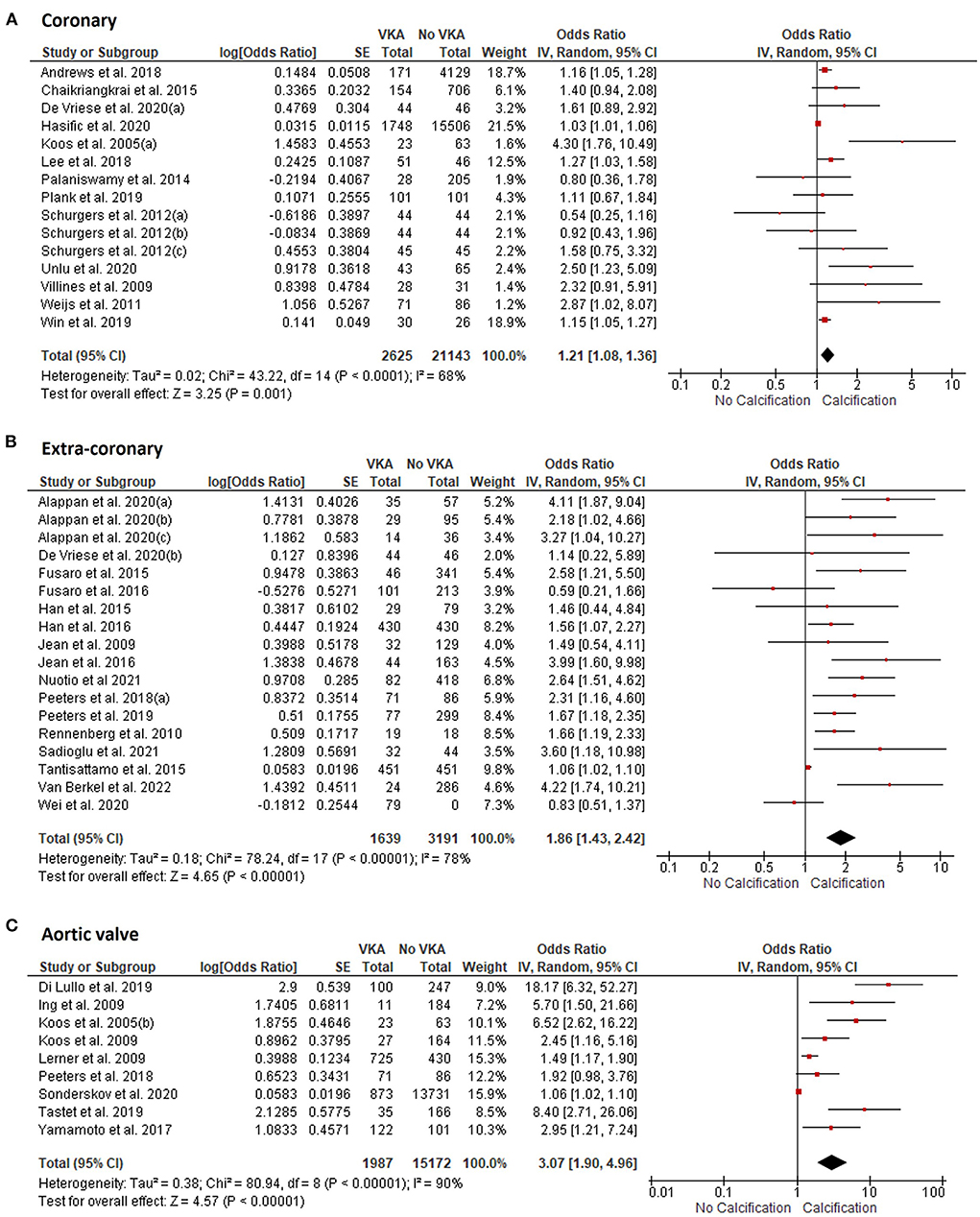
Figure 2. Meta-analysis of vascular and valvular calcification studies in VKA-treated patients. (A) coronary artery calcification; (B) extra-coronary calcification; (C) aortic valve calcification studies.
Publication bias
We constructed funnel plots of the effect sizes against their standard errors [log (OR), SE] and examined them using the Egger funnel plot asymmetry test and Begg & Mazumdar rank correlation test to evaluate the risks of publication bias. No significant risks of publication bias were found among the coronary artery calcification studies (Egger p = 0.097, Begg & Mazumdar p = 0.441) or the extra-coronary calcification studies (Egger p = 0.307, Begg & Mazumdar p = 0.172, Figures 3A,B). However, the risk of publication bias was significant in the studies of aortic valve calcification (Egger p = 0.0037, Begg & Mazumdar p = 0.0030, Figure 3C).
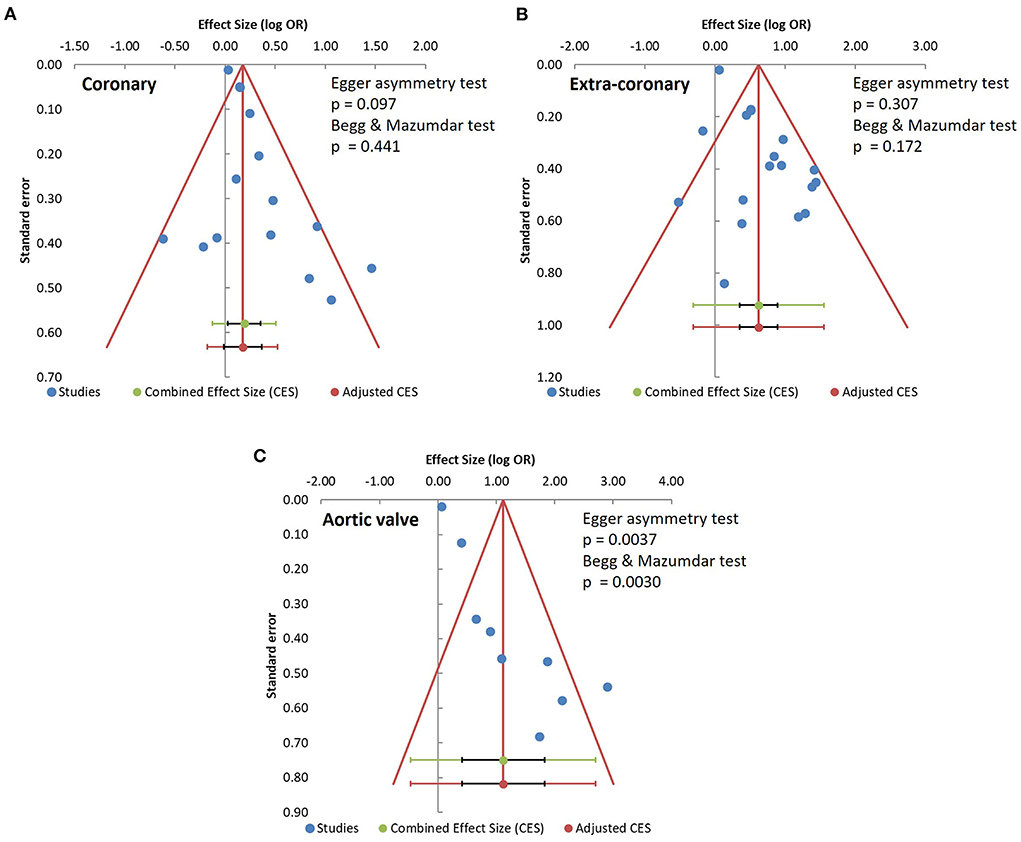
Figure 3. Analysis of publication bias. (A) coronary artery calcification; (B) extra-coronary calcification; (C) aortic valve calcification studies.
Meta-regression and subgroup analysis
We performed meta-regression analyses to identify potential effect modifiers. We calculated univariate random-effects regressions to assess the effects of the year of publication, geographic region (continent), study design, sample size, patient characteristics, median age, ratio of participants by sex, duration of VKA treatment, calcium imaging modality, and whether or not the effect estimates were reported adjusted for the confounders (Table 1). The estimates of coronary artery calcification were influenced by three explanatory variables, the year of publication [B regression coefficient of −0.04 (95% CI: −0.08, 0.00), p = 0.035]; the gender ratio expressed as a percent of male participants [B = −0.01 (−0.03, 0.00), p = 0.039]; and the duration of VKA treatment [B = 0.08 (0.03, 0.13), p = 0.0005, Table 2; Figure 4A]. The effects on the extra-coronary vascular calcification were modified by whether or not the reported estimates were adjusted or not adjusted for the confounders [B = −0.63 (−1.19, −0.08), p = 0.016, Table 2; Figure 5A]. Although the number of the aortic valve studies was low (n = 9) and suffered from a significant risk of publication bias, we performed a meta-regression analysis and found that the effect estimates were potentially modified by the sample size [B = −0.32 (−2.35, −0.04), p = 0.009, Table 2].
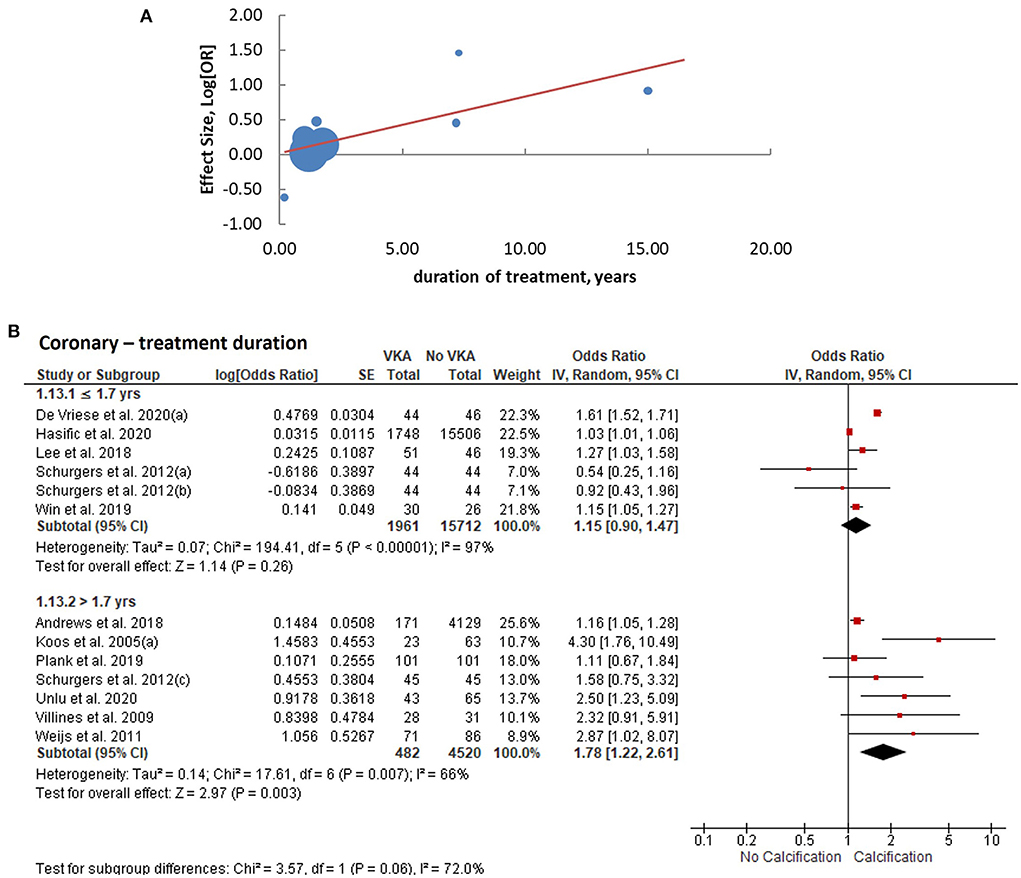
Figure 4. Analysis of coronary arterial calcification according to the duration of VKA treatment. (A) meta-regression of the effect sizes—less or equal vs. more than the median duration; (B) subgroup analysis based on the duration of treatment.
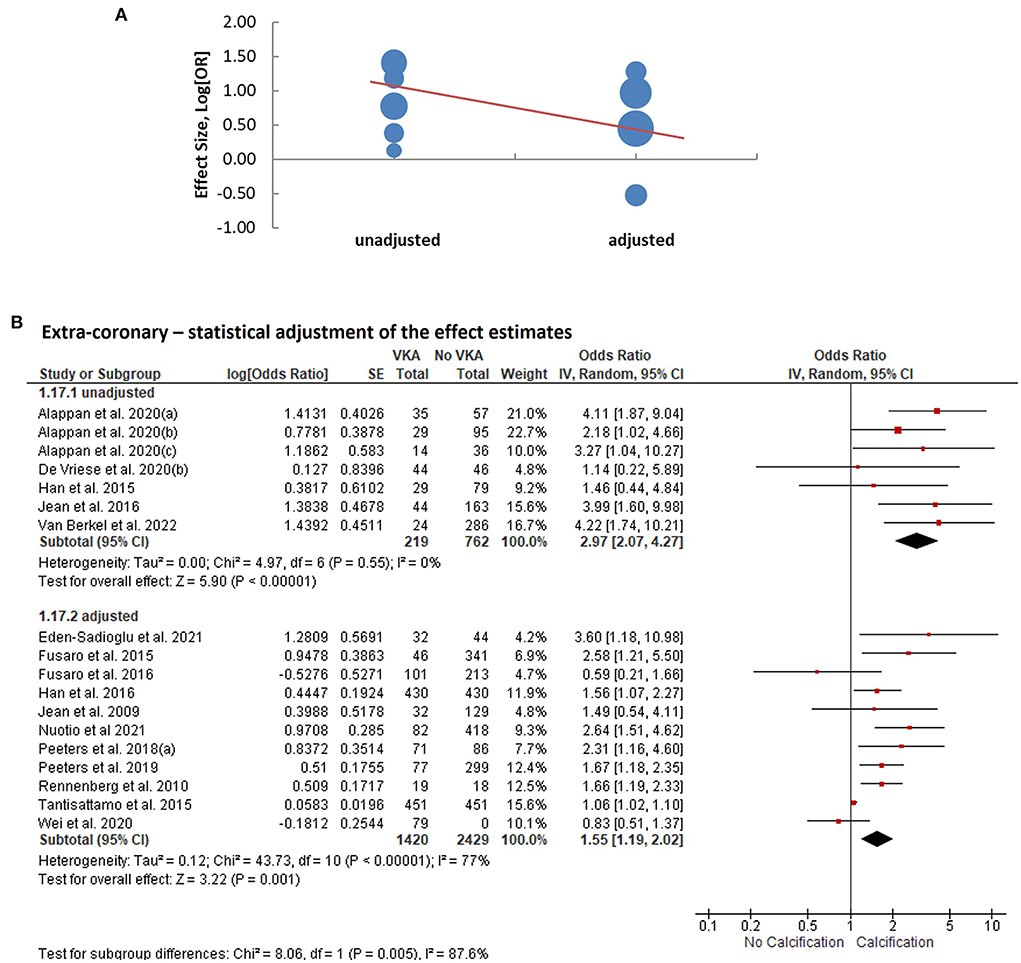
Figure 5. Analysis of extra-coronary arterial calcification according to statistical adjustment for confounding variables. (A) Meta-regression of the effect sizes—adjusted vs. unadjusted. (B) Subgroup analysis based on whether or not statistical adjustments were applied to calculate the effect estimates.
We consequently performed subgroup analyses comparing the top and bottom half of the studies with respect to each of the identified modifiers (publication year, sex ratio, duration of VKA treatment, and statistical adjustment). We found that the effect of VKA duration on the coronary artery calcification was borderline significant when comparing studies of a longer duration (>1.7 years) and studies of ≤ 1.7 years duration (groups difference test I2 = 72%, p = 0.06; Table 3; Figure 4B). We also found that, as predicted by meta-regression, the estimates of VKA effects of the extra-coronary calcification were modified by whether or not the reported models were adjusted for plausible confounders (I2 = 79%, p = 0.005, Table 3; Figure 5B).
Sensitivity analysis
To explore the influence of any single study on the overall effect sizes, we excluded studies from the corresponding analyses, one at a time. No significant effects of any individual study on the effect sizes of VKA were observed (Supplementary Table 4).
Discussion
We present the first meta-analysis of the effects of vitamin K antagonists on vascular and valvular calcification. Our results confirmed a strong association between VKA use and vascular calcification in the absence of significant risks of publication bias. We also found evidence of a positive association between VKA use and aortic valve calcification; however, due to a smaller number of studies and evidence of publication bias, the confidence in this finding is low.
A large number of good-quality observational studies of the effects of VKA on vascular calcification have been published. These studies assessed calcification in thousands of VKA-treated patients. Improved imaging modalities and the use of calcium imaging in diagnostics studies allowed for the analysis of larger cohorts of patients with greater precision. Several authors took a propensity matching approach to eliminate the potential confounders; others used other comprehensive statistical methods to minimize the effects of confounding variables on calcification estimates. The quality of publications on VKA use and vascular calcification is also supported by a lack of significant risk of publication bias and the fact that we could detect that duration of treatment as a modifier of the effect estimates. Lastly, a recent introduction of a new class of non-vitamin K oral anticoagulants, NOAC, allowed for the analysis of calcification in the first head-to-head randomized trials with VKA, although each of the three randomized trials assessed <100 patients so far.
Of 45,000 participants included in this meta-analysis, 99.5% were from observational studies. Therefore, it is important to recognize limitations inherent to observational study designs, such as the difficulty of establishing a cause-and-effect relationship. We also observed that the magnitude of effect estimates was modified by several experimental parameters, including sample size, gender ratio, and adjustments for confounding variables. In addition, we found that studies of VKA in valvular calcification suffered from a significant risk of publication bias, limiting our confidence in that association.
For decades, warfarin, a commonly used VKA, has been a standard and effective treatment option for patients requiring anticoagulation. Early studies in the 1980 s found an association between dystrophic calcification and warfarin in an animal model examining bioprosthetic aortic valves (64). Since the early 1990 s, it has been known that warfarin is associated with soft tissue calcification, such as skin calcinosis and tracheobronchial calcification (65, 66). In 2005, Koos et al. documented for the first time the effects of warfarin on the coronary artery and aortic valve calcification (31).
Cardiovascular calcification presents several morphologically distinct forms, including the intimal, medial, and heart valve calcification. Coronary arteries are primarily affected by atherosclerotic intimal calcification, whereas the peripheral arteries and aorta show different degrees of medial and intimal involvement. Despite the anatomical difference, many significant correlations exist between calcification burdens at different vascular sites, suggesting a common mechanism and the influence of systemic factors. Thus, calcification in the abdominal aorta, breast and the arteries of the extremities artery correlates with coronary artery calcification (67–69). An independent association between aortic valve calcification and the severity of coronary artery calcification has also been reported (70).
Calcification is initiated by osteogenic transdifferentiation of vascular cells (71). Transdifferentiated cells secrete mineralizing matrix vesicles that serve as nucleating sites for extracellular calcium deposition (72–74). Osteogenic transdifferentiation is preceded by inflammation, as has been shown in a longitudinal study of patients' coronary artery calcification employing positron emission tomography (PET) combined with CT (75). Active inflammation and microcalcification detected by specific PET were also shown to co-exist in patients with peripheral arterial or calcific aortic valve disease (76, 77). MGP interferes with both the osteogenic cell transformation and physicochemical process of biomineralization (78). VKA reduces gamma-carboxylation leaving MGP in the inactive state.
Calcification can negatively affect the clinical course of cardiovascular disease in several ways, by increasing arterial stiffness, stability of atherosclerosis plaques, and, in the context of calcific aortic valve stenosis, reducing the opening of the valves. Arterial stiffness promotes microcirculatory damage by increasing the transmission of pressure pulsatility (79). Numerous studies have shown that arterial stiffness predicts cardiovascular outcomes after adjustments for conventional risk factors (80–82). Calcification also changes the composition of atherosclerotic plaque. An earlier study of culprit plaques' characteristics documented that surface erosion over a calcified nodule has likely precipitated an acute ischemic coronary event and death (83). The later studies using 18F-sodium fluoride positron emission tomography (18F-NaF PET), capable of detecting micro-calcification invisible to other imaging technologies, demonstrated that micro-calcification is associated with high-risk plaque features (84). Furthermore, aortic valve calcification and the rate of progression of calcification are strong predictors of aortic valve stenosis outcomes (85, 86). Thus, the association between VKA use and cardiovascular calcification is concerning because it might worsen the course of vascular or valvular disease.
It was suggested that warfarin-induced calcification could result in adverse clinical outcomes (87). One study included in this meta-analysis demonstrated that warfarin had a significant hazard ratio of 1.97 for the overall mortality in hemodialysis patients independent of the confounder variables, age, atrial fibrillation, and diabetes. Furthermore, adjustment for vascular calcification reduced the strength of this association, suggesting that warfarin-induced calcification might have contributed to mortality (42).
In conclusion, our meta-analysis demonstrates that VKA use is associated with vascular calcification. Thus, vascular calcification can be considered a side effect of VKA. However, the clinical significance of VKA-induced calcification and the risk benefits of VKA therapy requires further evaluation.
Data availability statement
The original contributions presented in the study are included in the article/Supplementary material, further inquiries can be directed to the corresponding author/s.
Author contributions
NDK and OVS conceived, designed the review, analyzed, interpreted the results, and edited the manuscript. NDK and MS performed the literature search and screened the data. NDK and DK extracted the data. OVS verified the extracted data. NDK wrote the first draft. All authors contributed to the article and approved the submitted version.
Funding
This work was supported by the National Heart, Lung, and Blood Institute of the National Institutes of Health under award number R01HL149864 (OVS) and the NYITCOM Academic Medicine Scholar Program (NDK).
Acknowledgments
We thank the authors who have responded to our requests for information.
Conflict of interest
The authors declare that the research was conducted in the absence of any commercial or financial relationships that could be construed as a potential conflict of interest.
Publisher's note
All claims expressed in this article are solely those of the authors and do not necessarily represent those of their affiliated organizations, or those of the publisher, the editors and the reviewers. Any product that may be evaluated in this article, or claim that may be made by its manufacturer, is not guaranteed or endorsed by the publisher.
Supplementary Material
The Supplementary Material for this article can be found online at: https://www.frontiersin.org/articles/10.3389/fcvm.2022.938567/full#supplementary-material
References
1. Shekar C, Budoff M. Calcification of the heart: mechanisms and therapeutic avenues. Exp Rev Cardiovasc Therapy. (2018) 16:527–36. doi: 10.1080/14779072.2018.1484282
2. Agatston AS, Janowitz WR, Hildner FJ, Zusmer NR, Viamonte M Jr, Detrano R. Quantification of coronary artery calcium using ultrafast computed tomography. J Am Coll Cardiol. (1990) 15:827–32. doi: 10.1016/0735-1097(90)90282-T
3. Breen JF, Sheedy PF 2nd, Schwartz RS, Stanson AW, Kaufmann RB, Moll PP, et al. Coronary artery calcification detected with ultrafast CT as an indication of coronary artery disease. Radiology. (1992) 185:435–9. doi: 10.1148/radiology.185.2.1410350
4. Margolis JR, Chen JT, Kong Y, Peter RH, Behar VS, Kisslo JA. The diagnostic and prognostic significance of coronary artery calcification. A report of 800 cases. Radiology. (1980) 137:609–16. doi: 10.1148/radiology.137.3.7444045
5. Sugiyama T, Yamamoto E, Fracassi F, Lee H, Yonetsu T, Kakuta T, et al. Calcified plaques in patients with acute coronary syndromes. JACC Cardiovasc Interv. (2019) 12:531–40. doi: 10.1016/j.jcin.2018.12.013
6. Iwai S, Watanabe M, Okamura A, Kyodo A, Nogi K, Kamon D, et al. Prognostic impact of calcified plaque morphology after drug eluting stent implantation- an optical coherence tomography study. Circ J. (2021). 85:2019–28. doi: 10.1253/circj.CJ-20-1233
7. Aladin AI, Al Rifai M, Rasool SH, Dardari Z, Yeboah J, Nasir K, et al. Relation of coronary artery calcium and extra-coronary aortic calcium to incident hypertension (from the multi-ethnic study of atherosclerosis). Am J Cardiol. (2018) 121:210–6. doi: 10.1016/j.amjcard.2017.10.018
8. Pawade TA, Newby DE, Dweck MR. Calcification in aortic stenosis: the skeleton key. J Am Coll Cardiol. (2015) 66:561–77. doi: 10.1016/j.jacc.2015.05.066
9. Golub I, Lakshmanan S, Dahal S, Budoff MJ. Utilizing coronary artery calcium to guide statin use. Atherosclerosis. (2021) 326:17–24. doi: 10.1016/j.atherosclerosis.2021.04.011
10. Grundy SM, Stone NJ, Bailey AL, Beam C, Birtcher KK, Blumenthal RS, et al. 2018 AHA/ACC/AACVPR/AAPA/ABC/ACPM/ADA/AGS/APhA/ASPC/NLA/PCNA guideline on the management of blood cholesterol: a report of the American college of cardiology/American heart association task force on clinical practice guidelines. Circulation. (2019) 139:e1082–43. doi: 10.1161/CIR.0000000000000624
11. Nicholson JH. Clinical experiences with anticoagulants; a comparison of coumadin (warfarin) sodium and dicumarol (bishydroxycoumarin). Angiology. (1957) 8:456–65. doi: 10.1177/000331975700800508
12. Horton JD, Bushwick BM. Warfarin therapy: evolving strategies in anticoagulation. Am Fam Physician. (1999) 59:635–46.
13. Ortel TL, Neumann I, Ageno W, Beyth R, Clark NP, Cuker A, et al. American society of hematology 2020 guidelines for management of venous thromboembolism: treatment of deep vein thrombosis and pulmonary embolism. Blood Advances. (2020) 4:4693–738. doi: 10.1182/bloodadvances.2020001830
14. January CT, Wann LS, Calkins H, Chen LY, Cigarroa JE, Cleveland JC, et al. 2019 AHA/ACC/HRS focused update of the 2014 AHA/ACC/HRS guideline for the management of patients with atrial fibrillation: a report of the American college of cardiology/American heart association task force on clinical practice guidelines and the heart rhythm society in collaboration with the society of thoracic surgeons. Circulation. (2019) 140:e125–51. doi: 10.1161/CIR.0000000000000665
15. Wetmore JB, Roetker NS, Yan H, Reyes JL, Herzog CA. Direct-acting oral anticoagulants versus warfarin in medicare patients with chronic kidney disease and atrial fibrillation. Stroke. (2020) 51:2364–73. doi: 10.1161/STROKEAHA.120.028934
16. Otto CM, Nishimura RA, Bonow RO, Carabello BA, Erwin JP, Gentile F, et al. 2020 ACC/AHA guideline for the management of patients with valvular heart disease: a report of the American college of cardiology/American heart association joint committee on clinical practice guidelines. Circulation. (2021) 143:e72–227. doi: 10.1161/CIR.0000000000000923
17. Mills C, Snider MJ, Ortman TC, Dush A, Hevezi MS, Li J, et al. Trends in anticoagulation management services following incorporation of direct oral anticoagulants at a large academic medical center. J Thromb Thrombol. (2021) 51:1050–8. doi: 10.1007/s11239-020-02286-2
18. Willems BA, Vermeer C, Reutelingsperger CP, Schurgers LJ. The realm of vitamin K dependent proteins: shifting from coagulation toward calcification. Mol Nutr Food Res. (2014) 58:1620–35. doi: 10.1002/mnfr.201300743
19. Bäck M, Aranyi T, Cancela ML, Carracedo M, Conceição N, Leftheriotis G, et al. Endogenous calcification inhibitors in the prevention of vascular calcification: a consensus statement from the COST action EuroSoftCalcNet. Front Cardiovasc Med. (2018) 5:196. doi: 10.3389/fcvm.2018.00196
20. Kaesler N, Schurgers LJ, Floege J. Vitamin K and cardiovascular complications in chronic kidney disease patients. Kidney Int. (2021). 100:1023–36. doi: 10.1016/j.kint.2021.06.037
21. Schurgers LJ, Teunissen KJ, Knapen MH, Kwaijtaal M, van Diest R, Appels A, et al. Novel conformation-specific antibodies against matrix gamma-carboxyglutamic acid (Gla) protein: undercarboxylated matrix Gla protein as marker for vascular calcification. Arterioscl Thromb Vasc Biol. (2005) 25:1629–33. doi: 10.1161/01.ATV.0000173313.46222.43
22. van Gorp RH, Schurgers LJ. New insights into the pros and cons of the clinical use of vitamin K antagonists (VKAs) versus direct oral anticoagulants (DOACs). Nutrients. (2015) 7:9538–57. doi: 10.3390/nu7115479
23. Sterne JAC, Savović J, Page MJ, Elbers RG, Blencowe NS, Boutron I, et al. RoB 2: a revised tool for assessing risk of bias in randomised trials. BMJ. (2019) 366:l4898. doi: 10.1136/bmj.l4898
24. Wells G, SB O'Connell D, Peterson J, Welch V, Losos M, Tugwell P. The Newcastle-Ottawa Scale (NOS) for Assessing the Quality of Nonrandomised Studies in Meta-Analyses. (2013). Available online at: https://www.ohri.ca//programs/clinical_epidemiology/oxford.asp (accessed March 29, 2022).
25. Wilson D. Practical Meta-Analysis Effect Size Calculator [Online calculator] Available online at: https://campbellcollaboration.org/research-resources/effect-size-calculator.html (accessed March 29, 2022).
26. Suurmond R, van Rhee H, Hak T. Introduction, comparison, and validation of meta-essentials: a free and simple tool for meta-analysis. Res Synthesis Meth. (2017) 8:537–53. doi: 10.1002/jrsm.1260
27. Andrews J, Psaltis PJ, Bayturan O, Shao M, Stegman B, Elshazly M, et al. Warfarin use is associated with progressive coronary arterial calcification: insights from serial intravascular ultrasound. JACC Cardiovasc Imag. (2018) 11:1315–23. doi: 10.1016/j.jcmg.2017.04.010
28. Chaikriangkrai K, Valderrabano M, Bala SK, Alchalabi S, Graviss EA, Nabi F, et al. Prevalence and implications of subclinical coronary artery disease in patients with atrial fibrillation. Am J Cardiol. (2015) 116:1219–23. doi: 10.1016/j.amjcard.2015.07.041
29. De Vriese AS, Caluwe R, Pyfferoen L, De Bacquer D, De Boeck K, Delanote J, et al. Multicenter randomized controlled trial of vitamin k antagonist replacement by rivaroxaban with or without vitamin K2 in hemodialysis patients with atrial fibrillation: the valkyrie study. J Am Soc Nephrol. (2020) 31:186–96. doi: 10.1681/ASN.2019060579
30. Hasific S, Øvrehus KA, Gerke O, Hallas J, Busk M, Lambrechtsen J, et al. Extent of arterial calcification by conventional vitamin K antagonist treatment. PLoS ONE. (2020) 15:e0241450. doi: 10.1371/journal.pone.0241450
31. Koos R, Mahnken AH, Mühlenbruch G, Brandenburg V, Pflueger B, Wildberger JE, et al. Relation of oral anticoagulation to cardiac valvular and coronary calcium assessed by multislice spiral computed tomography. Am J Cardiol. (2005) 96:747–9. doi: 10.1016/j.amjcard.2005.05.014
32. Lee J, Nakanishi R, Li D, Shaikh K, Shekar C, Osawa K, et al. Randomized trial of rivaroxaban versus warfarin in the evaluation of progression of coronary atherosclerosis. Am Heart J. (2018) 206:127–30. doi: 10.1016/j.ahj.2018.08.007
33. Palaniswamy C, Aronow WS, Sekhri A, Adapa S, Ahn C, Singh T, et al. Warfarin use and prevalence of coronary artery calcification assessed by multislice computed tomography. Am J Ther. (2014) 21:148–51. doi: 10.1097/MJT.0b013e318249a1c6
34. Plank F, Beyer C, Friedrich G, Stühlinger M, Hintringer F, Dichtl W, et al. Influence of vitamin K antagonists and direct oral anticoagulation on coronary artery disease: a CTA analysis. Int J Cardiol. (2018) 260:11–5. doi: 10.1016/j.ijcard.2018.03.019
35. Schurgers LJ, Joosen IA, Laufer EM, Chatrou ML, Herfs M, Winkens MH, et al. Vitamin K-antagonists accelerate atherosclerotic calcification and induce a vulnerable plaque phenotype. PLoS ONE. (2012) 7:e43229. doi: 10.1371/journal.pone.0043229
36. Ünlü S, Sahinarslan A, Kiliç HK, Gökalp G, Sezenöz B, Erbaş G, et al. Long-term vitamin-K antagonist use and coronary artery calcification. Herz. (2020) 45:580–5. doi: 10.1007/s00059-018-4760-9
37. Villines TC, O'Malley PG, Feuerstein IM, Thomas S, Taylor AJ. Does prolonged warfarin exposure potentiate coronary calcification in humans? Results of the warfarin and coronary calcification study. Calcif Tissue Int. (2009) 85:494–500. doi: 10.1007/s00223-009-9300-4
38. Weijs B, Blaauw Y, Rennenberg RJ, Schurgers LJ, Timmermans CC, Pison L, et al. Patients using vitamin K antagonists show increased levels of coronary calcification: an observational study in low-risk atrial fibrillation patients. Eur Heart J. (2011) 32:2555–62. doi: 10.1093/eurheartj/ehr226
39. Win TT, Nakanishi R, Osawa K, Li D, Susaria SS, Jayawardena E, et al. Apixaban versus warfarin in evaluation of progression of atherosclerotic and calcified plaques (prospective randomized trial). Am Heart J. (2019) 212:129–33. doi: 10.1016/j.ahj.2019.02.014
40. Alappan HR, Kaur G, Manzoor S, Navarrete J, O'Neill WC. Warfarin accelerates medial arterial calcification in humans. Arterioscl Thromb Vasc Biol. (2020) 40:1413–9. doi: 10.1161/ATVBAHA.119.313879
41. Eren Sadioglu R, Ustuner E, Ergun I, Ecder ST, Nergizoglu G, Keven K. Warfarin is associated with the risk of vascular calcification in abdominal aorta in hemodialysis patients: a multicenter case-control study. Turk J Med Sci. (2021). 51:2607–15. doi: 10.3906/sag-2104-221
42. Fusaro M, Tripepi G, Noale M, Plebani M, Zaninotto M, Piccoli A, et al. Prevalence of vertebral fractures, vascular calcifications, and mortality in warfarin treated hemodialysis patients. Curr Vasc Pharmacol. (2015) 13:248–58. doi: 10.2174/15701611113119990146
43. Fusaro M, Gallieni M, Rebora P, Rizzo MA, Luise MC, Riva H, et al. Atrial fibrillation and low vitamin D levels are associated with severe vascular calcifications in hemodialysis patients. J Nephrol. (2016) 29:419–26. doi: 10.1007/s40620-015-0236-7
44. Han KH, Hennigar RA, O'Neill WC. The association of bone and osteoclasts with vascular calcification. Vasc Med. (2015) 20:527–33. doi: 10.1177/1358863X15597076
45. Han KH, O'Neill WC. Increased peripheral arterial calcification in patients receiving warfarin. J Am Heart Assoc. (2016) 5:e002665. doi: 10.1161/JAHA.115.002665
46. Jean G, Bresson E, Terrat JC, Vanel T, Hurot JM, Lorriaux C, et al. Peripheral vascular calcification in long-haemodialysis patients: associated factors and survival consequences. Nephrol Dial Transplant. (2009) 24:948–55. doi: 10.1093/ndt/gfn571
47. Jean G, Chazot C, Bresson E, Zaoui E, Cavalier E. High serum sclerostin levels are associated with a better outcome in haemodialysis patients. Nephron. (2016) 132:181–90. doi: 10.1159/000443845
48. Nuotio K, Koskinen SM, Mäkitie L, Tuimala J, Ijäs P, Heikkilä HM, et al. Warfarin treatment is associated to increased internal carotid artery calcification. Front Neurol. (2021) 12:696244. doi: 10.3389/fneur.2021.696244
49. Peeters F, Dudink E, Kimenai DM, Weijs B, Altintas S, Heckman LIB, et al. Vitamin K antagonists, non-vitamin K antagonist oral anticoagulants, and vascular calcification in patients with atrial fibrillation. TH Open. (2018) 2:e391–e8. doi: 10.1055/s-0038-1675578
50. Peeters MTJ, Houben R, Postma AA, van Oostenbrugge RJ, Schurgers LJ, Staals J. Vitamin K antagonist use and risk for intracranial carotid artery calcification in patients with intracerebral hemorrhage. Front Neurol. (2019) 10:1278. doi: 10.3389/fneur.2019.01278
51. Rennenberg RJ, van Varik BJ, Schurgers LJ, Hamulyak K, Ten Cate H, Leiner T, et al. Chronic coumarin treatment is associated with increased extracoronary arterial calcification in humans. Blood. (2010) 115:5121–3. doi: 10.1182/blood-2010-01-264598
52. Tantisattamo E, Han KH, O'Neill WC. Increased vascular calcification in patients receiving warfarin. Arterioscl Thromb Vasc Biol. (2015) 35:237–42. doi: 10.1161/ATVBAHA.114.304392
53. Van Berkel B, Van Ongeval C, Van Craenenbroeck AH, Pottel H, De Vusser K, Evenepoel P. Prevalence, progression and implications of breast artery calcification in patients with chronic kidney disease. Clin Kidney J. (2022) 15:295–302. doi: 10.1093/ckj/sfab178
54. Wei N, Lu L, Zhang H, Gao M, Ghosh S, Liu Z, et al. Warfarin accelerates aortic calcification by upregulating senescence-associated secretory phenotype maker expression. Oxid Med Cell Longev. (2020) 2020:2043762. doi: 10.1155/2020/2043762
55. Di Lullo L, Tripepi G, Ronco C, D'Arrigo G, Barbera V, Russo D, et al. Cardiac valve calcification and use of anticoagulants: preliminary observation of a potentially modifiable risk factor. Int J Cardiol. (2019) 278:243–9. doi: 10.1016/j.ijcard.2018.11.119
56. Ing SW, Mohler Iii ER, Putt ME, Torigian D, Leonard MB. Correlates of valvular ossification in patients with aortic valve stenosis. Clin Transl Sci. (2009) 2:431–5. doi: 10.1111/j.1752-8062.2009.00168.x
57. Koos R, Krueger T, Westenfeld R, Kühl HP, Brandenburg V, Mahnken AH, et al. Relation of circulating matrix gla-protein and anticoagulation status in patients with aortic valve calcification. Thromb Haemost. (2009) 101:706–13. doi: 10.1160/TH08-09-0611
58. Lerner RG, Aronow WS, Sekhri A, Palaniswamy C, Ahn C, Singh T, et al. Warfarin use and the risk of valvular calcification. J Thromb Haemost. (2009) 7:2023–7. doi: 10.1111/j.1538-7836.2009.03630.x
59. Sonderskov PS, Lindholt JS, Hallas J, Gerke O, Hasific S, Lambrechtsen J, et al. Association of aortic valve calcification and vitamin K antagonist treatment. Eur Heart J Cardiovasc Imag. (2020) 21:718–24. doi: 10.1093/ehjci/jeaa065
60. Tastet L, Pibarot P, Shen M, Clisson M, Côté N, Salaun E, et al. Oral anticoagulation therapy and progression of calcific aortic valve stenosis. J Am Coll Cardiol. (2019) 73:1869–71. doi: 10.1016/j.jacc.2019.01.043
61. Yamamoto K, Koretsune Y, Akasaka T, Kisanuki A, Ohte N, Takenaka T, et al. Effects of vitamin K antagonist on aortic valve degeneration in non-valvular atrial fibrillation patients: prospective 4-year observational study. Thromb Res. (2017) 160:69–75. doi: 10.1016/j.thromres.2017.10.027
62. Kauppila L, Polak J, Cupples L, Hannan M, Kiel D, Wilson P. New indices to classify location, severity and progression of calcific lesions in the abdominal aorta: a 25- year follow-up study. Atherosclerosis. (1997) 132:245–50. doi: 10.1016/S0021-9150(97)00106-8
63. Witteman JC, Grobbee DE, Valkenburg HA, van Hemert AM, Stijnen T, Burger H, et al. J-shaped relation between change in diastolic blood pressure and progression of aortic atherosclerosis. Lancet. (1994) 343:504–7. doi: 10.1016/S0140-6736(94)91459-1
64. Levy RJ, Schoen FJ, Levy JT, Nelson AC, Howard SL, Oshry LJ. Biologic determinants of dystrophic calcification and osteocalcin deposition in glutaraldehyde-preserved porcine aortic valve leaflets implanted subcutaneously in rats. Am J Pathol. (1983) 113:143–55.
65. Taybi H, Capitanio MA. Tracheobronchial calcification: an observation in three children after mitral valve replacement and warfarin sodium therapy. Radiology. (1990) 176:728–30. doi: 10.1148/radiology.176.3.2389031
66. Yoshida S, Torikai K. The effects of warfarin on calcinosis in a patient with systemic sclerosis. J Rheumatol. (1993) 20:1233–5.
67. Jurgens PT, Carr JJ, Terry JG, Rana JS, Jacobs DR, Duprez DA. Association of abdominal aorta calcium and coronary artery calcium with incident cardiovascular and coronary heart disease events in black and white middle‐ aged people: the coronary artery risk development in young adults study. J Am Heart Assoc. (2021) 10:e023037. doi: 10.1161/JAHA.121.023037
68. Takayama Y, Yasuda Y, Suzuki S, Shibata Y, Tatami Y, Shibata K, et al. Relationship between abdominal aortic and coronary artery calcification as detected by computed tomography in chronic kidney disease patients. Heart Vessels. (2016) 31:1030–7. doi: 10.1007/s00380-015-0712-y
69. Minssen L, Dao TH, Quang AV, Martin L, Andureau E, Luciani A, et al. Breast arterial calcifications on mammography: a new marker of cardiovascular risk in asymptomatic middle age women? Eur Radiol. (2022) 32:4889–97. doi: 10.1007/s00330-022-08571-3
70. Nasir K, Katz R, Al-Mallah M, Takasu J, Shavelle DM, Carr JJ, et al. Relationship of aortic valve calcification with coronary artery calcium severity: the multi-ethnic study of atherosclerosis (MESA). J Cardiovasc Comp Tomog. (2010) 4:41–6. doi: 10.1016/j.jcct.2009.12.002
71. Tintut Y, Honda HM, Demer LL. Biomolecules orchestrating cardiovascular calcification. Biomolecules. (2021) 11:1482. doi: 10.3390/biom11101482
72. Aikawa E, Blaser MC, Jeffrey M. Hoeg award lecture: calcifying extracellular vesicles as building blocks of microcalcifications in cardiovascular disorders. Arterioscl Thromb Vasc Biol. (2021) 41:117–27. doi: 10.1161/ATVBAHA.120.314704
73. Kim KM. Calcification of matrix vesicles in human aortic valve and aortic media. Fed Proc. (1976) 35:156–62.
74. Bertazzo S, Gentleman E, Cloyd KL, Chester AH, Yacoub MH, Stevens MM. Nano-analytical electron microscopy reveals fundamental insights into human cardiovascular tissue calcification. Nat Mater. (2013) 12:576–83. doi: 10.1038/nmat3627
75. Abdelbaky A, Corsini E, Figueroa AL, Fontanez S, Subramanian S, Ferencik M, et al. Focal arterial inflammation precedes subsequent calcification in the same location: a longitudinal FDG-PET/CT study. Circ Cardiovasc Imag. (2013) 6:747–54. doi: 10.1161/CIRCIMAGING.113.000382
76. Chowdhury MM, Tarkin JM, Albaghdadi MS, Evans NR, Le EPV, Berrett TB, et al. Vascular positron emission tomography and restenosis in symptomatic peripheral arterial disease: a prospective clinical study. JACC Cardiovasc Imag. (2020) 13:1008–17. doi: 10.1016/j.jcmg.2019.03.031
77. Dweck MR, Jones C, Joshi NV, Fletcher AM, Richardson H, White A, et al. Assessment of valvular calcification and inflammation by positron emission tomography in patients with aortic stenosis. Circulation. (2012) 125:76–86. doi: 10.1161/CIRCULATIONAHA.111.051052
78. Bjørklund G, Svanberg E, Dadar M, Card DJ, Chirumbolo S, Harrington DJ, et al. The role of matrix gla protein (MGP) in vascular calcification. Curr Med Chem. (2020) 27:1647–60. doi: 10.2174/0929867325666180716104159
79. Vasan RS, Short MI, Niiranen TJ, Xanthakis V, DeCarli C, Cheng S, et al. Interrelations between arterial stiffness, target organ damage, and cardiovascular disease outcomes. J Am Heart Assoc. (2019) 8:e012141. doi: 10.1161/JAHA.119.012141
80. Lee GP, Kim HL. Incremental value of the measures of arterial stiffness in cardiovascular risk assessment. Rev Cardiovasc Med. (2022) 23:6. doi: 10.31083/j.rcm2301006
81. Vasan RS, Pan S, Xanthakis V, Beiser A, Larson MG, Seshadri S, et al. Arterial stiffness and long-term risk of health outcomes: the framingham heart study. Hypertension. (2022) 79:1045–56. doi: 10.1161/HYPERTENSIONAHA.121.18776
82. Maroules CD, Khera A, Ayers C, Goel A, Peshock RM, Abbara S, et al. Cardiovascular outcome associations among cardiovascular magnetic resonance measures of arterial stiffness: the dallas heart study. J Cardiovasc Magn Reson. (2014) 16:33. doi: 10.1186/1532-429X-16-33
83. Virmani R, Kolodgie FD, Burke AP, Farb A, Schwartz SM. Lessons from sudden coronary death: a comprehensive morphological classification scheme for atherosclerotic lesions. Arterioscl Thromb Vasc Biol. (2000) 20:1262–75. doi: 10.1161/01.ATV.20.5.1262
84. Høilund-Carlsen PF, Sturek M, Alavi A, Gerke O. Atherosclerosis imaging with (18)F-sodium fluoride PET: state-of-the-art review. Eur J Nucl Med Mol Imag. (2020) 47:1538–51. doi: 10.1007/s00259-019-04603-1
85. Nishimura S, Izumi C, Nishiga M, Amano M, Imamura S, Onishi N, et al. Predictors of rapid progression and clinical outcome of asymptomatic severe aortic stenosis. Circ J. (2016) 80:1863–9. doi: 10.1253/circj.CJ-16-0333
86. Rosenhek R, Binder T, Porenta G, Lang I, Christ G, Schemper M, et al. Predictors of outcome in severe, asymptomatic aortic stenosis. N Engl J Med. (2000) 343:611–7. doi: 10.1056/NEJM200008313430903
Keywords: cardiovascular calcifications, atherosclerosis, coronary artery disease, breast arterial calcifications (BAC), peripheral arterial disease (PAD), aortic calcification index, carotid atheroma, calcific aortic valve disease (CAVD)
Citation: Kosciuszek ND, Kalta D, Singh M and Savinova OV (2022) Vitamin K antagonists and cardiovascular calcification: A systematic review and meta-analysis. Front. Cardiovasc. Med. 9:938567. doi: 10.3389/fcvm.2022.938567
Received: 07 May 2022; Accepted: 22 July 2022;
Published: 19 August 2022.
Edited by:
Leon J. Schurgers, Maastricht University, NetherlandsReviewed by:
Eisuke Amiya, The University of Tokyo Hospital, JapanRoger Rennenberg, Maastricht University Medical Centre, Netherlands
Copyright © 2022 Kosciuszek, Kalta, Singh and Savinova. This is an open-access article distributed under the terms of the Creative Commons Attribution License (CC BY). The use, distribution or reproduction in other forums is permitted, provided the original author(s) and the copyright owner(s) are credited and that the original publication in this journal is cited, in accordance with accepted academic practice. No use, distribution or reproduction is permitted which does not comply with these terms.
*Correspondence: Olga V. Savinova, b3Nhdmlub3YmI3gwMDA0MDtueWl0LmVkdQ==